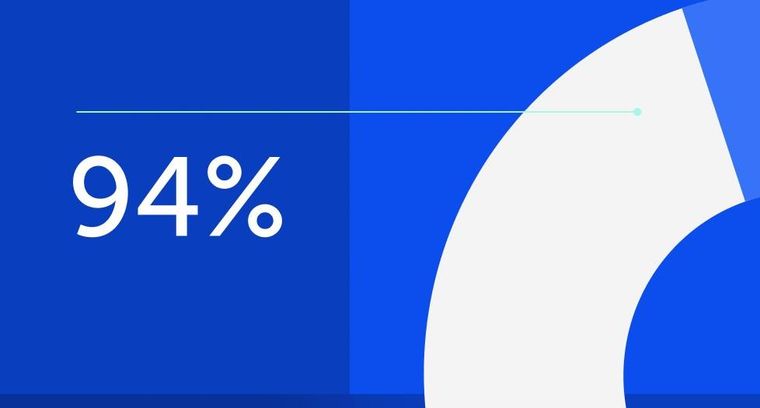
94% of researchers rate our articles as excellent or good
Learn more about the work of our research integrity team to safeguard the quality of each article we publish.
Find out more
ORIGINAL RESEARCH article
Front. Mar. Sci., 08 April 2025
Sec. Ocean Observation
Volume 12 - 2025 | https://doi.org/10.3389/fmars.2025.1569261
This article presents the results of three experiments conducted in 2022–2023 using an Argo autonomous profiling float anchored to the seabed with a thin line. The aim of the study was to prevent the float from drifting in order to perform measurements in a precisely defined location. The experiments were carried out in the Baltic Sea - in Puck Bay and the vicinity of the Hel Peninsula. Puck Bay, a shallow and sheltered body of water with a unique and sensitive ecosystem, provided an excellent location for testing the method under calm conditions. In contrast, the northern areas of the Hel Peninsula, exposed to strong currents and high waves, allowed for the evaluation of the method’s effectiveness in more dynamic conditions. The results of the experiments demonstrated that anchoring the Argo float is effective in shallow and sheltered waters with low wave activity and weak currents, such as Puck Bay. However, in the more challenging environment of the Hel Peninsula, the method proved to be less effective, indicating its limitations in harsher environmental conditions. Standard Argo floats, which are most often deployed in deeper parts of the Baltic Sea, provide limited data from shallow-water areas, particularly those near the coast and in enclosed waters such as lagoons and bays. The introduction of anchored Argo floats could complement the existing network of drifting floats, ensuring better coverage of shallow-water areas and improving the quality of marine environmental monitoring.
Argo floats are among the most important tools in modern oceanography, enabling automated, long-term monitoring of the physical and biogeochemical properties of the global ocean. The international Argo program, initiated in 1999, brought a revolution in operational oceanography (Le Traon, 2013). Today, the Argo program is a key component of both the Global Ocean Observing System (GOOS) and the Global Climate Observing System (GCOS) (Roemmich et al., 2019). Nearly 4,000 standard Argo floats cover the deep oceans, each profiling the water column down to a depth of 2,000 meters every 10 days. In 2024, the number of profiles obtained by Argo exceeded 3 million.
Argo floats are designed for measurements in the open ocean. They do not have their own propulsion system but drift freely with ocean currents. Depth changes are controlled by adjusting the float’s buoyancy, which is achieved by pumping oil from the interior of the float into an external rubber bladder or back into its internal reservoir placed in a rigid body. Each Argo float has a unique identification number assigned by the World Meteorological Organization (WMO). When a float surfaces, its data are transmitted to Data Assembly Centers (DAC), where they undergo preliminary quality control based on an agreed set of tests. These processed data are made available in near-real time, typically within 12 hours of measurement. The data are then forwarded to the Global Data Assembly Centers (GDAC) in Brest, France, and Monterey, California, where they become publicly accessible online within 24 hours of transmission. Within a year of data collection, the data undergo delayed-mode quality control (DMQC) conducted by GDAC. The entire data management process is coordinated by the Argo Data Management Team (ADMT).
The significance of Argo floats in ocean studies is undeniable, and technological advancements continue to enable increasingly sophisticated and precise measurements tailored to the specific conditions of different marine environments. Moreover, Argo floats can be utilized for purposes beyond basic (Core) and BioGeoChemical (BGC) measurements. The success of the global Argo program has led to the decision to expand and enhance the network through the OneArgo initiative, which aims to achieve three key objectives:
1. Supplementing Core Argo to cover marginal seas and seasonal ice zones,
2. Increasing Core Argo float density in key areas such as the tropics and western boundary current regions,
3. Implementing major new missions, including BioGeoChemical Argo, capable of measuring chlorophyll fluorescence, particle backscatter, oxygen, nitrate, pH, and irradiance, and Deep Argo, designed to monitor ocean variability at depths of up to 6,000 meters (Owens et al., 2022; Roemmich et al., 2019).
The United Nations Decade of Ocean Science for Sustainable Development (2021–2030) provides an excellent opportunity to realize these ambitious plans.
Changes in the Argo observing network began earlier, primarily focusing on the deployment of floats in marginal seas. Particular attention should be given to the use of Argo in the Baltic Sea, one of the most diverse and challenging water bodies in the world, where unique environmental conditions pose significant challenges for research teams (Haavisto et al., 2018; Roiha et al., 2018; Siiriä et al., 2018; Walczowski et al., 2020; Merchel et al., 2024). The Baltic Sea, a small, shallow, and semi-enclosed basin with low salinity, is characterized by strong water stratification and intense seasonal and regional variability (Bulczak et al., 2024; Lehmann et al., 2022; Liblik and Lips, 2019; Rak et al., 2024). These conditions create difficulties for standard Argo floats, which were originally designed for open-ocean operations. Standard floats may struggle to overcome the strong pycnocline, drift into areas with heavy vessel traffic, or be affected by highly variable coastal currents. However, the Baltic Sea also offers advantages for Argo deployments. Its small and semi-enclosed nature allows for easier recovery and redeployment of floats, facilitating diverse research experiments. Moreover, the Baltic is particularly sensitive to human activities, such as eutrophication and climate change, which increases the demand for precise and regular monitoring of its physical and biogeochemical properties. Modern float versions, such as BGC-Argo (BioGeoChemical Argo), provide enhanced capabilities by measuring dissolved oxygen, particle backscatter, irradiance, chlorophyll-a, nitrates, and pH, enriching analyses of key biogeochemical processes in the region. Despite the risk of float damage from intense ship traffic and fishing activities, the Baltic Sea’s research potential makes it an excellent testbed for developing and refining advanced oceanographic technologies.
The first Argo floats in the Baltic Sea were deployed in its northern part in 2012 by Finnish oceanographers (Haavisto et al., 2018; Roiha et al., 2018; Siiriä et al., 2018). In 2016, Polish oceanographers joined this initiative, starting regular deployments of Argo floats in the southern Baltic Sea as part of the European EuroArgo ERIC infrastructure (Walczowski et al., 2020; Merchel et al., 2024). Since 2021, German oceanographers have also been actively involved in the program, deploying floats in the central and western Baltic Sea. In 2023, Danish oceanographers also joined the group of researchers contributing to the monitoring of the Baltic Sea, initiating the deployment of floats in the Kattegat region and the western Baltic. Thanks to the collaborative efforts of multiple Baltic countries, the Argo project has become one of the key oceanographic research tools in the region. It provides critical data on the variability of temperature, salinity, and biogeochemical parameters, significantly enhancing the understanding of oceanographic processes in the Baltic Sea and the impact of climate change on this fragile ecosystem.
Argo-Poland, over more than eight years of operation in the Baltic Sea, has deployed 16 Argo floats. Of these, seven were equipped with sensors for measuring only basic oceanographic parameters: pressure, temperature, and salinity (CTD). Another eight floats included additional sensors for measuring dissolved oxygen concentration (DO), enabling more detailed monitoring of biogeochemical processes. Additionally, one specialized BGC float was enhanced with advanced sensors capable of measuring chlorophyll-a concentration, suspended particle, and irradiance - key parameters for assessing biological processes and the state of the marine ecosystem. During this period, Argo floats have conducted over 8,000 CTD profiles. Among these, 4,100 profiles included dissolved oxygen data, and nearly 600 were BGC profiles.
During standard missions in the southern part of the Baltic Sea, the issue of rapid float drift was frequently encountered. Within a few months, the floats moved from the Bornholm Basin through the Slupsk Furrow to the Gdansk Basin and then to the Eastern Gotland Basin, where they remained for an extended period. To ensure measurements in the area of interest, these floats can be retrieved and redeployed in the Bornholm Basin. However, this procedure is costly and time-consuming. To address these challenges, Argo floats were used as “virtual moorings.” This approach involved configuring the floats to remain on the bottom between profiles. This solution significantly reduced float drift and allowed for measurements to be conducted within a limited area for a longer period (Merchel et al., 2024).
Parking on the bottom has many advantages but is not always effective in limiting float drift. It often proves insufficient to keep the float within the selected basin, which led us to conduct further experiments involving anchoring the float to the seabed. This article presents the results of three experiments conducted in 2022–2023 using an autonomous Argo profiling float anchored to the seabed with a thin line. This solution allows for complete control over the device’s position. By eliminating drift, tethered floats enable the collection of high-temporal-resolution time series data, which is crucial for monitoring the diverse and dynamic ecosystem of the Baltic Sea. This type of innovation could enhance research in coastal waters worldwide, contributing to a deeper understanding of processes occurring in marine ecosystems. Integrating these floats into the Argo network could align with the measurement approach outlined in the OneArgo doctrine.
All three experiments were conducted using an Argo float of the ARVOR-I type, manufactured by the French company NKE (serial number A12632-18EU005, IMEI 300234065969030). The device is equipped with an Iridium telemetry system, enabling two-way communication between the float and the control center. This technology allows for remote real-time monitoring of the float and interventions when necessary, increasing operational efficiency and flexibility. In addition to standard CTD measurements, the float also measures the concentration of dissolved oxygen in the water.
The float used in the experiment was not registered in the Argo network because it was anchored to the seabed, whereas Argo requirements specify that floats should drift freely in the water column. As a result, its data was not transmitted to the Data Assembly Centers and did not undergo preliminary real-time quality control based on the standard set of tests. Instead, the data was sent directly from the satellite network operator to IOPAN in the form of emails with attachments containing raw data in *.sbd format. After decoding using software provided by the float manufacturer, the data underwent further processing.
In addition to CTD profiles from the surface to the bottom, the device also performed measurements while parking (in this case, at the bottom), near-surface, and surface. Near-surface data can be used for cross-calibration of CTD data (Wong et al., 2024), while surface data are valuable for calibrating oxygen measurements (Johnson et al., 2015; Bittig et al., 2018). Near-surface and surface data are considered specialized and differ from standard CTD profiles. For most floats equipped with a temperature and conductivity sensor with a pump-driven circulation system, the pump is turned off during ascent at a depth of approximately 5 meters to prevent contamination of the conductivity cell. Some float types continue sampling up to the sea surface, either with the pump turned off or with the pump reactivated closer to the surface (Wong et al., 2024). Floats operating in the Baltic Sea have their CTD pump turned off at a depth of 2 meters.
While temperature data collected without the pump activated is of good quality, conductivity data is significantly less reliable. This occurs because the flow through the conductivity cell is incorrect when the pump is turned off. Consequently, salinity data obtained without an active pump (or with a partially functioning pump) is flagged in real-time as “probably bad data” (Wong et al., 2024).
Most near-surface profiles extend up to the sea surface, where they transition into the surface part. This means that the shallowest part of the profile may contain measurements of a mixture of air and water. Data from this shallowest section, which potentially includes measurements of this mixture, are also flagged in real-time as “probably bad data” (Wong et al., 2024).
In 2022, IOPAN conducted two experiments using an Argo float in the very shallow waters of the southern Baltic Sea. In both cases, the float was deployed in Puck Bay (Figure 1). This is a shallow bay with a maximum depth of 54 meters, forming the western part of the Gulf of Gdansk, separated from the open sea to the northeast by the Hel Peninsula (Kruk-Dowgiałło and Szaniawska, 2008). Puck Bay was chosen for several reasons. One of them was the shallow depth and sheltered nature of the area, which results in smaller waves and weaker currents. Another important factor was its proximity to the Institute of Oceanology PAN in Sopot, allowing for a quick response in case of device failure.
The first experiment involved anchoring the float to the seabed at a depth of 15 m. A set consisting of an acoustic release, a buoyancy buoy, and a 25-meter-long line was attached to a 25-kilogram deadweight anchor. The Argo float was secured at the end of the line. The line length (approximately 1.7 times the depth of the basin) allowed the device to ascend freely while preventing the line (which had slight positive buoyancy) from reaching the surface when the float remained at the seabed.
The float was deployed on May 25, 2022, in the sheltered waters of Puck Bay (54.5918°N, 18.5887°E) (Figure 1). The experiment was conducted under wind conditions up to 6°B and wave heights of up to 1 m. The initial profiling interval was set to 1 hour, the sampling frequency to 8 seconds, and the parking depth to 25 m. The parking depth was significantly greater than the depth of the water area, ensuring that the float remained on the seabed between profiles. The float did not transmit data until the following day (May 26), when the profiling period was remotely changed to 2 hours, enabling proper data transmission. However, the profiling frequency was still too high, causing data collection issues while the float was parked on the seabed (up to profile 53). Therefore, on May 30, the profiling period was adjusted to 7 hours, which resolved the problem.
The test lasted until June 19, 2022. By that time, the float had completed 124 profiles, measuring pressure, temperature, conductivity, and dissolved oxygen content (Supplementary Figure 1). Additionally, it collected data from the near-bottom layer during parking, as well as from the near-surface and surface layers (Supplementary Figures 2–4). The experiment was successful, leading to the decision to retrieve the float and repeat the experiment in a deeper area of Puck Bay. The acoustic release was used to detach the buoyancy buoy from the ballast. The entire setup, including the acoustic release, float, and buoyancy buoy, was recovered in an intact condition, allowing it to be reused in the next experiment. The ballast (deadweight anchor, 25 kg) was left on the seabed.
On August 26, 2022, the second experiment was conducted, using the results of the previous study to further optimize the system’s operation. The float was once again anchored to the seabed in a sheltered area of Puck Bay (54.5856°N, 18.6768°E), this time at a depth of 40 meters, approximately 6 km east of the previous deployment site (Figure 1). A 60-meter-long rope, an acoustic release, a buoyancy buoy, and another 25-kilogram deadweight anchor were used. The sampling frequency was set to 8 seconds, as in the previous experiment, while the parking depth was increased from 25 to 60 meters.
The float was programmed to perform profiling cycles every 7 hours from cycle 1 to 60 (August 26 – September 14, 2022). Then, the cycle duration was shortened to 6 hours from cycle 61 to 297 (September 14 – December 5, 2022), which did not affect the float’s operation, as it continued to take measurements and transmit data correctly. After cycle 275 (November 8, 2022), the device stopped transmitting, prompting the research team to recover it. The float, along with all its equipment, was retrieved on December 5, 2022, from the deck of the IOPAN research vessel r/v Oceania during a routine hydrographic cruise. A visual inspection of the float after the experiment revealed no damage. Upon surfacing, the device transmitted data from an additional 22 profiles. During the initial phase of the experiment, the float measured parameters throughout the entire water column. However, after cycle 275, it only collected data from a depth of about 32 meters (up to profile 297). The line to which the float was attached was severely tangled, which most likely prevented it from completing full profiles and surfacing.
During the experiment, the float completed 240 full and 35 incomplete profiles, providing valuable CTD and DO data. Additionally, it conducted measurements while parked at the seabed as well as in the near-surface and surface layers. The second experiment was also considered a success, delivering valuable hydrographic data from Puck Bay.
Encouraged by the success of the two previous experiments, in 2023 we decided to conduct another one, this time outside the bay, in the area of the Hel Peninsula, at the position 54.5856°N, 18.6768°E (Figure 1). The choice of this location was not accidental. The region north of the Hel Peninsula is characterized by a specific seabed structure. The natural slope toward the open sea results in a rapid increase in depth in this region. This topography favors the occurrence of strong alongshore currents and the phenomenon of upwelling, where cold, nutrient-rich, deep waters are brought to the surface. This process, frequently observed in the region, has a significant impact on the local marine ecosystem (Jankowski, 2002; Kowalewska-Kalkowska and Kowalewski, 2019; Kowalewski and Ostrowski, 2005; Lehmann and Myrberg, 2008). The unique topography and open character of the area also expose this region to intense wave activity, which influences both water dynamics and the performance of measurement systems. The experiment aimed to test the effectiveness of our methods in more challenging hydrodynamic conditions and to gather new data that could contribute to a better understanding of the processes occurring in this area.
The float was deployed on May 9, 2023, from the deck of the research vessel r/v Oceania at the beginning of a routine hydrographic cruise. Weather conditions during deployment were favorable, with a wind force of 3°B and moderate waves. The experiment was conducted at a water depth of 45 meters. A 78-meter-long line, an acoustic release, a buoyancy buoy, and a 25-kilogram deadweight anchor were used. The sampling frequency was set to 8 seconds, as in previous experiments, while the parking depth was set to 60 meters. The device was programmed to perform profiling cycles every 6 hours. However, for several days, the float did not transmit data, prompting the retrieval of the measurement system on May 16, 2023, during the same cruise. Upon retrieval, the float transmitted data from 20 profiling cycles. Only 4 cycles contained data from the entire water column, while the remaining 16 cycles had incomplete or missing data (Supplementary Figure 5). Near-surface and surface data were complete, but the depth of surface measurements varied irregularly, ranging from -3 m (in-air) to 10 m instead of the standard depth of approximately 0 m (Supplementary Figures 7, 8), which may indicate very intense wave action. Most parking depth measurements were taken at approximately 15 meters instead of the planned 45 meters (Supplementary Figure 6). The float never reached the seabed, profiling to a maximum depth of 34 meters instead of 45 meters. This suggests that strong alongshore currents likely prevented the float from reaching the bottom and performing measurements across the full water column. The float was retrieved with a tangled line, which may have further impeded its proper operation.
The aim of this article is to present the potential of temperature, salinity, and oxygen content data obtained using an anchored Argo float. In this context, incomplete data and measurements taken at depths different from the intended ones have been deliberately included. This approach not only allows for an assessment of the possibilities offered by such measurements but also provides a better understanding of their limitations and potential drawbacks associated with this technique. As a result, the article highlights both the advantages of the system and helps identify areas that require improvement and optimization.
Data from the second experiment conducted in the Bay of Puck are discussed in more detail. This experiment lasted the longest, exceeding three months. During this time, the float collected a very interesting time series of data. Figures presenting data from the other two experiments are included in the appendix (Supplementary Figures 1-8).
The profiles of seawater properties from the entire measurement period (August 26 – December 4, 2022) illustrate their seasonal variability (Figure 2). A clear division into two seasons is visible: summer (blue color) and autumn (other colors). Since this is a shallow area (approximately 40 m), changes are evident throughout the entire water column. During the summer period (until mid-September), the thermocline is present at a depth of approximately 10–15 m, then it deepens to around 30 m during the autumn period. The surface layer is more stable in autumn, whereas in summer, there are greater fluctuations in temperature, salinity, and dissolved oxygen content. During autumn, periodic inflows of more saline, oxygen-poor, dense bottom water from the Gdansk Deep are observed.
Figure 2. Temperature, salinity, and dissolved oxygen profiles recorded by the anchored Argo float during the second experiment in Puck Bay (August 26 – December 4, 2022). The color scale indicates the measurement time.
A significant variability in temperature was observed throughout the entire water column (Figure 2). In the surface layer, the temperature ranged from 12°C (November 8, 2022) to 22°C (August 29, 2022). In the bottom layer, it varied from approximately 5°C (August 28, 2022) to 14°C (September 22, 2022).
Salinity in the surface layer showed slight fluctuations, remaining within the range of 7–7.5 throughout the study period (Figure 2). In contrast, salinity in the bottom layer was more variable, with the lowest daily value recorded at 7.4 (September 22, 2022) and the highest at 9.0 (November 5, 2022). The six-hour differences were even greater, ranging between 7.4 and 10.2.
The dissolved oxygen concentration in the surface layer was lowest at the beginning of the measurement period, reaching 201 µmol/kg on August 27, 2022, while the highest concentration was recorded at the end of the period, at 302 µmol/kg on November 4, 2022. In the bottom layer, the situation was reversed: the lowest value, 125 µmol/kg, was recorded on November 4, 2022, while the highest value, 295 µmol/kg, was observed on August 29, 2022 (Figure 2).
During each cycle, the float parked near the seabed (drift depth) for one hour, during which it measured temperature, salinity, and dissolved oxygen concentration (Figure 3). Until cycle 275, the float parked at a depth of approximately 40 meters. During this period, there were instances where the float did not reach the seabed, likely due to difficult storm conditions.
Figure 3. Temperature, salinity, and dissolved oxygen data recorded during the second experiment in Puck Bay (August 26 – December 4, 2022) while parking at the seabed.
From cycle 276 to 297, there are no data available for profiles, the near-surface or surface layers. The only available measurements from this period are from parking at a depth of approximately 30 meters. This was most likely caused by a tangled line, which was confirmed upon float retrieval—the line was severely entangled, and the float was covered in seaweed (Figure 4).
Figure 4. Photos of the Argo float retrieved on board r/v Oceania during the second experiment in Puck Bay on December 5, 2022 (Photos: Daniel Rak).
The data from parking depth show distinct episodes of bottom water inflows from the Gdansk Basin (cycles 30–53, 110–120, 165–290). This water was characterized by higher salinity (around 8–10) and lower oxygen concentrations (70–200 µmol/kg) (Figure 3). In the bottom layer, the Pearson correlation coefficient between salinity and oxygen concentration was -0.94, indicating a strong negative correlation. However, no significant relationship with temperature was observed.
During each cycle, the float also performed measurements in the near-surface and surface layers. The near-surface layer covered depths ranging from 0 to 10 meters (Figure 5), while the surface layer was generally within the range of -0.5 to 0.5 meters (result from the wave motion). However, under stronger wave conditions, it could extend beyond these boundaries (Figure 6). These measurements provided valuable information on the variability of environmental conditions in shallow water layers and in direct contact with the atmosphere.
Figure 5. Temperature, salinity, and dissolved oxygen data recorded during the second experiment in Puck Bay (August 26 – December 4, 2022) from the near-surface.
Figure 6. Temperature, salinity, and dissolved oxygen data recorded during the second experiment in Puck Bay (August 26 – December 4, 2022) from and the surface.
Until cycle 275, data from the near-surface layer indicated stable salinity with a slight increase from approximately 7.2 to 7.4 and a decrease in temperature from around 22°C to 12°C. The oxygen concentration in this layer showed a gradual increase from about 220 to 350 µmol/kg (Figure 5). Similar changes were observed in the surface layer: salinity increased from 7.0 to 7.4, temperature decreased from approximately 25°C to 12°C, and oxygen concentration rose from about 250 to 370 µmol/kg (Figure 6). From cycle 276 to 297, data from the near-surface and surface layers are missing, likely due to the previously mentioned issues with the float, most probably caused by a tangled tether. Data from the surface layer suggest stable conditions without strong wave activity. However, anomalies such as pressure fluctuations observed on September 12–14 and October 3–6 may indicate the occurrence of storm conditions.
Near-surface and surface data collected by the anchored float demonstrate that they can be used not only for calibration purposes. After thorough quality control in delayed mode, these measurements can also serve as valuable scientific data.
Argo floats operating within the global Argo network are primarily used for measurements in the open waters of seas and oceans. Finnish oceanographers were the first to introduce Argo floats into the northern regions of the Baltic Sea. Through collaboration within Euro-Argo ERIC, Polish oceanographers began deploying Argo floats in the southern Baltic Sea. Conventional oceanic Argo practices were modified: the profiling frequency was reduced to 1–2 days, and bottom parking was implemented. These modifications proved to be in line with the currently promoted One Argo doctrine, which aims to cover marginal seas with a dense Argo measurement network. In the case of the Baltic Sea, however, these efforts may still be insufficient, as measurements primarily cover deep basins. A map displaying the locations of all Argo profiles in the Baltic Sea clearly shows regions where data is lacking. The most significant data gaps occur in coastal areas and enclosed water bodies such as lagoons and bays (Figure 7). Therefore, the objective of the conducted experiments was to enable data collection from coastal, shallow-water areas that had previously remained inaccessible to Argo floats.
The experiments described in this article aimed to test the feasibility of anchoring an Argo float in very shallow waters to prevent its movement into deeper areas. This solution is effective in calm, sheltered regions where strong currents and intense wave action are limited. In the shallow and enclosed waters of Puck Bay, the anchored Argo float performed as expected. However, in the area of the Hel Peninsula, where currents are stronger and wave action is more intense, this method proved less effective and requires further improvements.
These conclusions are supported by a similar experiment conducted by Bulgarian oceanographers as part of the Euro-Argo RISE project. The experiment, carried out by the Institute of Oceanology of the Bulgarian Academy of Sciences (IO BAS) using an Argo float of the Arvor-I type (WMO 6903865), aimed to assess the feasibility of collecting data in the shallow waters of the Black Sea by anchoring the float at a depth of 50 meters. The float was deployed on July 24, 2020, on the western shelf of the Black Sea near the mouth of the Kamchiya River. To limit the device’s movement, a 100-meter-long, 2-mm-diameter fishing line with nearly neutral buoyancy was used. The mission was configured to allow for daily cycles, during which the float parked at a depth of 30 meters and conducted profiles down to 50 meters. To analyze the float’s performance under different environmental conditions, simulation tools were used to predict the behavior of the line as well as the effects of currents and wave action on the float (Palazov et al., 2021).
However, this experiment encountered significant technical problems. The float had difficulty rising to the surface, which led to an unstable GPS signal and irregular data transmission. During many cycles, the device failed to send any information, and in some cases, the CTD data were of low quality. Additionally, problems with the float’s buoyancy were observed, which may have been caused by the low salinity of the Black Sea’s surface waters, preventing proper antenna surfacing. On August 25, 2020, the line securing the float was likely broken, causing the device to start drifting freely. By November of the same year, the float was located in the Bosporus Strait region, but issues with GPS data accuracy prevented its recovery (Palazov et al., 2021).
Building on the acquired experience, oceanographers from IOPAN, in collaboration with oceanographers from the Institute of Geophysics of the Polish Academy of Sciences in Warsaw, successfully conducted experiments using an anchored Argo profiling float in Hornsund Fjord, Svalbard. In the challenging Arctic conditions, characterized by drifting ice and proximity to a calving glacier, a 14-day time series was obtained (June 11, 2024 – June 24, 2024), with measurements taken every 4 hours. During this period, 77 ascending profiles and 77 descending profiles were recorded.
The conducted experiments provided promising results and indicate the feasibility of using Argo floats anchored to the seabed with a properly sized line for data collection in shallow waters. The tests demonstrated that profiling in such conditions is technically possible, although it requires appropriate adjustments to mission parameters. For instance, the profiling interval of 1–2 hours used in the initial experiment proved too short. Extending this period to approximately 6 hours allowed for more complete and reliable results.
The experiments conducted in Puck Bay, the vicinity of the Hel Peninsula, and in the Black Sea have shown that the use of anchored Argo floats is not a universal solution. In shallow and sheltered waters, such as Puck Bay, this method proved to be quite effective as long as environmental conditions remained stable and currents and wave action were moderate. In these cases, an anchored float can serve as a valuable tool for continuous monitoring of the aquatic environment, providing data that was previously difficult to obtain. However, in open sea conditions, where strong currents and intense wave action are present, anchored floats face significant limitations. Both the experiment conducted by Bulgarian researchers in the Black Sea and the tests near the Hel Peninsula demonstrated that in such environments, this type of installation is prone to failures, such as line breakage or issues with communication and device stability. For this reason, an anchored Argo float is not suitable for use in open, dynamic waters.
Despite these limitations, the experiments provided valuable insights for future research. They highlight the need for further technological improvements, particularly in optimizing float settings to ensure the highest data quality and long-term device reliability. This could enable anchored Argo floats to be effectively used for environmental monitoring in semi-enclosed, small water bodies such as lagoons, bays, lakes, or river deltas. Such installations could become a key component of aquatic ecosystem monitoring systems, which is especially important in the context of climate change.
Of course, other technologies also allow for water column profiling using mobile measurement systems, and these are widely used in the Baltic Sea (Lips et al., 2016; Prien and Schulz-Bull, 2016; Rak et al., 2020; Stoicescu et al., 2019). However, these devices are expensive, and few of them have real-time data transmission capabilities; retrieving the entire profiling system is often necessary to access the data. A key advantage of using an anchored Argo float compared to conventional moorings is its lower cost, both in terms of equipment and operational expenses. While it requires additional components such as a ballast, a buoy, a release mechanism, and a line, its overall setup remains simpler and more cost-effective than a full-scale mooring system with a large surface buoy and heavy anchoring. Furthermore, deployment and recovery can be conducted from smaller research vessels without the need for specialized lifting equipment, significantly reducing operational costs. Unlike conventional moorings, which require regular maintenance and sensor replacements, the anchored Argo float can operate autonomously for extended periods, minimizing long-term servicing expenses while ensuring reliable water column profiling. Additionally, it offers the ability to cycle along the water column without being affected by mooring line tilting. Traditional moorings often require post-processing corrections to account for deviations in sensor positioning due to strong currents, which can introduce measurement inaccuracies. Therefore, anchored floats can serve as an excellent complement to the Argo network. Properly flagged data will contribute to better coverage and monitoring of coastal zones and sheltered water bodies.
Data from standard, freely drifting Argo floats, combined with data from floats using seabed parking techniques and anchored floats in coastal regions, can provide a comprehensive dataset available in near-real time.
The raw data supporting the conclusions of this article will be made available by the authors, without undue reservation.
Written informed consent was obtained from the individual(s) for the publication of any identifiable images or data included in this article.
MM: Conceptualization, Data curation, Formal Analysis, Investigation, Methodology, Visualization, Writing – original draft, Writing – review & editing, Validation. WW: Funding acquisition, Supervision, Writing – original draft, Writing – review & editing, Conceptualization, Investigation, Methodology. PW: Investigation, Resources, Writing – review & editing, Methodology.
The author(s) declare that financial support was received for the research and/or publication of this article. This publication was supported by the following projects: the Argo-Poland project, funded by the Polish Minister of Education and Science (grant number 2022/WK/04), and the Euro-Argo ONE project, funded by the HORIZON EUROPE program (HORIZON-INFRA-2024-DEV-01-03).
We sincerely thank Daniel Rak for his valuable assistance, both at sea and on land. Our gratitude also goes to the crew of r/v Oceania for their help and cooperation at sea.
The authors declare that the research was conducted in the absence of any commercial or financial relationships that could be construed as a potential conflict of interest.
The author(s) declare that no Generative AI was used in the creation of this manuscript.
All claims expressed in this article are solely those of the authors and do not necessarily represent those of their affiliated organizations, or those of the publisher, the editors and the reviewers. Any product that may be evaluated in this article, or claim that may be made by its manufacturer, is not guaranteed or endorsed by the publisher.
The Supplementary Material for this article can be found online at: https://www.frontiersin.org/articles/10.3389/fmars.2025.1569261/full#supplementary-material
Bittig H. C., Körtzinger A., Neill C., van Ooijen E., Plant J. N., Hahn J., et al. (2018). Oxygen optode sensors: principle, characterization, calibration, and application in the ocean. Front. Mar. Sci. 4. doi: 10.3389/fmars.2017.00429
Bulczak A. I., Nowak K., Jakacki J., Muzyka M., Rak D., Walczowski W. (2024). Seasonal variability and long-term winter shoaling of the upper mixed layer in the southern Baltic Sea. Cont. Shelf Res. 276, 105232. doi: 10.1016/j.csr.2024.105232
Haavisto N., Tuomi L., Roiha P., Siiriä S. M., Alenius P., Purokoski T. (2018). Argo floats as a novel part of monitoring the hydrography of the Bothnian Sea. Front. Mar. Sci. 5. doi: 10.3389/fmars.2018.00324
Jankowski A. (2002). Variability of coastal water hydrodynamics in the southern Baltic–hindcast modelling of an upwelling event along the Polish coast. Oceanologia 44, 395–418. https://www.iopan.gda.pl/oceanologia/444ston.pdf.
Johnson K. S., Plant J. N., Riser S. C., Gilbert D. (2015). Air oxygen calibration of oxygen optodes on a profiling float array. J. Atmos. Oceanic Technol. 32, 2160–2172. doi: 10.1175/JTECH-D-15-0101.1
Kowalewska-Kalkowska H., Kowalewski M. (2019). Combining satellite imagery and numerical modelling to study the occurrence of warm upwellings in the Southern Baltic Sea in winter. Remote Sens. 11, 2982. doi: 10.3390/rs11242982
Kowalewski M., Ostrowski M. (2005). Coastal up- and downwelling in the southern Baltic. Oceanologia 47, 421–432. https://www.iopan.gda.pl/oceanologia/474kowa1.pdf.
Kruk-Dowgiałło L., Szaniawska A. (2008). “Gulf of gdańsk and puck bay,” in Ecology of Baltic Coastal Waters. Ed. Schiewer U. (Springer, Berlin, Heidelberg), 139–165. doi: 10.1007/978-3-540-73524-3_7
Lehmann A., Myrberg K. (2008). Upwelling in the baltic sea—A review. J. Mar. Syst. 74, S3–S12. doi: 10.1016/j.jmarsys.2008.02.010
Lehmann A., Myrberg K., Post P., Chubarenko I., Dailidiene I., Hinrichsen H. H., et al. (2022). Salinity dynamics of the baltic sea. Earth Syst. Dyn. 13, 373–392. doi: 10.5194/esd-13-373-2022
Le Traon P. Y. (2013). From satellite altimetry to Argo and operational oceanography: three revolutions in oceanography. Ocean Sci. 9, 901–915. doi: 10.5194/os-9-901-2013
Liblik T., Lips U. (2019). Stratification has strengthened in the Baltic Sea—An analysis of 35 years of observational data. Front. Earth Sci. 7. doi: 10.3389/feart.2019.00174
Lips U., Kikas V., Liblik T., Lips I. (2016). Multi-sensor in situ observations to resolve the sub-mesoscale features in the stratified Gulf of Finland, Baltic Sea. Ocean Sci. 12, 715–732. doi: 10.5194/os-12-715-2016
Merchel M., Walczowski W., Rak D., Wieczorek P. (2024). The use of Argo floats as virtual moorings for monitoring the South Baltic Sea. Oceanologia 66, 99–110. doi: 10.1016/j.oceano.2024.01.002
Owens W. B., Zilberman N., Johnson K. S., Claustre H., Scanderbeg M., Wijffels S., et al. (2022). OneArgo: a new paradigm for observing the global ocean. Mar. Technol. Soc J. 56, 84–90. doi: 10.4031/MTSJ.56.3.5
Palazov A., Notarstefano G., Slabakova V., Cancouët R. (2021). D6.3: Preliminary results of shallow coastal float operations in the Black Sea. Zenodo. doi: 10.5281/zenodo.7103961
Prien R. D., Schulz-Bull D. E. (2016). Technical note: GODESS—a profiling mooring in the Gotland Basin. Ocean Sci. 12, 899–907. doi: 10.5194/os-12-899-2016
Rak D., Przyborska A., Bulczak A. I., Dzierzbicka-Głowacka L. (2024). Energy fluxes and vertical heat transfer in the Southern Baltic Sea. Front. Mar. Sci. 11. doi: 10.3389/fmars.2024.1365759
Rak D., Walczowski W., Dzierzbicka-Głowacka L., Shchuka S. (2020). Dissolved oxygen variability in the southern Baltic Sea in 2013–2018. Oceanologia 62, 525–537. doi: 10.1016/j.oceano.2020.08.005
Roemmich D., Alford M. H., Claustre H., Johnson K., King B., Moum J., et al. (2019). On the future of Argo: a global, full-depth, multi-disciplinary array. Front. Mar. Sci. 6. doi: 10.3389/fmars.2019.00439
Roiha P., Siiriä S. M., Haavisto N., Alenius P., Westerlund A., Purokoski T. (2018). Estimating currents from Argo trajectories in the Bothnian Sea, Baltic Sea. Front. Mar. Sci. 5. doi: 10.3389/fmars.2018.00308
Siiriä S., Roiha P., Tuomi L., Purokoski T., Haavisto N., Alenius P. (2018). Applying area-locked, shallow water Argo floats in Baltic Sea monitoring. J. Oper. Oceanogr. 12, 58–72. doi: 10.1080/1755876X.2018.1544783
Stoicescu S. T., Lips U., Liblik T. (2019). Assessment of eutrophication status based on sub-surface oxygen conditions in the Gulf of Finland (Baltic Sea). Front. Mar. Sci. 6. doi: 10.3389/fmars.2019.00054
Walczowski W., Merchel M., Rak D., Wieczorek P., Goszczko I. (2020). Argo floats in the southern Baltic Sea. Oceanologia 62, 478–488. doi: 10.1016/j.oceano.2020.07.001
Wong A., Keeley R., Carval T., the Argo Data Management Team (2024). Argo quality control manual for CTD and trajectory data. doi: 10.13155/33951
Keywords: Argo floats, anchored Argo, temperature, salinity, oxygen content, Baltic Sea, Puck Bay, Hel Peninsula
Citation: Merchel M, Walczowski W and Wieczorek P (2025) Anchored Argo profiling float experiments in the southern Baltic Sea: Puck Bay and Hel Peninsula. Front. Mar. Sci. 12:1569261. doi: 10.3389/fmars.2025.1569261
Received: 31 January 2025; Accepted: 24 March 2025;
Published: 08 April 2025.
Edited by:
Takafumi Hirata, Hokkaido University, JapanReviewed by:
Simone Cosoli, University of Western Australia, AustraliaCopyright © 2025 Merchel, Walczowski and Wieczorek. This is an open-access article distributed under the terms of the Creative Commons Attribution License (CC BY). The use, distribution or reproduction in other forums is permitted, provided the original author(s) and the copyright owner(s) are credited and that the original publication in this journal is cited, in accordance with accepted academic practice. No use, distribution or reproduction is permitted which does not comply with these terms.
*Correspondence: Małgorzata Merchel, bWVyY2hlbEBpb3Bhbi5wbA==
Disclaimer: All claims expressed in this article are solely those of the authors and do not necessarily represent those of their affiliated organizations, or those of the publisher, the editors and the reviewers. Any product that may be evaluated in this article or claim that may be made by its manufacturer is not guaranteed or endorsed by the publisher.
Research integrity at Frontiers
Learn more about the work of our research integrity team to safeguard the quality of each article we publish.