- 1Biology, Geology, Physics and Inorganic Chemistry Department, School of Experimental Sciences and Technology (ESCET), Rey Juan Carlos University, Madrid, Spain
- 2Research Group in Environmental Geophysics and Geochemistry, Universidad Rey Juan Carlos, Madrid, Spain
- 3Applied Physics Department, Sea Sciences Faculty, University of Cádiz, CASEM, Cádiz, Spain
While the internal structure of transgressive dunes is relatively well understood, little is known about that of climbing dunes. The Valdevaqueros dune, located 10 kilometers northwest of Tarifa (Cádiz), is considered one of the largest and most active transgressive dunes in Europe, as well as one of the main climbing dunes of the Iberian Peninsula. This study analyzes the internal structure of the Valdevaqueros dune using ground-penetrating radar (GPR) profiles. The results reveal a variety of sedimentary structures that reflect different wind intensities and directions. The findings highlight the significant role of topography in the development of these structures. These results contribute to expanding knowledge about climbing dunes, and particularly the dynamics of the Valdevaqueros dune.
1 Introduction
Coastal dunes form in areas of the coast where there is sufficient sand availability and winds capable of transporting it inland. While a sufficiently large post-beach surface is typically required to accommodate and promote maximum sedimentation, smaller and steeper areas can also sustain dunes, provided the sediment supply is substantial and wind transport capacity is exceptionally high (Ley et al., 2007). Transgressive dunes develop under conditions of significant sand transport inland, driven by strong wind regimes. Moreover, transgressive dune fields, characterized by abundant sediment volume and high mobility, exhibit a wide variety of aeolian landforms (Hesp, 2011). These include dunes stabilized by vegetation (e.g., nebkhas and shadow dunes), free dunes (e.g., barchan dunes and barchanoid ridges), erosive features (e.g., deflation surfaces and blowouts), and relief-dependent dunes (e.g., rampant dunes and drop dunes) (San Romualdo Collado, 2022).
When sand is transported towards a physical barrier (such as a cliff, hill, or building), it accumulates at its base or on the windward slopes, giving rise to echo dunes, [dunes formed upwind of an obstacle where the wind generates a secondary airflow in front of the obstacle, creating a reverse vortex that deposits single sand ridges parallel to the obstacle (Tsoar, 1983)]. In climbing dunes (essentially dunes that climb slopes), sediment is transported upslope by the persistent action of the wind, which deposits the sand on the slope until it reaches the crest. Once there, if the wind and transport conditions are favorable, the sand can move to the leeward side, forming secondary deposits as sand sheets or smaller dunes. A directionally persistent wind regime with sufficient energy drives the formation of climbing dunes, while the material foundation is an abundant sand source (Dong et al., 2023).
In climbing dunes, the slope plays a fundamental role in the sand transport and accumulation process. Although there is no fixed threshold of inclination that defines this kind of dune, Tsoar (1983) found that climbing dunes are formed at a slope angle of 50° or less, while echo dunes were formed at higher slope angles indicating a correspondence between the formation of reversing flow separation vortices at the toe of the steeper slopes and echo dune development. Dłużewski et al. (2023) noted that a prerequisite for climbing dune formation is that the slope inclination must not exceed 55°. On slopes of this inclination, airflow accelerates as it moves upslope, facilitating the transport of sand in the same direction. Gentler slopes tend to favor the formation of dispersed deposits or transverse dunes rather than climbing dunes (Hesp, 2011). In any case, these require a sufficiently steep slope, so that the wind does not completely transport the sand over the obstacle and allows its accumulation along the windward slope. On the other hand, although climbing dunes can develop on steeper slopes, sand hardly accumulates stably on inclinations that exceed the angle of repose of the sediment, which is generally between 30° and 34°for dry sand (Tsoar et al., 1996).
As previously stated, under specific conditions influenced by factors such as wind speed and direction, sand grain size, topographical discontinuities, and the stability of the surface beyond the crest (Núñez Ravelo et al., 2016), some sand may be deposited on the leeward side, facilitating the advance of the dune. This highlights the complex interplay between aeolian processes and the relief morphology of climbing dunes.
Although several studies have focused on climbing dunes (Tsoar, 1983; Clemmensen et al., 1997; White and Tsoar, 1998; Rodríguez-Santalla et al., 2009; Núñez Ravelo et al., 2016; Dong et al., 2023; Wang et al., 2022; Hesp, 2011; Dłużewski et al., 2023), only a few have specifically analyzed their internal structure (Ford et al., 2010; Flor-Blanco et al., 2012; Zhao et al., 2018). Understanding the internal architecture of these dunes is essential for reconstructing their formation processes and evaluating their response to environmental changes. Ground Penetrating Radar (GPR) is a highly effective tool for this purpose, as it allows for the reconstruction of past depositional environments and the identification of sedimentary processes in a variety of settings (Neal, 2004). Given its non-invasive nature, GPR is particularly suitable for studying fragile environments such as coastal dunes without causing environmental damage (Gómez-Ortiz et al., 2009; Zhao et al., 2018; Rodríguez-Santalla et al., 2021).
On the other hand, Hesp and Smyth (2019) highlight that scarping processes are likely to become more prevalent in coastal environments as sea levels rise and beaches and dunes retreat or shift landwards. They emphasize the vital importance of understanding wind flow and aerodynamics over scarps. Similar considerations are made by Vallarino-Castillo et al. (2023) on the coasts of Latin America. Moreover, given the growing concern about the effects of climate change on coastal zones, there is an increasing tendency to regenerate dunes in areas where they have disappeared, as dunes are considered one of the most effective mechanisms for beach conservation and defense (Rodríguez-Santalla et al., 2009). Building on these ideas, it is essential to deepen our understanding of the processes and mechanisms that influence the formation and evolution of climbing dunes. Ground Penetrating Radar (GPR) offers valuable insights into the stratigraphic configuration, depositional patterns, and subsurface features of dune systems, making it a powerful tool for analyzing their internal structure and sedimentary dynamics. In this study, we use GPR to examine the internal architecture of climbing dunes, with a particular focus on identifying bounding surfaces, sedimentary structures, and stratigraphic relationships. By doing so, we aim to improve the understanding of their formation processes and sedimentary evolution. This study presents an analysis of the sedimentary structures obtained through GPR associated with the climbing dune of Valdevaqueros, located on the coast of Cádiz. The objective is to elucidate the processes governing sand accumulation during upslope dune migration and to assess how wind dynamics and topographic relief interact to shape these sedimentary patterns. The results provide key insights into the aeolian depositional systems of coastal dune environment.
2 Study area
The Valdevaqueros dune, located 10 kilometers northwest of Tarifa (Cádiz) (Figure 1) is considered one of the largest transgressive dunes in Europe, measuring 700 m in length, 600 m in width, and rising 50 m above sea level. The dune migrates inland from southeast to northwest, forming ridges oriented perpendicular to the dominant easterly wind flow in the area. This wind pattern (Martins et al., 2018) drives an average migration rate of nearly 18 m/year, making it one of the most active dunes in Spain (Navarro, 2011). This high migration rate is attributed to the fact that 70% of wind speeds in the region exceed the threshold velocity required for sediment transport. Wind action has been observed to cause upslope displacements of up to 1 meter per day during easterly wind events (Navarro et al., 2015; Martínez-García et al., 2021, 2023). Navarro et al. (2011) calculated the drift potential (DP) and resultant drift potential (RDP) indices following the method of Fryberger (1979). The results indicated a very high DP, approaching 10,000, with about the same order of magnitude for RDP. The RDP/DP ratio was approximately 0.80, suggesting that the wind regime is predominantly unidirectional in the E-W direction. This is further supported by the resultant drift direction (RDD) value of 92.23°. Additionally, easterly winds accounted for 89% of the total wind power on average. These dynamics classify the Valdevaqueros dune as one of the main climbing dunes on the Iberian Peninsula. The climate of the study area is classified as a hot-summer Mediterranean climate (Csa, according to the Köppen classification), with annual average values of 17 degrees Celsius for temperature and 600 mm for rainfall, based on data from the Spanish Meteorological Agency (AEMET) recorded at the Tarifa station (AEMET (Agencia Estatal de Meteorología), 2024).
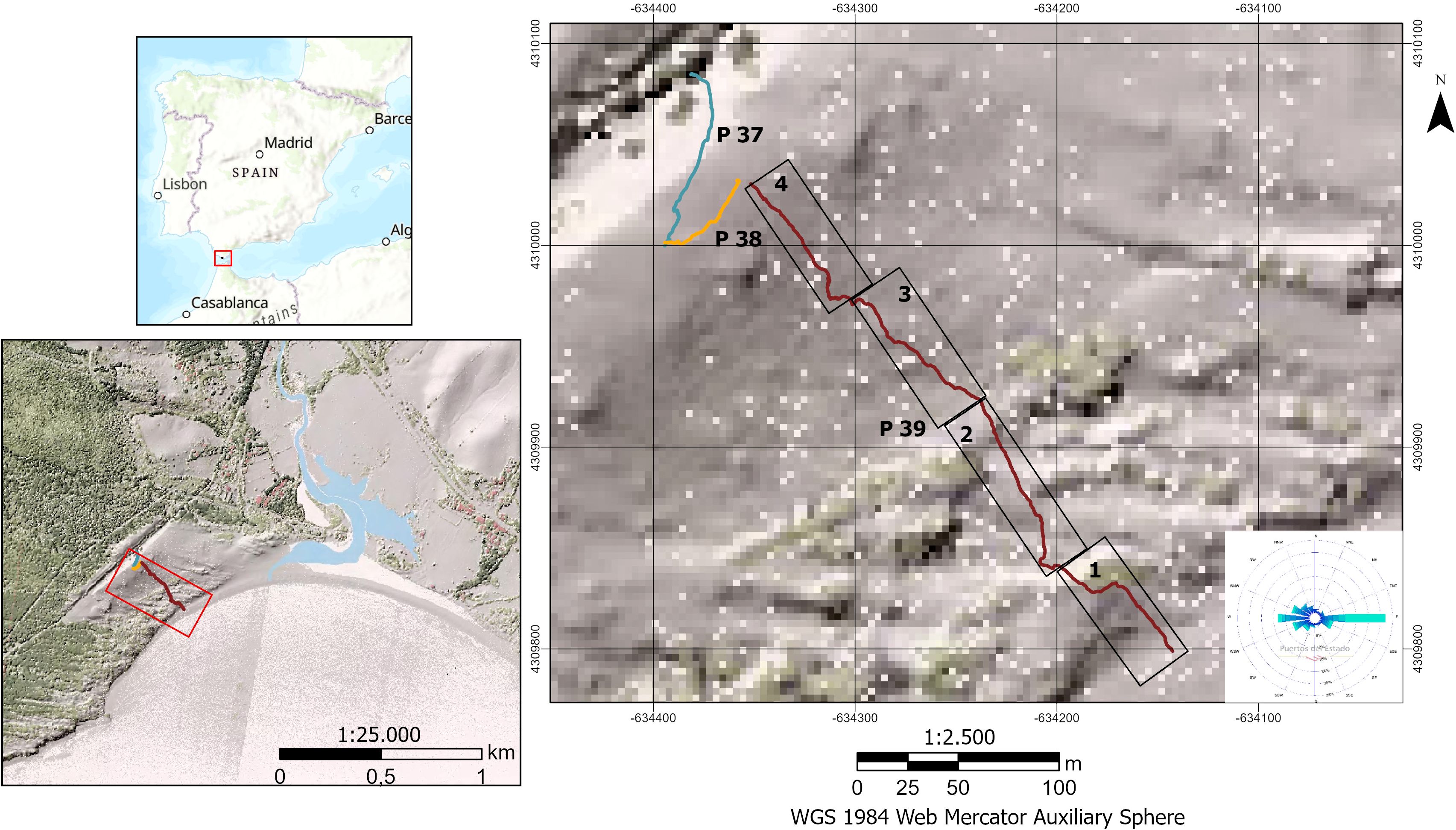
Figure 1. Location of the Valdevaqueros dune and the position of the ground-penetrating radar (GPR) profiles. Profile 39 has been divided into four zones, which are marked. The global wind rose corresponds to the period 2011–2018. (Data sources: Digital Terrain Model (DTM) from the National Geographic Institute; Wind rose from Puertos del Estado, SIMAR Point 6048018).
The sediment transported to the Valdevaqueros dune crest is well-sorted and primarily composed of medium-grained sand (0.3 mm), predominantly quartz (Muñoz-Pérez et al., 2009). González-Martín and Rodríguez-Santalla (2021) estimated a 50% increase in dune volume between 2008 and 2015, from 2,848,296 m³ to 4,320,089 m³, with most sediment accumulation concentrated along the dune crest and its leeward side.
The surrounding beach morphology follows the shape of a Z-shaped bay (Del Río et al., 2013) and includes a small seasonal coastal lagoon formed by the mouth of the Valle River, located at the eastern end of the beach. Typical of this type of beach, erosion is observed in the shaded zone, with an average retreat of 10.4 m and a maximum of 18.5 m, while accretion occurs in the distal zone, east of the Valle River’s mouth, with an average growth of 9.56 m and a maximum of 11.8 m (González-Martín and Rodríguez-Santalla, 2021).
Although coastal dunes provide a wide range of ecosystem services, the rapid migration of the Valdevaqueros dune poses challenges by encroaching on the A-2325 regional road, which provides access to the town of Paloma Baja (Figure 2). This is due to human intervention on the dune corridor that began in the early 1940s that has modified the processes and evolution of the entire physiographic unit (Navarro-Pons et al., 2011, 2016). To date, no effective method has been identified to stabilize the dune, as the strong winds and the large sediment load they carry exceed the retention capacity of vegetation or artificial barriers (Román-Sierra et al., 2004).Various alternatives have been proposed to mitigate this issue (Fages et al., 2007; Bello-Millán et al., 2016), but the dune continues its advance, already covering a significant portion of the adjacent pine forest which was planted in the mid-20th century to halt its progression (Figure 2).
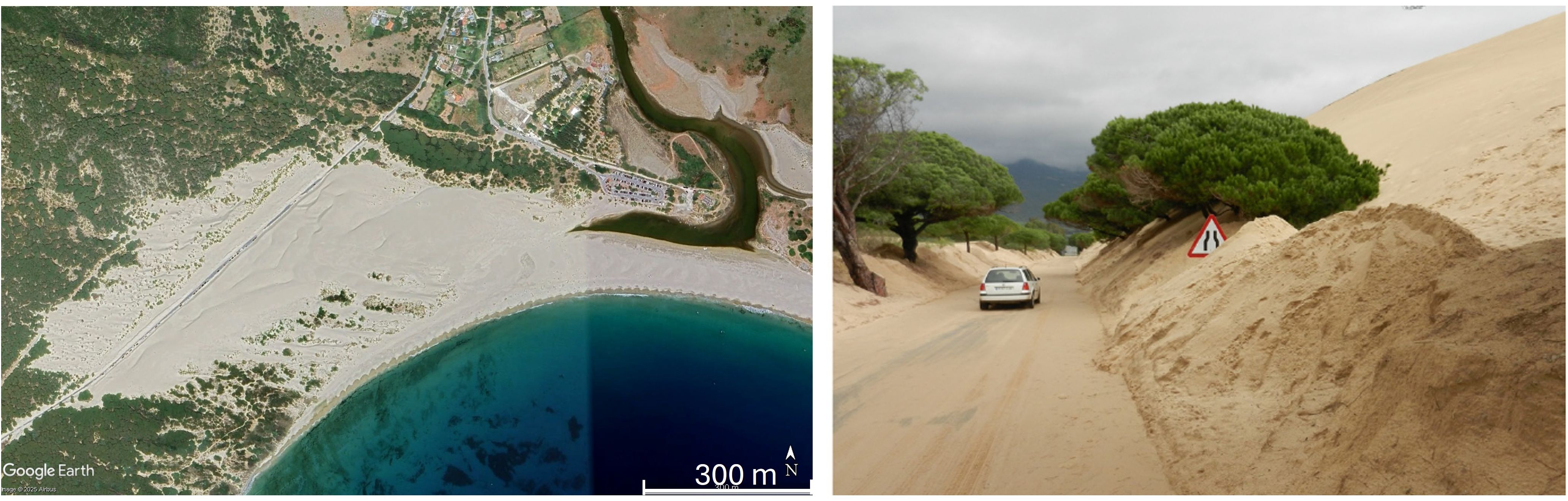
Figure 2. E-2325 regional road affected by sand invasion from the Valdevaqueros dune. Image sources: Google Earth, 2023 (left); Bello-Millán et al., 2016 (right).
Stabilizing the movement of a dune of this magnitude is extremely complex, as all the conditions favoring its mobility are present: abundant sand availability, a sandy fetch exposed to wind, favorable topography, and frequent episodes of strong, dry winds (Gómez-Pina et al., 2002). Figure 3 shows a schematic profile of the Valdevaqueros dune and the maximum, mean and minimum values of the windward and leeward slope angles measured by Muñoz-Pérez et al. (2009) from topographic profiles taken in the cross-dune direction in different surveys (1995, 1999, 2000, 2003 and 2006). Note that sometimes the leeward slope exceeds the angle of repose of the dry sand, so grainflow and avalanche processes are frequent.
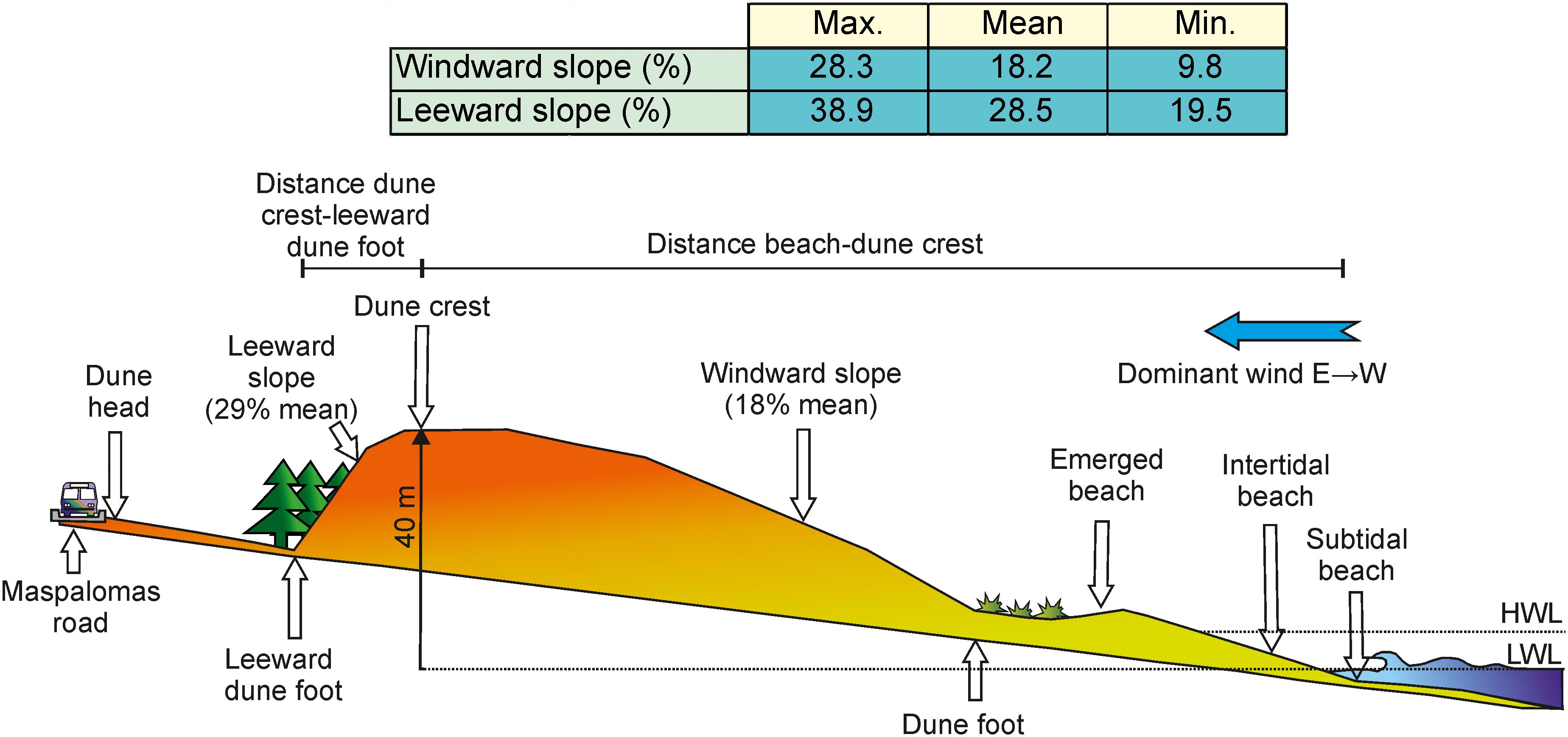
Figure 3. Schematic profile of the Valdevaqueros dune, and the maximum, mean and minimum values of the windward and leeward slope angles. HWL and LWL are High Water Level and Low Water Level respectively. (Modified of Muñoz-Pérez et al., 2009).
3 Materials and methods
According to Martínez-García (2024), dune ecosystems are highly dependent on sedimentary dynamics, wind patterns, and sediment properties. Consequently, understanding their evolution and development requires analyzing the internal structure of the dunes. To address this, three consecutive ground-penetrating radar (GPR) profiles were collected. The position of the profiles is located in the Figure 1. The profiles were obtained using RAMAC equipment with a shielded antenna operating at a central frequency of 250 MHz. The spacing between profile traces was 2 cm, and a calibrated marker wheel ensured equidistance between traces. The topography along the profiles was surveyed using differential GPS, and these data were subsequently used to apply topographic corrections during data processing.
Although published data for EM wave velocities in sedimentary materials are available, each specific study area displays particular dielectric features, due to specific inherent heterogeneities of each of its sedimentary lithologies. For this reason, specific estimations are necessary in order to obtain a mean EM wave velocity value applicable to all profiles, so that a representative dielectric constant could be calculated.
In order to determine the velocity of electromagnetic waves propagating through the sand dunes, the analysis of diffraction hyperbolas observed in the ground-penetrating radar (GPR) profiles was performed. Diffraction hyperbolas arise when an electromagnetic wave encounters a point scatterer or a sharp boundary contrast within the subsurface, leading to a characteristic hyperbolic reflection pattern in the radargram. The shape and curvature of these hyperbolas are directly related to the velocity of wave propagation in the subsurface material. This method provides a reliable, non-invasive means of determining subsurface wave velocities and is particularly useful in environments such as sand dunes, where direct velocity measurements may be challenging.
In this case, a 0.14 m•ns-1 mean velocity was obtained from the analysis of diffraction hyperbolas. Therefore, we can conclude that this value can be taken as representative of the materials in this area. The obtained velocity value is very similar to those published by different authors (e.g. Smith and Jol, 1992; Reynolds, 1997; Costas et al., 2006) for dry sand, which range from 0.12 m•ns-1 to 0.17 m•ns-1. It must be pointed out that the estimated velocity is only valid for the sand material located above the water table, due to the fact that wet sand exhibits lower velocity values, as is well known. As the time windows were determined in order to study mainly the unsaturated zone, we consider that the obtained velocity value is valid for depth determination. Once the velocity data were obtained, a migration process was applied in order to collapse the diffraction hyperbolae and obtain true geometries and depths of the subsurface structures along the profiles.
The obtained velocities were then used to convert the GPR travel times into depth estimates, allowing for a more accurate interpretation of subsurface structures. This method provides a reliable, non-invasive means of determining subsurface wave velocities and is particularly useful in environments such as sand dunes, where direct velocity measurements may be challenging.
Taking all this into account, the radargram processing included the following steps: zero-time correction for each trace, signal saturation correction, application of automatic gain control (AGC), band-pass filtering, static correction, topographic correction, and Kirchhoff migration.
The northernmost profile (profile 37) was acquired on the leeward side of the dune and terminates at the A-2325 road, with a total length of just over 40 meters (Figure 1). The second profile (profile 38) follows a path parallel to the crest of the dune, extending 30 meters. The third profile (profile 39) with a total length of 198 meters is the longest one, covering the entire slope of the windward side and extending up to the coastline. It runs mostly transverse to the dune. The global trajectory of the profiles is oriented N35°W. A dashed line indicates the location of the water table where it was identified. Figure 4 shows a montage with the windward and leeward profiles to give an idea of the dimensions of the Valdevaqueros dune.
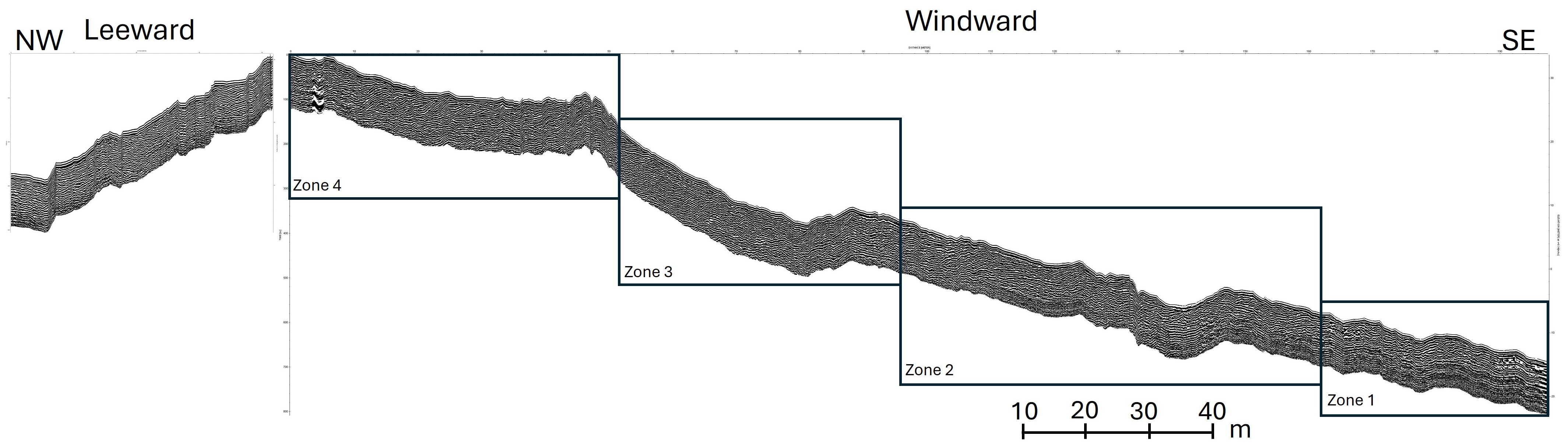
Figure 4. GPR profiles. The leeward side corresponds to profile 37 and windward side to profile 39, whose position is shown in Figure 1. The windward has been divided into four zones to facilitate profile analysis.
4 Results
The processed GPR profiles reveal detailed information about the internal geometry and subsurface characteristics of the climbing dunes, shedding light on the processes shaping their formation and evolution. Terminology used is the recommended by Neal (2004) to define and describe radar surfaces, radar packages, and radar facies. Figure 5 shows the radar facies identified in the GPR profiles.
4.1 Profile 39 – windward face
This profile has been divided into four zones due to its great length (Figure 4), following the upward trend of the dune, from the shoreline to the crest.
Zone 1 (Figure 6): begins with clear evidence of vertical accretion is represented by parallel, laterally continuous, and extensive reflections (1), which are associated with beach deposits (Costas et al., 2006). According to Rodríguez-Santalla et al. (2024) the unit at the base of profile 39 is related to the original dune morphology from the mid-20th century (Navarro-Pons et al., 2011). Between 180–182 m of the profile, convex, stacked, hyperbolic reflections correspond to the burial of protodunes and/or coastal scrub (2) (Costas et al., 2006; Gómez-Ortiz et al., 2009; Warner et al., 2022). Moving inland from the coast, the reflections become inclined, adapting to the slope of the system. Onlap and small roll-over patterns associated with upslope sediment transport by wind are also evident (3).
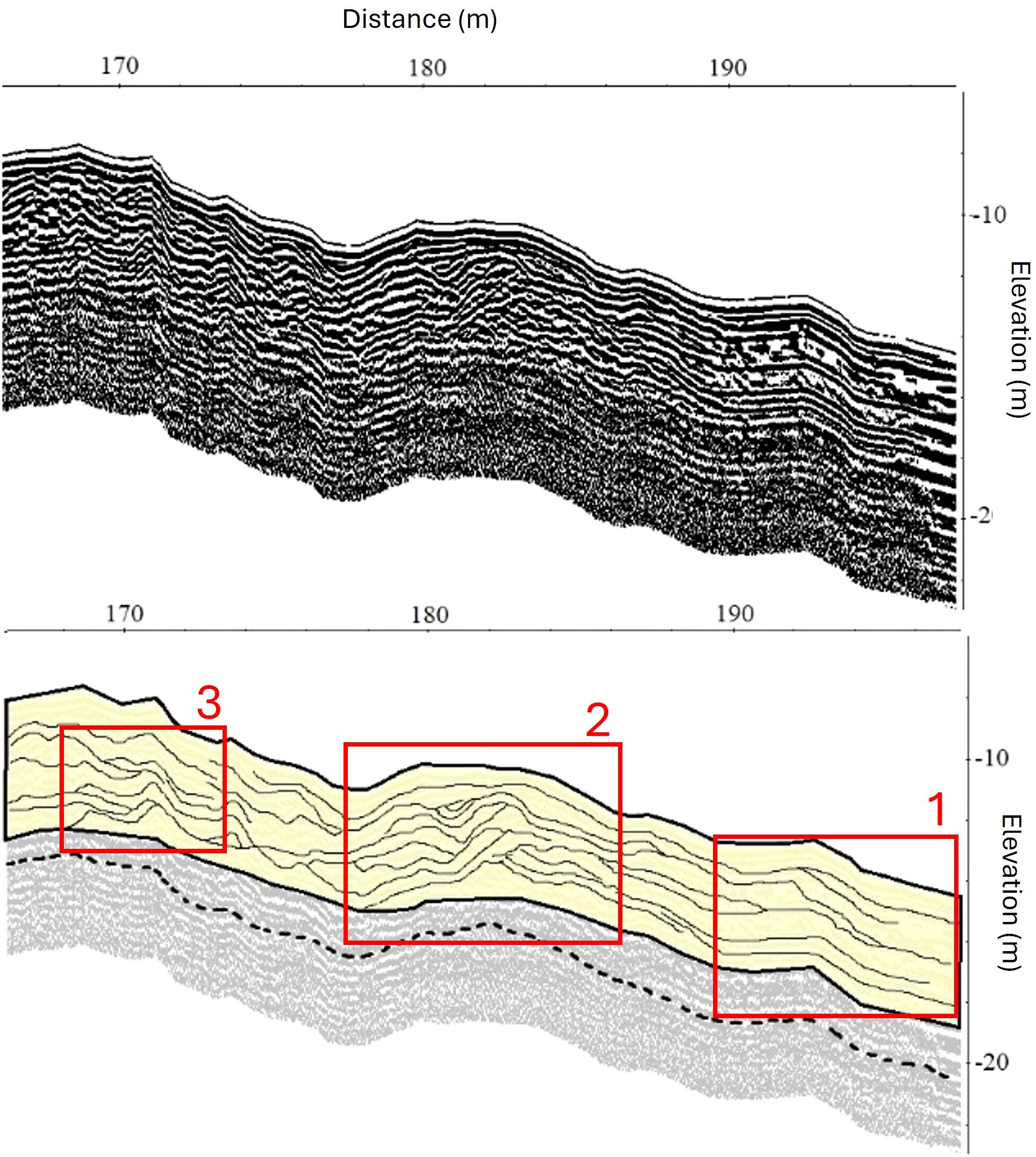
Figure 6. Profile 39 corresponding to Zone 1 of the windward side. Upper panel: processed radargram. Lower panel: interpretation of the radargram. The red boxes show key stratigraphic features. (1) Parallel and laterally continuous reflectors indicating vertical accretion related to beach deposits. (2) Convex, stacked, hyperbolic reflectors interpreted as the burial of protodunes and/or coastal vegetation. (3) Landward-inclined reflectors with onlap and roll-over patterns, associated with upslope sediment transport by wind.
Zone 2 (Figure 7): between 145 and 164 m, undulating, hummocky cross-stratification reflections typical of transgressive dune sequences can be observed (1). These suggest deposition under varying wind conditions (Warner et al., 2022). A central depression marked by toplap reflections terminations and erosional truncations is identified as a blowout (2) (González-Villanueva et al., 2011; Hesp, 2002). Above this structure, prograding and sigmoidal reflection shapes reappear, showing upward sediment transport under consistent wind flow, similar to those previously described in (1).
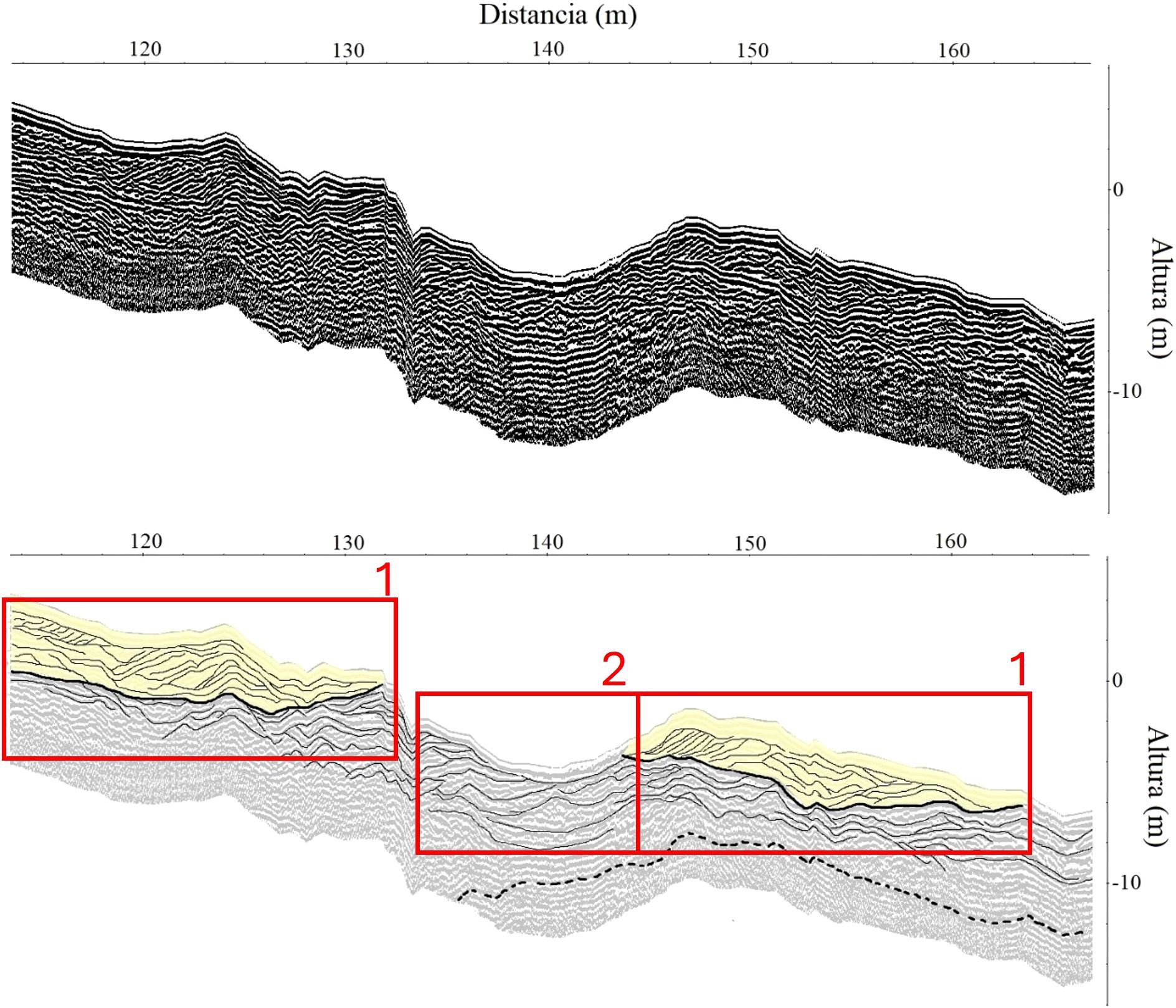
Figure 7. Profile 39 corresponding to Zone 2 of the windward side. Upper panel: processed radargram. Lower panel: interpretation of the radargram. The red boxes show key stratigraphic features. (1) Undulating, high-angle, truncated and discordant reflections interpreted as transgressive dune sequences deposited under variable wind conditions. (2) Central blowout structure characterized by toplap terminations and erosional truncations. Overlying the blowout, prograding sigmoidal reflections indicate renewed sediment accumulation under sustained wind flow.
Zone 3 (Figure 8): between 80 and 94 m, landward-dipping, high-angle, parallel reflections with downlap terminations and continuous stoss slope facies are observed. (1). In the 60–80 m section, convex, stacked, discontinuous, low-angle reflections with seaward dips are identified as foreslope accretion units (2). Above this, the slope becomes steeper, with roll-over structures and trough cross-stratifications developing (3).
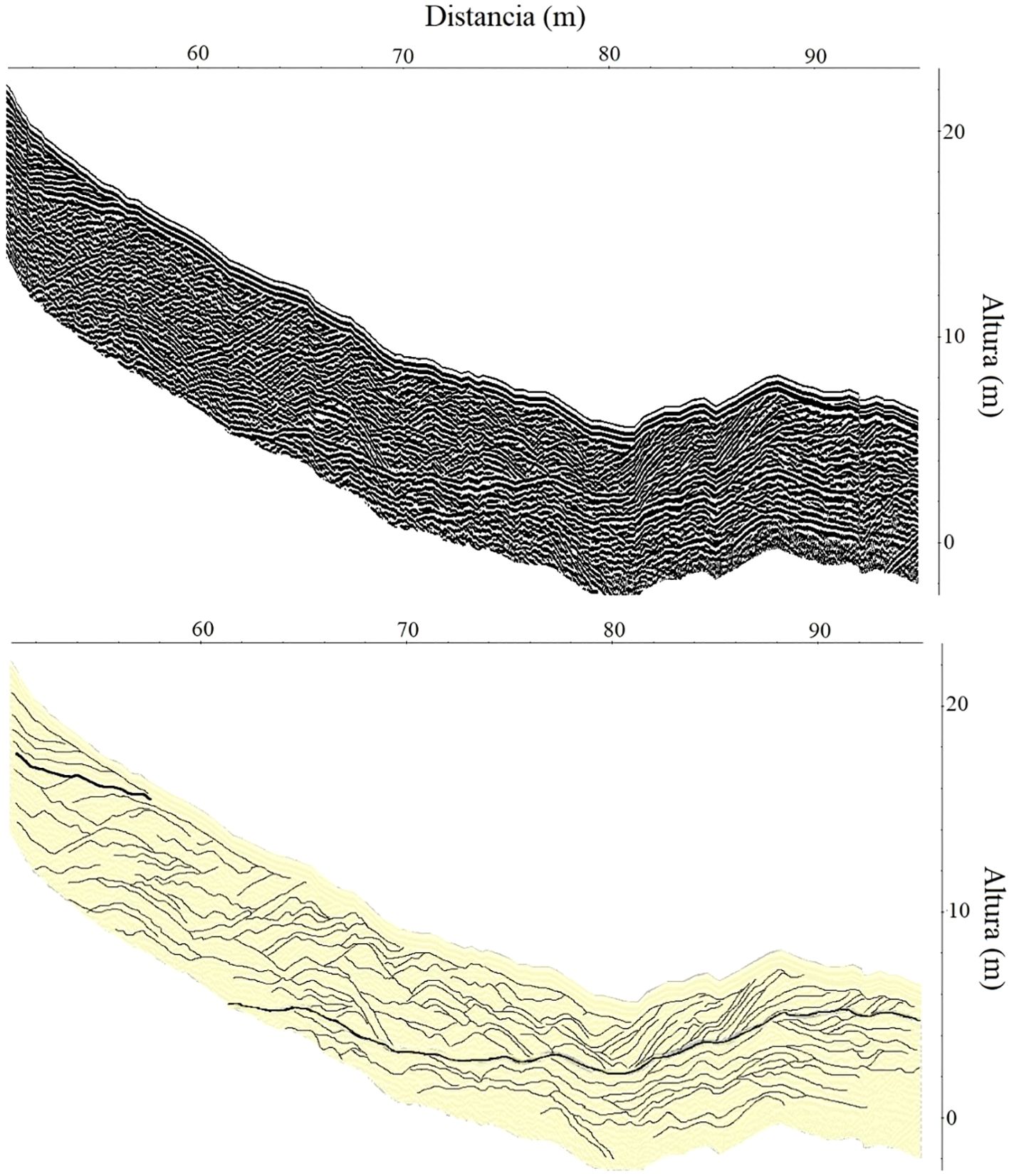
Figure 8. Profile 39 corresponding to Zone 3 of the windward side. Upper panel: processed radargram. Lower panel: interpretation of the radargram. The red boxes show key stratigraphic features. (1) Landward-dipping, high-angle parallel reflections with downlap terminations and continuous slope facies. (2) Below, convex, stacked, discontinuous low-angle reflections with seaward dips are interpreted as foreslope accretion units. (3) Higher up, steeper slopes exhibit roll-over structures and trough cross-stratifications.
Zone 4 (Figure 9): this corresponds to the highest part of the windward face and the dune crest. Between 40 and 60 m, convex, parallel, and concordant reflections suggest vertical accretion (1). In addition to this, on the windward side, erosional truncations caused by sediment being transported toward the crest can be distinguished (1). In the center of this profile (2), a continuous and concave reflection marks a bounding surface that separates different phases of sediment deposition. Above this surface, landward-dipping, high-angle, parallel reflections with downlap and toplap terminations are identified. In zone (3), undulating, landward-dipping, low-angle reflections are observed, showing vertical aggradation.
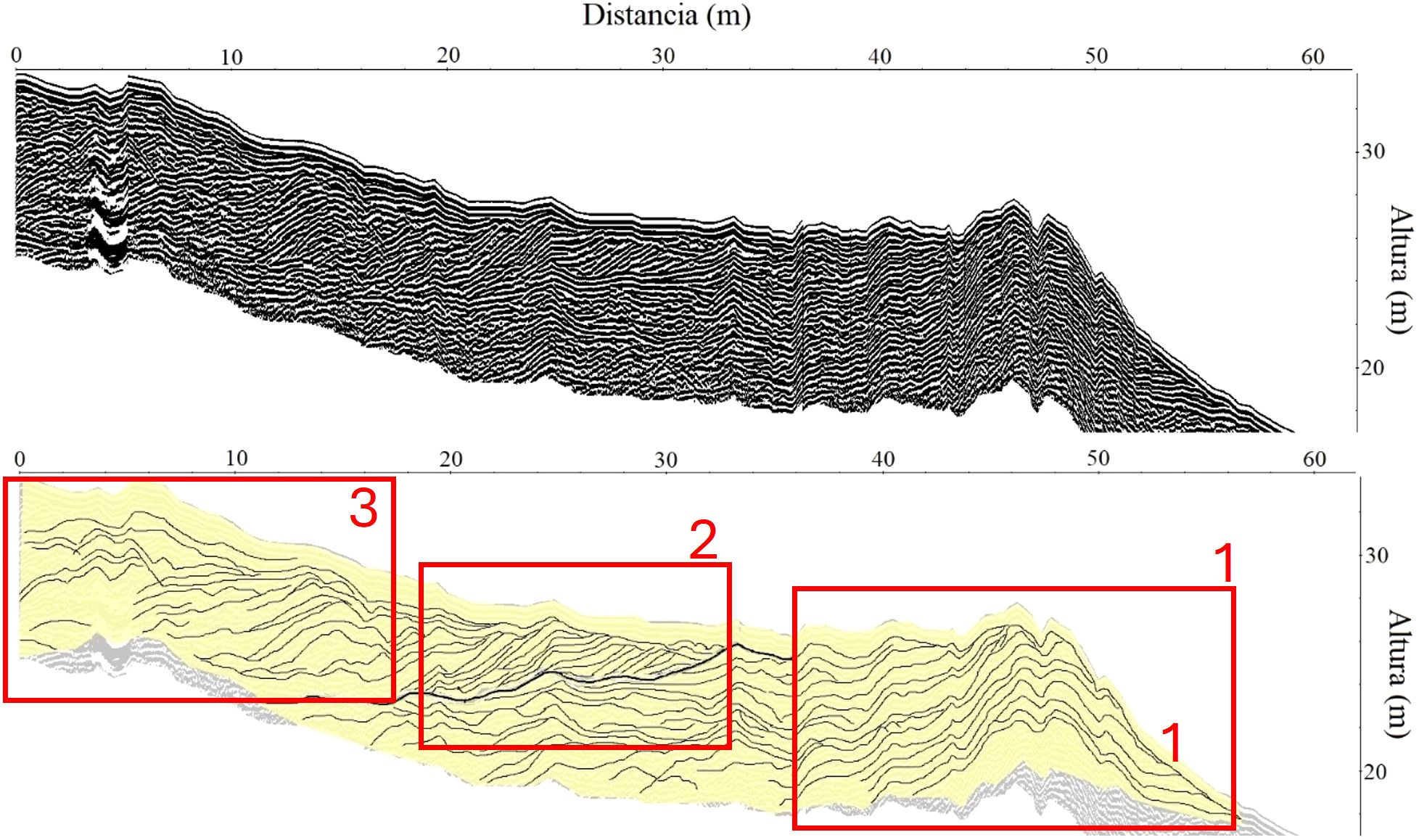
Figure 9. Profile 39 corresponding to Zone 4 of the windward side. Upper panel: processed radargram. Lower panel: interpretation of the radargram. The red boxes show key stratigraphic features. (1) Convex, parallel, concordant reflections indicate vertical accretion, with erosional truncations on the windward slope caused by upslope sediment transport. (2) A continuous concave reflector marks a bounding surface separating different depositional phases. (3) Above, landward-dipping, high- and low-angle reflections with downlap and toplap terminations indicate vertical aggradation.
4.2 Profile 37 – slipface of the Dune
The radargram (Figure 10) shows sand transported from the dune crest, deposited in layers inclined towards the base of the slope, showing cross-bedding with a prograding pattern. Between 20 and 30 m, the reflections exhibit an inclination of 34°, corresponding to the angle of repose of the sediment. These reflections are laterally continuous, subparallel, and show complex sigmoidal geometries. They form downlap relationships due to the aggradation and northward migration of the dune (3). Convex, stacked, and semi-continuous reflections are observed in zone (4) indicating multiple deposition and partial erosion (Warner et al., 2022). At the base of the slipface, sub-horizontal to undulating reflections are visible, showing grainflow (or avalanche) structures and vertical accretion processes (1). Additionally, truncations (2) can be identified near the Maspalomas road.
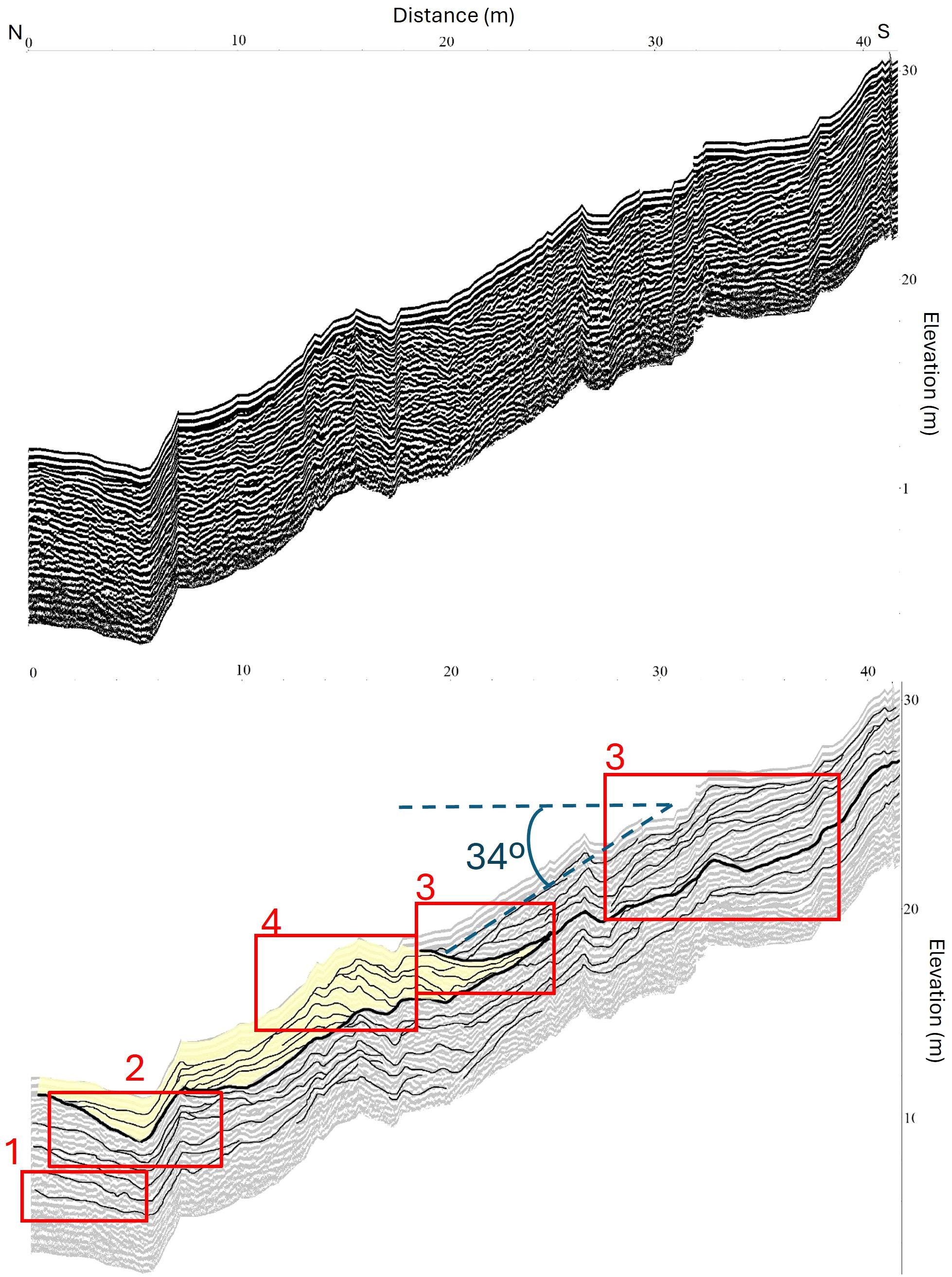
Figure 10. Profile 37 corresponding to the leeward side. Upper panel: processed radargram. Lower panel: interpretation of the radargram. The red boxes show key stratigraphic features. (1) Sub-horizontal to undulating reflections at the base indicate grainflow structures and vertical accretion. (2) Erosional truncations are visible near the Maspalomas road. (3) Inclined, laterally continuous, sigmoidal reflections (34°) with downlap terminations reflect prograding slipface migration. (4) Convex, stacked, semi-continuous reflections suggest multiple deposition events and partial erosion.
4.3 Profile 38 – dune Crest
This profile (Figure 11) represents a longitudinal section of the dune crest oriented SW–NE. This section is strongly influenced by easterly winds, as it is located in the highest area of the dune field. Between 0 and 5 m, continuous, parallel reflections with a convex-upward shape are observed (1). Between 10 and 20 m in zone (2), cross-stratification establishing onlap, downlap, and toplap relationships due to sediment movement towards the crest are imaged. In addition to this, older reflections are truncated by more recent ones, generating erosional truncations (3).
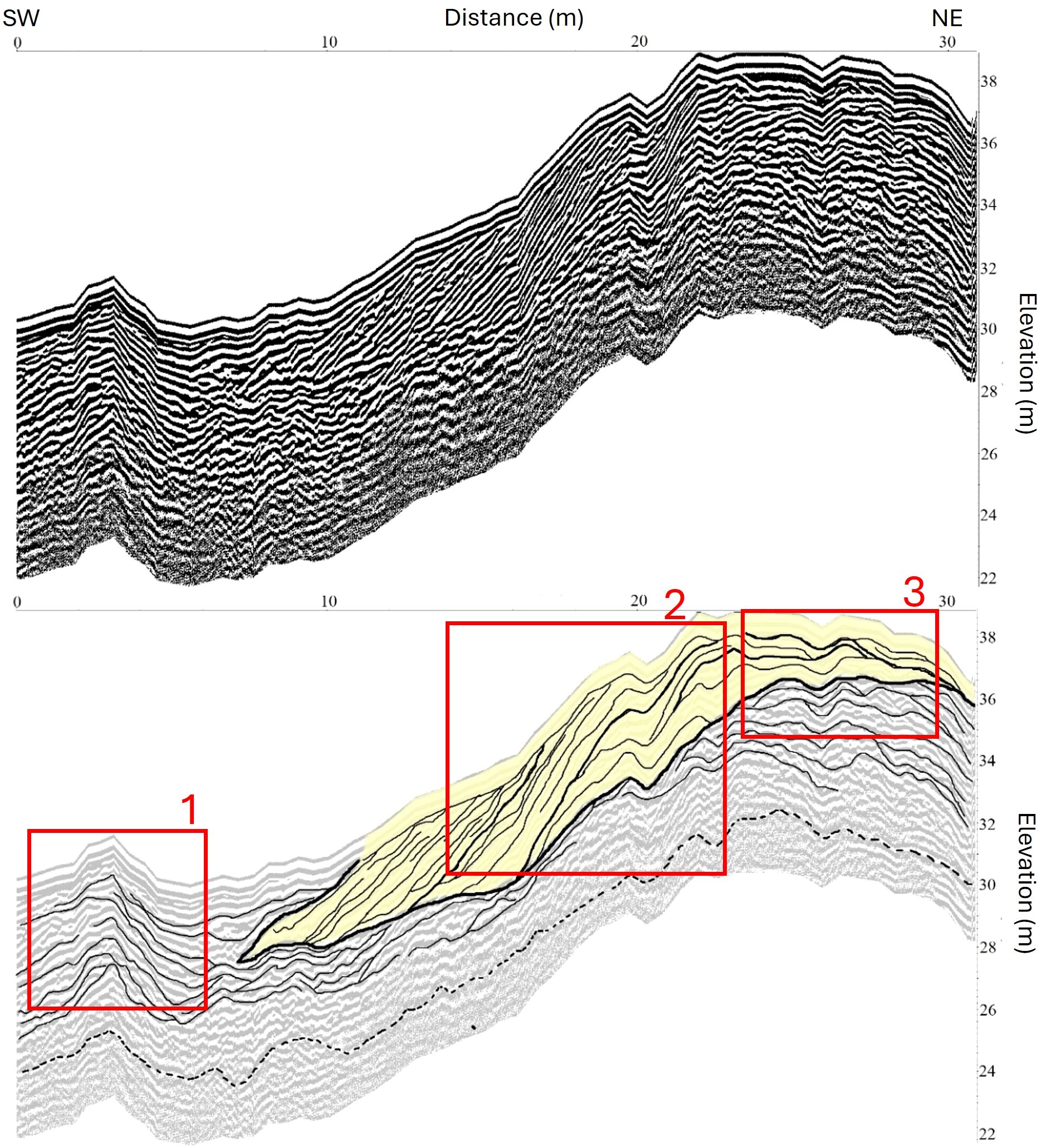
Figure 11. Profile 38 corresponding to the crest of the dune. Upper panel: processed radargram. Lower panel: interpretation of the radargram. The red boxes show key stratigraphic features. (1) Continuous, parallel, convex-upward reflections near the surface. (2) Cross-stratified reflections with onlap, downlap, and toplap terminations indicating sediment transport towards the crest. (3) Erosional truncations where older reflections are cut by more recent ones.
5 Discussion
Some stratigraphic relationships in the radargrams of Valdevaqueros dune resemble those in transgressive coastal dunes (McGourty and Wilson, 2000; Costas et al., 2006; Robin et al., 2022; Warner et al., 2022). However, climbing dunes show unique differences due to their topographic context and sand accumulation on an ascending slope. An interpretation of the different radar facies descriptions in the results section has been provided here.
5.1 Windward face processes
The windward face presents unique stratigraphic relationships resulting from the interaction between prevailing winds, the ascending slope of the terrain, and the constant transport of sand toward the crest and the leeward side. Across the entire windward face (zones 1 to 4), the reflections conform to the underlying topography, adjusting their inclination to the local slope variations. As a general trend, it can be stated that the upslope sand transport generates aggradation patterns characterized by pronounced onlap, downlap, and roll-over structures associated with the wind-driven movement of sediment up the slope. On the other hand, in steeper areas, the reflections are tilted in the direction of dune advancement (Rodríguez-Santalla et al., 2021), reflecting the dune progradation process. In addition to this, variations in wind intensity are recorded as truncations along the profile, which represents the disruption of the deposition process (Fu et al., 2019).
Near the coastline (Zone 1), the structures resemble those in modern foredunes (Hesp, 1988; Harari, 1996; Bristow et al., 2000; Bristow and Pucillo, 2006; Ramos et al., 2010; Fernández et al., 2016);, with parallel and continuous reflections indicating vertical accretion (Costas et al., 2006; Rodríguez-Santalla et al., 2021). Evidence of vegetation burial is also observed in Zone 1 (Figure 6).
The complex, undulating, high-angle inclined reflections, often truncated and discordant reflections described in Zone 2 (1, Figure 7) suggest deposition under varying wind conditions and are typical of dynamic environments where dunes are continuously advancing and reshaping due to changes in wind direction and intensity (Warner et al., 2022). In the same profile but in its central part (Zone 2, (2), Figure 7) a blowout associated with erosive processes has been previously described. According to Hesp (2002), a blowout is a saucer-, cup- or trough-shaped depression or hollow formed by wind erosion on a preexisting sand deposit. Blowouts are common features in coastal dune environments, and their internal structure has been analyzed through GPR by several authors (Neal and Roberts, 2001; González-Villanueva et al., 2011; Baird et al., 2021, among others).
In the same profile but in Zone 3 (Figure 8), different processes of dune dynamics can be inferred from the different radar facies observed. For example, between 80 and 94 m of the profile, the landward-dipping, high-angle, parallel, with downlap terminations and continuous stoss slope facies observed are interpreted as associated with dune accretion and migration (1). However, the convex, stacked, discontinuous, low-angle reflections with seaward dips and downlap terminations identified in (2) can be interpreted, according to Bristow et al. (2000), as foreslope accretion units. Moreover, the roll-over structures and trough cross-stratifications described in (3) indicate the migration of sediment up the windward slope toward the dune crest (Bristow and Pucillo, 2006; Costas et al., 2006; Rodríguez-Santalla et al., 2021).
Focusing on highest part of the windward face and the dune crest area (profile 39, Figure 9, Zone 4), different radar facies has been described. Whereas the convex, parallel, and concordant reflections observed indicate a process of successive vertical accretion of the dune (40-60 m of the profile in (1)), the erosional truncations imaged at the windward side are associated with dune accretion in the foreset (2) and the migration of sediment toward the dune crest. These dome-shaped reflections, inclined in favor of the slope, are similar to those described by Flor-Blanco et al. (2016) in the Xagó dune field, in Asturias. Different form this, the undulating, landward-dipping, low-angle reflection described in (3) reflect the tendency of the dune to advance due to the constant deposition of wind-transported sand and are characteristic of transgressive dune sequences (Warner et al., 2022).
5.2 Dune crest processes
The dune crest (Figure 11) is the area most affected by strong winds (Navarro-Pons et al., 2011), so it is normal to find truncations between the reflections associated with the removal of sediments. Again, different radar facies have been previously described in the results section. For example, the truncation of older reflections by more recent ones generating the erosional truncations described in (3) indicates processes such as sand removal by strong winds or changes and reactivations in wind direction that generate deflation at the crest (Rodríguez-Santalla et al., 2009).
Different to this, in the transition zone from the crest to the leeward slope, the cross-stratifications establishing onlap, downlap, and toplap relationships observed at (2) are a results of the easterly winds pushing sediment towards the dune crest. This process leads to dune aggradation by foreslope accretion, producing reflections with stratification patterns of cut-and-fill and rollover (2), as described by Bristow et al. (2000) and more recently by Rodríguez-Santalla et al. (2021). The material is deposited by sliding or falling sand (grainfall) (Eastwood, 2011) and the reflections are inclined downwind, from the brink of the dune, establishing downlap relationships. Sigmoidal or complex relationships are also observed, with curved reflections typical of dynamic sedimentation structures in active dunes (Hugenholtz et al., 2007). These relationships result from the interaction of transport and deposition processes combined with the morphology of the dune crest (Brothers et al., 2017).
Finally, aggradation process is also evident in (1), with continuous and parallel reflections with a convex-upward shape observed associated with the vertical aggradation of the topset This reflects the tendency of the dune to advance due to the constant deposition of wind-transported sand.
5.3 Slipface processes
In general, the structures defined on the slipface (profile 37, Figure 10) do not differ significantly from those described in transgressive coastal dunes (Clemmensen et al., 2001; Brothers et al., 2017; Fu et al., 2019; Ribolini et al., 2021). The stratigraphic relationships between the reflections are the result of the sedimentary processes associated with the grainfall (Eastwood, 2011) and vertical accumulation resulting in dune aggradation under a consistent sand supply over time. Grainfall deposits typically form cross-bedding with a prograding pattern (Hunter, 1977; Harari, 1996; Fu et al., 2019; Eastwood, 2011). The reflections are inclined toward the base of the slope, with inclinations close to the sediment’s angle of repose (30° to 34°for dry sand). They exhibit a parallel, laterally continuous arrangement, forming a prograding pattern. The progressive accumulation of sand layers on the slope as the dune advances generates onlap and downlap relationships between the reflections (Bristow et al., 2000; Bristow and Pucillo, 2006; Costas et al., 2006; Rodríguez-Santalla et al., 2021). Truncations are frequently associated with episodes of erosion caused by changes in wind direction or intensity, which remove previous layers before the deposition of new sand sheets, reflecting the interplay of depositional and erosive processes. At the base of the avalanche face, it is possible to find horizontal or slightly inclined reflections representing vertical sediment accumulation and grainflow deposits (avalanche) (Eastwood, 2011), marking older sand deposits or stabilized underlying units (González-Martín and Rodríguez-Santalla, 2021). However, in the case of the Valdevaqueros dune, these reflections are disrupted due to sand removal activities associated with road maintenance.
6 Conclusions
The internal structure of the climbing dunes shows a variety of sedimentary structures that differ along the profile due to the different intensities and directions of the wind, as well as the pronounced topographic control that influences them. These processes are supported by an abundant sand supply. The Valdevaqueros dune represents a wide catalogue of these structures. The reflections adapt to the slope of the underlying terrain, showing structures indicative of upward transport and aggradation. The different phases of sediment deposition associated with wind reactivations are represented by bounding surfaces. In the transition from the crest to the leeward slope, material is deposited through grainfall, At the base of the leeward face, the reflections form prograding patterns through grainflow and vertical accumulation.
The analysis of these radargrams further expands the description of sedimentary structures characteristic of climbing dunes, which is scarce in the literature. Additionally, this study expands the understanding of the dynamics of the Valdevaqueros dune.
Data availability statement
The original contributions presented in the study are included in the article/supplementary material. Further inquiries can be directed to the corresponding author.
Author contributions
IR-S: Conceptualization, Data curation, Formal Analysis, Investigation, Methodology, Supervision, Validation, Writing – original draft, Writing – review & editing. DG-O: Conceptualization, Data curation, Formal Analysis, Investigation, Methodology, Software, Supervision, Validation, Writing – review & editing. JJM-P: Conceptualization, Data curation, Funding acquisition, Investigation, Project administration, Resources, Validation, Writing – review & editing.
Funding
The author(s) declare that no financial support was received for the research and/or publication of this article.
Conflict of interest
The authors declare that the research was conducted in the absence of any commercial or financial relationships that could be construed as a potential conflict of interest.
Generative AI statement
The author(s) declare that no Generative AI was used in the creation of this manuscript.
Publisher’s note
All claims expressed in this article are solely those of the authors and do not necessarily represent those of their affiliated organizations, or those of the publisher, the editors and the reviewers. Any product that may be evaluated in this article, or claim that may be made by its manufacturer, is not guaranteed or endorsed by the publisher.
References
AEMET (Agencia Estatal de Meteorología) (2024). Climatology of Spain: Data for the period 1981-2010. Available online at: https://www.aemet.es (Accessed October 10, 2024).
Baird T. R., Bristow C. S., Luo W., Du E., Bryant R. G., Mitchell T. M., et al. (2021). Blowout morphometrics and mass balances. Front. Earth Sci. 9. doi: 10.3389/feart.2021.669440
Bello-Millán F. J., Somoano M., Clavero M., Gómez-Pina G., Losada M. A. (2016). El sistema dunar de Valdevaqueros: evolución histórica y alternativas de gestión. RIBAGUA - Rev. Iberoamericana del Agua 3, 46–55. doi: 10.1016/j.riba.2016.07.001
Bristow C., Chroston P. N., Bailey S. D. (2000). The structure and development of foredunes on a locally prograding coast: insights from ground penetrating radar surveys. Sedimentology 47, 923–944. doi: 10.1046/j.1365-3091.2000.00330.x
Bristow C., Pucillo K. (2006). Quantifying rates of coastal progradation from sediment volume using GPR and OSL: The Holocene fill of Guichen Bay, south-east South Australia. Sedimentology. 53, 769–788. doi: 10.1111/j.1365-3091.2006.00792.x
Brothers S. C., Kocurek G., Brothers T. C., Buynevich I. V. (2017). Stratigraphic architecture resulting from dune interactions: White Sands Dune Field, New Mexico. Sedimentology 64, 686–713. doi: 10.1111/sed.2017.64.issue-3
Clemmensen L. B., Fornós J. J., Rodríguez-Perea A. (1997). Morphology and architecture of a late Pleistocene cliff-front dune, Mallorca, Western Mediterranean. Terra Nova 9, 251–254. doi: 10.1111/j.1365-3121.1997.tb00023.x
Clemmensen L. B., Pye K., Murray A., Heinemeier J. (2001). Sedimentology, stratigraphy and landscape evolution of a Holocene coastal dune system, Lodbjerg, NW Jutland, Denmark. Sedimentology 48, 3–27.
Costas S., Alejo I., Rial F., Lorenzo H., Nombela M. A. (2006). Cyclical evolution of a modern transgressive sand barrier in northwestern Spain elucidated by GPR and aerial photos. J. Sedimentary Res. 76, 1077–1092. doi: 10.2110/jsr.2006.094
Del Río L., Gracia F. J., Benavente J. (2013). Morphological and evolutionary classification of sandy beaches in Cadiz coast (SW Spain). J. Coast. Res. 65, 2113–2118. doi: 10.2112/SI65-357.1
Dłużewski M., Rotnicka J., Hesp P., Tomczak J. (2023). Airflow dynamics and aeolian sand transport across a beach-climbing dune-clifftop dune system. J. Geophysical Research: Earth Surface 128. doi: 10.1029/2023JF007316
Dong M., Yan P., Wang X., Zhang X., Wu W., Wang Y., et al. (2023). Morphological characteristics and dynamic changes of typical climbing dunes on the Qinghai-Tibet Plateau. Geomorphology 440, 108869. doi: 10.1016/j.geomorph.2023.108869
Eastwood E. N. (2011). Reconstructing environmental forcings on aeolian dune fields: results from modern, ancient, and numerically-simulated dunes, 177 pp. Dissertation presented to the Faculty of the Graduate School of The University of Texas at Austin. (Austin (USA): University of Texas).
Fages L., Gómez-Pina G., Navarro M., Román-Sierra J., Giménez-Cuenca M., Ruiz J. A., et al. (2007). “Coastal management activities in Valdevaqueros Dune Area (Tarifa, SW Spain),” in International Conference on Management and Restoration of Coastal Dunes (ICCD 2007). (Santander (Spain): Universidad de Cantabria), 168–171.
Fernández G. B., Figueiredo M. S., Rocha T. B., Maluf V. B., Martins C., Moulton M. B. A. (2016). Foredunes morphological changes by offshore winds revealed by grund-penetrating radar: massambaba beach – Rio de Janeiro, Brazil. J. Coast. Res. 75, 278–282. doi: 10.2112/SI75-56.1
Flor-Blanco G., Rubio D., Flor G., Fernández J. P. (2012). “Estructura interna e interpretación de la evolución del campo dunar eólico de Xagó (costa central de Asturias, NO de España),” in 7° Simpósio sobre a Margem Ibérica Atlântica, Lisboa, Vol. 2012. (Lisboa (Portugal): Universidad de Lisboa), 71–76.
Flor-Blanco G., Rubio-Melendi D., Flor G., Fernández-Álvarez J. P., Jackson D. W. T. (2016). Holocene evolution of the Xagó dune field (Asturias, NW Spain) reconstructed by means of morphological mapping and ground penetrating radar surveys. Geo-Marine Lett. 36, 35–50. doi: 10.1007/s00367-015-0427-1
Ford R., Gillman S. L., Wilkins D., Clement W. P., Kathleen N. (2010). Geology and geomorphology of coral pink sand dunes state park. Utah Geological Assoc. Publication. 28, 379–406.
Fryberger S. G. (1979). “Dune forms and wind regime.” in A Study of Global Sand Seas, Geological Survey Professional Paper 1052, ed. McKee E. D. (Washington, DC: United States Department of Interior), 137–169.
Fu T., Wu Y., Tan L., Li D., Wen Y. (2019). Imaging the structure and reconstructing the development of a barchan dune using ground-penetrating radar. Geomorphology 341, 192–202. doi: 10.1016/j.geomorph.2019.05.014
Gómez-Ortiz D., Martín-Crespo T., Rodríguez I., Sánchez M. J., Montoya I. (2009). The internal structure of modern barchan dunes of the Ebro River Delta (Spain) from ground penetrating radar. J. Appl. Geophysics 68, 159–170. doi: 10.1016/j.jappgeo.2008.11.007
Gómez-Pina G., Muñoz-Pérez J. J., Ramírez J. L., Ley C. (2002). Sand dune management problems and techniques, Spain. J. Coast. Res. 36, 325–332. doi: 10.2112/1551-5036-36.sp1.325
González-Martín L., Rodríguez-Santalla I. (2021). Analysis of the dynamics of the Valdevaqueros dune. Environ. Sci. Proceeding. Available online at: https://sciforum.net/manuscripts/11643/manuscript.pdf.
González-Villanueva R., Costas S., Duarte H., Pérez-Arlucea M., Alejo I. (2011). Blowout evolution in a coastal dune using GPR, aerial imagery and core records. J. Coast. Res. 64, 278–282. Available at: https://www.jstor.org/stable/26482177 (Accessed January 15, 2025).
Harari Z. (1996). Ground-penetrating radar (GPR) for imaging stratigraphic features and groundwater in sand dunes. J. Appl. Geophysics 36, 43–52. doi: 10.1016/S0926-9851(96)00031-6
Hesp P. A. (1988). Morphology, dynamics and internal stratification of some established foredunes in Southeast Australia. Sedimentary Geology. 55, 17–41. doi: 10.1016/0037-0738(88)90088-7
Hesp P. A. (2002). Foredunes and blowouts: initiation, geomorphology and dynamics. Geomorphology 48, 245–268. doi: 10.1016/S0169-555X(02)00184-8
Hesp P. A. (2011). “Dune coasts,” in Treatise on Estuarine and Coastal Science, vol. 3. Eds. Wolanski E., McLusky D. S. (Academic Press, Waltham), 193–221. doi: 10.1016/B978-0-12-374711-2.00310-7
Hesp P. A., Smyth T. A. G. (2019). CFD flow dynamics over model scarps and slopes. Phys. Geogr. 42, 1–24. doi: 10.1080/02723646.2019.1706215
Hugenholtz C. H., Moorman B. J., Wolfe S. A. (2007). “Ground penetrating radar (GPR) imaging of the internal structure of an active parabolic sand dune,” in Stratigraphic Analyses Using GPR: Geological Society of America Special Paper, vol. 432 . Eds. Baker G. S., Jol H. M.. (Boulder, Colorado, USA: Geological Society of America), 35–45. doi: 10.1130/2007.2432(03
Hunter R. E. (1977). Basic types of stratification in small eolian dunes. Sedimentology 24, 361–387. doi: 10.1111/j.1365-3091.1977.tb00128.x
Ley C., Gallego J. B., Vidal C. (2007). Manual de restauración de dunas costeras. Ministerio de Medio Ambiente y Medio Rural y Marino (Madrid: Centro de Publicaciones), 251pp.
Martínez-García F. P. (2024). Transferencia espacial de datos de viento. Aplicación a la Duna de Valdevaqueros (Cádiz). Cádiz (Spain): Universidad de Cádiz, 197 pp. Tesis Doctoral.
Martínez-García F. P., Contreras-de-Villar A., Muñoz-Perez J. J. (2021). Review of wind models at a local scale: advantages and disadvantages. J. Mar. Sci. Eng. 9, 318. doi: 10.3390/jmse9030318
Martínez-Garcia F. P., Muñoz-Perez J. J., Contreras-de-Villar A., Contreras F., Jigena-Antelo B. (2023). A methodology to design a wind transfer function: application to the valdevaqueros dune (SW Spain). J. Mar. Sci. Eng. 11, 923. doi: 10.3390/jmse11050923
Martins M. V. A., Rey D., Pereira E., Plaza-Morlote M., Salgueiro E., Moreno J., et al. (2018). Influence of dominant wind patterns in a distal region of the NW Iberian Margin during the last glaciation. J. Geological Soc. 175, 321–335. doi: 10.1144/jgs2017-075
McGourty J., Wilson P. (2000). “Investigating the internal structure of Holocene coastal sand dunes using ground-penetrating radar: example from the north coast of Northern Ireland.” in Proceedings of the Eighth International Conference on Ground Penetrating Radar. (Washington (USA): International Society for Optics and Photonics), 14–19. doi: 10.1117/12.383554
Muñoz-Pérez J. J., Navarro M., Román-Sierra J., Tejedor B., Rodríguez-Santalla I., Gómez-Pina G. (2009). Long-term evolution of a transgressive migration dune using reconstruction of the EOF method. Geomorphology 112, 167–177. doi: 10.1016/j.geomorph.2009.05.016
Navarro M. (2011). Modelización de la evolución morfodinámica de la duna de Valdevaqueros (T.M. Tarifa) mediante la aplicación de funciones empíricas ortogonales a corto, medio y largo plazo. Universidad de Cádiz, España, Cádiz (Spain). Tesis Doctoral.
Navarro M., Muñoz-Pérez J. J., Román-Sierra J., Ruiz-Cañavante A., Gómez-Pina G. (2015). Characterization of wind-blow sediment transport with height in a highly mobile dune (SW Spain). Geologica Acta 13, 155–166. doi: 10.1344/GeologicaActa2015.13.2.6
Navarro-Pons M., Muñoz-Pérez J. J., Román-Sierra J., García S. (2016). Evidence of coastal dune mobility increases over the last half century in response to historical human intervention. Scientia Marina 80, 1–12. doi: 10.3989/scimar.04336.16A
Navarro-Pons M., Muñoz-Pérez J. J., Román-Sierra J., Tsoar H., Rodríguez I., y Gómez-Pina G. (2011). Assessment of highly active dune mobility in the medium, short and very term. Geomorphology 129, 14–28. doi: 10.1016/j.geomorph.2011.01.009
Neal A. (2004). Ground-penetrating radar and its use in sedimentology: principles, problems and progress. Earth-Science Rev. 66, 261–330. doi: 10.1016/j.earscirev.2004.01.004
Neal A., Roberts C. L. (2001). Internal structure of a trough blowout, determined from migrated ground-penetrating radar profiles. Sedimentology 4, 791–810. doi: 10.1046/j.1365-3091.2001.00382.x
Núñez Ravelo F., Hernández Labrador M., Ugas Pérez M. (2016). Geomorfología de la duna remontante ubicada en el acantilado sur del Castillo de Araya, estado Sucre (Venezuela). Cuadernos Geografía: Rev. Colombiana Geografía 26, 51–63. doi: 10.15446/rcdg.v26n1.52804
Ramos R., Freitas M., Andrade C., Costas S., Bristow C., Grangeia C., et al. (2010). “Sedimentary structure of the Nazaré coastal dunes (Portugal),” in Proceedings of the 13th International Conference on Ground Penetrating Radar, GPR 2009. (New York, (USA): Institute of Electrical and Electronics Engineers), 1–6. doi: 10.1109/ICGPR.2010.5550148
Reynolds J. M. (1997). An Introduction to Applied and Environmental Geophysics (New York, USA: John Wiley), 796 p.
Ribolini A., Bertoni D., Bini M., Sarti G. (2021). Ground-penetrating radar prospections to image the inner structure of coastal dunes at sites characterized by erosion and accretion (Northern Tuscany, Italy). Appl. Sci. 11, 11260. doi: 10.3390/app112311260
Robin N., Billy J., Alexandre N.-L., Castelle B., Hesp P., Rosebery D., et al. (2022). Natural remobilization and historical evolution of a modern coastal transgressive dunefield. Earth Surface Processes Landforms 48, 1–20. doi: 10.1002/esp.5535
Rodríguez-Santalla I., Gómez-Ortiz D., González-Martin L., Muñoz-Pérez J. J. (2024). “Estructura interna de la duna de Valdevaqueros, Cádiz,” in Proceeding of the XII Jornadas de Geomorfología Litoral. (Valencia (Spain): Universitat Politècnica de València), 25-27 de septiembre de 2024. doi: 10.4995/GEOLIT24.2024.18707
Rodríguez-Santalla I., Gómez-Ortiz D., Martín-Crespo T., Sánchez M. J., Montoya-Montes I., Martín-Velázquez S., et al. (2021). Study and evolution of the dune field of la banva spit in ebro delta (Spain) using liDAR data and GPR. Remote Sens. 13, 17. doi: 10.3390/rs13040802
Rodríguez-Santalla I., Sánchez M. J., Montoya I., Gómez D., Martín T., Serra J. (2009). Internal structure of the aeolian sand dunes of El Fangar spit, Ebro Delta (Tarragona, Spain). Geomorphology 104, 238–252. doi: 10.1016/j.geomorph.2008.08.017
Román-Sierra J., Navarro-Pons M., Gomez-Pina G., Fages-Antiñolo L., Muñoz-Pérez J. J. (2004). Ecosistemas dunares en la provincia de Cádiz: Estabilizaciones en Bolonia y Valdevaqueros, TM Tarifa. Rev. Obras Públicas N. 3450, 65–76.
San Romualdo Collado A. (2022). Caracterización biogeomorfológica de la duna costera (foredune) en sistemas playa-duna áridos: naturaleza, amenazas y gestión. PhD Programa de Doctorado en Oceanografía y Cambio Global por la Universidad de Las Palmas de Gran Canaria. (Las Palmas de Gran Canaria (Spain): Universidad de Las Palmas de Gran Canaria).
Smith D. G., Jol H. M. (1992). Ground penetrating radar results to infer depositional processes of coastal spits in large lakes. Geological Survey Finland Special Paper 16, 169–177.
Tsoar H. (1983). “Wind tunnel modelling of echo and climbing dunes,” in Eolian Sediments and Processes. Eds. Brookfield M. E., Ahlbrandt T. S. (Elsevier, Amsterdam), 247–259.
Tsoar H., White B. R., Berman E. (1996). The effect of slopes on sand transport - numerical modelling. Landscape Urban Plann. 34, 171–181. doi: 10.1016/0169-2046(95)00235-9
Vallarino-Castillo R., Negro-Valdecantos V., del Campo J. M. (2023). Understanding the impact of hydrodynamics on coastal erosion in Latin America: a systematic review. Front. Environ. Sci. 11, 1267402. doi: 10.3389/fenvs.2023.1267402
Wang X., Ping Y., Dong M., Zhang X., Wang Y., Wang Y., et al. (2022). The effect of gradient and shape on the formation process of climbing dunes in a wind-tunnel experiment. J. Geophysical Research: Earth Surface 127. doi: 10.1029/2022JF006858
Warner A. L., Hesp P. A., Keane R., Gontz A. M. (2022). Ground penetrating radar of paleoblowouts and transgressive dunes, younghusband peninsula, south Australia. J. Coast. Res. 38, 885–895. doi: 10.2112/JCOASTRES-D-20-00107.1
White B., Tsoar H. (1998). Slope effect on saltation over a climbing sand dune. Geomorphology 22, 159–180. doi: 10.1016/S0169-555X(97)00058-5
Keywords: climbing dunes, ground penetrating radar (GPR), sedimentary structure, radar facies, Valdevaqueros dune, Cádiz
Citation: Rodríguez-Santalla I, Gomez-Ortiz D and Muñoz-Perez JJ (2025) Review of the internal structure of a climbing dune. The case of the Valdevaqueros dune (Cádiz, Spain). Front. Mar. Sci. 12:1568474. doi: 10.3389/fmars.2025.1568474
Received: 29 January 2025; Accepted: 28 March 2025;
Published: 24 April 2025.
Edited by:
Giandomenico Foti, Mediterranea University of Reggio Calabria, ItalyReviewed by:
Charles Bristow, University of London, United KingdomLucas Vimpere, University of Geneva, Switzerland
Copyright © 2025 Rodríguez-Santalla, Gomez-Ortiz and Muñoz-Perez. This is an open-access article distributed under the terms of the Creative Commons Attribution License (CC BY). The use, distribution or reproduction in other forums is permitted, provided the original author(s) and the copyright owner(s) are credited and that the original publication in this journal is cited, in accordance with accepted academic practice. No use, distribution or reproduction is permitted which does not comply with these terms.
*Correspondence: Inmaculada Rodríguez-Santalla, aW5tYWN1bGFkYS5yb2RyaWd1ZXpAdXJqYy5lcw==; Juan J. Muñoz-Perez, anVhbmpvc2UubXVub3pAdWNhLmVz