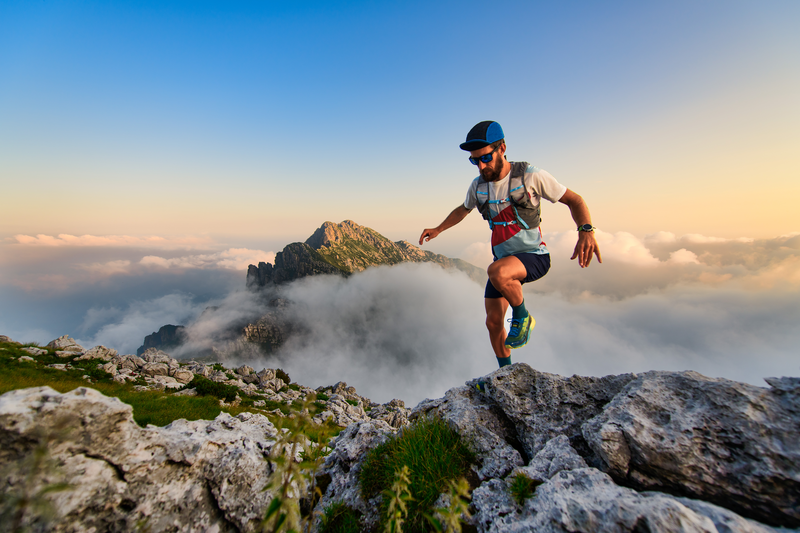
94% of researchers rate our articles as excellent or good
Learn more about the work of our research integrity team to safeguard the quality of each article we publish.
Find out more
ORIGINAL RESEARCH article
Front. Mar. Sci.
Sec. Coastal Ocean Processes
Volume 12 - 2025 | doi: 10.3389/fmars.2025.1564173
This article is part of the Research Topic Processes, Mechanisms and Solutions in Coastal Wetland to Adapt to Changing Environment View all 10 articles
The final, formatted version of the article will be published soon.
You have multiple emails registered with Frontiers:
Please enter your email address:
If you already have an account, please login
You don't have a Frontiers account ? You can register here
Unraveling the relationships between archaea and factors influencing their diversity and distribution is a critical issue in marine ecosystems. Here, the archaeal diversity and community structure in 0 - 20 cm soils from freshwater influenced wetlands (FIW), flooding freshwater and underground seawater influenced wetlands (MIW) and seawater influenced wetlands (SIW) in the Yellow River Nature Reserve were examined utilizing high-throughput sequencing of 16S rRNA gene sequencing. Based on the comparison of the alpha diversity indices, the abundance and diversity of the archaeal community in wetlands with varying hydrologic conditions did not significantly change (p > 0.05), with Thaumarchaeota and Marine_Group_I as the predominant archaeal phylum and class in all the three sampled sites, respectively. Thaumarchaeota, Woesearchaeota and Euryarchaeota constituted more than 90% of the total soil archaeal community in all wetlands. However, beta diversity indices revealed that significantly different distribution patterns of archaea were found among the three wetlands (p < 0.05). And the archaeal community structure in different wetlands varied as the hydrologic conditions changed. Less discriminated archaeal taxa were found in MIW (1 taxon) than in FIW (24 taxa) and SIW (18 taxa). Furthermore, statistical analysis confirmed that the difference in soil salinity caused by different hydrologic conditions was the major driver of archaeal community structure. Overall, this study highlights the role of hydrologic conditions in structuring the soil archaeal community in coastal wetlands.
Keywords: Archaea, Diversity and community structure, Soil salinity, Hydrologic conditions, coastal wetlands
Received: 21 Jan 2025; Accepted: 03 Mar 2025.
Copyright: © 2025 Zhao, Jia, Song, Li, Zhang and Huang. This is an open-access article distributed under the terms of the Creative Commons Attribution License (CC BY). The use, distribution or reproduction in other forums is permitted, provided the original author(s) or licensor are credited and that the original publication in this journal is cited, in accordance with accepted academic practice. No use, distribution or reproduction is permitted which does not comply with these terms.
* Correspondence:
Qingqing Zhao, Qilu University of Technology, Jinan, China
Jia Jia, Yellow River Institute of Hydraulic Research, Zhengzhou, Henan Province, China
Disclaimer: All claims expressed in this article are solely those of the authors and do not necessarily represent those of their affiliated organizations, or those of the publisher, the editors and the reviewers. Any product that may be evaluated in this article or claim that may be made by its manufacturer is not guaranteed or endorsed by the publisher.
Research integrity at Frontiers
Learn more about the work of our research integrity team to safeguard the quality of each article we publish.