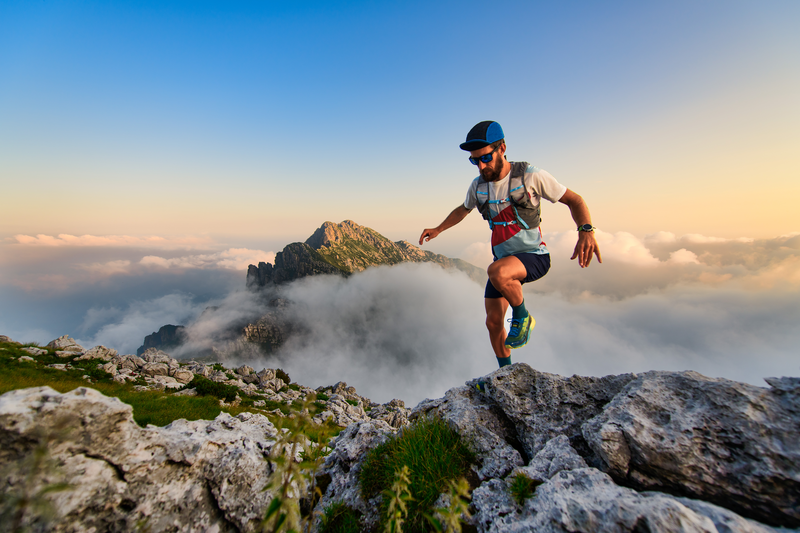
94% of researchers rate our articles as excellent or good
Learn more about the work of our research integrity team to safeguard the quality of each article we publish.
Find out more
ORIGINAL RESEARCH article
Front. Mar. Sci.
Sec. Marine Pollution
Volume 12 - 2025 | doi: 10.3389/fmars.2025.1556592
This article is part of the Research Topic Fate and Effects of Sediment and Emerging Pollutants in Marine and Estuarine Environments View all 4 articles
The final, formatted version of the article will be published soon.
You have multiple emails registered with Frontiers:
Please enter your email address:
If you already have an account, please login
You don't have a Frontiers account ? You can register here
Microplastic pollution has emerged as an undeniable marine environmental issue.While a distribution map of microplastics in the upper ocean has been established, the patterns of microplastics within the water column remain unclear. In this study, a largevolume in situ filtration device with filtration efficiency of 30 m 3 /h was employed to investigate microplastics in the deep waters of the South China Sea. The abundance of microplastics ranged from 0.2 to 1.5 items per cubic meter (n/m 3 ), with an average of 0.56 ± 0.40 n/m 3 . Microplastics are primarily fragments (72.58%) and fibers (20.97%), with the predominant polymer types being polypropylene (PP) and polyethylene terephthalate (PET). The average size of microplastics is 0.91 ± 0.97 mm, with no statistically significant differences observed across different water layers from 50 to 1000 meter (m). Non-metric Multidimensional Scaling (NMDS) analysis indicated that microplastics in the water column primarily originated from surface waters in the studied region. The occurrence of microplastics in the marine water column is a complex environmental process, influenced by a range of oceanographic mechanisms, including biological, chemical, and physical interactions. Our results provided reliable baseline data on microplastics in the water column of the South China Sea, contributing a better understanding to the vertical transport and fate of microplastics in this region.
Keywords: Microplastics, South China Sea, source, distribution, Vertical transport
Received: 07 Jan 2025; Accepted: 21 Feb 2025.
Copyright: © 2025 Li, Zhu, Wang and Li. This is an open-access article distributed under the terms of the Creative Commons Attribution License (CC BY). The use, distribution or reproduction in other forums is permitted, provided the original author(s) or licensor are credited and that the original publication in this journal is cited, in accordance with accepted academic practice. No use, distribution or reproduction is permitted which does not comply with these terms.
* Correspondence:
Lixin Zhu, East China Normal University, Shanghai, China
Disclaimer: All claims expressed in this article are solely those of the authors and do not necessarily represent those of their affiliated organizations, or those of the publisher, the editors and the reviewers. Any product that may be evaluated in this article or claim that may be made by its manufacturer is not guaranteed or endorsed by the publisher.
Research integrity at Frontiers
Learn more about the work of our research integrity team to safeguard the quality of each article we publish.