- 1School of Ocean, Yantai University, Yantai, China
- 2Shandong Engineering Research Center of Healthy Land-Sea Relay Farming of Economic Fish, Yantai, China
- 3Yantai Engineering Research Center of Deep-sea Aquaculture of Economic Fish, Yantai, China
- 4Rizhao Ocean and Fishery Research Institute, Rizhao, China
- 5National Fisheries Technology Extension Center, Beijing, China
- 6Guangxi Academy of Marine Sciences, Guangxi Academy of Sciences, Nanning, China
- 7East China Sea Fisheries Research Institute, Chinese Academy of Fishery Sciences, Shanghai, China
The greenfin horse-faced filefish, Thamnaconus septentrionalis, which is widely distributed in the Indo-West Pacific Ocean, is an important commercial fish species with characteristic blue-green fins, rough skin, and a spine-like first dorsal fin. Recently, T. septentrionalis has captured the attention of conservation and development due to its sharply declining population, and its great economic value as an important marine aquaculture fish species. To protect the resources of T. septentrionalis and develop its fishery industry, artificial breeding is essential and has been carried out gradually in local farms in China. A previous study has shown that temperature can effectively influence the gonadal development in T. septentrionalis in its overwintering state. However, the molecular mechanisms underlying the effects of temperature on the gonadal development in T. septentrionalis are still unrevealed and worth further study. In the present study, we systematically investigated the effects of temperature on ovary development in T. septentrionalis through transcriptomics and metabolomics analysis. The results showed that temperature rises significantly regulated the metabolic status of T. septentrionalis and promoted ovary development and maturation process through the regulation of the pathways of ECM-receptor interaction, regulation of actin cytoskeleton, oocyte meiosis, steroid hormone biosynthesis, lipid metabolism, ABC transporters, protein digestion and absorption, biosynthesis of amino acids pathways, etc. The histological, scanning electron microscope, and transmission electron microscope analyses showed that the oocytes in the ovaries of T. septentrionalis experiencing the temperature rises developed from phase II to III with increased gonadal somatic index and 17β-estradiol level. The present study for the first time elucidated the potential molecular regulatory mechanism of ovary development in T. septentrionalis induced by temperature and will provide valuable information for the artificial breeding of T. septentrionalis and conservation of T. septentrionalis fishery resources in the future.
1 Introduction
The greenfin horse-faced filefish Thamnaconus septentrionalis is a temperate water fish of Tetraodontiformes distributed widely in the Indo-West Pacific Ocean (Bian et al., 2020). Due to its appealing taste, rich nutritional benefits, and high economic value, T. septentrionalis has become an important commercially cultured marine fish species in Japan, Korea, and China (Bian et al., 2020). The natural resources of T. septentrionalis have rapidly decreased over the past decades, mainly as a result of serious overfishing and habitat loss. To develop the fishery industry of T. septentrionalis and meet market demands, artificial breeding is essential and has increased rapidly in recent years. With the rapid expansion of the T. septentrionalis aquaculture industry and market demands, the seedling production of the species was substantially enhanced.
The natural breeding period of T. septentrionalis is fairly late, in May–June along Shandong coastal waters, and parent fishes in the waters are affected by low temperature in winter and have a longer gonad development time (Mao et al., 2024). Therefore, the fry coming from parent fish cultivated under normal temperatures cannot be cultivated to commercial fish size in the same year. Reproductive regulation of parent T. septentrionalis through temperature rises could prolong the culture and growth period and likely achieve commercial fish sizes to meet the market demands. In teleosts, gonad development is influenced by many environmental factors (water temperature, photoperiod, food availability, etc.), and water temperature could significantly influence gonadal development and maturation (Urbatzka et al., 2011; Flores et al., 2020). During the artificial breeding period, the parent fish must undergo a three-stage process of temperature dropping (November), temperature rising for overwintering (December to January), and temperature rising for ripening (February) to facilitate successful spawning. In a previous study, we found temperature rises for overwintering could break ovary maintenance in stageII and start the development (Mao et al., 2024). However, the molecular mechanism underlying the induction of gonadal development in T. septentrionalis through temperature rises has been scarcely studied.
In addition, the aquaculture industry and sustainability have been increasingly impacted by climate change such as global warming (McKenzie et al., 2021; Yadav et al., 2024). The temperature rise induced by global warming affects the reproductive biology and population structure of commercially important tropical fish species (Brulé et al., 2022; Lema et al., 2024). The climate-induced temperature changes influenced the growth performance, fecundity, and population size of the marine fish Gadus morhua and Zoarces viviparous (Pörtner et al., 2001). Wanzenböck et al. (2022) found that ocean warming has an effect on population polymorphism and range shifts in stickleback by altering its fecundity and offspring development. Recent studies have also been conducted to explore the temperature-dependent effects on ovary development in teleosts. A high-temperature treatment intensified and promoted the emergence of sex-differentiated females in Leporinus friderici (Souza et al., 2024), induced Nile tilapia female sex reversal (Zhao et al., 2020), and increased the breeding probability in female coral reef damselfish Acanthochromis polyacanthus (Spinks et al., 2021). Besides the promoting effects on ovarian development (Souza et al., 2024; Zhao et al., 2020), a high-temperature treatment could also negatively affect the ovarian development in female pupfish by impairing ovarian steroidogenesis (Housh et al., 2024), and delayed ovarian development in the lesser sand eel with oocyte maturation over 2 months (Wright et al., 2017).
Considering the variability in responses of teleost reproductive systems to thermal challenges and the effects of climate change on aquaculture and fisheries, it is crucial to explore the effects of temperature rises on other fish species. Our preliminary study found that green horse-faced filefish is a thermo-sensitive species vulnerable to gonadal development disturbance due to rising temperature, and is suitable for scientific research on temperature-induced reproductive development in fish. Thus, we applied transcriptomics and metabolomics techniques to systematically elucidate the molecular mechanisms of ovary development in T. septentrionalis induced by temperature rises in the present study. Histological and ultrastructural analyses of T. septentrionalis ovaries were conducted in this study. The concentration of two key steroid hormones (17β-estradiol and testosterone) was quantified in T. septentrionalis plasma. The major findings in the present study will reveal the critical biological pathways and the genes involved in ovary development in T. septentrionali, which will provide theoretical scientific evidence for the artificial breeding of T. septentrionali, enrich the scientific knowledge of the molecular mechanism underlying teleost ovary development, and potentially provide theoretical scientific evidence for the conservation efforts in fish stocks or fisheries management under global warming.
2 Materials and methods
2.1 Experiment animals, design and sampling
Three-year-old female parent greenfin horse-faced filefish T. septentrionalis without a reproductive history were collected from a local aquatic hatchery located in Yantai, China. Our preliminary investigation found that the ovaries of female fish will be maintained at stageII in the process of T. septentrionalis breeding without appropriate temperature stimulation (Supplementary Figure 1), which provided an important basis and evidence for conceiving the experiment design and determining the sampling time points in this study. Furthermore, the experiment was carried out under the same culture condition as recorded in the preliminary investigation to exclude the potential influence of the other factors (e.g. diet, temperature, salinity, and photoperiod) on ovarian development of the T. septentrionalis used in this study. During the overwintering period controlled by water temperature rises, T. septentrionalis ovaries (n = 6 for each group) were sampled before the water rising (the LO group), while those sampled after the water rising were designated as the HO group. Detailed information on the experimental design and sampling in this study are presented in Supplementary Figure 2. Prior to sampling, fish were not fed for 24 hours (h) and anesthetized with 0.05% MS-222. The body’s full length, body weight, and gonad weight were measured and listed in Table 1. The gonadal somatic index (GSI) of each fish was measured as described in Flores et al. (2015). Using heparinized syringes, the blood samples were collected from the caudal vein of 12 fish (n = 6 for the LO group, n=6 for the HO group) and centrifuged for 15 min at 800 g to separate plasma for hormone extraction and quantification of 17β-estradiol (E2) and testosterone (T) by enzyme-linked immunosorbent assay (ELISA) following the method as described in Sánchez-Hernández et al. (2013). Then, the tissues of the ovaries were collected for further molecular and cellular analysis. For each ovary sample, approximately 1 cm3 of ovary tissue was preserved in Bouin’s solution for histopathological analysis and 1 cm3 of ovary tissue was preserved in 2.5% glutaraldehyde solution for the scanning electron microscope (SEM) and transmission electron microscope (TEM) analyses. Further ovary tissues of T. septentrionalis (n = 3/group for transcriptomic analysis; n = 6/group for metabolomics analysis) were collected and preserved at -80°C after quick freezing in liquid nitrogen until being further processed.
2.2 Histological analysis of T. septentrionalis ovaries
The ovary tissues of T. septentrionalis were dissected and further sampled for histological examination. Briefly, the ovary tissues were excised and fixed in Bouin’s solution for less than 24 h, and the fixative solution was replaced several times with 70% ethanol in which samples were eventually stored. All tissue samples were then processed using paraffin histological techniques and stained with hematoxylin and eosin (H and E) as described in previous studies (Johnson et al., 2009; Sharma et al., 2023). The prepared slides were carefully examined and documented with an Olympus BX53 microscope equipped with an Olympus DP73 digital camera (200×, 400×).
2.3 SEM and TEM analysis of T. septentrionalis ovary
The gonads were removed and individually immersed in sodium cacodylate buffer (0.1 M, pH 7.4) supplemented with 2.5% glutaraldehyde. After 24 h at 4°C, tissue samples were washed three times for 5 min with the sodium cacodylate buffer and post-fixed in the same buffer containing 1% osmium tetroxide (OsO4) for 1.5 h. For the SEM analysis, the tissue samples were dehydrated in a graded ethanol series (30%–100%), critical point dried using CO2, mounted on metallic stubs using carbon stickers, sputter-coated with gold, and examined using a HITACHI SU8100 SEM. For the TEM analysis, the tissue samples were dehydrated in an ascending acetone series and embedded in Epon 812. Ultra-thin sections were cut in a microtome and contrasted with uranyl acetate and lead citrate, followed by examination using a HITACHI HT7800 electron microscope with a HITACHI TEM system.
2.4 RNA extraction, library construction, transcriptome sequencing, and analysis
RNA extraction, library preparation, sequencing, and bioinformatics analyses were performed at Beijing Novogene Technology Co., LTD (Beijing, China). The sequencing library was prepared using the NEBNext mRNA Sample Prep Reagent Set for Illumina (NEB, USA). Qubit 2.0 (Life Technologies, Carlsbad, CA, USA) was used for preliminary library quantification, and qRT-PCR was conducted for accurate quantification of the effective concentration of the library (>2 nM) to ensure the quality of the library. The libraries were subjected to paired-end HiSeq sequencing and generated 6G of data for each sample. Novaseq 6000 was used with the PE150 sequencing strategy. Trimmomatic software (v. 0.39) was used to remove reads with sequencing adapters (Bolger et al., 2014), reads with N (uncertain base) content >10%, and reads with low-quality base (Q ≤ 20) content > 50%. FASTP (version 0.19.7) served as the low-quality reads removal software with the parameter FASTP-Q 20-U 50-N 15-L 150. The clean reads were aligned to a reference genome using HISAT2 software (Pertea et al., 2016), based on the bowtie2 method (Langmead, 2010), and genes were annotated.
The annotated genes were counted using RNASep by Expectation Maximization v1.3.0 (Li and Dewey, 2011), and FPKM values were determined to obtain transcript levels. Differentially expressed genes (DEGs) among the samples were identified using the DEseq2 R package and based on FDR < 0.05 and | log2 (fold change) | > 1 (Love et al., 2014). The DEGs were clustered in heatmaps and subjected to Gene Ontology (GO) enrichment for functional analysis (http://www.geneontology.org/) based on molecular function, biological process, and cellular component terms using GoSeq (v. 1.22) (Young et al., 2010). For KEGG pathway enrichment analysis (Kanehisa et al., 2004), a hypergeometric test was applied to identify the pathways significantly enriched in DEGs based on FDR < 0.05. R software and a self-written script were used for pathway enrichment analysis using Benjamini–Hochberg-corrected FDR values.
2.5 Untargeted metabolomics analysis of T. septentrionalis ovaries
2.5.1 Sample collection and preparation
The fish ovary tissues were quickly frozen in liquid nitrogen immediately after dissection. Then, the tissue was cut on dry ice (~ 80 mg) and placed in an Eppendorf tube (2 mL). The tissue samples, with 200 μL of H2O and five ceramic beads, were homogenized using the homogenizer, and 800 μL methanol/acetonitrile (1:1, v/v) was added to the homogenized solution for metabolite extraction. The mixture was centrifuged for 20 min (14,000 g, 4°C), and the supernatant was dried in a vacuum centrifuge. For LC-MS analysis, the samples were re-dissolved in 100 μL acetonitrile/water (1:1, v/v) solvent and centrifuged at 14,000 g at 4°C for 15 min, and then the supernatant was injected.
2.5.2 LC-MS/MS analysis
Analysis was performed using a UHPLC (1290 Infinity LC, Agilent Technologies) coupled to a quadrupole time-of-flight (AB Sciex TripleTOF 6600) at Shanghai Applied Protein Technology Co., Ltd. For HILIC separation, samples were analyzed using a 2.1 mm × 100 mm ACQUIY UPLC BEH Amide 1.7 µm column (Waters, Ireland). In both ESI positive and negative modes, the mobile phase contained A = 25 mM ammonium acetate and 25 mM ammonium hydroxide in water and B = acetonitrile. The gradient was 95% B for 0.5 min and was linearly reduced to 65% for 6.5 min, and then was reduced to 40% for 1 min, and then increased to 95% for 0.1 min, with a 3 min re-equilibration period employed. The ESI source conditions were set as follows: Ion Source Gas1 (Gas1): 60, Ion Source Gas2 (Gas2): 60, curtain gas (CUR): 30, source temperature: 600°C, IonSpray Voltage Floating (ISVF) ± 5500 V. In the MS-only acquisition, the instrument was set to acquire over the m/z range of 60–1000 Da, and the accumulation time for the TOF MS scan was set at 0.20 s/spectra. In auto MS/MS acquisition, the instrument was set to acquire over the m/z range 25-1000 Da, and the accumulation time for the product ion scan was set at 0.05 s/spectra. The product ion scan was acquired using information-dependent acquisition (IDA) with high sensitivity mode selected. The parameters were set as follows: the collision energy (CE) was fixed at 35 V with ± 15 eV; declustering potential (DP), 60 V (+) and −60 V (−); exclude isotopes within 4 Da, candidate ions to monitor per cycle: 10.
2.5.3 Data processing and statistical analysis
The raw MS data were converted to MzXML files using ProteoWizard MSConvert before being imported into freely available XCMS software. For peak picking, the following parameters were used: centWave m/z = 10 ppm, peakwidth = c (10, 60), and prefilter = c (10, 100). For peak grouping, bw = 5, mzwid = 0.025, and minfrac = 0.5 were used. CAMERA (Collection of Algorithms of MEtabolite pRofile Annotation) was used for annotation of isotopes and adducts. In the extracted ion features, only the variables with more than 50% of the non-zero measurement values in at least one group were kept. Compound identification of metabolites was performed by comparing the accuracy m/z value (< 10 ppm) and MS/MS spectra with an in-house database established with available authentication standards.
After sum-normalization, the processed data were analyzed in an R package, where it was subjected to multivariate data analysis, including Pareto-scaled principal component analysis (PCA) and orthogonal partial least-squares discriminant analysis (OPLS-DA). Seven-fold cross-validation and response permutation testing were used to evaluate the robustness of the model. The variable importance in the projection (VIP) value of each variable in the OPLS-DA model was calculated to indicate its contribution to the classification. Student’s t-test was applied to determine the significance of differences between two groups of independent samples. The metabolites were considered to be significantly different between the LO and HO groups when VIP > 1 and P-value < 0.05. Pearson’s correlation analysis was performed to determine the correlation between two variables.
2.6 Validation of representative DEGs with qRT-PCR assays
To verify the reliability of the sequencing data, the transcriptional profiles of 12 DEGs involved in critical fish gonadal development processes with significant alterations were further analyzed by qRT-PCR assays. Total RNAs were isolated from the same subset of fish ovary samples using Trizol reagent (TaKaRa, Japan). The extracted RNAs were treated with RNase-free DNase I (TaKaRa, Japan) to remove contaminating genomic DNAs. The transcripts of the DEGs were analyzed and validated by qRT-PCR assays using the PrimeScript™ RT reagent Kit (TaKaRa, Japan) as described in Li et al. (2019). The qRT-PCR assays were carried out in triplicate. The transcripts of the target genes were normalized by the geometric mean of three endogenous reference genes, including β-actin, glyceraldehyde-3-phosphate dehydrogenase (gapdh), and elongation factor 1-alpha (ef1a), according to the MIQE guidelines (Bustin et al., 2009). The qRT-PCR primers used in this study were designed in the online software qPrimerDB 2.0 (Li et al., 2025) and are listed in Supplementary Table 1. The gene expression levels were calculated by the 2-ΔΔCt method as described in Livak and Schmittgen (2001). Data were shown as the mean ± standard deviation (SD) and analyzed via the one-way analysis of variance (ANOVA), followed by Duncan’s multiple range test using the SPSS statistics software (version 20.0, SPSS, USA). Differences were considered significant when P < 0.05.
3 Results
3.1 The physiological index of the sampled female T. septentrionalis
No significant difference was found in body length, full length, body weight, and gonad weight between the LO and HO groups, while the GSI was significantly increased in the HO group compared to the LO group (Table 1). Compared to the LO group, the concentrations of E2 (1.86-fold, P < 0.05) and T (1.54-fold, P < 0.05) were significantly increased in the plasma of T. septentrionali in the HO group (Figure 1).
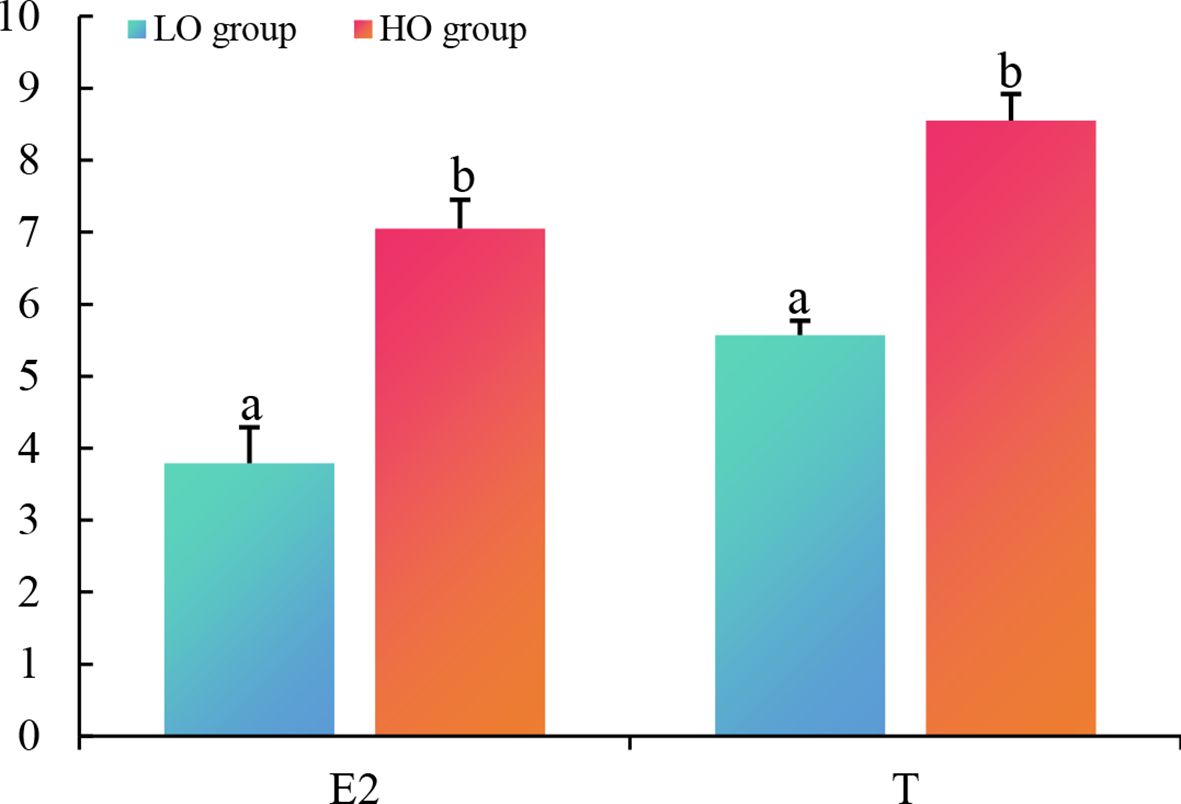
Figure 1. The concentrations of plasma 17β-estradiol (E2) and testosterone (T) in the LO and HO groups. Statistics significance (P < 0.05) was indicated by the different letters on bars. LO, T. septentrionalis ovaries treated with low temperature; HO, T. septentrionalis ovaries treated with high temperature.
3.2 Histological, SEM, and TEM analyses of T. septentrionalis ovary
In the previous histological staining analysis, the oocyte development stages of T. septentrionalis were described in detail and classified into six stages (Zhang, 2011). In the present study, the histological observation showed that the oocytes in the LO ovaries were predominantly in phaseII with a minority of oocytes in phase I, indicating the LO ovaries were in stage II. Then, after the temperature rise, the oocytes in the HO ovaries started to develop with increased cell volume and the partial oocytes transitioned to phase III;, indicating the development of the HO ovaries to stage III (Figure 2). The SEM analysis showed that the overall structure of the sampled ovaries was tree-branch-like and nearly spherical oocytes could be clearly observed (Figures 3A, B). Similar to the histological results, the oocytes in the LO ovaries were mainly in phaseII, while those in HO ovaries developed in a stepwise fashion to phase III. In addition, the ultrastructure of the nucleolus, nuclear membrane, and radiation zone could be clearly observed in the sampled ovaries in the TEM analysis (Figures 3C, D). Compared to the oocytes in the LO ovaries, the nuclei in the HO ovaries were enlarged with more condensed nucleoli, and big vacuoles appeared in the HO ovaries.
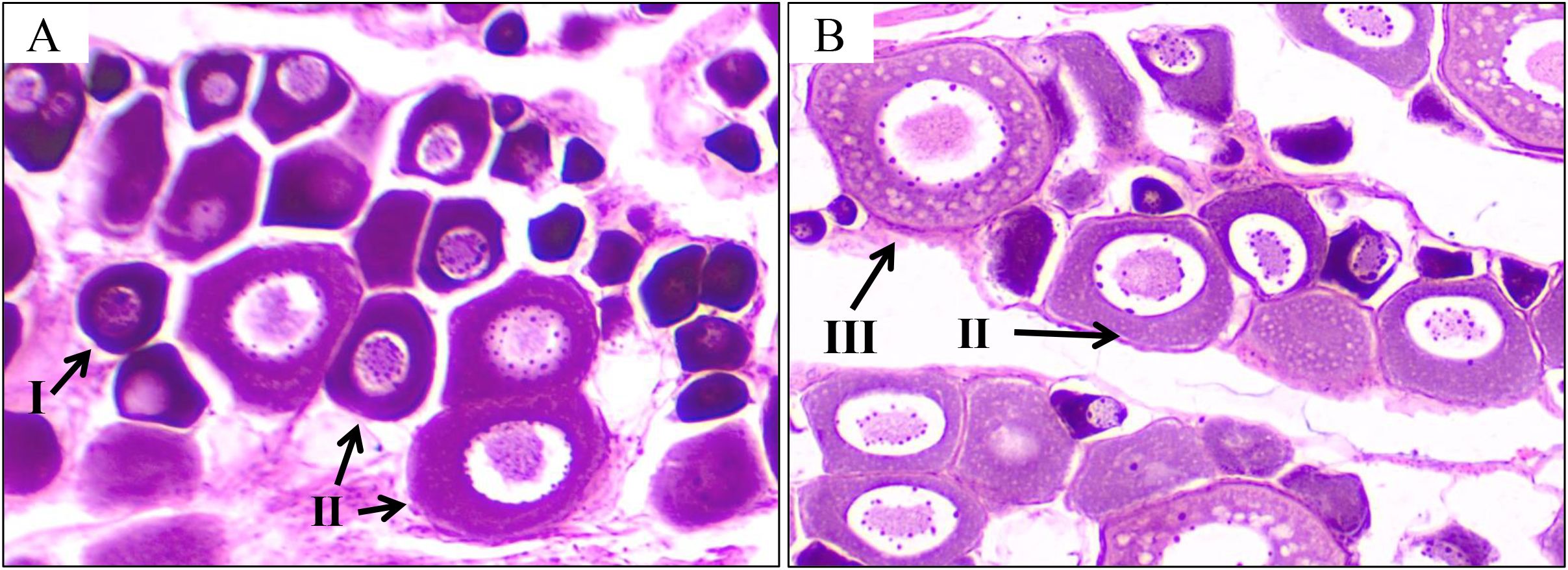
Figure 2. Histological observation of T. septentrionalis ovaries in the LO (A) and HO (B) groups. I, oocyte in phase I; II, oocyte in phase II; III, oocyte in phase III; scale bar: 100 μm.
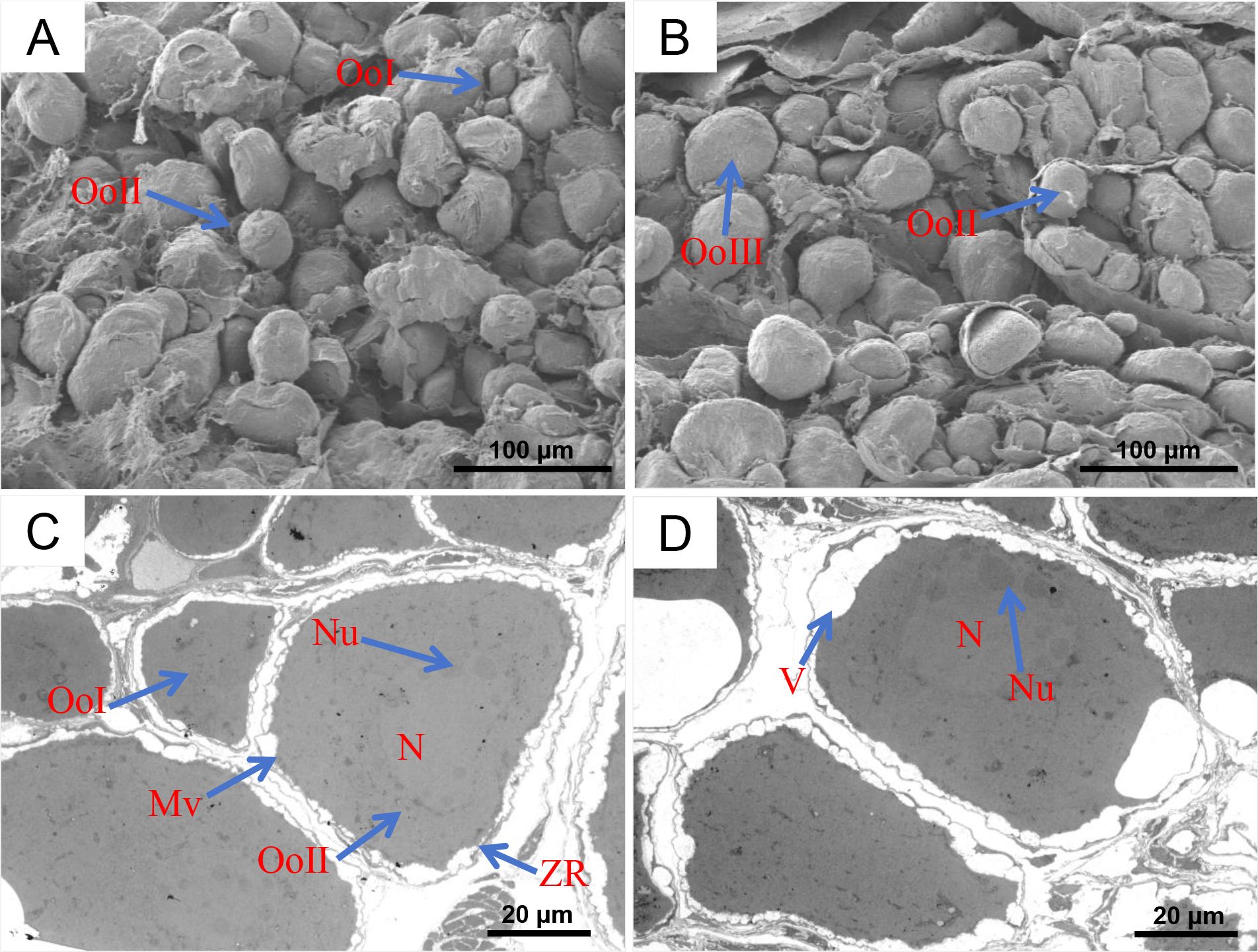
Figure 3. SEM and TEM analysis of T. septentrionalis ovaries in the LO and HO groups. SEM photograph of T. septentrionalis ovaries in the LO (A) and HO (B) groups. TEM analysis of the T. septentrionalis ovaries in the LO (C) and HO (D) groups. OoI, oocyte in phase I; OoII, oocyte in phase II; OoIII, oocyte in phase III; Nu, nucleolus; CA, cortical alveoli; V, vacuole; Oo, oocyte; Mv, microvilli; ZR, zone radiate. Scale bar: 2 μm. SEM, scanning electron microscope; TEM, transmission electron microscope.
3.3 Transcriptome responses of T. septentrionalis ovary to rising temperature
3.3.1 Quality assessment of sequencing data
A total of 271,432,936 raw sequencing reads were obtained by the transcriptomics analysis of ovary tissues from the LO and HO groups. After removing low-quality and junction sequence reads, a total of 262,230,538 clean reads were obtained. The results of transcriptomics quality analysis showed that the average GC content in the LO and HO groups was 53.15% and 53.18%, respectively; the average Q30 values were 91.52% and 91.36%, respectively; and the average Q20 values were 96.66% and 96.56%, respectively. The clean reads were mapped to the T. septentrionalis genome; the average mapping rates of the LO and HO groups were 81.16% and 81.03%, respectively, indicating that the quality of transcriptome sequencing was good and reliable (Supplementary Table 2).
3.3.2 Identification and enrichment analysis of DEGs
In the comparative analysis of the transcriptomic sequencing of the LO and HO groups under the screening conditions of FDR < 0.05 and | log2 FC | ≥ 1, a total of 146 DEGs were identified, including 97 upregulated and 49 downregulated genes (Figure 4A). In order to deeply understand the biological processes in which the DEGs were involved, GO enrichment analysis was conducted. The results showed that the DEGs were mainly divided into three functional categories: biological process, molecular function, and biological adhesion. The biological processes mainly include proteolysis, cell adhesion, and DNA metabolic processes. The cellular components mainly comprise the extracellular matrix, parts of the extracellular region, and the extracellular region. The molecular functions are mostly related to the metallopeptidase activity, metallocarboxypeptidase activity, and structural constituents of the extracellular matrix (Figure 4B). KEGG pathway enrichment analysis suggested that a total of 146 DEGs were divided into six categories, which included metabolism, organic system, human diseases, environmental information processing, cellular processes, and genetic information processing, of which six KEGG pathways, including ECM-receptor interaction, focal adhesion, vascular smooth muscle contraction, regulation of actin cytoskeleton, oocyte meiosis, and steroid hormone biosynthesis, were significantly enriched (Figure 5).
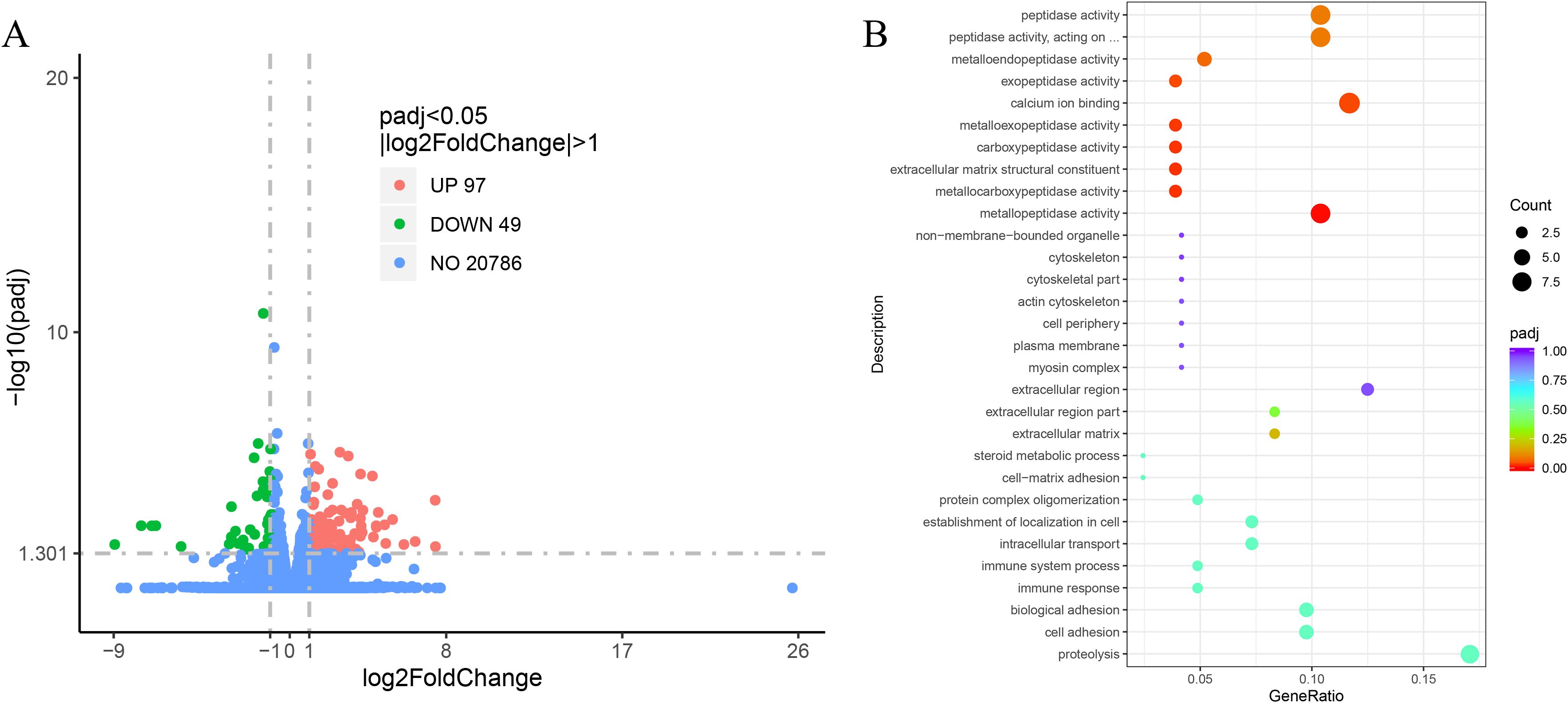
Figure 4. (A) The volcano map of the DEGs between the LO and HO groups. Red dots: significantly upregulated DEGs, green dots: significantly downregulated DEGs. (B) GO enrichment analysis of the DEGs between the LO and HO groups. DEGs, differentially expressed genes; GO, Gene Ontology.
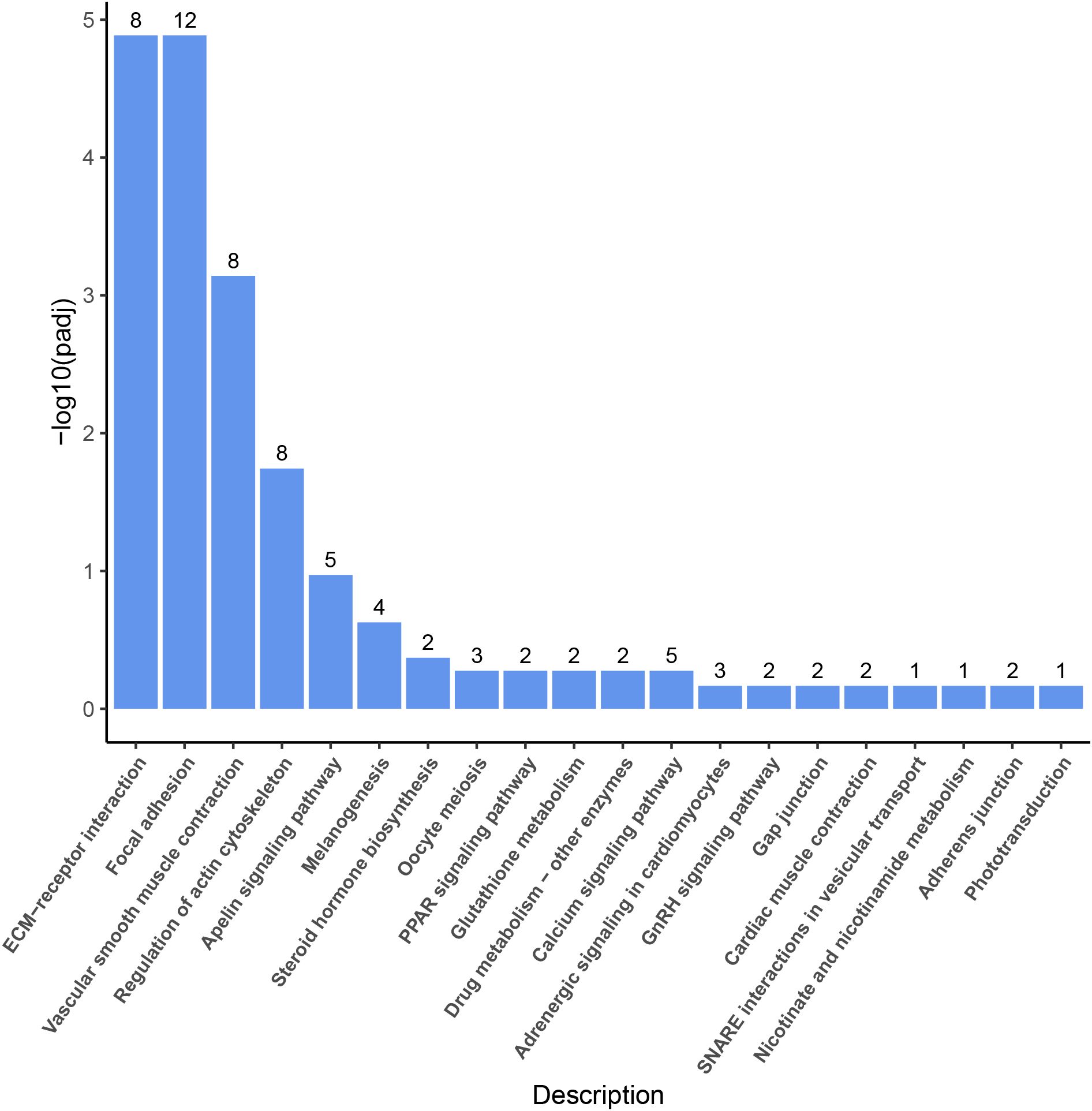
Figure 5. KEGG pathway enrichment analysis of the DEGs between the LO and HO groups. KEGG, Kyoto Encyclopedia of Genes and Genomes; DEGs, differentially expressed genes. Each column indicates for one pathway with the number of DEGs in it.
3.4 qRT-PCR assays of critical DEGs in T. septentrionalis ovaries responding to rising temperature
In order to verify the reliability and accuracy of the RNA-Seq results, 12 DEGs in T. septentrionalis ovaries enriched in the ECM-receptor interaction (collagen α1, femp2, and integrin α1), focal adhesion (collagen α2 and vinculin), regulation of actin cytoskeleton (protein phosphatase 1 and myosin), steroid hormone biosynthesis (hsd3b and hsd17b1), oocyte meiosis (adenylate cyclase 8, cyclin-E2, and calmodulin-1) pathways were subjected to qRT-PCR analysis. The transcripts of collagen α1 (2.16-fold), FEMP2 (3.58-fold), integrin α1 (2.78-fold), collagen α2 (5.62-fold), vinculin (13.68-fold), protein phosphatase 1 (15.21-fold), myosin (9.16-fold), hsd3b (6.21-fold), hsd17b1 (3.62-fold), and calmodulin-1 (2.69-fold) were significantly increased in T. septentrionalis ovaries responding to rising temperature (Supplementary Figure 3). The transcripts of adenylate cyclase 8 (0.15-fold) and cyclin-E2 (0.28-fold) were significantly decreased in T. septentrionalis ovaries after temperature rises (Supplementary Figure 3). The qRT-PCR results showed that the DEGs exhibited a similar expression trend as that of RNA-seq results (Supplementary Figure 3), reflecting that the transcriptomic results were accurate and reliable in the present study.
3.5 Metabolomic responses of T. septentrionalis ovaries to rising temperature
3.5.1 Identification and multivariate statistical analysis of metabolites
The effects of rising temperature on ovary metabolism in T. septentrionalis were studied using non-targeted metabolomics analysis using the LC-MS platform. The ionization source of LC-MS was ESI, including two ion modes: positive ion mode (POS) and negative ion mode (NEG). The peak retention time (RT) and peak area of the total ion chromatogram of all the quality control (QC) samples overlapped well, indicating that the analysis system was stable and reliable (Supplementary Figure 4). In the positive and the negative ion modes, a total of 558 and 543 effective peaks were identified, respectively. The effective peaks were compared to the databases of the metabolomes (MS1 and MS2), which identified 558 and 543 metabolites in the positive and negative ion modes, respectively. The 1,101 metabolites were classified into 15 superclasses including lipids and lipid-like molecules; organic acids and derivatives; undefined, organoheterocyclic compounds; organic oxygen compounds; benzenoids; nucleosides, nucleotides, and analogs; organic nitrogen compounds; phenylpropanoids and polyketides; alkaloids and derivatives; homogeneous non-metal compounds; lignans, neolignans, and related compounds; organoheterocyclic compounds; organometallic compounds; and organosulfur compounds (Supplementary Figure 5).
The PCA results showed that the LO and HO groups were significantly differentiated in the negative and positive ion modes (Figure 6), respectively. The cross-validation of the OPLS-DA model showed that R2X, R2Y, and Q2 were 0.544, 0.994, and 0.77 in the positive ion mode respectively, and those in the negative ion mode were 0.468, 0.979, and 0.798, respectively. Q2 was greater than 0.5, indicating the model had good prediction ability. Then, the OPLS-DA model was established with a permutation test, and the order of the categorical variable Y was randomly changed to obtain the R2 and Q2 of the random model. All the Q2 points were lower than the original black Q2 points from left to right, which indicated the model’s reliability with no overfitting and further ensured that the metabolomics data and instrument analysis system was reliable and stable in the present study.
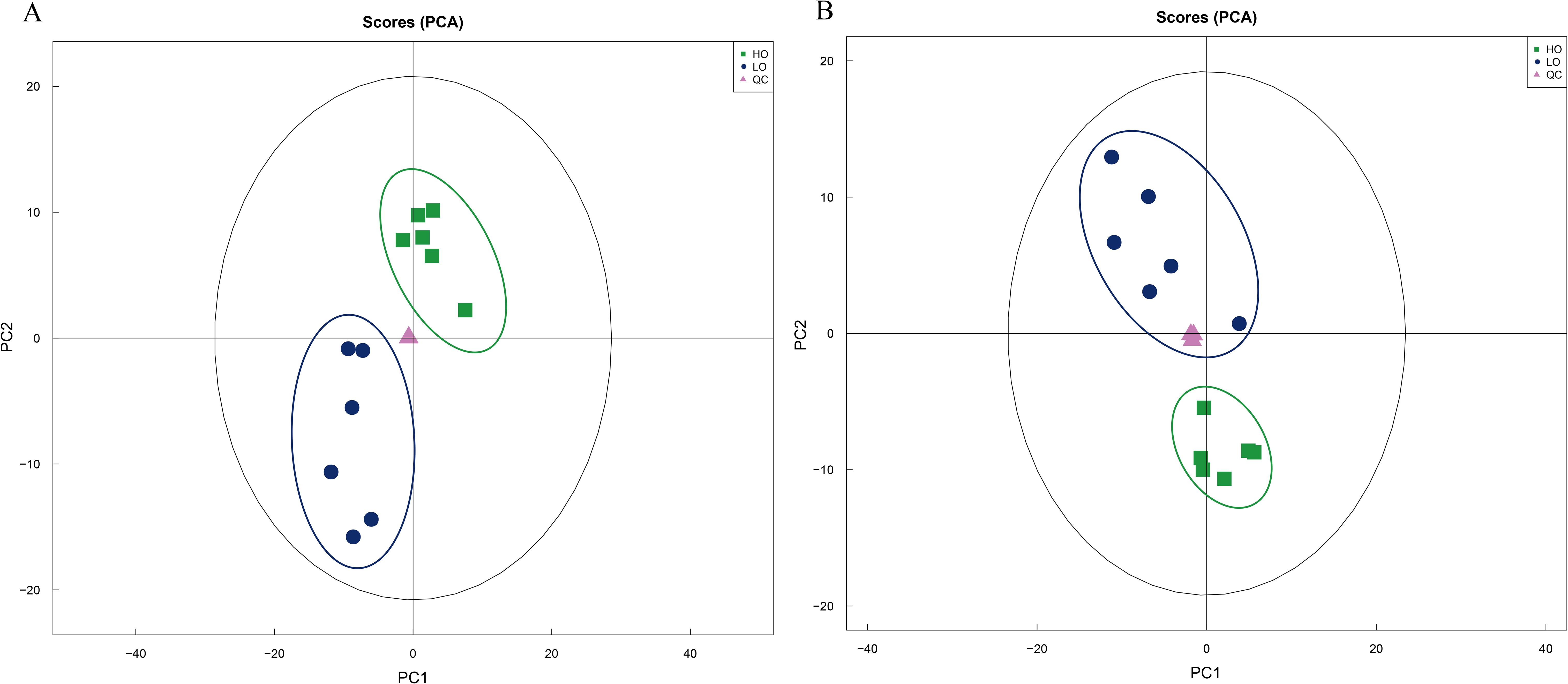
Figure 6. Principal component analysis (PCA) of the metabolomics data from T. septentrionalis ovaries. (A, B) are the negative and positive ion modes, respectively. The green squares in the green circle are the cluster of the ovary samples treated with high temperature. The blue dots in the blue circle are the cluster of ovary samples treated with low temperature. The pink triangles are the quality control samples.
3.5.2 Analysis of SDMs and their metabolic pathways
According to the screening conditions (VIP > 1 and P < 0.05), the significantly different metabolites (SDMs) between the two groups were obtained, and their fold changes (FCs) were calculated. The results showed that 65 SDMs were identified in the HO vs. LO groups, among which 54 were upregulated, and 11 were downregulated under the negative ion mode (Figure 7A). There was a total of 69 SDMs in the HO vs. LO groups under the positive ion mode, among which 53 were upregulated, and 16 were downregulated (Figure 7B). Based on the screening conditions of VIP > 1 and P < 0.05, a total of 25 KEGG pathways were significantly enriched by the SDMs between the LO and HO groups, and the top 10 significantly enriched pathways were protein digestion and absorption, pyrimidine metabolism, ABC transporters, mineral absorption, aminoacyl-tRNA biosynthesis, biosynthesis of amino acids, glycerophospholipid metabolism, cysteine and methionine metabolism, glucosinolate biosynthesis, and metabolic pathways (Figure 7C). In addition, three significantly enriched pathways, glycerophospholipid metabolism, ether lipid metabolism, and sphingolipid metabolism, belonged to the Pathway Hierarchy 2 “Lipid metabolism” category.
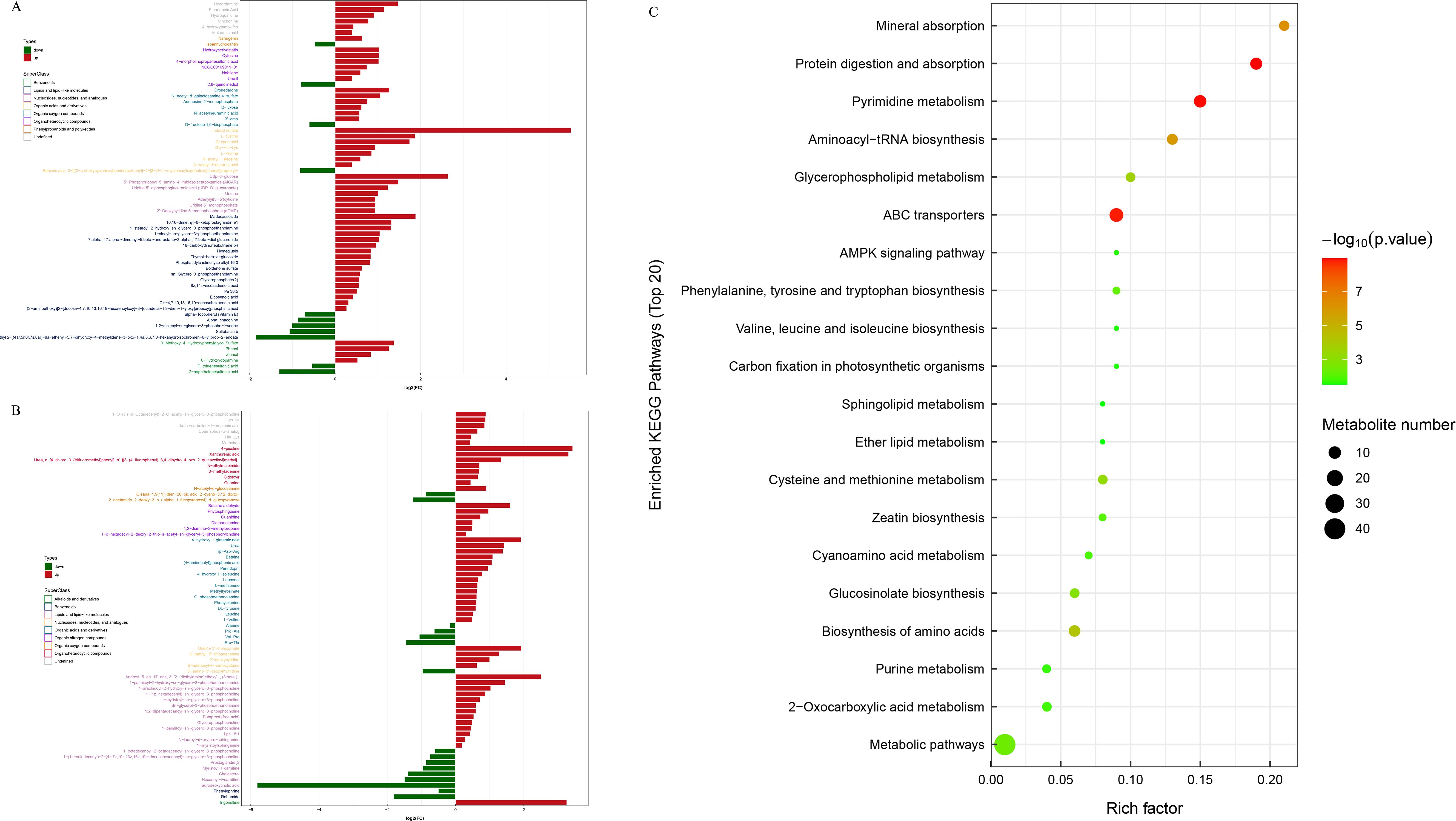
Figure 7. The types and superclasses of the SDMs between the LO and HO groups. Red bars: significantly upregulated SDMs, green bars: significantly downregulated SDMs. (A, B) are the negative and positive ion modes, respectively. The red and green columns represent the significantly up- and downregulated metabolites (| Fold change> 1.2 and P –value < 0.05), respectively. KEGG pathway enrichment analysis of SDMs between the LO and HO groups (C). The size of the dots represents the number of metabolites involved in each pathway, with larger dots indicating a higher number. The color gradient from green to red signifies the P-value, with red indicating a more significant enrichment. SDMs, significantly differential metabolites.
4 Discussion
The greenfin horse-faced filefish T. septentrionalis is a valuable commercial fish species widely distributed in the Indo-West Pacific Ocean and has become an important marine aquaculture fish species in China. In recent years, there has been a sharp decline in the population resources and overfishing of T. septentrionalis. Studies have focused on the reproductive development and artificial propagation of T. septentrionalis under industrial aquaculture conditions. However, the molecular regulatory mechanism underlying the reproductive development in T. septentrionalis has been scarcely studied until now. In the present study, we systematically studied the molecular regulatory mechanism of temperature-induced ovary development in T. septentrionalis under industrial aquaculture conditions. The key genes, metabolites, and the biological pathways contributing to the temperature-induced development of T. septentrionalis were revealed. In addition, the present study found that rising temperature promotes ovarian maturation in female T. septentrionalis primarily by enhancing the synthesis of E2 and metabolic regulation during the overwintering period.
The transcriptomics results showed that the pathways of “ECM-receptor interaction”, “Focal adhesion”, and “Regulation of actin cytoskeleton” were overtly modulated in T. septentrionalis ovaries after temperature rises. ECM is an important structure essential for normal tissue homeostasis (Bonnans et al., 2014) and participates in regulating cellular adhesion, proliferation, and differentiation (Liu et al., 2015). The ECM-receptor interaction pathway plays a crucial regulatory role in ovarian development and oocyte proliferation, differentiation, and maturation (Irving-Rodgers and Rodgers, 2005; Cassel, 2019; Curry and Osteen, 2003; Dong et al., 2021). ECM must be involved in ovarian development as ECM provides structural support for follicles and regulates follicle proliferation, survival, and differentiation, and steroid formation to promote follicle development and maturation (Cha et al., 1991). Wang et al. (2024) showed that the genes (collagen and Integrin) involved in the ECM-receptor interaction pathway were significantly upregulated in Procambarus clarkii ovaries, indicating their important regulatory function in promoting ovarian development and oocyte maturation. Yue et al. (2004) demonstrated that integrins could initiate the reorganization of cytoskeletal protein in mouse oocytes and were likely involved in transmembrane signal transduction and egg activation during fertilization. Pallotta et al. (2022) suggested that the FRAS1-related extracellular matrix protein (FEMP), an important ECM protein, is increased in female embryonic gonads of the leopard gecko Eublepharis macularius and participate in the gonad differentiation and embryonic development in E. macularius. Gautier et al. (2008) suggested that FREM proteins participate in the development of various tissues (e.g. pharyngeal arches, hypochord, musculature, fin) in zebrafish Danio rerio. In the present study, the transcripts of three genes (collagen α1, Integrin α1, and femp2) involved in the ECM-receptor interaction pathway were significantly increased in T. septentrionalis ovaries after temperature rises, which suggested the critical function of the ECM-receptor interaction pathway in the ovarian development in T. septentrionalis in response to rising temperature.
In addition, focal adhesion and the regulation of actin cytoskeleton pathways were also significantly influenced in T. septentrionalis ovary after temperature rises. Focal adhesion regulates cell morphology, migration, and proliferation during ovarian development (Wang et al., 2024). The actin cytoskeleton plays an important role in regulating meiotic maturation and fertilization (Santella et al., 2014). In the present study, four genes (Protein phosphatase 1, Myosin, Vinculin, and collagen α2) involved in these two pathways were significantly upregulated in T. septentrionalis ovaries after temperature rises. Mishra and Joy (2006) suggested that protein phosphatase (PP) may be involved in the PKC (protein kinase C) modulation of steroidogenesis, oocyte maturation, and ovulation in animals. Vinculin is a cytoskeletal protein that localizes to cell-ECM and cell-cell adhesion sites, with crucial roles in the maintenance and regulation of cell adhesion and migration (Paz et al., 2007), and is involved in the PKC modulation of the cytoplasmic compartment during the development of oocytes (Johnson and Capco, 1997). Myosins were found to play a role in regulating the migration of zebrafish primordial germ cells (Blaser et al., 2006). Combining the results of this study and previous findings, the present study suggests that focal adhesion and regulation of actin cytoskeleton pathways were significantly involved in the temperature-induced ovarian development in T. septentrionalis.
Furthermore, the steroid hormone biosynthesis pathway was significantly influenced in T. septentrionalis after temperature rises. Two key steroidogenesis-related genes (hsd3b and hsd17b1) were significantly increased in T. septentrionalis ovaries after temperature rises in this study. Hasegawa et al. (2022) found rapid upregulation of hsd3b expression during oocyte maturation in Amur sturgeons Acipenser schrenckii. Recent studies also established that hsd17b1 participates in regulating gonad differentiation and ovarian development by regulating the synthesis of sex steroid hormones (e.g. E2 and testosterone) in teleosts including Japanese sardine Sardinops melanostictus (Nyuji et al., 2024), olive flounder Paralichthys olivaceus (Zou et al., 2020), and Siberian sturgeon Acipenser baerii (Lasalle et al., 2024). Cai et al. (2023) also reported enhanced transcripts of hsd3b and hsd17b1, suggesting an important role in ovarian development in common carp Cyprinus carpio by catalyzing the synthesis of E2. E2 stimulates hepatic cells to synthesize and secrete vitellogenin to promote oocyte development in female fish (Hiramatsu et al., 2015; Hara et al., 2016; Maldonado-García et al., 2018). It is also known that E2 increases from the first stage of ovarian development and peaks during puberty in coho salmon Oncorhynchus kisutch and yellow tail flounder Limanda ferruginea (Campbell et al., 2006; Manning et al., 2008). In the present study, the transcripts of hsd3b and hsd17b1 were significantly increased in T. septentrionalis ovaries after temperature rises, which contributed to enhancing the synthesis of E2. Then, the significantly increased E2 level promoted the development of T. septentrionalis ovaries with oocytes developed from stage II to stage III as indicated by the histological analysis and the significantly increased GSI. The combined results suggested that rising temperature could regulate sex-related steroid hormone synthesis such as E2 by influencing the steroid hormone biosynthesis pathway to promote the development and maturation of T. septentrionalis ovaries (Figure 8). Moreover, the findings of the stimulation effects of rising temperature on T. septentrionalis ovary development were consistent with recent studies, which showed that a temperature rise promoted ovarian development in Leporinus friderici (Souza et al., 2024), Oreochromis niloticus (Zhao et al., 2020), and Acanthochromis polyacanthus (Spinks et al., 2020).
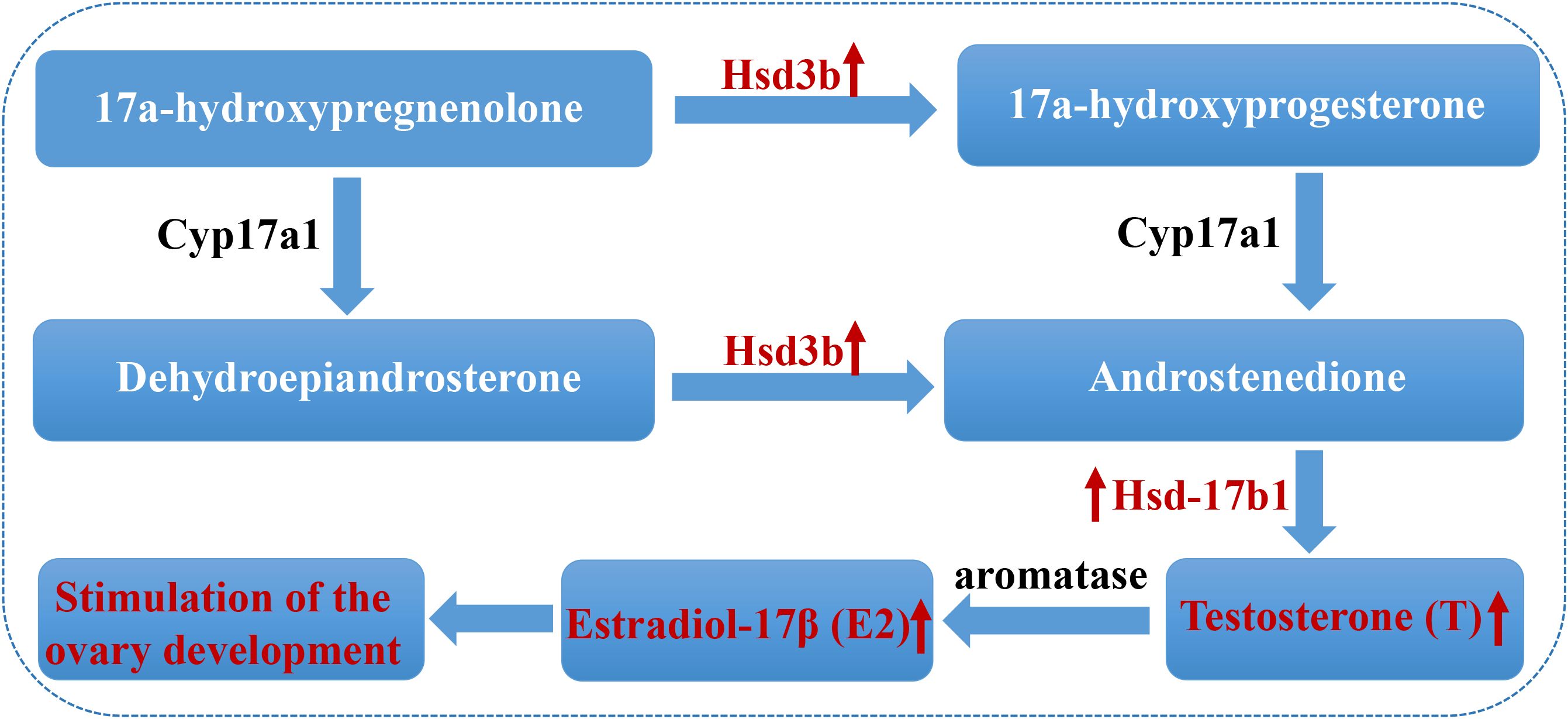
Figure 8. The potential mechanism of promoting ovarian development in Thamnaconus septentrionalis by increasing the E2 level via regulation of the key steroidogenic genes. The two significantly upregulated genes (Hsd3b and Hsd-17b1) and the increased levels of hormones (E2, T) were labeled in red and indicated by red arrows. Hsd3b, 3β-hydroxysteroid dehydrogenase; hsd17b1, 17β-hydroxysteroid dehydrogenase 1; E2, Estradiol-17β; T, Testosterone.
In addition, the “Oocyte meiosis” pathway was significantly altered with three genes (Adenylate cyclase type 8, Cyclin-E2, and Calmodulin-1) differentially expressed in T. septentrionalis ovary after temperature rises in this study. Distinct expression patterns of Adenylate cyclases (ACs) and Cyclins were observed during oocyte growth and maturation of ricefield eels Monopterus albus (Meng et al., 2022), reflecting the roles of ACs and Cyclins in regulating fish oocyte meiosis and ovarian development. Tang et al. (2019) reported the significant downregulation of AC8 in Nile tilapia Oreochromis niloticus and suggested its involvement in regulating ovary development in Nile tilapia under photoperiod conditions. In the present study, the transcripts of AC type 8 and G2/S1-specific Cyclin-E2 decreased significantly in T. septentrionalis ovaries after temperature rises, which was consistent with previous studies (Meng et al., 2022; Tang et al., 2019), further suggesting that ACs and Cyclin-E2 play regulatory roles in fish ovary development. Moreover, the transcripts of calmodulin 1 (CALM1) involved in the oocyte meiosis pathway were significantly increased in T. septentrionalis ovaries after temperature rises in this study. Previous studies showed that CALM1 is a prototypical calcium sensor and promotes the secretion of steroid hormones (Levorse et al., 1991; Chin and Means, 2000). Moreover, CALM1 has been reported to regulate androgen production in chicken follicular membrane cells (Xu et al., 2016). Thus, the increased transcripts of CALM1 suggest its critical role in regulating temperature-induced ovarian development in T. septentrionalis.
In female animals, reproduction and metabolism are tightly connected and reciprocally regulated, with energy metabolism tuned to meet reproductive needs during the reproductive cycle (Fontana and Torre, 2016). In fish, reproduction requires energy investment to metabolically support ovary maturation and the production of massive eggs in females. Gonad development was found to be accompanied by metabolic and energy-demanding processes, and metabolic changes were closed related to the reproductive cycle to sustain this energy-demanding process specific to the stage of gonadal development or period of growth in female goldfish (Ladisa et al., 2022). In addition, Ladisa et al. (2022) found enhanced lipid metabolism, particularly glycerophospholipid and sphingolipid metabolism, and fatty acids biosynthesis during the reproductive cycle of the mature female goldfish Carassius auratus. In the present study, we also found that lipid metabolism was significantly regulated by the rising temperature, with three pathways, “Glycerophospholipid metabolism”, “Ether lipid metabolism”, and “Sphingolipid metabolism”, significantly enhanced after the temperature rise. The lipid content of ovaries accounts for a large proportion of the fish’s weight, ranging from 12.2% to 25.5% of the wet weight in wild Tilapia nilotica (James Henderson and Tocher, 1987). Furthermore, elevated lipid synthesis during the peak of vitellogenesis and lipid mobilization contributed to supporting maximal gonadal maturation (Ladisa et al., 2022). Thus, we suggest that the higher temperature had promoting effects on the maturation of T. septentrionalis ovary partially by enhancing fish lipid metabolism.
In addition, three metabolic pathways involved in protein and amino acids including “Protein digestion and absorption”, “Biosynthesis of amino acids pathways”, and “Cysteine and methionine metabolism” were also enhanced by the rising temperature in T. septentrionalis ovaries. Zhu et al. (2020) revealed the metabolic changes of fish bodies in harmony with ovarian development and indicated that there were increased active amino acids, lipid metabolism, and energy dynamics in the fish body during ovary development from stage II to IV in female Chinese sturgeon Acipenser sinensis. Urich et al. (2020) showed that the development of oocytes likely resulted from the differences in energy metabolism, protein synthesis, phospholipid production, and elevated levels of amino acids in the gonads of largemouth bass Micropterus salmoides. Thus, the results in this study revealed active metabolism of lipids, proteins, and amino acids in response to the rising temperature, which likely played an important role in promoting the development of T. septentrionalis ovaries.
It was also noteworthy that the ABC transporter pathway was found to be significantly enriched in the T. septentrionalis ovaries in response to rising temperature by the metabolomics analysis. The role of the ABC transporter pathway in fish reproductive processes has increasingly attracted research interest in recent years. Huang et al. (2023) found that ABC transporters were involved in regulating gonad development and oocyte maturation in zebrafish using comparative transcriptome analysis. Shu et al. (2023) showed that the ABC transporter pathway played a crucial role in follicular development and oocyte maturation in the ovaries of Ctenopharyngodon idellus. Sun et al. (2023) found that the ABC transporter pathway was significantly involved in gonad differentiation and development in golden pompano Trachinotus blochii. Previous studies have established that ABC transporters transport ligands across cellular lipid membranes, which is critical for many aspects of cell physiology, including the uptake of nutrients and energy generation (Linton, 2007; Wilkens, 2015). Thus, in the present study, the higher-temperature-induced ovarian development in T. septentrionalis during the overwintering period was closely related to the active processes of nutrition uptake and energy metabolism, which needs to be further studied.
5 Conclusion
In the present study, the potential underlying molecular mechanism of higher-temperature-induced ovary development in T. septentrionalis during the overwintering period was systematically investigated at the cellular and molecular levels. The transcriptomic and metabolomic analyses revealed the key genes, metabolites, and critical regulatory pathways significantly associated with ovary development in T. septentrionalis. The histological and ultrastructural analyses showed the rising temperature contributed to the development of oocytes in T. septentrionalis, which was further supported by the significantly increased plasma E2 concentration. In terms of female gonadal development, rising water temperature was suitable for the development of T. septentrionalis ovaries. The major findings in the present study will provide theoretical scientific evidence for the artificial breeding of T. septentrionali, enrich the scientific acknowledge of the molecular mechanism underlying teleost ovary development, and potentially provide theoretical scientific evidence the conservation efforts in wild fish stocks or fisheries management under global warming.
Data availability statement
The original contributions presented in the study are included in the article/Supplementary Material, further inquiries can be directed to the corresponding author/s.
Ethics statement
The animal study was approved by Experimental animal Ethics Committee of Yantai University. The study was conducted in accordance with the local legislation and institutional requirements.
Author contributions
YL: Conceptualization, Formal Analysis, Funding acquisition, Writing – original draft. WX: Data curation, Formal Analysis, Writing – review & editing. XZ: Data curation, Formal Analysis, Methodology, Writing – review & editing. JW: Writing – review & editing. XC: Data curation, Writing – review & editing. XY: Formal Analysis, Writing – review & editing. JZ: Funding acquisition, Writing – review & editing. YW: Funding acquisition, Writing – review & editing. LL: Conceptualization, Funding acquisition, Project administration, Writing – review & editing.
Funding
The author(s) declare that financial support was received for the research and/or publication of this article. This work was funded by the youth project of the National Natural Science Foundation of China (NNSFC) (grant no. 32302997), the Youth Project of Natural Science Foundation of Shandong Province (ZR2023QC113), Yantai Science and Technology Innovation Development Plan Project (2022XDRH022), Guangxi Academy of Sciences Special Project for Reform and Development (2024YGFZ505-901), Fangchenggang Science and Technology Innovation Development Plan Project (AB23006013), and Lianyungang City Key Research and Development Program (Social Development) (SF2304).
Conflict of interest
The authors declare that the research was conducted in the absence of any commercial or financial relationships that could be construed as a potential conflict of interest.
Generative AI statement
The author(s) declare that no Generative AI was used in the creation of this manuscript.
Publisher’s note
All claims expressed in this article are solely those of the authors and do not necessarily represent those of their affiliated organizations, or those of the publisher, the editors and the reviewers. Any product that may be evaluated in this article, or claim that may be made by its manufacturer, is not guaranteed or endorsed by the publisher.
Supplementary material
The Supplementary Material for this article can be found online at: https://www.frontiersin.org/articles/10.3389/fmars.2025.1556002/full#supplementary-material
Supplementary Figure 1 | Histological observation of T. septentrionalis ovaries. (A) ovary of 6-month-old T. septentrionalis; (B) ovary of 12-month-old T. septentrionalis; (C) ovary of 18-month-old T. septentrionalis; (D) ovary of 24-month-old T. septentrionalis; (E) ovary of 3-year-old T. septentrionalis before rising temperature for overwintering; (F) ovary of 3-year-old T. septentrionalis after the overwintering period. Oo(I): oocyte in phase I; Oo(II): oocyte in phase II; Oo(III): oocyte in phase III. Scale bar: 25 μm.
Supplementary Figure 2 | Detailed information of the experimental design and sampling in this study.
Supplementary Figure 3 | Comparison of qRT-PCR and RNA-Seq results for gene expression of 12 DEGs in T. septentrionalis ovaries responding to temperature treatment. The qRT-PCR and the RNA-Seq results are represented by the red and blue curves, respectively. DEGs, differentially expressed genes.
Supplementary Figure 4 | Total Ion Chromatorgraphy (TIC) of QC samples. The x and y axis represents the time and the sum of ionic strength respectively at each time point. (A) TIC of QC samples in POS mode; (B) TIC of QC sample in NEG. QC, quality control.
Supplementary Figure 5 | The classification of the 1,101 metabolites detected in T. septentrionalis ovaries.
References
Bian L., Li F. H., Ge J. L., Wang P. F., Chang Q., Zhang S. N., et al. (2020). Chromosome-level genome assembly of the greenfin horse-faced filefish (Thamnaconus septentrionalis) using Oxford Nanopore PromethION sequencing and Hi-C technology. Mol. Ecol. Res 20, 1069–1079. doi: 10.1111/1755-0998.13183
Blaser H., Reichman-Fried M., Castanon I., Dumstrei K., Marlow F. L., Kawakami K., et al. (2006). Migration of zebrafish primordial germ cells: a role for myosin contraction and cytoplasmic flow. Dev. Cell 11, 613–627. doi: 10.1016/j.devcel.2006.09.023
Bolger A. M., Lohse M., Usade B. (2014). Trimmomatic: A flexible read trimming tool for Illumina NGS data. Bioinformatics 30, 2114–2120. doi: 10.1093/bioinformatics/btu170
Bonnans C., Chou J., Werb Z. (2014). Remodelling the extracellular matrix in development and disease. Nat. Rev. Mol. Cell Biol 15, 786–801. doi: 10.1038/nrm3904
Brulé T., Renán X., Colás-Marrufo T. (2022). Potential impact of climate change on fish reproductive phenology: A case study in gonochoric and hermaphrodite commercially important species from the southern gulf of Mexico. Fishes 7, 156. doi: 10.3390/fishes7040156
Bustin S. A., Benes V., Garson J. A., Hellemans J., Huggett J., Kubista M., et al. (2009). The MIQE guidelines: minimum information for publication of quantitative real-time PCR experiments. Clin. Chem 55, 611–622. doi: 10.1373/clinchem.2008.112797
Cai S., Li Y., Xu R., He C., Chen K., Chen J., et al. (2023). Transcriptome analysis provides new insights into ovarian follicle growth in common carp (Cyprinus carpio). Aquaculture Rep 33, 101864. doi: 10.1016/j.aqrep.2023.101864
Campbell B., Dickey J., Beckman B., Young G., Pierce A., Fukada H., et al. (2006). Previtellogenic oocyte growth in salmon: relationships among body growth, plasma insulin-like growth factor-1, estradiol-17beta, follicle-stimulating hormone and expression of ovarian genes for insulin-like growth factors, steroidogenic-acute regulatory protein and receptors for gonadotropins, growth hormone, and somatolactin. Biol. Reprod 75, 34–44. doi: 10.1095/biolreprod.105.049494
Cassel M. (2019). A review of the macroscopic, microscopic, and ultramicroscopic characteristics of some key oocyte developmental processes in fish species. IntechOpen. doi: 10.5772/intechopen.87967
Cha K. Y., Koo J. J., K J. J., Choi D. H., Han S. Y., Yoon T. K. (1991). Pregnancy after in vitro fertilization of human follicular oocytes collected from nonstimulated cycles, their culture in vitro and their transfer in a donor oocyte program. Fertil. Steril 55, 109–113. doi: 10.1016/S0015-0282(16)54068-0
Chin D., Means A. R. (2000). Calmodulin: A prototypical calcium sensor. Trends Cell Biol 10, 322–328. doi: 10.1016/S0962-8924(00)01800-6
Curry J., Osteen K. E. (2003). The matrix metalloproteinase system: changes, regulation, and impact throughout the ovarian and uterine reproductive cycle. Endocr. Rev 24, 428–465. doi: 10.1210/er.2002-0005
Dong Y. N., Lyu L. K., Zhang D. Q., Li J., Wen H. S., Bao S. (2021). Integrated lncRNA and mRNA transcriptome analyses in the ovary of Cynoglossus semilaevis reveal genes and pathways potentially involved in reproduction. Front. Genet 12, 671729. doi: 10.3389/fgene.2021.671729
Flores A., Wiff R., Brown D. I., Larrea-Meza S. (2020). Reproductive traits of female of pink cusk-eel (Genypterus blacodes Schneide) in the fjords of the Chilean patagonia. Estuar. Coast. Shelf Sci 233, 106554. doi: 10.1016/j.ecss.2019.106554
Flores A., Wiff R., Díaz E. (2015). Using the gonadosomatic index to estimate the maturity ogive: application to Chilean hake (Merluccius gayi gayi). ICES J. Mari. Sci 72, 508–514. doi: 10.1093/icesjms/fsu155
Fontana R., Torre S. (2016). The deep correlation between energy metabolism and reproduction: a view on the effects of nutrition for women fertility. Nutrients 8, 1–34. doi: 10.3390/nu8020087
Gautier P., Naranjo-Golborne C., Taylor M. S., Jackson I. J., Smyth I. (2008). Expression of the fras1/frem gene family during zebrafish development and fin morphogenesis. Dev. Dyn 237, 3295–3304. doi: 10.1002/dvdy.v237:11
Hara A., Hiramatsu N., Fujita T. (2016). Vitellogenesis and choriogenesis in fishes. Fish. Sci 82, 187–202. doi: 10.1007/s12562-015-0957-5
Hasegawa Y., Surugaya R., Adachi S., Ijiri S. (2022). Regulation of 17α-hydroxyprogesterone production during induced oocyte maturation and ovulation in Amur sturgeon (Acipenser schrenckii). J. Mari. Sci. Eng 10, 86. doi: 10.3390/jmse10010086
Hiramatsu N., Todo T., Sullivan C. V., Schilling J., Reading B. J., Matsubara T. (2015). Ovarian yolk formation in fishes: Molecular mechanisms underlying formation of lipid droplets and vitellogenin-derived yolk proteins. Gen. Comp. Endocrinol 221, 9–15. doi: 10.1016/j.ygcen.2015.01.025
Housh M. J., Telish J., Forsgren K. L., Lema S. C. (2024). Fluctuating and stable high temperatures differentially affect reproductive endocrinology of female pupfish. Integr. Organism. Biol 6, obae003. doi: 10.1093/iob/obae003
Huang Y., Yang H., Li Y., Guo Y., Li G., Chen H. (2023). Comparative transcriptome analysis reveals the effect of Aurantiochytrium sp. on gonadal development in zebrafish. Animals, 13, 2482. doi: 10.3390/ani13152482
Irving-Rodgers H. F., Rodgers R. J. (2005). Extracellular matrix in ovarian follicular development and disease. Cell Tissue Res 322, 89–98. doi: 10.1007/s00441-005-0042-y
James Henderson R., Tocher D. R. (1987). The lipid composition and biochemistry of freshwater fish. Prog. Lipid Res 26, 281–347. doi: 10.1016/0163-7827(87)90002-6
Johnson J., Capco D. G. (1997). Progesterone acts through protein kinase C to remodel the cytoplasm as the amphibian oocyte becomes the fertilization-competent egg. Mech. Dev 67, 215–226. doi: 10.1016/S0925-4773(97)00122-6
Johnson R., Wolf J., Braunbeck T. (2009). OECD guidance document for the diagnosis of endocrine-related histopathology of fish gonads. Organ. Economic Co-operation Dev 96, 1–96. doi: 10.1787/8f7cf3b5-en
Kanehisa M., Goto S., Kawashima S., Okuno Y., Hattori M. (2004). The KEGG resource for deciphering the genome. Nucleic Acids Res 32, D277–D280. doi: 10.1093/nar/gkh063
Ladisa C., Ma Y., Habibi H. R. (2022). Metabolic changes during growth and reproductive phases in the liver of female goldfish (Carassius auratus). Front. Cell Dev. Biol. 10, 834688. doi: 10.3389/fcell.2022.834688
Langmead B. (2010). Aligning short sequencing reads with Bowtie. Curr. Protoc. Bioinf. 32, 11–17. doi: 10.1002/0471250953.2010.32.issue-1
Lasalle A., Benech-Correa G., Brunet F. G., Vizziano-Cantonnet D. (2024). hsd17b1 is a key gene for ovarian differentiation of the Siberian sturgeon. Mol. Reprod. Dev 91, e23729. doi: 10.1002/mrd.23729
Lema S. C., Luckenbach J. A., Yamamoto Y., Housh M. J. (2024). Fish reproduction in a warming world: vulnerable points in hormone regulation from sex determination to spawning. Philos. Trans. R Soc London Ser. B Biol. Sci 379, 20220516. doi: 10.1098/rstb.2022.0516
Levorse J. M., Tilly J. L., Johnson A. L. (1991). Role of calcium in the regulation of theca cell androstenedione production in the domestic hen. J. Reprod. Fertil 92, 159–167. doi: 10.1530/jrf.0.0920159
Li B., Dewey C. N. (2011). RSEM: accurate transcript quantification from RNA-Seq data with or without a reference genome. BMC Bioinform 12, 323. doi: 10.1186/1471-2105-12-323
Li M., Wang J., Huang Q., Li C. (2019). Proteomic analysis highlights the immune responses of the hepatopancreas against Hematodinium infection in Portunus trituberculatus. J. Proteomics 197, 92–105. doi: 10.1016/j.jprot.2018.11.012
Li X., Meng B., Zhang Z., Wei L., Chang W., Wang Y., et al. (2025). qPrimerDB 2.0: an updated comprehensive gene-specific qPCR primer database for 1172 organisms. Nucleic Acids Res 53, D205–D210. doi: 10.1093/nar/gkae684
Linton K. J. (2007). Structure and function of ABC transporters. Physiology 22, 122–130. doi: 10.1152/physiol.00046.2006
Liu X., Qu T., Sachar A., Svovoda K. K. (2015). Cell-Martix Interactions and Signal Transduction. Elsevier Inc. Acad. Press.
Livak K. J., Schmittgen T. D. (2001). Analysis of relative gene expression data using real-time quantitative PCR and the 2-ΔΔCT method. Methods 25, 402–408. doi: 10.1006/meth.2001.1262
Love M. I., Huber W., Anders S. (2014). Moderated estimation of fold change and dispersion for RNA-seq data with DESeq2. Genome Biol 15, 550. doi: 10.1186/s13059-014-0550-8
Maldonado-García M., Gracia-Lopez V., Kiewek-Martínez M., Carrillo M., Zanuy S. (2018). Reproductive cycle of leopard grouper Mycteroperca rosacea (street) held in captivity: relationship between gonad development and sex steroid concentration. La. Am. J. Aquat. Res 46, 83–90. doi: 10.3856/vol46-issue1-fulltext-10
Manning A. J., Burton M. P. M., Crim L. W. (2008). The timing of puberty in cultured female yellowtail flounder, Limanda ferruginea (Storer): oogenesis and sex steroid production in vivo and in vitro. Aquaculture 279, 188–196. doi: 10.1016/j.aquaculture.2008.03.059
Mao X., Liu L., Wang J., Liu Y., Wu Y., Jiang H., et al. (2024). Histological observation on the gonadal development of the parental Thamnaconus modestus during early reproductive regulation. J. Fisher. Res 46, 11–18. doi: 10.14012/j.cnki.fjsc.2024.01.002
McKenzie D. J., Geffroy B., Farrell A. P. (2021). Effects of global warming on fishes and fisheries. J. Fish Biol 98, 1489–1492. doi: 10.1111/jfb.14762
Meng F., Sun S., Xu X., Yu W., Gan R., Zhang L., et al. (2022). Transcriptomic analysis provides insights into the growth and maturation of ovarian follicles in the ricefield eel (Monopterus albus). Aquaculture 555, 738251. doi: 10.1016/j.aquaculture.2022.738251
Mishra A., Joy K. P. (2006). 2-Hydroxyestradiol-17β-induced oocyte maturation in catfish (Heteropneustes fossilis) involves protein kinase C and its interaction with protein phosphatases. Comp. Biochem. Physiol. A Mol. Integr. Physiol 144, 416–422. doi: 10.1016/j.cbpa.2006.03.006
Nyuji M., Hongo Y., Kazeto Y., Yoneda M. (2024). Characterization of eight types of 17β-hydroxysteroid dehydrogenases from the Japanese sardine Sardinops melanostictus: The probable role of type 12a in ovarian estradiol synthesis. Gen. Comp. Endocrinol 347, 114423. doi: 10.1016/j.ygcen.2023.114423
Pallotta M. M., Fogliano C., Carotenuto R. (2022). Temperature incubation influences gonadal gene expression during Leopard gecko development. Animals 12, 3186. doi: 10.3390/ani12223186
Paz M., López-Casas P. P., Mazo J. D. (2007). Changes in vinexin expression patterns in the mouse testis induced by developmental exposure to 17beta-estradiol. Biol. Reprod 77, 605–613. doi: 10.1095/biolreprod.107.060020
Pertea M., Kim D., Pertea G. M., Leek J. T., Salzberg S. L. (2016). Transcript-level expression analysis of RNA-seq experimentswith HISAT, StringTie and Ballgown. Nat. Protoc 11, 1650–1667. doi: 10.1038/nprot.2016.095
Pörtner H. O., Berdal B., Blust R., Brix O., Colosimo A., De Wachter B., et al. (2001). Climate induced temperature effects on growth performance, fecundity and recruitment in marine fish: developing a hypothesis for cause and effect relationships in Atlantic cod (Gadus morhua) and common eelpout (Zoarces viviparus). Cont. Shelf Res 21, 1975–1997. doi: 10.1016/S0278-4343(01)00038-3
Sánchez-Hernández M., Chaves-Pozo E., Cabas I., Mulero V., García-Ayala A., García-Alcázar A. (2013). Testosterone implants modify the steroid hormone balance and the gonadal physiology of gilthead seabream (Sparus aurata L.) males. J. Steroid Biochem. Mol. Biol 138, 183–194. doi: 10.1016/j.jsbmb.2013.05.014
Santella L., Limatola N., Chun J. T. (2014). Actin cytoskeleton and fertilization in starfish eggs. Springer Open. doi: 10.1007/978-4-431-54589-7_13
Sharma P., Purohit S., Bhatt G., Kothiyal S., Singh M., Nautiyal P., et al. (2023). A study on the seasonal cyclicity of ovarian development in adult himalayan snow trout, Schizothorax plagiostomus. J. Fish Biol 103, 292–304. doi: 10.1111/jfb.v103.2
Shu T., Chen Y., Xiao K., Huang H., Jia J., Yu Z., et al. (2023). Effects of short-term water velocity stimulation on the biochemical and transcriptional responses of grass carp (Ctenopharyngodon idellus). Front. Physiol 14, 1248999. doi: 10.3389/fphys.2023.1248999
Souza T. G., Abreu M. R., Kuradomi R. Y., Batlouni S. R. (2024). Effect of temperature on gonadal differentiation and growth of Leporinus friderici. Anim. Reprod 21, e20230158. doi: 10.1590/1984-3143-ar2023-0158
Spinks R. K., Bonzi L. C., Ravasi T., Munday P. L., Donelson J. M. (2021). Sex- and time-specific parental effects of warming on reproduction and offspring quality in a coral reef fish. Evol. Appl 14, 1145–1158. doi: 10.1111/eva.13187
Sun S., Song F., Shi L., Zhang K., Gu Y., Sun J., et al. (2023). Transcriptome analysis of differentially expressed circular RNAs in the testis and ovary of golden pompano (Trachinotus blochii). Com. Biochem. Physiol. Part D Genomics Proteomics 45, 101052. doi: 10.1016/j.cbd.2022.101052
Tang Z., Zhou Y., Xiao J., Zhong H., Miao W., Guo Z., et al. (2019). Transcriptome analysis of ovary development in Nile Tilapia under different photoperiod regimes. Front. Genet 10, 894. doi: 10.3389/fgene.2019.00894
Urbatzka R., Rocha M. J., Rocha E. (2011). Regulation of ovarian development and function in teleosts. Hormones Reprod. Vertebrates 35, 65–82. doi: 10.1016/B978-0-12-375009-9.10004-9
Urich M. L., Henderson W. M., MacLeod A. H., Yonkos L. T., Bringolf R. B. (2020). Gonad metabolomics and blood biochemical analysis reveal differences associated with testicular oocytes in wild largemouth bass (Micropterus salmoides). Com. Biochem. Physiol. Biochem. Mol. Biol 250, 110491. doi: 10.1016/j.cbpb.2020.110491
Wang L., Zhu J., Hu M., Cai L., Wang Y., Zhou X., et al. (2024). Comparative transcriptome analysis reveals molecular mechanisms of the effects of light intensity and photoperiod on ovarian development in Procambarus clarkii (Girar). Comp. Biochem. Physiol. Part D Genomics Proteomics. 52, 101329. doi: 10.1016/j.cbd.2024.101329
Wanzenböck S., Fuxjäger L., Ringler E., Ahnelt H., Shama L. (2022). Temperature-dependent reproductive success of stickleback lateral plate morphs: implications for population polymorphism and range shifts under ocean warming. Front. Mari. Sci 9, 759450. doi: 10.3389/fmars.2022.759450
Wilkens S. (2015). Structure and mechanism of ABC transporters. F1000prime Rep 7, 14. doi: 10.12703/P7-14
Wright P. J., Orpwood J. E., Boulcott P. (2017). Warming delays ovarian development in a capital breeder. Mari. Biol 164, 80. doi: 10.1007/s00227-017-3116-y
Xu Q., He H., Ma R., Chen Z., Yi Z., Kang B., et al. (2016). Characterization and differential expression profile of calm1 gene in sichuan white goose. Xi Bei Nong Ye Xue Bao 31, 128–133. doi: 10.7668/hbnxb.2016.01.021
Yadav N. K., Patel A. B., Singh S. K., Mehta N. K., Anand V., Lal J., et al. (2024). Climate change effects on aquaculture production and its sustainable management through climate-resilient adaptation strategies: a review. Environ. Sci. pollut. Res 31, 31731–31751. doi: 10.1007/s11356-024-33397-5
Young M. D., Wakeeld M. J., Smyth G. K., Oshlack A. (2010). GOseq: Gene Ontology testing for RNA-seq datasets. Genome Biol 11, R14. doi: 10.1186/gb-2010-11-2-r14
Yue L., Zhang L., He Y., Zhang J., Zheng J., He Y., et al. (2004). Reorganization of cytoskeletal proteins of mouse oocytes mediated by integrins. Sci. China C Life Sci 47, 540–544. doi: 10.1360/03yc0155
Zhang J. N. (2011). Reproductive regulation and fry breeding technology of Thamnaconus septentrionalis Günther. [master’s thesis] (China: Ocean University of China).
Zhao Y., Chen H., Wang Y., Mei Y., Huang L., Wang H., et al. (2020). Gonad development examination of high-temperature–treated genetically female Nile tilapia. Aquaculture 515, 734535. doi: 10.1016/j.aquaculture.2019.734535
Zhu Y., Wu J., Leng X., Du H., Wu J., He S., et al. (2020). Metabolomics and gene expressions revealed the metabolic changes of lipid and amino acids and the related energetic mechanism in response to ovary development of Chinese sturgeon (Acipenser sinensis). PloS One 15, e0235043. doi: 10.1371/journal.pone.0235043
Keywords: Thamnaconus septentrionalis, ovary development, molecular mechanism, transcriptomics, metabolomics
Citation: Liu Y, Xu W, Zhang X, Wang J, Chen X, Yu X, Zeng J, Wu Y and Liu L (2025) The molecular mechanism of ovary development in Thamnaconus septentrionalis induced by rising temperature via transcriptomics and metabolomics analysis. Front. Mar. Sci. 12:1556002. doi: 10.3389/fmars.2025.1556002
Received: 06 January 2025; Accepted: 18 March 2025;
Published: 11 April 2025.
Edited by:
Gustavo M. Somoza, Instituto Tecnológico de Chascomús (CONICET-UNSAM), ArgentinaReviewed by:
Juan Ignacio Fernandino, CONICET Institute of Biotechnological Research (IIB-INTECH), ArgentinaKhaled Mohammed Geba, Menoufia University, Egypt
Copyright © 2025 Liu, Xu, Zhang, Wang, Chen, Yu, Zeng, Wu and Liu. This is an open-access article distributed under the terms of the Creative Commons Attribution License (CC BY). The use, distribution or reproduction in other forums is permitted, provided the original author(s) and the copyright owner(s) are credited and that the original publication in this journal is cited, in accordance with accepted academic practice. No use, distribution or reproduction is permitted which does not comply with these terms.
*Correspondence: Yan Liu, bGl1eWFuc2RAeXR1LmVkdS5jbg==; Liming Liu, bGl1X2xpbWluZzcxQDE2My5jb20=