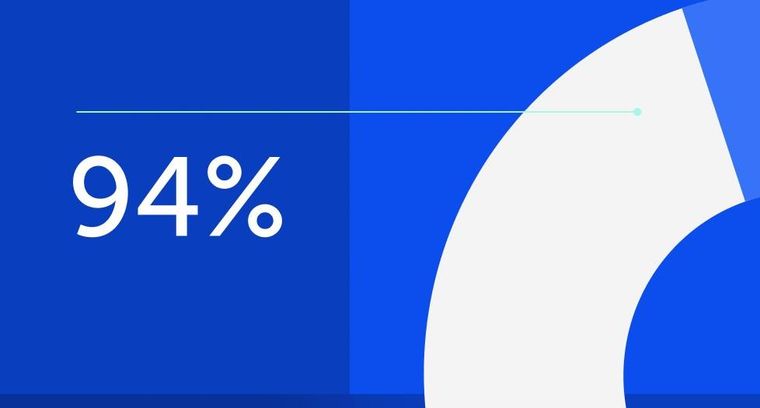
94% of researchers rate our articles as excellent or good
Learn more about the work of our research integrity team to safeguard the quality of each article we publish.
Find out more
ORIGINAL RESEARCH article
Front. Mar. Sci., 11 April 2025
Sec. Marine Fisheries, Aquaculture and Living Resources
Volume 12 - 2025 | https://doi.org/10.3389/fmars.2025.1553629
This article is part of the Research TopicDevelopment of Sustainable Aquafeed: Novel Ingredients and FormulationsView all 4 articles
Due to the increasing trend in aquaculture and fisheries production, conventional feed resources, especially protein ingredients, are overburdened, and sometimes their price fluctuations limit quality feed formulations for farmers, thus leading to the exploration of alternative protein feed resources. Antarctic krill meal (AKM) has emerged as a potential candidate ingredient for sustainable and suitable partial replacement of conventional fish meal. A 10-week feeding trial was designed to investigate the effects of different concentrations of AKM on the growth performance, antioxidant capacity, and serum biochemical indices of coho salmon (Oncorhynchus kisutch) post-smolts. In total, 150 post-smolt fish with an average initial weight of 178.17 ± 0.73 g were divided into five groups with three replicates in each group and 10 fish in each replicate. Five experimental diets were formulated by substituting 0%, 3%, 6%, 9%, and 12% fish meal (protein source) with AKM (protein source), which were designated AKM0 (the control), AKM3, AKM6, AKM9, and AKM12, respectively. The results showed that the addition of AKM to the salmon diet increased (P < 0.05) the weight gain (WG), specific growth rate (SGR), feed conversion ratio (FCR), and protein efficiency ratio (PER). There was no effect (P > 0.05) on body composition and essential amino acid profile of the meat except for methionine, isoleucine, lysine, and threonine. The liver concentrations of alanine aminotransferase (ALT), aspartate aminotransferase (AST), and malondialdehyde (MDA) significantly decreased while the concentration of catalase and superoxide dismutase (SOD) increased in the AKM supplemented groups compared to the control group. The serum concentrations of ALT and AST decreased (P < 0.05) while total cholesterol, triglyceride, and total protein content increased (P < 0.05) in the AKM treatment groups compared to the control. Based on the results of this study, supported by polynomial quadratic regression analyses of WG, SGR, FCR, and PER, we conclude that 6.73%–7.08% AKM is the best possible suitable inclusion level range to partially replace conventional fish meal in the diet of coho salmon post-smolt.
Fish consumption is increasing with the growing awareness of public health improvement through healthy food. The Food and Agriculture Organization (FAO) is expecting a 10% growth rise in aquatic production by 2032 to fulfill the increasing demand for fish products (FAO, 2024). The world’s production of aquaculture and fisheries has witnessed tremendous growth with a total production of 223.20 million tons during 2022 (FAO, 2024). Owing to this, in recent years, the demand for fish feed has also increased due to the increasing farming area (Waagbø et al., 2001). Of the various available protein ingredients for fish feed, fish meal is the best and contributes a major proportion of the protein source in quality feed formulations, which may no longer meet the growing feed demand for aquatic animals (Choi et al., 2020; Yan et al., 2024). Additionally, the availability and pricing pattern of various protein meals varies during different production seasons (Yan et al., 2024). Therefore, it is urgent to investigate, explore, and choose other high-quality sustainable aquatic animal feeds to combat this problem. Antarctic krill meal (AKM) is a potential candidate as a protein feed ingredient in fish feeds to contribute to sustainable aquaculture (Kiranpreet et al., 2022) and partially replace fish meal (Choi et al., 2020; Yan et al., 2024).
Antarctic krill is a small crustacean with black-colored eyes and covered with a semitransparent reddish-tinted shell. Its resource is estimated to reach 500 million tons, which is the largest source of animal protein on the earth, and the development prospect is very broad (Atkinson et al., 2009; Kiranpreet et al., 2022). Although Antarctic krill is an important species in the food chain of the marine ecosystem in the ocean and is regulated by certain rules and regulations to limit its harvesting, there is still a huge and abundant biomass of krill available to be used in animal feed (Kiranpreet et al., 2022). AKM is a good source of protein, lipids, and omega-3 fatty acids (Kiranpreet et al., 2022), and is usually used to partially replace fish meal in fish diets (Kubitza and Lovshin, 1997; Olsen et al., 2006; Suontama et al., 2007; Atkinson et al., 2009; Kiranpreet et al., 2022; Yan et al., 2024). Because its phospholipid, vitamin, chitin, nucleotide, and natural carotenoid levels are significantly higher than fish meal, it is even more suitable for aquatic feed than fish meal (Kubitza and Lovshin, 1997; Everson, 2000; Olsen et al., 2006; Suontama et al., 2007; Hansen et al., 2011; Tibbetts et al., 2011; Lu et al., 2014), and due to its sustainability and high palatability characteristics, this has greatly increased the interest of the aquaculture industry in adding AKM to the feed (Kiranpreet et al., 2022).
AKM inclusion in the diet can stimulate the appetite of fish, thereby having a direct influence on improving feed intake and growth (Anderson et al., 1997; Oikawa and March, 1997; Olsen et al., 2006; Tibbetts et al., 2011; Yan et al., 2018; Wei et al., 2019), and it also has a certain impact on the immune system, meat quality, fat metabolism, and gonad development of fish (Wang et al., 2021). However, although AKM has many advantages, it cannot completely replace fish meal and they can sometimes be interchanged or partially replaced (Vens-Capell and Horstmann, 1978; Papuktchieva et al., 1981). AKM addition to the diet of Atlantic salmon (Salmo salar) resulted in increased feed intake, growth performance, and fillet quality (Hatlen et al., 2017). AKM has the potential to effectively improve the reproductive performance in sole (Cynoglossus semilaevis) broodstock (Xu et al., 2016). For rainbow trout, partially replacing fish meal with AKM can increase its growth performance or will not negatively affect the feed economics (Pfeffer and Becker, 1977; Jahn et al., 1978; Koops et al., 1979).
Coho salmon belongs to the genus Oncorhynchus and normally inhabits the Pacific Ocean with a wide range from the Russian Bering Sea to Alaska, moving from the north coastal side of America to California, and has huge commercial importance (Hart, 1973). It is known to spend its lifespan both in fresh water and marine water in its hatching and spawning cycles. Salmon farming is gaining popularity worldwide and was the most valuable trading species group among other aquatic groups in 2022 (FAO, 2024). Coho salmon is rich in healthy nutritional components, containing high quantities of protein and polyunsaturated fatty acids (PUFA) and significantly high omega-3 fatty acids, astaxanthin, and phospholipids, which are well known for having various public health benefits (Tinsley et al., 1973; Ma et al., 2007; Swanson et al., 2012; Kiranpreet et al., 2022). Owing to its rich nutritional qualities and high economic value, coho salmon has gained popularity in China in recent years (Yu et al., 2022). With these recent advancements, it is important to assess the nutritional needs of coho salmon and explore alternative protein sources for quality feed formulation to achieve sustainable farming by addressing the feed shortage and pricing challenges faced in coho salmon farming. In this context, to the best of our knowledge, to date, there have been very few or no reports on the effects of adding AKM to the diet on coho salmon (Oncorhynchus kisutch) post-smolt growth and development, antioxidant capacity, and hematological indicators, especially during the early growing stage. Therefore, this trial explored the effect of adding different levels of AKM to the diet on coho salmon (O. kisutch) post-smolt growth and development, antioxidant capacity, and blood biochemical indices, aiming to find the most suitable and safe inclusion level of AKM to be added to the diet of coho salmon post-smolt for sustainable farming.
The present study was carried out according to the recommendations in the “Guide for the Ethical Use of Experimental Animals” of Weifang University, Weifang, Shandong, China (No. 20200601) and it was approved by the Institutional Animal Care and Use Committee of the Institute of Modern Facility Fisheries, Weifang University, China (IACUC NO. 20220516003).
Imported ultra-steamed fish meal, soya bean meal, and shrimp meal were used as the main sources of protein, while soyabean oil and fish oil were the main sources of fat in the diets. Five diets with the inclusion of 0%, 3%, 6%, 9%, and 12% AKM fish meal [i.e., AKM0 (control), AKM3, AKM6, AKM9, and AKM12, respectively] were formulated. All of the five diets were formulated to be isonitrogenous and isocaloric. The chemical composition and amino acid profile of fish meal and AKM are given in Table 1. The dietary ingredient composition, analyzed nutrient composition, and amino acid profile of the experimental diets are shown in Tables 2, 3.
Table 1. Nutrient composition and amino acid profile of fish meal and Antarctic krill meal (% dry matter).
Table 2. Formulation and nutrient composition of the experimental diets for post-smolt coho salmon (Oncorhynchus kisutch) (% dry matter).
Table 3. Amino acid (AA) profile of the experimental diets for post-smolt coho salmon (Oncorhynchus kisutch), g/kg crude protein.
The post-smolt coho salmon used in the trial were procured from the hatchery of the National Aquatic Product Introduction and Breeding Centre (Beijing, China), and were placed in the cemented ponds of the aquaculture base of Shandong Wanda Fishery Co., Ltd (Weifang, China). One week of adaptation and acclimatization was provided to the salmon with the control feed before the formal trial. Then, 150 experimental fish with strong adaptability, uniform size, and initial weights of 178.17 ± 0.73 g were selected to be used in the trial. The experimental fish were randomly divided into five groups with three replicates and 10 fish in each replicate. Experimental groups were offered diets with AKM0 (control), AKM3, AKM6, AKM9, and AKM12. The fish were fed three times a day (at 7:00, 12:00, and 17:00), with a feeding rate of approximately 3% of body weight for 10 weeks, ensuring consumption of all the offered feed. During the whole 10-week feeding trial, the temperature of the water was maintained at 15.0 ± 1.5°C, the pH value was kept between 7.1 and 7.5, and the dissolved oxygen concentration was higher than 7.5 mg/L. The post-smolt coho salmon were reared in the freshwater under natural sunlight.
At the end of the 10-week feeding trial, all fish were starved for 24 h and then the number of fish and the weight of all fish in each replicate were recorded. After that, three fish were randomly selected from each replicate to record their body weight and body length and blood was collected from the tail vein with a 1-ml disposable medical syringe and kept at 4°C for 3–4 hours to clot. Serum was separated by centrifugation at 3,000 RPM for 10 minutes at 4°C and then stored at -80°C to analyze the biochemical parameters and antioxidant capacity. The fish was then quickly dissected to collect the viscera and liver. The weight of the viscera and liver were recorded to calculate the hepatosomatic index (HSI) and viscerosomatic index (VSI). Then, the whole-body muscle and liver samples were collected and kept at -20°C for further analysis. The fish muscle samples were used for body composition and amino acid profile analyses. Liver samples were used to assess liver function enzyme activity and antioxidant capacity.
At the start of the trial, the initial body weight of all fish and fish number were recorded. Then, at the end of the trial (10th week), final body weight, feed intake, and fish number were also recorded. This data was then used to calculate the survival rate (SR), weight gain (WG), specific growth rate (SGR), feed conversion ratio (FCR), protein efficiency ratio (PER), and condition factor (CF) during the trial. The liver and visceral weights, as mentioned in the above paragraph, were used to calculate the HSI and VSI during the whole 10 weeks of the trial using the following standard equations:
As mentioned in the above section, the collected body muscle samples were analyzed using methods as prescribed in AOAC (1990) (AOAC (Association of Official Analytical Chemists), 1990) for nutrient composition analysis; crude protein was determined by Kjeldhal apparatus (Automatic Apparatus Kjeltec 8400, FOSS, Denmark) by calculating nitrogen (N × 6.25); crude fat was determined using Soxhlet (ST 243, FOSS, Denmark); moisture was determined at 105°C to constant weight; and feed samples were burnt at 550°C for 12 h for crude ash analysis. Muscle essential amino acid profile analysis was performed as described by (Palliyeguru et al., 2010); briefly, meat samples were minced and lyophilization was performed using a freeze dryer (EYELA FDU-1200, RIKAKIKAI, Tokyo, Japan; Glass vacuum chamber DRC-3L, Serial 1113594, RIKAKIKAI, Tokyo, Japan) and well-ground to 500 microns. Then, a 100 mg sample was oxidized with a 5 ml performic acid and phenol mixture for 16 hours. Afterward, sodium disulfide (840 mg) was added to decompose the excess oxidizing agent, stirred for 10 minutes, and then hydrolysis was performed using 15 ml of 6 N phenolic HCL solution at 110°C for 24 hours. The pH of the hydrolysate was adjusted to 2.20. After that, the hydrolysate was centrifuged and filtered to determine the amino acids profile using an automatic amino acid analyzer (Serial No. 199.16-01, 2016, Model A300, PMA GmbH, Germany).
As mentioned in the above section, the liver and serum samples were prepared and stored at -20°C and -80°C respectively. The collected liver samples for liver enzyme analysis were homogenized and placed in 0.1 M pH 7.4 Tris-HCl buffer at 4°C, and then used for analysis. The liver and serum enzyme activity and related indicators, including glutamate transaminase (ALT), glutamate transaminase (AST) activity, liver malondialdehyde (MDA) content, superoxide dismutase activity (SOD), serum triglycerides (TG), and total cholesterol (TC) content, were determined by a commercial reagent kit (Nanjing Jiancheng Bioengineering Institute, Nanjing, China) using automatic analyzer.
The data obtained from the experiment were confirmed for homogeneity and normality using the Shapiro–Wilk and Levene’s tests and were then subjected to statistical analysis using one-way ANOVA in SPSS software (IBM SPSS Statistics 21). The significance between the mean values was tested using Duncan’s test. The values in the table are presented as the mean ± SE (standard error), and the level of significant difference was P < 0.05. Polynomial regression analysis was used to determine the optimal inclusion level of AKM to replace fish meal, based on WG, SGR, FCR, and PER in the fish diet.
The research results for growth performance are shown in Table 4. Results showed that FBW, WG, DWG, SGR, FCR, and PER significantly improved (P < 0.001) in all treatment groups compared to the control group. The highest (P < 0.001) values for these parameters were observed in the AKM6 group. FBW, WG, and DWG were the highest (P < 0.001) in the AKM6 group followed by the AKM9, AKM12, and AKM3 groups and the lowest was the AKM0 group. SGR was significantly higher in groups AKM6 and AKM9 compared to other groups, followed by the AKM3 and AKM12 groups, which differed significantly from the AKM0 group. FCR was better in the AKM6 and AKM9 groups (P < 0.001) followed by the AKM3 and AKM12 groups with significantly lowest value in the AKM0 group. However, PER was greater (P < 0.001) in the AKM6 group compared to all the other groups, followed by the AKM9 and then the AKM6 and AKM3 groups, but the significantly lowest value was observed in the AKM0 group. There were no significant differences in SR, initial body weights, CF, HIS, and VSI (P > 0.05). The polynomial regression analysis showed that there was a significant positive (P < 0.001) correlation between the growth performance parameters and AKM (Figures 1–4). The quadratic regression analysis showed the highest (P < 0.001) WG, SGR, and PER, and lowest (P < 0.001) FCR in coho salmon at the AKM6 supplementation level and predicted that the inclusion of 6.93%, 6.73%, 7.08%, and 6.83% AKM would lead to optimal performance on the basis of WG, SGR, FCR, and PER, respectively.
Table 4. Growth performance of coho salmon post-smolts (Oncorhynchus kisutch) fed diets for 10 weeks with Antarctic krill meal (AKM) partially substituting for fish meal (FM) (Mean ± SE).
Figure 1. A polynomial quadratic regression analysis of weight gain with various inclusion levels of Antarctic krill meal in coho salmon (Oncorhynchus kisutch) post-smolts diet revealed that the predicted suitable inclusion level of AKM was 6.93% of the diet for optimal WG.
Figure 2. A polynomial regression analysis of specific growth rate with various inclusion levels of Antarctic krill meal in coho salmon (Oncorhynchus kisutch) post-smolts diet revealed that the predicted suitable inclusion level of AKM was 6.73% of the diet for optimal SGR.
Figure 3. A polynomial regression analysis of the relationship between feed conversion ratio and Antarctic krill meal inclusion level predicted that the suitable inclusion level of AKM was 7.08% of diet for optimal FCR in coho salmon (Oncorhynchus kisutch) post-smolts.
Figure 4. A polynomial regression analysis of the relationship between protein efficiency ratio and Antarctic krill meal inclusion level evaluated that the suitable inclusion level of AKM was 6.83% of diet for optimal PER in coho salmon (Oncorhynchus kisutch) post-smolts.
The results of the proximate analysis of fish muscle meat samples and their essential amino acid profiles showed that methionine concentration increased (P < 0.001) in the krill meal treatment groups with the highest in the AKM12 group and it gradually decreased with decreases in AKM levels, but still showed a significantly improved trend in all treatment groups as compared to AKM0. Isoleucine, lysine, and threonine concentrations decreased (P < 0.001) in the supplemented groups compared to the control group (AKM0). However, cystine concentration was only better (P < 0.05) in the AKM3 group while the other groups did not differ (Table 5). All other parameters of proximate composition of muscle including moisture, crude protein, lipid, ash content, and essential amino acid content remained unaffected (P > 0.05).
Table 5. Muscle proximate composition and essential amino acid profile of post-smolt coho salmon (Oncorhynchus kisutch) fed diets for 10 weeks with Antarctic krill meal (AKM) partially substituting for fish meal (FM) (Mean ± SE).
The research results for ALT, AST, MDA, and catalase and SOD activity in the liver showed significant differences in the treatment groups compared to the control group (P < 0.001), as shown in Table 6. ALT, AST, and MDA concentrations in the salmon livers were lower (P < 0.001) while catalase and SOD activity was increased (P < 0.001) in the AKM treatment groups compared to the control group. There was a gradual decrease in ALT, AST, and MDA concentrations with increasing dietary levels of AKM. The lowest values (P < 0.001) of ALT, AST, and MDA were observed in the high supplementation group (AKM12). Catalase content significantly increased with the increase in dietary levels of AKM, showing the highest values in the AKM6, AKM9, and AKM12 groups followed by the AKM3 group compared to the control group. Catalase values did not differ significantly in the AKM6, AKM9, and AKM12 groups. SOD activity was highest (P < 0.001) in the AKM6 group followed by the AKM9, AKM12, and AKM3 groups, and lowest (P < 0.001) in the non-supplemented control group.
Table 6. Liver function and anti-oxidative enzyme activities of post-smolt coho salmon (Oncorhynchus kisutch) fed diets for 10 weeks with Antarctic krill meal (AKM) partially substituting for fish meal (FM) (Mean ± SE).
The results of the serum indices showed a decrease (P < 0.001) in ALT and AST enzyme activity while TC, TG, and TP concentrations were increased (P < 0.05) in the AKM-supplemented groups compared to the control group. ALT and AST enzyme concentrations showed a gradual decreasing (P < 0.001) trend with an increase in dietary levels of AKM, showing the lowest (P < 0.001) values of these enzymes in the AKM 12 and AKM9 groups compared to the control. The TC value was significantly higher (P < 0.001) in the AKM6, AKM9, and AKM12 groups followed by the AKM3 and AKM0 groups. TG was higher (P < 0.001) in the AKM9 and AKM12 groups compared to the other groups while TP was better (P < 0.001) in all the treatment groups with the highest values in the AKM6, AKM12, and AKM9 groups followed by the AKM3 group compared to the control group (Table 7).
Table 7. Serum biochemical parameters of post-smolt coho salmon (Oncorhynchus kisutch) fed diets for 10 weeks with Antarctic krill meal (AKM) partially substituting for fish meal (FM) (Mean ± SE).
AKM contains an ample quantity of high-quality protein and unsaturated fatty acids, which can be effectively used in aquatic feed (Vens-Capell and Horstmann, 1978). The crude protein content of AKM is 49%–80%, the fat content is 4%–26%, and the crude ash content is 4%–17% (Gigliotti et al., 2008; Xu et al., 2019; Wang, 2020). Its protein contains nine essential amino acids, the lipid components are mainly polar lipids and triglycerides, and its bio-availability is higher than that of fish oil (Sun, 2019). AKM has a similar fat level to fish meal, but it has 10% less protein than fish meal and contains more ash and salt (Olsen et al., 2006). AKM plays an extremely important role in the growth and development of fish, fish quality, liver and kidney health, and reproduction.
The growth of the fish is directly related to the quality of feed, feed intake, absorption, and utilization of feed nutrients along with growth factors that may directly or indirectly influence the digestive mechanism, leading to improved performance. AKM is known to have a specific growth factor that was found to be a residue of the growth hormone from the neurosecretory X-organ-Sinus gland responsible for regulating the metabolism in the body (Williams et al., 2005). In addition, the high content of n-3 fatty acids and low content of n-6 fatty acids in AKM are also very important (Roncarati et al., 2011) for improving the growth performance of fish. Due to the presence of these factors, AKM seems to have more growth-promoting benefits than conventional fish meal. In this study, we found that adding AKM improved the FW, WG, SGR, FCR, and PER of coho salmon. We speculated that it might be because feeding AKM could promote the ability of coho salmon to absorb nutrients from the feed, thereby boosting their growth performance. Previous studies have found that supplementation of 7.5% of AKM increased growth performance, FCR, and PER in European sea bass (Dicentrarchus labrax) (Torrecillas et al., 2021); Turid et al. (Mørkøre et al., 2020), in Atlantic salmon, reported safe replacement of fish meal with 12% KM; in Salmo salar (Atlantic salmon), a 10%-30% replacement was reported (Julshamn et al., 2004); Saleh et al. (Saleh et al., 2018) reported improved FCR at 9% AKM supplementation. Similarly, many researchers reported that in Oncorhynchus tshawytscha (Anderson et al., 1997), Micropterus salmoides (Zhu et al., 2019), Gadus chalcogramus (Choi et al., 2020), and Larimichthys crocea (Wei et al., 2019) fish meal can be safely replaced with AKM. Further, in small aquaculture species, research has shown that the substitution of different amounts of fish meal with krill meal has significantly increased the growth performance in prawn (Yan et al., 2024) and shrimp (Wei et al., 2022), with up to 20% inclusion levels. Some other studies showed that the addition of AKM up to 15% has increased performance while 30% KM led to a decrease in the growth rate (Yoshitomi et al., 2006), presumably due to the different rearing environment and high inclusion level of AKM. It was also found by Xie et al. (Xie et al., 2022) that there was no significant effect on Pelteobagrus fulvidraco growth and survival rate with the inclusion of krill meal up to 10%. Moreover, it was found that the fish SGR was higher in those supplemented with 20% and 30% AKM than that in the control group (Julshamn et al., 2004). This variation in growth performance with variable inclusion levels of AKM can be attributed to the different species, environments, feed compositions, and water quality (Kiranpreet et al., 2022). Fish meal can be completely replaced with AKM (Wei et al., 2019; Wei et al., 2022) without adverse effects on the growth, however, in our study, the advantageous inclusion level of AKM in coho salmon was predicted to range between 6.93% and 7.07% in comparison to the control group fed fish meal. Although the AKM12 group showed improved growth performance compared to the control, it showed less growth when we compared it with the AKM6 group. Besides many other reasons and differences in rearing environment (Kiranpreet et al., 2022), this might be linked with the increased intake of certain minerals, including copper, fluoride, anti-nutritional factors (Albrektsen et al., 2022), and chitin (Olsen et al., 2006; Rødde et al., 2008; Hansen et al., 2010), present in AKM in greater concentrations by the salmon when AKM is supplemented in higher amounts, thereby decreasing the digestive efficiency and resulting in reduced growth (Yan et al., 2024). Additionally, the quadratic regression analysis in our study showed that the predicted optimal addition of AKM in the coho salmon diet was 6.93%, 6.73%, and 7.07% for WG, SGR, and FCR respectively. Therefore, can be concluded that the substitution of fish meal with krill meal has no adverse effects and could improve the growth and developmental performance of post-smolt coho salmon with an inclusion level of 6%.
The muscle composition analysis showed that there were no effects of AKM supplementation on the contents of moisture, crude protein, lipids, and ash. The essential amino acid profile also remained unchanged except for lysine, methionine, and threionine. The concentration of methionine was increased while lysine and methionine decreased compared to the control group. We hypothesize that this change in these amino acids may be associated with the dietary composition having more and less concentrations of these, respectively. Similarly, it has been reported that muscle composition was not affected by the dietary inclusion of AKM (Khosravi et al., 2018; Choi et al., 2020; Wei et al., 2022) and muscle composition is dependent on the fish species. The no major change in muscle composition indicates the good acceptance of AKM by the coho salmon and shows the safe substitution of fish meal with krill meal.
MDA is the ultimate product of lipid peroxidation reactions, which indicates the status of oxidative cellular and tissue damage induced by reactive oxygen species under stimulation of reactive nitric oxide species (Venditti and Di Meo, 2006; Zhang et al., 2021). MDA is usually used as an important measure of oxidative cell damage (Mu et al., 2012). Catalase and SOD are the enzymes of the antioxidant system of the body and serve as the first line of defense against reactive oxygen species; thus the antioxidant enzymes catalase and SOD can detoxify superoxide anion radicals to reduce the damage caused by lipid peroxidation (Vespasiani-Gentilucci et al., 2014; Sara et al., 2017). In this trial, the addition of AKM significantly reduced the content of MDA in the liver and serum (Wei et al., 2022) of the post-smolt coho salmon and significantly increased the content of catalase and SOD (P < 0.05) in both the liver and serum. Similar results were also reported in an experiment on juvenile spotted halibut, Veraper variegatus (Yan et al., 2018; Yan et al., 2023). Khosravi et al. (Khosravi et al., 2015) demonstrated that the SOD activity in red sea bream Pagrus major fed Antarctic krill proteolysate was greatly improved compared with those fed fish meal. AKM has potential to increase the content of hepatopancreatic glutathione (GSH), which is an important endogenous antioxidant in fish and scavenges the free radicals (Wei, 2015; Liu et al., 2020; Yan et al., 2024). Astaxanthin, zinc, and selenium content in AKM could also be the possible reasons for these described results (Zhang et al., 2013; Wei et al., 2022). Astaxanthin can remove oxygen free radicals in the liver and improve disease resistance. Selenium can improve the activity of antioxidant enzymes, eliminate hydrogen peroxide, reduce the toxicity of fluorine, and affect the cell antioxidant system. Zinc can promote the expression of metallosulfur protein, protect the integrity of cell membrane, and increase its antioxidant capacity (Xiao et al., 2006; Zhang et al., 2013; Xie et al., 2019). The most pronounced efficacy of AKM inclusion on antioxidant status was found in the AKM6 group in this study. Conclusively, this showed that AKM has certain elements that are responsible for reducing oxidation and improving the antioxidant status in coho salmon, maintaining its health (Wei et al., 2022).
During the routine normal process of metabolism, the concentration of transaminases is maintained at a normal range in the serum (Chen et al., 2022). However, the serum concentration of liver enzymes increases when there is abnormal metabolism stress and the liver is somewhat damaged (Nyblom et al., 2004). Therefore, serum ALT and AST activity are two important indicators to reflect liver function and animal health status (Lin et al., 2007). Under normal circumstances, they should be present in the liver and are barely detectable or present in lower concentrations in the blood (Nyblom et al., 2004; Xiao et al., 2006; Lin et al., 2007; Mu et al., 2012; Zhang et al., 2013; Liu et al., 2014; Vespasiani-Gentilucci et al., 2014; Khosravi et al., 2015; Wei, 2015; Sara et al., 2017; Xie et al., 2019; Liu et al., 2020; Chen et al., 2022; Yan et al., 2023). However, when the liver is damaged, both enzymes can leak into the plasma chamber (Sandnes et al., 1988), thus causing an increase in the concentration levels of the enzymes in the blood (Yan et al., 2023). In this trial, the addition of different levels of AKM significantly decreased the contents of ALT and AST in the liver and serum ALT and AST were also decreased compared with the control group (Yan et al., 2023). Similarly, the results of another study showed that both the ALT and AST contents of Atlantic salmon after dietary supplementation with AKM were within the normal range (Sandnes et al., 1988; Hansen et al., 2010; Mørkøre et al., 2020). The reduced levels of ALT and AST are symbols of good liver and fish health and it shows that AKM can protect hepatocytes from damage.
Lipids are the general term for fats (also known as triglycerides) and lipid metabolism has a crucial impact on animal carcass quality and health (Cheng et al., 2006). In this trial, the serum content of TG and TC was not significantly affected by the addition of AKM up to the inclusion level of 6% but an increase was observed in AKM9 and AKM12, which is consistent with the findings of Kong et al (Kong et al., 2012). Some other studies also demonstrated that TC and TG are vital components for the synthesis of bile, hormones, cell membrane integrity, and vitamin D metabolism (Xie et al., 2020), and they decrease when the liver is not functioning properly (Chen et al., 2021). It has been reported that the addition of AKM results in an increase in TC and TG contents (Wei, 2015; Zhu et al., 2019; Yan et al., 2024), which is similar to our study results. In the studies of Wei et al. and Zhang et al. (Zhang et al., 2013; Wei et al., 2019), it was also observed that the lipid content in the serum increased after adding AKM to the diet. However, during another study in Atlantic salmon, it was reported that the serum TC content in the AKM supplementation group was reduced compared with the control group (Hansen et al., 2010). This was presumed to be a result of the obstruction of TC uptake due to the presence of chitin (Zacour et al., 1992). The reasons for the above different conclusions may be related to the different animal types, inclusion levels, the chitin content, and the breeding environment of the Antarctic krill (Zacour et al., 1992).
Protein is a vital component in the body and optimum concentration of TP in the blood indicates efficient protein utilization from the diet (Hansen et al., 2010; Yan et al., 2023). Certain feeds have greater biological values for certain nutrients and transfer the maximum amount nutrients from the feed to the body, resulting in increased concentrations of total protein and leading to an improvement in the overall immune status and protein synthesis in the body (Coma et al., 1995; Kanjanapruthipong, 1998). AKM addition has be shown to increase the total protein concentration in prawns (Yan et al., 2024), which is in consistent with our findings. Some studies have already reported that the inclusion of AKM has the potential to increase the digestion of protein (Kofuji et al., 2006), thereby increasing TP levels in blood. The higher values of TP may suggest that krill meal diet protein is readily digestible and utilized by salmon (Hansen et al., 2010).
This study demonstrated that dietary Antarctic krill meal supplementation has the potential to be used as a suitable protein feed ingredient and significantly improved the growth performance, liver function, and antioxidant status of coho salmon when partially substituting for fish meal. The performance of post-smolt coho salmon was the highest at the dietary inclusion level of 6% of AKM without any adverse effects. The prediction analysis using polynomial quadratic regression suggested the use of 6.73% to 7.08% AKM for the best performance. However, over-supplementation of AKM may lead to reduced performance.
The original contributions presented in the study are included in the article/Supplementary Material. Further inquiries can be directed to the corresponding authors.
The animal study was approved by the ethics committee for “Guide for the Ethical Use of Experimental Animals” of the Weifang University, Weifang, Shandong, China (No. 20200601). The study was conducted in accordance with the local legislation and institutional requirements.
HY: Conceptualization, Data curation, Methodology, Supervision, Writing – review & editing. LY: Methodology, Supervision, Writing – review & editing. AR: Data curation, Software, Writing – review & editing. SG: Data curation, Formal analysis, Writing – review & editing. YZ: Methodology, Supervision, Writing – original draft. GW: Formal analysis, Software, Validation, Writing – review & editing. LL: Data curation, Formal Analysis, Writing – original draft.
The author(s) declare that financial support was received for the research and/or publication of this article. Funding for this research was provided by the Shandong Provincial Key Research and Development Programs (Major Scientific and Technological Innovation Projects, MSTIP), Grant/Award Numbers: 2018CXGC0102 and 2019JZZY020710; Scientific and Technological Development Program of Weifang, Grant/Award Number: 2019ZJ1046; Shandong Provincial Natural Science Foundation, Grant/Award Number: ZR2020MC174.
Authors AR and SG were employed by the company Conqueren Leading Fresh Science and Technology Inc. Ltd. Author LL was employed by the company Shandong Conqueren Marine Technology Co. Ltd.
The remaining authors declare that the research was conducted in the absence of any commercial or financial relationships that could be construed as a potential conflict of interest.
The author(s) declare that no Generative AI was used in the creation of this manuscript.
All claims expressed in this article are solely those of the authors and do not necessarily represent those of their affiliated organizations, or those of the publisher, the editors and the reviewers. Any product that may be evaluated in this article, or claim that may be made by its manufacturer, is not guaranteed or endorsed by the publisher.
Albrektsen S., Kortet R., Skov P. V., Ytteborg E., Gitlesen S., Kleinegris D., et al. (2022). Future feed resources in sustainable salmonid production: a review. Rev. Aquac, 14 (4), 1790–1812. doi: 10.1111/raq.12673
Anderson J. S., Richardson N. L., Higgs D. A., Dosanjh B. S. (1997). The evaluation of air-dried krill meal as a dietary protein supplement for juvenile Chinook salmon (Oncorhynchus tshawytscha). Can. Tech Rep. Fish Aquac Sci. 17, 17–33.
AOAC (Association of Official Analytical Chemists) (1990). Official methods of analysis. Assoc. Anal. Chem. 62, 2742–2744.
Atkinson A., Siegel V., Pakhomov E., Jessopp M., Loeb V. (2009). A re-appraisal of the total biomass and annual production of Antarctic krill. Deep Sea Res. Part I: Oceanogr Res. Papers 56, 727–740. doi: 10.1016/j.dsr.2008.12.007
Chen S. Y., Lin Y., Miao L. H., Pan W. J., Jiang W. Q., Qian L. J., et al. (2021). Ferulic acid alleviates lipopolysaccharide-induced acute liver injury in Megalobrama amblycephala. Aquaculture 532, 735972. doi: 10.1016/j.aquaculture.2020.735972
Chen S. Y., Lin Y., Shi H. Q., Miao L. H., Liu B., Ge X. P. (2022). Dietary ferulic acid supplementation improved cottonseed meal-based diet utilization by enhancing intestinal physical barrier function and liver antioxidant capacity in grass carp (Ctenopharyngodon Idellus). Front. Physiol. 13. doi: 10.3389/fphys.2022.922037
Cheng H. L., Xia D. Q., Wu T. T. (2006). Fatty liver and regulation of lipids metabolism in fish. Chin. J. Anim. Nutr. 18, 294–298.
Choi J., Lee K. W., Han. G. S., Byun S. G., Lim. H. J., Kim H. S. (2020). Dietary inclusion effect of krill meal and various fish meal sources on growth performance, feed utilization, and plasma chemistry of grower walleye pollock (Gadus chalcogrammus, pallas 1811). Aquac Rep. 17, 10031. doi: 10.1016/j.aqrep.2020.100331
Coma J., Carrion D., Zimmerman D. R. (1995). Use of plasma urea nitrogen as a rapid response criterion to determine the lysine requirement of pigs. J. Anim. Sci. 73, 472–481. doi: 10.2527/1995.732472X
FAO (2024). “In brief to the state of world fisheries and aquaculture 2024,” in Blue transformation in action. (Rome: Food and Agriculture Organization). doi: 10.4060/cd0690en
Gigliotti J. C., Jaczynski J., Tou J. C. (2008). Determination of the nutritional value, protein quality and safety of krill protein concentrate isolated using an isoelectric solubilization/precipitation technique. Food Chem. 111, 209–214. doi: 10.1016/j.foodchem.2008.03.030
Hansen J.Ø., Penn M., Øverland M., Shearer K. D., Krogdahl. Å., Mydland L. T., et al. (2010). High inclusion of partially deshelled and whole krill meals in diets for Atlantic salmon (salmo salar). Aquaculture 310, 164–172. doi: 10.1016/j.aquaculture.2010.10.003
Hansen J.Ø., Shearer K. D., Øverland M., Penn M. H., Krogdahl Å., Mydland L. T., et al. (2011). Replacement of LT fish meal with a mixture of partially deshelled krill meal and pea protein concentrates in diets for Atlantic salmon (Salmo salar). Aquaculture 315, 275–282. doi: 10.1016/j.aquaculture.2011.02.038
Hart J. L. (1973). Pacific fishes of Canada. (Ottawa, Canada: Fisheries Research Board of Canada), 180. 1–740.
Hatlen B., Berge K., Nordrum S., Johnsen K., Kolstad K., Mørkøre T. (2017). The effect of low inclusion levels of Antarctic krill (Euphausia superba) meal on growth performance, apparent digestibility and slaughter quality of Atlantic salmon (Salmo salar). Aquac Nutr. 23, 721–729. doi: 10.1111/anu.12439
Jahn F., Lukowicz M., Lo¨rz R. (1978). Erfahrungen mit Krillmehl im Forellenfutter. Fisch Teichwirt 29, 67–68.
Julshamn K., Malde M. K., Bjorvatn K., Krogedal P. (2004). Fluoride retention of Atlantic salmon (Salmo salar) fed krill meal. Aquac Nutr. 10, 9–13. doi: 10.1046/j.1365-2095.2003.00273.x
Kanjanapruthipong J. (1998). Supplementation of milk replacers containing soy protein with threonine, methionine, and lysine in the diets of calves. J. Dairy Sci. 81, 2912–2915. doi: 10.3168/jds.S0022-0302(98)75852-7
Khosravi S., Kim E., Lee Y., Lee S. (2018). Dietary inclusion of mealworm (Tenebrio molitor) meal as an alternative protein source in practical diets for juvenile rockfish (Sebastes schlegeli). Entomol Res. 48, 214–221. doi: 10.1111/enr.2018.48.issue-3
Khosravi S., Rahimnejad S., Herault M., Fournier V., Lee C. R., Bui H. T. D., et al. (2015). Effects of protein hydrolysates supplementation in low-fish meal diets on growth, innate immunity and disease resistance of red sea bream pagrus major. Fish Shellfish Immunol. 45, 858–868. doi: 10.1016/j.fsi.2015.05.039
Kiranpreet K., Trond M. K., Tibiabin B. S., Lena B. (2022). Effects of antarctic krill products on feed intake, growth performance, fillet quality, and health in salmonids. Aquac Nutr., 3170854. doi: 10.1155/2022/3170854
Kofuji M. P. Y., Hosokawa H., Masumoto T. (2006). Effects of dietary supplementation with feeding stimulants on yellowtail Seriola quinqueradiata (Temminck & Schlegel; Carangidae) protein digestion at low water temperatures. Aquac Res. 37, 366–373. doi: 10.1111/j.1365-2109.2005.01435.x
Kong F. H., Liang M. Q., Wu L. X., Zheng K. K., Wang X. X., Chang Q., et al. (2012). Effects of different level of Antarctic krill meal on the growth, non-specific immunity and fluorine retention in diets of turbot Scophthalmus maximus. Adv. Fish Sci. 33, 54–60. doi: 10.3969/j.issn.1000-7075.2012.01.008
Kubitza F., Lovshin L. L. (1997). Effects of initial weight and genetic strain on feed training largemouth bass Micropterus salmoides using ground fish flesh and freeze dried krill as starter diets. Aquaculture 148, 179–190. doi: 10.1016/S0044-8486(96)01419-6
Lin S. M., Mai K. S., Tan B. P. (2007). Effect of soybean meal replacement by rapeseed cottonseed compound on growth, body composition and immunity of Tilapia Oreochromis niloticus x O. Aureus Oceanologia Limnol Sin. 38, 168–173. doi: 10.1016/j.aquaculture.2008.07.030
Liu L., Chen Y. F., Yang L. (2014). Inhibition study of alanine aminotransferase enzyme using sequential online capillary electrophoresis analysis. Anal Biochem. 467, 28–30. doi: 10.1016/j.ab.2014.08.035
Liu J.-D., Wen-Bin L., Cai-Yan Z., Chen-Yuan X., Zheng X.-C., Zhang D.-D., et al. (2020). Dietary glutathione supplementation enhances antioxidant activity and protects against lipopolysaccharide-induced acute hepatopancreatic injury and cell apoptosis in Chinese mitten crab, Eriocheir sinensis. Fish Shellfish Immunol. 97, 440–454. doi: 10.1016/j.fsi.2019.12.049
Lu H. F. S., Bruheim I., Jacobsen C. (2014). Oxidative stability and nonenzymatic browning reactions in Antarctic krill oil (Euphausia superba). Lipid Tech 26, 111–114. doi: 10.1002/lite.201400027
Ma Q. L., Teter B., Ubeda O. J., Morihara T., Dhoot D., Nyby M. D., et al. (2007). Omega-3 fatty acid docosahexaenoic acid increases SorLA/LR11, a sorting protein with reduced expression in sporadic Alzheimer’s disease (AD): Relevance to AD prevention. J. Neurosci. 27, 14299–14307. doi: 10.1523/jneurosci.3593-07.2007
Mørkøre T., Moreno HM., Borderías J., Larsson T., Hellberg H., Hatlen B., et al. (2020). Dietary inclusion of Antarctic krill meal during the finishing feed period improves health and fillet quality of Atlantic salmon (Salmo salar L.). Br. J. Nutr. 124, 418–431. doi: 10.1017/S0007114520001282
Mu X. Y., Qi L., Qiao J., Zhang H. Z., Ma H. M. (2012). Study on alanine aminotransferase kinetics by microchip electrophoresis. Anal Biochem. 421, 499–505. doi: 10.1016/j.ab.201111.037
Nyblom H., Berggren U., Balldin J., Olsson R. (2004). High AST/ALT ratio may indicate advanced alcoholic liver disease rather than heavy drinking. Alcohol AUD 39, 336–339. doi: 10.1093/alcalc/agh074
Oikawa C. K., March B. E. (1997). Communications: a method for assessment of the efficacy of feed attractants for fish. Prog Fish-Cult 59, 213–217. doi: 10.1577/1548-8640(1997)059<0213:CAMFAO>2.3.CO;2
Olsen R. E., Suontama J., Langmyhr E., Mundheim H., Ringø E., Melle W., et al. (2006). The replacement of fish meal with Antarctic krill, Euphausia superba in diets. Aquac Nutr. 12, 280–290. doi: 10.1111/j.1365-2095.2006.00400.x
Palliyeguru M. W., Rose S. P., Mackenzie A. M. (2010). Effect of dietary protein concentrates on the incidence of subclinical necrotic enteritis and growth performance of broiler chickens. Poult Sci. 89, 34–43. doi: 10.3382/ps.2009-00105
Papuktchieva T., Tomassian H., Zaikov A., Gadjeva M., Simovska M., Ribarov Z. (1981). Testing of combined granules, containing krill meal, for feeding carp and trout for consumption. Proc. Freshw. Fish Res. Inst. Plovdiv 15, 79–107.
Pfeffer E., Becker K. (1977). Untersuchungen an Regenbogenforellen u¨ber den Futterwert verschiedener Handelsfu¨tter und u¨ber den weitgehenden Ersatz von Fishmehl durch Krillmehl im Futter. Arch. Fischereiwiss 28, 19–29.
Rødde R. H., Einbu A., Vårum. K. M. (2008). A seasonal study of the chemical composition and chitin quality of shrimp shells obtained from northern shrimp (Pandalus borealis). Carbohydr Polym 71, 388–393. doi: 10.1016/j.carbpol.2007.06.006
Roncarati A., Sirri F., Felici A., Stocchi L., Melotti P., Meluzzi A. (2011). Effects of dietary supplementation with krill meal on pigmentation and quality of flesh of rainbow trout (Oncorhynchus mykiss). Ital. J. Anim. Sci 10, 2. doi: 10.4081/ijas.2011.e27
Saleh R., Burri L., Benitez-Santana T., Turkmen S., Castro P., Izquierdo M. (2018). Dietary krill meal inclusion contributes to better growth performance of gilthead seabream juveniles. Aquac Res. 49, 3289–3295. doi: 10.1111/are.2018.49.issue-10
Sandnes K., Lie Ø., Waagbø R. (1988). Normal ranges of someblood chemistry parameters in adult farmed Atlantic salmon, Salmo salar. Fish Biol. 32, 129–136. doi: 10.1111/j.1095-8649.1988.tb05341.x
Sara Z., Massoud M. A. B., Massoud A. (2017). Inhibition of liver alanine aminotransferase and aspartate aminotransferase by hesperidin and its aglycone hesperetin:An in vitro and in silico study. Life Sci. 178, 49–55. doi: 10.1016/j.lfs.2017.04.001
Sun C. H. (2019). Research on the main biological characteristics of Antarctic krill oil and its mechanism of blood lipid lowering (Beijing, China: China Agricultural University).
Suontama J., Karlsen Ø., Moren M., Hemre G. I., Melle W., Langmyhr E., et al. (2007). Growth, feed conversion and chemical composition of Atlantic salmon (Salmo salar L.) and Atlantic halibut (Hippoglossus hippoglossus L.) fed diets supplemented with krill or amphipods. Aquac Nutr. 13, 241–255. doi: 10.1111/j.1365-2095.2007.00466.x
Swanson D., Block R., Mousa S. A. (2012). Omega-3 fatty acids EPA and DHA: Health benefits throughout life. Adv. Nutr. 1, 1–7. doi: 10.3945/an.111.000893
Tibbetts S. M., Olsen R. E., Lall S. P. (2011). Effects of partial or total replacement of fish meal with freeze-dried krill (Euphausia superba) on growth and nutrient utilization of juvenile Atlantic cod (Gadus morhua) and Atlantic halibut (Hippoglossus hippoglossus) fed the same practical diets. Aquac Nutr. 17, 287–303. doi: 10.1111/j.1365-2095.2010.00753.x
Tinsley I. J., Krueger H. M., Saddler J. B. (1973). Fatty acid content of coho salmon,Oncorhynchus kisutch— a statistical approach to changes produced by diet. J. Fish Board Canada 30, 1661–1666. doi: 10.1139/f73-266
Torrecillas S., Montero D., Carvalho M., Benitez-Santana T., Izquierdo M. (2021). Replacement of fish meal by Antarctic krill meal in diets for European sea bass Dicentrarchus labrax: Growth performance, feed utilization and liver lipid metabolism. Aquaculture 545, 737166. doi: 10.1016/j.aquaculture.2021.737166
Venditti P., Di Meo S. (2006). Thyroid hormone-induced oxidative stress. Cell. Mol. Life Sci. 63, 414–434. doi: 10.1007/s00018-005-5457-9
Vens-Capell B., Horstmann H. J. (1978). Untersuchungenu¨ber die Eignung des Krillmehls als Ersatz fu¨r Fischmehl im Trockenmischfutter fu¨r Forellen. O¨sterr Fisch 31, 35–46.
Vespasiani-Gentilucci U., Gallo P., Piccinocchi G., Piccinocchi R., Schena E., Galati G., et al. (2014). Determinants of alanine aminotransferase levels in a large population from Southern Italy: Relationship between alanine aminotransferase and age. Dig Liver Dis. 46, 909–915. doi: 10.1016/j.dld.2014.05.021
Waagbø R., Torrissen. O. J., Austreng E. (2001). “Fôr og fôrmidlerden største utfordringen for vekst i norsk havbruk,” in Et utredningsarbeid utført for Norge Forskningsråd, Bioproduksjon og foredling, Havbrukssprogrammet (in Norwegian)(Oslo, Norway).
Wang X. Y. (2020). Effect of Antarctic krill powder on the growth performance, protein metabolism and antioxidant capacity of young Chinese crab crabs (Shanghai, China: East China Normal University).
Wang Y. Y., Zhao L., Guo X. H., Zhang X. M. (2021). Nutritional characteristics of antarctic krill meal and its application in aquatic feed. Chin. J. Anim. Nutr. 33, 6601–6611. doi: 10.3969/j.issn.1006-267x.2021.12.001
Wei J. L. (2015). Application effects of krill meal in feeds for juvenile starry flounder (Platichthys stellatus) and pearl gentian grouper (♀ Epinephelus fuscoguttatus×♂ Epinephelus lanceolatu) (China: Shanghai Ocean University). Available at: https://kns.cnki.net/kcms/detail/detail.aspx?FileName=1015974728.nh&DbName=CMFD2016 (Accessed December 30, 2024).
Wei Y. T., Shen H. H., Xu W. Q., Pan Y., Chen J., Zhang W. B., et al. (2019). Replacement of dietary fishmeal by Antarctic krill meal on growth performance, intestinal morphology, body composition and organoleptic quality of large yellow croaker Larimichthys crocea. Aquaculture 512, 734281. doi: 10.1016/j.aquaculture.2019.734281
Wei Y., Xiaoxia W., Fangli X., Haohao S., Weihua G., Wenbing Z., et al. (2022). Influences of replacing dietary fish meal by Antarctic krill meal on growth performance, immunity and muscle quality of white shrimp Litopenaeus vannamei. Aquac Rep. 25, 101256. doi: 10.1016/j.aqrep.2022.101256
Williams K. C., David M. S., Margaret C. B., Simon J. T., George R. (2005). Evidence of a growth factor in some crustacean-based feed ingredients in diets for the giant tiger shrimp Penaeus monodon. Aquaculture 250, 377–390. doi: 10.1016/j.aquaculture.2005.04.002
Xiao Y. X., Li J. L., Xu S. W. (2006). Progress in studying the effects of cadmium, selenium, fluorine, copper, zinc, and phosphorus on cell membranes. Chin. J. Loc Path 25, 225–226. doi: 10.3760/cma.j.issn.1000-4955.2006.02.047
Xie D., Gong M. Y., Wei W., Jin J., Wang X. S., Wang X. G., et al. (2019). Antarctic krill (Euphausia superba) oil: a comprehensive review of chemical composition, extraction technologies, health benefits, and current applications. Compr. Rev. Food Sci. Food Safety 18, 514–534. doi: 10.1111/1541-4337.12427
Xie K., Shi Y., He H., Ma X. K., Li Z. L., Zhong L., et al. (2022). Effect of Antarctic krill powder on replacing fish meal on the growth, immunity and muscle quality of yellow catfish. J. Fish 46, 410–419.
Xie S., Yin P., Tian L., Liu Y., Niu J. (2020). Lipid metabolism and plasma metabolomics of juvenile largemouth bass Micropterus salmoides were affected by dietary oxidized fish oil. Aquaculture 522, 735158. doi: 10.1016/j.aquaculture.2020.735158
Xu B. B., Jiang X. M., Xue Y. (2019). Nutritional value evaluation of proteins in different Antarctic krill products. Food Ind Tech 40, 17–23, 30. doi: 10.13386/j.issn1002-0306.2019.23.003
Xu H. G., Zhao M., Zheng K. K., Wei Y. L., Yan L., Liang M. Q. (2016). Antarctic krill (Euphausia superba) meal in the diets improved the reproductive performance of tongue sole (Cynoglossus semilaevis) broodstock. Aquac Nutr. 23, 1287–1295. doi: 10.1111/anu.12503
Yan J. L., Chen S. Q., Chang Q., Wang Z. J., Lu B., Liu C. L., et al. (2018). Effect of dietary Antarctic krill meal on growth performance, muscle proximate composition, and antioxidative capacity of juvenile spotted halibut, Verasper variegatus. J. World Aquac Soc 49, 761–769. doi: 10.1111/jwas.12455
Yan Y., Lin Y., Gu Z., Lu S., Zhou Q., Zhao Y., et al. (2024). Dietary fishmeal substitution with Antarctic krill meal improves the growth performance, lipid metabolism, and health status of oriental river prawn (Macrobrachium nipponense). Aquac Rep. 37. doi: 10.1016/j.aqrep.2024.102202
Yan Y., Lin Y., Zhang L., Gao G., Chen S., Chi C., et al. (2023). Dietary supplementation with fermented antarctic krill shell improved the growth performance, digestive and antioxidant capability of Macrobrachium nipponense. Aquac Rep 30, 101587. doi: 10.1016/j.aqrep.2023.101587
Yoshitomi B., Aoki M., Oshima S. I., Hata K. (2006). Evaluation of krill (Euphausia superba) meal as a partial replacement for fish meal in rainbow trout (Oncorhynchus mykiss) diets. Aquaculture 261, 440–446. doi: 10.1016/j.aquaculture.2006.06.036
Yu H.-R., Guo M.-J., Yu L.-Y., Li L.-Y., Wang Q.-H., Li F.-H., et al. (2022). Effects of dietary riboflavin supplementation on the growth performance, body composition and anti-oxidative capacity of coho salmon (Oncorhynchus kisutch) post-smolts. Animals 12, 3218. doi: 10.3390/ani12223218
Zacour A. C., Silva M. E., Cecon P. R., Bambirra E. A., Vieira E. C. (1992). Effect of dietary chitin on cholesterol absorption and metabolism in rats. J. Nutr. Sci. Vitaminol 38, 609. doi: 10.3177/jnsv.38.609
Zhang Z., Lu M., Chen C., Tong X., Li Y., Yang K., et al. (2021). Holo lactoferrin: the link between ferroptosis and radiotherapy in triple-negative breast cancer. Theranostics 11, 3167–3182. doi: 10.7150/thno.52028
Zhang L., Lu X. Q., Wang Z. M., Qin L. Q., Yuan L. X., Yin X. B. (2013). Evaluation of the toxicity of fluorine in antarctic krill on soft tissues of wistar rats. Adv. Polar Sci 24, 128–132. doi: 10.3724/SP.J.1084.2013.00078
Zhu W. M., Tan Y. G., Ma H. J., Chen Y. M., Lan H. B., Lin S. M. (2019). Effect of different levels of Antarctic krill powder replacing fish meal on the growth performance and some physiological and biochemical indicators of largemouth sea bass. Chin. Feed 15, 85–92. doi: 10.15906/j.cnki.cn11-2975/s.20191520
Keywords: sustainable feed, alternative solutions, fish nutrition, health promoters, non-conventional feed ingredients
Citation: Yu H, Yu L, Rahman A, Govindharajan S, Zhang Y, Wang G and Li L (2025) Impact of dietary supplementation with Antarctic krill meal on growth performance, biochemical parameters, and antioxidant status in Coho Salmon (Oncorhynchus kisutch) post-smolts. Front. Mar. Sci. 12:1553629. doi: 10.3389/fmars.2025.1553629
Received: 31 December 2024; Accepted: 17 March 2025;
Published: 11 April 2025.
Edited by:
Yngvar Olsen, Norwegian University of Science and Technology, NorwayReviewed by:
Yun Wang, Chinese Academy of Fishery Sciences (CAFS), ChinaCopyright © 2025 Yu, Yu, Rahman, Govindharajan, Zhang, Wang and Li. This is an open-access article distributed under the terms of the Creative Commons Attribution License (CC BY). The use, distribution or reproduction in other forums is permitted, provided the original author(s) and the copyright owner(s) are credited and that the original publication in this journal is cited, in accordance with accepted academic practice. No use, distribution or reproduction is permitted which does not comply with these terms.
*Correspondence: Hairui Yu, eWhyNjAwM0Bob3RtYWlsLmNvbQ==; Abdur Rahman, YWJkdXJyZWhtYW5AdXZhcy5lZHUucGs=
Disclaimer: All claims expressed in this article are solely those of the authors and do not necessarily represent those of their affiliated organizations, or those of the publisher, the editors and the reviewers. Any product that may be evaluated in this article or claim that may be made by its manufacturer is not guaranteed or endorsed by the publisher.
Research integrity at Frontiers
Learn more about the work of our research integrity team to safeguard the quality of each article we publish.