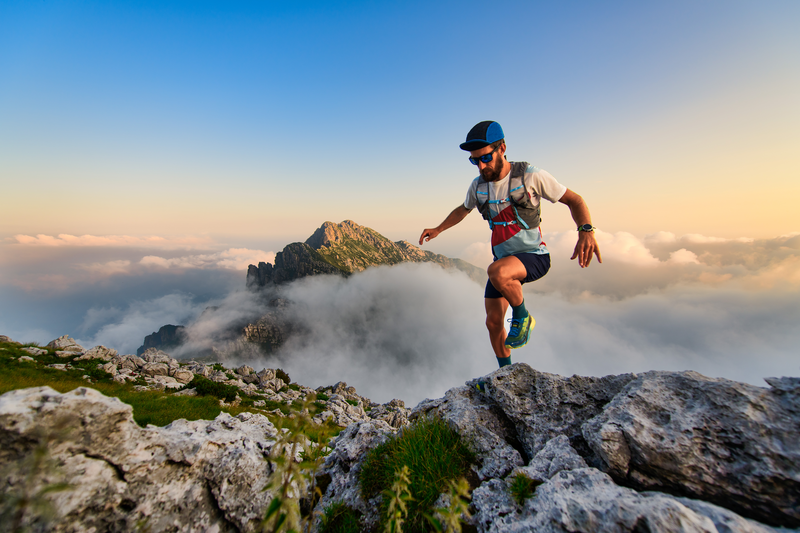
94% of researchers rate our articles as excellent or good
Learn more about the work of our research integrity team to safeguard the quality of each article we publish.
Find out more
ORIGINAL RESEARCH article
Front. Mar. Sci. , 26 March 2025
Sec. Marine Ecosystem Ecology
Volume 12 - 2025 | https://doi.org/10.3389/fmars.2025.1553583
This article is part of the Research Topic Turning with the Tide and Time in the Salish Sea: Change in Estuary and Nearshore Habitats and Species Dependent on Them View all 9 articles
While conducting early detection monitoring for invasive European green crab (Carcinus maenas), Washington Sea Grant Crab Team discovered a non-native shrimp Palaemon macrodactylus, hitherto unreported along Washington’s portion of the Salish Sea. By examining prior data, and tracking this species closely over subsequent years, we were able to consistently monitor the spread and increase of this species across the region. The program and dataset enabled a highly quality-controlled and verified record of this species. Originating near the Canadian border, P. macrodactylus has spread southward into the main basins of Puget Sound and has been observed in Hood Canal as well. At sites where this shrimp has been consistently documented, the relative abundance over time has increased, indicating that the invasion has become established but not yet reached equilibrium. Similar to studies in other regions of the globe where it is found, we observed that P. macrodactylus favors estuarine habitats and demonstrates seasonal migration within creek systems. The Salish Sea population is within demographic values published for other invasions, but females achieve a smaller maximum size, and reproductive maturity at a smaller size than those reported from the native range. This might suggest the potential for an altered life history strategy favored by introduction to a novel evolutionary context. Given the rate of spread over the last decade, and density of suitable habitat, we anticipate that P. macrodactylus will continue to expand its range within the Salish Sea. No ecological impacts of this species have been documented elsewhere. Nevertheless, this approach demonstrates the benefit of participatory science monitoring in tracking cryptic or otherwise unnoticed species invasions.
Tracking a biological invasion or range expansion in progress is often challenged by lack of resources for monitoring sufficient in scope to track rare and stochastic encounters. This limitation is even more difficult when a non-native species is not perceived to pose a substantial risk, either of spread or of impact. This means that the invasions of all but a few species are relatively poorly documented in real time or at the early stages of invasion/expansion.
Participatory science programs can often offer the opportunity to conduct monitoring on a broader scale, and for longer time spans, than single research projects afford (Tulloch et al., 2013; Likens and Lindenmayer, 2018). This modality is commonly used for early detection of invasive species (Crall et al., 2010; Encarnação et al., 2021), but many such programs focus data collection only on the target species (e.g., Delaney et al., 2008) presumably to minimize the demand on the participants and, in turn, overhead of the program. Unfortunately, this strategy can miss the opportunity to leverage the efforts of participants to detect and track changes in the ecological community or habitats being surveyed (Wintle et al., 2010; Tulloch et al., 2013). This could include changes caused by the invasive species, “surprise” pulse disturbances related to natural disasters or climate events, the addition of other non-native species or decline of native populations.
We document and characterize the first reports of the globally invasive palaemonid shrimp, Palaemon macrodactylus, into the Washington portion of the Salish Sea, through a participatory science monitoring network, Washington Sea Grant Crab Team. P. macrodactylus, native to the northwestern Pacific Ocean, has established populations in several temperate estuarine regions worldwide, including the northeastern Pacific (Newman, 1963), as well as the northwestern (Warkentine and Rachlin, 2010), southwestern (Spivak et al., 2006), and northeastern (Cuesta et al., 2004) Atlantic oceans. Detection in 1957 in San Francisco Bay marked the first occurrence of P. macrodactylus outside the native range, and introduction is supposed to have occurred a few years prior, likely resulting from transport from the native range in the fouled seawater systems of naval vessels returning to Alameda from the Korean War (Newman, 1963). Subsequent regional spread of P. macrodactylus likely has been mediated both by unassisted larval dispersal, as well as human-vectored transport (Carlton, 1985; Ashelby et al., 2013). The first report of P. macrodactylus in Washington State came from Willapa Bay, a shallow coastal estuary (Jensen, 1995), but the species was not found in an extensive survey of non-native species in Puget Sound conducted around that time (Cohen et al., 1998). Subsequently, two reports have been made from British Columbia, in the Canadian portion of the Salish Sea: Boundary Bay at the Nicomekl River, directly adjacent to the United States Border (Lamb and Hanby, 2005), and in Victoria on Vancouver Island (Jensen, 2014). But to date, there are no published reports of P. macrodactylus within the Washington portion of the Salish Sea. The shrimp is not included on the Washington Invasive Species Council’s list of 50 priority invasive animals or in the USGS AIS database.
The Crab Team monitoring network was created to detect the early arrival and establishment phases of invasive European green crab (Carcinus maenas, hereafter referred to as green crab) in the Washington portion of the Salish Sea. Green crab is a level 1 (highest priority as established by the state) prohibited species in Washington State and monitoring was established prior to any confirmed detections in the region (Grason et al., 2018). Crab Team monitoring protocols consist of three surveys including an overnight set of baited traps. Because monitors record every organism in the trap, the surveys characterize a broad swath of the community of nearshore mobile epifauna, including crabs, fishes, gastropods, and shrimps. While initially designed to enable a before-after control-impact (BACI) sampling design focused on impacts and habitat associations of green crab, by recording all species in traps, Crab Team monitoring also enables investigation of other phenomena unrelated to green crab, including habitat restoration, extreme events, and community change.
In 2020, P. macrodactylus was discovered for the first time to have been captured in a Crab Team trapping survey at one monitoring site, Drayton Harbor, near the border between the United States and Canada (Figure 1). In this paper, we leverage the Crab Team dataset to assess the presence, abundance, spread and seasonality of P. macrodactylus within the Salish Sea, including reexamining data from years prior to the discovery.
Figure 1. Study region overview map, including P. macrodactylus detections prior to this study (black triangles, with date of publication) and Crab Team sampling sites 2015 - 2024 (circles). Sites where P. macrodactylus has not been found are white circles, black circles, labeled with site names, indicate sites where P. macrodactylus has been captured during regular monitoring.
We used data from three main sources to describe the spread of Palaemon macrodactylus in within the Washington portion of the Salish Sea (Puget Sound, the Strait of Juan de Fuca and the San Juan Islands):
1. To assess distribution, relative abundance, and seasonality at the regional scale, we investigated the existing participatory science dataset from Washington Sea Grant Crab Team.
2. To explore local site- and habitat-level distribution, and seasonal estuarine migration, we drew from capture data opportunistically collected as part of a local green crab trapping effort in Drayton Harbor.
3. To characterize population demographics, we collected specimens from both of the above trapping efforts, along with additional opportunistic and targeted efforts.
Crab Team manages a statewide network of early detection and monitoring sites for invasive European green crab (Carcinus maenas) (Figure 1). The network presently consists of 68 active sites, but since launching in 2015, a total of 75 sites has been sampled at least once as part of the program. At each site, trained volunteers and technical staff from partner agencies and tribes implement a standardized baited trapping protocol monthly from April through September annually. While the baited trapping protocol does not target P. macrodactylus directly, drawing on this consistent dataset enabled detection at multiple sites and comparison across the region and over a decade.
Sites were selected for inclusion in the monitoring network based primarily on suitability of the habitat for green crabs at the early stages of invasion. Observations of green crab habitat use on the U.S. West Coast (Grosholz and Ruiz, 1996) suggest that green crabs perform best in low energy, soft-sediment, estuarine shorelines. Sites fell into three categories: pocket estuary lagoons, salt marsh channel, and muddy tide flats. Pocket estuary lagoons (n = 30) were characterized by shallow depth of an impounded water body, connected to open marine waters on only high tides, and might or might not have additional freshwater input, e.g., a small stream. Salt marsh channels (n = 32) were relatively narrow, linear waterways with vertical banks, bordered on both sides by salt marsh habitat, including halophytic vegetation (e.g., Salicornia). Channels generally were selected which retained some water, at least in pools, at low tide to reduce stress on captured animals. Tide flats (n = 13) were the least common site across the monitoring network, and, in accordance with the goal to prioritize habitat most suitable for green crab, targeted an intertidal elevation range of approximately +1-2 m relative to mean lower-low water (MLLW), and adjacent to physical structure such as pilings, riprap, or pedestal marsh. Particularly relevant for this study, at the site level, freshwater influence varied significantly across the sites in the network.
A total of 75 sites were sampled with baited traps at least once, but up to 58 times, between August 2015 and September 2024 (Figure 1). As part of the standardized early detection trapping protocols (Grason et al., 2018, and updated annually thereafter), monitors used three traps of two types, a Gee-40 galvanized steel minnow trap (ca. 6.35mm mesh size, 25mm opening), and a square Fukui-style folding fish trap (FT-100, 13mm mesh, with a horizontal slit entrance 40cm wide). The Fukui traps were modified using a cable tie to connect the top and bottom of the ramp openings at the middle, reducing the width of the entrance (to two 20cm wide segments) in order to reduce capture of large non-target animals that experience high mortality rates in traps. For each monthly survey, traps were baited with ca. 150-175g of Pacific mackerel (Scomber japonicus) and arranged in a transect parallel to shore (for tideflat and lagoon sites) or parallel to the channel axis (for saltmarsh channel sites), alternating by trap type, for an overnight high-tide soak, approximately 16-22 hours depending on trap elevation.
The number of individuals for all taxa captured in traps was recorded according to Crab Team’s standard taxonomic resolution. Most taxa are identified to species, but some that are more difficult to distinguish are grouped to genus or family level. Surveyors also submit photographs of trap contents, which are reviewed by program staff to verify species identification and data quality.
P. macrodactylus was initially discovered opportunistically in a data submission during the summer of 2020, originally misreported as a native shrimp (Family Pandalidae or Hippolytidae, but later identified to species through review of trap photos (G. Jensen, personal communication, August 6, 2020). We subsequently conducted a systematic review of all trapping data collected since the initiation of the program in August 2015 for other possible misidentifications. In total, 1,084 previous trapping survey efforts were reviewed across 59 sites, and we reevaluated trap photographs for all surveys that reported any shrimp taxa (n = 152 records). Photographs were ranked on a scale of 1 - 5 based on the level of confidence that shrimp could be positively identified as P. macrodactylus:
1. High confidence that shrimp in photograph was a species other than P. macrodactylus.
2. Medium confidence that shrimp in photograph was species other than P. macrodactylus.
3. Unable to distinguish any identifying features on shrimp in image due to poor image quality.
4. Medium confidence that shrimp in photograph could be positively identified as P. macrodactylus.
5. High confidence that shrimp in photograph could be identified as P. macrodactylus supported by visibility of multiple confirmatory features.
In photographs, P. macrodactylus was distinguished from Cragonid shrimp in that the latter are dorso-ventrally compressed, have sub-chelate claws, and have a narrow last abdominal segment relative to the width of the uropods when viewed dorsally (as was the most common presentation in photographs). Additionally, P. macrodactylus differed from hippolytid and pandalid shrimp also captured in traps in that the former had first antennae that were long and bifurcated, and second antennae longer than the length of the body.
Only those photographs for which shrimp could be identified as P. macrodactylus with a high degree of confidence (rated 5 on our scale) were included in this report.
Concurrent with retrospective data review, Crab Team implemented training and identification resources for monitors targeting P. macrodactylus to enable ongoing collection of species-level data, including retention of all specimens for species verification. Notably, no shrimp identified by monitors and confirmed from photos by staff to be P. macrodactylus through the standard photographic QA/QC process (Grason et al., 2018) was subsequently reclassified as another species as a result of specimen evaluation. Therefore, while it is possible that some P. macrodactylus were missed from historical photos due to image quality, we are confident that P. macrodactylus confirmed from historic photos are correctly identified.
To explore site-level distribution and habitat use, we analyzed a dataset from green crab trapping collected by Washington Department of Fish and Wildlife (WDFW) and Northwest Straits Commission (NWSC) from 2020 - 2022 in Drayton Harbor. This was the location of the initial detection of P. macrodactylus within the Crab Team dataset, and the northernmost monitoring site within the Crab Team network. Within Drayton Harbor, Crab Team monitoring occurs only in a 50m stretch at the mouth of one of two tidal creek systems, California Creek. WDFW and NWSC agency staff conduct control trapping for green crab broadly throughout the Harbor, across a range of depths and habitat types, collecting data on P. macrodactylus among other bycatch appearing in traps. Like Crab Team monitoring, these efforts utilized minnow and Fukui traps, typically in alternating arrangement, with the difference that the opening diameter of the minnow traps was larger than those used for Crab Team monitoring (ca. 50mm).
Additionally, in 2021, both creek systems in Drayton Harbor were trapped with seasonal index sites twice per month from April through September. On each creek, three index sites spanned the estuarine gradient extending upstream from the mouth. Sites were sampled similar to Crab Team monitoring, with three minnow (with a large opening, as above) and three Fukui traps baited with mackerel for a single overnight soak.
To further characterize the population of P. macrodactylus at multiple sites where it was detected (Figure 1), we collected specimens in several ways:
1. A synoptic sampling event across three sites (Drayton Harbor, Post Point, and Davis Slough; Figure 1) was conducted 16 September 2020. P. macrodactylus were captured opportunistically with dip nets from shoreline on a low tide (n = 124).
2. We aggregated specimens that were opportunistically captured by agency staff during trapping efforts for green crab from three water bodies (Drayton Harbor, Samish Bay, and Padilla Bay) during summer 2020 - 2022 (n = 316).
3. Crab Team network monitors retained P. macrodactylus captured during trapping surveys. Staff retrieved specimens for identity verification and measurement (n = 313).
All specimens (n = 753) were returned to the lab and frozen. Shrimp species identity, sex, and ovigery was confirmed using a dissecting scope, and size was measured using image analysis (ImageJ, Schneider et al., 2012). In determining species identity of specimens, crangonid shrimp could be quickly ruled out based on gross morphology; the body shape of P. macrodactylus shows strong lateral compression relative to the crangonids. Close examination of the first and second pereiopods enabled us to rule out species from other local groups, including Hippolytidae and Pandalidae. The first pereiopod is chelate only in P. macrodactylus and among Hippolytidae, but smaller than the chela of the second pereiopod in P. macrodactylus, and larger than the chela of the second pereiopod in Hippolytidae. The number of articulations in the carpus of the second pereiopod also differed across the three groups observed: P. macrodactylus lacks articulations, while local species of Hippolytidae (3-7) and Pandalidae(20 or more) possess articulations on this portion of the appendage.
Lateral photographs of thawed individual shrimp were taken with a DSLR camera and scale bar, attempting to position the shrimp with abdomen extended for consistent measurements. Because several metrics of shrimp size have been utilized by researchers, and were not always precisely described, P. macrodactylus specimens were measured using three metrics (Supplementary Figure S1):
1. Carapace length (CL): measured from the posterior orbital margin to the dorsal ridge on the posterior margin of the carapace as a single line segment.
2. Total length (TL): measured from the anterior tip of the rostrum to the posterior tip of the telson. Due to the curvature of the shrimp, this was always measured in three line segments with the following landmark points (anterior to posterior): a) tip of the rostrum, b) dorsal ridge on the posterior margin of the carapace, c) apex of the dorsal ridge on the third abdominal segment (i.e., pleon), and d) distal end of the posterior spine of the telson.
3. Vázquez total length (VTL): From Vázquez et al. (2012), this metric measures from the posterior orbital margin to the tip of the telson, with three line segments: a) Posterior orbital margin, b) dorsal ridge on the posterior margin of the carapace, c) apex of the dorsal ridge on the third abdominal segment, and, d) distal end of the posterior spine of the telson.
Metrics that include the rostrum and abdomen may be subject to error, due to vulnerability to damage in the former, and due to variability in shrimp positioning for the latter. However, measurements that include the abdomen and rostrum might better account for allometric differences between the sexes. Moreover, converting across metrics enables comparison across previous studies. We calculated regressions for each two-way comparison of size metrics (Supplementary Figure S2, Supplementary Table S1), but use carapace length as the most robust metric as it was the one most frequently measurable on our specimens.
Of the 152 records evaluated, only 19 (12.5%) were unable to be assigned (rating of 3) due to poor visibility of the target specimens. About a third of the total records assessed were identified as likely P. macrodactylus with either medium (8 records) or high (44 records) confidence. The small fraction of records (5%) assessed as P. macrodactylus with only medium confidence (a rating of 4) was not reassigned from the original identification by monitors. It is notable, however, that all but a single record in this category came from sites and years where P. macrodactylus have been confidently confirmed from both photos and specimens (Post Point, Davis Slough, and Shore Trail). Thus, the relative abundances reported at these sites might be slight underestimates. The other record of medium confidence occurred at Dungeness Spit Base Lagoon, a waterbody where P. macrodactylus has never otherwise been confirmed prior or since. The majority of shrimp captured that we could identify as a species other than P. macrodactylus with either medium (14 records) or high (67 records) confidence fell into two taxonomic groups: sand shrimps (Crangonidae) and broken back shrimps (Pandalidae or Hippolytidaeae).
Review of photographs prior to 2020 revealed P. macrodactylus had been captured at three monitoring sites within the Crab Team network dating back as early as 2016 (Figure 2, Table 1). The majority of observations occurred at the northernmost two sites in the network, Drayton Harbor, which is directly adjacent to Boundary Bay, B.C., and Post Point, in Bellingham Bay. At the third site, Davis Slough, P. macrodactylus was first captured in 2018. Notably, this site is 55 km to the south of Post Point, with a dozen network monitoring sites between where no detections had been made.
Figure 2. Site maps showing annual sampling sites and P. macrodactylus capture rate (standardized as number of shrimp per 100 trap sets).
Table 1. Characteristics and location of Crab Team monitoring sites where P. macrodactylus has been captured and confirmed at least once since 2015.
Subsequently, P. macrodactylus has been captured at additional monitoring sites, including several sites between Post Point and Davis Slough, in Samish, Padilla, and Fidalgo Bays (Table 1; Figures 3, 4). Additionally, in 2021, P. macrodactylus was captured for the first time well into central Puget Sound at Best Lagoon, nearly 100 km south of the nearest detections.
Figure 3. Annual relative abundance of P. macrodactylus (standardized as number of shrimp per 100 trap sets) in monitoring trapping across Crab Team network. Relative abundance (CPUE) has been log transformed to enable comparisons across sites with a wide range of observed capture rates.
Figure 4. Map of Drayton Harbor site, showing extent of all trapping (white circles, with total effort emphasized with a heatmap), and P. macrodactylus captures (red circles) from 2021-2022. Basemap aerial imagery via QGIS (v 3.36.1 Maidenhead) and QuickMapServices plug-in.
At sites where P. macrodactylus is most consistently captured, abundance, as estimated by catch per unit effort, has generally increased over time (Figure 3). One notable exception is Davis Slough, at which the trend has been toward decline. This site is connected to a large estuary restoration project, which has likely altered the salinity regime experienced in this blind saltmarsh channel substantially since several dikes were first breached in 2019.
Trapping efforts distributed broadly across Drayton Harbor from 2019-2022 detected P. macrodactylus only at a subset of sites, nearly all associated with freshwater inputs (Figure 4). Most observations were made within or near the mouth of the two tidal creeks that empty into the estuary, Dakota and California creeks. On the north side of the Harbor, a few detections were made in a small stream outflow in Semiahmoo Bay, immediately outside the Harbor and opening into Boundary Bay, B.C. Inside the mouth of the Harbor, a small number of P. macrodactylus were captured in a tight concentration around the base of a derelict pier. This area is a focus of intensive green crab trapping and thus a greater search effort was concentrated at this location. Within the creeks, P. macrodactylus were captured along the entire extent trapped, but not at all sites. Locations with more consistent trapping effort, including the index sites and the mouth of the creeks, generally had more detections.
Regionally, seasonality of captures varied across sites (Figure 5). Several sites had peaks in relative abundance that were consistent across years (Drayton Harbor, Post Point, Alice Bay, Davis Slough), though the timing of those peaks varied across sites. At Alice Bay, P. macrodactylus was captured only during spring, while the single seasonal peak at Drayton Harbor and Davis Slough occurred in late summer (August). At Post Point, seasonality was more variable, but averaged across all years, P. macrodactylus peaked in mid spring (May), and again late summer (August-September). At other sites, seasonal patterns were either not consistent (Sharpe’s Corner and Best Lagoon) or P. macrodactylus were too sparse for trends to be apparent.
Figure 5. Monthly relative abundance (CPUE: standardized as number of shrimp per 100 trap sets) at all sites within the Crab Team network at which P. macrodactylus has been captured by year. Note that not all sites were sampled all years, for site launch dates, see Table 1.
At the two stream systems in Drayton Harbor, California and Dakota creeks, P. macrodactylus showed a seasonal pattern of abundance that changed with distance from the mouth of the creek (Figure 6). Shrimp appeared in traps slightly earlier at the upstream sites, beginning in late spring. At the sites closest to the mouth, P. macrodactylus were only detected in July and August. It was rare to capture P. macrodactylus at the middle sites at either of the two creeks, but at Dakota Creek, during the peak month of August, captures occurred in several traps.
Figure 6. Monthly capture rates (in shrimp per trap) of P. macrodactylus captured in 2021 at Drayton Harbor creek index stations by distance from the mouth of the creek. The site closest to the mouth of California Creek is in the same location as the Crab Team network monitoring site for Drayton Harbor.
Across all collection effort types, P. macrodactylus specimens ranged from 2.9 - 13.2 mm CL and females were larger than males (Figure 7). The largest male measured 8.0 mm CL. Regressions of the three measurement types indicated that the metrics are tightly correlated and can be used to translate measurements from prior studies where the measured length parameters are well defined (Supplementary Figure S2, Supplementary Table S1).
Figure 7. Log-log plot of Vázquez total length versus carapace length of all P. macrodactylus captured (n = 753) showing sex and ovigery status (for females).
We observed that the size range of shrimp captured depended on the sampling method. Both types of sampling that utilized baited traps (Crab Team and Dispersed trapping) captured much larger shrimp than sampling with dip nets (Synoptic) at the same locations (Figure 8). Consequently, trapping yielded substantially female-biased samples (1 male: 25.1 females) relative to dip netting, which, in contrast, resulted in a slightly male-biased sample (1.24 males: 1 female).
Figure 8. Beanplot showing size (CL) of P. macrodactylus captured based on capture method. Dotted line shows global mean for all groups, wide solid lines show individual group means, and narrow bands show individual shrimp measurements. Gray area indicates kernel density estimate for each group.
More than two thirds (69%) of all females captured were gravid and the smallest gravid female was 6.05mm CL. The number females captured and proportion of females that were gravid varied across the months sampled (Figure 9). Gravid females were only captured from May through September, and the proportion of the females that were gravid was highest from June through August. Of the females carrying broods, the prevalence of late-stage ova (eggs with developed eyes), peaked in July. Nevertheless, accounting for the increase in number of gravid females captured in August, the absolute number of broods of late-stage ova was greatest in August.
Figure 9. Seasonality of P. macrodactylus reproduction across Crab Team network sampling from 2020 - 2022. Top panel (A) is the number of females captured total across all sites, with ovigery condition. Bottom panel (B) shows the proportion of gravid females with broods in early versus late developmental state (eggs with visible eyes, or no visible eyes).
Observations of the early stages of invasions can be particularly challenging to obtain if the non-native species is not of management interest. In documenting the spread of Palaemon macrodactylus into the southern portion of the Salish Sea, we demonstrate the opportunity afforded by participatory science programs to provide rich ecological records of changing environments. Two specific features of the program that enabled this opportunity included consistent protocol implementation by a broad range of participants and verifiable, archivable data.
The Crab Team network monitoring dataset captures not only the first record of the spread of P. macrodactylus into the Washington portion of the Salish Sea, but also the increasing abundance and ongoing geographic range expansion of this shrimp into Puget Sound, as well as spatially variable patterns of seasonal abundance. The opportunistic documentation of this phenomenon was enabled by sampling at a scale only feasible with extensive volunteer/partner participation. Further, the addition of fine spatial scale trapping data from Drayton Harbor enabled a closer look at habitat use and seasonality, contextualizing point observations (i.e., Crab Team monitoring data) within a larger mosaic of habitats at the water body-scale. P. macrodactylus are highly concentrated at the mouths of tidally influenced creeks, particularly in the late summer months. We also observed that the capture method can exert a strong bias on which segments of the population are sampled. In combination, these factors should be carefully considered when attempting to characterize a population.
Though P. macrodactylus was not the target species of the monitoring effort, the consistent sampling design of the Crab Team trapping provided the first systematic set of observations for this species within the Salish Sea. Notably, these are likely not to have been the first arriving individuals, however, given the timing and demographics of the shrimp captured and detected.
P. macrodactylus was first detected in San Francisco Bay more than 70 years ago, but spread into the Salish Sea is almost certainly quite recent, as, prior to this study, only two point observations were referenced in the last two decades (Lamb and Hanby, 2005; Jensen, 2014). The increase in abundance and ongoing spread observed at Crab Team sites aligns with the interpretation that the P. macrodactylus expansion is continuing to unfold in the Salish Sea, and that populations have not yet reached equilibrium. As of 2024, P. macrodactylus is consistently found at sites spanning 160 km of shoreline within the Washington portion of the Salish Sea. No P. macrodactylus were confirmable at sites along the Strait of Juan de Fuca as the main entry to the Salish Sea, though one photograph record evaluated from Dungeness Spit, which is approximately in the center of the Strait, was rated as medium confidence of being a P. macrodactylus. Dungeness Spit is a site of intense green crab trapping activity, yet no other reports of P. macrodactylus have been made in either Crab Team monitoring or other trapping activities since that time.
Within the Salish Sea, a few locations of capture for this species have been reported by agency staff alerted to their possible presence. Most of these detections have occurred within sites at which the presence of P. macrodactylus also is captured in the Crab Team dataset. Of particular note, however, are a few detections made in Quilcene Bay, within the Hood Canal sub-basin of Puget Sound, where no monitoring efforts have detected P. macrodactylus (Washington Department of Fish and Wildlife, personal communication, 10-25-2024), though these are not photo-verifiable.
Despite initially being reported from Willapa Bay, Washington by 2005, P. macrodactylus has not been captured in any monitoring traps in the coastal estuary sites, the majority of which have been sampled since 2020. All Crab Team sites in Willapa Bay are associated with tidal freshwater sloughs, as would be expected to be suitable for this species, yet only a single opportunistic observation is confirmable from the last decade (J. Ruesink, personal communication, 11-11-2024).
Because P. macrodactylus were captured in the first year of monitoring at the northernmost two sites (Drayton Harbor and Post Point), it is almost certain that this species had already been present for at least a few years prior to their discovery. Yet the rate of spread and increase following these detections suggests that establishment likely occurred quite recently. Nevertheless, given the species’ estimated 2-3 year life span (Table 2), it can be difficult to age shrimp beyond year one. The earliest samples that could be measured were collected in 2020 and included adult shrimp at all sites sampled. Indeed, the largest individual shrimp measured in the study (13.2 mm CL) was captured during this early effort. Moreover, trapping, which was the primary mode of initial detections, is more sensitive for larger shrimp, and could fail to capture the first cohorts of juveniles. Thus, it might not be possible to accurately assign a date of arrival at any site.
Table 2. Reported size and life history parameters for P. macrodactylus studied in six regions, including the native range (Japan).
Seasonality and habitat distribution of P. macrodactylus across sites and within creek systems aligns with observations from other researchers (Siegfried, 1980; Omori and Chida, 1988a; González-Ortegón et al., 2006; Béguer et al., 2011; Ashelby et al., 2013). The extent to which sites demonstrated seasonality varied by site; the majority of sites with high abundances of P. macrodactylus experienced the greatest relative abundance in late summer. This might in part be explained by the bias of traps toward large females, which were themselves most abundant at that time of year. Additionally, selection of specific habitats for each network monitoring site also impacts apparent changes in seasonality across sites within the network. This inference was supported by the observation of seasonal patterns along the two creek systems in Drayton Harbor, showing that P. macrodactylus increased in abundance high in the creeks, near the upper limits of marine influence, slightly earlier in the season than downstream increases (where the network monitoring site within Drayton Harbor Was located). Previous researchers have documented seasonal estuarine migrations, and thus, to account for landscape-scale habitat use, combining regional monitoring at low spatial resolution with local high spatial resolution sampling is important to fully resolve population dynamics. Nevertheless, the current dataset does not allow us to determine whether these spatial patterns differ by shrimp sex or size. It’s also important to note that, based on the salinity tolerances for this species, P. macrodactylus distribution in tidal creeks almost certainly extends further upstream than was sampled for this effort.
Though sampling biases of capture methods make it difficult to directly compare populations from the Salish Sea to those in other invaded ranges, several demographic parameters observed in P. macrodactylus from the Salish Sea, including maximum size and ovigery appear to fall within observations and estimates from other regions. The largest female we observed was within the range of values for both observed maximum size as well as asymptotic size estimated by cohort analysis for both the native and non-native range (Table 2). By contrast, the maximum size of males we observed was substantially smaller than asymptotic size estimated by cohort analysis for either of the prior two studies, as well as the observed maximum size in the native range. Interestingly, Vázquez et al. (2012) only observed males as large as 7.5mm CL, but reported undersampling males in that study. Given the majority of the specimens we measured were captured by trap, and traps demonstrated size bias toward larger shrimp, it is likely that males were also undersampled relative to females in this effort.
The size of the smallest ovigerous female we captured (6.05mm CL) was substantially smaller than that reported from the native range, but more similar to sizes reported from the other two non-native populations described in the literature. Including the present study, there is a trend whereby females from invasive populations of P. macrodactylus obtain a smaller maximum size but achieve reproductive maturity at a smaller size than females from the native range. This might indicate a change in life history strategy, toward earlier reproduction, is favored by the invasion process for this species (Jaspers et al., 2018).
Definitive vectors and pathways for the invasion of P. macrodactylus into and across the Salish Sea remain unidentified. Three likely mechanisms of spread include oceanographic larval dispersal, ballast water transport (Newman, 1963; Wasson et al., 2001), and human-mediated transport of P. macrodactylus for use as bait (Standing, 1981; Carlton, 1985; Ashelby et al., 2013). It is difficult to assess the importance of larval dispersal in the spread of the species, in part because the behavior of larvae relative to salinity is not yet fully resolved. Little (1969) characterized zoeal progression of P. macrodactylus through 6-7 stages taking 12-18 days, with an additional megalopal stage. Thus, P. macrodactylus has a relatively short pelagic duration that might limit dispersal distances. Conversely, Vázquez et al. (2015) suggested that a lower freshwater tolerance of P. macrodactylus larvae relative to adults indicates an export strategy. It is perhaps notable, however, that Vázquez studied a population of the species that has the relatively uncommon characteristic of being distributed in marine waters for its entire life cycle. Regardless of these tendencies, P. macrodactylus has been observed in estuaries at all life stages (González-Ortegón et al., 2006). If larvae do not disperse well through saline waters, this could have the effect of slowing larval spread, particularly in open coastal environments. The Salish Sea is brackish relative to the open coastal waters of the Pacific Ocean (26-29 PSU, Walker et al., 2022). Combined with the high density of moderate freshwater inputs and low-grade tidal creeks, the Salish Sea might create more favorable conditions for accelerated spread of P. macrodactylus than open coastal waters of Washington state.
Initial spread into the Salish Sea from coastal waters likely faced an additional oceanographic barrier to larval dispersal in the predominant outward (westward) surface flow of waters (Brasseale et al., 2019). This occurs due to substantial freshwater input through large mountain-fed river systems, particularly the Fraser and Skagit, creating a low-density surface layer that conveys oceanward. This prevailing condition has been hypothesized to reduce the advection of larval European green crab from coastal embayments into and through the Strait of Juan de Fuca, but can also exhibit seasonal reversals, particularly along the southern shoreline. These reversals could allow opportunistic episodic spread if their timing aligns with larval presence. Based on the disappearance of ovigerous females during late summer and early fall, we infer larvae would be most abundant in fall, which indeed coincides with the typical timing of greatest probability of reversals (Brasseale et al., 2019). This provides at least circumstantial support for advection as a possible vector of this species from coastal waters.
It is also possible that human-mediated vectors of dispersal, e.g., ballast water transport, or intentional movement for use as bait, might have helped P. macrodactylus bypass the semi-permeable circulation barrier described above and establish founding populations in British Columbia, the first documented locations for P. macrodactylus within the Salish Sea. Namely, if oceanographic larval advection were the predominant pathway of dispersal into the Salish Sea, we would have expected to detect P. macrodactylus at monitoring sites along the southern side of Strait of Juan de Fuca, but have found no confirmable evidence this is the case. Indeed, the only two sites (Victoria and Boundary Bay) where this species was earlier reported were north of the Strait. Both of these are sites near major international shipping activities and ballast water has previously been inferred as an important vector for this species’ dispersal (Lejeusne et al., 2014).
The timing and locations of spread suggest that human mediated transport of one fashion or another may still be playing a role in expansion of this species. In particular, the occurrence of P. macrodactylus at Best Lagoon may represent an instance of intentional or accidental movement because the site is nearly 100 km south of other infested sites and nearby monitoring sites have not detected the species. Humans could be helping to accelerate the spread by enabling P. macrodactylus to overcome partial natural barriers and allowing them to access broader ranges of suitable habitat that they would have been less likely to encounter without human assistance. Leapfrog dispersal is a common characteristic of human-mediated spread of non-native species.
Several observations from our data highlighted ways in which sampling design can influence inference on population demographics. We observed that small scale changes in sample timing, location, and collection method can not only influence the probability of detection, but also which segments of the population are sampled. The traps we used, which were set targeting green crab, can catch P. macrodactylus as well, but bias the capture toward large shrimp, which consequently means that females are sampled more heavily than males. A few small individuals (as small as 4 mm CL) did appear in traps, but at a much lower frequency than shrimp of that size collected from the same site and month with dip nets. Conversely, dip net collection caught a very small number of larger individuals. This discrepancy could be related to either size selectivity of the traps, or to behavioral differences across shrimp population segments. For instance, if larger females are more mobile than smaller males, they might migrate further with tidal cycles and be less catchable by dipnetting which took place on a daytime low tide. Similarly, larger shrimp may have a more robust rapid escape response via tail flip. Smaller males may also be more reluctant to enter traps in which larger animals are already present. Cannibalism and territoriality of large females has been observed in captivity, particularly of gravid females (Omori and Chida, 1988b). Regardless of the mechanism, neither of the two sampling methods alone captures the full-size range of shrimp present, and thus researchers should use caution when interpreting population parameters such as size frequency distribution or sex ratio from a single capture method.
In a parallel fashion, the seasonal movements and habitat specificity of P. macrodactylus mean that sampling is particularly sensitive to location and timing of collection. Notably regarding the Crab Team data set, the probability of detecting P. macrodactylus within a given water body is likely variable across Crab Team sites. This is because these sites were selected for suitability for European green crab at the early stages of invasion, as well as for logistical feasibility of monitoring. Thus, some sites do possess habitat features that are also highly favorable for P. macrodactylus, but not all. For instance, at Chuckanut Bay, a site just south of Post Point, the Crab Team site is located near the mouth of the bay, along a riprap wall. However, a freshwater stream that feeds the estuary from the other side might be more likely to support P. macrodactylus if they are present in the water body. Thus, a more sensitive effort to specifically assess the presence of P. macrodactylus in a particular water body might preferentially select stream mouths with significant upstream tidal incursion.
Arrival and spread of non-native species can go undetected, particularly those that are not known to have negative impacts. Nevertheless, monitoring these species can be valuable, both to inform invasion theory, and because lag times can sometimes precede a change (i.e., sleeper invaders, Spear et al., 2021). Participatory science affords opportunities for discovery beyond initial project goals, yet such efforts must be designed carefully to balance the increased demands on program participants and staff as protocols become more expansive and complex (Likens and Lindenmayer, 2018).
Three criteria of programs could support achieving these multiple goals. First, collecting data types that can be both closely QA/QC’ed and retrospectively reviewed, such as photos, or archived water samples. Second, choosing methods capable of sampling a broad range of taxa, and ensuring protocols account for recording these taxa as carefully as target species. Lastly, selecting sites with characteristics susceptible to invasion, such as proximity to human activities, or habitat types suitable for multiple likely species likely to invade a given region. In this way programs can increase the potential impact of monitoring efforts.
The Crab Team protocols utilize one approach to this balance, by requesting photographic documentation alongside specified data for verification. Photographs enabled low-effort preliminary data verification and enabled us to revisit data from years prior to the “discovery” of P. macrodactylus. They further provide an archive to explore spread, as well as other questions not conceived at the start of the project.
In Washington, many restoration efforts that aim to improve migration habitat for salmonids are also creating highly suitable habitats for P. macrodactylus. Many of these efforts, by removing barriers to fish migration, also restore natural tidal flow and enlarge the extent and physical complexity of estuarine habitat favored by P. macrodactylus (Newman, 1963). Additionally, restoration of marine and freshwater connectivity will also augment tidal creeks that are similar to those in Drayton Harbor, which offer additional recruitment habitat and seasonal migratory corridors for this species.
Thus, it appears almost certain that this species will become increasingly abundant and widespread within the Salish Sea. The impacts of this expansion are difficult to anticipate, however. This region has no native palaemonid shrimp, but P. macrodactylus shows dietary overlap with several species of Crangonid shrimp; mysid shrimps make up a significant portion of the diet of both types of shrimp (Siegfried, 1980, 1982). The geographic overlap of these species observed across the Crab Team network is limited. While Crangonid shrimps have been trapped at two thirds of sites where P. macrodactylus has also been captured, this overlap is rare relative to the wider distribution of Crangonids within the network or monitoring sites; P. macrodactylus has been captured at only 6 of 36 sites where Crangonids have been observed (Unpublished data, Crab Team). Moreover, no substantial ecological impacts by this species have been documented from other regions where it is invasive. Nevertheless, by capturing the relatively early stage of invasion, this opportunistic documentation of a cryptic range expansion enables future work to more carefully assess the evidence for such impacts.
The datasets presented in this study can be found in online repositories. The names of the repository/repositories and accession number(s) can be found below: Repository: URL: http://datadryad.org/stash/share/dDf9KY9W1Wito5knfpJR_1oLT7O2OHpH1NnehJQ4O84.
The manuscript presents research on animals that do not require ethical approval for their study.
EG: Conceptualization, Data curation, Formal analysis, Funding acquisition, Investigation, Methodology, Project administration, Resources, Software, Supervision, Validation, Visualization, Writing – original draft, Writing – review & editing. JP: Data curation, Investigation, Methodology, Writing – review & editing. PM: Conceptualization, Funding acquisition, Investigation, Methodology, Supervision, Writing – review & editing.
The author(s) declare that financial support was received for the research and/or publication of this article. The following funding sources have contributed to this project United States Environmental Protection Agency assistance agreements PC 00J29801, PC-00J90701, PC-01J22301, The Washington Department of Fish and Wildlife contracts 17-09728, 19-13737, 20-15421, 23-24088 The National Science Foundation Division of Ocean Sciences award #1850945. The contents of this document do not necessarily reflect the views and policies of the Environmental Protection Agency, the Washington Department of Fish and Wildlife, or the National Science Foundation, nor does mention of trade names or commercial products constitute endorsement or recommendation for use.
The authors are grateful to Gregory Jensen for originally identifying this shrimp at our request, and for graciously contributing many species identifications to the Crab Team program. Lindsey Parker at the Washington Department of Fish and Wildlife supported data sharing from green crab trapping in Drayton Harbor and Samish Bay, and the authors greatly appreciate the efforts of WDFW and Northwest Straits Commission staff to identify and collect specimens they encountered. Jennifer Ruesink also provided helpful reference to recent observations, and lack thereof, of P. macrodactylus in Willapa Bay. Not least of all, we are grateful to the hundreds of Crab Team monitors that looked carefully at each and every one of their shrimp captures, and particularly those who took additional time and marital capital to retain and convey those specimens to the authors. Lastly, the preparation of this manuscript was a joy thanks to support for a writing retreat provided by Washington Sea Grant and held at the Whitely Center at Friday Harbor Laboratories at the University of Washington.
The authors declare that the research was conducted in the absence of any commercial or financial relationships that could be construed as a potential conflict of interest.
The author(s) declare that no Generative AI was used in the creation of this manuscript.
All claims expressed in this article are solely those of the authors and do not necessarily represent those of their affiliated organizations, or those of the publisher, the editors and the reviewers. Any product that may be evaluated in this article, or claim that may be made by its manufacturer, is not guaranteed or endorsed by the publisher.
The Supplementary Material for this article can be found online at: https://www.frontiersin.org/articles/10.3389/fmars.2025.1553583/full#supplementary-material
Ashelby C., De Grave S., Johnson M. (2013). The global invader Palaemon macrodactylus (Decapoda, Palaemonidae): an interrogation of records and a synthesis of data. Crustaceana 86, 594–624. doi: 10.1163/15685403-00003203
Béguer M., Bergé J., Martin J., Martinet J., Pauliac G., Girardin M., et al. (2011). Presence of Palaemon macrodactylus in a Europe estuary: evidence for a successful invasion of the Gironde (SW France). Aquat. Invasions 6, 301–318. doi: 10.339/ai.2011.6.3.07
Brasseale E., Grason E. W., McDonald P. S., Adams J., MacCready P. (2019). Larval transport modeling support for identifying population sources of European green crab in the Salish Sea. Estuaries Coasts 42, 1586–1599. doi: 10.1007/s12237-019-00586-2
Carlton J. T. (1985). Transoceanic and interoceanic dispersal of coastal marine organisms: The biology of ballast. Oceanography Mar. Biol. 23, 313–371.
Cohen A., Mills C., Berry H., Wonahm M. J., Bingham B., Bookheim B., et al. (1998). A rapid assessment survey of non-indigenous species in the shallow waters of Puget Sound (Olympia, WA: Washington State Department of Natural Resources).
Crall A. W., Newman G. J., Jarnevich C. S., Stohlgren T. J., Waller D. M., Graham J. (2010). Improving and integrating data on invasive species collected by citizen scientists. Biol. Invasions 12, 3419–3428. doi: 10.1007/s10530-010-9740-9
Cuesta J. A., González-Ortegón E., Drake P., Rodríguez A. (2004). First record of Palaemon macrodactylus Rathbun 1902 (Decapoda, Caridea, Palaemonidae) from European waters. Crustaceana 77, 377–380. doi: 10.12681/mms.309
Delaney D. G., Sperling C. D., Adams C. S., Leung B. (2008). Marine invasive species: validation of citizen science and implications for national monitoring networks. Biol. Invasions 10, 117–128. doi: 10.1007/s10530-007-9114-0
Encarnação J., Teodósio M. A., Morais P. (2021). Citizen science and biological invasions: A review. Front. Environ. Sci. 8, 1–13. doi: 10.3389/fenvs.2020.602980
González-Ortegón E., Pascual E., Cuesta J. A., Drake P. (2006). Field distribution and osmoregulatory capacity of shrimps in a temperate European estuary (SW Spain). Estuarine Coast. Shelf Sci. 67, 293–302. doi: 10.1016/j.ecss.2005.11.025
Grason E., McDonald S., Adams J., Litle K., Apple J., Pleus A. (2018). Citizen science program detects range expansion of the globally invasive European green crab in Washington State (USA). Manage. Biol. Invasions 9, 39–47. doi: 10.3391/mbi.2018.9.1.04
Grosholz E. D., Ruiz G. M. (1996). Predicting the impact of introduced marine species: Lessons from the multiple invasions of the European green crab Carcinus maenas. Biol. Conserv. 78, 59–66. doi: 10.1016/0006-3207(94)00018-2
Jaspers C., Marty L., Kiørboe T. (2018). Selection for life-history traits to maximize population growth in an invasive marine species. Global Change Biol. 24, 1164–1174. doi: 10.1111/gcb.13955
Jensen G. C. (2014). Crabs and Shrimps of the Pacific Coast: A guide to shallow-water decapods from southeastern Alaska to the Mexican border (Bremerton, WA: Mola Marine).
Lamb A., Hanby B. P. (2005). Marine life of the Pacific Northwest: A photographic encyclopedia of invertebrates, seaweeds and selected fishes (Madeira Park, British Columbia, Canada: Harbour Publishing).
Lejeusne C., Saunier A., Petit N., Béguer M., Otani M., Carlton J. T., et al. (2014). High genetic diversity and absence of founder effects in a worldwide aquatic invader. Sci. Repports 4, 5808. doi: 10.1038/srep05808
Likens G., Lindenmayer D. (2018). Effective ecological monitoring (Collingwood, Australia: Csiro Publishing).
Little G. (1969). The larval development of the shrimp, Palaemon macrodactylus Rathbun, reared in the laboratory, and the effect of eyestalk extirpation on development. Crustaceana 17, 69–87. doi: 10.1163/156854069X00079
Newman W. A. (1963). On the introduction of an edible Oriental shrimp (Caridea, Palaemonidae) to San Francisco Bay. Crustaceana 5, 119–132. doi: 10.1163/156854063X00390
Omori M., Chida Y. (1988a). Life history of a Caridean shrimp Palaemon macrodactylus with special reference to the difference in reproductive features among ages. Nippon Suisan Gakkaishi 54, 365–375. doi: 10.2331/suisan.54.365
Omori M., Chida Y. (1988b). Reproductive ecology of a caridean shrimp Palaemon macrodactylus in captivity. Nippon Suisan Gakkaishi 54, 377–383. doi: 10.2331/suisan.54.377
Schneider C. A., Rasband W. S., Eliceiri K. W. (2012). NIH Image to ImageJ: 25 years of image analysis. Nat. Methods 9, 671–675. doi: 10.1038/nmeth.2089
Siegfried C. A. (1980). Seasonal abundance and distribution of Crangon franciscorum and Palaemon macrodactylus (Decapoda, Caridea) in the San Francisco Bay-delta. Biol. Bull. 159, 177–192. doi: 10.2307/1541017
Siegfried C. A. (1982). Trophic relations of Crangon franciscorum Stimpson and Palaemon macrodactylus Rathbun: predation on the opossum shrimp, Neomysis mercedis Holmes. Hydrobiologia 89, 129–139. doi: 10.1007/BF00006166
Spear M. J., Walsh J. R., Ricciardi A., Zanden M. J. V. (2021). The invasion ecology of sleeper populations: prevalence, persistence, and abrupt shifts. BioScience 71, 357–369. doi: 10.1093/biosci/biaa168
Spivak E. D., Boschi E. E., Martorelli S. R. (2006). Presence of Palaemon macrodactylus Rathbun 1902 (Crustacea: Decapoda: Caridea: Palaemonidae) in Mar del Plata Harbor, Argentina: First record from southwestern Atlantic waters. Biol. Invasions 8, 673–676. doi: 10.1007/s10530-005-2063-6
Standing J. D. (1981). Occurrences of shrimps Natantia, Penaeidea, and Caridea in central California and Oregon USA. Proc. Biol. Soc. Washington 94, 774–786. doi: 10.5281/zenodo.13485512
Tulloch A. I. T., Possingham H. P., Joseph L. N., Szaby J., Martin T. G. (2013). Realising the full potential of citizen science monitoring programs. Biol. Conserv. 165, 128–138. doi: 10.1016/j.biocon.2013.05.025
Vázquez M. G., Bas C. C., Kittlein M., Spivak E. D. (2015). Effects of temperature and salinity on larval survival and development in the invasive shrimp Palaemon macrodactylus (Caridea: Palaemonidae) along the reproductive season. J. Sea Res. 99, 56–60. doi: 10.1016/j.seares.2015.02.002
Vázquez M. G., Bas C. C., Spivak E. D. (2012). Life history traits of the invasive estuarine shrimp Palaemon macrodactylus (Caridea: Palaemonidae) in a marine environment (Mar del Plata, Argentina). Scientia Marina 76, 2–210. doi: 10.3989/scimar.03506.02F
Vejan A., Patimar P., Jafaryan H., Gholizadeh M., Adineh H., Aghilinezhad S. M. (2023). Population parameters of the non-indigenous invasive shrimp Palaemon macrodactylus Rathbun, 1902 (Caridea: Palaemonidae) from the southeastern Caspian Sea, with implications for range expansions, threats and conservation. Marine Environmental Research 190, 106078. doi: 10.1016/j.marenvres.2023.106078
Walker S., Mozaria-Luna H. N., Kaplan I., Petatán-Ramírez D. (2022). Future temperature and salinity in Puget Sound, Washington State, under CMIP6 climate change scenarios. J. Water Climate Change 13, 4255–4272. doi: 10.2166/wcc.2022.282
Warkentine B. E., Rachlin J. W. (2010). The first Record of Palaemon macrodactylus (Oriental shrimp) from the eastern coast of North America. Northeastern Nat. 17, 91–102. doi: 10.1656/045.017.0107
Wasson K., Zabin C. J., Bedinger L., Cristina Diaz M., Pearse J. S. (2001). Biological invasions of estuaries without international shipping: the importance of intraregional transport. Biol. Conserv. 102, 143–153. doi: 10.1016/S0006-3207(01)00098-2
Keywords: invasive species, citizen science, estuaries, palaemonid shrimp, ecological monitoring
Citation: Grason EW, Pineda J and McDonald PS (2025) Tracking two invasions for the cost of one: opportunistically tracking the range expansion of non-native Palaemon macrodactylus in the Salish Sea through participatory science. Front. Mar. Sci. 12:1553583. doi: 10.3389/fmars.2025.1553583
Received: 31 December 2024; Accepted: 28 February 2025;
Published: 26 March 2025.
Edited by:
Elene Trujillo, Cramer Fish Sciences, United StatesReviewed by:
Patricia Briones-Fourzan, National Autonomous University of Mexico, MexicoCopyright © 2025 Grason, Pineda and McDonald. This is an open-access article distributed under the terms of the Creative Commons Attribution License (CC BY). The use, distribution or reproduction in other forums is permitted, provided the original author(s) and the copyright owner(s) are credited and that the original publication in this journal is cited, in accordance with accepted academic practice. No use, distribution or reproduction is permitted which does not comply with these terms.
*Correspondence: Emily W. Grason, ZWdyYXNvbkB1dy5lZHU=
Disclaimer: All claims expressed in this article are solely those of the authors and do not necessarily represent those of their affiliated organizations, or those of the publisher, the editors and the reviewers. Any product that may be evaluated in this article or claim that may be made by its manufacturer is not guaranteed or endorsed by the publisher.
Research integrity at Frontiers
Learn more about the work of our research integrity team to safeguard the quality of each article we publish.