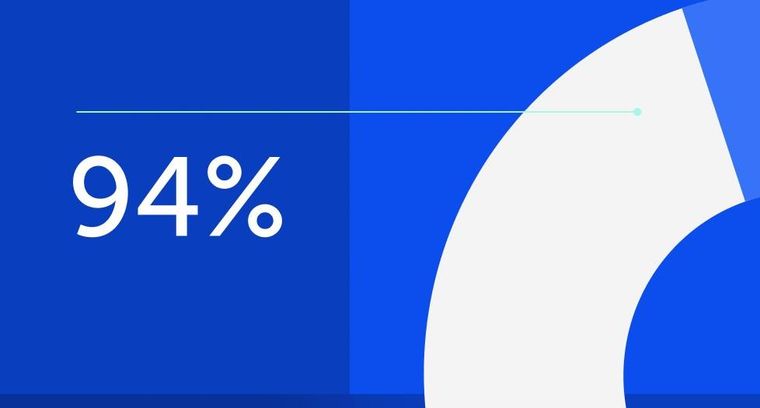
94% of researchers rate our articles as excellent or good
Learn more about the work of our research integrity team to safeguard the quality of each article we publish.
Find out more
ORIGINAL RESEARCH article
Front. Mar. Sci., 14 April 2025
Sec. Marine Megafauna
Volume 12 - 2025 | https://doi.org/10.3389/fmars.2025.1553509
For highly mobile marine species such as pelagic elasmobranchs, the development of effective spatial management requires a comprehensive understanding of movement ecology. Research incorporating movement data across seasons and life stages, including reproductive states, is valuable for informing spatial management, yet is absent for most species. In the Northwest (NW) Atlantic Ocean (hereafter referred to as NW Atlantic), the porbeagle shark Lamna nasus is a pelagic species that is overfished, has a live retention ban (Canada) or landings regulations (United States), and is also commonly captured incidentally as bycatch. Research on the spatiotemporal dynamics of NW Atlantic porbeagle habitat use is limited, with all previous research utilizing pop-off satellite archival tags that are prone to large uncertainty in location estimates. This study used higher-accuracy fin-mount satellite tags to identify patterns in habitat use across life stages and seasons for porbeagle sharks tagged off the northeastern coast of the United States. During the summer and fall, the 95% kernel density estimate (referred to as “activity space”) of tagged porbeagles occurred almost exclusively on the continental shelf in the Gulf of Maine and Georges Bank. Activity space expanded and shifted southwards to include offshore environments during the winter and spring for juveniles, mature non-gravid females, and mature females of unknown reproductive states, while the activity space of mature males and gravid females remained in shelf waters year-round. This finding differs from the previous assumption that southward migrations are linked to reproduction for NW Atlantic porbeagles. Tagged porbeagles were also found to have a relatively small 50% kernel density estimate (referred to as “high occupancy area”) located around Cape Cod, Massachusetts that was well-conserved across life stages and seasons. This relatively static, small high occupancy area has implications for the population’s conservation given the high amount of fishing activity (rod-and-reel, trawl, gillnet) occurring within this region. Given the overlap between porbeagle high occupancy area and fishing activity, as well as the relatively high recapture rate of tagged sharks (10.5%), the coastal waters around Cape Cod, Massachusetts should be considered for spatial management of the NW Atlantic porbeagle.
Global declines in elasmobranch populations (Dulvy et al., 2021) have driven widespread prioritization of research and development of conservation strategies (Jorgensen et al., 2022). A relatively common approach to elasmobranch conservation is spatial management, in which critical habitats are protected from anthropogenic impacts, albeit with varying degrees of efficacy (i.e., Edgar et al., 2014; Jaiteh et al., 2016; White et al., 2017; Birkmanis et al., 2020; Crear et al., 2021; Shea et al., 2023). Not only does the highly mobile nature of many elasmobranch species challenge effective spatial management, the tendency for elasmobranch species to segregate by sex, size or maturity, and/or reproductive states further confounds this effort (Cartamil et al., 2010; Bansemer and Bennet, 2011; Chapman et al., 2015; Sulikowski et al., 2016; Maxwell et al., 2019). Space use may be related to a combination of many factors, including competition and predation, food availability, reproduction, energetic constraints, and abiotic needs (Bowler and Benton, 2005; Shaw, 2020; Bowlby et al., 2022). Collectively, these factors make spatial management decisions inherently complex and highlight the value of comprehensive data on spatiotemporal movement patterns across all life stages of a population in order to identify and prioritize the most critical conservation areas. In fact, a recent survey of experts identified making tagging studies more applicable to elasmobranch conservation as a key research priority and suggested that using representative life-history sampling schemes was essential to accomplishing this goal (Jorgensen et al., 2022).
The porbeagle Lamna nasus is a large, pelagic, and regionally endothermic shark species that inhabits cold-temperate waters in the North Atlantic and the Southern Hemisphere, with the warm equatorial waters acting as a present-day barrier for inter-hemispheric dispersal (Francis et al., 2008). Like most large sharks, the porbeagle exhibits life history characteristics (long lifespans, slow growth, low reproductive output) which make the species vulnerable to overexploitation and depletion (Campana et al., 2002; Jensen et al., 2002; Natanson et al., 2002, 2019), and the species is assessed as “Vulnerable” to extinction on a global scale (Rigby et al., 2019). Furthermore, although the Northwest (NW) Atlantic population was originally suggested to have an annual reproductive cycle (Jensen et al., 2002), a more recent study found evidence of a biennial cycle in some individuals (Natanson et al., 2019), which suggested lower reproductive output and greater risk of population decline. The NW Atlantic porbeagle population was once the target of a commercial longline fishery (Aesen, 1963; Campana et al., 2002). However, overexploitation led this stock to be designated as an endangered species in Canada (COSEWIC, 2004, 2014), and an overfished species in the United States (U.S.) (Curtis et al., 2016). Conservation concern for the population also led to landings restrictions, including a ban on live retention in Canada (Campana et al., 2015) and catch and size limits in the U.S. (NMFS, 2007; ICCAT, 2015). Despite these efforts to minimize fishing mortality, porbeagles continue to be captured incidentally as bycatch in numerous commercial (longline, rod-and-reel, trawl, gillnet) and recreational (rod-and-reel) fisheries in the region (Hurley, 1998; Curtis et al., 2016; Haugen, 2020; NOAA, 2023). The NW Atlantic porbeagle may benefit from spatial management strategies given the predictions of stock assessments which suggested population rebuilding could take up to 100 years (NOAA, 2023).
A limited number of studies have used pop-off satellite archival tags (PSATs) to explore the spatiotemporal dynamics of horizontal habitat use for NW Atlantic porbeagles (Campana et al., 2010; Skomal et al., 2021; Braun et al., 2023). Skomal et al. (2021) found that large juveniles were shelf-oriented in the summer and early fall and made more extensive movements into offshore habitat in the winter and spring. In comparison, Campana et al. (2010) found large juvenile females predominately utilized shelf-waters (although tag deployments were more limited to summer through early winter), one mature male utilized shelf-waters while another made southeasterly offshore movement in the winter, and all mature females moved to offshore habitats in winter, several of which migrated as far south as the Sargasso Sea in the spring before tag pop-off (Campana et al., 2010). Based on the assumed annual reproductive cycle of the population during the time of the study (Jensen et al., 2002) and the apparent disparity in movements between mature females and other life stages, Campana et al. (2010) suggested that the Sargasso Sea was a pupping ground for the population. However, there are two key assumptions which limit the validity of these conclusions. First, the tag deployment periods limited the comparisons of habitat use among life stages during all seasons, as most data from late winter and spring was from mature females. Second, more recent data indicate that at least a portion of the NW Atlantic porbeagle population reproduces biennially (Natanson et al., 2019). As mature females tagged by Campana et al. (2010) were not confirmed to be gravid by any recognized method, their reproductive states remain uncertain, and therefore it is possible that movement patterns differ by reproductive state.
A more directed study of seasonal movement that spans all life stages, including all reproductive states, would provide a more comprehensive understanding of the spatiotemporal dynamics of porbeagle habitat use in the NW Atlantic. Moreover, all previous telemetry work on NW Atlantic porbeagles have used PSATs to estimate geolocation. There are numerous recognized trade-offs associated with the type of tags used in telemetry studies. While PSATs provide depth and temperature data as well as daily light-based geolocation estimates that do not rely on the animal being at the sea surface, it is at the expense of reduced location accuracy (i.e., Wilson et al., 2007; Winship et al., 2012), with uncertainty bounds on the order of several hundred km (Wilson et al., 2007). In comparison, fin-mount satellite tags transmit more accurate location information, with uncertainty bounds on the order of hundreds of m to <5 km (i.e., Tougaard et al., 2008), but at irregular intervals (reviewed by Hammerschlag et al., 2011; Renshaw et al., 2023), and most fin-mount tag models do not provide depth or temperature data. Successful transmission by fin-mount satellite tags is dependent on the animal being at the sea surface and in proximity to an orbiting satellite (i.e., Winship et al., 2012). Given that porbeagles spend a large proportion of time at the sea surface (Pade et al., 2009; Skomal et al., 2021; Anderson et al., 2024a) and the recent successful use of fin-mount tags on porbeagles in the Northeast Atlantic (Bortoluzzi et al., 2024), the NW Atlantic population could be a suitable candidate for using the more accurate fin-mount satellite tags to study its habitat use. Here, we advance the study of movement ecology of porbeagles found in the NW Atlantic by using high-accuracy fin-mount satellite tags deployed on all life history stages (including confirmed reproductive states) over a span of five years, and with several tag deployments lasting longer than one year. Specifically, we used satellite-tag derived data to advance the following overarching goals: (1) assessing seasonal habitat use of porbeagles in the NW Atlantic and (2) comparing movement patterns by life stage by integrating empirical reproductive data.
Porbeagles (n = 38) were captured using rod-and-reel in the NW Atlantic from 2017 to 2022, in locations spanning continental shelf waters from southern Maine (ME) to southern Massachusetts (MA) (Table 1). Captured sharks were either left in the water and secured alongside the vessel or brought onboard the vessel. For sharks that were brought onboard the vessel, a saltwater pump was placed in the mouth to facilitate respiration throughout sampling. Sex, fork length (FL; cm), total length (TL; cm), and capture location were recorded. The life stage of each shark was determined based on a length-at-age relationship (Natanson et al., 2002) and the size at 50% maturity (174 and 218 cm FL for males and females, respectively; Jensen et al., 2002) for this population, and/or ultrasonography for some females (described below). Individuals with FL < 107 cm were considered young-of-the-year (YOY; Natanson et al., 2002). Males were considered juveniles if FL was 107-174 cm or mature if FL was >174 cm (Jensen et al., 2002). For females that were not formally assessed for reproductive stage (described below), sharks were considered juveniles if FL was 107-218 cm or mature if FL was >218 cm (Jensen et al., 2002). However, ultrasonography was performed on most females near or above the size range at maturity (210-230 cm FL; Jensen et al., 2002) to determine the reproductive stage (immature, gravid, or non-gravid) following Sulikowski and Hammerschlag (2023). An Ibex EVO II portable ultrasound (E.I Medical Imaging) with a 60 mm curved linear array 5-2.5 MHz transducer (model 290470) capable of a 24 cm scan depth was used to obtain images of the reproductive tract. Scanning was performed on the ventral surface from the pectoral to the pelvic fin in both a transverse and longitudinal orientation to obtain cross-sectional and lengthwise images of the uterus, respectively.
One of a variety of fin-mount satellite transmitters was fitted to the first dorsal fin of each shark following established protocols (i.e., Hammerschlag et al., 2012; Gibson et al., 2021; Supplementary Figure S1). Tag models included: Sirtrack K2F transmitter (Lotek Wireless; n = 15), Sirtrack F6F FastGPS Argos transmitter (Lotek Wireless; n = 2), Sirtrack Kiwisat K4 transmitter (Lotek Wireless; n = 2), Sirtrack K2F 176D dive transmitter (Lotek Wireless; n = 4), or SPOT 6 transmitter (Wildlife Computers; n = 15). Additionally, a subset of porbeagles (n = 14) were double-tagged with a 365-day PSATFLEX (Lotek Wireless) or sPAT (Wildlife Computers) for other studies (Unpublished data, B. Anderson and J. Sulikowski).
Porbeagle position estimates were downloaded from Argos satellites (CLS America, Inc.). Argos provides location accuracy using the following location classes (LC): 3, 2, 1, 0, A, B, and Z (in decreasing accuracy). These LCs are associated with the following error estimates: LC3: < 250 m, LC2: 250-500 m, LC1: 500-1500 m, and LC0: > 1500 m. Argos does not provide error estimates for LC A or B, but LC A and LC B have been found to be accurate to > 1 km and > 5 km radius, respectively (Tougaard et al., 2008). The lowest accuracy category transmissions (LC Z) are poor, unreliable location estimates and were removed from the dataset (<1% of all transmissions; Supplementary Table S1).
Due to irregular surfacing of sharks (and thus irregular transmission rates) and variation in satellite coverage at any given time, fin-mount satellite tracking data often have large data gaps, and may also be subject to autocorrelation and spatial biases. Therefore, all positional data were regularized and interpolated following an approach comparable to Hammerschlag et al. (2022). Specifically, using the R package ‘aniMotum’ (Jonsen et al., 2023), a continuous-time correlated random walk (CTCRW) state-space model (SSM), with a 2 m/s speed filter (per Skomal et al., 2021), was used to interpolate and regularize positional data along an estimated movement path of each shark. Data were regularized to daily position estimates. Since interpolating track sections with large temporal gaps increases uncertainty in the location estimates (Jonsen et al., 2023), we did not interpolate gaps in the data that exceeded 10 days (Supplementary Figure S2). Instead, full tracks with gaps exceeding 10 days were segmented into multiple sub-tracks prior to model fitting and interpolation was completed separately for each sub-track. Additionally, we did not interpolate sub-tracks with <10 positions.
To examine habitat use, kernel utilization distributions (KUDs) were calculated with the interpolated positions using the ‘adehabitatHR’ R package with the “href” bandwidth estimator (Calenge, 2024). Kernel density estimates (KDEs) were used to quantify areas of higher and lower shark use within the KUDs (Worton, 1989). Specifically, we calculated 95% and 50% density volume contours from the KDEs, with the 95% KDE volume contours representing areas with the lowest densities of shark position estimates (referred to as an “activity space”) and the 50% KDE volume contours representing areas with the highest densities of shark position estimates (referred to as “high occupancy area”). Prior to KUD analyses, any positional data from the first three days of tag deployment were removed to reduce tagging location bias. Additionally, any overlap of KDE contours with land were removed from the estimation of activity space and high occupancy area sizes. At first, movement data from all interpolated tracks were combined to make an overall KUD. Then, to examine seasonal differences in habitat use, seasonal KUDs were calculated using interpolated tracks. Seasons were defined as follows: winter: December-February, spring: March-May, summer: June-August, and fall: September-November. Finally, differences in activity space and high occupancy areas by life stage were observed by calculating life-stage based KUDs with the interpolated tracks. Distance of each SSM-estimated location from the tagging location was calculated for each shark included in habitat use analyses. Dispersion from the tagging location was subsequently plotted by life stage and as a function of time since tagging. Additionally, the proportion of time spent within different national boundaries was determined using exclusive economic zone (EEZ) data from Flanders Marine Institute (2019).
A total of 38 porbeagles were tagged with fin-mount satellite transmitters between June 2017 and October 2022 (Table 1). Tagging locations were concentrated off the northeastern coast of the U.S., particularly offshore of Gloucester and Cape Cod, Massachusetts (MA), as well as offshore of southern Maine (ME). Tagged sharks ranged in size from 81.2 to 244 cm FL and included 10 males (3 YOY, 3 juvenile, 4 mature) and 28 females (4 YOY, 8 juvenile, 3 mature but not assessed for reproductive stage, 3 mature non-gravid, 10 mature gravid), representing all life stages.
Thirty-one of the tagged sharks (81.6%) appeared to survive post-release. However, mortality was evident during the tag deployment for seven (18.4%) of the tagged sharks, and four of these mortalities (57%) were related to recapture in fisheries. Immediate mortality was confirmed in shark 37 (175434, gravid female) based on depth data provided by a PSAT attached to the shark and failure of the fin-mount tag to report. Shark 37 was foul hooked in the ventral surface and experienced an extended fight time (47 min) on rod-and-reel before reaching the vessel. Predation was inferred in shark 36 (207870, gravid female) near Bermuda, approximately five months after tagging, based on the depth and temperature data transmitted by a PSAT attached to the shark (Anderson et al., 2024b). Shark 38 (221129, mature non-gravid female) died from unknown cause south of Bermuda on 12/15/2022, approximately 50 days after tagging, based on depth data provided by a PSAT attached to the shark indicating the shark sunk to 2,600 m and remained at depth until the PSAT mortality release was triggered. The other four sharks appeared to be recaptured in fishing gear during their tag deployment. Shark 23 (33148, mature non-gravid female) appeared to be landed approximately 71 days after tagging based on satellite transmissions occurring on land north of Gloucester, MA for a month before the tag stopped transmitting. Shark 31 (33098, YOY) appeared to be landed based on all satellite transmissions occurring on land (on Cape Cod, MA). Shark 35 (234533, gravid female) is known to have been recaught in a monkfish gillnet south of Cape Cod, MA on 03/27/2023, 154 days after tagging, and suffered at-vessel mortality. Constant depth data from a PSAT attached to shark 35 suggested the shark was in the gillnet for approximately 48 hours before removal. Finally, shark 8 (175793, juvenile female) is known to have been recaught in a trawl targeting squid south of Cape Cod, MA on 06/26/2023, 244 days after tagging, and suffered at-vessel mortality.
Overall, tags reported a total of 11,999 Argos locations, with 54% of locations assigned a LC of 1-3 and 35% assigned a LC of A or B(Supplementary Table S1). The number of days at liberty (days between tagging and the last tag transmission received) for tagged porbeagles ranged widely, from 0 to 963 days (mean = 252 days, median = 170 days) with 24 sharks tracked for ≥100 days. Following the removal of non-reporting tags and tags with limited or infrequent transmissions (i.e., <10 positions in subtracks), tracks segments were available from 26 out of the 38 tagged porbeagles (68%) for data regularization and interpolation with the SSM. For the 12 sharks that could not be used in the SSM and habitat use analysis, one shark died immediately (shark 37; 175434), one was predicted to have been landed shortly after tagging (shark 31; 33098), one had a tag that failed to transmit (shark 27; 33111), five were YOY sharks that had very short periods of transmissions before the tags stopped transmitting (sharks 28, 29, 30, 32, 33), three had transmissions that were too infrequent before they were recaptured (shark 35; 221533) or died (shark 36, 38), and one had infrequent transmissions and a sub-track that did not converge in the SSM (shark 34; 163579).
The 26 sharks incorporated into the SSM included 1 YOY, 9 juveniles, 4 mature males, 7 gravid females, 2 mature non-gravid females, and 3 mature females of unknown reproductive states. The SSM produced a total of 4,447 position estimates from these 26 individuals for habitat use analyses. The number of days with regularized positions for these tagged porbeagles ranged between 25 and 457 days (mean = 174 days, median = 121 days). Track data was most limited for YOY porbeagles, with only one YOY shark (shark 1; 220902) providing enough data for track interpolation. As such, the interpolated track from the single YOY shark was included with the tracks from larger juveniles for further analyses. Additionally, location estimates were limited for mature non-gravid females due to the sample size of individuals and short track durations for this group. There were no SSM location estimates for mature non-gravid females during the winter. Otherwise, location estimates were relatively evenly distributed across life stages and seasons (Supplementary Figure S3).
Regularized daily position estimates were largely concentrated on the northeastern U.S. continental shelf, ranging from ME to southern MA between latitudes of approximately 39°N to 45°N and longitudes of approximately 71°W to 65°W (Figure 1). A limited number of tracks extended outside this range. One mature male (shark 13; 175432) made excursions north into the Bay of Fundy two years in a row. One mature female of unknown reproductive state (shark 26; 220982) migrated to waters southeast of Bermuda. Two juveniles (shark 7; 221136, shark 8; 175793), two mature females (one non-gravid (shark 22; 33131), one of unknown reproductive state (shark 24; 172014)), and one mature male (shark 12; 175435) made excursions to the continental shelf region near Long Island, New York and/or New Jersey (NJ). Finally, one juvenile (shark 8; 175793) and one mature female of unknown reproductive state (shark 26; 220982) moved east of 60°W. When considering national boundaries, 89.7% of porbeagle location estimates occurred within the U.S. EEZ, 9.5% occurred within the Canadian EEZ, and less than 1% of location estimates occurred in the high seas (international waters).
Figure 1. Map of the activity space (95% kernel density estimate; black outer polygon), high occupancy area (50% kernel density estimate; white inner polygon), and regularized state-space model location estimates (grey points) for 26 porbeagles tagged in the Northwest Atlantic, including all life stages and seasons. The 200 m depth contour is represented by blue lines and the exclusive economic zones (EEZ) are represented by grey lines.
In regard to activity space, the overall KDE (95% KDE, including all regularized tracks) was approximately 222,600 km2 and encompassed the continental shelf ranging from the lower Bay of Fundy to NJ, with an extension into offshore habitat along the eastern and southern edges of Georges Bank (black polygon, Figure 1). The overall high occupancy area (50% KDE) was approximately 36,774 km2 and included only continental shelf waters east of Cape Cod, MA, and in a region ranging from 40.2° to 42.7°N latitude and 70.5° to 67.7°W longitude (white polygon, Figure 1).
When considering the amount of time spent in national boundaries seasonally, the highest proportion of time spent in U.S. waters occurred during the fall and winter, with 93.7% of fall and 90.2% of winter SSM locations occurring in U.S. waters. In comparison, 88.8% of summer and 87.1% of spring locations were in U.S. waters. The proportion of time spent in Canadian waters was highest during the spring and summer, with 11.1% of spring and 11.0% of summer locations occurring in Canadian waters. In comparison, 8.3% of winter and 6.2% of fall locations were in Canadian waters. Finally, the highest proportion of time spent in the high seas occurred during the spring and winter, with 1.8% of spring and 1.5% of winter SSM locations occurring in the high seas, respectively. Less than 1% of summer and fall location estimates were in the high seas.
Seasonal level KUDs demonstrated year-round use of the Gulf of Maine, Georges Bank, and particularly the shelf-waters surrounding Cape Cod, MA (Figures 2, 3). The size of activity spaces (95% KDEs) and high occupancy areas (50% KDEs) were greatly reduced during the summer and fall (Table 2), with habitat occurring almost exclusively on continental shelf waters from ME to MA during these seasons (Figures 2C, D). During the winter and spring, activity space and high occupancy areas expanded to approximately 1.5-3 times the size of those in the summer and fall (Table 2), and activity space shifted southward to include portions of the offshore environment along the edge of Georges Bank and east of NJ (Figures 2A, B). During the spring, a second activity space area was present southeast of Bermuda at approximately 31°N, 56°W (Figure 2B). High occupancy areas were in the vicinity of Cape Cod, MA year-round (Figures 2, 3), and spatial overlap in seasonal high occupancy area was located southeast of Cape Cod, MA (Figure 3). Specifically, during the summer there was a high occupancy area encompassing shelf waters southeast, east, and north of Cape Cod and a smaller high occupancy area on the northern edge of Georges Bank. During the fall, there was a high occupancy area southeast and east of Cape Cod and a smaller high occupancy area northeast of Cape Cod. However, high occupancy area expanded southward in the winter and spring and also rotated eastward to include most of Georges Bank in the spring (Figure 2B).
Figure 2. Maps of activity space (95% kernel density estimate; dark outer polygons), high occupancy area (50% kernel density estimate; light inner polygons), and regularized state-space model location estimates (points) for porbeagles tagged in the Northwest Atlantic during the following seasons: winter (A; n = 16 sharks), spring (B; n = 18 sharks), summer (C; n = 22 sharks), and fall (D; n = 18 sharks). The 200 m depth contour is represented by blue lines and the exclusive economic zones (EEZ) are represented by grey lines.
Figure 3. Map showing the seasonal overlap in high occupancy areas (50% kernel density estimates) for tagged porbeagles. The 200 m depth contour is represented by blue lines and the exclusive economic zones (EEZ) are represented by grey lines.
Table 2. Seasonal activity space (95% kernel density estimate) and high occupancy area (50% kernel density estimate) size for tagged porbeagles.
Based on SSM location estimates, most tagged porbeagles (85%; n = 22) stayed within approximately 500 km of their tagging location, regardless of life stage (Supplementary Figure S4). Non-gravid mature females and mature females of unknown reproductive states had the highest proportions of SSM locations in U.S. waters, 100% and 96.3% respectively. Gravid females, juveniles, and mature males had similar proportions of SSM locations in U.S. waters, 88.1%, 88.1%, and 87.3%, respectively. Alternatively, mature males, gravid females, and juveniles had higher proportions of location estimates in Canadian waters (12.7%, 11.8%, and 11.3%, respectively) compared to females of unknown reproductive state and non-gravid mature females (0.7% and 0% respectively). Finally, mature females of unknown reproductive state had the highest proportion of locations in the high seas (3.0%), followed by juveniles (0.6%) and gravid females (0.1%). Mature males and non-gravid mature females did not have any SSM location estimates in the high seas.
Although the geographic ranges of porbeagles were relatively similar among life stages and were restricted in general, seasonal latitudinal and longitudinal movements appeared to be most prevalent in juveniles and mature females that were either non-gravid or were of unknown reproductive state (Figure 4). In particular, these life stages occupied more southern latitudes during the winter and/or spring, and more northern latitudes during the summer and fall (Figure 4). Additionally, these life stages occupied larger longitudinal ranges during the winter and/or spring compared to the summer and fall (Figure 4). On the other hand, mature males and gravid females exhibited relatively minor latitudinal and longitudinal shifts throughout the year compared to the other life stages, and gravid females had the smallest and most consistent latitudinal range in this study (Figure 4).
Figure 4. Seasonal latitudinal (A) and longitudinal (B) distribution for regularized state-space model location estimates for tagged porbeagles, grouped by life stage. Circles represent outliers.
A high occupancy area was concentrated around Cape Cod, MA for all life stages (Figure 5) and there was some degree of spatial overlap in high occupancy areas among all groups except between gravid and non-gravid mature females (Figure 5). Additionally, activity space overlapped between life stages but varied in size (Figure 6; Table 3). Mature males (Figure 6B) and gravid females (Figure 6E) had the smallest activity spaces, and gravid females also had the smallest high occupancy areas (Table 3). The activity spaces for these life stages were almost exclusively in continental shelf waters from ME to southern MA (Figures 6B, E). High occupancy area was concentrated northeast, east, and southeast of Cape Cod, MA and the northern edge of Georges Bank for mature males (Figure 6B) and southeast of Cape Cod, MA for gravid females (Figure 6E). Non-gravid mature females had the next largest activity space (Table 3), ranging from southern ME to NJ and extending south of Georges Bank into offshore environments (Figure 6D). High occupancy area for this life stage was exclusively in shelf waters north of Cape Cod, MA (Figure 6D). Juveniles (Figure 6A) and mature females of unknown reproductive states (Figure 6C) had the largest activity spaces and high occupancy areas (Table 3). The juvenile activity space encompassed the entire Gulf of Maine, the continental shelf region from southern ME to NJ, and extended south of Georges Bank into offshore environments (Figure 6A). Juvenile high occupancy areas surrounded Cape Cod, MA, the northern edge of Georges Bank, and a small area off of southern ME (Figure 6A). Mature females of unknown reproductive state had three main activity space areas (Figure 6C). The largest area encompassed the continental shelf region from southern ME to southern MA and extended south of Georges Bank into offshore habitat (Figure 6C). A second area included waters surrounding the Delaware Bay, and a third area was present southeast of Bermuda at approximately 31°N, 56°W (Figure 6C). However, the high occupancy area of this group was southeast of Cape Cod, MA (Figure 6C).
Figure 5. Map showing the overlap in high occupancy areas (50% kernel density estimates) across life stages for tagged porbeagles. The 200 m depth contour is represented by blue lines and the exclusive economic zones (EEZ) are represented by grey lines.
Figure 6. Maps of activity space (95% kernel density estimate; dark outer polygons), high occupancy area (50% kernel density estimate; light inner polygons), and regularized state-space model location estimates (points) for tagged porbeagles in the Northwest Atlantic of the following life stages: juveniles (A; n = 10 sharks), mature males (B; n = 4 sharks), mature females of unknown reproductive state (C; n = 3 sharks), mature non-gravid females (D; n = 2 sharks), and gravid females (E; n = 7 sharks). The 200 m depth contour is represented by blue lines and the exclusive economic zones (EEZ) are represented by grey lines.
Table 3. Life-stage-based activity space (95% kernel density estimate) and high occupancy area (50% kernel density estimate) size for tagged porbeagles.
Our study provides one of the first comparative analyses on the movements and habitat use of a large elasmobranch across all of its life history stages, including female reproductive states, and is the first study to do so for porbeagle sharks within the NW Atlantic. While sample size was limited among life history stages (particularly YOY), these data help address knowledge gaps for the population and can be incorporated into future large-scale comparative studies. Taken together, our results indicate relatively restricted space use for a pelagic shark and a high degree of overlap in habitat use among life stages and seasons. There was, however, also some evidence of population structuring by life stage in the extent and location of activity spaces and high occupancy areas for porbeagles in this region. Tagged porbeagles were present on the continental shelf surrounding the northeastern U.S. year-round, although movements into offshore environments were occasionally recorded in the winter and spring for some life stages. While limited to just a few individuals, larger-scale movements and broader distributions were mainly observed in juveniles and mature females that were of unknown reproductive state.
Many previous studies on the movement ecology of sharks have found evidence of ontogenetic differences in habitat use, including changes in environments used (i.e., shifts from estuarine nurseries to coastal or oceanic habitats, latitudinal shifts; Bansemer and Bennet, 2011; Ajemian et al., 2020; Franks et al., 2021; Kock et al., 2022) and/or by expansions in space use as sharks grow (e.g., Speed et al., 2010; Bansemer and Bennet, 2011; Franks et al., 2021; Kock et al., 2022). Interestingly, activity space and high occupancy area were actually larger for juveniles compared to most mature life stages in our study. Two previous studies have tagged juvenile porbeagles with PSATs in the NW Atlantic to assess their horizontal habitat use. Campana et al. (2010) found juvenile porbeagles predominantly occupied continental shelf habitats, although tag deployments were limited to summer through early winter and thus may have missed offshore movements for this life stage. In comparison, Skomal et al. (2021) found large juveniles to be relatively shelf-oriented in the summer and fall, but make seasonal offshore movements into mesopelagic environments during the winter and spring. Our results most closely align with those found by Skomal et al. (2021), although the KUDs for our juvenile porbeagles were smaller and did not overlap with the regions used by juvenile porbeagles tagged in Skomal et al. (2021). Juvenile porbeagles were tagged in U.S. coastal waters from ME to MA in our study, whereas juvenile porbeagles were tagged on Georges Bank in Skomal et al. (2021), and on Georges Bank, the Scotian Shelf, and the Grand Banks in Campana et al. (2010). Tagging location could thus have a large influence on any comparison among juvenile habitat use from these three studies, as they potentially sampled from different components of the juvenile porbeagle population in the NW Atlantic.
Mature male porbeagles had the second smallest activity space in this study, which appeared to be restricted to the Gulf of Maine, Georges Bank, and shelf waters south of MA. High occupancy areas included continental shelf waters around Cape Cod, MA. There were a very limited number of SSM location estimates in offshore habitats for this subset of tagged individuals. Although tracking data for mature male porbeagles is limited globally, the spatial patterns of animals tagged in this study were similar to previous tracking data for this life stage in the NW Atlantic (Campana et al., 2010) and the South Pacific (Francis et al., 2015). For example, Francis et al. (2015) found no evidence of a seasonal latitudinal migration for the two mature males tagged in their study. Of the two mature males tagged by Campana et al. (2010) in the NW Atlantic, one remained in continental shelf waters for the duration of the tag deployment while another moved southeast to approximately 37°N, 55°W (northeast of Bermuda) in January. Most of the mature males tagged in this study remained in continental shelf waters year-round, although raw, unfiltered location estimates suggested one mature male (Shark 24; ID 175432) migrated south to approximately 33°N, 65°W (north of Bermuda) during late December of 2022 and again moved south of Georges Bank into offshore waters (approximately 39°N, 69.6°W) in late December of 2023. Collectively, mature male porbeagles appear to be mostly shelf-oriented in the NW Atlantic, but further tagging efforts are needed to determine the frequency of southward migrations for this life stage.
Campana et al. (2010) found that mature female porbeagles (which were assumed gravid, but not confirmed) made extensive southward migrations to the Sargasso Sea during the spring. Based on the timing and the disparity in movements between those mature females and other life stages, Campana et al. (2010) suggested that mature females were migrating to the Sargasso Sea for parturition. However, YOY porbeagles are found in the continental shelf waters of the U.S. and Canada by June or early July (Natanson et al., 2002; Anderson et al., 2021a, 2024a). Any metabolic or evolutionary advantage of the suggestion that gravid porbeagles migrate 2,000 km to give birth in unproductive subtropical waters, followed by a return migration for the post-partum females and neonates within a couple months of parturition (Campana et al., 2010), remains unidentified. Furthermore, the fin-mount tracking data collected from our confirmed gravid porbeagles differ substantially from this pattern. Gravid porbeagles had the smallest activity space and high occupancy area of all life stages tagged in this study. Activity space for this reproductive stage was concentrated in the Gulf of Maine and Georges Bank, while high occupancy area included the region southeast of Cape Cod, MA. Moreover, while tracking data during the expected pupping season (April through June) were limited to a few individuals, based on the available fin-mount tag transmissions during this time, gravid females did not appear to be in the Sargasso Sea. As such, it is possible that at least some of the gravid females tagged in this study did not migrate to give birth.
Although tag data was very limited for this reproductive stage, the non-gravid mature female porbeagles had a relatively large activity space and high occupancy area over their short tag durations (1-3 months) and one non-gravid mature female dispersed over 450 km within 8 days of tagging. The activity space of non-gravid mature females was approximately two times larger than those for gravid females. The activity space of non-gravid mature females included the southwestern Gulf of Maine, western Georges Bank, shelf waters near New York and NJ, and offshore habitat south of Georges Bank. The high occupancy area for non-gravid mature females was north of Cape Cod, MA, including Stellwagen Bank, and did not overlap with the high occupancy area of confirmed gravid females. A previous study (Natanson et al., 2019) performed dissections on several female porbeagles caught in the area of Stellwagen Bank to assess maturity and reproductive state. This subset of mature female porbeagles were found to be reproductively resting (i.e., not actively undergoing vitellogenesis, ovulation or gestation), suggesting that Stellwagen Bank was a geographically segregated habitat used by mature females during a resting phase of the reproductive cycle (Natanson et al., 2019). Although sample size is limited, the tracking data from our non-gravid mature females support the previous work suggesting Stellwagen Bank may be an important habitat for non-gravid mature female porbeagles (Natanson et al., 2019).
Three female porbeagles tagged in this study were above the size at 50% maturity but were not formally assessed for reproductive state. These sharks made the most extensive movements and had the largest activity space in this study. For example, two of the three mature females of unknown reproductive states dispersed over 500 km from the tagging location, with one (Shark 24; 172014) dispersing approximately 650 km and one (Shark 26; 220982) dispersing over 1,600 km. The activity space of this group included the western Gulf of Maine, Georges Bank, shelf waters near NJ, and extended into offshore habitats, including a region southeast of Bermuda. Interestingly, the activity space of mature females of unknown reproductive state included habitats that were important to both gravid females (southeast of Cape Cod, MA) and non-gravid mature females (Stellwagen Bank) tagged in this study. It is possible that some of the mature females that were not assessed for reproductive state were gravid while some were resting. However, there was some degree of overlap in the activity spaces of gravid and non-gravid mature females, so it was not possible to predict the reproductive states of these unassessed females based on movement patterns. Overall, this work highlights that making assumptions about the reproductive states of sharks is unadvisable and demonstrates the importance of combining telemetry with formal assessment of reproductive state (i.e., via ultrasound) when attempting to identify reproductive habitats for this taxon. Otherwise, inaccurate assumptions about the geographic locations associated with reproduction may be used as the basis for conservation and management decisions.
The current understanding of NW Atlantic porbeagle reproductive movements and pupping grounds is based on the tracking data of females of unknown reproductive states. While we recognize that the population was thought to reproduce annually (Jensen et al., 2002) and there was limited accessibility of non-lethal reproductive analyses (Hammerschlag and Sulikowski, 2011) at the time of the previous work, the conclusions provided in Campana et al. (2010) nevertheless require further evaluation given their relevance to conservation and management of the population. It is important to consider that direct comparisons between studies are complicated by differences in tag types (and their different capabilities) and tagging locations for mature females (northeastern U.S. in our study, eastern Georges Bank and the Scotian Shelf in Campana et al. (2010)). Nevertheless, movement patterns made by mature female porbeagles tagged in Campana et al. (2010) were more comparable to the patterns observed in the non-gravid mature females in this study rather than the gravid females, even while considering data for non-gravid females was limited to a few months. For example, several of the mature females tagged in Campana et al. (2010) visited Stellwagen Bank during the tag deployment, a documented female resting habitat (Natanson et al., 2019). Although none of the SSM location estimates from our tagged females were as far south as the Sargasso Sea, when considering raw, unfiltered fin-mount tag transmission data, one of the non-gravid mature females (Shark 38; ID 221129) migrated south near Bermuda during the winter before suffering mortality. However, given the lack of SSM location estimates for our tagged non-gravid females in winter and early spring, further tagging of this life stage is needed to assess their habitat use patterns throughout the year.
While we did not document movements as far south into the Sargasso Sea, it is possible that the mature females tagged by Campana et al. (2010) were not gravid given the general similarity to our results. However, we also cannot rule out the possibility that there are different behavioral characteristics among gravid females, in which a portion of the population migrates for parturition, while another portion remains in cold-temperate waters to give birth (i.e., McMillan et al., 2019), especially considering mature female porbeagles were tagged in different locations in our study compared to Campana et al. (2010). For example, previous research has found evidence of partial migration for gravid female school sharks Galeorhinus galeus in Australia (McMillan et al., 2019) and blacktip reef sharks C. melanopterus in French Polynesia (Mourier and Planes, 2013), in which some females migrate to give birth while others remain near aggregation sites. Moreover, given the broad distributions of YOY shortfin makos (Natanson et al., 2020) and common thresher sharks Alopias vulpinas (Kneebone et al., 2020) in the Northwest Atlantic, including during their expected pupping seasons, it is possible that lamnid sharks, including porbeagles, may not exhibit distinct reproductive migrations or have discrete pupping grounds that have been documented in coastal species.
We discovered several limitations regarding the use of fin-mount satellite tags for porbeagles. First, as with all fin-mount satellite tag studies, deployment lengths were not consistent among individuals, with tag transmission lengths ranging from approximately four days to two years. While this was partially mitigated by not including sharks with fewer than 10 track days into habitat use analyses, it is important to note that our understanding of habitat use (e.g., KDEs) in this study will be biased to an extent toward individuals with longer track durations. Additionally, the utility of these finmount tags was particularly low for YOY porbeagles, as only one of our tagged YOY sharks had enough tag transmissions to be included in habitat use analyses. We do not believe the low number of YOY shark tag transmissions was related to time spent at the sea surface, as YOY porbeagles have been found to spend greater time in surface waters than larger conspecifics (Anderson et al., 2024a). We also do not believe initial capture- and tagging-induced mortality was a principal factor in the lack of transmissions for tags deployed on YOY sharks in our study given post-release survival of YOY and immature porbeagles has been found to be high (100%) using rod-and-reel (Anderson et al., 2021a). However, we recognize that data are lacking on the impact of SPOT tags on survival of YOY sharks in general. Still, we suspect that the fin-mount tag attachment style is more likely leading to reduced transmissions from tags on YOY sharks, as it is likely that the dorsal fins of YOY porbeagles are not large enough or dense enough to support the weight of the fin-mount tag for extended periods (e.g., Anderson et al., 2021b). Additionally, rapid growth during early life stages (i.e., Natanson et al., 2002) could cause the fin-mount tags to fall off the dorsal fin as it grows. In an attempt to mitigate these concerns, a prototype fin-mount tag design (Sirtrack Kiwisat K4) that is much smaller and lighter (and is designed to move on the fin as the shark grows) was deployed on two YOY porbeagles (shark 1; 221902 and shark 28; 221906) at the later stage of this study. One of these YOY sharks (shark 1; 221902) fitted with the prototype tag design provided enough transmissions to be used in habitat use analyses, although data from this individual was still limited.
Another limitation of the fin-mount tags was the sparsity of transmissions when porbeagles were offshore of the continental shelf (Braun et al., 2023). Raw, unprocessed fin-mount tag data, as well as PSAT data for double-tagged sharks (unpublished data, B. Anderson and J. Sulikowski), indicated that several of our porbeagles occupied waters offshore of the continental shelf for several weeks during the winter and spring, but fin-mount transmissions were infrequent and had gaps that exceeded our SSM threshold during these forays, preventing incorporation into habitat use analyses. Previous studies using PSATs have also found porbeagles to migrate south into offshore waters and these sharks exhibited a submergence diving behavior in which they infrequently inhabited surface waters (Campana et al., 2010; Skomal et al., 2021; Braun et al., 2023; Anderson et al., 2024a, b). It is likely that porbeagles in this study exhibited similar submergence behavior when in warmer offshore waters that caused the infrequent transmission from fin-mount satellite tags. This represents a major limitation of using fin-mount tags to study the habitat use of pelagic sharks which have displayed surface-avoidance behavior. Given this consideration, it is important to note that the KUDs calculated in this study are likely to be underestimated during the winter and spring, may be missing habitats used by porbeagles during offshore excursions, and may overall be biased toward shelf-based habitats. Nevertheless, the major benefit to using fin-mount tags to assess porbeagle habitat use in this study was the high location accuracy, which enabled the consistent identification of rather fine-scale important habitat within a national boundary that can have direct management impacts. Overall, based on these findings, future studies deploying multiple tag types (fin-mount tags, PSATs) would best optimize the accuracy and amount of location estimates for this population, particularly during winter and spring.
Conservation and management of highly mobile sharks, such as porbeagles, requires a detailed understanding of spatial dynamics. Data on movement patterns can inform vulnerability to interactions with fisheries as well as provide support for the development of spatial management strategies (e.g., Cortés et al., 2010; Bowlby et al., 2020b). For example, space use of porbeagles in this study was limited, which increases vulnerability while also making the population a candidate for spatial management. The overall 50% KDE for our porbeagles was approximately 2-3.5 times smaller than those for great hammerheads Sphyrna mokarran and tiger sharks (Graham et al., 2016), and seasonal 50% and 95% KDEs for our porbeagles were approximately 1.5-26 times smaller than for closely related salmon sharks L. ditropis (Weng et al., 2008) and shortfin makos (Kresge, 2024). Moreover, the vast majority of our porbeagle SSM location estimates were within U.S. waters (89.7%), with limited location estimates occurring in Canadian waters (9.5%), and even fewer in the high seas (<1%), suggesting that national regulations on porbeagles may be effective at supporting recovery. However, this finding differs from previous research suggesting NW Atlantic porbeagles frequently cross national jurisdictions (i.e., Campana et al., 2010; Campana, 2016; Kohler and Turner, 2019; Skomal et al., 2021), and may be partially related to limitations of the tagging method biasing SSM location estimates toward continental shelf habitats. It is likely that the proportion of time spent in the high seas is underestimated in this study due to reduced fin-mount transmissions while porbeagles are offshore. Regardless, this is the first study to deploy satellite tags on NW Atlantic porbeagle sharks west of Georges Bank and therefore provides electronic tag-based movement data on a relatively understudied component of the population. Our results suggest it is likely that these individuals behave differently than those occupying more eastern portions of the populations’ range.
Essential fish habitat (EFH) is defined under the U.S. Magnuson-Stevens Act as habitat required for spawning/breeding, feeding, and/or growth (NMFS, 1996). EFH is mandated to be identified and described for each life stage of a managed species, and adverse effects on such habitat is to be minimized to the extent practicable (NMFS, 1996). EFH was first described for porbeagles (of all life stages combined) in 1999 (NMFS, 2009) and was most recently updated in 2017 to include the Gulf of Maine (excluding Cape Cod Bay and Massachusetts Bay) and shelf-break habitats from Georges Bank to NJ (NMFS, 2017). The coastal waters north and south of Cape Cod, MA are not included in the current map of porbeagle EFH, yet these areas were important habitat year-round for porbeagles tagged in this study, as they were consistently identified as high occupancy areas. The coastal habitat south of Cape Cod, MA is particularly significant to at least a portion of this population given its apparent importance to gravid females. The data from this study support the future inclusion of the coastal waters north and south of Cape Cod, MA into the NW Atlantic porbeagle EFH.
In addition to consideration for designation of EFH, the relatively small high occupancy area surrounding Cape Cod, MA warrants further attention given the concentration of fishing activity (rod-and-reel, trawl, gillnet; NOAA, 2023) that occurs year-round within this region. The overlap between porbeagle high occupancy areas and U.S. fishing activity suggests vulnerability to capture is high (e.g., Queiroz et al., 2019). A recent (2021) U.S. catch statistics report indicate an estimated 1.2 metric tons (mt) of porbeagle were landed in the recreational rod-and-reel fishery while 25 individuals were discarded, and 1 mt were caught in pelagic longline fisheries (NOAA, 2023). While gillnet and trawl catch data are limited to a number of observed sets, porbeagle is the most frequently bycaught highly migratory shark in these fisheries in the Northeast region of the U.S (NOAA, 2023). In 2021 for example, 202 individuals were discarded in 251 observed gillnet sets and 132 individuals were discarded in 208 observed bottom otter trawl trips (41% of which were dead at discard) (NOAA, 2023). At least four (10.5%) of the porbeagles tagged in our study were confirmed or predicted to be recaptured in U.S. fisheries within several months of tagging, providing evidence of high fishing pressure on the stock within the U.S. that warrants further exploration. Recaptured porbeagles included one YOY believed to be illegally landed based on tag transmissions, one juvenile (larger than the minimum legal size limit) caught dead in a bottom trawl, one gravid female caught dead in a gillnet, and one non-gravid mature female believed to be landed based on tag transmissions. Although 75% of the captured sharks were above the legal size limit, most of them were caught as bycatch and suffered at-vessel mortality. Moreover, this work raises questions regarding the frequency of illegal landing of undersized porbeagles. Overall, this study highlights the need to quantify porbeagle mortality (including post-release mortality) in fisheries in the region, and data are particularly scarce for gillnet and otter trawl fisheries. Most work on porbeagle bycatch mortality has been done in pelagic longline fisheries, with at-vessel mortality estimated at approximately 44% and post-release mortality estimates varying from approximately 14-27.2% depending on the fishery (e.g., Gallagher et al., 2014; Campana et al., 2016; Bowlby et al., 2020a). Despite limited sample size, the tracking data presented here should help justify the need for a spatial management strategy for the high occupancy area surrounding Cape Cod, MA to promote population recovery. For example, U.S. fisheries management entities should consider the high occupancy area identified in Figure 1 (white polygon) for porbeagle spatial protective measures.
The raw data supporting the conclusions of this article will be made available by the authors, without undue reservation.
The animal study was approved by Arizona State University Institutional Animal Care and Use Committee. The study was conducted in accordance with the local legislation and institutional requirements.
BA: Conceptualization, Data curation, Formal analysis, Funding acquisition, Investigation, Methodology, Project administration, Visualization, Writing – original draft, Writing – review & editing. NH: Investigation, Methodology, Resources, Writing – review & editing. SS: Writing – review & editing. JD: Conceptualization, Funding acquisition, Investigation, Methodology, Project administration, Resources, Writing – review & editing. HB: Writing – review & editing. YK: Writing – review & editing. AG: Data curation, Investigation, Resources, Writing – review & editing. JS: Conceptualization, Funding acquisition, Investigation, Methodology, Project administration, Resources, Supervision, Writing – review & editing.
The author(s) declare that financial support was received for the research and/or publication of this article. The authors gratefully acknowledge the American Elasmobranch Society Donald R. Nelson award and the Arizona State University Graduate and Professional Student Associate Graduate Research and Support Program for contributing funds for this research.
We would like to thank boat captains, particularly W. Hatch, S. Riddle, and D. Johnson, and crew members for providing access to fishing vessels and assistance in the fieldwork for this study. We also gratefully acknowledge graduate and undergraduate students of the Sulikowski Shark and Fish Research Lab that assisted in the fieldwork for this study, particularly H. Verkamp, S. Hylton, and B. Campbell. Thank you to the Animal Telemetry Network for providing satellite time and the fishermen who were willing to share recapture information and return tags. All capture, handling, and sampling protocols were approved by the Arizona State University Institutional Animal Care and Use Committee (20-1745R). Research activities were conducted with a NMFS letter of acknowledgement.
The authors declare that the research was conducted in the absence of any commercial or financial relationships that could be construed as a potential conflict of interest.
The author(s) declare that no Generative AI was used in the creation of this manuscript.
All claims expressed in this article are solely those of the authors and do not necessarily represent those of their affiliated organizations, or those of the publisher, the editors and the reviewers. Any product that may be evaluated in this article, or claim that may be made by its manufacturer, is not guaranteed or endorsed by the publisher.
The Supplementary Material for this article can be found online at: https://www.frontiersin.org/articles/10.3389/fmars.2025.1553509/full#supplementary-material
Aesen O. (1963). Length and growth of the porbeagle (Lamna nasus, Bonnaterre) in the North West Atlantic. Rep. Norwegian Fisheries Mar. Investigations 13, 20–37.
Ajemian M. J., Drymon J. M., Hammerschlag N., Wells R. J. D., Street G., Falterman B., et al. (2020). Movement patterns and habitat use of tiger sharks (Galeocerdo cuvier) across ontogeny in the Gulf of Mexico. PloS One 15, e0234868. doi: 10.1371/journal.pone.0234868
Anderson B. N., Bowlby H. D., Natanson L. J., Coelho R., Cortes E., Domingo A., et al. (2021a). Preliminary estimate of post-release survival of immature porbeagles caught with rod-and-reel in the Northwest Atlantic Ocean. Mar. Ecol. Prog. Ser. 660, 153–159. doi: 10.3354/meps13603
Anderson B. N., Bowlby H. D., Saul S., Kang Y., Hammerschlag N., Natanson J. L., et al. (2024a). First insights into the vertical habitat use of young porbeagles in the north-western Atlantic with implications for bycatch reduction strategies. Mar. Freshw. Res. 75, Mf23182. doi: 10.1071/MF23182
Anderson J. M., Burns E. S., Meese E. N., Farrugia T. J., Stirling B. S., White C. F., et al. (2021b). Interannual nearshore habitat use of young of the year white sharks off southern California. Front. Mar. Sci. 8. doi: 10.3389/fmars.2021.645142
Anderson B. N., Horstmyer L., Ballard K. L., Dodd J. F., Sulikowski J. A. (2024b). First evidence of predation on an adult porbeagle equipped with a pop-off satellite archival tag in the Northwest Atlantic. Front. Mar. Sci. 11. doi: 10.3389/fmars.2024.1406973
Bansemer C. S., Bennet M. B. (2011). Sex- and maturity-based differences movement and migration patterns of grey nurse shark, Carcharias taurus, along the eastern coast of Australia. Mar. Freshw. Res. 62, 596–606. doi: 10.1071/MF10152
Birkmanis C. A., Partridge J. C., Simmons L. W., Heupel M. R., Sequeira A. M. M. (2020). Shark conservation hindered by lack of habitat protection. Glob. Ecol. Conserv. 21, e00862. doi: 10.1016/j.gecco.2019.e00862
Bortoluzzi J. R., McNicholas G. E., Jackson A. L., Klocker C. A., Ferter K., Junge C., et al. (2024). Transboundary movements of porbeagle sharks support need for continued cooperative research and management approaches. Fish. Res. 275, 107007. doi: 10.1016/j.fishres.2024.107007
Bowlby H., Joyce W., Benoit H., Sulikowski J. (2020a). Evaluation of post-release mortality for porbeagle and shortfin mako sharks from the Canadian pelagic longline fishery. ICCAT Col. Vol. Sci. Papers 76, 365–373.
Bowlby H. D., Joyce W. N., Winton M. V., Coates P. J., Skomal G. B. (2022). Conservation implications of white shark (Carcharodon carcharias) behavior at the northern extent of their range in the Northwest Atlantic. Can. J. Fish. Aquat. Sci. 79, 1843–1859. doi: 10.1139/cjfas-2021-0313
Bowlby H. D., Taylor N., Carlson J. (2020b). Quantifying horizontal overlap between longline fleets and porbeagle distribution for ecological risk assessment. ICCAT Col. Vol. Sci. Papers 77, 169–179.
Bowler D. E., Benton T. G. (2005). Causes and consequences of animal dispersal strategies: relating individual behaviour to spatial dynamics. Biol. Rev. 80, 205–225. doi: 10.1017/S1464793104006645
Braun C. D., Penna A. D., Arostegui M. C., Afonso P., Berumen M. L., Block B. A., et al. (2023). Linking vertical movements of large pelagic predators with distribution patterns of biomass in the open ocean. PNAS 120, e2306357120. doi: 10.1073/pnas.2306357120
Campana S. E. (2016). Transboundary movements, unmonitored fishing mortality, and ineffective internal fisheries management pose risks for pelagic sharks in the Northwest Atlantic. Can. J. Fish. Aquat. Sci. 73, 1599–1607. doi: 10.1139/cjfas-2015-0502
Campana S. E., Fowler M., Houlihan D., Joyce W. N., Showell M., Simpson M., et al. (2015). Recovery potential assessment for porbeagle (Lamna nasus) in Atlantic Canada. DFO Can. Sci. Advis. Sec. Res. Doc., iv + 45p.
Campana S. E., Joyce W., Fowler M. (2010). Subtropical pupping ground for a cold-water shark. Can. J. Fish. Aquat. Sci. 67, 769–773. doi: 10.1139/F10-020
Campana S. E., Joyce W., Fowler M., Showell M. (2016). Discards, hooking, and post-release mortality of porbeagle (Lamna nasus), shortfin mako (Isurus oxyrinchus), and blue shark (Prionace glauca) in the Canadian pelagic longline fishery. ICES J. Mar. Sci. 73, 520–528. doi: 10.1093/icesjms/fsv234
Campana S. E., Joyce W., Marks L., Natanson L. J., Kohler N. E., Jensen C. F., et al. (2002). Population dynamics of the porbeagle in the Northwest Atlantic Ocean. N. Am. J. Fish. Manage. 22, 106–121. doi: 10.1577/1548-8675(2002)022<0106:PDOTPI>2.0.CO;2
Cartamil D., Wegner N. C., Kacev D., Ben-aderet N., Kohin S., Graham J. B. (2010). Movement patterns and nursery habitat of juvenile thresher sharks Alopias vulpinus in the Southern California Bight. Mar. Ecol. Prog. Ser. 404, 249–258. doi: 10.3354/meps08495
Chapman D. D., Feldheim K. A., Papastamatiou Y. P., Hueter R. E. (2015). There and back again: a review of residency and return migrations in sharks, with implications for population structure and management. Annu. Rev. Mar. Sci. 7, 547–570. doi: 10.1146/annurev-marine-010814-015730
Cortés E., Arocha F., Beerkircher L., Carvalho F., Domingo A., Heupel M., et al. (2010). Ecological risk assessment of pelagic sharks caught in Atlantic pelagic longline fisheries. Aquat. Living Resour. 23, 25–34. doi: 10.1051/alr/2009044
COSEWIC (2004). COSEWIC assessment and status report on the porbeagle shark Lamna nasus in Canada (Ottawa, Ontario, Canada: Committee on the Status of Endangered Wildlife in Canada), viii+43 p.
COSEWIC (2014). COSEWIC assessment and status report on the porbeagle Lamna nasus in Canada (Ottawa, Onterio, Canada: Committee on the Status of Endangered Wildlife in Canada), xi+40 p.
Crear D. P., Curtis T. H., Durkee S. J., Carlson J. K. (2021). Highly migratory species predictive spatial modeling (PRiSM): an analytical framework for assessing the performance of spatial fisheries management. Mar. Biol. 168, 148. doi: 10.1007/s00227-021-03951-7
Curtis T. H., Laporte S., Cortes E., DuBeck G., McCandless C. (2016). Status review report: porbeagle shark (Lamna nasus). Final Report to National Marine Fisheries Service (Office of Protected Resources).
Dulvy N. K., Pacoureau N., Rigby C. L., Pollom R. A., Jabado R. W., Ebert D. A., et al. (2021). Overfishing drives over one-third of all sharks and rays toward a global extinction crisis. Curr. Biol. 31, 4773–4787. doi: 10.1016/j.cub.2021.08.062
Edgar G., Stuart-Smith R., Willis T., Kininmonth S., Baker S. C., Banks S., et al. (2014). Global conservation outcomes depend on marine protected areas with five key features. Nature 506, 216–220. doi: 10.1038/nature13022
Flanders Marine Institute (2019). Maritime boundaries geodatabase: maritime boundaries and exclusive economic zones (200NM), version 11. doi: 10.14284/386
Francis M. P., Holdsworth J. C., Block B. A. (2015). Life in the open ocean: seasonal migration and diel diving behaviour of Southern Hemisphere porbeagle sharks (Lamna nasus). Mar. Biol. 162, 2305–2323. doi: 10.1007/s00227-015-2756-z
Francis M. P., Natanson L. J., Campana S. E. (2008). “The biology and ecology of the porbeagle shark, Lamna nasus,” in Sharks of the open ocean. Eds. Camhi M. D., Pikitch E. K., Babcock E. A. (Blackwell Publishing Ltd., Oxford), 105–111.
Franks B. R., Tyminski J. P., Hussey N. E., Braun C. D., Newton A. L., Thorrold S. R., et al. (2021). Spatio-temporal variability in white shark (Carcharodon carcharias) movement ecology during residency and migration phases in the western North Atlantic. Front. Mar. Sci. 8. doi: 10.3389/fmars.2021.744202
Gallagher A. J., Orbesen E. S., Hammerschlag N., Serafy J. E. (2014). Vulnerability of oceanic sharks as pelagic longline bycatch. Glob. Ecol. Evol. 1, 50–59. doi: 10.1016/j.gecco.2014.06.003
Gibson K. J., Streich M. K., Topping T. S., Stunz G. W. (2021). New insights into the seasonal movement patterns of shortfin mako sharks in the Gulf of Mexico. Front. Mar. Sci. 8. doi: 10.3389/fmars.2021.623104
Graham F., Rynne P., Estevanez M., Luo J., Ault J. S., Hammerschlag N. (2016). Use of marine protected areas and exclusive economic zones in the subtropical western North Atlantic Ocean by large highly mobile sharks. Diversity Distrib. 22, 534–546. doi: 10.1111/ddi.2016.22.issue-5
Hammerschlag N., Gallagher A. J., Lazarre D. M. (2011). A review of shark satellite tagging studies. J. Exp. Mar. Biol. Ecol. 398, 1–8. doi: 10.1016/j.jembe.2010.12.012
Hammerschlag N., Luo J., Irschick D. J., Ault J. S. (2012). A comparison of spatial and movement patterns between sympatric predators: bull sharks (Carcharhinus leucas) and Atlantic tarpon (Megalops atlanticus). PloS One 7(9):e45958. doi: 10.1371/journal.pone.0045958
Hammerschlag N., McDonnell L. H., Rider M. J., Street G. M., Hazen E. L., Natanson L. J., et al. (2022). Ocean warming alters the distributional range, migratory timing, and spatial protections of an apex predator, the tiger shark (Galeocerdo cuvier). Global Change Biol. 28, 1990–2005. doi: 10.1111/gcb.16045
Hammerschlag N., Sulikowski J. (2011). Killing for conservation: the need for alternatives to lethal sampling of apex predatory sharks. Endanger. Species Res. 14, 135–140. doi: 10.3354/esr00354
Haugen J. B. (2020). Fishery management, conservation, and bycatch of North Atlantic porbeagle (Dartmouth (MA: University of Massachusetts Dartmouth).
Hurley P. C. F. (1998). A review of the fishery for pelagic sharks in Atlantic Canada. Fish. Res. 39, 107–113. doi: 10.1016/S0165-7836(98)00177-5
ICCAT (2015). Recommendation by ICCAT on porbeagle caught in association with ICCAT Fisheries. Recommendation 15-06. International Commission for the Conservation of Atlantic Tunas, Madrid.
Jaiteh V. F., Lindfield S. J., Mangubhai S., Warren C., Fitzpatrick B., Loneragan N. R. (2016). Higher abundance of marine predators and changes in fishers’ behavior following spatial protection within the world’s biggest shark fishery. Front. Mar. Sci. 3. doi: 10.3389/fmars.2016.00043
Jensen C. F., Natanson L. J., Pratt H. L. Jr., Kohler N., Campana S. E. (2002). The reproductive biology of the porbeagle shark (Lamna nasus) in the western North Atlantic Ocean. Fish. Bull. 100, 727–738.
Jonsen I. D., Grecian W. J., Phillips L., Carroll G., McMahon C., Harcourt R. G., et al. (2023). aniMotum, an R package for animal movement data: rapid quality control, behavioural estimation and simulation. Methods Ecol. Evol. 14, 806–816. doi: 10.1111/2041-210X.14060
Jorgensen S. J., Micheli F., White T. D., Van Houtan K. S., Alfaro-Shigueto J., Andrzejaczek S., et al. (2022). Emergent research and priorities for shark and ray conservation. Endanger. Species Res. 47, 171–203. doi: 10.3354/esr01169
Kneebone J., Bowlby H., Mello J., McCandless C. T., Natanson L. J., Gervelis B., et al. (2020). Seasonal distribution and habitat use of the common thresher shark (Alopias vulpinus) in the western North Atlantic Ocean inferred from fishery-dependent data. Fish. Bull. 118, 399–412. doi: 10.7755/FB.118.4.8
Kock A. A., Lombard A. T., Daly R., Goodall V., Meÿer M., Johnson R., et al. (2022). Sex and size influence the spatiotemporal distribution of white sharks, with implications for interactions with fisheries and spatial management in the Southwest Indian Ocean. Front. Mar. Sci. 9. doi: 10.3389/fmars.2022.811985
Kohler N. E., Turner P. A. (2019). Distributions and movements of Atlantic shark species: a 50-year retrospective atlas of mark and recapture data. Mar. Fish. Rev. 81, 1–93. doi: 10.7755/MFR.81.2.1
Kresge C. D. (2024). Investigating the movements, behaviors, and interactions with fisheries of the endangered shortfin mako shark in the western North Atlantic Ocean. University of Rhode Island, South Kingstown (RI.
Maxwell S. M., Scales K. L., Bograd S. J., Briscoe D. K., Dewar H., Hazen E. L., et al. (2019). Seasonal spatial segregation in blue sharks (Prionace glauca) by sex and size class in the Northeast Pacific Ocean. Diversity Distributions 25, 1304–1317. doi: 10.1111/ddi.12941
McMillan M. N., Huveneers C., Semmens J. M., Gillanders B. M. (2019). Partial female migration and cool-water migration pathways in an overfished shark. ICES J. Mar. Sci. 76, 1083–1093. doi: 10.1093/icesjms/fsy181
Mourier J., Planes S. (2013). Direct evidence for reproductive philopatry and associated fine-scale migrations in female blacktip reef sharks (Carcharhinus melanopterus) in French Polynesia. Mol. Ecol. 22, 201–214. doi: 10.1111/mec.2012.22.issue-1
Natanson L. J., Deacy B. M., Joyce W., Sulikowski J. (2019). Presence of resting population of female porbeagles (Lamna nasus), indicating a biennial reproductive cycle, in the western North Atlantic Ocean. Fish. Bull. 117, 70–77. doi: 10.7755/FB.117.1-2.8
Natanson L. J., Mello J. J., Campana S. E. (2002). Validated age and growth of the porbeagle shark (Lamna nasus) in the western North Atlantic Ocean. Fish. Bull. 100, 266–278.
Natanson L. J., Winton M., Bowlby H., Joyce W., Deacy B., Coelho R., et al. (2020). Updated reproductive parameters for the shortfin mako (Isurus oxyrinchus) in the North Atlantic Ocean with inferences of distribution by sex and reproductive stage. Fish. Bull. 118, 21–36. doi: 10.7755/FB.118.1.3
NMFS (1996). Magnuson–stevens fishery conservation and management act. NOAA technical memorandum NMFS-F/SPO-23 (Washington, DC: US Government Printing Office).
NMFS (2007). Final amendment 2 to the consolidated Atlantic highly migratory species fishery management plan (Silver Spring, MD: NOAA, NMFS, Off. Sustainable Fish., Highly Migratory Species Management Division).
NMFS (2009). Amendment 1 to the consolidated Atlantic highly migratory species fishery management plan: essential fish habitat (Silver Spring, MD: NOAA/NMFS), 410 pp.
NMFS (2017). Amendment 10 to the 2006 consolidated Atlantic highly migratory species fishery management plan: essential fish habitat (Silver Spring, MD: NOAA/NMFS), 442 pp.
NOAA (2023). 2022 stock assessment and fishery evaluation (SAFE) report for Atlantic highly migratory species (Silver Spring, MD: NOAA, Atlantic Highly Migratory Species Management Division), 296 p.
Pade N. G., Queiroz N., Humphries N. E., Witt M. J., Jones C. S., Noble L. R., et al. (2009). First results from satellite-linked archival tagging of porbeagle shark, Lamna nasus: area fidelity, wider-scale movements and plasticity in diel depth changes. J. Exp. Mar. Biol. Ecol. 370, 64–74. doi: 10.1016/j.jembe.2008.12.002
Queiroz N., Humphries N. E., Couto A., Vedor M., da Costa I., Sequeira A. M. M., et al. (2019). Global spatial risk assessment of sharks under the footprint of fisheries. Nature 572, 461–466. doi: 10.1038/s41586-019-1444-4
Renshaw S., Hammerschlag N., Gallagher N. J., Lubitz N., Sims D. W. (2023). Global tracking of shark movements, behaviour and ecology: a review of the renaissance years of satellite tagging studies 2010-2020. J. Exp. Mar. Biol. Ecol. 560, 151841. doi: 10.1016/j.jembe.2022.151841
Rigby C. L., Barreto R., Carlson J., Fernando D., Fordham S., Francis M. P., et al. (2019). Lamna nasus. The IUCN red list of threatened species 2019. E.T11200A500969. doi: 10.2305/IUCN.UK.2019-3.RLTS.T11200A500969.en
Shaw A. K. (2020). Causes and consequences of individual variation in animal movement. Move. Ecol. 8, 12. doi: 10.1186/s40462-020-0197
Shea B. D., Gallagher A. J., Bomgardner L. K., Ferretti F. (2023). Quantifying longline bycatch mortality for pelagic sharks in western Pacific shark sanctuaries. Sci. Adv. 9, eadg3527. doi: 10.1126/sciadv.adg3527
Skomal G., Marshall H., Galuardi B., Natanson L., Braun C. D., Bernal D. (2021). Horizontal and vertical movement patterns and habitat use of juvenile porbeagles (Lamna nasus) in the western North Atlantic. Front. Mar. Sci. 8. doi: 10.3389/fmars.2021.624158
Speed C. W., Field I. C., Meekan M. G., Bradshaw C. J. A. (2010). Complexities of coastal shark movements and their implications for management. Mar. Ecol. Prog. Ser. 408, 275–293. doi: 10.3354/meps08581
Sulikowski J. A., Hammerschlag N. (2023). A novel intrauterine satellite transmitter to identify parturition in large sharks. Sci. Adv. 9, eadd6340. doi: 10.1126/sciadv.add6340
Sulikowski J. A., Wheeler C. R., Gallagher A. J., Prohaska B. K., Langan J. A., Hammerschlag N. (2016). Seasonal and life-stage variation in the reproductive ecology of a marine apex predator, the tiger shark Galeocerdo cuvier, at a protected female-dominated site. Aquat. Biol. 24, 175–184. doi: 10.3354/ab00648
Tougaard J., Teilmann J., Tougaard S. (2008). Harbour seal spatial distribution estimated from Argos satellite telemetry: overcoming positioning errors. Endanger. Species Res. 4, 113–122. doi: 10.3354/esr00068
Weng K. C., Foley D. G., Ganong J. E., Perle C., Shillinger G. L., Block B. A. (2008). Migration of an upper trophic level predator, the salmon shark Lamna ditropis, between distant ecoregions. Mar. Ecol. Prog. Ser. 372, 253–274. doi: 10.3354/meps07706
White T. D., Carlisle A. B., Kroodsma D. A., Block B. A., Casagrandi R., De Leo G. A., et al. (2017). Assessing the effectiveness of a large marine protected area for reef shark conservation. Biol. Conserv. 207, 64–71. doi: 10.1016/j.biocon.2017.01.009
Wilson S. G., Stewart B. S., Polovina J. J., Meekan M. G., Stevens J. D., Galuardi B. (2007). Accuracy and precision of archival tag data: a multiple-tagging study conducted on a whale shark (Rhincodon typus) in the Indian Ocean. Fish. Oceanogr. 16, 547–554. doi: 10.1111/j.1365-2419.2007.00450.x
Winship A. J., Jorgensen S. J., Shaffer S. A., Jonsen I. D., Robinson P. W., Costa D. P., et al. (2012). State-space framework for estimating measurement error from double-tagging telemetry experiments. Methods Ecol. Ecol. 3, 291–302. doi: 10.1111/j.2041-210X.2011.00161.x
Keywords: satellite tagging, elasmobranch, spatial management, conservation, porbeagle shark, life stage, reproduction
Citation: Anderson BN, Hammerschlag N, Saul S, Dodd JF, Bowlby HD, Kang Y, Gallagher AJ and Sulikowski JA (2025) Life stage and seasonal habitat use of the porbeagle Lamna nasus in the Northwest Atlantic Ocean. Front. Mar. Sci. 12:1553509. doi: 10.3389/fmars.2025.1553509
Received: 30 December 2024; Accepted: 25 March 2025;
Published: 14 April 2025.
Edited by:
Adrian C Gleiss, Murdoch University, AustraliaReviewed by:
Peter Gausmann, Ruhr University Bochum, GermanyCopyright © 2025 Anderson, Hammerschlag, Saul, Dodd, Bowlby, Kang, Gallagher and Sulikowski. This is an open-access article distributed under the terms of the Creative Commons Attribution License (CC BY). The use, distribution or reproduction in other forums is permitted, provided the original author(s) and the copyright owner(s) are credited and that the original publication in this journal is cited, in accordance with accepted academic practice. No use, distribution or reproduction is permitted which does not comply with these terms.
*Correspondence: Brooke N. Anderson, Ym5hbmRlcnNvQGdtYWlsLmNvbQ==
†Present addresses: Neil Hammerschlag, Shark Research Foundation Inc., Boutiliers Point, NS, Canada
James A. Sulikowski, Hatfield Marine Science Center, Oregon State University, Newport, OR, United States
Disclaimer: All claims expressed in this article are solely those of the authors and do not necessarily represent those of their affiliated organizations, or those of the publisher, the editors and the reviewers. Any product that may be evaluated in this article or claim that may be made by its manufacturer is not guaranteed or endorsed by the publisher.
Research integrity at Frontiers
Learn more about the work of our research integrity team to safeguard the quality of each article we publish.