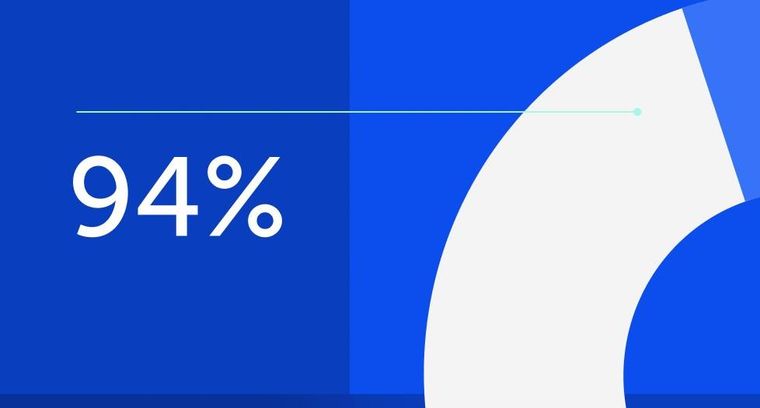
94% of researchers rate our articles as excellent or good
Learn more about the work of our research integrity team to safeguard the quality of each article we publish.
Find out more
ORIGINAL RESEARCH article
Front. Mar. Sci., 06 February 2025
Sec. Marine Fisheries, Aquaculture and Living Resources
Volume 12 - 2025 | https://doi.org/10.3389/fmars.2025.1548955
This article is part of the Research TopicBeneficial Effects of Microorganisms and Their Metabolites, Including Probiotics, Fungi and Microalgae, on the Growth and Physiological Health of Aquatic AnimalsView all 4 articles
Introduction: Bacillus species are probiotics commonly utilized in aquaculture to enhance aquatic animal growth, inhibit pathogens, and strengthen immunity. However, research comparing the effects of probiotic bacterial cells and cultures is limited. This study aimed to evaluate the probiotic potential of Bacillus licheniformis strain HN318 and compare its impact on growth, immunity, and disease resistance in hybrid grouper when administered as bacterial cells or cultures.
Methods: The study involved assessing the auto-aggregation capability, gastrointestinal stress resistance, and safety of HN318. Enzymatic activities and antibacterial properties of HN318 cultures and bacterial cells were also compared. Hybrid grouper were fed with HN318 cultures and bacterial cells for 8 weeks, and their growth, feed utilization, immune responses, and survival rates upon challenge with Vibrio harveyi were evaluated. Additionally, the expression of immune-related genes was analyzed.
Results: HN318 exhibited high auto-aggregation and gastrointestinal stress resistance, and was found to be safe for use in aquaculture. Cultures of HN318 displayed higher protease, amylase, and antibacterial activities compared to bacterial cells. Both forms significantly improved growth and feed utilization in hybrid grouper. Notably, HN318 cultures induced higher levels of immune enzymes and activities, and provided better protection against V. harveyi challenge, with a higher relative percent survival compared to bacterial cells. Furthermore, HN318 cultures upregulated the expression of immune-related genes more than bacterial cells.
Discussion: This study highlights the potential of both HN318 cultures and bacterial cells as immune enhancers for hybrid grouper. However, HN318 cultures demonstrated superior probiotic properties, including higher enzymatic activities, antibacterial properties, and immunomodulatory effects. These findings provide new insights and references for the diverse application forms of probiotics in aquaculture, suggesting that cultures may be more effective than bacterial cells in enhancing the health and performance of aquatic animals.
The hybrid grouper is a first-generation hybrid between Epinephelus polyphekadion (♂) and E. fuscoguttatus (♀), which has emerged as one of the significant varieties in Hainan’s grouper aquaculture in recent years (Huang et al., 2018). However, the shift towards large-scale and intensive farming practices has increasingly exposed the aquaculture industry to various diseases (Torrecillas et al., 2007). Bacterial infections are a significant concern in grouper aquaculture, resulting in substantial economic losses and environmental impacts (Xu et al., 2018). While antibiotics have been commonly used to control these diseases, their overuse has resulted in antibiotic-resistant bacteria, posing risks to both human health and the environment (Baquero et al., 2008; Magnadottir, 2010; Resende et al., 2012; Mathialagan et al., 2018). To address these concerns, probiotics are emerging as a promising alternative (Qi et al., 2009; Dawood and Koshio, 2016). Probiotics, or beneficial microorganisms, enhance the health of aquatic animals by competing with pathogens, producing antimicrobial substances, and boosting the immune system (Gómez and Shen, 2008; Kesarcodi-Watson et al., 2008). This approach not only reduces the need for antibiotics but also supports sustainable aquaculture by maintaining ecosystem balance and ensuring the production of safe, high-quality seafood.
Probiotics, when applied in their live bacterial form to aquaculture, offer several advantages. It has been reported that the addition of Bacillus licheniformis Dahb1 bacterial cells can enhance the growth performance and antibacterial resistance of the Pangasius hypophthalmus (Gobi et al., 2016). Huang et al. (2023) revealed the beneficial effects of B. licheniformis VLPPro® SB538 supplementation on the culture water quality, growth performance, and intestinal health of hybrid grouper. In Labeo rohita, B. licheniformis supplementation or combination supplementation of B. licheniformis and B. methylotrophicus could improved the growth response, immune, hematological parameters, and post-challenge survival rate (Mukherjee et al., 2019). Consequently, dietary supplementation of B. licheniformis was noticed to enhance growth, immunity and disease resistance in fish. Moreover, the presence of live probiotic cells in the water can contribute to a stable and healthy aquatic environment by modulating the microbial community.
In addition to the use of live probiotic cells, probiotic cultures and their bacteria-free supernatants also have potential benefits, especially in terms of improving host disease resistance (Chen et al., 2009; Xiong et al., 2015; Midhun et al., 2017; Lin et al., 2021; Zhang et al., 2022). For example, studies had shown that probiotic cultures are superior to probiotic cells in improving fish growth performance and disease resistance (Pichard et al., 1995; Lin et al., 2021). The cultures of B. amyloliquefaciens has superior antibacterial properties compared to the bacterial cells, which is attributed to the production of secondary metabolites with antibacterial activity (Chen et al., 2009). Paenibacillus polymyxa, heat-inactivated P. polymyxa, and sterile fermentation supernatant all exhibited bactericidal activity against the tested pathogens, with the supernatant showing the strongest inhibitory effect (Midhun et al., 2017). The dietary levan produced by B. licheniformis FRI MY-55 could be an effective method for enhancing the growth performance and disease resistance in orange-spotted grouper (Huang et al., 2014). The primary reason for the enhanced disease resistance in fish provided by probiotics may be due to the production of bacteriocins, siderophores, antibiotics, lysozymes, and other bioactive substances (Gram and Melchiorsen, 1996). Nevertheless, the application of probiotic cultures in aquaculture has been less explored compared to the use of probiotics bacterial cells. In particular, the probiotic effects of B. licheniformis cultures are unclear and there is no comparative study on the application effect of B. licheniformis cultures and its bacterial cells. Further research is needed to fully understand the impact of these fermentation products on the health and well-being of aquatic animals, as they may provide an alternative or complementary approach to traditional probiotic applications.
This study assessed the probiotic characteristics of B. licheniformis HN318 by evaluating its hemolytic activity, adhesiveness, tolerance to simulated gastric and intestinal fluids, and safety. In addition, the extracellular enzyme activity and antibacterial activity of both the B. licheniformis bacterial cells and its cultures were compared. Based on this, we further explored and compared the effects of dietary supplementation with B. licheniformis HN318 bacterial cells and cultures on the growth, non-specific immunity, and disease resistance of hybrid grouper, providing a theoretical basis for its application in the growth and disease prevention of groupers.
Bacillus licheniformis strain HN318 used in the present study was isolated from the intestine of healthy grouper, identified by 16S rDNA sequencing, and preserved by our laboratory. The well-preserved HN318 strain was activated, inoculated into Zobell Marine Broth 2216 (2216E, Qingdao Hope Bio-Technology Co. Ltd, China), and cultured at 37°C until the OD600 reached 1.0, which was obtained as the HN318 cultures. To prepare the HN318 bacterial cells, the HN318 cultures were obtained and then added to a 50 mL centrifuge tube, centrifuged at 4°C and 4000 rpm for 12 min. The supernatant was discarded, and the pellets were washed twice with phosphate buffered saline (PBS). The bacterial concentration was then adjusted to match the original culture concentration using PBS.
Cell surface hydrophobic activity was determined according to our previously described method (Sun et al., 2018). Briefly, 1.5 mL of HN318 bacterial cells was taken out and mixed with chloroform, xylene or ethyl acetate in 1:1 ratio, respectively, and shaken with a vortex shaker for 2 min. Then let the mixture stand at room temperature for 8 h. 100 μL of the aqueous phases (the aqueous phases of xylene and ethyl acetate in the lower layer, and the chloroform one in the upper layer) were pipetted at 0, 1, 2, 4, 6, and 8 h, respectively, and the absorbance values at 600 nm were measured in a microplate reader (Thermo Fisher Scientific, BioTek, USA). The hydrophobicity was determined using the following expression: Hydrophobicity (%) = (A0-At)/A0 × 100, A0 is the absorbance without mixing with carbohydrates and At is the absorbance value of the aqueous phase after mixing with organic solvents and resting. The experiment was repeated three times.
The auto-aggregation activity was detected following our previously outlined methodology (Sun et al., 2018). 4 mL of HN318 bacterial cells was taken and vortexed for 10 s, left at room temperature, and the change in absorbance of the supernatant at 600 nm was measured at 1, 2, 4, 6, and 8 h. The percentage of auto-aggregation was determined by the following formula: auto-aggregation (%) = [1-(At/A0)] × 100%, where At denotes the absorbance at different times, and A0 denotes the absorbance at 0 h. The experiment was repeated three times.
Simulated gastrointestinal stress tolerance was analyzed according to the method described in a previous study (Manhar et al., 2015). Briefly, simulated gastric fluid (SGF) was formulated in PBS through the addition of pepsin at a concentration of 0.3 mg/mL and NaCl at 0.5% (w/v), with the pH adjusted to either 3.0 or 4.0. This mixture was subsequently filtered utilizing a 0.22 μm filter membrane. Differently, simulate intestinal fluid (SIF) was formulated by incorporating pancreatin (Sigma, Germany) at a concentration of 0.1 mg/mL and 0.3% (w/v) of Oxgall (Solarbio, Beijing, China) into PBS. The pH of this mixture was adjusted to either 6.8 or 8, and subsequently, the solution was filtered through a 0.22 μm filter membrane. Then, 100 μL of HN318 bacterial cells was withdrawn and added separately to SGF and SIF. Subsequently, both mixtures were incubated at 37°C in a constant temperature incubator. After 0, 1, 2 and 4 h of incubation, the 10-fold gradient dilution mixture was coated and then counted, and the survival rate of HN318 was calculated using the formula: survival rate (%) = (Nt/N0) × 100, where Nt is the number of surviving cells at 1, 2 or 4 h, and N0 is the number of cells at 0 h. The experiment was repeated three times.
Under sterile conditions, the HN318 bacterial cells was streaked onto the blood agar plate (HuanKai Biology, Guangdong, China) and cultured in a 37°C incubator for 24 h to observe whether hemolysis occurred on the plate. In addition, Staphylococcus aureus was streaked onto the blood agar plate as a positive control. The experiment was repeated three times.
100 μL of HN318 bacterial cells was taken and spread evenly with a sterile applicator stick on the 2216E solid medium. Thirty-five kinds of drug sensitivity disks were attached to the plates coated with HN318 with sterile tweezers. Each drug sensitivity disk was set up in 3 parallels and incubated overnight in an incubator at 37°C. The diameter of each inhibition circle was measured to determine the sensitivity of HN318 to various antibiotics, using the standard of interpretation for the range of inhibition provided by the drug sensitivity disks. The experiment was repeated three times.
HN318 bacterial cells was prepared by following the method outlined in 2.1. Healthy hybrid grouper (average weight: 15.18 ± 1.25 g, average length: 8.57 ± 1.32 cm) were purchased from Changjiang Chengzhe Biological Limited Liability Company. Before experiments, fish were transiently reared in a recirculating water system for seven days. A total of 60 fish were divided into two groups. Within each group, 30 fish were then randomly assigned to three sub-groups, with each sub-group consisting of 10 fish. The experimental group was intraperitoneally injected with 100 μL of HN318 bacterial cells, whereas the control group received an equivalent volume of PBS via the same route. The health status of hybrid grouper was observed within 14 days after injection, and the mortality rate was recorded daily to evaluate the safety of HN318.
The HN318 cultures and its bacterial cells were prepared and their activities of amylase production and protease production were compared. Briefly, two 7 mm holes were drilled on 2216E solid medium containing 1% soluble starch, and 80 μL of HN318 cultures and HN318 bacterial cells were added to the holes, respectively, and cultured in a constant temperature incubator at 37°C for 12 h. Then, the plate is covered with a 10-fold dilution of Luckett’s iodine solution, and left it to stand until a transparent ring appears. The activity of amylase production was judged according to the diameter of the transparent ring (mm). Similarly, 2216E solid medium containing 1% skimmed milk powder were prepared and two 7 mm holes containing 80 μL of HN318 cultures or HN318 bacterial cells were created in the medium respectively. After incubation at 37°C for 12 h, the protease production activity was determined according to the hydrolysis circle size (mm) including the 7 mm hole size. The experiment was repeated three times.
The antimicrobial activities of the HN318 cultures and HN318 bacterial cells were evaluated against various pathogenic bacteria (Vibrio harveyi, V. alginolyticus, Edwardsiella tarda and Staphylococcus aureus) using the agar disc diffusion method. HN318 cultures and its bacterial cells were prepared according to the method described above. Then, four pathogenic bacteria were activated and expanded for culture, and their concentration was adjusted to 1× 107 CFU/mL. 1 mL of pathogenic bacteria was then added into 20 mL LB semi-solid medium, mixed evenly, and poured into a plate. After solidification, 8 mm sterile filter paper discs were attached to the surface of the plate, and 50 μL of HN318 cultures or its bacterial cells were absorbed and added to the filter paper. Finally, the plate was placed in the incubator for culture, and the diameter of antibacterial zone including the 8 mm sterile filter paper disc was measured after 24 h. The experiment was repeated three times.
Commercial diet (SANTON CENTRAL FEEDS CO., LTD., CHINA) was used as a basal ingredient of diets, and its nutrition composition was shown in Supplementary Table S1. The bacterial concentrations of both the HN318 cultures and HN318 bacterial cells were adjusted to 1 × 108 CFU/g diet, and then evenly sprayed on the commercial diet respectively, whereas the control diet received the same volume of sterile PBS in an identical manner. Following spraying, three diets were air-dried under controlled conditions, stored at 4°C, and prepared on a weekly basis to ensure freshness.
Healthy hybrid grouper (average weight 31.21 ± 3.23 g) were purchased from Changjiang Chengzhe Biological Co. Before the experiment started, the fish were temporarily reared for a week and fed with a commercial Haitong feed twice a day (9 a.m. and 5 p.m.). Subsequently, 360 fish were randomly divided into three groups, with three parallels in each group and 40 fish in each parallel. Fish in each group were bulk weighed as the initial weight. Three diets, namely the control diet, HN318 cultures diet, and HN318 bacterial cells diet, were individually fed to one group of fish. The feeding experiment was conducted for 8 weeks (W) and fish were fed three experimental diets twice a day (9 a.m. and 5 p.m.). After feeding for 1 h, uneaten diets were collected, dried to constant weight at 70°C, and re-weighed to calculate subsequent feed intake. The water in each tank was replaced daily by two-thirds of its volume. The experimental conditions were rigorously controlled, maintaining water temperature within the range of 28-30°C, dissolved oxygen levels at 7 mg/L, and ensuring that total ammonia nitrogen remained consistently below 0.03 mg/L.
At the end of the feeding experiment, fish were first starved for 24 h, and then fish in each group were anesthetized with tricaine mesylate (Sigma-Aldrich, St. Louis, MO, USA) and weighed for the final weight. Percentage weight gain (PWG) and feed utilization rate (FUR) were calculated to evaluate the growth of hybrid grouper. The calculation formula is: PWG (%) = [(final weight (g) - initial weight (g))/initial weight (g)] × 100, FUR (%) = [(final weight (g) - initial weight (g)]/total feed intake (g).
To analyze non-specific immune parameters, the head kidneys and blood of 9 fish in each group (3 fish per parallel) were respectively collected at 1 W, 4 W, and 8 W of feeding, with one day of food withdrawal before sampling. Prior to sampling, fish were anesthetized using tricaine mesylate. Blood was collected from the tail vein of fish with a 1 mL sterile syringe, gently pressed into a sterile 1.5 mL centrifuge tube, and left at 4°C overnight. The serum was obtained by centrifugation at 3000 rpm for 10 min at 4°C and stored at -80°C for further use. The serum level of superoxide dismutase (SOD), acid phosphatase (ACP), and serum lysozyme (LZM) activities were determined by commercial kits (Nanjing Jianjian Bioengineering Research Institute, Nanjing, Jiangsu, China) according to the manufacturer’s instructions (Yang et al., 2015). The head kidney was extracted under sterile conditions and macrophages from the head kidney were collected as previously described (Liu et al., 2017). Cell viability was calculated using the Taipan blue exclusion test. The concentration of harvested cells was adjusted to 2×106 cells/mL. Then, the respiratory burst (RB) activity of head kidney macrophages (HKMs) was determined by the nitroblue tetrazolium (NBT, Sigma Aldrich) assay as described in previous studies (Sun et al., 2010). The assay wavelength was 630 nm and KOH/DMSO was used as a blank. The samples were analyzed in triplicate.
V. harveyi strain QT520 stored in our laboratory was used in this study, which was isolated from diseased Trachinotus ovatus and showed strong pathogenicity to T. ovatus (Tu et al., 2017). V. harveyi was cultured overnight and the concentration was adjusted to 1×105 CFU/mL. Then, 150 μL of serum described in the previous paragraph were incubated with 150 μL of V. harveyi in a 96-well plate for 1 h at 30°C, with five parallel wells set up for each sample. Subsequently, 100 μL of culture was absorbed and spread on LB agar plate, and the number of surviving V. harveyi was counted after 24 h of incubation at 30°C.
To evaluate the protective effect of the experimental diets on disease resistance of fish, a challenge test was carried out after 8 weeks of feeding trial. V. harveyi was cultured overnight and the concentration was adjusted to 1×104 CFU/mL. Eighty four fish were randomly selected from the remaining fish in each group (28 fish per parallel) and intraperitoneally injected with 100 μL of V. harveyi. At 12 h after injection, the spleens of 9 fish from each group (3 fish per parallel) were taken for subsequent quantitative real time PCR (qRT-PCR) analysis. 75 fish from each group (25 fish per parallel) were used to calculate mortality. Mortality was monitored for 7 d following the injection. Relative percent of survival (RPS) was calculated according to the following formula: RPS = [1 - (% mortality of treated fish/% mortality of control fish)] × 100.
RNA extraction from spleens and cDNA synthesis were performed as reported previously (Sun et al., 2018). qRT-PCR was performed using the Eastep qPCR Master Mix Kit (Promega, Madison, USA) on a QuantStudio™ 6 FLEX Real-Time PCR System (ABI, USA). The expressions levels of immune genes, including interferon-gamma (IFNγ), interleukin-1 beta (IL1β), interleukin-8 (IL8), transforming growth factor beta-1 (TGFβ1), tumor necrosis factor alpha (TNFα), and interleukin-10 (IL10), were quantified. Specific primer sequences for these genes are listed in Table 1. The housekeeping gene β-2-microglobulin (B2M) was used for normalization. The 2-ΔΔCt method was used for calculating the gene expression levels. The samples were analyzed in triplicate.
All statistical analysis were executed using SPSS 17.0 software (SPSS. Inc., Chicago, IL, USA) and GraphPad Prism 9.0 (GraphPad, CA, USA). Data was presented as mean ± standard deviation (SD). Results were analyzed using one-way analysis of variance (ANOVA), and were considered statistically significant when the probability (p) value was less than 0.05.
Cell surface hydrophobic activity and auto-aggregation capacity are related to bacterial adhesion to epithelial cells. The cell surface hydrophobic activities of B. licheniformis HN318 in chloroform, xylene and ethyl acetate were as high as 69.5%, 85.8% and 64.7% (Figure 1A). Meanwhile, the auto-aggregation activity of HN318 increased with incubation time, reaching 84% at 8 h (Figure 1B).
Figure 1. Cell surface hydrophobic activity (A) and auto-aggregation capacity (B) of B. licheniformis HN318.
Under the simulated gastric fluid condition, HN318 was well tolerated. Compared to 0 h, at pH = 3, the bacterial counts of HN318 gently decreased in simulated gastric fluid after 1 h, 2 h, and 4 h, with survival rates of 42%, 37%, and 34%, respectively (Table 2). At pH = 4, the survival rate of HN318 at 1 h-4 h firstly increased and then decreased, with the survival rate reaching 52.3% at 2 h and 31.5% at 4 h. The stress capacity of HN318 in simulated intestinal fluid was also shown in Table 2. When pH = 6.8, the number of surviving cells of HN318 remained basically unchanged from 1 h to 4 h, with cell counts of (0.18 ± 0.02) × 108 CFU/mL, (0.15 ± 0.00) × 108 CFU/mL, (0.15 ± 0.01) × 108 CFU/mL, indicating a slow decrease compared to the initial cell count at 0 h, which was (2.16 ± 0.04) × 108 CFU/mL. At pH = 8.0, the number of surviving cells of HN318 at 1 h was (0.41 ± 0.08) × 108 CFU/mL, and the number of HN318 at 2 h and 4 h was almost unchanged.
The results of the drug resistance test of HN318 are shown in Table 3. The results showed that strain HN318 was resistant to 10 antibiotics, including azlocillin, mezlocillin, piperacillin, penicillin, oxacillin, and carbenicillin from β-lactams; ceftazidime from cephalosporins; erythromycin from macrolides; and polymyxin B and clindamycin from quinolones. In addition, HN318 was sensitive to 20 antibiotics, including 3 cephalosporins; 6 aminoglycoside; 7 quinolones; and other 4 antibiotics. Furthermore, the HN318 exhibited moderately sensitive to 5 antibiotics, including ampicillin, amoxicillin, cefoperazone, streptomycin and chloromycetin.
By observing the growth of HN318 on the surface of the blood plate, it can be seen that HN318 didn’t produce a transparent hemolytic ring, indicating that HN318 does not hemolysis (Figure 2). In contrast, S. aureus produced a transparent hemolysis circle around the colony, showing α-hemolysis (Figure 2). HN318 was then intraperitoneally injected into the hybrid grouper to evaluated its pathogenicity. The results showed that no pathological symptoms, disease or death occurred in HN318 group and control group within 14 days after injection, indicating that HN318 is safe and harmless to hybrid grouper.
As can be seen from Table 4, both HN318 cultures and bacterial cells had certain amylase and protease activities. In contrast, the extracellular amylase and protease activities of HN318 cultures were significantly higher than those of HN318 bacterial cells (p < 0.05).
Both HN318 cultures and bacterial cells showed inhibitory effect on all four indicator pathogens (Table 5). Notably, the antagonistic effects of HN318 cultures on V. harveyi and S. aureus were significantly higher than that of the HN318 bacterial cells (p < 0.05). However, there was no significant difference in the antagonistic effects of HN318 culture and HN318 bacterial cells against E. tarda and V. alginolyticus (p > 0.05), although the diameter of the HN318 culture against both pathogens was higher than that of the HN318 bacterial cells.
The growth performance was measured after 8 W of feeding and the results are shown in Table 6. The final weight and PWG in both of HN318 culture group and HN318 bacterial cells group were significantly higher than those in control group (p < 0.05), but there was no significant difference in these two indexes between HN318 culture group and HN318 bacterial cell group (p > 0.05). Similarly, the difference of feed utilization rate among three groups also showed the same trend.
Table 6. Effects of B. licheniformis HN318 bacterial cells and cultures on growth performance of hybrid grouper.
At 1 W, ACP activity of both HN318 cultures and HN318 bacterial cells groups was significantly higher than the control group (p < 0.05). At 4 W, HN318 cultures group showed the highest ACP activity, significantly higher than both the control and HN318 bacterial cells groups (p < 0.05) (Figure 3A). The SOD activity of the HN318 cultures and bacterial cells groups were not significantly different from the control at 1 W and 4 W, but significantly higher than the control group at 8 W (Figure 3B). At 1 W, 4 W, and 8 W, HN318 cultures exhibited significantly higher LZM activity than that of HN318 bacterial cells and control groups (p < 0.05), whereas no significant difference was found in bacterial cells group and control group (p > 0.05) (Figure 3C). Moreover, the RB activity in HN318 cultures group was significantly higher than that of the bacterial cells group and control group at both 1 W and 4 W (p < 0.05), whereas the RB activity in the bacterial cells group showed no significant difference from the control group throughout the feeding period (p > 0.05) (Figure 3D).
Figure 3. The immune-related enzyme activities of hybrid grouper after feeding B. licheniformis HN318 cultures and bacterial cells diets. Serum acid phosphatase (ACP) activity (A), serum superoxide dismutase (SOD) activity (B), serum lysozyme (LZM) activity (C), and macrophages respiratory burst (RB) activity (D) were detected. Different letters indicate significant differences (p < 0.05) in data collected at the same sampling time across treatments.
After 4 W and 8 W of feeding, the serum bactericidal activities against V. harveyi in HN318 cultures and HN318 bacterial cells groups were significantly higher than that of the control group (p < 0.05). Amongst all, fish fed the HN318 cultures diet showed significantly higher bactericidal activity than the bacterial cells group at 4 W (p < 0.01) (Figure 4).
Figure 4. Serum bactericidal activity of hybrid grouper after feeding after feeding B. licheniformis HN318 cultures and bacterial cells diets. Different letters indicate significant differences (p < 0.05) in data collected at the same sampling time across treatments.
Fish were injected with V. harveyi and monitored for mortality after 8 W of feeding diets containing HN318 cultures, HN318 bacterial cells, or control. The highest survival rate (72%) was observed in HN318 culture group, which was significantly higher than that of the HN318 bacterial cells groups (36%) and control group (20%) (p < 0.05) (Figure 5). Meanwhile, the HN318 bacterial cells group also showed a significantly higher survival rate than the control group (p < 0.05). By calculation, the RPS of the HN318 cultures group and bacterial cells group were 65.10% and 20.13%, respectively.
Figure 5. Survival percentages of different administrating fish. Different letters indicate significant differences (p < 0.05) in data collected at the same sampling time across treatments.
To examine the effect on immune-related genes expression induced by HN318 bacterial cells or cultures, including IFNγ, IL1β, IL8, TGFβ1, TNFα and IL10 were selected for qRT-PCR analysis. The results showed that compared with the control group, the expression levels of all the examined genes in the HN318 culture group were significantly up-regulated (p < 0.05) (Figure 6). Meanwhile, IL1β, IL8, TNFα and IL10 were significantly overexpressed in the HN318 bacterial cells group compared to the control group (p < 0.05).
Figure 6. Effect of B. licheniformis HN318 bacterial cells and cultures on immune gene expression. The fold change of gene expression was normalized to β-2-microglobulin (B2M) and relative to the control group samples. The statistical significance is analyzed. Different letters indicate significant differences (p < 0.05) in data collected at the same sampling time across treatments.
In recent years, the use of probiotics in aquatic animal feeds has received increasing attention due to the need for environmentally friendly health management in aquaculture (Harikrishnan et al., 2010; Kim et al., 2013; Nguyen et al., 2017). A large number of studies have shown that probiotics added to feed in the form of bacterial cells or cultures can improve the health performance of various aquatic animals, but few studies have compared the differences in the effects of the two uses on growth, immunity, and disease resistance in aquatic animals. In this study, it has been demonstrated that B. licheniformis HN318 exhibits pronounced probiotic properties. Furthermore, the diet containing HN318 cultures achieved a better health benefit on the growth, immunity, and disease resistance in the hybrid grouper than using the bacterial cells.
The health benefits of probiotics are widely recognized. However, this beneficial effect is strain specific. Therefore, probiotics must undergo strict screening before being developed and applied, and only bacteria with significant in vitro probiotic properties and biosafety can become probiotic candidate strains. Bacterial adhesion ability is an important index to evaluate whether potential probiotics can accumulate and colonize in the intestine to exert multiple effects, such as maintaining body metabolism, promoting intestinal development, and inhibiting the adhesion and spread of pathogenic bacteria (Boonaert and Rouxhet, 2000; Polak-Berecka et al., 2014). Studies have shown that auto-aggregation capacity and cell surface hydrophobic activity are positively correlated with the adhesion ability of strains (Liu et al., 2017; Sun et al., 2018). In our study, HN318 exhibited high hydrophobic activity in solvents. Additionally, its auto-aggregation activity increased progressively over time, indicating that HN318 possesses a strong capability to adhere to the intestinal tract. In addition, the factors that limit the survival and colonization of probiotics in the gastrointestinal tract of animals also include the destructive effect of gastric and intestinal fluids on probiotics (De Boever et al., 2001; Ringø et al., 2003). Many studies have used in vitro tests to screen probiotics that can resist simulated gastrointestinal fluid, such as Bacillus and lactic acid bacteria, and fed aquatic animals to prove that they can improve the growth performance and immunity (Chen et al., 2010; Zhou et al., 2019). In this study, HN318 was able to survive in simulated gastric fluid with pH 3.0 and simulated intestinal fluid with pH 8.0, with a survival rate of 34% and 18% after 4 h, respectively, demonstrating its potential as a probiotic.
The safety evaluation of probiotics is the key link in the research and application process, including hemolysis detection, animal pathogenicity test, drug resistance test, etc (Sahu et al., 2008; Wang et al., 2017; Yang et al., 2017). If probiotics are to be used in organisms, it should be the first prerequisite that they are harmless to the host. Although Bacillus is widely recognized as a good probiotic, some strains of Bacillus cereus and Bacillus subtilis have been shown to be pathogenic to fish (Luo et al., 2009; Nie et al., 2015; Velmurugan et al., 2015; Yang et al., 2017). Hemolysis is thought to be the cause of the disease caused by B. cereus in Ictalurus punctatus and Carassius auratus (Zhang et al., 2019; Chen et al., 2019). Fortunately, our study found that HN318 exhibited no hemolysis, and the hybrid grouper remained healthy and grew well after HN318 was injected into grouper, indicating that HN318 could be used as a candidate probiotic in hybrid grouper culture. Additionally, probiotics, as common strains in the environment, may serve as significant reservoirs of antibiotic resistance genes, which can spread through the food chain and pose a threat to human health (Wang et al., 2017; Jiao et al., 2017). Determining the antibiotic resistance of probiotics can help quickly identify and eliminate strains with potential safety hazards. Some strains of Lactobacillus and Bifidobacterium have shown resistance to antibiotics such as tetracycline, erythromycin, and chloramphenicol, and may promote horizontal gene transfer between bacteria in the gut, increasing the risk of human pathogens acquiring resistance (Sánchez et al., 2017). This study investigated the antibiotic resistance of HN318 and found that HN318 was sensitive to 25 common antibiotics, including tetracycline, gentamicin, oxytetracycline, vancomycin, norfloxacin, tobramycin, etc., demonstrating that the potential safety risk of HN318 is low. Combining the results of the probiotic properties and safety risk assessment, HN318 is an excellent candidate probiotic strain with potential for development and application.
The positive effects of using probiotics as feed additives in fish farming, in terms of nutrition, growth, immune modulation, and disease resistance, are unquestionable. In previous studies, the addition of probiotics in the form of live bacterial cells to feed has been shown to improve the health of some aquatic economic animals (de Souza et al., 2012; Darafsh et al., 2020). However, few studies on the effects of addition in the form of bacterial cultures on aquatic animals, even though bacterial cultures are actually more convenient to obtain compared to collecting bacterial bodies. Probiotic cultures contain complex probiotic fermentation products, including living cells, cell wall components, metabolites, and culture media for probiotic bacterial cells (Nilnaj et al., 2011; Ayiku et al., 2020). Moreover, the cultures contain non-antibiotic antibacterial substances and bioactive compounds that stimulate the immune and antioxidant systems and improve the health of the fish (Midhun et al., 2017, 2018). Therefore, we speculate that there is a potential effect of B. licheniformis HN318 culture on improving the health status of grouper. Following an 8 weeks of feeding period, our study’s findings supported the above hypothesis, with a marked enhancement in the final weight, PWG, and feed utilization rate of hybrid grouper observed upon supplementing the HN318 cultures and bacterial cells into the feed. Considering that this study also found that both HN318 cultures and bacterial cells showed excellent amylase and protease production activities, the growth promoting effect may be due to the increased activity of digestive enzymes produced by HN318, which enhances the fish’s appetite and promotes metabolism. Similarly, the addition of B. amyloliquefaciens, B. subtilis, and B. cereus to the diets significantly increased the activities of protease, lipase, and amylase in Lates calcarifer and Clarias gariepinus, thus promoting the growth performance (Reda et al., 2018; Adorian et al., 2019). Inconsistently, Tao (2023) reported that dietary administration of Clostridium butyricum cultures did not significantly affect the growth performance and feed utilization rate of Micropterus salmoides, but significantly reduced the hepatosomatic index and viscerosomatic index. This difference may be due to the addition dose, probiotic species, fermentation medium and fish species.
When pathogens invade, fish primarily deploy their defense through the non-specific immune system (Zhou et al., 2015). Macrophages, as one of the most effective cells in non-specific immune defense, possess a strong capability for non-specific phagocytosis and elimination of various pathogens. The intensity of the RB activity directly reflects the capacity of macrophages to kill pathogens (Abreu et al., 2009; Rieger and Barreda, 2011). Furthermore, serum indicators (such as ACP, LZM, etc.) are usually used to reflect the physiological and pathological changes of aquatic animals to determine the health status (Pedro et al., 2005). Previous research showed that administering probiotics stimulated the immune system of O. niloticus, enhance the RB activity of macrophages, and increase the number of white blood cells and lymphocytes, thereby enhancing the organism’s immunity to pathogens (Tachibana et al., 2020). Feng et al. (2020) indicated that feeding Bacillus can significantly enhance the ACP activity in the Apostichopus japonicus. Gobi et al. (2018) also observed that feeding B. licheniformis led to a significant increase in the AKP, LZM, SOD activities of O. niloticus. Consistent with these studies, in our study, the addition of HN318 cultures to the feed significantly enhanced the RB activity of macrophages, SOD, LZM, and ACP activities in serum. By contrast, HN318 bacterial cells only positively affected the serum ACP and SOD activities, but did not significantly increase LZM and RB activities. In line with these results, the administration of HN318 cultures significantly enhanced the in vitro bactericidal activity of the serum against V. harveyi, compared to the bacterial cells and control groups. Similar findings were shown in Nile tilapia (Aly et al., 2008; Selim and Reda, 2015) and hybrid Hulong grouper (Zhou et al., 2019), which demonstrated that the addition of Bacillus spp. can enhance the in vitro bactericidal ability of the host serum. Not only that, compared with HN318 bacterial cells, HN318 cultures showed significantly higher in vitro antimicrobial effect against V. harveyi and S. aureus, and better against E. tarda and V. alginolyticus. Bacillus can exert inhibitory effects on pathogenic bacteria by secreting substances such as lysozyme, proteases, siderophores, and bacteriocins (Doan et al., 2021; Zhang et al., 2022). Multiple studies have compared the in vitro antibacterial effects of Bacillus cultures and bacterial cells. The results showed that the cultures exhibited stronger antibacterial activity than the bacterial cells, which may be attributed to the fact that the cultures contain a variety of antibacterial proteins and peptides antibacterial substances (Xiong et al., 2015; Lin et al., 2021; Zhang et al., 2022). Therefore, the results from the study indicated that HN318 cultures might induce a stronger innate immunity in fish compared to HN318 bacterial cells. This is most likely due to two reasons: first, HN318 cultures itself possess stronger direct antagonistic properties against pathogens, and the antibacterial components in the cultures still need to be further studied; second, HN318 cultures enhanced the RB activity of macrophages and stimulated macrophages to produce more cytokines into the blood circulation, thereby improving the innate immunity of hybrid grouper.
Once the innate immune system is activated, it will maintain homeostasis under the regulation of the organism (Varin and Gordon, 2009; Kim et al., 2013). Probiotics can activate the fish’s innate immune system, enhancing the host’s immunity and thereby increasing its disease resistance (Wang et al., 2013; Dash et al., 2015). Li et al. (2019) demonstrated that feeding a diet containing B. velezensis to the pearl grouper enhanced its resistance to V. harveyi. Similar protective effects were observed in Ctenopharyngodon idella against A. hydrophila (Qin et al., 2019), in O. niloticus against Streptococcus iniae (Kuebutornye et al., 2020), and in other aquaculture species (Nguyen et al., 2017; Sun et al., 2018). Supporting earlier findings, our study revealed that the addition of HN318 cultures and bacterial cells to the diets displayed significantly greater resistance to V. harveyi than those in the control group, with the corresponding RPS of 65.10% and 20.13%, respectively. Additionally, the survival rate in the cultures group was significantly higher than in the bacterial cells group. Research on Litopenaeus vannamei had shown that Lactobacillus pentosus cells and its supernatant significantly improved its resistance to V. parahaemolyticus. Moreover, the lower mortality in the supernatant group suggested that antimicrobial substances in the supernatant may have enhanced immunity (Sha et al., 2016). The cultures group in this study was composed of both the bacterium and the supernatant, so this may be also due to the fact that the supernatant contains certain secondary metabolites that stimulate the immune system of the fish, thus increasing its resistance to disease. To better understand the reasons for the immune-protective effect of HN318 cultures and bacterial cells, their immunomodulatory activities at the transcriptional level were explored. Midhun et al. (2019) confirmed that the anti-inflammatory cytokine IL10 in O. niloticus was significantly upregulated after B. licheniformis HGA8B supplementation. According to a study by Selim and Reda (2015), increased expression of TNFα and IL1β genes, leading to enhanced disease resistance, has been reported in tilapia (Selim and Reda, 2015). Moreover, Bacillus as the feed additive can upregulate the expression of immune genes such as IFNγ, TLR2, TGFβ1, IL10, IL1β, IFN1, and TNFα in various fish species (Chen et al., 2015; Midhun et al., 2019). Correspondingly, qRT-PCR results from the current study showed that the addition of HN318 cultures to the feed significantly up-regulated the expression of immune genes IFNγ, IL1β, IL8, TGFβ1, TNFα and IL10. In contrast, only IL1β, IL8, TNFα and IL10 were up-regulated in the HN318 bacterial cells group. This confirms the superior immunomodulatory effect of HN318 cultures, which also explains the good protective effect of HN318 on hybrid grouper.
In conclusion, B. licheniformis HN318 is a candidate probiotic with good probiotic properties, including excellent potential for gut colonization, extracellular enzyme production, and in vitro antibacterial function, as well as being harmless to the host. When added to feed in the form of cultures or bacterial cells, it can improve the growth performance, nonspecific immunity, and disease resistance of hybrid grouper, with the advantages of the cultures being more pronounced. Overall, this study provides a reference and new perspectives for the selection and diversified application forms of probiotics in aquaculture.
The original contributions presented in the study are included in the article/Supplementary Material. Further inquiries can be directed to the corresponding authors.
The animal study was approved by Animal Care and Use Committee of the Hainan University. The study was conducted in accordance with the local legislation and institutional requirements.
ZC: Conceptualization, Data curation, Formal analysis, Project administration, Writing – original draft, Writing – review & editing. QY: Project administration, Conceptualization, Data curation, Formal analysis, Writing – review & editing. AK: Data curation, Investigation, Methodology, Writing – review & editing. JW: Data curation, Investigation, Methodology, Writing – review & editing. GW: Data curation, Investigation, Methodology, Writing – review & editing. PL: Data curation, Investigation, Methodology, Writing – review & editing. GQ: Data curation, Investigation, Methodology, Writing – review & editing. CL: Funding acquisition, Writing – review & editing. YS: Funding acquisition, Project administration, Writing – review & editing.
The author(s) declare financial support was received for the research, authorship, and/or publication of this article. This work was supported by the Hainan Province Science and Technology Special Fund (ZDYF2022XDNY246) and the Innovational Fund for Scientific and Technological Personnel of Hainan Province (KJRC2023C38).
Author QY was employed by the company Yantai Scibio Biotechnology Co., Ltd.
The remaining authors declare that the research was conducted in the absence of any commercial or financial relationships that could be construed as a potential conflict of interest.
The author(s) declare that no Generative AI was used in the creation of this manuscript.
All claims expressed in this article are solely those of the authors and do not necessarily represent those of their affiliated organizations, or those of the publisher, the editors and the reviewers. Any product that may be evaluated in this article, or claim that may be made by its manufacturer, is not guaranteed or endorsed by the publisher.
The Supplementary Material for this article can be found online at: https://www.frontiersin.org/articles/10.3389/fmars.2025.1548955/full#supplementary-material
Supplementary Table 1 | Main nutrition components of the commercial diet.
Abreu J. S., Marzocchi-MaChado C. M., Urbaczek A. V., Fonseca L. M., Urbinati E. C. (2009). Leukocytes respiratory burst and lysozyme level in pacu (Piaractus mesopotamicus Holmberg 1887). Braz. J. Biol. 69, 1133–1139. doi: 10.1590/s1519-69842009000500018
Adorian T. J., Jamali H., Farsani H. G., Darvishi P., Hasanpour S., Bagheri T., et al. (2019). Effects of probiotic bacteria Bacillus on growth performance, digestive enzyme activity, and hematological parameters of Asian Sea Bass, Lates calcarifer (Bloch). Probiotics Antimicro. 11, 248–255. doi: 10.1007/s12602-018-9393-z
Aly S. M., Abdel-Galil-Ahmed Y., Abdel-Aziz-Ghareeb A., Mohamed M. F. (2008). Studies on Bacillus subtilis and Lactobacillus acidophilus, as potential probiotics, on the immune response and resistance of Tilapia nilotica (Oreochromis niloticus) to challenge infections. Fish Shellfish Immunol. 25, 128–136. doi: 10.1016/j.fsi.2008.03.013
Ayiku S., Shen J. F., Tan B. P., Dong X. H., Liu H. Y. (2020). Effects of dietary yeast culture on shrimp growth, immune response, intestinal health and disease resistance against Vibrio harveyi. Fish Shellfish Immunol. 102, 286–295. doi: 10.1016/j.fsi.2020.04.036
Baquero F., Martínez J. L., Cantón R. (2008). Antibiotics and antibiotic resistance in water environments. Curr. Opin. Biotechnol. 19, 260–265. doi: 10.1016/j.copbio.2008.05.006
Boonaert C. J., Rouxhet P. G. (2000). Surface of lactic acid bacteria: relationships between chemical composition and physicochemical properties. Appl. Environ. Microbiol. 66, 2548–2554. doi: 10.1128/AEM.66.6.2548-2554.2000
Chen F., Hunag Y. D., Sun J. F., LÜ A. J., Hu C. X., Shi H. Y., et al. (2019). Isolation, identification and biological characteristics of Bacillus cereus from intestine of Carassius auratus. Jiangsu Agric. Sci. 47, 191–194. doi: 10.15889/j.issn.1002-1302.2019.18.041
Chen L., Peng H. N., Kong C. B., Wang Z. Y., LÜ Z. H., Zhen L. (2010). The evaluation of the stress resistance of several probiotics to simulated gastrointestinal circumstance in vitro. Chin. J. Microecol. 22, 701–702+704. doi: 10.13381/j.cnki.cjm.2010.08.012
Chen X. H., Koumoutsi A., Scholz R., Schneider K., Vater J., Süssmuth R., et al. (2009). Genome analysis of Bacillus amyloliquefaciens FZB42 reveals its potential for biocontrol of plant pathogens. J. Biotechnol. 140, 27–37. doi: 10.1016/j.jbiotec.2008.10.011
Chen X. M., Lu H. M., Niu X. T., Wang G. Q., Zhang D. M. (2015). Enhancement of secondary metabolites from Bacillus Licheniformis XY-52 on immune response and expression of some immune-related genes in common carp, Cyprinus carpio. Fish Shellfish Immunol. 45, 124–131. doi: 10.1016/j.fsi.2015.02.019
Darafsh F., Soltani M., Abdolhay H. A., Shamsaei Mehrejan M. (2020). Efficacy of dietary supplementation of Bacillus licheniformis and Bacillus subtilis probiotics and Saccharomyces cerevisiae (yeast) on the hematological, immune response, and biochemical features of Persian sturgeon (Acipenser persicus) fingerlings. Iran. J. Fish. Sci. 19, 2024–2038. doi: 10.22092/ijfs.2018.117847
Dash G., Raman R. P., Pani Prasad K. P., Makesh M., Pradeep M. A., Sen S. (2015). Evaluation of paraprobiotic applicability of Lactobacillus plantarum in improving the immune response and disease protection in giant freshwater prawn, Macrobrachium rosenbergii (de Man 1879). Fish Shellfish Immunol. 43, 167–174. doi: 10.1016/j.fsi.2014.12.007
Dawood M. A. O., Koshio S. (2016). Recent advances in the role of probiotics and prebiotics in carp aquaculture: A review. Aquaculture 454, 243–251. doi: 10.1016/j.aquaculture.2015.12.033
De Boever P., Wouters R., Verstraete W. (2001). Combined use of Lactobacillus reuteri and soygerm powder as food supplement. Lett. Appl. Microbiol. 33, 420–424. doi: 10.1046/j.1472-765x.2001.01023.x
de Souza D. M., Suita S. M., Leite F. P. L., Romano L. A., Wasielesky W., Ballester E. L. C. (2012). The use of probiotics during the nursery rearing of the pink shrimp Farfantepenaeus brasiliensis (Latreille 1817) in a zero exchange system. Aquac. Res. 43, 1828–1837. doi: 10.1111/j.1365-2109.2011.02992.x
Doan H. V., Soltani M., Ringø E. (2021). In vitro antagonistic effect and in vivo protective efficacy of Gram-positive probiotics versus Gram-negative bacterial pathogens in finfish and shellfish. Aquaculture 540, 736581. doi: 10.1016/j.aquaculture.2021.736581
Feng Z. F., Song X. J., Zhao L. T., Zhu W. (2020). Isolation of probiotics and their effects on growth, antioxidant and non-specific immunity of sea cucumber Apostichopus japonicus. Fish Shellfish Immunol. 106, 1087–1094. doi: 10.1016/j.fsi.2020.08.049
Gobi N., Malaikozhundan B., Sekar V., Shanthi S., Vaseeharan B., Jayakumar R., et al. (2016). GFP tagged Vibrio parahaemolyticus Dahv2 infection and the protective effects of the probiotic Bacillus licheniformis Dahb1 on the growth, immune and antioxidant responses in Pangasius hypophthalmus. Fish Shellfish Immunol. 52, 230–238. doi: 10.1016/j.fsi.2016.03.006
Gobi N., Vaseeharan B., Chen J. C., Rekha R., Vijayakumar S., Anjugam M., et al. (2018). Dietary supplementation of probiotic Bacillus licheniformis Dahb1 improves growth performance, mucus and serum immune parameters, antioxidant enzyme activity as well as resistance against Aeromonas hydrophila in tilapia Oreochromis mossambicus. Fish Shellfish Immunol. 74, 501–508. doi: 10.1016/j.fsi.2017.12.066
Gómez R., Shen M. (2008). Influence of probiotics on the growth and digestive enzyme activity of white pacific shrimp (Litopenaeus vannamei). J. Ocean Univ. China. 7, 215–218. doi: 10.1007/s11802-008-0215-x
Gram L., Melchiorsen J. (1996). Interaction between fish spoilage bacteria Pseudomonas sp. and Shewanella putrefaciens in fish extracts and on fish tissue. J. Appl. Bacteriol. 80, 589–595. doi: 10.1111/j.1365-2672.1996.tb03262.x
Harikrishnan R., Balasundaram C., Heo M. S. (2010). Lactobacillus sakei BK19 enriched diet enhances the immunity status and disease resistance to Streptococcosis infection in kelp grouper, Epinephelus bruneus. Fish Shellfish Immunol. 29, 1037–1043. doi: 10.1016/j.fsi.2010.08.017
Huang J. P., Amenyogbe E., Wen Z. W., Ou G. H., Li Y., Jiang X. T., et al. (2023). Effect of Bacillus licheniformis probiotic on the culture of hybrid grouper (♀ Epinephelus fuscoguttatus × ♂ Epinephelus polyphekadion). Aquac. Rep. 33, 101798. doi: 10.1016/j.aqrep.2023.101798
Huang M. Y., Chang C. I., Chang C. C., Tseng L. W., Pan C. L. (2014). Effects of dietary levan on growth performance, nonspecific immunity, pathogen resistance and body composition of orange-spotted grouper (Epinephelus coioides H.). Aquac. Res., 1–16. doi: 10.1111/are.12430
Huang J. H., Chen G., Wang Z. L., Zhang J. D. (2018). Use of response surface methodology to study the combined effects of temperature and salinity on hatching and deformity of the hybrid grouper, Epinephelus fuscoguttatus (♀) × Epinephelus polyphekadion (♂). Aquac. Res. 49, 1997–2005. doi: 10.1111/are.13655
Jiao Y. N., Chen H., Gao R. X., Zhu Y. G., Rensing C. (2017). Organic compounds stimulate horizontal transfer of antibiotic resistance genes in mixed wastewater treatment systems. Chemosphere 184, 53–61. doi: 10.1016/j.chemosphere.2017.05.149
Kesarcodi-Watson A., Kaspar H., Lategan M. J., Gibson L. (2008). Probiotics in aquaculture: The need, principles and mechanisms of action and screening processes. Aquaculture 274, 1–14. doi: 10.1016/j.aquaculture.2007.11.019
Kim D., Beck B. R., Heo S. B., Kim J., Kim H. D., Lee S. M., et al. (2013). Lactococcus lactis BFE920 activates the innate immune system of olive flounder (Paralichthys olivaceus), resulting in protection against Streptococcus iniae infection and enhancing feed efficiency and weight gain in large-scale field studies. Fish Shellfish Immunol. 35, 1585–1590. doi: 10.1016/j.fsi.2013.09.008
Kuebutornye F. K. A., Tang J. F., Cai J., Yu H., Wang Z. W., Abarike E. D., et al. (2020). In vivo assessment of the probiotic potentials of three host-associated Bacillus species on growth performance, health status and disease resistance of Oreochromis niloticus against Streptococcus agalactiae. Aquaculture 527, 735440. doi: 10.1016/j.aquaculture.2020.735440
Li J., Wu Z. B., Zhang Z., Zha J. W., Qu S. Y., Qi X. Z., et al. (2019). Effects of potential probiotic Bacillus velezensis K2 on growth, immunity and resistance to Vibrio harveyi infection of hybrid grouper (Epinephelus lanceolatus♂ × E. fuscoguttatus♀). Fish Shellfish Immunol. 93, 1047–1055. doi: 10.1016/j.fsi.2019.08.047
Lin S. Q., Zhuang R. F., Liu B., Qiao X. J., Lin Z. X., Zhou W. Z. (2021). Study on biological characteristics of a strain of Bacillus coagulans ZC-1. Feed Res. 44, 70–74. doi: 10.13557/j.cnki.issn1002-2813.2021.17.017
Liu H. T., Wang S. F., Cai Y., Guo X. H., Cao Z. J., Zhang Y. Z., et al. (2017). Dietary administration of Bacillus subtilis HAINUP40 enhances growth, digestive enzyme activities, innate immune responses and disease resistance of tilapia, Oreochromis niloticus. Fish Shellfish Immunol. 60, 326–333. doi: 10.1016/j.fsi.2016.12.003
Luo Y. W., Hao Z. K., Wang Y. G., Qu J. B. (2009). A Bacillus cereus isolates causing “rot-skin syndrome” of Stichopus japonicus. Fish. Sci. Technol. Information 36, 60–63. doi: 10.3969/j.issn.1001-1994.2009.02.011
Magnadottir B. (2010). Immunological control of fish diseases. Mar. Biotechnol. 12, 361–379. doi: 10.1007/s10126-010-9279-x
Manhar A. K., Saikia D., Bashir Y., Mech R. K., Nath D., Konwar B. K., et al. (2015). In vitro evaluation of celluloytic Bacillus amyloliquefaciens AMS1 isolated from traditional fermented soybean (Churpi) as an animal probiotic. Res. Vet. Sci. 99, 149–156. doi: 10.1016/j.rvsc.2015.01.008
Mathialagan K., Manickam R., Pachiappan P. (2018). Evaluation of probiotic potential of Bacillus spp. isolated from the digestive tract of freshwater fish Labeo calbasu (Hamilton 1822). Aquac. Rep. 11, 59–69. doi: 10.1016/j.aqrep.2018.07.001
Midhun S. J., Neethu S., Arun D., Vysakh A., Divya L., Radhakrishnan E. K., et al. (2019). Dietary supplementation of Bacillus licheniformis HGA8B improves growth parameters, enzymatic profile and gene expression of Oreochromis niloticus. Aquaculture 505, 289–296. doi: 10.1016/j.aquaculture.2019.02.064
Midhun S. J., Neethu S., Vysakh A., Arun D., Radhakrishnan E. K., Jyothis M. (2017). Antibacterial activity and probiotic characterization of autochthonous Paenibacillus polymyxa isolated from Anabas testudineus (Bloch 1792). Microb. Pathogen. 113, 403–411. doi: 10.1016/j.micpath.2017.11.019
Midhun S. J., Neethu S., Vysakh A., Radhakrishnan E. K., Jyothis M. (2018). Antagonism against fish pathogens by cellular components/preparations of Bacillus coagulans (MTCC-9872) and It’s in vitro probiotic characterization. Curr. Microbiol. 75, 1174–1181. doi: 10.1007/s00284-018-1506-0
Mukherjee A., Chandra G., Ghosh K. (2019). Single or conjoint application of autochthonous Bacillus strains as potential probiotics: Effcts on growth, feed utilization, immunity and disease resistance in Rohu, Labeo rohita (Hamilton). Aquaculture 512, 734302. doi: 10.1016/j.aquaculture.2019.734302
Nguyen T. L., Park C. I., Kim D. H. (2017). Improved growth rate and disease resistance in olive flounder, Paralichthys olivaceus, by probiotic Lactococcus lactis WFLU12 isolated from wild marine fish. Aquaculture 471, 113–120. doi: 10.1016/j.aquaculture.2017.01.008
Nie W., Liu L. H., Chai S., Wang J. Y. (2015). Toxic effects of Bacillus subtilis on goldfish. J. Wuhan Polytechnic Univ. 34, 1–9.
Nilnaj C., Chatchaleeya C., Somkiat P. (2011). Effect of dietary supplementation of brewers yeast and nucleotide singularly on growth, survival and vibriosis resistance on juveniles of the gastropod spotted babylon (Babylonia areolata). Aquacult. Int. 19, 489–496. doi: 10.1007/s10499-010-9364-1
Pedro N. D., Guijarro A. I., López-Patiño M. A., Martínez-Álvarez R., Delgado M. J. (2005). Daily and seasonal variations in haematological and blood biochemical parameters in the tench, Tinca tinca Linnaeus 1758. Aquacult. Res. 36, 85–96. doi: 10.1111/j.1365-2109.2005.01338.x
Pichard B., Larue J. P., Thouvenot D. (1995). Gavaserin and saltavalin, new peptide antibiotics produced by Bacillus polymyxa. FEMS Microbiol. Lett. 133, 215–218. doi: 10.1111/j.1574-6968.1995.tb07887.x
Polak-Berecka M., Waśko A., Paduch R., Skrzypek T., Sroka-Bartnicka A. (2014). The effect of cell surface components on adhesion ability of Lactobacillus rhamnosus. Antonie Van Leeuwenhoek 106, 751–762. doi: 10.1007/s10482-014-0245-x
Qi Z., Zhang X. H., Boon N., Bossier P. (2009). Probiotics in aquaculture of China — Current state, problems and prospect. Aquaculture 290, 15–21. doi: 10.1016/j.aquaculture.2009.02.012
Qin L., Xiang J. H., Xiong F., Wang G. T., Zou H., Li W. X., et al. (2019). Effects of Bacillus licheniformis on the growth, antioxidant capacity, intestinal barrier and disease resistance of grass carp (Ctenopharyngodon idella). Fish Shellfish Immunol. 97, 344–350. doi: 10.1016/j.fsi.2019.12.040
Reda R. M., El-Hady M. A., Selim K. M., El-Sayed H. M. (2018). Comparative study of three predominant gut Bacillus strains and a commercial B. amyloliquefaciens as probiotics on the performance of Clarias gariepinus. Fish Shellfish Immunol. 80, 416–425. doi: 10.1016/j.fsi.2018.06.031
Resende J. A., Silva V. L., Fontes C. O., Souza-Filho J. A., de Oliveira T. L. R., Coelho C. M., et al. (2012). Multidrug-resistance and toxic metal tolerance of medically important bacteria isolated from an aquaculture system. Microbes Environ. 27, 449–455. doi: 10.1264/jsme2.ME12049
Rieger A. M., Barreda D. R. (2011). Antimicrobial mechanisms of fish leukocytes. Dev. Comp. Immunol. 35, 1238–1245. doi: 10.1016/j.dci.2011.03.009
Ringø E., Olsen R. E., Mayhew T. M., Myklebust R. (2003). Electron microscopy of the intestinal microflora of fish. Aquaculture 227, 395–415. doi: 10.1016/j.aquaculture.2003.05.001
Sahu M. K., Swarnakumar N. S., Sivakumar K., Thangaradjou T., Kannan L. (2008). Probiotics in aquaculture: Importance and future perspectives. Indian J. Microbiol. 48, 299–308. doi: 10.1007/s12088-008-0024-3
Sánchez B., Delgado S., Blanco-Míguez A. B., Lourenço A., Gueimonde M., Margolles A. (2017). Probiotics, gut microbiota, and their influence on host health and disease. Mol. Nutr. Food Res. 61, 1600240. doi: 10.1002/mnfr.201600240
Selim K. M., Reda R. M. (2015). Improvement of immunity and disease resistance in the Nile tilapia, Oreochromis niloticus, by dietary supplementation with Bacillus amyloliquefaciens. Fish Shellfish Immunol. 44, 496–503. doi: 10.1016/j.fsi.2015.03.004
Sha Y. J., Wang L., Liu M., Jiang K. Y., Xin F., Wang B. J. (2016). Effects of lactic acid bacteria and the corresponding supernatant on the survival, growth performance, immune response and disease resistance of Litopenaeus vannamei. Aquaculture 452, 28–36. doi: 10.1016/j.aquaculture.2015.10.014
Sun Y., He M. W., Cao Z. J., Xie Z. Y., Liu C. S., Wang S. F., et al. (2018). Effects of dietary administration of Lactococcus lactis HNL12 on growth, innate immune response, and disease resistance of humpback grouper (Cromileptes altivelis). Fish Shellfish Immunol. 82, 296–303. doi: 10.1016/j.fsi.2018.08.039
Sun Y., Hu Y. H., Liu C. S., Sun L. (2010). Construction and analysis of an experimental Streptococcus iniae DNA vaccine. Vaccine 28, 3905–3912. doi: 10.1016/j.vaccine.2010.03.071
Tachibana L., Telli G. S., de Carla Dias D., Gonçalves G. S., Ishikawa C. M., Cavalcante R. B., et al. (2020). Effect of feeding strategy of probiotic Enterococcus faecium on growth performance, hematologic, biochemical parameters and non-specific immune response of Nile tilapia. Aquac. Rep. 16, 100277. doi: 10.1016/j.aqrep.2020.100277
Tao J. J. (2023). Effects of dietary supplementation of probiotic cultures on growth, antioxidant capacity and inflammatory response in juvenile largemouth bass, Micropterus salmoides. Shanghai Ocean University.
Torrecillas S., Makol A., Caballero M. J., Montero D., Robaina L., Real F., et al. (2007). Immune stimulation and improved infection resistance in European sea bass (Dicentrarchus labrax) fed mannan oligosaccharides. Fish Shellfish Immun. 23, 969–981. doi: 10.1016/j.fsi.2007.03.007
Tu Z., Li H., Zhang X., Sun Y., Zhou Y. C. (2017). Complete genome sequence and comparative genomics of the golden pompano (Trachinotus ovatus) pathogen, Vibrio harveyi strain QT520. PeerJ 5, e4127. doi: 10.7717/peerj.4127
Varin A., Gordon S. (2009). Alternative activation of macrophages: immune function and cellular biology. Immunobiology 214, 630–641. doi: 10.1016/j.imbio.2008.11.009
Velmurugan S., Palanikumar P., Velayuthani P., Donio M. B. S., Babu M. M., Lelin C., et al. (2015). Bacterial white patch disease caused by Bacillus cereus, a new emerging disease in semi-intensive culture of Litopenaeus vannamei. Aquaculture 444, 49–54. doi: 10.1016/j.aquaculture.2015.03.017
Wang W., Li M., Fang W. H., Pradhan A. K., Li Y. B. (2013). A predictive model for assessment of decontamination effects of lactic acid and chitosan used in combination on Vibrio parahaemolyticus in shrimps. Int. J. Food Microbiol. 167, 124–130. doi: 10.1016/j.ijfoodmicro.2013.07.012
Wang Q., Lu Q., Mao D. Q., Cui Y. X., Luo Y. (2017). The horizontal transfer of antibiotic resistance genes is enhanced by ionic liquid with different structure of varying alkyl chain length. Front. Microbiol. 6. doi: 10.3389/fmicb.2015.00864
Xiong T., Deng Y. J., Liao L. K., Song S. H., Guan Q. Q. (2015). Identification and antibacterial experiments of Lactobacillus reuteri NCU801. Food Fermentation Industries 41, 24–29. doi: 10.13995/j.cnki.11-1802/ts.201502005
Xu Y., Wang Y. G., Zhou Y. C., Zhang Z., Liao M. J., Li B., et al. (2018). Isolation, identification, and pathogen analysis of skin-ulcer disease in cultured groupers in Hainan Province. J. Hainan Univ. (Nat. Sci.) 36, 153–163.
Yang B. Y., Wang C. C., Hu H. H., Tu Y. Q., Han D. H., Zhu X. M., et al. (2015). Repeated handling compromises the immune suppression and improves the disease resistance in overwintering channel catfish (Ictalurus punctatus). Fish Shellfish Immunol. 47, 418–428. doi: 10.1016/j.fsi.2015.09.010
Yang Y. B., Yu L. X., Liu Y. T., Yang Q. H., Su Z. J., Song Y., et al. (2017). Isolation, identification and antibiotic sensitivity of Bacillus cereus from tilapia. Freshw. Fish. 47, 51–56. doi: 10.13721/j.cnki.dsyy.2017.04.008
Zhang H. Y., Wang H. B., Zhang Z. W., Zhao M. J., Xia L. (2019). Toxicological safety assessment of a potential probiotic strain Bcillus cereus D7 isolated from shrimp. Prog. Vet. Med. 40, 59–65. doi: 10.16437/j.cnki.1007-5038.2019.03.010
Zhang J., Wu Y., Gu S. B., Ma J. L., Tian P. P., Zhao L. N., et al. (2022). Genome mining, expression and activity determination of a novel antimicrobial peptide from Bacillus coagulans CGMCC 9951. Food Fermentation Industries 48, 71–78. doi: 10.13995/j.cnki.11-1802/ts.030314
Zhou Z. J., Qiu R., Zhang J. (2015). Molecular characterization of the cathepsin B of turbot (Scophthalmus maximus). Fish Physiol. Biochem. 41, 473–483. doi: 10.1007/s10695-014-9998-4
Zhou S., Song D. L., Zhou X. F., Mao X. L., Zhou X. F., Wang S. L., et al. (2019). Characterization of Bacillus subtilis from gastrointestinal tract of hybrid Hulong grouper (Epinephelus fuscoguttatus × E. lanceolatus) and its effects as probiotic additives. Fish Shellfish Immunol. 84, 1115–1124. doi: 10.1016/j.fsi.2018.10.058
Keywords: probiotics, probiotic cells, probiotic cultures, probiotic effects, immunity, disease resistance
Citation: Cao Z, Yang Q, Kang A, Wang G, Li P, Qiu G, Wang J, Liu C and Sun Y (2025) Probiotic properties of Bacillus licheniformis HN318 and comparison of the effects of its bacterial cells and cultures on growth, immunity and disease resistance of hybrid grouper (Epinephelus polyphekadion♂ × Epinephelus fuscoguttatus♀). Front. Mar. Sci. 12:1548955. doi: 10.3389/fmars.2025.1548955
Received: 20 December 2024; Accepted: 21 January 2025;
Published: 06 February 2025.
Edited by:
Jin Niu, Sun Yat-sen University, ChinaReviewed by:
Xulu Chang, Henan Normal University, ChinaCopyright © 2025 Cao, Yang, Kang, Wang, Li, Qiu, Wang, Liu and Sun. This is an open-access article distributed under the terms of the Creative Commons Attribution License (CC BY). The use, distribution or reproduction in other forums is permitted, provided the original author(s) and the copyright owner(s) are credited and that the original publication in this journal is cited, in accordance with accepted academic practice. No use, distribution or reproduction is permitted which does not comply with these terms.
*Correspondence: Chunsheng Liu, bGNzNTExM0AxNjMuY29t; Yun Sun, c3lzaHVpMjA3QDEyNi5jb20=
†These authors have contributed equally to this work
Disclaimer: All claims expressed in this article are solely those of the authors and do not necessarily represent those of their affiliated organizations, or those of the publisher, the editors and the reviewers. Any product that may be evaluated in this article or claim that may be made by its manufacturer is not guaranteed or endorsed by the publisher.
Research integrity at Frontiers
Learn more about the work of our research integrity team to safeguard the quality of each article we publish.