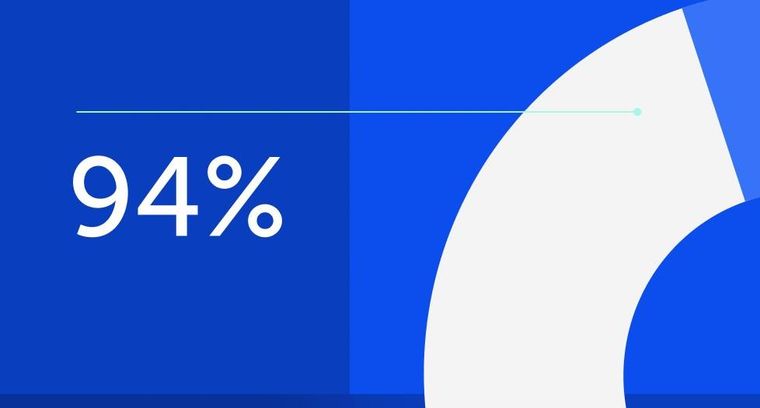
94% of researchers rate our articles as excellent or good
Learn more about the work of our research integrity team to safeguard the quality of each article we publish.
Find out more
REVIEW article
Front. Mar. Sci., 26 March 2025
Sec. Coral Reef Research
Volume 12 - 2025 | https://doi.org/10.3389/fmars.2025.1547870
Coral bleaching poses a severe threat to the health and survival of global coral reef ecosystems, with recent events surpassing historical heat stress records. To address this crisis, improved long-term monitoring, communication, and coordination are urgently required to enhance conservation, management, and policy responses. This study reviews global coral bleaching survey methodologies and datasets spanning 1963 to 2022, identifying key challenges in methodological standardization, including database duplication and inconsistencies in naming and reporting bleaching metrics. These issues hinder comparative analyses and contribute to discrepancies in global bleaching impact assessments. We developed a typology of twenty-nine coral bleaching methods used across various scales, encompassing remote sensing tools, underwater surveys, and specimen collection. Analysis of 77,370 observations from three major datasets revealed that 9.36% of entries lacked methodological descriptions. Among recorded methods, belt transects (42%), line and point intercept transects (33%), and random surveys (17%) were the most widely applied. Practitioner surveys underscored the dominance of in situ transect and visual methods, highlighting the growing adoption of photo quadrats—an emerging yet underrepresented technique in existing datasets. To enhance global coral bleaching assessments, we propose a standardized framework that ensures open access and accessible data that aligns with decision-makers’ needs for efficient data aggregation and interoperability to better understand temporal and spatial bleaching events. A globally coordinated coalition should unify protocols, improve data-sharing capabilities, and empower regional networks through targeted training, incentives, and open communication channels. Strengthening field capacity in coral taxonomy and standardized survey methodologies, alongside integrating advanced tools, will improve data quality and comparability. Additionally, creating precise geolocated datasets will bridge on-the-ground observations with advanced remote sensing systems, refining the accuracy of satellite-based early warning tools. Establishing interoperable online platforms will further streamline data integration and accessibility, providing a robust foundation to support global responses to coral bleaching and foster impactful conservation initiatives.
Coral bleaching is one of the greatest current threats to reefs worldwide (Heron et al., 2016; Hughes et al., 2017) and occurs when water temperatures exceed the thermal tolerance of corals (Glynn and D’Croz, 1990; Glynn, 1993). Although bleaching can occur naturally due to fluctuations in seasonal temperatures, sedimentation, and salinity, mass bleaching events have begun to emerge during all phases of the El Niño-Southern Oscillation (ENSO) due to the high heat content in the oceans (Claar et al., 2018; Muñiz-Castillo et al., 2019; Spady et al., 2022). These events have increased in frequency across all coral reef regions of the globe (Eakin et al., 2010; Hughes et al, 2018b; Sully et al., 2022; Virgen-Urcelay and Donner, 2023), presenting limited recovery from one event to the next (Mason et al., 2020). Global estimates suggest we have lost half of the world’s corals (Eddy et al., 2021), and the International Union for Conservation of Nature (IUCN) Red List Coral Assessment estimates that 44% of reef-building coral species globally are threatened with extinction due to climate change and other stressors (Gutierrez et al., 2024). These staggering losses demand an urgent reduction of global carbon emissions and an effective robust approach to global coral bleaching monitoring (Hoegh-Guldberg et al., 2023).
Data from ongoing monitoring efforts have significantly influenced international policy (Obura et al., 2019). The Paris Agreement under the United Nations Framework Convention on Climate Change (UNFCCC) was shaped by data highlighting the severe impact of rising sea temperatures on coral reefs, driving the inclusion of temperature rise limits to keep the global average temperature increase below 2°C above pre industrial levels, and ideally 1.5°C, to protect reefs (Obura et al., 2019). Similarly, the impacts of coral bleaching have guided the Convention on Biological Diversity (CBD) Aichi Biodiversity goals, particularly Target 8, which aims to minimize the impacts of climate change on biodiversity and build resilience (CBD, 2024). The Intergovernmental Panel on Climate Change (IPCC) incorporates extensive heat stress projections in its assessment reports, emphasizing the urgent need for mitigation and adaptation measures to protect coral reefs from climate change (IPCC, 2023). Furthermore, based on these data, the United Nations Environment Program (UNEP) has developed global guidelines and policy recommendations for coral reef management and climate change adaptation, integrating reef resilience into marine spatial planning (Souter et al., 2021). Lastly, the International Coral Reef Initiative (ICRI) and its operative network, the Global Coral Reef Monitoring Network (GCRMN), use long-term data sets on coral reef status and trends to generate comprehensive reports that guide international policies and conservation strategies (Donovan et al., 2021; Souter et al., 2021; Sully et al., 2019, Sully et al., 2022).
Several global monitoring networks and regional initiatives with standardized survey methodologies currently contribute data to many prominent online platforms, facilitating meta-analyzing of coral reef conditions (Donovan et al., 2021; Lachs et al., 2021; McClanahan et al., 2019; Sully et al., 2019, Sully et al., 2022). These platforms monitor key indicators of reef health with set monitoring time frames that may not coincide with bleaching events. However, these programs offer bleaching and non-bleaching observations that allow the creation of comprehensive global databases (Donner et al., 2017; Spady et al., 2022; van Woesik and Kratochwill, 2022; Virgen-Urcelay and Donner, 2023). These efforts encompass surveys conducted by organizations such as Reef Check (Sully et al., 2019), Wildlife Conservation Society (McClanahan et al., 2019, McClanahan et al., 2020) and CoralWatch (Siebeck et al., 2006; Marshall et al., 2012) alongside long-standing regional programs such as Atlantic and Gulf Rapid Reef Assessment (AGRRA) in the Caribbean (Ginsburg and Lang, 2003), the National Coral Reef Monitoring Program (NCRMP) across the United States and territories, the Coastal Ocean Research and Development in the Indian Ocean (CORDIO), and the Australian Institute of Marine Science’s Long-Term Monitoring Program, among many others (van Woesik and Kratochwill, 2022; Virgen-Urcelay and Donner, 2023). These networks and other bleaching monitoring groups provide data to the National Oceanic and Atmospheric Administration (NOAA) Coral Reef Watch Program to help validate early warning systems based on sea surface temperature anomalies (Eakin et al., 2010).
Different methodologies and approaches can evolve naturally when led by different organizations. However, methodological incompatibility between organizations inhibits globally integrated datasets to determine the status and trends of coral bleaching. Currently, a plethora of in situ coral bleaching assessment methods are in use, from data gathered at the cellular level to information gleaned from satellites and from basic visual field estimates to more precise digital calculations from in situ survey photos and machine learning programs (Donovan et al., 2023; Hedley et al., 2016; Madin et al., 2019; Obura et al., 2019; Teague et al., 2020; van Woesik et al., 2022). Given the breadth of available assessment options, it remains challenging to compare data gathered at different reef locations across space and time, complicating our understanding of coral reef health (Delaval et al., 2021; Wicquart et al., 2022). For instance, the GCRMN has noted that a lack of integrated datasets hampers seamless cross-compatibility and large-scale analyses of ecological datasets of coral reefs (Wicquart et al., 2022). The differences in resources and capacities between regions further compound this issue. To successfully monitor reef health in an era of rapid change, we must coordinate globally to guide policy and urgently address longstanding data integration and methodological standardization issues, which have persisted since the inception of global coral bleaching protocols over three decades ago (Ginsburg and Lang, 2003; Hill and Wilkinson, 2004; Marshall et al., 2004).
Here, we provide a comprehensive review of the methodologies and data sets available from 1963 to 2022 for global coral bleaching surveys, detailing the methods used and their temporal and spatial coverage. Our objective is to inform and improve conservation efforts by synthesizing the current state of coral bleaching methods and offering recommendations for future spatiotemporal data harmonization of bleaching events. We emphasize that improving data standardization will benefit and facilitate the integration of advanced technology and predictive tools for coral reef management and conservation. Standardization is imperative to support links to globally relevant policies such as target eight of the Global Biodiversity Framework (“Minimize the Impacts of Climate Change on Biodiversity and Build Resilience”) and other efforts to address the threats of coral reefs worldwide (CBD, 2024).
Based on an analysis of three global coral bleaching databases, a practitioner survey, and a review of gray and scientific literature, we developed a conceptual framework to summarize the current coral bleaching assessment methods. We structured our framework using broad categories of methods (remote sensing, underwater surveys, and specimen collection) and metrics used to measure bleaching. This typology constitutes a visual aid in understanding the breadth of bleaching monitoring methods and data collection types.
We compiled three of the most comprehensive global databases of coral bleaching surveys, covering observations from 1963 to 2022: (a) van Woesik and Kratochwill, 2022 (1980–2020) with 34,846 observations; (b) Virgen-Urcelay and Donner, 2023 (1963-2017) with 37,774 observations; and (c) CoralWatch, 2022 (2003-2022) with 367,350 observations obtained from https://biocollect.ala.org.au/coralwatch (Siebeck et al., 2006; Marshall et al, 2012). These databases included data on bleaching and non-bleaching observations. Incorporation of other available regional coral bleaching databases was beyond the scope of this paper.
To eliminate duplicate observations and locations, we comprehensively cross-referenced the database (Figure 1). This involved comparing entries according to date, geographic coordinates, depth, and survey method. All data entries were standardized by method name according to our typology and reclassified accordingly. Since many observations did not explicitly indicate an assessment method, we manually searched sources to find this missing information when possible. We considered these two independent methods if two distinct types of surveys were done at a single site (e.g., point intercept transects and belt transects). We used the following quality control workflows for each database:
A. van Woesik and Kratochwill, 2022: we reviewed each data provider and removed duplicate data. For example, we removed Reef Check and CoralWatch from Kumagai and Yamano, 2018 (668 observations) to prevent duplicates. We obtained methods for the data provided by Safaie et al. (2018) by manually reviewing the supplementary material and associating a method with observations when possible. We also removed data from (Donner et al, 2017) (7429 observations) since we included a more recent version of this data set. After filtering by date, geographic coordinates, depth, and survey method, we obtained 32,954 site-level observations.
B. Virgen-Urcelay and Donner (2023) database: we recategorized observations with an associated percentage of bleaching to visual estimates but did not specify a visual assessment method. We did this for data compiled by Eakin et al. (2010), personal communications, and CORDIO data originally submitted to NOAA Coral Reef Watch (CRW). After filtering for date, geographic coordinates, depth, and survey method, we obtained 24,309 site-level observations.
C. CoralWatch database: All observations were made using the Coral Health Chart (Siebeck et al., 2006; Marshall et al., 2012) and were labeled random surveys. At some sites in the CoralWatch database, two methods were used, including the random survey and georeferenced photo-quadrat methods; in these cases, we included two methods in our database conducted at the same site. After filtering for date, geographic coordinates, depth, and survey method, we obtained 12,863 site-level observations.
Figure 1. Workflow analysis of three coral bleaching databases, resulting in 70,126 individual observations with associated methods from 1963 to 2022.
We also developed a map to visualize the density and distribution of global bleaching monitoring efforts. To do so, we overlaid a longitude/latitude grid on the tropical/subtropical waters where coral reefs are found and quantified the number of observations within ≈ 0.698 square degrees (assuming values near the equator) hexagonal cells. These analyses were performed using QGIS tools from version 3.16.1-Hannover.
From June to December 2021, we sent a survey (via a Google form; see Supplementary Material) with 15 questions to various listservs and webmail sites such as Coral-List Digest and newsletters for ICRI, the Reef Resilience Network, Seven Seas, and Western Indian Ocean Marine Science Association, among other networks, to reach managers, scientists, and other practitioners across the globe with practical experience in the implementation of coral bleaching field methodologies. Participants were asked to provide information on the coral bleaching assessment method used by their organization and their location within NOAA’s 18 monitored regions that encompass the 214 virtual regions of Coral Reef Watch (https://coralreefwatch.noaa.gov/product/vs/data.php). These regions were then grouped into four areas: the Pacific Ocean, the Atlantic Ocean, the Indian Ocean, and the Middle East.
Respondents were also asked to select their preferred coral bleaching assessment method(s) from a list of twelve options, with the opportunity to supply additional methods in an open-ended ‘other’ submission field. We also asked respondents about their rationale for selecting certain methods, constraints that limited their choices, and the time frame of their monitoring efforts (these time frames incorporate major global bleaching events, that is, historical/before 1997, 1998–2004, 2005–2014 and 2015–2021).
Our conceptual diagram organizes the twenty-nine coral bleaching monitoring methods identified in our global database/literature review and practitioner survey (Figure 2). We grouped methods by their spatial scale and extent covered into three categories: (A) remote sensing tools (monitoring methods conducted from air, that is, drones, airplanes or satellites in space), (B) underwater surveys (both diver-based and remote vehicles) and (C) specimen collection (samples of corals further analyzed in a laboratory) (Hedley et al., 2016; Obura et al., 2019; Teague et al., 2020; McLachlan et al., 2020; Thurber et al., 2022; Donovan et al., 2021; van Woesik et al., 2022).
Figure 2. Typology of coral bleaching monitoring methods at different scales of measurement: (A) Remote sensing tools (scale: global to cm), (B) underwater surveys (scale: km to cm), and (C) specimen collection (scale: m to mm). This progression from satellites to divers offers increasing detail but covers smaller geographic areas. The colored lines refer to the seven types of bleaching metrics shown in the legend (see Table 1). Methods with multiple-colored lines were associated with more than one type of metric. Further details are provided in Table 2 and Table 3.
Coral bleaching is evaluated and monitored using a range of methods and metrics (Figure 2). These methods - remote sensing tools, underwater surveys, and specimen collection - allow data collection across seven types of bleaching metrics: (1) predicted bleaching risk, (2) extent of bleached area, (3) presence or absence of bleaching at a site, (4) cover of bleached corals at the site level, (5) extent and severity at the coral colony level, (6) spectral signature, and (7) bleaching at the cellular level (Table 1). These metrics are broad, capturing various indicators related to coral health based on the data collected. Together, these methods and metrics offer a multifaceted approach to understanding and monitoring coral bleaching.
Table 1. Detailed descriptions of the types of bleaching metrics and data collection categories, with examples of the methods and programs that implement these methods.
Remote sensing tools (Table 2) provide broad sea surface temperature (SST) measurements to develop heat stress indices that help predict coral bleaching events (Hedley et al., 2016). Satellite imagery can also reveal bleaching through spectral and bottom reflectance analysis (Ziskin, 2011; Li, 2020; Bonelli et al., 2024). Airborne tools, such as planes, helicopters, and Unmanned Aerial Systems (UASs, also known as drones), enable visual bleaching identification, and with specialized sensors, capture more detailed images using airborne spectroscopy (Hedley et al., 2016; Levy et al., 2018). Even more advanced technologies, such as hyperspectral sensors available on both satellites and airborne platforms, offer high precision but are limited by costs and smaller coverage areas (Asner et al., 2021a; Nguyen et al., 2021).
Table 2. Description of the methods, bleaching metrics and scale used for remote sensing tools used for bleaching monitoring.
Underwater methods (Table 3) to assess coral bleaching in the field encompass remote technologies (3 methods), direct visual surveys (10 methods), digital imaging (5 methods), and bio-optical analysis (3 methods). Each method provides a unique perspective on evaluating bleaching. For underwater observations, remotely operated vehicles (ROVs) and unmanned underwater vehicles (UUVs) provide fine-scale detail (Cardenas et al., 2024; Obura et al., 2019; Teague et al., 2020). Visual methods include techniques in which observers swim along transects or freely to identify bleaching at the genera or species level (Donovan et al., 2021; Jokiel et al., 2015; Teague et al., 2020; van Woesik et al., 2022). Digital methods require cameras for video or photographs, which can be stitched together to form mosaics and/or 3D reef models of a set area (Jokiel et al., 2015; Kopecky et al., 2023; Teague et al., 2020). Specimen collection (Table 3; three methods) involves divers taking coral samples, which are then further analyzed in a laboratory for genetic, physiological, or histological analysis (McLachlan et al., 2020; Thurber et al., 2022).
Table 3. Description of the methods, bleaching metrics and scale used for underwater surveys and specimen collection for bleaching monitoring.
Of our initial duplicated total of 77,370 surveys, 7,244 (9.36%) could not be assigned a method even after a thorough manual review. Therefore, we assessed the remaining 70,126 surveys, which included bleaching and non-bleaching observations from van Woesik and Kratochwill, 2022 (1980–2020) with 32,954 observations, Virgen-Urcelay and Donner, 2023 (1963-2017) with 24,309 site-level observations, and CoralWatch, 2022 (2003-2022) with 12,863 observations (Figure 1). Although a total of 15 different coral bleaching field methods were identified, three methods accounted for ~92% of the observations that specified a method. These where belt transects (41.88%), line and point intercept transects (33%), and random surveys (16.90%, most of which were color cards). The methods associated with the remaining ~8% of the data points included visual estimates (2.68%), aerial surveys (1.87%), photo quadrats and transects (1.84%), and to a lesser extent quadrats surveys (0.5%), another unspecified type of transect (0.4%), manta tow (0.3%), among other methods (Figure 3A).
Figure 3. (A) Main methods used for coral bleaching assessments based on three global databases, (B) Temporal distribution of coral bleaching methods from all databases during 1990-2022.
The first major global bleaching event in 1998 resulted in numerous monitoring efforts (Berkelmans et al., 2004; McClanahan et al., 2001; McField, 1999). Most observations were made using belt transects and line and point intercept methods until ~2015, after which additional methods such as photo quadrats and transects became more common (Figure 3B). Aerial surveys appeared only in the databases in 1998 and 2002 and for a specific geographic area (Great Barrier Reef), while visual estimates were common between 2005 and 2015. Random surveys started to increase from 2010 onward, peaking in 2019. Bleaching observations were highest in 2005-2006 (coinciding with a mass bleaching event in the Caribbean), with a notable dip in surveys in 2018 when no mass bleaching occurred.
Bleaching observations were unevenly distributed (Figures 4A–D). Within the tropical and subtropical waters where coral reefs are found, the density of coral bleaching survey observations ranged from 1 to 3,000 per 0.698 square degrees (~ 8,602.25 km² assuming values near the equator), with the highest densities in the Bay Islands of Honduras and Florida in the Caribbean (Figure 4B), in a section of the coral reef triangle that includes Malaysia, Thailand, Hong Kong and the Philippines in East Asia (Figure 4C); and along the Great Barrier Reef of Australia (Figure 4D).
Figure 4. (A) Spatial distribution and density of coral bleaching surveys in the habitable range of coral reefs globally, shown as the number of observations per 0.698 square degrees (~8,602.25 km² assuming values near the equator) hexagonal cells, (B) in the Caribbean, (C) in Southeast Asia, and (D) the Great Barrier Reef of Australia.
In the practitioner survey, 122 respondents identified fourteen different methods for monitoring coral bleaching, many using multiple approaches, resulting in a combined total of 384 responses. Most of the respondents monitored sites in the Pacific (52.60%, n = 81), followed by the Atlantic (28.57%, n = 44), the Indian Ocean (13.64%, n = 21) and the Middle East (5.19%, n = 8). The four most common methods were combined line and point intercept transects (17.97%), photo-quadrats (16.93%), belt transects (16.67%), and visual estimates (16.67%), followed by random surveys (7.55%), video transects (6.51%), permanent transects (5.21%) and manta tows (3.91%) (Figure 5). The less frequently used methods in the other category included bar drop (2.34%), drone imagery (2.34%), aerial surveys (1.82%), photogrammetry (1.56%), quadrat (0.26%), and photos (0.26%) (Figure 5).
Figure 5. The main coral bleaching methods identified in practitioner surveys conducted from June to December 2021.
Monitoring participation evolved, with 11% of respondents involved before 1997. Engagement increased slightly to 12.62% during 1998–2004, the first global coral bleaching event in 1998. A marked increase was observed between 2005 and 2014, with 27.67% of respondents contributing during this period, which included the mass bleaching event in the Caribbean (2005) and the second global bleaching event (2010). Most respondents (53.40%) reported monitoring between 2015 and 2020, coinciding with the third global bleaching event (2014–2017). It is important to note that this survey was conducted before the onset of the fourth global bleaching event (2023-2024).
The preferences of the survey method were mainly influenced by long-term institutional implementation (35%) and the use of the scientific literature (29%), with fewer based on feasibility for citizen scientists (23%) and the least common reason being the funding of specific methods (5%). The survey results showed that 67.7% of the respondents collaborate with a network response team or a group of stakeholders to monitor coral bleaching, while 32.3% do not participate in such collaborations. The main constraints on the implementation of the method reported by the respondents were a lack of funding (44%), an untrained monitoring team (28%), logistical challenges (20%), and other factors such as limitations in rapid responses, staffing, and the impact of COVID-19 (7%).
As part of the practitioner review, a list of coral health monitoring programs, online platforms, dashboards, and their respective geographic regions was identified and synthesized in Table 4.
The declaration of a fourth global bleaching event, coupled with increasingly urgent climate forecasts, underscores the critical need for improved communication and coordination in coral bleaching monitoring (Reimer et al., 2024). While past research has documented the impacts of bleaching (Eakin et al., 2019; Hughes et al., 2018a; van Woesik and Kratochwill, 2022), there has been limited focus on the diverse methodologies used in data collection. This gap contributes to inconsistencies in global bleaching metrics, as variations in methods, scales, and timing obscure the specific findings and underlying drivers of coral bleaching variability (Khen et al., 2023). Integrating multiple data collection techniques across spatial scales would improve comparability and enhance regional trend analysis. A hierarchical approach to survey methods that provide metrics of percent coral cover and bleaching percent cover, ranging from beginner-friendly techniques like Reef Check point intercept transects and quadrats, to more advanced photo quadrats and belt transect-based assessments (e.g., AGRRA), could help bridge this gap. Standardized approaches are urgently needed to accurately track and address this growing crisis.
To address this, we provide the following key recommendations to help improve coordination, interoperability, and standardization in coral bleaching assessments.
a. Global coalition and standardized indicators: Strengthen and formalize a global coalition of organizations (e.g., such as GCRMN, ICRI, and NOAA among others) and monitoring platforms (e.g., Reef Check, AGRRA, MERMAID among others) and promote partnership with local, regional, and global networks. A working group of bleaching-monitoring organizations could define key policy- indicators and standardize metrics (e.g., percentage cover bleaching, percentage cover of bleached colonies) by harmonizing protocols to enable seamless data integration. To maximize impact, the coalition should drive consistent communication, provide incentives, facilitate comprehensive training in key methods, and secure dedicated funding.
b. Capacity building and monitoring expansion: Strengthening expertise in identifying bleaching taxa and training diverse stakeholders (scientists, communities, citizen scientists) is crucial. This effort should be complemented by expanding survey areas to improve spatial coverage, ensuring a more even distribution of monitoring sites, and enhancing geolocation accuracy for precise data collection. A concrete step forward would be to establish regional training programs with secured funding and certification frameworks. This approach would ensure consistency in data collection and empower local communities to contribute effectively to long-term reef health assessments.
c. Integration of technologies and consistency: Combining advanced technologies (photogrammetry or georeferenced photo quadrats) and AI-assisted image analysis with traditional methods (line and point intercept) enhances data accuracy, ensures consistent collection, and supports regional and global comparisons to better understand coral bleaching dynamics. We argue that all major bleaching monitoring organizations and entities should continue to incorporate these tools into their protocols now and in the future.
d. Integrate coral bleaching assessment data using interoperable online platforms. To create global coral bleaching databases, online management systems must actively integrate regional networks and local programs while enforcing common standards such as FAIR principles (Findable, Accessible, Interoperable, and Reusable). We encourage the major bleaching monitoring programs and online platforms (Table 4) to develop collaborative application programming interfaces (APIs), open access datasets with standardization of methods and metrics or data outputs to facilitate data sharing.
These recommendations will strengthen and unify global coral health monitoring, producing a single, powerful, data-driven voice that can highlight the impacts of heat stress on reefs and inform regional decision-making, global policy, and climate targets. Establishing interoperable information systems and fostering cross-network collaboration will support the streamlining of data sharing, ensure consistency across diverse datasets, and enable comprehensive large-scale analyses critical to driving impactful coral conservation initiatives (Wicquart et al., 2022). Operationalizing these recommendations will ensure accurate assessments of bleaching trends, support more informed conservation strategies, and help decision makers prioritize interventions and allocate resources effectively, advancing global efforts to protect and preserve coral reefs in the face of climate change.
Our study extensively reviewed the temporal and spatial coverage of global coral bleaching monitoring methods. In particular, belt, line, and point intercept transects emerged as the most used methods worldwide, a trend supported by both database analyses and practitioner surveys (van Woesik and Kratochwill, 2022; Virgen-Urcelay and Donner, 2023). These results highlight that trained citizen science programs are the primary collectors of publicly available coral bleaching data. We also identified widespread duplication across databases and inconsistencies in the naming and reporting of bleaching measurements (Reef Base, 2016; van Woesik and Kratochwill, 2022; Virgen-Urcelay and Donner, 2023; Spady et al., 2022).
Our analysis highlighted discrepancies between the most widely reported methods in global databases and those prevalent among surveyed practitioners. While belt, line, and point intercept transects predominated in the databases, practitioners indicated a higher use of photo quadrats, an emerging method. This suggests that much of the data generated from photo-quadrat data has only recently begun to be used and thus has not yet been integrated into global databases. Another hypothesis is that these photo-quadrat data remain confined to individual studies and are not yet fully integrated into public databases. A key modern challenge is scaling up survey data consistently to the island or country level, where NGOs and local marine resource agencies coordinate multiple photo quadrat surveys across reefs, integrating and standardizing individual datasets for broader ecological assessments. Despite the availability of open-source tools like Coral Net (coralnet.ucsd.edu) and ReefCloud (reeflcloud.org), which facilitate the analysis and synthesis of photo quadrats on a global scale, further efforts are required to incorporate these data into larger datasets. The emergence of artificial intelligence tools, which streamline image annotation and analysis, holds promise to enable bridging this gap by enabling faster data integration. Furthermore, high-resolution methods, such as video surveys and photogrammetry techniques, were described in the literature but were conspicuously absent from global databases and rarely reported by practitioners (Kopecky et al., 2023; Mallet and Pelletier, 2014). This gap underscores a lag in the integration of these methods. Furthermore, photogrammetry is an emerging advanced technique; its high cost and resource-intensive nature may render it less accessible.
Large-scale programs like Reef Check, AGRRA, NCRMP, and AIMS conduct coral health monitoring surveys over specific intervals, across various habitats worldwide; however, programs may not necessarily target bleaching events. Although these datasets contribute valuable non-bleaching observations to coral bleaching databases, their monitoring protocols during bleaching events often differ from their standard methodologies, introducing further inconsistencies. Also, vast yet understudied areas, such as the Coral Triangle, home to the world’s highest coral diversity, face inconsistent bleaching assessments, reducing the reliability of regional risk projections. Consequently, many programs are designed for rapid assessments during specific bleaching events, rather than comprehensive, long-term monitoring, complicating efforts to achieve globally comparable datasets.
Bleaching is often captured at different spatial scales and data may not be interchangeable or need to be transformed to be compared. The lack of reporting standards of different survey techniques can create inconsistencies in coral bleaching data and may lead to discrepancies in interpretation (Eakin et al., 2019; Hughes et al., 2018c; van Woesik and Kratochwill, 2022). Understanding the different bleaching metrics across methods and within organizations’ protocols (e.g. severity indices, percent cover estimates, or colony-level assessments) supports comparability and integration of global data sets (Figure 6A–H) (Bruno et al., 2001; Glynn et al., 2001; McClanahan, 2004; Carroll et al., 2017; Khen et al., 2023). Standardized metrics, clear definitions (in terms such as bleaching prevalence, severity, susceptibility, and frequency (Khen et al., 2023), and observer training are essential to minimize differences in data collection to ensure bleaching assessments are comparable.
Figure 6. Schematic visual showing detailed bleaching metrics that can be obtained from eight different methods (base image was modified from Bongaerts et al. 2021).
Figure 6. provides examples of bleaching metrics measured in eight methods. For example, visual assessments may rely on qualitative descriptions (e.g., pale, fully bleached) used in scoring systems (e.g., None (<1%), low (1-10%), medium (10-50%), high (50-90%), extreme (>90%) qualitatively based on bleached coral cover (Obura, 2015) (Figure 6A) and are usually conducted in timed counts or per site. Timed counts depend on the protocol, coral diversity, and survey conditions, requiring divers to identify healthy and bleached corals while swimming through a reef, making them effective for rare species. However, in regions with high coral diversity, it can be challenging to apply, though it still provides a valuable bleaching metric by calculating the percentage of colonies affected, offering insights distinct from traditional percent cover assessments. The Coral Health Chart from CoralWatch, designed for citizen scientists, uses 24 color shades to assess coral condition, requiring a minimum of 20 colonies per site and focusing on boulder, branching, plate, and soft corals; itis often difficult to compare across bleaching studies (Marshall et al., 2012) (Figure 6B) Line intercept transects are among the most common methods that can obtain coral cover, species composition, and bleaching percent cover among other benthic attributes, yet outputs vary substantially depending on the protocol (Figure 6C) (Hill and Wilkinson, 2004; Marshall et al., 2004; Obura, 2015). Even the same type of method may calculate different bleaching metrics. For example, Belt transects in Reef Check obtain the proportion of bleaching colonies at the site-level (Figure 6D). Belt transects from AGRRA obtain bleaching percentages in the planar view of individual colonies (Ginsburg and Lang, 2003). Other methods such as the WCS rapid bleaching protocol include area estimates and seven bleaching intensity categories (Figure 6E). Photo quadrats’ bleaching metrics may vary but are usually based on percent cover bleached with the support of photo software (Figure 6F). In the case of bar drop, used in the Mesoamerican Region, the percent bleached live tissue is calculated within two hundred colonies in a site with no metrics calculated for the surveyed area (Figure 6G).
Overall, there is limited data comparing bleaching across methods, although some exist, they show varying results estimating benthic attributes. A study by Couch et al. (2021) found that annotators analyzing photogrammetry recorded significantly higher bleaching levels than diver transect surveys. Similarly, in situ line-intercept transects produced higher coral cover estimates than photo quadrats and photogrammetry. Among all methods, surface analyses on orthomosaics yielded the lowest and most consistent coral cover estimates (Urbina-Barreto et al., 2021). Overall, photogrammetry provides the most detailed assessments, offering a high-resolution perspective on reef conditions (Figure 6H).
Furthermore, the lack of specified methods across datasets significantly hinders the ability to objectively compare and assess the impact of coral bleaching over time. In this study, 9% of the analyzed observations lacked an associated method, underscoring the urgent need for improved data quality and mandatory documentation of methods. Knowing the survey method allows for more accurate comparisons and calculations, so without that information, the comparison may become inaccurate. Additionally, understanding the area assessed is equally critical. Since different methods capture bleaching at varying spatial scales, the absence of methodological context makes it difficult to separate actual coral responses from survey bias. This issue extends beyond bleaching data to coral monitoring in general. The Status of Coral Reefs of the World: 2020, the most comprehensive compilation of long-term coral reef condition data, revealed that while many countries featured data collected by point intercept transects (47%) and photo quadrats (20%), a substantial number of database entries (27%) lacked information on the method type (Delaval et al., 2021). This lack of methods metadata is a pervasive problem, further complicating efforts not only to compare and analyze coral reef data on a global scale, but also at the country- or island-level.
Our study revealed a significant upward trend in coral bleaching data collection over time, with notable surges during major bleaching events. Monitoring efforts intensified in the late 1990s (Figure 3B), following the first recorded global coral bleaching event (Berkelmans et al., 2004; Donner et al., 2017). It is important to note that the decline during 2020-2022 is since Coral Watch was our only database that included data from 2020 until early 2022.
The emergence of innovative approaches to monitoring coral bleaching, particularly the integration of remote sensing technologies with standardized field techniques, marks a pivotal advancement in global coral monitoring efforts. The increase in satellite-based methods and airborne sensors over the past decade highlights the transformative potential of these technologies to enhance our ability to monitor coral bleaching on a large scale. These innovations leverage higher resolution satellite imagery, integrate biophysical parameters such as depth and waves, object-based contextual editing, advanced processing algorithms, and consistent reef mapping, providing cost-effective and extensive monitoring capabilities (Lyons et al., 2020; Kennedy et al., 2021; Roelfsema et al., 2021). Moreover, the latest remote sensing mapping approaches allow the integration of multiple criteria - such as water quality, wave exposure, spectral wavelengths, and light stress - into comprehensive datasets that can strengthen prediction models for coral bleaching (Li, 2020; Mason et al., 2020; Skirving et al., 2019). Linking satellite-based predictions with in-situ datasets from long term monitoring sites across spatial scales as well as on-site bleaching responses is a critical modern challenge to further refine predictions. Academic institutions have long term ecological research stations that can provide multi-parameter monitoring to help refine predictions (such as Centre for Island Research and Environmental Observatory (CRIOBE) in French Polynesia). Satellite-derived temperature metrics remain invaluable in predicting heat stress events globally consistently across time and space, allowing reliable long-term comparisons and early warning systems, a capability that can be further reinforced by advances in technology. However, while satellite data provide broad and consistent global coverage, it may lack the fine-scale resolution necessary to assess localized coral health.
Airborne imaging spectroscopy, when combined with in situ spectral data has the potential to map the phenotypic variations and thermal tolerance of coral species during bleaching events (Drury et al., 2022). Although these methods can be expensive and have seen limited application thus far, advancements in artificial intelligence (AI), photogrammetry, machine learning, and image analysis are poised to significantly enhance our ability to identify patterns and trends within extensive datasets, leading to more informed decision-making (González-Rivero et al., 2020; McCarthy et al., 2024).
Emerging technologies can be integrated with traditional monitoring methods (Urbina-Barreto et al., 2021). Techniques such as photo quadrats and photogrammetry offer high-detail insights into reef changes and enable cross-referencing with established methods, such as belt, line, and point-intercept surveys, to support long-term monitoring and data sets (Urbina-Barreto et al., 2021). These go-to methods may have the most direct inter-compatibility with past data. The fusion of diverse, georeferenced, and standardized field techniques, enriched with comprehensive metadata, creates a solid framework for refining satellite-based early warning systems, such as those by NOAA Coral Reef Watch (CRW) and the Allen Coral Atlas (Hedley et al., 2016; Xu et al., 2020). However, it is crucial to assess the feasibility of these high-tech methods, as they may be impractical for communities and countries in tropical regions, especially in Small Island Developing States. Setting resource-intensive monitoring standards could inadvertently widen existing geographic disparities in monitoring capabilities, potentially leaving some regions without accessible tools for coral health assessment.
NOAA CRW, which operates the world’s most widely used global early warning system for coral bleaching, utilizes in situ coral bleaching data to validate its metrics based on sea surface temperature (SST) that are used for predictions (e.g., hotspots, DHW, and bleaching alerts) (Skirving et al., 2020). In situ data are essential for ground-truth satellite-derived SST heat stress metrics and other predictive products, ensuring they accurately represent real-world conditions. This localized data is critical not only for refining model predictions, but also for supporting proactive monitoring, enabling targeted actions to mitigate bleaching impacts before and during thermal stress events. By providing direct insight into coral responses, in situ observations allow NOAA CRW to improve prediction accuracy and strengthen risk assessments and communication of bleaching threats across regions.
Similarly, the Allen Coral Atlas, another remote sensing tool, offers a coral bleaching monitoring system that tracks changes in bottom reflectance of coral and algae-mapped habitats, supported by various global coral reef mapping products (Asner et al., 2021b; Lyons et al., 2020). Although the Atlas’s first-generation algorithms have been tested in areas such as the Hawaiian Islands, French Polynesia, Fiji, and parts of the Great Barrier Reef, ongoing refinement is needed based on feedback on locations, extents, and severity of bleaching events (Bonelli et al., 2024; Carlson et al., 2022; Xu et al., 2020). Although this broader-scale technology provides high spatial and temporal resolution for coral bleaching data, its effectiveness is based on calibration with standardized field data. To further improve the accuracy and validation of satellite-based monitoring products, it is essential to expand geographical coverage and geolocate field data from in-water reef surveys. For example, georeferenced photo transects provide an opportunity to record geotagged reef photographs over a larger reef track (200m up to 1km) and may provide a detailed level of georeferenced observations across multiple habitats and reef composition. This complements further strategies that record one latitude and longitude of a site where surveys have been conducted. Additional advances in hyperspectral data to better distinguish between algal growth and living coral tissue will allow for adjustments of spectral data and help reduce errors of false positives or allow for the identification of potential bleaching from live coral. Using robotics and AI-driven image analysis will be instrumental in strengthening satellite-based monitoring products (Hoegh-Guldberg et al., 2023). Such innovations not only enhance monitoring reliability but also address the urgent need for more robust early warning methodologies for coral reefs. Globally consistent data sets with standardized bleaching information are critical to validating these monitoring tools and supporting data-informed policy decisions that guide global conservation strategies and climate adaptation actions for coral reef protection.
To improve comparability and facilitate global-scale analyses of coral health and coral bleaching observations, it is essential to improve standardized databases and support collaborative frameworks led by ICRI, GCRMN, and NOAA. Monitoring platforms must work with GCRMN to establish bottom-up networks and adhere to guidelines such as the FAIR principles (Findable, Accessible, Interoperable, and Reusable) and GCRMN’s workflows (e.g., GitHub gcrmndb_benthos). If more programs included standardized reporting parameters (such as coordinates, method types, and measured areas) while submitting data, it would facilitate comparison across efforts of data standardization that can be incorporated into existing global databases (Wicquart et al., 2022). Although efforts are already underway, the success of this standardization approach depends on effective collaboration and communication among local efforts, monitoring programs, online platforms, scientists who conduct database consolidations, regional institutions, and global organizations to ensure long-term sustainability, as well as on securing long-term funding. Lessons from past initiatives such as Reef Base highlight the need for continued support to prevent funding shortages. Reef Base, once the official database of the GCRMN and the International Coral Reef Action Network (ICRAN) in the 1990s, was a comprehensive information repository hosted by the WorldFish Center, but it is no longer available (https://catalogue.odis.org/view/612).
There is precedent for this process, as standardization has already been implemented for coral bleaching experiments, both in laboratory and specimen collection from the field. Recent reviews have stressed the importance of comparability and transparency and offered detailed recommendations on data collection, reporting parameters, and sample preservation to optimize cross-study comparisons (Grottoli et al., 2021; McLachlan et al., 2020; Thurber et al., 2022). These reviews emphasize that similarly detailed reporting of field sampling is crucial, with parameters such as the date of sampling, the area measured, depth, sampling methods, habitat, and species/genus level identification (if available) should be presented in the survey metadata (Thurber et al., 2022). Our study revealed the critical importance of databases specifying coordinate systems (e.g., geographic latitude and longitude) and datum (e.g., World Geodetic datum) and differentiating between source data and entries consolidated from other databases versus those directly submitted.
Coral monitoring programs connected to online platforms and standardized datasets play pivotal roles in supporting GCRMN, ICRI, and NOAA, yet these networks have their own standard operating procedures, protocols, funding mechanisms, and training requirements. Therefore, prioritizing the coordination and sharing of lessons learned across international boundaries (ideally led by ICRI) is essential. The main bleaching monitoring programs and online platforms (Table 4) could collaboratively develop application programming interfaces (API) and integrated information systems to facilitate data sharing. One major challenge for organizations such as the GCRMN is the bureaucracy and time allocation associated with data-sharing agreements; thus, creating more seamless data collection and input systems could help mitigate this issue if data agreements are established long-term and under a unified platform. Also, investing in these APIs and training people to use advanced technologies effectively will help ensure accurate and efficient data collection and analysis. It is also important to integrate long-term legacy datasets into on-line platforms and enable data extraction in standardized formats, although this can also be time- and resource-intensive.
Ideally, technology could facilitate data sharing and collaboration between researchers, practitioners in local and regional networks, and global coral reef platforms, leading to more effective and coordinated conservation efforts. To maximize data interoperability, standardized data formatting is essential for ensuring datasets are comparable across space and time. The adoption of open and reproducible practices is becoming more prevalent in modern scientific approaches. If the same response variables are measured using consistent methods, data analysis can be significantly streamlined. Best practices include structuring datasets in a long format, using open-access file formats such as.csv and.rtf, maintaining a well-organized data spreadsheet paired with a corresponding metadata file, and posting data files on open-access repositories. Standardized reporting templates, unique ID numbers for datasets, and comprehensive metadata guidelines could further enable effective collaboration while addressing challenges with database duplication. Regional networks, including CORDIO and AGRRA, are already in place and could be linked to open-access global coral reef information systems. The following best practices for open and accessible data not only support reproducible analyses but also streamlines scientific workflows, enabling more efficient research by accelerating the analytical process (Lowndes et al., 2017).
A promising tool advancing these efforts is the Marine Ecological Research Management Aid (MERMAID), an open-source platform developed through a collaboration between WCS, the World Wildlife Fund, and Sparkgeo. MERMAID facilitates data standardization for coral reef data collection and analysis, supporting a wide array of underwater surveys, including benthic surveys (line intercept transect, point intercept transect, photo quadrat), fish surveys (belt transect), bleaching surveys (quadrat), and habitat complexity assessments (tape-and-chain). The platform continues to evolve based on user needs, offering a user-friendly dashboard, automated data management processes, and basic analytical outputs in Excel and CSV formats. Additionally, the open source mermaidr R package in RStudio enhances advanced data accessibility and analysis, allowing users to compile datasets from multiple projects and integrate legacy data. MERMAID aligns with international policy initiatives, further strengthening its role in global coral reef conservation (https://datamermaid.org).
One of the primary limitations encountered in this study was the possible duplication of observations arising from the databases utilized (van Woesik and Kratochwill, 2022; Virgen-Urcelay and Donner, 2023). To mitigate this issue, we meticulously cross-referenced data based on date, location, depth, and method to identify and eliminate duplicates. However, the integration of diverse data sources continues to pose significant challenges. Therefore, the establishment of standardized comparative criteria, the assurance of consistent training, and the fostering of collaborative monitoring networks are essential steps to enhance data reliability and coherence across various monitoring initiatives (Souter et al., 2021; Wicquart et al., 2022). The results of this study are heavily based on information from monitoring organizations that adhere to standardized methods and provide data to scientists to collate bleaching surveys, such as Reef Check and Coral Watch, which are significant data sources. We acknowledge the exclusion of other important long-term regional databases, such as AIMS’s Long Term Monitoring Program for the Great Barrier Reef, among others; however, incorporating additional databases was beyond the scope of our study.
Observation data was analyzed over a continuous time frame and not just during known periods of heat stress. However, while the primary sources are labeled as bleaching databases, many surveys derived from monitoring efforts were not strictly bleaching assessments. The databases were designed for in-water field surveys, with a single entry per site identified by the central coordinates of the survey, rendering them incompatible with airborne or other remote-sensing surveys that cover broader areas. The databases primarily draw from existing datasets maintained by large monitoring organizations that adhere to consistent protocols (e.g., van Woesik and Kratochwill, 2022, which utilized seven sources), which may account for discrepancies in the breakdown of survey methods between the databases and the practitioner survey.
Another limitation and constraint is the need to standardize the timing of bleaching surveys and contextualize surveys within a defined bleaching timeline to enhance data comparability (Khen et al., 2023; Grottoli et al., 2014). Coral bleaching is a physiological response that results from specific exposure to heat and light over varying timeframes. Experimental research has shown that bleaching can appear immediately after acute heat stress or can take one to four months of accumulated heat stress to manifest (Glynn and D’Croz, 1990; Grottoli et al., 2006; Grottoli et al., 2014; Schoepf et al., 2015). Similarly, coral recovery can occur within periods ranging from 20 days to two months (Rodríguez-Román et al., 2006; Grottoli et al., 2014; Schoepf et al., 2015).
Two approaches can enhance the effectiveness of coral bleaching monitoring. The first approach focuses on characterizing the maximum severity of a bleaching event by conducting surveys during the peak heat stress period in each region (Eakin et al., 2010; Hughes et al., 2018b; Muñiz-Castillo et al., 2019). This window of maximum severity of bleaching could vary across regions (DeCarlo, 2020). These intra-annual patterns may differ across subregions and should be considered when designing monitoring efforts. For instance, in the Caribbean, most subregions experience heat stress between August and December, with the highest levels of heat stress typically observed in October or November (Muñiz-Castillo et al., 2019). Based on this example, the monitoring season for Caribbean coral reefs could primarily occur from September to October. However, it is important to note that coral bleaching may sometimes be observed before or after this period.
The second approach involves categorizing data collection into standard bleaching phases: a) Pre-Bleaching (Baseline), occurring before significant thermal stress based on historical temperature thresholds; b) Early Bleaching, when the first signs of bleaching are reported or detected; c) Peak Bleaching, marking the maximum bleaching extent in the region; and d) Late Bleaching/Recovery, when bleaching subsides, and corals either recover or experience mortality. It is most likely that the effort of bleaching will depend on many variables such as funding, monitoring capacity, and other factors mentioned in the practitioner survey. Many programs are designed for rapid assessments during specific bleaching events, rather than comprehensive, long-term monitoring, complicating efforts to achieve globally comparable datasets. We recommend prioritizing longitudinal measurements by tracking coral bleaching over time. This approach provides critical insights into the progression and recovery of bleaching events, thereby offering a more comprehensive understanding of coral responses to thermal stress (Grottoli et al., 2014; Neely et al., 2024).
There is an urgent need for improved coordination and consolidation of coral bleaching monitoring data. The diversity of methods and inconsistent data entry present significant challenges to accurately understanding how coral reefs change over time, especially given the increasing frequency of coral bleaching events. Our work has identified the most widely used techniques, as well as issues such as missing methods in metadata, highlighting the need for standardization across methods. To address this, collaborations must be strengthened across regional monitoring organizations, and global platforms (e.g., MERMAID) could collaborate with GCRMN to establish bottom-up networks and adhere to guidelines (e.g., FAIR principles). In addition, major monitoring programs and online platforms should develop collaborative APIs and integrated information systems to streamline data sharing based on data agreements. These elements will enhance collaboration, communication, and coordination across the field and result in more integrated global databases.
Although traditional methods for assessing coral bleaching in belt, line, and point transects are widely used due to their established protocols, training programs, and institutionalization, the importance of integrating recent technology into coral bleaching monitoring cannot be overstated. Despite their widespread use, these legacy methods offer a limited scope of reef health assessment and require considerable time and effort to monitor even small areas. In contrast, advanced technologies such as photogrammetry at permanent sites (= 10m2) and geo-referenced photo quadrats provide a more accurate and comprehensive understanding of reef dynamics at larger scales (Obura et al., 2019; McCarthy et al., 2024). These technologies enable the extraction of data that are comparable to legacy data while simultaneously advancing monitoring capabilities. However, these approaches come with specific needs in technological equipment (software, servers, global positioning devices, and computing power), funding (investment in resources, training of personnel, and maintenance of equipment), and data management and analysis that can pose challenges, especially for under-resourced geographies (McCarthy et al, 2024). To overcome these barriers, initiatives may focus on low-cost, open-source alternatives for software and equipment, as well as collaborations with international organizations that can also provide access to training and resources. Incorporating modern methods can significantly enhance our ability to track and analyze reef health more effectively and link this to global policy communication and efforts.
Recent technology offers a promising path forward by addressing the limitations of traditional coral bleaching monitoring methods and fostering the creation of more coordinated and consistent datasets. By linking these data to high-resolution advanced remote sensing platforms, many of which are freely accessible, practitioners, managers, and decision-makers around the world could access and utilize critical information. Our recommendations would improve global capacity to monitor and manage coral reef ecosystems and consolidate data, enabling the coral reef community to set and achieve more effective conservation targets to protect the remaining coral reefs worldwide.
AR-S: Conceptualization, Data curation, Methodology, Writing – original draft, Writing – review & editing, Formal Analysis, Investigation, Visualization. AM-C: Conceptualization, Data curation, Formal Analysis, Methodology, Visualization, Writing – original draft, Writing – review & editing. BC: Data curation, Investigation, Methodology, Writing – original draft, Writing – review & editing. GA: Conceptualization, Methodology, Supervision, Writing – review & editing. CR: Investigation, Writing – review & editing. SD: Investigation, Methodology, Writing – review & editing. BB: Writing – review & editing. AB: Writing – review & editing. MP: Writing – review & editing. DM: Writing – review & editing. PM: Writing – review & editing. HF: Conceptualization, Funding acquisition, Investigation, Project administration, Supervision, Writing – original draft, Writing – review & editing.
The author(s) declare that financial support was received for the research and/or publication of this article. We acknowledge funding from the Paul M. Angell Family Foundation for their generous support, which enabled the Coral Reef Alliance, Arizona State University, and the Wildlife Conservation Society to advance the project, ¨Expanding the Impact of the Allen Coral Atlas through Global Reef Monitoring¨.
We gratefully acknowledge support from the Allen Coral Atlas, which was made possible primarily through funding from Vulcan, Inc. We also thank Robert van Woesik, Mark Eakin, Diana Kleine, Sophie Kalkowski-Pope, Emily Darling, Antonella Rivera, Annick Cros, Jeremy Wicquart, and Rebecca Green and the reviewers for their insightful feedback, which strengthened the manuscript.
Author BB was employed by company Resource & Ecosystem Management. Author MP was employed by the company Global Sciences and Technology, Inc. Greenbelt, MD, USA.
The remaining authors declare that the research was conducted in the absence of any commercial or financial relationships that could be construed as a potential conflict of interest.
The author(s) declare that no Generative AI was used in the creation of this manuscript.
All claims expressed in this article are solely those of the authors and do not necessarily represent those of their affiliated organizations, or those of the publisher, the editors and the reviewers. Any product that may be evaluated in this article, or claim that may be made by its manufacturer, is not guaranteed or endorsed by the publisher.
The Supplementary Material for this article can be found online at: https://www.frontiersin.org/articles/10.3389/fmars.2025.1547870/full#supplementary-material
Andréfouët S., Berkelmans R., Odriozola L., Done T., Oliver J., Müller-Karger F. (2002). Choosing the appropriate spatial resolution for monitoring coral bleaching events using remote sensing. Coral Reefs 21, 147–154. doi: 10.1007/s00338-002-0233-x
Asner G. P., Vaughn N. R., Foo S. A., Heckler J., Martin R. E. (2021a). Abiotic and human drivers of reef habitat complexity throughout the main hawaiian islands. Front. Mar. Sci. 8. doi: 10.3389/fmars.2021.631842
Asner G. P., Vaughn N. R., Heckler J., Knapp D. E., Balzotti C., Shafron E., et al. (2021b). Large-scale mapping of live corals to guide reef conservation. Proc. Natl. Acad. Sci. United States America 117, 33711–33718. doi: 10.1073/PNAS.2017628117
Berkelmans R., De’ath G., Kininmonth S., Skirving W. J. (2004). A comparison of the 1998 and 2002 coral bleaching events on the Great Barrier Reef: Spatial correlation, patterns, and predictions. Coral Reefs 23, 74–83. doi: 10.1007/s00338-003-0353-y
Bonelli A. G., Martin P., Noel P., Asner G. P. (2024). Global chlorophyll concentration distribution and effects on bottom reflectance of coral reefs. Oceans 5, 210–226. doi: 10.3390/oceans5020013
Bongaerts P., Dubé C. E., Prata K. E., Gijsbers J. C., Achlatis M., Hernandez-Agreda A. (2021). Reefscape genomics: leveraging advances in 3D imaging to assess fine-scale patterns of genomic variation on coral reefs. Front. Mar. Sci. 8). doi: 10.3389/fmars.2021.638979
Bruno J., Siddon C., Witman J., Colin P., Toscano M. (2001). El Niño related coral bleaching in Palau, Western Caroline Islands. Coral Reefs 20, 127–136. doi: 10.1007/s003380100151
Cardenas J. A., Samadikhoshkho Z., Rehman A. U., Valle-Pérez A. U., de León E. H. P., Hauser C. A. E., et al. (2024). “A systematic review of robotic efficacy in coral reef monitoring techniques,” in Marine pollution bulletin (Vol. 202) (Netherlands: Elsevier Ltd). doi: 10.1016/j.marpolbul.2024.116273
Carlson R. R., Li J., Crowder L. B., Asner G. P. (2022). Large-scale effects of turbidity on coral bleaching in the Hawaiian islands. Front. Mar. Sci. 9. doi: 10.3389/fmars.2022.969472
Carroll A. G., Harrison P. L., Adjeroud M. (2017). Susceptibility of coral assemblages to successive bleaching events at Moorea, French Polynesia. Mar. Freshw. Res. 68, 760–771. doi: 10.1071/MF15134
Claar D. C., Szostek L., McDevitt-Irwin J. M., Schanze J. J., Baum J. K. (2018). Global patterns and impacts of El Niño events on coral reefs: A meta-analysis. PloS One 13, 1–22. doi: 10.1371/journal.pone.0190957
Convention on Biological Diversity (2024)Target 8, minimize the impacts of climate change on biodiversity and build resilience. Available at: https://www.cbd.int/gbf/targets/8 (Accessed November 11, 2024).
Coral Watch (2022). Available at: https://biocollect.ala.org.au/coralwatch (Accessed June 2, 2022).
Couch C. S., Oliver T. A., Suka R., Lamirand M., Asbury M., Amir C., et al. (2021). Comparing coral colony surveys from in-water observations and structure-from-motion imagery shows low methodological bias. Front. Mar. Sci. 8. doi: 10.3389/fmars.2021.647943
DeCarlo T. M. (2020). Treating coral bleaching as weather: A framework to validate and optimize prediction skill. PeerJ 2020, 1–16. doi: 10.7717/peerj.9449
Delaval N. P., Wicquart J., Staub F., Planes S. (2021). Status of coral reef monitoring: an assessment of methods and data at the national level. Global Coral Reef Monitoring Network (GCRMN)/International Coral Reefs Initiative (ICRI)
Donner S. D., Rickbeil G. J. M., Heron S. F. (2017). A new, high-resolution global mass coral bleaching database. PloS One 12, 1–17. doi: 10.1371/journal.pone.0175490
Donovan M. K., Alves C., Burns J., Drury C., Meier O. W., Ritson-Williams R., et al. (2023). From polyps to pixels: understanding coral reef resilience to local and global change across scales. Landscape Ecol. 38, 737–752. doi: 10.1007/s10980-022-01463-3
Donovan M. K., Burkepile D. E., Kratochwill C., Shlesinger T., Sully S., Oliver T. A., et al. (2021). Local conditions magnify coral loss after marine heatwaves. Science 372, 977–980. doi: 10.1126/science.abd9464
Drury C., Martin R. E., Knapp D. E., Heckler J., Levy J., Gates R. D., et al. (2022). Ecosystem-scale mapping of coral species and thermal tolerance. Front. Ecol. Environ. 20, 285–291. doi: 10.1002/fee.2483
Eakin C. M., Morgan J. A., Heron S. F., Smith T. B., Liu G., Alvarez-Filip L., et al. (2010). Caribbean corals in crisis: Record thermal stress, bleaching, and mortality in 2005. PloS One 5, 1–9. doi: 10.1371/journal.pone.0013969
Eakin C. M., Sweatman H. P. A., Brainard R. E. (2019). The 2014–2017 global-scale coral bleaching event: insights and impacts. Coral Reefs 38, 539–545. doi: 10.1007/s00338-019-01844-2
Eddy T. D., Lam V. W. Y., Reygondeau G., Cisneros-Montemayor A. M., Greer K., Palomares M. L. D., et al. (2021). Global decline in capacity of coral reefs to provide ecosystem services. One Earth 4, 1278–1285. doi: 10.1016/j.oneear.2021.08.016
Ginsburg R. N., Lang J. C. (2003). Status of Coral Reefs in the Western Atlantic. Results of initial surveys, Atlantic and Gulf Rapid Reef Assessment (AGRRA) Program. Atoll Res. Bull. 496, 1–625.
Glynn P. W., D’Croz L. (1990). Experimental evidence for high temperature stress as the cause of El Niño-coincident coral mortality. Coral Reefs 8, 181–191. doi: 10.1007/BF00265009
Glynn P. W., Maté J. L., Baker A. C., Calderón M. O. (2001). Coral bleaching and mortality in panama and ecuador during the 1997-1998 el niño-southern oscillation event: Spatial/Temporal patterns and comparisons with the 1982-1983 event. Bull. Mar. Sci. 69, 79–109.
González-Rivero M., Beijbom O., Rodriguez-Ramirez A., Bryant D. E. P., Ganase A., Gonzalez-Marrero Y., et al. (2020). Monitoring of coral reefs using artificial intelligence: A feasible and cost-effective approach. Remote Sens. 12, 489. doi: 10.3390/rs12030489
Grottoli A. G., Rodrigues L. J., Palardy J. E. (2006). Heterotrophic plasticity and resilience in bleached corals. Nature 440, 1186–1189. doi: 10.1038/nature04565
Grottoli A. G., Toonen R. J., van Woesik R., Vega Thurber R., Warner M. E., McLachlan R. H., et al. (2021). ). Increasing comparability among coral bleaching experiments. Ecol. Appl. 31, 1–17. doi: 10.1002/eap.2262
Grottoli A. G., Warner M. E., Levas S. J., Aschaffenburg M. D., Schoepf V., Mcginley M., et al. (2014). The cumulative impact of annual coral bleaching can turn some coral species winners into losers. Global Change Biol. 20, 3823–3833. doi: 10.1111/gcb.12658
Gutierrez L., Polidoro B., Obura D., Cabada-Blanco F., Linardich C., Pettersson E., et al. (2024). Half of Atlantic reef-building corals at elevated risk of extinction due to climate change and other threats. PloS One 19, e0309354. doi: 10.1371/journal.pone.0309354
Hedley J. D., Roelfsema C. M., Chollett I., Harborne A. R., Heron S. F., Weeks S. J., et al. (2016). Remote sensing of coral reefs for monitoring and management: A review. Remote Sens. 8, 1–40. doi: 10.3390/rs8020118
Heron S. F., Maynard J. A., Van Hooidonk R., Eakin C. M. (2016). Warming trends and bleaching stress of the world’s coral reefs 1985-2012. Sci. Rep. 6, 1–16. doi: 10.1038/srep38402
Hill J., Wilkinson C. (2004). “Methods for ecological monitoring of coral reefs,” in Australian institute of marine science, townsville (Australian Institute of Marine Science).
Hoegh-Guldberg O., Skirving W., Dove S. G., Spady B. L., Norrie A., Geiger E. F., et al. (2023). Coral reefs in peril in a record-breaking year. Science 382, 1238–1240. doi: 10.1126/science.adk4532
Hughes T. P., Anderson K. D., Connolly S. R., Heron S. F., Kerry J. T., Lough J. M., et al. (2018a). Spatial and temporal patterns of mass bleaching of corals in the Anthropocene. Science 359, 80–83. doi: 10.1126/science.aan8048
Hughes T. P., Barnes M. L., Bellwood D. R., Cinner J. E., Cumming G. S., Jackson J. B. C., et al. (2017). Coral reefs in the anthropocene. Nature 546, 82–90. doi: 10.1038/nature22901
Hughes T. P., Kerry J. T., Baird A. H., Connolly S. R., Dietzel A., Eakin C. M., et al. (2018b). Global warming transforms coral reef assemblages. Nature 556, 492–496. doi: 10.1038/s41586-018-0041-2
Hughes T. P., Kerry J. T., Simpson T. (2018c). Large-scale bleaching of corals on the Great Barrier Reef. Ecology 99, 501–501. doi: 10.1002/ecy.2092
IPCC (2023). “Summary for policymakers,” in Climate change 2022 – impacts, adaptation and vulnerability. Eds. D. C. R. E. S. P. K. M. M. T. A. A. M. C. S. L. S. L. V. M. A. O, Pörtner H.-O. (Cambridge University Press), 3–34. doi: 10.1017/9781009325844.001
Jokiel P. L., Rodgers K. S., Brown E. K., Kenyon J. C., Aeby G., Smith W. R., et al. (2015). Comparison of methods used to estimate coral cover in the Hawaiian Islands. PeerJ 2015, 1–26. doi: 10.7717/peerj.954
Kennedy E. V., Roelfsema C. M., Lyons M. B., Kovacs E. M., Borrego-Acevedo R., Roe M., et al. (2021). Reef Cover, a coral reef classification for global habitat mapping from remote sensing. Sci. Data 8, 1–20. doi: 10.1038/s41597-021-00958-z
Khen A., Wall C. B., Smith J. E. (2023). Standardization of in situ coral bleaching measurements highlights the variability in responses across genera, morphologies, and regions. . PeerJ 11, 1–26. doi: 10.7717/peerj.16100
Kopecky K. L., Pavoni G., Nocerino E., Brooks A. J., Corsini M., Menna F., et al. (2023). Quantifying the loss of coral from a bleaching event using underwater photogrammetry and AI-assisted image segmentation. Remote Sens. 15, 1–18. doi: 10.3390/rs15164077
Kumagai N. H., Yamano H. (2018). High-resolution modeling of thermal thresholds and environmental influences on coral bleaching for local and regional reef management. PeerJ 6, e4382. doi: 10.7717/peerj.4382
Lachs L., Bythell J. C., East H. K., Edwards A. J., Mumby P. J., Skirving W. J., et al. (2021). Fine-tuning heat stress algorithms to optimise global predictions of mass coral bleaching. Remote Sens. 13, 1–18. doi: 10.3390/rs13142677
Levy J., Hunter C., Lukacazyk T., Franklin E. C. (2018). Assessing the spatial distribution of coral bleaching using small unmanned aerial systems. Coral Reefs 37, 373–387. doi: 10.1007/s00338-018-1662-5
Li J., Fabina N. S., Knapp D. E., Asner G. (2020). The sensitivity of multi-spectral satellite sensors to benthic habitat change. Remote Sens. 12, 532. doi: 10.3390/rs12030532
Lowndes J. S. S., Best B. D., Scarborough C., Afflerbach J. C., Frazier M. R., O’Hara C. C., et al. (2017). Our path to better science in less time using open data science tools. Nat. Ecol. Evol. 1, 1–7. doi: 10.1038/s41559-017-0160
Lyons M. B., Roelfsema C. M., Kennedy E. V., Kovacs E. M., Borrego-Acevedo R., Markey K., et al. (2020). Mapping the world’s coral reefs using a global multiscale earth observation framework. Remote Sens. Ecol. Conserv. 6, 557–568. doi: 10.1002/rse2.157
Madin E. M. P., Darling E. S., Hardt M. J. (2019). Emerging technologies and coral reef conservation: opportunities, challenges, and moving forward. Front. Mar. Sci. 6. doi: 10.3389/fmars.2019.00727
Mallet D., Pelletier D. (2014). Underwater video techniques for observing coastal marine biodiversity: A review of sixty years of publication-2012). Fisheries Res. 154, 44–62. doi: 10.1016/j.fishres.2014.01.019
Marshall N. J., Kleine D. A., Dean A. J. (2012). CoralWatch: Education, monitoring, and sustainability through citizen science. Front. Ecol. Environ. 10, 332–334. doi: 10.1890/110266
Marshall P., Oliver J., Setiasih N., Marshall P., Hansen L. (2004). ). A global protocol for monitoring of coral bleaching. WorldFish Center Q. 27. Available at: www.reefbase.org/input/bleachingreport/.
Mason R. A. B., Skirving W. J., Dove S. G. (2020). Integrating physiology with remote sensing to advance the prediction of coral bleaching events. Remote Sens. Environ. 246, 1–11. doi: 10.1016/j.rse.2020.111794
McCarthy O. S., Winston Pomeroy M., Smith J. E. (2024). Corals that survive repeated thermal stress show signs of selection and acclimatization. PloS One 19, e0303779. doi: 10.1371/journal.pone.0303779
McClanahan T. R. (2004). The relationship between bleaching and mortality of common corals. Mar. Biol. 144, 1239–1245. doi: 10.1007/s00227-003-1271-9
McClanahan T. R., Darling E. S., Maina J. M., Muthiga N. A., Agata S. D., Jupiter S. D., et al. (2019). Temperature patterns and mechanisms influencing coral bleaching during the 2016 El Niño. Nat. Climate Change 9, 845–851. doi: 10.1038/s41558-019-0576-8
McClanahan T. R., Darling E. S., Maina J. M., Muthiga N. A., D’Agata S., Leblond J., et al. (2020). Highly variable taxa-specific coral bleaching responses to thermal stresses. Mar. Ecol. Prog. Ser. 648, 135–151. doi: 10.3354/meps13402
McClanahan T., Muthiga N., Mangi S. (2001). Coral and algal changes after the 1998 coral bleaching: interaction with reef management and herbivores on Kenyan reefs. Coral Reefs 19, 380–391. doi: 10.1007/s003380000133
McField M. D. (1999). Coral response during and after mass bleaching in Belize. Bull. Mar. Sci. 64, 155–172.
McLachlan R. H., Price J. T., Solomon S. L., Grottoli A. G. (2020). Thirty years of coral heat-stress experiments: a review of methods. Coral Reefs 39, 885–902. doi: 10.1007/s00338-020-01931-9
Muñiz-Castillo A. I., Rivera-Sosa A., McField M., Chollett I., Eakin C. M., Enríquez S., et al. (2024). Underlying drivers of coral reef vulnerability to bleaching in the mesoamerican reef. Commun. Biol. 7, 1452. doi: 10.1038/s42003-024-07128-y
Muñiz-Castillo A. I., Rivera-Sosa A., Chollett I., Eakin C. M., Andrade-Gómez L., McField M., et al. (2019). Three decades of heat stress exposure in Caribbean coral reefs: a new regional delineation to enhance conservation. Sci. Rep. 9, 11013. doi: 10.1038/s41598-019-47307-0
Neal B. P., Khen A., Treibitz T., Beijbom O., O’Connor G., Coffroth M. A., et al. (2017). Caribbean massive corals not recovering from repeated thermal stress events during 2005–2013. Ecol. Evol. 7, 1339–1353. doi: 10.1002/ece3.2706
Neely K. L., Nowicki R. J., Dobler M. A., Chaparro A. A., Miller S. M., Toth K. A. (2024). Too hot to handle? The impact of the 2023 marine heatwave on Florida Keys coral. Front. Mar. Sci. 11. doi: 10.3389/fmars.2024.1489273
Nguyen T., Liquet B., Mengersen K., Sous D. (2021). Mapping of coral reefs with multispectral satellites: A review of recent papers. Remote Sens (Basel) 13, 4470.1–25. doi: 10.3390/rs13214470
Obura D. O., Aeby G., Amornthammarong N., Appeltans W., Bax N., Bishop J., et al. (2019). Coral reef monitoring, reef assessment technologies, and ecosystem-based management. Front. Mar. Sci. 6. doi: 10.3389/fmars.2019.00580
Reimer J. D., Peixoto R. S., Davies S. W., Traylor-Knowles N., Short M. L., Cabral-Tena R. A., et al. (2024). “The Fourth Global Coral Bleaching Event: Where do we go from here?,” in Coral reefs (Germany: Springer Nature). doi: 10.1007/s00338-024-02504-w
Roelfsema C. (2010). Integrating field data with high spatial resolution multispectral satellite imagery for calibration and validation of coral reef benthic community maps. J. Appl. Remote Sens 4, 043527. doi: 10.1117/1.3430107
Rodríguez-Román A., Hernández-Pech X., Thomé P. E., Enríquez S., Iglesias-Prieto R. (2006). Photosynthesis and light utilization in the Caribbean coral Montastraea faveolata recovering from a bleaching event. Limnology Oceanography 51, 2702–2710. doi: 10.4319/lo.2006.51.6.2702
Roelfsema C. M., Lyons M., Murray N., Kovacs E. M., Kennedy E., Markey K., et al. (2021). Workflow for the generation of expert-derived training and validation data: A view to global scale habitat mapping. Front. Mar. Sci. 8. doi: 10.3389/fmars.2021.643381
Safaie A., Silbiger N. J., McClanahan T. R., Pawlak G., Barshis D. J., Hench J. L., et al. (2018). High frequency temperature variability reduces the risk of coral bleaching. Nat. Commun. 9, 1671. doi: 10.1038/s41467-018-04074-2
Schoepf V., Grottoli A., Baumann J. H., Grottoli G., Levas S. J., Schoepf V., et al. (2015). Annual coral bleaching and the long-term recovery capacity of coral Annual coral bleaching and the long-term recovery capacity of coral. Proceedings of the Royal Society B: Biological Sciences. 282, 20151887. doi: 10.1098/rspb.2015.1887
Siebeck U. E., Marshall N. J., Klüter A., Hoegh-Guldberg O. (2006). Monitoring coral bleaching using a colour reference card. Coral Reefs 25, 453–460. doi: 10.1007/s00338-006-0123-8
Skirving W. J., Heron S. F., Marsh B. L., Liu G., de la Cour J. L., Geiger E. F., et al. (2019). The relentless march of mass coral bleaching: a global perspective of changing heat stress. Coral Reefs 38, 547–557. doi: 10.1007/s00338-019-01799-4
Skirving W., Marsh B., de la Cour J., Liu G., Harris A., Maturi E., et al. (2020). Coraltemp and the coral reef watch coral bleaching heat stress product suite version 3.1. Remote Sens. 12, 1–10. doi: 10.3390/rs12233856
Souter D., Planes S., Wicquart J., Logan M., Obura D., Staub F. (2021). Status of coral reefs of the world: 2020 report (Global Coral Reef Monitoring Network (GCRMN) and International Coral Reef Initiative (ICRI). doi: 10.59387/WOTJ9184
Spady B. L., Skirving W. J., Liu G., de la Cour J. L., McDonald C. J., Manzello D. P. (2022). Unprecedented early-summer heat stress and forecast of coral bleaching on the Great Barrier Reef 2021-2022. F1000Research 11, 127. doi: 10.12688/f1000research.108724.1
Sully S., Burkepile D. E., Donovan M. K., Hodgson G., van Woesik R. (2019). A global analysis of coral bleaching over the past two decades. Nat. Commun. 10, 1–5. doi: 10.1038/s41467-019-09238-2
Sully S., Hodgson G., van Woesik R. (2022). Present and future bright and dark spots for coral reefs through climate change. Global Change Biol. 28, 4509–4522. doi: 10.1111/gcb.16083
Teague J., Allen M. J., Day J. C. C., Scott T. B. (2020). A review of current coral monitoring tools/diagnostic methods & Introducing a new tool to the coral health toolkit. 1–20. doi: 10.20944/preprints202008.0045.v1
The Great Reef Census (2024). Available at: https://greatreefcensus.org/ (Accessed 08-10-2024).
Thurber R. V., Schmeltzer E. R., Grottoli A. G., van Woesik R., Toonen R. J., Warner M., et al. (2022). Unified methods in collecting, preserving, and archiving coral bleaching and restoration specimens to increase sample utility and interdisciplinary collaboration. PeerJ. 10, 1–32. https://doi: 10.7717/peerj.14176
Urbina-Barreto I., Garnier R., Elise S., Pinel R., Dumas P., Mahamadaly V., et al. (2021). Which method for which purpose? A comparison of line intercept transect and underwater photogrammetry methods for coral reef surveys. Front. Mar. Sci. 8. doi: 10.3389/fmars.2021.636902
van Woesik R., Kratochwill C. (2022). A global coral-bleaching database 1980–2020. Sci. Data 9, 1–7. doi: 10.1038/s41597-022-01121-y
van Woesik R., Shlesinger T., Grottoli A. G., Toonen R. J., Vega Thurber R., Warner M. E., et al. (2022). Coral-bleaching responses to climate change across biological scales. Global Change Biol. 28, 4229–4250. doi: 10.1111/gcb.16192
Virgen-Urcelay A., Donner S. D. (2023). Increase in the extent of mass coral bleaching over the past half-century, based on an updated global database. PloS One 18, 1–17. doi: 10.1371/journal.pone.0281719
Wicquart J., Gudka M., Obura D., Logan M., Staub F., Souter D., et al. (2022). A workflow to integrate ecological monitoring data from different sources. Ecol. Inf. 68, 1–10. doi: 10.1016/j.ecoinf.2021.101543
Winston M., Oliver T., Couch C., Donovan M. K., Asner G. P., Conklin E., et al. (2022). Coral taxonomy and local stressors drive bleaching prevalence across the hawaiian archipelago in 2019. PloS One 17, e0269068. doi: 10.1371/journal.pone.0269068
Xu J., Zhao J., Wang F., Chen Y., Lee Z. (2021). Detection of coral reef bleaching based on sentinel-2 multi-temporal imagery: simulation and case study. Front. Mar. Sci. 8, 1–15. doi: 10.3389/fmars.2021.584263
Xu Y., Vaughn N. R., Knapp D. E., Martin R. E., Balzotti C., Li J., et al. (2020). Coral bleaching detection in the hawaiian islands using spatio-temporal standardized bottom reflectance and planet dove satellites. Remote Sens. 12, 1–16. doi: 10.3390/rs12193219
Keywords: coral reefs, coral bleaching surveys, global bleaching review, marine heatwaves, underwater surveys
Citation: Rivera-Sosa A, Muñiz-Castillo AI, Charo B, Asner GP, Roelfsema CM, Donner SD, Bambic BD, Bonelli AG, Pomeroy M, Manzello D, Martin P and Fox HE (2025) Six decades of global coral bleaching monitoring: a review of methods and call for enhanced standardization and coordination. Front. Mar. Sci. 12:1547870. doi: 10.3389/fmars.2025.1547870
Received: 18 December 2024; Accepted: 26 February 2025;
Published: 26 March 2025.
Edited by:
Cliff Ross, University of North Florida, United StatesReviewed by:
Hajime Kayanne, The University of Tokyo, JapanCopyright © 2025 Rivera-Sosa, Muñiz-Castillo, Charo, Asner, Roelfsema, Donner, Bambic, Bonelli, Pomeroy, Manzello, Martin and Fox. This is an open-access article distributed under the terms of the Creative Commons Attribution License (CC BY). The use, distribution or reproduction in other forums is permitted, provided the original author(s) and the copyright owner(s) are credited and that the original publication in this journal is cited, in accordance with accepted academic practice. No use, distribution or reproduction is permitted which does not comply with these terms.
*Correspondence: Andrea Rivera-Sosa, YXJpdmVyYS1zb3NhQGNvcmFsLm9yZw==
Disclaimer: All claims expressed in this article are solely those of the authors and do not necessarily represent those of their affiliated organizations, or those of the publisher, the editors and the reviewers. Any product that may be evaluated in this article or claim that may be made by its manufacturer is not guaranteed or endorsed by the publisher.
Research integrity at Frontiers
Learn more about the work of our research integrity team to safeguard the quality of each article we publish.