- 1Sericultural and Agri-Food Research Institute, Guangdong Academy of Agricultural Sciences/Key Laboratory of Functional Foods, Ministry of Agriculture and Rural Affairs/Guangdong Key Laboratory of Agricultural Products Processing, Guangzhou, China
- 2College of Marine Sciences, South China Agricultural University, Guangzhou, China
- 3College of Food Science and Technology, Zhongkai University of Agriculture and Engineering, Guangzhou, China
Chronic heat stress (HS) induces oxidative damage, low immunity, and intestinal flora disturbance of fish, posing great challenges to the aquaculture industry. As an important plant extract, mulberry leaf extract (MLE) has been shown to have antioxidant and immune-boosting properties. This study evaluated the protective effect of dietary MLE on HS-induced liver injury and intestinal flora disturbance in Largemouth bass (Micropterus salmoides) based on oxidative damage parameters, immune parameters, and intestinal flora composition. Fish were randomly assigned into three groups: CON group (ambient temperature, 26°C, basal diet), HS group (33°C, basal diet), and HS + MLE group (33°C, basal diet supplemented with 9 g/kg MLE). HS significantly decreased the final body weight, specific growth rate, intestinal villus length, muscular layer thickness, ACE and Chao1 indices, and lipase, trypsin, total superoxide dismutase (T-SOD), glutathione peroxidase (GPx), alkaline phosphatase (AKP), and lysozyme (LZM) activities, but significantly increased the feed conversion rate, aspartate aminotransferase (AST) activity, reactive oxygen species (ROS), malondialdehyde (MDA), complement 3 (C3) contents, and the relative abundances of Proteobacteria and Plesiomonas. Compared with the HS group, dietary MLE significantly improved lipase, trypsin, GPx, ACP, and LZM activities, and ACE and Chao1 indices in intestine, but significantly decreased the numbers of vacuoles and inflammatory cells, AST activity, and the GLU, MDA, and ROS contents. Dietary MLE also significantly up-regulated the mRNA expressions of gpx, tgf-β, il-10, jnk2, and bcl-2, but down-regulated the mRNA expressions of il-8, tnf-α, caspase-3, atf4, chop, ire1, traf2, jnk1, TRPV, MCU, and VDAC in liver. In conclusion, dietary supplementation with 9 g/kg MLE could improve the growth, immunity, and diversity of intestinal flora in heat-stressed largemouth bass, and alleviate hepatic injuries by regulating inflammation, oxidative stress, and apoptosis, providing a theoretical basis for the development of MLE as a treatment against HS.
1 Introduction
Water temperature is an important environmental factor that affects the survival and growth of aquacultural organisms. Any subtle changes in water temperature can affect the survival, physiological functions, and immune defenses of fish (Kim et al., 2017; Islam et al., 2021; Mugwanya et al., 2022). Although increasing the water temperature within a certain range can improve fish growth by increasing metabolic enzyme activity, excessively high temperatures may lead to intestinal barrier disorders, increased infection probability, and even death, thereby resulting in oxidative stress (Chen et al., 2022; Li X. et al., 2023; Guo et al., 2023; Du et al., 2024). Stress-induced reactive oxygen species (ROS) have a key role in innate immune defense (Sun J. L. et al., 2020; Andrés et al., 2022). Antioxidant enzymes and endogenous antioxidants form the first line of defense for cells against oxidative stress and maintain the cellular redox status. However, excessive stress-induced levels of ROS can damage crucial biomolecules, including DNA, proteins, and lipids, resulting in decreased physiological functions and reduced growth (Ito et al., 2019; Andrés et al., 2021). New evidences suggested that heat stress (HS) also can cause endoplasmic reticulum stress and mitochondrial dysfunction, and induce hepatocyte apoptosis of fish (Zhao et al., 2022; Lin et al., 2024). Thus, a key objective of aquaculture research is to mitigate the adverse effects of excessive stress and to minimize economic losses for fishermen operating in high temperature breeding environments.
In aquaculture, breeding environment management, epigenetic regulation, and feed supplementation have been identified as effective strategies to mitigate the negative effects of HS (Islam et al., 2021; Liu Z. et al., 2022). Of these approaches, dietary management is the simplest, quickest and cheapest to manage. Mulberry leaf extract (MLE) is made from mulberry leaves through ultrasonic extraction, filtration, concentration, and freeze-drying. It is rich in bioactive substances, such as flavonoids, polysaccharides, and polyphenols, and has the advantages of high efficiency, naturalness, and low side effects. It is being increasingly used as a feed supplement for aquatic animals (Tingsen et al., 2022; Chen et al., 2023). Neamat-Allah et al. (2021) reported that diet supplemented with MLE significantly improved the antioxidant levels and enhanced the immune feedback of tilapia (Oreochromis niloticus), as also reported by Tang et al. (2022). Dietary MLE was also revealed to improve the growth performance, feed utilization, digestive capacity, and hepatic antioxidant status of the Chinese giant salamander (Andrias davidianus) (Li Z. et al., 2020). In addition, diet supplemented with MLE can reduce oxidative damage caused by hypoxia-reoxygenation stress by inhibiting ROS production and oxidation of cellular components (Li H. et al., 2020). Furthermore, MLE promoted liver lipid and carbohydrate metabolism in spotted sea bass (Lateolabrax maculatus), and enhanced digestive enzyme activity and improved intestinal flora composition (Zhou et al., 2023a, 2023b). Therefore, we hypothesize that MLE can also be used as an environmentally friendly and healthy antioxidant and immune enhancer for fish under HS.
Largemouth bass (Micropterus salmoides) is an economically important aquaculture species in East Asia, with an annual production of more than 800,000 tons in China (Gong et al., 2024). With the expansion of breeding scale, high-density pond farming has become the main breeding mode for M. salmoides. However, because of the increase in stocking density and lack of management, this farming model will inevitably experience the interaction of high temperature and hypoxic stress during the summer, leading to reduced yield and immunity, and high mortality (Sun L. et al., 2020; He Y. et al., 2022). However, research on the relationship between HS and dietary immune enhancer supplementation in M. salmoides is still in its early stage, and many traits related to HS and disease resistance remain unclear. Therefore, this study explored the effects of MLE on the growth performance, immune and antioxidant capacity, and intestinal flora of M. salmoides under chronic HS, focusing on environmentally friendly and efficient aquaculture in hot weather.
2 Materials and methods
2.1 Fish culture
Healthy M. salmoides were procured from a M. salmoides hatchery in Zhuhai, China, and then acclimated in a concrete tank (2 m × 1.5 m ×1.5 m) in an outdoor circulating aquaculture system of the Sericultural & Agri-Food Research Institute, Guangdong Academy of Agricultural Sciences (Guangzhou, China) for 1 week before the experiment. The fish were fed a basal diet (Foshan Jieda Feed Co., Ltd., Guangdong, China) twice daily (Table 1). All ingredients were blended and ultra-micronized, sieved (250-μm mesh), modulated by 102°C water vapor for 8 min, and then extruded and pelleted at 95°C, dried at 55°C, and stored at 4°C until use.
2.2 Heat stress treatments
A total of 108 M. salmoides were randomly divided into a control group (CON, fed a basal diet), a HS group (HS, fed a basal diet) and a HS supplemented with 9 g/kg MLE group (HS+MLE) with three tanks per group and 12 fish per tank (0.3 m × 0.3 m × 0.35 m). MLE (9 g) was fully dissolved in 50 g sterile water, evenly distributed into 1 kg basal diet using a sprayer, dried at 50°C for 1 h. The MLE (mainly containing 4.2% mulberry leaf flavonoids) was purchased from Xian Ruilin Biotechnology Co., Ltd. (Xian, China) and the supplemental level of MLE was based on previous studies (Tingsen et al., 2022; Zhou et al., 2023a). The water temperature was gradually increased in the HS and HS+MLE groups to 33°C from 26°C over 7 days (1°C per day) to prevent the thermal shock of the fish before the start of the experiment. Subsequently, the water temperature was controlled using thermostatically monitored electric heaters (J10, Laoyujiang Industrial Co., Ltd., Shenzhen, China) for the 3 weeks of the study. The fish were fed the diets above twice daily (08:30 h and 17:00 h) to achieve an apparent state of satiety for 3 weeks. Fish waste was siphoned twice daily, and a half of the aquarium water was replaced with dechlorinated water that had been previously adjusted to the temperature of the aquarium water (26°C or 33°C). The animal husbandry and handling protocols used in this study have been approved by the Animal Care and Use Committee, Sericultural & Agri-Food Research Institute, Guangdong Academy of Agricultural Sciences.
2.3 Sampling
At the end of the feeding trial, the fish were fasted for 24 h and then anesthetized with 40 mg/L tricaine methane sulphonate (MS-222, Sigma, Saint Louis, USA). Fish in each tank were counted and weighed to determine the final body weight, weight gain rate, specific growth rate, condition factor, feed conversion ratio and feed intake. The blood from six fish per tank was drawn from the caudal vein, kept at 4°C, centrifuged at 3,500 rpm for 10 min, and the supernatant was stored at −80°C for the analysis of the serum antioxidant, immunity and biochemistry indices. Afterward, the fish were killed and dissected using a sterile scalpel by cutting the belly on ice for the determination of the viscerosomatic index and hepatosomatic index.
The total intestines of six fish per tank were collected and stored at −80°C for the analysis of intestinal microbiota, antioxidant and immunity parameters. Some mid-intestine and liver samples were fixed in 4% formalin for hematoxy&lin-eosin (H&E) staining. The livers of six fish per tank were collected and stored at −80°C for genes expression, antioxidant and immunity parameters analysis.
2.4 Biochemistry, antioxidant and immunity parameters
The intestine or liver samples of M. salmoides were mixed with cooled phosphate buffered saline (PBS; 1:9, w/v) and homogenized using an automatic sample rapid grinder (Xinzhi Biotechnology Co., Ltd., Ningbo, China) on ice. After centrifugation for 10 min (2500 rpm/min, 4°C), the supernatant was collected and used to measure antioxidant and immunity parameters. ROS and complement3 (C3) contents were measured using commercial ELISA kits (Youxuan Biotechnology, Shanghai, China; Jiancheng Biotechnology, Nanjing, China, respectively). The remaining parameters were measured using commercial kits (Jiancheng Biotechnology, Nanjing, China) as follows: aspartate aminotransferase (AST, no.C010-2-1), alanine aminotransferase (ALT, no.C009-2-1), glucose (GLU, no.A154-1-1), triglyceride (TG, no.A110-1-1), total cholesterol (TCH, no.A111-1-1), low-density lipoprotein cholesterol (LDL-C, no.A113-1-1), high-density lipoprotein cholesterol (HDL-C, no.A112-1-1), malondialdehyde (MDA, no.A003-1-2), total superoxide dismutase (T-SOD, no.A001-1-1), catalase (CAT, no.A007-1-1), glutathione peroxidase (GPx, no.A005-1-2), alkaline phosphatase (AKP, no.A059-2-1), acid phosphatase (ACP, no.A060-2-1), and lysozyme (LZM, no.A050-1-1). All procedures were conducted following the manufacturer’s protocols.
For the ROS or C3 content assay, samples were added to a 96-well plate coated with ROS antibodies. After incubation at 37°C for 60 min and a thorough rinse, tetramethylbenzidine chromogen solution was added to each well to complete the reaction. The optical density was read at 450 nm, and the ROS and C3 contents were calculated using the curve formula, respectively.
The AST reaction product 2, 4 dinitrophenylhydrazone and ALT reaction product phenylhydrazone pyruvate appear reddish brown in alkaline solution, and both have a maximum absorption peak at 505 nm. Thus, AST and ALT activities were calculated from the standard curve. The GLU content was measured using the glucose oxidase method, with absorbance measured at 505 nm using a visible light spectrophotometer. The TG content was measured using the GPO-PAP enzymatic method and the absorbance was measured at 500 nm. The TCH content was determined by measuring the color depth of red quinide at 500 nm. The LDL-C and HDL-C contents were determined by measuring the color change in benzoquinone pigment at 550 nm.
The MDA content was measured using the TBA method. MDA in the peroxide lipid degradation product can condense with TBA to generate a red product with a maximum absorption peak at 532 nm. T-SOD activity was determined by the xanthine oxidase method. Absorbance was measured at 550 nm using a visible light spectrophotometer. GPx activity was measured by determining the reduced glutathione consumption rate at 412 nm.
For the CAT activity assay, the reaction of H2O2 decomposition by catalase can be rapidly suspended by adding ammonium molybdate. The remaining H2O2 and ammonium molybdate produces a yellow complex and the CAT activity was determined by measuring the degree of change at 405 nm.
The AKP and ACP activities were determined by measuring the color depth of red quinone derivatives at 520 nm. The LZM activity was determined by measuring the change in transmittance of turbid bacterial liquid at 530 nm.
2.5 Intestinal and liver histopathology
The mid-intestines (about 1 cm in length) and livers (0.5 cm × 0.5 cm × 0.5 cm) of three fish per tank were sampled and fixed immediately in 4% formalin solution for 24 h. The samples were then embedded in paraffin blocks from which 4-μm-thick sections were cut and stained with hematoxylin-eosin (H&E) according to a method described on a previous studies (Li et al., 2024).
2.6 Intestinal microbiota analysis
The DNA from the intestine (about 50 mg) of three fish per group was extracted using a TIANamp Stool DNA Kit (TIANGEN, Beijing, China). The extracted DNA then underwent quality control assessment and agarose gel electrophoresis to evaluate the integrity of the samples. Upon passing quality control, the DNA was amplified for the V3–V4 region of the 16S rDNA gene by Shanghai Majorbio Technology Co., Ltd. (Shanghai, China). The amplified products were then purified, quantified, and subjected to sequencing using the Illumina MiSeq platform. Finally, the obtained sequencing reads were analyzed utilizing QIIME2 software.
2.7 Determination of mRNA expression
The extraction and quality inspection of liver total RNA, the synthesis of cDNA, and the instruments and commercial kits for quantitative PCR were used as described previously (Li et al., 2024). The design of the gene primers are showed in Table 2.The gene expression levels were calculated using the 2−△△Ct method (Livak and Schmittgen, 2001).
2.8 Data calculation and statistical analysis
The following equations were used to assess the impact of MLE on various features of M. salmoides:
The significance of differences between groups was analyzed using a one-way ANOVA with Tukey’s test for multiple comparisons. Statistical analysis was performed using SPSS22 (SPSS, Inc., USA). P<0.05 was considered to indicate statistical significance, and 0.05 ≤ P< 0.10 was considered to be a tendency.
3 Results
3.1 Growth performance
Compared with the control group, the HS group showed a significant decrease in FBW, WGR, and SGR (P<0.05) (Table 3), but a significant increase in FCR, HSI, and VSI (P<0.05). Dietary MLE supplementation had a trend to increase the WGR and SGR (P =0.093, P =0.088, respectively), and a trend to decrease the FCR and VSI (P =0.090, P =0.080, respectively) in heat-stressed fish.
3.2 Intestinal digestive enzyme activity
As shown in Table 4, HS decreased lipase and trypsin activities compared with the control group (P<0.05), whereas dietary MLE supplementation increased the lipase and trypsin activities in heat-stressed fish (P<0.05).
3.3 Intestine and liver histomorphology
As presented in Table 5 and Figure 1, HS decreased the intestinal villus length and muscular layer thickness compared with the control group (P<0.05), whereas MLE supplementation had a trend to increase the intestinal villus length in heat-stress fish (P =0.065).
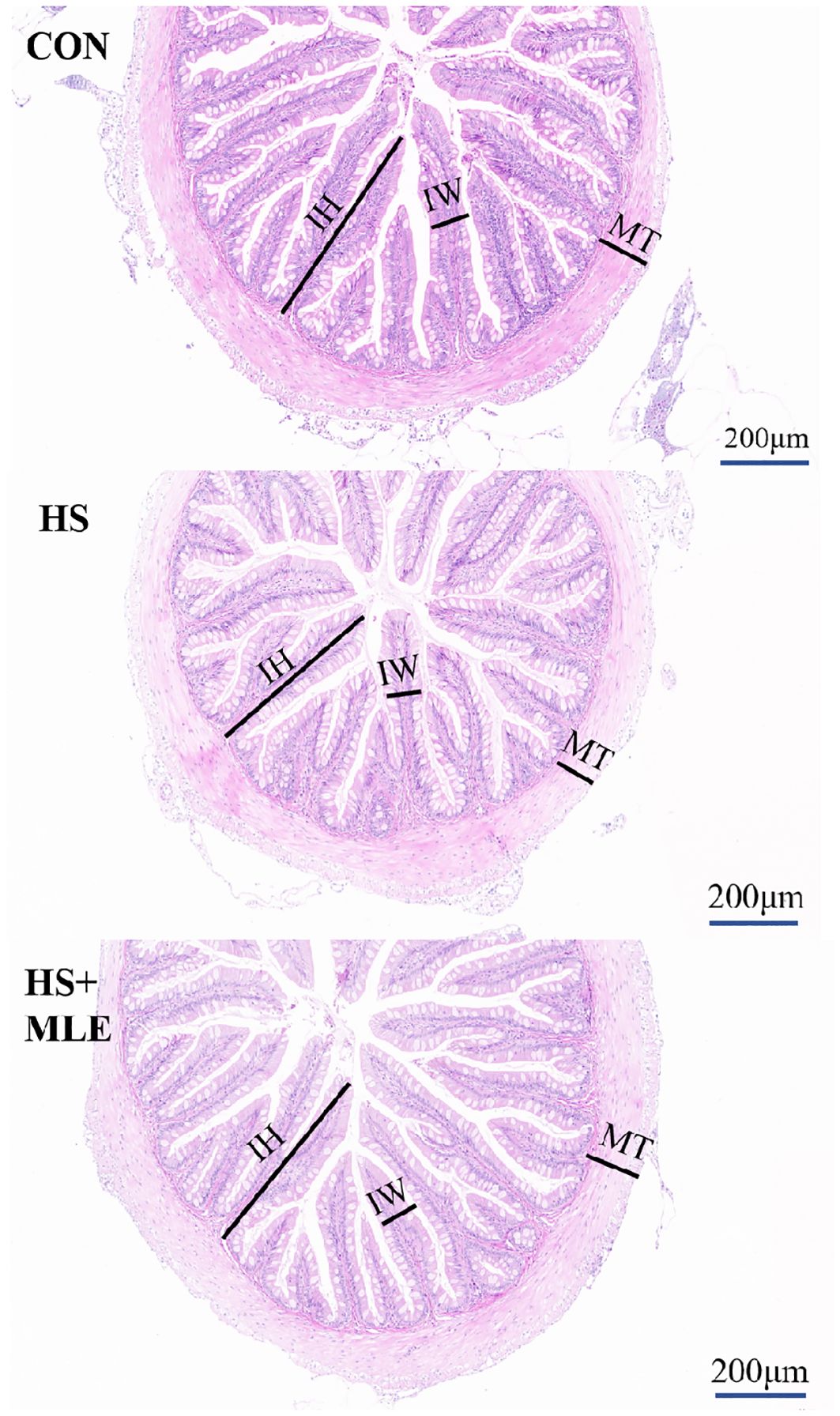
Figure 1. Intestinal histomorphology (hematoxylin&eosin stain) of Micropterus salmoides. CON, fish reared at 26°C, fed a basal diet; HS, fish reared at 33°C, fed a basal diet; HS+MLE, fish reared at 33°C, fed a basal diet supplemented with 9 g/kg mulberry leaf extract. IH, intestinal villus height; IW, intestinal villus width; MT, muscular layer thickness. Scale bar = 200 μm.
In liver, compared with the control group, the HS group showed an increase in intercellular space, numbers of vacuoles and inflammatory cells, whereas MLE supplementation reduced the numbers of vacuoles and inflammatory cells (Figure 2).
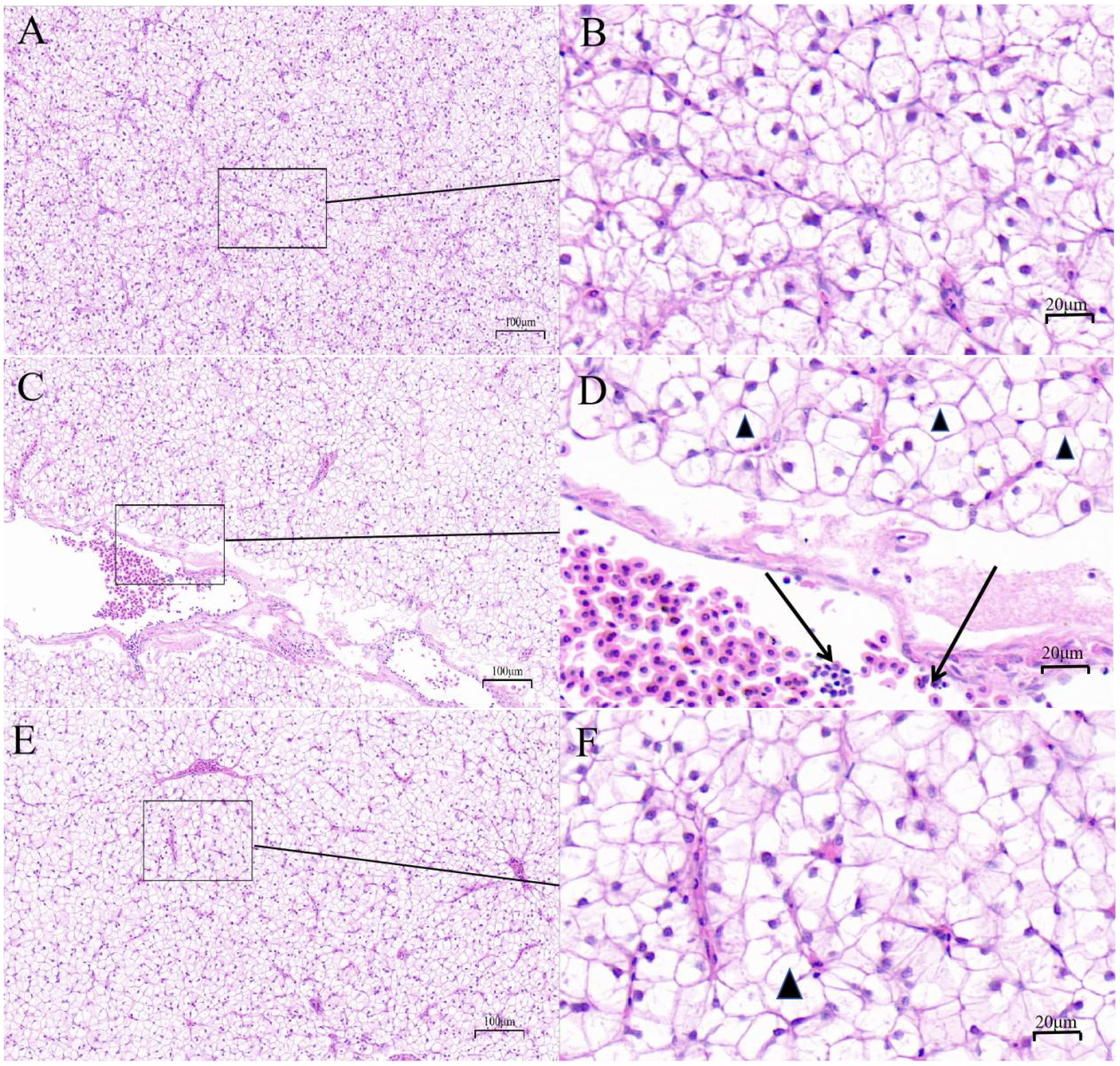
Figure 2. Liver histomorphology (hematoxylin&eosin stain) of Micropterus salmoides. Arrows indicate swelling cells; triangles indicate vacuolization. (A, B) CON group, fish reared at 26°C, fed a basal diet; (C, D) HS group, fish reared at 33°C, fed a basal diet; (E, F) HS+MLE group, fish reared at 33°C, fed a basal diet supplemented with 9 g/kg mulberry leaf extract. The scale bars of (A, C, E) are 100 μm; the scale bars of (B, D, F) are 20 μm.
3.4 Serum biochemistry parameters
As shown in Table 6, compared with the control group, HS increased the activities of AST and ALT, and the contents of TG, TCH and LDL-C (P<0.05), but decreased the HDL-C content (P<0.05). Compared with the HS group, dietary MLE supplementation reduced the activities of AST and ALT, and the contents of GLU, TG, and LDL-C (P<0.05). Meanwhile, compared with the control group, dietary MLE supplementation in heat-stressed fish enhanced the activity of ALT (P<0.05), while reducing the content of GLU (P<0.05).
3.5 Antioxidant parameters
In serum, fish in HS group had lower activities of GPx and CAT (P<0.05), but higher contents of ROS and MDA than those in control group (P<0.05) (Table 7). Dietary MLE supplementation enhanced the activity of GPx (P<0.05), while reducing the content of MDA in heat-stressed fish (P< 0.05).
In the intestine, fish in HS group had higher contents of ROS and MDA (P<0.05), but lower activities of GPx and T-SOD than those in control group (P<0.05). Dietary MLE supplementation had a trend to decrease the ROS content (P =0.064), and enhanced the activity of GPx (P<0.05), while reducing the content of MDA in heat-stressed fish (P<0.05). Meanwhile, compared with the control group, dietary MLE supplementation in heat-stressed fish reduced the activity of GPx (P =0.013).
In the liver, fish in HS group had lower activities of GPx, T-SOD and CAT, but higher contents of MDA and ROS than those in control group (P<0.05). Dietary MLE supplementation had a trend to increase the activities of GPx and CAT (P =0.070, P =0.070, respectively), and reduced the content of ROS in heat-stressed fish (P<0.05).
3.6 Immunity parameters
In serum, compared with control group, HS group had higher activity of AKP and content of C3 (P<0.05) (Table 8). Compared with HS group, dietary MLE supplementation enhanced the activity of ACP, while reducing the content of C3 (P<0.05). Meanwhile, compared with the control group, dietary MLE supplementation in heat-stressed fish enhanced the activities of AKP and ACP (P<0.05).
In intestine, compared with control group, HS group had lower activities of AKP, ACP and LZM (P<0.05). Compared with HS group, dietary MLE supplementation had a trend to increase the activities of LZM and AKP (P =0.066, P =0.066, respectively). Meanwhile, compared with the control group, dietary MLE supplementation in heat-stressed fish reduced the activities of AKP and ACP (P<0.05).
In liver, compared with control group, HS group had a trend to decrease the activity of AKP (P =0.071). Compared with HS group, dietary MLE supplementation enhanced the activity of AKP (P<0.05), and had a trend to increase the activity of LZM (P =0.077).
3.7 Intestinal microbial composition
3.7.1 Intestinal microbial OTUs and beta diversity
In total, 378, 111, and 201 operational taxonomic units (OTUs) were detected in CON, HS, and HS+MLE, respectively (Figure 3A). The specific OTUs of each group were 282, 13, and 90, respectively. The total number of mutual OTUs was 61.
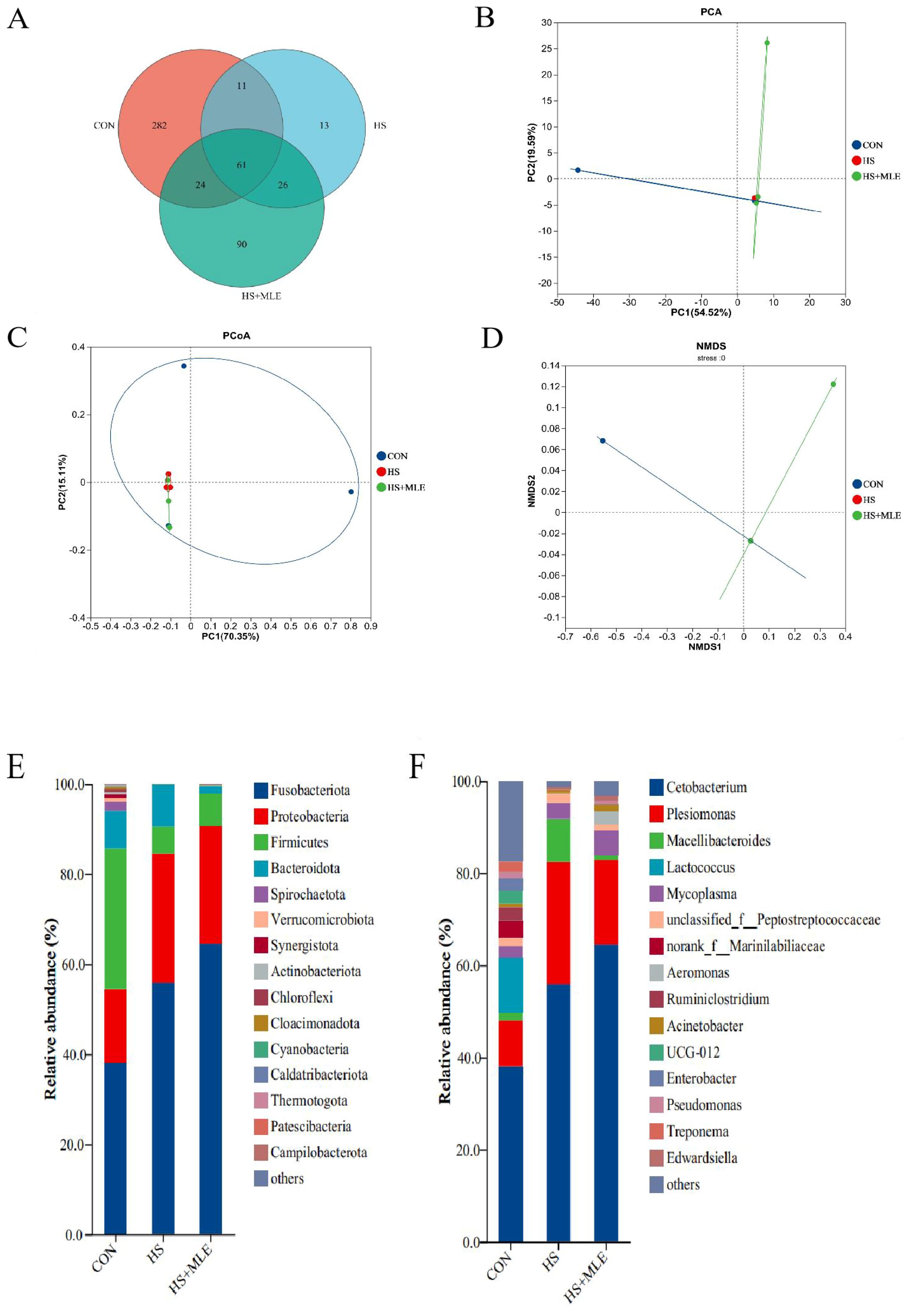
Figure 3. Intestinal microbial compositions of in Micropterus salmoides.Venn diagrams (A); OTUs-based PCA plot (B); Bray curtis distance-based PCoA plot (C); Binary-Jaccard distancebased NMDS plot (D); gut microbiota composition in the groups at the phylum level (E) and genus level (F). CON, fish reared at 26°C, fed a basal diet; HS, fish reared at 33°C, fed a basal diet; HS+MLE, fish reared at 33°C, fed a basal diet supplemented with 9 g/kg mulberry leaf extract.
The beta diversity analysis revealed significant differences in species diversity among the samples, as indicated by reliable data on the PCA, PCoA, and NMDS coordinate plots (Figures 3B–D). Furthermore, it was evident that the composition and distribution of intestinal microbiota among the three groups could be visually distinguished. Notably, the composition of the intestinal microbiota of M. salmoides in the HS group and the HS+MLE group was more similar.
3.7.2 Intestinal microbial alpha diversity
As presented in Table 9, fish in HS group had lower ACE, Chao1 and Shannon indices than those in control group (P<0.05). Compared with HS group, dietary MLE supplementation enhanced the ACE and Chao1 indices (P<0.05). Meanwhile, compared with the control group, dietary MLE supplementation in heat-stressed fish reduced the ACE, Chao1 and Shannon indices (P<0.05).
3.7.3 Species composition and abundance of intestinal microbes
The top 15 phyla with relative abundance in each group were sorted, and the composition structure was analyzed (Figure 3E). Fusobacteria, Proteobacteria, Firmicutes, and Bacteroidetes were the dominant flora in the intestinal tract of M. salmoides. Compared with the control group, HS significantly increased the relative abundances of Fusobacteria and Proteobacteria, significantly decreased the abundance of Firmicutes. Dietary MLE supplementation enhanced the relative abundances of Fusobacteria and Firmicutes, and reduced the relative abundance of Proteobacteria in heat-stressed fish. At the genus level (Figure 3F), Cetobacterium and Plesiomonas were the dominant flora in the intestinal tract of M. salmoides. Compared with the control group, HS significantly increased the abundances of Cetobacterium and Plesiomonas. Dietary MLE supplementation enhanced the relative abundance of Cetobacterium, and reduced the relative abundance of Plesiomonas in the gut of M. salmoides under HS.
3.8 Expression of genes related to antioxidant and immunity
Compared with the control group, HS exposure decreased the mRNA expression level of gpx (P<0.05) (Figure 4A), whereas increased the mRNA expressions levels of nf-κb, il-8 and tnf-a (P<0.05) (Figure 4B). HS exposure caused an increased tendency of nf-κb (P =0.061) and a decreased tendency of sod (P =0.060) on the mRNA expressions levels compared with the control group. In contract, dietary MLE supplementation decreased the mRNA expressions levels of il-8 and tnf-a (P<0.05), while increasing the mRNA expressions levels of gpx, tgf-β, and il-10 in fish under HS (P<0.05).
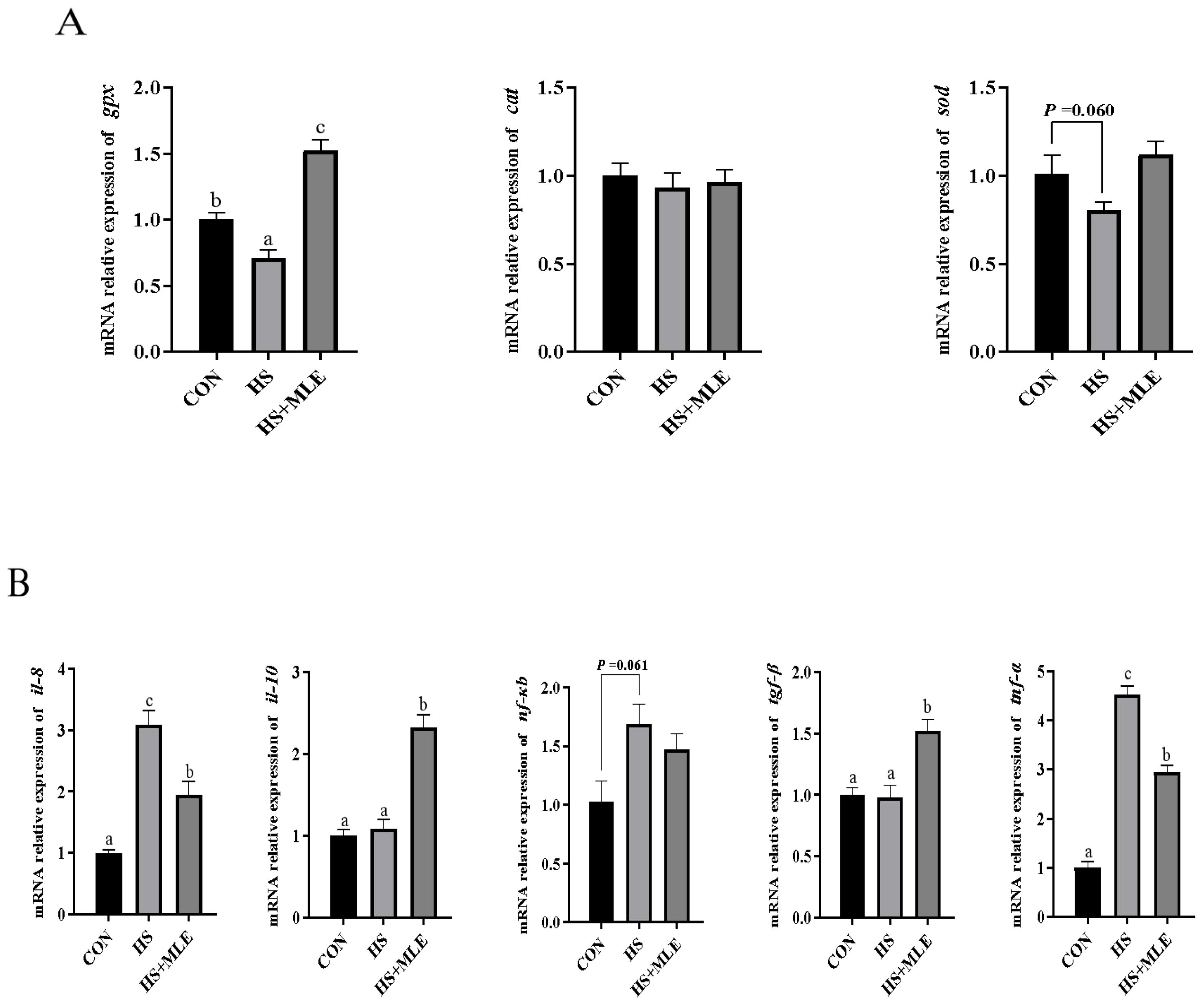
Figure 4. Relative expression levels of antioxidant (A) and immune (B) related genes in the liver of Micropterus salmoides. CON, fish reared at 26°C, fed a basal diet; HS, fish reared at 33°C, fed a basal diet; HS+MLE, fish reared at 33°C, fed a basal diet supplemented with 9 g/kg mulberry leaf extract. abc Indicates that the same columns carrying various superscripts are significantly different at P<0.05.
3.9 Expression of genes related to hepatocyte apoptosis
Compared with the control group, HS up-regulated the mRNA expressions levels of grp78, atf4, perk, chop, ire1, traf2, ask1, and jnk1 (P<0.05), and down-regulated the mRNA expressions levels of bcl-2 and jnk2 (P<0.05) (Figure 5A). In contract, dietary MLE supplementation down-regulated the mRNA expressions levels of atf4, chop, ire1, and jnk1 (P<0.05), and up-regulated the mRNA expressions levels of bcl-2 and jnk2 (P<0.05). In addition, dietary MLE down-regulated the mRNA expressions levels of TRPV, MCU, and VDAC in the liver of heat-stressed fish (P<0.05) (Figure 5B).
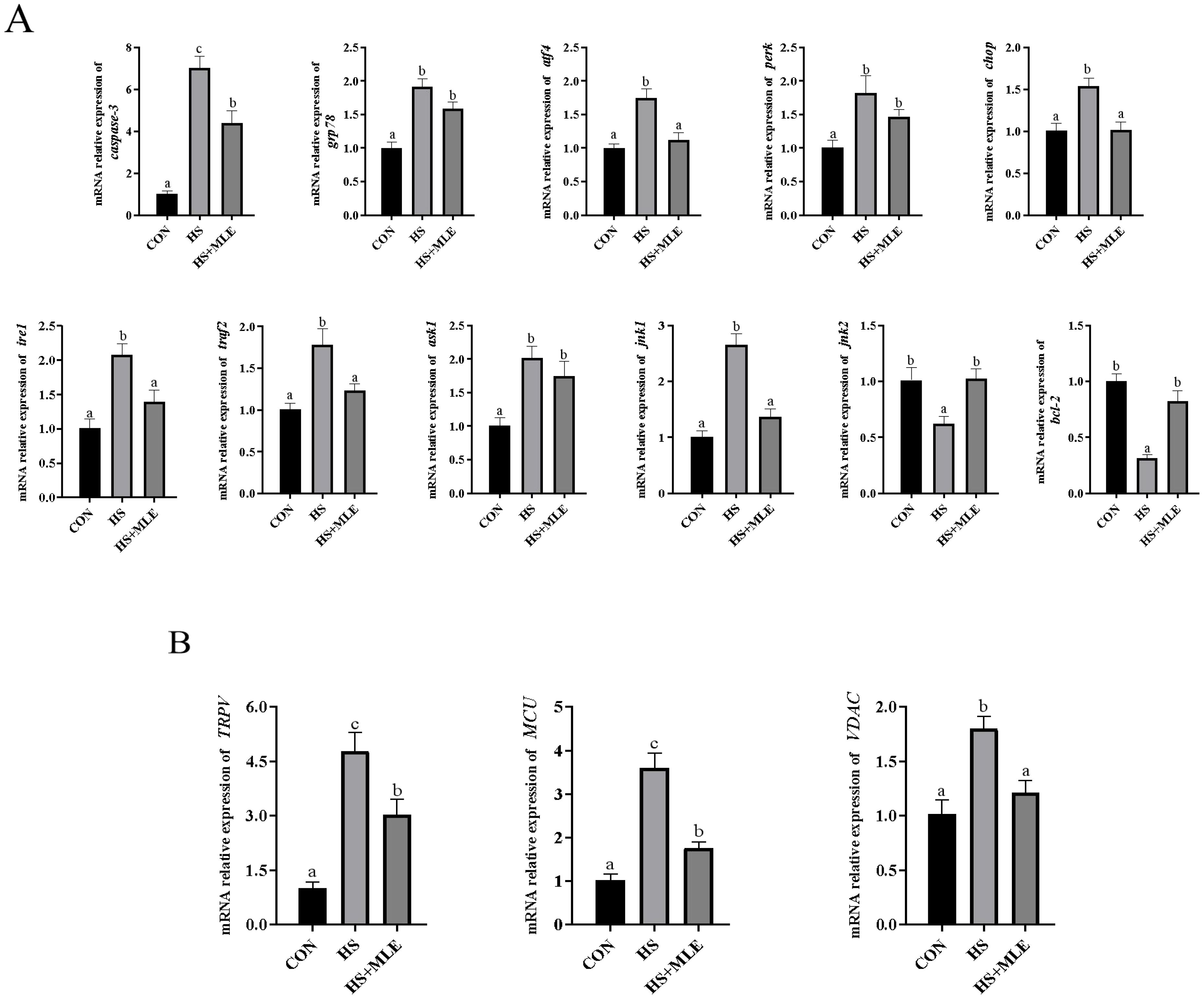
Figure 5. Relative expression levels of endoplasmic reticulum stress (A) and Ca2+ transporter (B) related genes in the liver of Micropterus salmoides. CON, fish reared at 26°C, fed a basal diet; HS, fish reared at 33°C, fed a basal diet; HS+MLE, fish reared at 33°C, fed a basal diet supplemented with 9 g/kg mulberry leaf extract. abc Indicates that the same columns carrying various superscripts are significantly different at P<0.05.
4 Discussion
4.1 Growth performance
As cold-blooded animals, aquatic organisms experience an increase in the energy required to maintain basal metabolism when exposed to high temperature. Concurrently, the energy allocated to food digestion decreases, resulting in a loss of appetite and reduced food intake. This cascade of effects ultimately leads to growth inhibition or mortality, as reported in O. niloticus, Atlantic salmon (Salmo salar), European seabass (Dicentrarchus labrax), and M. salmoides (Li Y. et al., 2023; Beemelmanns et al., 2021; Islam et al., 2020; Dinken et al., 2022). Similarly, in this study, after 30-day HS feeding trial, the FBW, WGR, and SGR of M. salmoides were significantly decreased, while FCR was significantly increased. Yu et al. (2023) reported that steroid biosynthesis, which is closely related to growth, reproduction, and development, was significantly down-regulated in the liver of M. salmoides under high temperature stress. This down-regulation might be one reason for the reduced growth performance observed in the HS group. Plant extracts have been reported to increase growth hormone secretion and up-regulate the expressions of growth factors (e.g., GH and IGF-1) (Tan et al., 2017; Ahmadifar et al., 2021). In the present study, supplementation with MLE showed an increased tendency of WGR and SGR in heat-stressed M. salmoides. However, some studies have also indicated that plant extracts do not significantly improve growth performance even under normal temperature condition (Peterson et al., 2015; Tan et al., 2018). These inconsistent results might be related to the plant species used, the amount of plant extract added, the extraction process, the compositions of the plant extract, and the animal species. The HSI is one of the parameters that indirectly reflects the healthy growth of fish. In this trial, HSI in the HS group was significantly higher than that in the control group, which could be attributed to disordered hepatic lipid metabolism induced by prolonged long-term high temperature stress. Flavonoids in MLE can reduce the expression levels of SREBP1, ACC, and FAS through the AMPK signaling pathway, thereby inhibiting lipid synthesis, while increasing the expression level of CPT1A and promoting lipid decomposition (He L. et al., 2022). This is evidenced by the significant decrease in TG and the increase in HDL-C in serum following MLE supplementation.
Furthermore, tissue damage is recognized as one of the primary causes of growth impairment. Khieokhajonkhet et al. (2023) found that 10 weeks of HS induced vacuolation and inflammatory cell infiltration in the liver cells of goldfish (Carassius auratus), which was associated with decreased WGR and increased FCR. Zhao et al. (2024) reported that intestinal mucosal epithelial cells in rainbow trout (Oncorhynchus mykiss) exhibited significant shedding and necrosis after 14 days of HS, resulting in a marked decrease in FBW. Similarly, the substantial reduction in WGR observed in the HS group in this study was closely linked to lesions in intestinal and liver tissues, as well as diminished intestinal digestive enzyme activity. However, normalization of intestinal development and enhancement of digestive enzyme activity were observed in the HS+MLE group. The above results of this study indicated that dietary MLE supplementation can preserve the integrity of tissue morphology in M. salmoides under prolonged HS, prevent liver lipid accumulation resulting from high temperature stress, protect normal physiological functions, and, thus, mitigate growth loss associated with HS.
4.2 Antioxidant and immune capacity
Antioxidant enzymes have a key role in oxidative stress responses during the balance of redox states within vertebrate cells and the maintenance of homeostasis. SOD, CAT, and GPx are involved in regulating cellular defenses against stress (Ighodaro and Akinloye, 2019). SOD converts intracellular oxygen free radicals into hydrogen peroxide, which is then eliminated by CAT and GPx, reducing its toxic effects (Chelikani et al., 2004; Gill and Tuteja, 2010). When exposed to HS, M. salmoides produced excessive ROS, leading to disruption of the redox balance and causing oxidative damage to tissues. Liu et al. (2010) reported that anthraquinone extract enhanced the activities of hepatic CAT and SOD in Giant River Prawn (Macrobrachium rosenbergii) following high temperature stress. In addition, dietary carotenoid was showed to increase the activities of CAT, SOD, and T-AOC in the liver of yellow catfish (Pelteobagrus fulvidraco) after 7 days of HS (Liu et al., 2019). Similarly, after supplementation with MLE, the activity of GPx in the intestine and serum of the HS group was significantly increased, whereas the ROS content was markedly reduced. Flavonoid have been shown to protect against oxidative stress by eliminating free radicals and up-regulating the expression of antioxidant genes mediated by electrophilic response elements (Hassan et al., 2020). MDA is produced by lipid peroxidation, which causes oxidative damage to cell membranes and is considered a biomarker reflecting the level of oxidative stress (Giera et al., 2012). Oxidative stress also leads to the accumulation of aldehydes and cytotoxicity through cross-linking with nucleophilic groups of proteins, nucleic acids, and aminophospholipids (Pohl and Jovanovic, 2019). Yousefi et al. (2022) reported that dietary Hyssop extract can significantly reduce the MDA content in the serum and gill of O. mykiss after 7 days of high temperature stress. Supplementing with Perovskia abrotanoides extract significantly reduced the MDA content in the serum of carp (Cyprinus carpio) (Adineh et al., 2024). Similarly, MLE supplementation significantly reduced the MDA content of the HS group in the present study. This shows that adding MLE can effectively alleviate oxidative stress in M. salmoides under high temperature conditions. Furthermore, the increased expression levels of liver antioxidant genes in the HS+MLE group further clarified the alleviating effect of MLE on oxidative stress under HS.
The immune system of fish can be affected by temperature. LZM is a crucial component of the innate immune response, responsible for maintaining immune defense by degrading the peptidoglycan layer of major Gram-positive bacteria and certain Gram-negative bacteria (Ferraboschi et al., 2021). When fish are exposed to pathogenic bacteria and various stress-inducing substances, the activity of LZM is stimulated, thereby enhancing immune defense through modifications of immune regulatory functions (Kumaresan et al., 2015; Hwang et al., 2016; Yilmaz et al., 2021). As key lysosomal enzymes, AKP and ACP are not only involved in energy homeostasis in the body, but also respond to environmental changes, serving as important components of the nonspecific immune regulatory system (Li et al., 2018; Chen et al., 2021). Ibrahim et al. (2019) found that rocket (Eruca sativa) leaves restored the LZM and IgM levels of O. niloticus under high temperature culture conditions. Reported by Hao et al. (2024) showed that the ACP and LZM activities in juvenile greater amberjack (Seriola dumerili) were significantly higher than those of the control group within 24 h of high temperature stress; however, these activities were significantly reduced after 120 h. Dietary quercetin significantly increased the activity and gene expression of LZM in C. carpio after 48 h of high temperature stress (Armobin et al., 2023). Our results indicated that long-term HS significantly reduced the activities of AKP, ACP, and LZM in the intestines of M. salmoides, as well as the AKP activity in serum. By contrast, supplementation with MLE restored the activities of ACP and AKP in the intestines and the ACP activity in serum, suggesting that MLE supplementation effectively enhances the immunity of M. salmoides under long-term HS. C3 is the primary soluble protein of the innate immune system and exhibits various biological activities, including the promotion of inflammatory responses, the marking of foreign organisms, and the stimulation of B lymphocytes (Alcorlo et al., 2015; Page et al., 2018). Notably, we observed that C3 content in the serum of the HS group was remained higher than that of the control group even after prolonged exposure to HS. This finding aligns with the significant up-regulation of pro-inflammatory factors (il-8, nf-kb, and tnf-α), the pronounced inflammatory response in liver tissue cells, and the marked increase in serum ALT and AST activities observed in the HS group. Furthermore, C3 content in the HS group was effectively inhibited following MLE supplementation. These results suggest that MLE effectively mitigates inflammation of liver under HS through the NF-κB/TLRs signaling pathways.
4.3 Intestinal microbiota
A relatively stable intestinal microbiota is vital for maintaining normal feeding behavior, growth performance, digestive capacity, and fecundity of fish (Ghanbari et al., 2015; Butt and Volkoff, 2019; Yukgehnaish et al., 2020). Under normal temperature, the dominant flora of intestinal microorganisms in M. salmoides are Fusobacteria, Proteobacteria, Firmicutes and Bacteroidetes (Lin et al., 2020; Yu et al., 2021), which is consistent with the results of this study. It indicated that high temperature stress does not change the composition of the main bacterial community of this species. The current results showed that HS significantly increased the relative abundance of Proteobacteria and decreased the relative abundance of Firmicutes, but the change in the relative abundances of the two bacterial phyla showed opposite trends in heat-stressed M. salmoides. These findings are similar to previous studies conducted on C. carpio and D. labrax (Vaz-Moreira et al., 2021; Zhou et al., 2023a). Proteobacteria is generally considered to be the main pathogenic bacteria in fish, while Firmicutes is considered beneficial bacteria (Shin et al., 2015; Liu Y. et al., 2022). Research shows that rising temperatures can disrupt the balance of gut microbiota and promote the growth of Proteobacteria, Pleuromonas, Mycoplasma, and Aeromonas (Yang et al., 2022; Zhou et al., 2022). Similarly, in this study, HS increased the relative abundance of Pleuromonas, while supplementation with MLE in heat-stressed fish not only reduced the relative abundance of Pleuromonas, but also significantly increased the relative abundance of Cetobacterium. Cetobacterium belongs to the phylum Fusobacteria and produces acetic acid, which can regulate insulin expression, enhance amino acid metabolism, and promote protein utilization (Wang et al., 2021; Zhang et al., 2022). The results of the current study show that MLE supplementation effectively reduced the relative abundance of pathogenic bacteria in the intestinal tract of heat-stressed M. salmoides, increased the relative abundance of beneficial bacteria, and reduced blood glucose content and disease probability. High temperature stress led to an increase in the diversity and richness of the intestinal flora of yellowtail kingfish (Seriola lalandi) and tsinling lenok trout (Brachymystax lenok tsinlingensis) (as indicated by the Simpson, Chao1, and Shannon indices) (Soriano et al., 2018; Fang et al., 2023). By contrast, in the current study observed a significant reduction in the ACE and Chao1 indices of the HS group, while these indices were significantly increased in the HS+MLE group. This discrepancy might be attributed to the varying adaptability of different fish species to temperature changes.
4.4 Hepatocyte apoptosis
The latest evidence indicated that HS activates the IRE1-TRAF2-ASK-JNK pathway, resulting in endoplasmic reticulum stress and mitochondrial dysfunction, which ultimately induces hepatocyte apoptosis (Zhao et al., 2022). The c-Jun N-terminal kinase (JNK) modulates various cellular processes, including cell proliferation, differentiation, inflammation, and cell death; however, its effects differ depending on the specific subtype (Zeke et al., 2016). Further research has demonstrated that HS activates JNK1, leading to the induction of P53 expression. The activation of P53 subsequently triggers the downstream effector Bax, causing the release of cytochromec into the cytoplasm and a decrease in mitochondrial membrane potential, which ultimately initiates caspase-dependent intrinsic apoptosis in hepatocytes (Lin et al., 2024). Conversely, JNK2 and Bcl-2 exhibit opposing functions to those of JNK1. In this study, HS was observed to up-regulate the mRNA levels of caspase-3, grp78, chop, perk, atf4, ire1α, traf2, ask1, and jnk1, while down-regulating the mRNA levels of jnk2 and bcl-2. It is worth nothing that dietary MLE supplementation up-regulated the mRNA levels of jnk2 and bcl-2, and down-regulated the mRNA levels of caspase-3, chop, atf4, ire1α, traf2, and jnk1 in heat-stressed M. salmoides. This observation aligns with the protective effect of polyphenol-rich propolis on organelles in the liver of catfish (Clarias gariepinus) under HS (Alfons et al., 2023). Importantly, mitochondrial dysfunction and endoplasmic reticulum stress are accompanied by disruptions in Ca2+ homeostasis within the intracellular environment. Ca2+ homeostasis is primarily regulated by membrane transporters and receptors, including transient receptor potential (TRPV), mitochondrial calcium uniporter (MCU), osteoporosis risk assessment instrument (ORAI), and voltage-dependent anion channel (VADC) (Clapham, 2007; Zhao et al., 2023). Previous study shown that hypoxic stress increased cytoplasmic Ca2+ concentration and upregulated the mRNA levels of TRPV, MCU, ORAI, and VADC of M. salmoides (Zhao et al., 2023). Consistent with this finding, our study revealed that the mRNA levels of TRPV, MCU, and VADC were significantly up-regulated in the HS group and markedly down-regulated following MLE supplementation. Suggesting that MLE might mitigate the detrimental effects of HS on hepatocytes of M. salmoides by inhibiting the expressions of apoptotic factors and preserving intracellular Ca2+ homeostasis.
5 Conclusion
Dietary MLE supplementation can improve the growth, immunity, and diversity of intestinal flora, and inhibit the proliferation of harmful intestinal bacteria in heat-stressed M. salmoides. In addition, MLE is able to alleviate inflammation and reduce apoptosis of liver under HS through NF-κB/TLRs and IRE1-TRAF2-ASK-JNK signaling pathways. This provides an effective strategy for culturing M. salmoides in high temperature conditions.
Data availability statement
The original contributions presented in the study are included in the article/supplementary material. Further inquiries can be directed to the corresponding authors.
Ethics statement
The animal study was approved by the Animal Care and Use Committee, Sericultural & Agri-Food Research Institute, Guangdong Academy of Agricultural Sciences. The study was conducted in accordance with the local legislation and institutional requirements.
Author contributions
BF: Writing – original draft, Project administration, Resources. DZ: Writing – original draft, Funding acquisition. XQ: Writing – original draft. JZ: Writing – original draft. QY: Resources, Writing – original draft. YX: Resources, Writing – original draft. SL: Conceptualization, Writing – original draft. QL: Writing – review & editing. DX: Writing – review & editing, Funding acquisition.
Funding
The author(s) declare financial support was received for the research, authorship, and/or publication of this article. the High-level Guangdong Province Agricultural Science and Technology Demonstration City Construction Fund Project in 2023 (2220060000087, 232006002429), the Collaborative Innovation Center of Guangdong Academy of Agricultural Sciences (XT202304), and the Key Science and Technology Research Projects in Foshan City (2220001018588).
Conflict of interest
The authors declare that the research was conducted in the absence of any commercial or financial relationships that could be construed as a potential conflict of interest.
Generative AI statement
The author(s) declare that no Generative AI was used in the creation of this manuscript.
Publisher’s note
All claims expressed in this article are solely those of the authors and do not necessarily represent those of their affiliated organizations, or those of the publisher, the editors and the reviewers. Any product that may be evaluated in this article, or claim that may be made by its manufacturer, is not guaranteed or endorsed by the publisher.
References
Adineh H., Zahedi S., Yousefi M., Sedaghat Z., Yilmaz S., Gholamalipour Alamdari E., et al. (2024). The use of Perovskia abrotanoides extract in ameliorating heat stress-induced oxidative damage and improving growth efficiency in carp juveniles (Cyprinus carpio). Aquacult. Nutr. 2024, 5526562. doi: 10.1155/2024/5526562
Ahmadifar E., Pourmohammadi Fallah H., Yousefi M., Dawood M. A. O., Hoseinifar S. H., Adineh H., et al. (2021). The gene regulatory roles of herbal extracts on the growth, immune system, and reproduction of fish. Animals 11, 2167. doi: 10.3390/ani11082167
Alcorlo M., López-Perrote A., Delgado S., Yébenes H., Subías M., Rodríguez-Gallego C., et al. (2015). Structural insights on complement activation. FEBS J. 282, 3883–3891. doi: 10.1111/febs.13399
Alfons M. S., Ibrahim A. T. A., Harabawy A. S., Al-Salahy M. B., Badr G. (2023). Cytoprotective effect of propolis on heat stress induces alteration to histological, ultrastructural, and oxidative stress in catfish (Clarias gariepinus). Environ. Sci. pollut. R. 30, 114152–114165. doi: 10.1007/s11356-023-30386-y
Andrés C., Pérez de la Lastra J., Juan C., Plou F., Pérez-Lebeña E. (2022). The role of reactive species on innate immunity. Vaccines 10, 1375. doi: 10.3390/vaccines10101735
Andrés J. C., Pérez de la Lastra J. M., Plou F. J., Eduardo P. L. (2021). The chemistry of reactive oxygen species (ROS) revisited:outlining their role in biological macromolecules (DNA, lipids and proteins) and induced pathologies. Int. J. Mol. Sci. 22, 4642. doi: 10.3390/ijms
Armobin K., Ahmadifar E., Adineh H., Samani M. N., Kalhor N., Yilmaz S., et al. (2023). Quercetin application for common carp (Cyprinus carpio): I. effects on growth performance, humoral immunity, antioxidant status, immune-related genes, and resistance against heat stress. Aquacult. Nutr. 2023, 1168262. doi: 10.1155/2023/1168262
Beemelmanns A., Zanuzzo F. S., Xue X., Sandrelli R. M., Rise M. L., Gamperl A. K. (2021). The transcriptomic responses of Atlantic salmon (Salmo salar) to high temperature stress alone, and in combination with moderate hypoxia. BMC Genomics 22, 1–33. doi: 10.1186/s12864-021-07464-x
Butt R. L., Volkoff H. (2019). Gut microbiota and energy homeostasis in fish. Front. Endocrinol. 10. doi: 10.3389/fendo.2019.00009
Chelikani P., Fita I., Loewen P. C. (2004). Diversity of structures and properties among catalases. Cell. Mol. Life Sci. (CMLS). 61, 192–208. doi: 10.1007/s00018-003-3206-5
Chen Y., Dong X., Yang Q., Liu H., Nie Q., Hu J., et al. (2021). Effect of yeast hydrolysate supplementation on the resistance of Pacific white shrimp, Litopenaeus vannamei, challenged with low salinity stress. Aquac. Res. 53, 409–418. doi: 10.1111/are.15582
Chen H., Hu Q., Kong L., Rong H., Bi B. (2022). Effects of temperature on the growth performance, biochemical indexes and growth and development-related genes expression of juvenile hybrid sturgeon (Acipenser baerii♀ × Acipenser schrenckii♂). Water 14, 2368. doi: 10.3390/w14152368
Chen G., Long J., Li H., Xu J., Yuan J., Yang Q., et al. (2023). The protective effect of a dietary extract of mulberry (Morus alba L.) leaves against a high stocking density, copper and trichlorfon in crucian carp (Carassius auratus). Animals 13, 2652. doi: 10.3390/ani13162652
Dinken C. P., Keretz K. R., Schramm H. L., Petrie-Hanson L., Schilling M. W., Allen P. J. (2022). The effects of water temperature and simulated angling on the physiological stress response of largemouth bass. T. Am. Fish. Soc 151, 487–506. doi: 10.1002/tafs.10365
Du J., Xie Y., Li M., Zhu T., Lei C., Song H., et al. (2024). Effects of chronic heat stress on growth performance, liver histology, digestive enzyme activities, and expressions of HSP genes in different populations of largemouth bass (Micropterus salmoides). Aquacult. Rep. 35, 101972. doi: 10.1016/j.aqrep.2024.101972
Fang M., Lei Z., Ruilin M., Jing W., Leqiang D. (2023). High temperature stress induced oxidative stress, gut inflammation and disordered metabolome and microbiome in tsinling lenok trout. Ecotox. Environ. Safe. 266, 115607. doi: 10.1016/j.ecoenv.2023.115607
Ferraboschi P., Ciceri S., Grisenti P. (2021). Applications of lysozyme, an innate immune defense factor, as an alternative antibiotic. Antibiotics 10, 1534. doi: 10.3390/antibiotics10121534
Ghanbari M., Kneifel W., Domig K. J. (2015). A new view of the fish gut microbiome: Advances from next-generation sequencing. Aquacult. 448, 464–475. doi: 10.1016/j.aquaculture.2015.06.033
Giera M., Lingeman H., Niessen W. M. A. (2012). Recent advancements in the LC- and GC-based analysis of malondialdehyde (MDA): a brief overview. Chromatographia 75, 433–440. doi: 10.1007/s10337-012-2237-1
Gill S. S., Tuteja N. (2010). Reactive oxygen species and antioxidant machinery in abiotic stress tolerance in crop plants. Plant Physiol. Bioch. 48, 909–930. doi: 10.1016/j.plaphy.2010.08.016
Gong Y., Chen S., Wang Z., Li W., Xie R., Zhang H., et al. (2024). Dietary lipid sources affect growth performance, lipid deposition, antioxidant capacity and inflammatory response of largemouth bass (Micropterus salmoides). Fish Shellfish Immun. 150, 109635. doi: 10.1016/j.fsi.2024.109635
Guo K., Zhang R., Luo L., Wang S., Xu W., Zhao Z. (2023). Effects of thermal stress on the antioxidant capacity, blood biochemistry, intestinal microbiota and metabolomic responses of Luciobarbus capito. Antioxidants 12, 198. doi: 10.3390/antiox12010198
Hao R., Li H., Tian Y., Ru X., Deng Q., Zhu K., et al. (2024). The effect of heat stress on energy metabolism, immune function, and oxidative stress of Juvenile greater amberjack Seriola dumerili. Aquac. Res. 2024, 4406165. doi: 10.1155/2024/4406151
Hassan F.-u., Arshad M. A., Li M., Rehman M.S.-u., Loor J. J., Huang J. (2020). Potential of mulberry leaf biomass and its flavonoids to improve production and health in ruminants: mechanistic insights and prospects. Animals 10, 2076. doi: 10.3390/ani10112076
He L., Xing Y., Ren X., Zheng M., Yu S., Wang Y., et al. (2022). Mulberry leaf extract improves metabolic syndrome by alleviating lipid accumulation in vitro and in vivo. Molecules 27, 5111. doi: 10.3390/molecules27165111
He Y., Yu H., Zhang Z., Zhang J., Kang S., Zhang X. (2022). Effects of chronic hypoxia on growth performance, antioxidant capacity and protein turnover of largemouth bass (Micropterus salmoides). Aquacult. 561, 738673. doi: 10.1016/j.aquaculture.2022.738673
Hwang B. O., Kim Y. K., Nam Y. K. (2016). Effect of hydrogen peroxide exposures on mucous cells and lysozymes of gill tissues of olive flounder Paralichthys olivaeceus. Aquac. Res. 47, 433–444. doi: 10.1111/are.12504
Ibrahim R. E., El-Houseiny W., Behairy A., Abo-Elmaaty A., Al-Sagheer A. A. (2019). The palliative role of Eruca sativa leaves dietary supplementation against oxidative stress, immunosuppression, and growth retardation in temperature-stressed Oreochromis niloticus. J. Therm. Biol. 84, 26–35. doi: 10.1016/j.jtherbio.2019.05.026
Ighodaro O. M., Akinloye O. A. (2019). First line defence antioxidants-superoxide dismutase (SOD), catalase (CAT) and glutathione peroxidase (GPX): their fundamental role in the entire antioxidant defence grid. Alex. J. Med. 54, 287–293. doi: 10.1016/j.ajme.2017.09.001
Islam M. J., Kunzmann A., Bögner M., Meyer A., Thiele R., James Slater M. (2020). Metabolic and molecular stress responses of European seabass, Dicentrarchus labrax at low and high temperature extremes. Ecol. Indic. 112, 106118. doi: 10.1016/j.ecolind.2020.106118
Islam M. J., Kunzmann A., Slater M. J. (2021). Responses of aquaculture fish to climate change-induced extreme temperatures: a review. J. World Aquacult. Soc 53, 314–366. doi: 10.1111/jwas.12853
Ito F., Sono Y., Ito T. (2019). Measurement and clinical significance of lipid peroxidation as a biomarker of oxidative stress: oxidative stress in diabetes, atherosclerosis, and chronic inflammation. Antioxidants 8, 72. doi: 10.3390/antiox8030072
Khieokhajonkhet A., Phoprakot M., Aeksiri N., Kaneko G., Phromkunthong W. (2023). Effects of thermal stress responses in goldfish (Carassius auratus): growth performance, total carotenoids and coloration, hematology, liver histology, and critical thermal maximum. Fish Physiol. Biochem. 49, 1391–1407. doi: 10.1007/s10695-023-01263-9
Kim J. H., Park H. J., Kim K. W., Hwang I. K., Kim D. H., Oh C. W., et al. (2017). Growth performance, oxidative stress, and non-specific immune responses in juvenile sablefish, Anoplopoma fimbria, by changes of water temperature and salinity. Fish Physiol. Biochem. 43, 1421–1431. doi: 10.1007/s10695-017-0382-z
Kumaresan V., Bhatt P., Ganesh M. R., Harikrishnan R., Arasu M., Al-Dhabi N. A., et al. (2015). A novel antimicrobial peptide derived from fish goose type lysozyme disrupts the membrane of Salmonella enterica. Mol. Immunol. 68, 421–433. doi: 10.1016/j.molimm.2015.10.001
Li Z., Chen X., Chen Y., Li W., Feng Q., Zhang H., et al. (2020). Effects of dietary mulberry leaf extract on the growth, gastrointestinal, hepatic functions of Chinese giant salamander (Andrias davidianus). Aquac. Res. 51, 2613–2623. doi: 10.1111/are.14639
Li Q., Fu B., Huang L., Wang F., Zhou D., Yang Q., et al. (2024). Effects of silkworm pupae powder on growth performance, muscle fatty acid composition, and intestinal function in mandarin fish (Siniperca chuatsi). Aquacult. Rep. 39, 102435. doi: 10.1016/j.aqrep.2024.102435
Li Y., Fu B., Zhang J., Wang G., Gong W., Tian J., et al. (2023). Effects of heat stress on the chemical composition, oxidative stability, muscle metabolism, and meat quality of Nile tilapia (Oreochromis niloticus). Food Chem. 426, 136590. doi: 10.1016/j.foodchem.2023.136590
Li H., Lu L., Wu M., Xiong X., Luo L., Ma Y., et al. (2020). The effects of dietary extract of mulberry leaf on growth performance, hypoxia-reoxygenation stress and biochemical parameters in various organs of fish. Aquacult. Rep. 18, 100494. doi: 10.1016/j.aqrep.2020.100494
Li C., Ren Y., Jiang S., Zhou S., Zhao J., Wang R., et al. (2018). Effects of dietary supplementation of four strains of lactic acid bacteria on growth, immune-related response and genes expression of the juvenile sea cucumber Apostichopus japonicus Selenka. Fish Shellfish Immun. 74, 69–75. doi: 10.1016/j.fsi.2017.12.037
Li X., Wu X., Li X., Zhu T., Zhu Y., Chen Y., et al. (2023). Effects of water temperature on growth performance, digestive enzymes activities, and serum indices of juvenile Coreius guichenoti. J. Therm. Biol. 115, 103595. doi: 10.1016/j.jtherbio.2023.103595
Lin Z., Cai Z., Li L., Wei Y., Ling Q. (2024). c-Jun N-terminal kinase 1/P53 signaling mediates intrinsic apoptosis of largemouth bass (Micropterus salmoides) hepatocytes under heat stress. Sci. Total Environ. 947, 174664. doi: 10.1016/j.scitotenv.2024.174664
Lin S. M., Zhou X. M., Zhou Y. L., Kuang W. M., Chen Y. J., Luo L., et al. (2020). Intestinal morphology, immunity and microbiota response to dietary fibers in largemouth bass, Micropterus salmoide. Fish Shellfish Immun. 103, 135–142. doi: 10.1016/j.fsi.2020.04.070
Liu Y., Huang H., Fan J., Zhou H., Zhang Y., Cao Y., et al. (2022). Effects of dietary non-starch polysaccharides level on the growth, intestinal flora and intestinal health of juvenile largemouth bass Micropterus salmoides. Aquacult. 557, 738343. doi: 10.1016/j.aquaculture.2022.738343
Liu F., Qu Y. K., Wang A. M., Yu Y. B., Yang W. P., Lv F., et al. (2019). Effects of carotenoids on the growth performance, biochemical parameters, immune responses and disease resistance of yellow catfish (Pelteobagrus fulvidraco) under high-temperature stress. Aquacult. 503, 293–303. doi: 10.1016/j.aquaculture.2019.01.008
Liu B., Xie J., Ge X., Xu P., Wang A., He Y., et al. (2010). Effects of anthraquinone extract from Rheum officinale Bail on the growth performance and physiological responses of Macrobrachium rosenbergii under high temperature stress. Fish Shellfish Immun. 29, 49–57. doi: 10.1016/j.fsi.2010.02.018
Liu Z., Zhou T., Gao D. (2022). Genetic and epigenetic regulation of growth, reproduction, disease resistance and stress responses in aquaculture. Front. Genet. 13. doi: 10.3389/fgene.2022.994471
Livak K. J., Schmittgen T. D. (2001). Analysis of relative gene expression data using real-time quantitative PCR and the 2–ΔΔCt method. Methods 25, 402–408. doi: 10.1006/meth.2001.1262
Mugwanya M., Dawood M. A. O., Kimera F., Sewilam H. (2022). Anthropogenic temperature fluctuations and their effect on aquaculture: a comprehensive review. Aquacult. Fish. 7, 223–243. doi: 10.1016/j.aaf.2021.12.005
Neamat-Allah A. N. F., Mahmoud E. A., Mahsoub Y. (2021). Effects of dietary white mulberry leaves on hemato-biochemical alterations, immunosuppression and oxidative stress induced by Aeromonas hydrophila in Oreochromis niloticus. Fish Shellfish Immun. 108, 147–156. doi: 10.1016/j.fsi.2020.11.028
Page M. J., Bester J., Pretorius E. (2018). The inflammatory effects of TNF-α and complement component 3 on coagulation. Sci. Rep. 8, 1812. doi: 10.1038/s41598-018-20220-8
Peterson B. C., Peatman E., Ourth D. D., Waldbieser G. C. (2015). Effects of a phytogenic feed additive on growth performance, susceptibility of channel catfish to Edwardsiella ictaluri and levels of mannose binding lectin. Fish Shellfish Immun. 44, 21–25. doi: 10.1016/j.fsi.2015.01.027
Pohl E. E., Jovanovic O. (2019). The role of phosphatidylethanolamine adducts in modification of the activity of membrane proteins under oxidative stress. Molecules 24, 4545. doi: 10.3390/molecules24244545
Shin N. R., Whon T. W., Bae J. W. (2015). Proteobacteria: microbial signature of dysbiosis in gut microbiota. Trends Biotechnol. 33, 496–503. doi: 10.1016/j.tibtech.2015.06.011
Soriano E. L., Ramírez D. T., Araujo D. R., Gómez-Gil B., Castro L. I., Sánchez C. G. (2018). Effect of temperature and dietary lipid proportion on gut microbiota in yellowtail kingfish Seriola lalandi juveniles. Aquacult. 497, 269–277. doi: 10.1016/j.aquaculture.2018.07.065
Sun L., Wang X., Saredy J., Yuan Z., Yang X., Wang H. (2020). Innate-adaptive immunity interplay and redox regulation in immune response. Redox Biol. 37, 101759. doi: 10.1016/j.redox.2020.101759
Sun J. L., Zhao L. L., Liao L., Tang X. H., Cui C., Liu Q., et al. (2020). Interactive effect of thermal and hypoxia on largemouth bass (Micropterus salmoides) gill and liver: aggravation of oxidative stress, inhibition of immunity and promotion of cell apoptosis. Fish Shellfish Immun. 98, 923–936. doi: 10.1016/j.fsi.2019.11.056
Tan X., Sun Z., Huang Z., Zhou C., Lin H., Tan L., et al. (2017). Effects of dietary hawthorn extract on growth performance, immune responses, growth- and immune-related genes expression of juvenile golden pompano (Trachinotus ovatus) and its susceptibility to Vibrio harveyi infection. Fish Shellfish Immun. 70, 656–664. doi: 10.1016/j.fsi.2017.09.041
Tan X., Sun Z., Liu Q., Ye H., Zou C., Ye C., et al. (2018). Effects of dietary ginkgo biloba leaf extract on growth performance, plasma biochemical parameters, fish composition, immune responses, liver histology, and immune and apoptosis-related genes expression of hybrid grouper (Epinephelus lanceolatus♂ × Epinephelus fuscoguttatus♀) fed high lipid diets. Fish Shellfish Immun. 72, 399–409. doi: 10.1016/j.fsi.2017.10.022
Tang X., Fu J., Gao Q., Liu G., Ye J., Guan W., et al. (2022). Effects of mulberry (Morus alba L.) leaf extracts on growth, immune response, and antioxidant functions in Nile tilapia (Oreochromis niloticus). Ann. Anim. Sci. 22, 349–369. doi: 10.2478/aoas-2021-0038
Tingsen J., Hui L., Junwa H., Zhe L., Yu L., Honghao J., et al. (2022). Mulberry leaf extract improves non-specific immunity and antioxidant capacity of largemouth bass (Micropterus salmoides) fed a high-starch diet. Front. Mar. Sci. 9. doi: 10.3389/fmars.2022.1029360
Vaz-Moreira I., Feher M., Fauszt P., Tolnai E., Fidler G., Pesti-Asboth G., et al. (2021). Effects of phytonutrient-supplemented diets on the intestinal microbiota of Cyprinus carpio. PloS One 16, e0248537. doi: 10.1371/journal.pone.0248537
Wang A., Zhang Z., Ding Q., Yang Y., Bindelle J., Ran C., et al. (2021). Intestinal Cetobacterium and acetate modify glucose homeostasis via parasympathetic activation in zebrafish. Gut Microbes 13, 1–15. doi: 10.1080/19490976.2021.1900996
Yang S., Zhang C., Xu W., Li D., Feng Y., Wu J., et al. (2022). Heat stress decreases intestinal physiological function and facilitates the proliferation of harmful intestinal microbiota in sturgeons. Front. Microbiol. 13. doi: 10.3389/fmicb.2022.755369
Yilmaz S., Ergün S., Çelik E. Ş., Banni M., Ahmadifar E., Dawood M. A. O. (2021). The impact of acute cold water stress on blood parameters, mortality rate and stress-related genes in Oreochromis niloticus, Oreochromis mossambicus and their hybrids. J. Therm. Biol. 100, 103049. doi: 10.1016/j.jtherbio.2021.103049
Yousefi M., Hoseini S. M., Kulikov E. V., Seleznev S. B., Petrov A. K., Babichev N. V., et al. (2022). Effects of dietary Hyssop, Hyssopus officinalis, extract on physiological and antioxidant responses of rainbow trout, Oncorhynchus mykiss, juveniles to thermal stress. Front. Vet. Sci. 9. doi: 10.3389/fvets.2022.1042063
Yu Y., Liu Y., Tan Y., Kumkhong S., Gu Y., Yu H., et al. (2021). Effects of deoxynivalenol-contaminated diet on the composition and diversity of the intestinal microbial community and intestinal ultrastructure of juvenile largemouth bass (Micropterus salmoides). Aquacult. 538, 736544. doi: 10.1016/j.aquaculture.2021.736544
Yu J., Zhong D., Li S., Zhang Z., Mo H., Wang L. (2023). Acute temperature stresses trigger liver transcriptome and microbial community remodeling in largemouth bass (Micropterus salmoides). Aquacult. 573, 739573. doi: 10.1016/j.aquaculture.2023.739573
Yukgehnaish K., Kumar P., Sivachandran P., Marimuthu K., Arshad A., Paray B. A., et al. (2020). Gut microbiota metagenomics in aquaculture: factors influencing gut microbiome and its physiological role in fish. Rev. Aquacult. 12, 1903–1927. doi: 10.1111/raq.12416
Zeke A., Misheva M., Reményi A., Bogoyevitch M. A. (2016). JNK signaling: regulation and functions based on complex protein-protein partnerships. Microbiol. Mol. Biol. Rev. 80, 793–835. doi: 10.1128/MMBR.00043-14
Zhang Z., Fan Z., Yi M., Liu Z., Ke X., Gao F., et al. (2022). Characterization of the core gut microbiota of Nile tilapia (Oreochromis niloticus): indication of a putative novel Cetobacterium species and analysis of its potential function on nutrition. Arch. Microbiol. 204, 690. doi: 10.1007/s00203-022-03301-1
Zhao X., Li L., Li C., Liu E., Zhu H., Ling Q. (2022). Heat stress-induced endoplasmic reticulum stress promotes liver apoptosis in largemouth bass (Micropterus salmoides). Aquacult. 546, 737401. doi: 10.1016/j.aquaculture.2021.737401
Zhao L. L., Liao L., Yan H. X., Tang X. H., He K., Liu Q., et al. (2023). Physiological responses to acute hypoxia in the liver of largemouth bass by alteration of mitochondrial function and Ca2+ exchange. Aquat. Toxicol. 256, 106436. doi: 10.1016/j.aquatox.2023.106436
Zhao C., Wang J., Ren W., Zheng S., Ren Y. (2024). Histological, immune, and intestine microbiota responses of the intestine of rainbow trout (Oncorhynchus mykiss) to high temperature stress. Aquacult. 582, 740465. doi: 10.1016/j.aquaculture.2023.740465
Zhou S., Huang Z., Lin H., Kong L., Ma J., Long Z., et al. (2023a). Effects of mulberry leaf extract on the intestinal health of spotted sea bass (Lateolabrax maculatus). Front. Mar. Sci. 10. doi: 10.3389/fmars.2023.1185795
Zhou S., Lin H., Kong L., Ma J., Long Z., Qin H., et al. (2023b). Effects of mulberry leaf extract on the liver function of Juvenile spotted sea bass (Lateolabrax maculatus). Aquacult. Nutr. 2023, 2892463. doi: 10.1155/2023/2892463
Keywords: mulberry leaf extract, largemouth bass, heat stress, intestinal flora, liver injury, immune ability
Citation: Fu B, Zhou D, Qiu X, Zheng J, Yang Q, Xiao Y, Liao S, Li Q and Xing D (2025) Mulberry leaf extract reduces damage by regulating the oxidative response, immune response and intestinal flora of largemouth bass (Micropterus salmoides) under chronic heat stress. Front. Mar. Sci. 12:1543540. doi: 10.3389/fmars.2025.1543540
Received: 11 December 2024; Accepted: 22 January 2025;
Published: 07 February 2025.
Edited by:
Luca Parma, University of Bologna, ItalyReviewed by:
Yuwen Dong, University of Pennsylvania, United StatesAmit Ranjan, Tamil Nadu Fisheries University, India
Copyright © 2025 Fu, Zhou, Qiu, Zheng, Yang, Xiao, Liao, Li and Xing. This is an open-access article distributed under the terms of the Creative Commons Attribution License (CC BY). The use, distribution or reproduction in other forums is permitted, provided the original author(s) and the copyright owner(s) are credited and that the original publication in this journal is cited, in accordance with accepted academic practice. No use, distribution or reproduction is permitted which does not comply with these terms.
*Correspondence: Qingrong Li, bGlxaW5ncm9uZ0BnZGFhcy5jbg==; Dongxu Xing, ZG9uZ3h1eGluZ0AxMjYuY29t
†These authors have contributed equally to this work