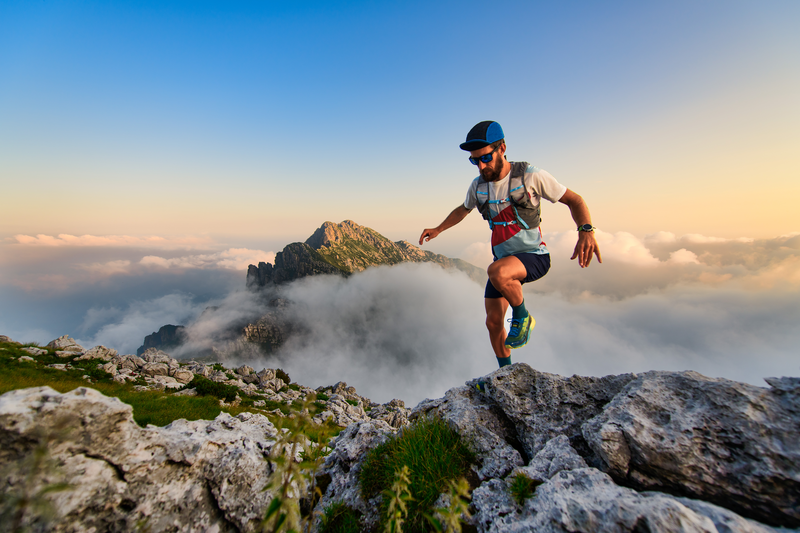
94% of researchers rate our articles as excellent or good
Learn more about the work of our research integrity team to safeguard the quality of each article we publish.
Find out more
ORIGINAL RESEARCH article
Front. Mar. Sci.
Sec. Coral Reef Research
Volume 12 - 2025 | doi: 10.3389/fmars.2025.1539066
The final, formatted version of the article will be published soon.
You have multiple emails registered with Frontiers:
Please enter your email address:
If you already have an account, please login
You don't have a Frontiers account ? You can register here
Mesophotic coral ecosystems (MCEs) – reefs below 30m depth - represent distinct ecological communities that are under threat from local (e.g., fishing) and global scale (e.g., climate change) disturbance. However, most MCEs remain unexplored, and their ecological communities are not well characterized. MCEs on remote offshore seamounts are further unexplored and provide the opportunity to assess assembly rules from comparatively less disturbed MCEs given their remoteness from settlements with high human population densities. Here we characterize the fish community from the remote offshore seamount of Pickle Bank in the Central Caribbean Sea, exploring differences in the fish community between 25m depth compared to the mesophotic zone at 45m depth. We found differences in species composition between depths, with significantly higher abundance of fish at mesophotic depths, while species diversity and species richness were significantly higher at the shallow sites. Species from Labridae and Scaridae dominated the biomass on shallow sites, while species in the family Carangidae dominated biomass in the mesophotic zone. There were also differences in the community composition of trophic guilds between depths, with higher macrocarnivore biomass, macrocarnivore abundance, and omnivore abundance at deep sites compared to shallow sites. Despite the logistical challenges and limitations associated with accessing MCEs on offshore seamounts, these data provide compelling evidence to the growing body of literature documenting MCEs as unique habitats warranting further data collection to obtain a holistic understanding of shallow and mesophotic seamount ecosystems.
Keywords: Caribbean, Cayman Islands, Cuba, coral reef, Remote, offshore, Rebreather, Fisheries
Received: 03 Dec 2024; Accepted: 04 Mar 2025.
Copyright: © 2025 Johnson, Chequer and Goodbody-Gringley. This is an open-access article distributed under the terms of the Creative Commons Attribution License (CC BY). The use, distribution or reproduction in other forums is permitted, provided the original author(s) or licensor are credited and that the original publication in this journal is cited, in accordance with accepted academic practice. No use, distribution or reproduction is permitted which does not comply with these terms.
* Correspondence:
Jack V Johnson, Central Caribbean Marine Institute, Little Cayman, Cayman Islands
Disclaimer: All claims expressed in this article are solely those of the authors and do not necessarily represent those of their affiliated organizations, or those of the publisher, the editors and the reviewers. Any product that may be evaluated in this article or claim that may be made by its manufacturer is not guaranteed or endorsed by the publisher.
Research integrity at Frontiers
Learn more about the work of our research integrity team to safeguard the quality of each article we publish.