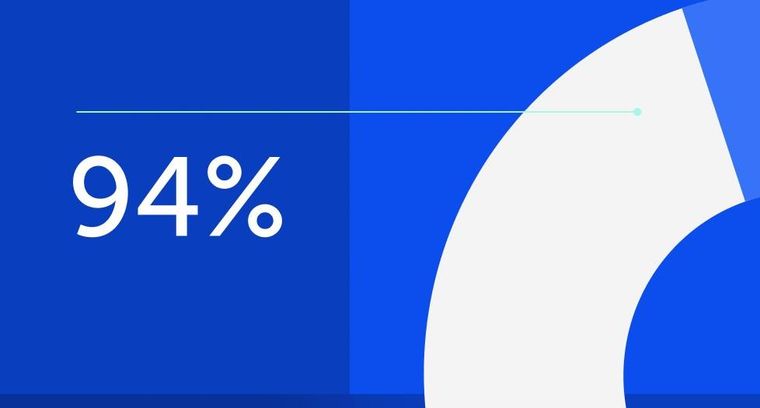
94% of researchers rate our articles as excellent or good
Learn more about the work of our research integrity team to safeguard the quality of each article we publish.
Find out more
ORIGINAL RESEARCH article
Front. Mar. Sci., 11 March 2025
Sec. Marine Fisheries, Aquaculture and Living Resources
Volume 12 - 2025 | https://doi.org/10.3389/fmars.2025.1519832
Sponges are now requested for their commercial, industrial and pharmaceutical importance. This led to an increase in demand in the global markets with uncontrolled and excessive harvesting pressure that, together with diseases outbreaks, put wild populations at risk, with several habitats completely looted. Aquaculture of sponge fragments poses an alternative to wild collection since fragment regeneration is easy, cheap and efficient. We chose as subject of our study Spongia anclotea, common to the tropical Western Atlantic, due to its high request on the market for cosmetics, body care and pharmaceutical applications. We set a low-cost sponge farm made of cheap materials, thus affordable for local communities, which did not require significant maintenance. The growth and survival rate of 384 out of 2304 randomly chosen sponges have been investigated over a 4-year period. At the end of the study, sponges increased by an average rate of 380% ± 275% of their initial volume (with a maximum of 1480%) and 87.5% of sponges survived (death and detach during the whole period were considered as mortality). Farmed sponges from our structure resulted characterized by superior quality and shape compared to collected wild individuals from the area and, after six years of cultivation (4 years of the present study + 2 years of forecasted growth based on our findings), this improved quality could yield higher profits (618 USD vs. 547 USD, respectively, for a standard stock size). This experimental setup can be considered a good alternative to sponge harvesting and a good economic opportunity for developing countries.
Sponges (phylum Porifera) are a monophyletic (Wörheide et al., 2012) group which is estimated to include over 9000 species in 852 genera (de Voogd et al., 2024).
Historically, since the era of the Roman Empire, a small fraction of sponges, belonging to the order Dictyoceratida (family Spongiidae), characterized by a high quality spongin skeleton due to the almost total absence of spicules, great water retention, elasticity and resistance to usage overtime, were harvested for cosmetics in the Mediterranean Sea (Pronzato and Manconi, 2008; Ehrlich et al., 2018; Jesionowski et al., 2018). Recently, sponges have been demonstrated to be usable and profitable in various fields as biomedicine and pharmaceutical, due to the discovery and applications of their secondary metabolites (Faulkner et al., 1993; Amigó et al., 2008; Indraningrat et al., 2016; Abdelaleem et al., 2020; Pawlik and McMurray, 2020), biomonitoring and bioremediation, owing to their proficiency in filtering sea water, removing and accumulating different classes of pollutants (Patel et al., 1985; Olesen and Weeks, 1994; Hansen et al., 1995; Philp, 1999; Perez et al., 2002, 2003, 2005; Milanese et al., 2003; Cebrian et al., 2007; Santos-Gandelman et al., 2014; Orani et al., 2018), and aquaculture, as both farmed target species or as water filtration tool for bioremediation and reduction ecological impacts of farming in integrated multi-trophic aquaculture systems (IMTA) (Longo et al., 2016, 2020; Giangrande et al., 2020; Gökalp et al., 2021; Aguilo-Arce et al., 2023). This increase in demand made sponges a target organism in the global markets. While data before 1900 are not reliable for commercial sponges, a peak of 2 million collected sponges was reported in 1902 (Sella, 1912) and demand in the early 20th century was estimated at 30 tons/year. Wild sponges’ gathering increased over time with over 100 tons/year in England and Germany in the 1930’s (Arndt, 1938), 200 tons per year in 1985 up to around 2000 tons in 2003 (FAO Fishery Information and Unit (FAO-FIDI), 2005).
Since the end of the 20th century, new discoveries on applications of sponges put these organisms under excessive fishing pressure and, together with diseases outbreaks (Webster, 2007), wild populations become severely threatened and several areas faced significant reduction in both sponge abundance and species richness (Croft, 1990; Pronzato, 2003; Bertolino et al., 2017).
This situation led to an increase in sponge aquaculture efforts all over the world, from the Mediterranean Sea to the Atlantic and Pacific Ocean (Cahn, 1948; Storr, 1957, 1964; Croft, 1990; Handley et al., 2003). Researchers tried to develop new farming methods aimed at maximizing efficiency and reducing costs. Over time, the effects of new farming techniques (such as cages, fences, tendales, ropes, net pockets, spikes, and plastic pins (Duckworth et al., 2007; De Caralt et al., 2010; Osinga et al., 2010; Zea et al., 2010; Page et al., 2011; Betanzos-Vega et al., 2019)) on sponge growth, survival, and secondary metabolites production were evaluated, alongside other tests on integrated mariculture with the placement of sponges in association with fish and mussels’ aquaculture systems (Gökalp et al., 2019, 2021; Giangrande et al., 2020; Li et al., 2023). To date, the accepted idea is that sponge aquaculture is species-specific, and each farming strategy must be calibrated to the needs of the target organism (Duckworth, 2009; Schippers et al., 2012). Currently, one of the most common farming techniques is growing fragments taken from natural individuals, taking advantage of the remarkable regenerative ability of these organisms. This practice was first adopted in the Mediterranean Sea (Cavolini, 1785, 1853), then it spread to America, Asia and Oceania (Duckworth et al., 1997, 2007; Louden et al., 2007). Aquaculture of sponge fragments gave new opportunities to developing countries which were facing ecological issues related to the past harvesting of natural individuals. In fact, this fragment regeneration method has been demonstrated to be simple, fast, inexpensive and efficient (Ayling, 1983; Duckworth, 2003). With proper protocols and conditions, a single individual may be a “source” for multiple generations (Osinga et al., 1999; Duckworth, 2001; Belarbi, 2003; Louden et al., 2007) thus shrinking the need of natural donors (Duckworth, 2009). This evidence made farming sponges a win-win choice for communities in developing nations, since it does not require expensive technologies and tools, it is easy to run, profitable and, ecologically speaking, it can decrease the anthropogenic pressure on natural stocks (Yi et al., 2005; Betanzos-Vega et al., 2019).
The genus Spongia is highly rated on the markets its specific features related to personal care, including fine texture and water absorption (Verdenal and Verdenal, 1987). The well-known Spongia pertusa, now accepted as Spongia (Spongia) anclotea de Laubenfels & Storr, 1958, is typical of the Atlantic Ocean coasts of America from Florida to Brazil. This species was chosen due to its high market value for cosmetics and body care and for the newly discovered pharmaceutical applications of its secondary metabolites as both antifungal agent against human pathogens and breast cancer CSC-like cell proliferation inhibitors (Osinga et al., 1999; Tang et al., 2018, 2022; Tian et al., 2023). Thus, improved understanding of its biology and physiology is fundamental for successful aquaculture farming. To our knowledge, only one study by Oronti et al. (2012) did a short-term preliminary investigation on growth and survival of S. anclotea in the Bahamas. Our study is the first long term investigation to address this species’ adaptability to farming. In this work, we aimed to investigate S. anclotea regeneration and growth rates, together with survival and detachment, in an experimental site at Cape Eleuthera, Bahamas. Then, the productivity of the farm was estimated (in US $) and the revenue made by selling farmed sponges was compared to the profits made by selling similar size natural sponges collected from the study area. This allowed us to evaluate the actual applicability and the benefits of this farming system to help the economy of local communities and alleviate the stress on natural sponges’ populations.
The study took place in Cape Eleuthera Bay (Bahamas), near the Cape Eleuthera Island School and Institute (Figure 1). The farm was set in a dredged channel (3 m depth) 15 m far from the shore. The area was chosen basing on a past pilot study (Oronti et al., 2012) in which Spongia anclotea farming at this location resulted in higher growth rates and lower mortality compared to other sites in the area, probably due to higher nutrient terrestrial runoff and weaker wave energy and water turbulence. Furthermore, the vicinity to the shore made setup, sampling and analyses much easier compared to offshore areas.
Figure 1. Study area. Red triangle indicates Spongia anclotea farm setup location at Cape Eleuthera, Eleuthera Island, Bahamas.
A total of 8 farming cells (A-B-C-D-E-F-G-H) were built in November 2010 following the scheme reported in Figure 2, at a depth of 3 m. Cell X was already present from the previous pilot study (Oronti et al., 2012) and filled with older sponges, so it was not considered in this study. Every cell involved 6 strands made of 5 modules 105 cm far from each other. To do so, 240 concrete blocks (15 kg each) were deposited on the bottom, each presenting a vertical iron bar (1 meters long, 19 mm diameter) where two bitumen coated nylon twines were fixed at different heights (15 cm and 45 cm from the top of the bar) and pulled between bars of the same strand.
Figure 2. Scheme of the sponge farm. (A) details of a single unit of a strand (a full strand is made by 4 units). Two concrete blocks (cb) support two iron bars, between which two nylon twines are pulled; 6 sponges (black circles) per twine at the two depths, up-low, are placed (24 on the complete strand). (B) structure of a single cell made of six strands (1-6) viewed from above: dimensions approximately 4 x 5 meters in length and width, respectively. (C) comprehensive map of the farm viewed from above, all cells (A–H) are shown. The left side is near the coastline while the right side is exposed to open sea. The main water current in the area flows downwards.
The upper portions (2/3 of total volume) of donor sponges from the area (estimated between 576 and 768 native individuals, explant volume ranging between 450-600 cm3 per donor sponge) were cut with a knife to obtain fragments, the remaining 1/3 were left sticked to the substrate for recovery as suggested by Duckworth and Battershill (2003). Sponge portions where then divided, ensuring that at least a side had undamaged pinacoderm, into multiple smaller sub-spherical fragments (usually 3-4 fragments per donor sponge). During every processing step, sponges were always kept underwater to avoid air exposition and minimize stress. Fragments were then tied with zip ties 15 cm apart from each other for a total of 48 individuals per strand numbered as 1-24 up for the upper thread and 1-24 down for the lower one. Strands orientation was set parallel to the local water current to minimize hydrodynamic stress.
For growth analysis, due to the large number of samples, only one randomly chosen strand per cell was processed for a total of 2304 samples (6 strands x 2 rows x 24 samples x 8 cells).
The experiment ran from 2010 to 2014. It began in November 2010 (setup and first measures) with subsequent measurements in July 2011, January 2012, July 2012, February 2013 and September 2014. Dimensions were measured underwater with precision calipers assessing the length of the three main axis of the sponges (length, width, height: x, y, z). Volumes were calculated using the ellipsoid as a reference shape (Formula 1), due to its similarity to samples shapes.
Formula 1. Ellipsoid volume.
Growth rate expressed as a percentage was calculated as in Formula 2.
Formula 2. Sponge growth rate. Volume at tx indicates the volume of a sample measured at one of the five sampling times (t1-t5), while volume at t0 represents the initial measurement of that sample’s volume.
The survival rate was assessed considered death or detachment of sponges as one, since both fates resulted as an individual lost for final sale.
The total costs of initial setup and annual maintenance comprising materials (concrete blocks, iron bars, bitumen coated nylon twine, zip ties) and manpower (scuba diving preparation of the structures, monitoring and sponge measuring) have been assessed. At the end of the study, the quality and the market value (wholesale price) of farmed sponges has been explored by experts in the field from the Spugnificio Rosenfield sponge farm of Muggia, Italy, which professional activities consist in importing wholesale farmed or harvested sponges, processing and modelling sponges to obtain highly requested spherical shapes and final resale. The quality of sponges primarily depends on their skeleton shape and texture. Wild sponges, which grow attached to the substrate, develop irregular morphologies and textures that are less commercially desirable. In contrast, sponges cultivated on suspended lines, experiencing no spatial constraints, grow freely in all directions resulting in more spherical shapes and homogeneous texture, which are likely to be defined as 1st quality and highly requested on the market. Last, potential profits for the farmers (revenues minus costs) were estimated and compared with mean profits made by selling collected wild sponges of similar size. Two estimations were made, at t4 (3 years), where sponges reached the commercial size and a forecasted t6 (4 years of the current study, plus 2 years of forecasted growth based on our findings, assuming a constant growth and the absence of potential stressful events), when the farm productivity would be stable and settled. The number of marketable sponges was estimated based on survived individuals at t4. At t6, no difference in numbers was assumed, based on the premise that in an operational farm (unlike our study, where dead/detached sponges were intentionally not replaced) blank spaces are constantly refilled, thereby minimizing the loss of individuals. We considered as revenue only the wholesale price of sponges, excluding additional costs for buyers, as these were not relevant to the study’s focus on the farm’s economic feasibility. Similarly, for collected sponges, we decided to consider as profit the wholesale price for buyers. We excluded the presence of significant additional expenses for sponge fishers, since most of sponge collection in the Caribbean is performed by “staff and hook” or apnea diving in shallow waters with small artisanal boats as support, with almost absent expenses and equipment. In the Bahamas, sponge collection in rural local communities is a sporadic activity carried out by fishermen. For this reason, the cost of the boat has not been considered, as it is already covered by their main fishing operations and does not represent an additional expense. Since the study ended in 2014, prices were updated not by a simple conversion of the past vs current global value of the dollar. We investigated the current local value at the Bahamas of each component for both costs and revenues and reported in American dollars (USD).
To provide a general overview of the physical conditions during the experiment, we reported the seasonal variations and anomalies of several environmental variables during the study period. Satellite data of seawater temperature, chlorophyll-a, oxygen, salinity, nitrates, phosphates, silicates and biomass for the experimental site and timeframe were obtained from the product Global Ocean Physics Reanalysis (doi.org/10.48670/moi-00021), dataset “cmems_mod_glo_phy_my_0.083deg_P1D-m”, and the product Global Ocean Biogeochemistry Hindcast (https://doi.org/10.48670/moi-00019), dataset “cmems_mod_glo_bgc_my_0.25deg_P1D-m”, on Copernicus Marine Service. Details are described in Supplementary Data 1.
Temperature data (from 1987 to 2021) were analyzed using the R package “heatwaveR” to assess Heatwaves “MHW” and Colds Spells “MCS” during the study period, details of the protocol are reported in Supplementary Data 2. Following the same procedure, anomalies over 90th and under 10th percentile were also detected for each other variable (in this case the climatology was calculated over the 1993-2021 period due to limited extent of the available datasets).
Generalized Linear Mixed Models (GLMM) were run to investigate statistical differences in growth rate among different (1) rows (upper-lower), (2) position (1-24), (3) cells (A-H) and their interaction with time (Supplementary Table 1). For (1), we set a GLMM with growth rate as dependent variable, the interaction of row and time as fixed factors, and sample id as random factor. For (2), a GLMM was set with growth rate as dependent variable, the interaction of position and time as fixed factors, and sample id as random factor. For (3), a GLMM was set with growth rate as dependent variable, the interaction of cell and time as fixed factors, and sample id as random factor. To assess differences in survival (death + detach) of sponges among rows, position, cells, three binomial GLMM has been set with alive/dead organisms (as 0/1) as dependent variable (Supplementary Table 1). As for growth rate, the first model included the interaction of row and time as fixed factors, and sample id as random factor. The second model included the interaction of position and time as fixed factors, and sample id as random factor. The third model included the interaction of cells and time as fixed factors, and sample id as random factor. For all models, pairwise comparisons (Tukey test) were run when significant differences were found. The statistical models were developed under the R statistical environment v.3.6.2 (R Core Team, 2021) using package stats (R Core Team, 2021), lme4 (Bates et al., 2015) and emmeans (Lenth, 2023).
A total of 384 sponges were analyzed 6 times during a 4 years period for a total of 2304 measurements (1152 up and 1152 down) (Figures 3B–F). Sampling intervals were November 2010, July 2011, January 2012, July 2012, February 2013, and September 2014.
Figure 3. Experimental setup. (A) wild Spongia anclotea specimen, (B) t0, orange color indicates the fresh cutting surfaces, (C, D) overview of farming area and a zoom on a detached individual at t1, (E) t4, (F) individuals at final sampling time t5.
The average sponge volumes increase rate at t5 measured 380% ± 275%, with the minimum individual value recorded in cell C (-80%) and the highest record in cell D (1480%) (Table 1).
Analyzing the different part of our farming structure, no differences in growth were observed between up and down rows of cells at every time interval and no differences were found between the positions 1-24 on the two rows (Supplementary Table 1). Considering the whole period t0-t5, no differences in growth rate were observed between cells. At t5, differences were observed only between cells C-D and C-G (Figure 4; Supplementary Table 1).
Figure 4. Growth analysis. Growth (log scale) of S. anclotea for each farming cell (A–H) at different time intervals (t0-t5).
A total of 54 out of 384 sponges (14%) were lost to death or detachment during the study, with no statistical differences between rows, position, and cells (Figure 5; Supplementary Table 1).
Figure 5. Mortality analysis. (A) boxplot of detached/dead individuals for each time interval, (B) boxplot of detached/dead individuals for each farming Cell, (C) total number of detached/dead individuals for each farming Cell.
As a personal observation of the authors, since t0, the sandy-orange cut surfaces of the fragments were covered with regenerated pinacoderm within a couple of weeks. We observed that the self-cleaning capacity of the sponges, so evident in wild specimens (Figure 3A), was initially weakened in the transplanted fragments, which tended to be covered by both sediments and epibionts, and it was recovered only one or two years after the start of culturing. Interestingly, this restoration corresponded with the recovery of the initially lost, probably due to cutting and transplantation, endobiont organisms as worms, shrimps, etc. To date, the role of organisms living inside sponges and the nature of their associations are still under debate; if benefits for endobionts (food, shelter) are easier to assess, the benefit for the sponge have not been determined (Westinga and Hoetjes, 1981; Wulff, 2006; Martin and Britayev, 2018; Goren et al., 2021; Lira et al., 2024). Here, we could roughly suggest, as a personal reflection based on qualitative observations, a potential role of these organisms in cleaning the surface of sponges.
Heatwaves and Cold spells, together with Chl-a, oxygen, salinity, nitrates, phosphates, silicates, biomass anomalies calculated for the study period are reported in Figure 6 and Supplementary Figure 1. The climatic conditions of the area have been rather stable for each variable during the study without co-occurring anomalies, with the exception of October/November 2012, during t3-t4, where an intense drop of temperature from the 90th percentile down to a cold spell event (below 10th percentile) took place. Concomitantly, a salinity anomaly below the 10th percentile and anomalous peaks over the 90th percentile of primary productivity and biomass in the area were observed. These anomalies co-occurred with Hurricane Sandy, hitting the Bahamas around the end of October 2012. Nitrates, phosphates and silicates did not show any associated anomaly.
Figure 6. Environmental factors anomalies. Red line is the observed value of the variable, green indicates the climatology, blue and purple line indicates the 90th and 10th percentile for each variable. (A) Temperature, (B) Chlorophyll-a, (C) Oxygen and (D) Salinity. Dotted lines are time intervals t1-t5. Black arrow indicates the arrival of Hurricane Sandy at the study site.
Experts from the Spugnificio Rosenfield sponge farm of Muggia, in the person of Elena Pesle, stated that sponges reached selling size at t4 and defined their quality as first quality for the 80% of sponges, the remaining 20% as second quality. Under their supervision, we assessed the costs (all values reported were updated at 2023) for the initial setup to $387 plus $15 for the annual maintenance activities for a total of $432 at t4. Maintenance consisted mainly in checking the structure integrity and the cleaning of ropes from fouling, activities that can be performed by farm operators on a monthly basis, without the need of external experts and extra costs (Table 2). A common stock of collected wild sponges at entry-level commercial size, usually made by 50% first quality, 50% second quality, is worth $1 per sponge, while a same size farmed high-quality stock is worth about $2/2.5 per sponge. At t4, 348 out of 384 sponges survived and this number was used for the analysis. Based on our results about growth and survival rate of farmed sponges, at a hypothetical forecasted t6 (assuming a constant growth and the absence of potential stressful events), profits made by Spongia anclotea farmed individuals would exceed wild sponges’ collection profits ($618 vs $547) (Table 3).
Marine sponges are a fundamental component of the benthic ecosystem due to their abundance, function and services like shelter provision (Herrnkind et al., 1997; Coppock et al., 2022), bioremediation (Amato et al., 2024) and water filtration (Dayton et al., 1974; Ayling, 1981; Costello and Myers, 1987; Maldonado et al., 2012, 2017; De Goeij et al., 2013; Pawlik and McMurray, 2020). Commercially, sponges request is constantly increasing for cosmetic, industrial, pharmaceutic applications and the supply from natural populations, which are mainly located in developing countries and put under excessive stress, is becoming increasingly unable to support the demand. At the current state of the art, farming sponges requires simple setups and cheap tools (Verdenal, 1990; Duckworth and Battershill, 2003; Page et al., 2005), which could benefit local communities in developing countries who lack large capitals to invest. For these reasons, in our study, we decided to adopt a structure of sponge farming which potentially meets these needs and can be assembled with simple materials (concrete blocks, iron bars and nylon twines), involving low-cost processing and maintenance while guaranteeing efficient sponge growth, as already observed in multiple studies (Corriero et al., 2004; Friday, 2011; Çelik et al., 2011; Maslin et al., 2021; Bierwirth et al., 2022).
The two most important parameters in sponge farming are growth and survival rates (Duckworth, 2009; Santiago et al., 2019; Mohite et al., 2020; Ou et al., 2020). Looking at our results, no significant differences in both growth and mortality were found for the two depths, the position on the row, and cell. From a structural point of view, these homogeneous results indicate our farm setup as a strong and efficient farming environment where, at least for this species, the different depths of farming do not affect sponges’ growth and so does the position on the twine or the position of the cell. According to Duckworth et al. (2007) farmed sponges’ volume should increase by 100% per year in order to be considered profitable. In our study, at t5 (4 years), the mean rate of volume increase accounted for 380% (individual values ranged from a minimum of -80% to a maximum of 1480%). This wide range of results may be related to the variability in terms of state of health, resistance, and resilience among the numerous donor individuals. Within donor sponges, some individuals could have been already in suffering health condition, or they could be less genetically gifted, leading to a shrinkage in volume over time, while others, more suited for growth, exhibited the highest values. However, compared to other taxa, records about sponge growth and shrinkage rates in literature are scarce and limited to a few species (Ayling, 1983; Barthel, 1986; Hoppe, 1988; Garrabou and Zabala, 2001; Duckworth et al., 2007; De Caralt et al., 2010; Osinga et al., 2010; Zea et al., 2010; Page et al., 2011; Padiglia et al., 2018; Gökalp et al., 2019; Li et al., 2023). Moreover, within this deficiency of data, farmed sponge growth has been demonstrated to be species specific, highly variable [from negative growth (Hoppe, 1988; De Caralt et al., 2008; Page et al., 2011; Di Camillo et al., 2012), to 2000% increase per year (Pronzato and Manconi, 2008; Page et al., 2011; Schippers et al., 2012)] and easily influenced by several factors like seasonality, water temperature, water transparency and currents (Barthel and Theede, 1986). Thus, a clear picture of growth performance in relation with different farming techniques is not available (Corriero et al., 2004). The only study on Spongia anclotea was carried out by Oronti et al. (2012), which reported a growth rate of 6.2 cm3/month over a three year period. We recorded a better growth rate (11.28 cm3/month), but it should be considered that the smaller initial size of sponge fragments in Oronti et al. (2012) may have influenced the velocity of growth. Within the genus Spongia, Çelik et al. (2011) investigated line-farmed Spongia officinalis specimens and registered growth rates of 5-17% in two years. Our farming system of S. anclotea from Cape Eleuthera resulted in better performance. Environmental factors, like seasonal variations of water temperature, were demonstrated to potentially influence sponge growth. In locations with marked seasonality like temperate areas, growth is higher during the warmer season (Handley et al., 2003; Kelly et al., 2004; Page et al., 2005). Similarly, studies in Australia found a positive relationship between growth rates and temperature (Duckworth et al., 2007). Other research works, on the contrary, found no effect of seasonality on sponges’ growth (Ayling, 1983; Hoppe, 1988; Costa et al., 2015). Being the Bahamas in a semi-tropical area, the temperature range in our study area may be too limited to affect sponge growth rate significantly or S. anclotea may be one of those species which are not particularly affected by the variations of water conditions. However, during t3 - t4, a drop in growth was observed in some farm cells. At that time, a long cold spell took place at Cape Eleuthera, caused by the impact of Hurricane Sandy on the area, potentially slowing down the growth of sponges. Considering the scarcity of studies on sponge growth, our results could help to fill this gap in sponge science.
In aquaculture, another important issue is mortality of organisms since it means a complete loss of time and money invested. In our study, mortality was limited, only 54 out of 384 sponges died (or have been lost) during the study. Verdenal (1990) stated that a good farm should yield about 90% of survival of organisms per year. Our farming system accounted for a final 86% in a 3-year span, thus being in line with the standard. This result is noticeable if compared with survival rates reported in literature, which ranged from 0 to 100% of survival across different farming methodologies and species (Osinga, 2010; Gökalp et al., 2019, 2020; Bierwirth et al., 2022). Oronti et al. (2012) recorded a 12.5% mortality rate for S. anclotea in three years of farming. The higher mortality (14%) we observed may be due to the longer duration of our study. For S. officinalis, Çelik et al. (2011) reported a survival rate of 82-85% of specimen farmed with a similar line technique to the one we adopted. Due to the absence of existing literature data on S. anclotea, we were unable to determine if our results on growth and survival represent just the intrinsic growth rate of this species or if they are influenced by the farming methodology and location. In our farm, most of lost sponges were due to detach. Sponge death was observed only during the first 12-18 months after transplantation. We decided for scientific purposes to not add new sponges on blank spaces but, in a context of economic activities, it would be quite easy to fill the holes with new sponges collected in the area (sometimes detached sponges are still in the vicinity of the cells) and maintain the farm fully operational.
Another key factor for both growth and survival rates is the hydrodynamics of the area. Growth rates of farmed sponges were shown to increase with current flow (Wilkinson and Vacelet, 1979), which provides more food particles, until it becomes strong enough to cause physical damage to sponge tissues (Duckworth et al., 1997; Bannister et al., 2007). Duckworth (2003) found that individuals of Latrunculia wellingtonensis farmed in areas with strong water motion grew three times bigger compared to areas with weak currents. Conversely, in area where strong currents cause excessive suspension or where weak currents deposit large amounts of particles, sponges may be buried, with detrimental effects on their ability to filter water and, consequently, on their survival (Reiswig, 1971; Osinga et al., 2001; Gökalp et al., 2020). During our study, damage by strong currents and increased suspension caused by Hurricane Sandy could justify the weak growth observed during t3 – t4. Plus, the registered peak of Chl-a (most probably an algal bloom), may have additionally impaired the filtering capacity of sponges leading to an even stronger state of stress. Except for the potential acute impact of the hurricane, no other evidence of the effect of hydrodynamics were observed since sponge growth and mortality in different cell positions, and consequent different exposure to currents, did not show clear picture of potential interactions. This suggest, on one hand, that S. anclotea may be tolerant to average currents and suspension, and, at the same time, that the choice of the area (nearshore and not affected by strong currents and waves) and the design of the sponge farm were proficient for this species. Thus, this confirm that finding a proper location and plan a smart farm design is a key factor to maximize the overall efficiency and productivity.
The following step of this study was assessing the commercial quality of the farmed sponges. Experts from the Spugnificio Rosenfeld sponge farm of Muggia stated that most of the sponges were over the minimal commercial size already at time t4 and that about 80% of them were defined as first quality sponges, the remaining 20% as second quality. At t4, the overall estimated profit for farmers for 348 sponges was $264, while a standard stock of natural harvested sponges (usually made by 50-50 of 1st and 2nd quality sponges) is usually worth $348. On a longer time scale, 6 years, the profitability of our farm exceeded the standard stock at same sponge volume ($618 vs $547). It must be considered that the time needed to reach commercial size and the consequent t6 could be easily reduced by using initial fragments of larger size. Here, being a pilot study, we chose to obtain 3-4 small fragments for donor sponge. Just starting from fragments of double the size would cut the farming time in half with consequent economic benefits for producers and avoiding issues with mortality at smaller sizes.
As a conclusion, our results suggest this farming system as a valid alternative to harvested sponges since its profitability in the short/mid-term is higher. In addition to the economic and commercial aspects, our results are also significant from an ecological perspective since finding new sources of sponges, like aquaculture, could be a key tool to reduce stress on their populations. Furthermore, the presence of open-sea sponge farms, acting as high-density reproduction areas, could provide a significant amount of fragments, propagules and larvae for the surrounding regions, thus favoring the restocking of wild populations affected by fishing pressure and natural mortality events (Leong and Pawlik, 2010; Pawlik, 2011). On the other hand, long-term farming could increase the risk of confined in-situ reproduction with consequent genetic degradation over time impairing their fitness and survival (Pérez-Portela et al., 2014). Plus, the high density of individuals could favor the outbreak of sponge diseases, predators and parasites, as observed worldwide in different species and locations (Webster, 2007; Maldonado et al., 2010; Page et al., 2011; Wulff, 2012; Choudhury et al., 2015), that could then spread and harm wild populations.
Further studies are needed to test the efficiency of this farming technique in the long term both on S. anclotea and other commercially important sponge species. This would allow to obtain a clearer picture of all the potential benefits, both economic and ecological, or unexpected detrimental effects, on the ecosystems and economy of local communities all over the world.
The raw data supporting the conclusions of this article will be made available by the authors, without undue reservation.
The manuscript presents research on animals that do not require ethical approval for their study.
GM: Data curation, Formal Analysis, Writing – original draft, Writing – review & editing. RA: Conceptualization, Investigation, Writing – review & editing. AB: Conceptualization, Investigation, Writing – review & editing. MN: Writing – review & editing, Formal Analysis. EP: Writing – review & editing, Funding acquisition, Project administration, Supervision. AT: Writing – review & editing. MA: Conceptualization, Funding acquisition, Investigation, Project administration, Writing – review & editing.
The author(s) declare that financial support was received for the research, authorship, and/or publication of this article. This work was realized as a part of the research project funded by the Italian Ministry of University and Research PRIN-PNRR 2022 “Turning an environmental threat into an opportunity: the invasive sponge Paraleucilla magna as a source of ecofriendly antifouling agents (PARAFOULING). PRIN n. 2022R5JJFC - CUP: J53D23006700006. This study was partially supported either by the Spugnificio Rosenfeld, Muggia, Italy, and co-financed by the Friuli-Venezia Giulia Region in the years 2011-2012 (FERRERO-CTSPUGNE)
We wish to thank the Cape Eleuthera Island School-CEI and the Cape Eleuthera Institute, Rock Sound, Eleuthera, The Bahamas, which hosted the experimental farming and provided the facilities for its maintenance and control.
The authors declare that the research was conducted in the absence of any commercial or financial relationships that could be construed as a potential conflict of interest.
The author(s) declare that no Generative AI was used in the creation of this manuscript.
All claims expressed in this article are solely those of the authors and do not necessarily represent those of their affiliated organizations, or those of the publisher, the editors and the reviewers. Any product that may be evaluated in this article, or claim that may be made by its manufacturer, is not guaranteed or endorsed by the publisher.
The Supplementary Material for this article can be found online at: https://www.frontiersin.org/articles/10.3389/fmars.2025.1519832/full#supplementary-material
Abdelaleem E. R., Samy M. N., Desoukey S. Y., Liu M., Quinn R. J., Abdelmohsen U. R. (2020). Marine natural products from sponges (Porifera) of the order Dictyoceratida, (2013to 2019); a promising source for drug discovery. RSC Adv. 10, 34959–34976. doi: 10.1039/D0RA04408C
Aguilo-Arce J., Ferriol P., Trani R., Puthod P., Pierri C., Longo C. (2023). Sponges as emerging by-product of integrated multitrophic aquaculture (IMTA). J. Mar. Sci. Eng. 11, 80. doi: 10.3390/jmse11010080
Amato A., Esposito R., Federico S., Pozzolini M., Giovine M., Bertolino M., et al. (2024). Marine sponges as promising candidates for integrated aquaculture combining biomass increase and bioremediation: an updated review. Front. Mar. Sci. 10. doi: 10.3389/fmars.2023.1234225
Amigó M., Payá M., Braza-Boïls A., De Rosa S., Terencio M. C. (2008). Avarol inhibits TNF-α generation and NF-κB activation in human cells and in animal models. Life Sci. 82, 256–264. doi: 10.1016/j.lfs.2007.11.017
Arndt W. (1938). “Schwamme,” in Die Rohstoffe des Tierreichs 1, 2 Hälfte, Gebr. Eds. Arndt W., Pax F. (Borntraeger, Berlin), 1577–2000.
Ayling A. L. (1983). Growth and regeneration rates in thinly encrusting demospongiae from temperate waters. Biol. Bull. 165, 343–352. doi: 10.2307/1541200
Ayling A. M. (1981). The role of biological disturbance in temperate subtidal encrusting communities. Ecology 62, 830–847. doi: 10.2307/1937749
Bannister R. J., Brinkman R., Wolff C., Battershill C., De Nys R. (2007). The distribution and abundance of dictyoceratid sponges in relation to hydrodynamic features: identifying candidates and environmental conditions for sponge aquaculture. Mar. Freshw. Res. 58, 624. doi: 10.1071/MF07011
Barthel D. (1986). On the ecophysiology of the sponge Halichondria panicea in Kiel Bight. I. Substrate specificity, growth and reproduction. Mar. Ecol. Prog. Ser. 32, 291–298. doi: 10.3354/meps032291
Barthel D., Theede H. (1986). A new method for the culture of marine sponges and its application for experimental studies. Ophelia 25, 75–82. doi: 10.1080/00785326.1986.10429715
Bates D., Mächler M., Bolker B., Walker S. (2015). Fitting linear mixed-effects models using lme4. J. Stat. Software 67, 1–48. doi: 10.18637/jss.v067.i01
Belarbi E. (2003). Producing drugs from marine sponges. Biotechnol. Adv. 21, 585–598. doi: 10.1016/S0734-9750(03)00100-9
Bertolino M., Costa G., Carella M., Cattaneo-Vietti R., Cerrano C., Pansini M., et al. (2017). The dynamics of a Mediterranean coralligenous sponge assemblage at decennial and millennial temporal scales. PloS One 12, e0177945. doi: 10.1371/journal.pone.0177945
Betanzos-Vega A., Mazón-Suástegui J. M., Formoso-García M., Avilés-Quevedo M. A. (2019). “Sponge fishery and aquaculture in Cuba: impacts and challenges,” in Invertebrates - Ecophysiology and Management. Eds. Ray S., Diarte-Plata G., Escamilla-Montes R. (London, UK: IntechOpen), 103–117. doi: 10.5772/intechopen.84785
Bierwirth J., Mantas T. P., Villechanoux J., Cerrano C. (2022). Restoration of marine sponges—What can we learn from over a century of experimental cultivation? Water 14, 1055. doi: 10.3390/w14071055
Cahn A. R. (1948). Japanese sponge culture experiments in the South Pacific Islands, General Headquarters, Supreme Commander for the Allied Powers, National Resources Section. (Washington DC: U.S Fish and Wildlife Service, U.S Department of the Interior).
Cavolini F. (1785). Memorie per servire alla storia de’polipi marini (Napoli, Italy: Wentworth Press).
Cavolini F. (1853). Memorie postume sceverate dalle schede autografice di Filippo Cavolini per cura ed a spese di SD Chiaie. Tip. delle Streghe. (Benevento, Italy: Tipografia delle streghe).
Cebrian E., Uriz M.-J., Turon X. (2007). Sponges as biomonitors of heavy metals in spatial and temporal surveys in northwestern Mediterranean: multispecies comparison. Environ. Toxicol. Chem. Int. J. 26, 2430–2439. doi: 10.1897/07-292.1
Çelik İ., Cirik Ş., Altιnağaç U., Ayaz A., Çelik P., Tekeşoğlu H., et al. (2011). Growth performance of bath sponge (Spongia officinalis Linnaeus 1759) farmed on suspended ropes in the Dardanelles (Turkey): Growth performance of bath sponge (Spongia officinalis) in the Dardanelles (Turkey). Aquac. Res. 42, 1807–1815. doi: 10.1111/j.1365-2109.2010.02781.x
Choudhury J. D., Pramanik A., Webster N. S., Llewellyn L. E., Gachhui R., Mukherjee J. (2015). The pathogen of the Great Barrier Reef sponge Rhopaloeides odorabile is a new strain of Pseudoalteromonas agarivorans containing abundant and diverse virulence-related genes. Mar. Biotechnol. 17, 463–478. doi: 10.1007/s10126-015-9627-y
Coppock A. G., Kingsford M. J., Battershill C. N., Jones G. P. (2022). Significance of fish–sponge interactions in coral reef ecosystems. Coral Reefs 41, 1285–1308. doi: 10.1007/s00338-022-02253-8
Corriero G., Longo C., Mercurio M., Nonnis Marzano C., Lembo G., Spedicato M. T. (2004). Rearing performance of Spongia officinalis on suspended ropes off the Southern Italian Coast (Central Mediterranean Sea). Aquaculture 238, 195–205. doi: 10.1016/j.aquaculture.2004.04.030
Costa M. F. D. B., Mansur K. F. R., Leite F. P. P. (2015). Temporal variation of the gammaridean fauna (Crustacea, Amphipoda) associated with the sponge Mycale angulosa (Porifera, Demospongiae) in southeastern Brazil. Nauplius 23, 79–87. doi: 10.1590/S0104-64972015002312
Costello M. J., Myers A. A. (1987). Amphipod fauna of the sponges Halichondria panicea and Hymeniacidon perleve in Lough Hine, Ireland. Mar. Ecol. Prog. Ser. 41, 115–121. doi: 10.3354/meps041115
Croft R. A. (1990). Recommendations for Establishing a Commercial Sponge Industry Within the Pohnpei Region (Waimanalo, Hawaii: US Department of Agriculture, Center for Tropical and Subtropical Aquaculture).
Dayton P. K., Robilliard G. A., Paine R. T., Dayton L. B. (1974). Biological accommodation in the benthic community at McMurdo Sound, Antarctica. Ecol. Monogr. 44, 105–128. doi: 10.2307/1942321
De Caralt S., Sánchez-Fontenla J., Uriz M. J., Wijffels R. H. (2010). In situ aquaculture methods for Dysidea avara (Demospongiae, Porifera) in the Northwestern Mediterranean. Mar. Drugs 8, 1731–1742. doi: 10.3390/md8061731
De Caralt S., Uriz M. J., Wijffels R. H. (2008). Grazing, differential size-class dynamics and survival of the Mediterranean sponge Corticium candelabrum. Mar. Ecol. Prog. Ser. 360, 97–106. doi: 10.3354/meps07365
De Goeij J. M., Van Oevelen D., Vermeij M. J. A., Osinga R., Middelburg J. J., De Goeij A. F. P. M., et al. (2013). Surviving in a marine desert: the sponge loop retains resources within coral reefs. Science 342, 108–110. doi: 10.1126/science.1241981
de Voogd N., Alvarez B., Boury-Esnault N., Cárdenas P., Díaz M.-C., Dohrmann M., et al. (2024). World Porifera Database. doi: 10.14284/359
Di Camillo C., Coppari M., Bartolucci I., Bo M., Betti F., Bertolino M., et al. (2012). Temporal variations in growth and reproduction of Tedania anhelans and Chondrosia reniformis in the North Adriatic Sea. Anc. Anim. New Chall. Dev. Sponge Res. 219, 299–313. doi: 10.1007/s10750-011-0877-z
Duckworth A. (2001). Farming sponges for chemicals with pharmaceutical potential. World Aquac. Magazine 14, 16–18.
Duckworth A. R. (2003). Effect of wound size on the growth and regeneration of two temperate subtidal sponges. J. Exp. Mar. Biol. Ecol. 287, 139–153. doi: 10.1016/S0022-0981(02)00552-X
Duckworth A. (2009). Farming sponges to supply bioactive metabolites and bath sponges: A review. Mar. Biotechnol. 11, 669–679. doi: 10.1007/s10126-009-9213-2
Duckworth A., Battershill C. (2003). Sponge aquaculture for the production of biologically active metabolites: the influence of farming protocols and environment. Aquaculture 221, 311–329. doi: 10.1016/S0044-8486(03)00070-X
Duckworth A. R., Battershill C. N., Bergquist P. R. (1997). Influence of explant procedures and environmental factors on culture success of three sponges. Aquaculture 156, 251–267. doi: 10.1016/S0044-8486(97)00131-2
Duckworth A. R., Wolff C., Evans-Illidge E. (2007). “Developing methods for commercially farming bath sponges in tropical Australia,” in Porifera Res.-Biodivers. Innov. Sustain. Eds. Custodio M. R., Lobo-Hajdu G., Hajdu E., Muricy G. (Museu Nacional, Rio de Janeiro, Brazil).
Ehrlich H., Wysokowski M., Żółtowska-Aksamitowska S., Petrenko I., Jesionowski T. (2018). Collagens of Poriferan origin. Mar. Drugs 16, 79. doi: 10.3390/md16030079
FAO Fishery Information, D, Unit (FAO-FIDI), S. (2005). Collation, Analysis and Dissemination of Global and Regional Fishery Statistics. (Rome, Italy: Food and Agriculture Organization of the United Nations (FAO)).
Faulkner D., Hai-yin H., Unson M., Bewley C., Garson M. (1993). New metabolites from marine sponges: are symbionts important? Gazzetta Chim. Ital. 123, 301–307.
Friday S. (2011). A Study of Sponge Aquaculture in Jambiani: Is Shallow Farming Feasible? (Brattleboro, USA: Independent Study Project (ISP) Collection).
Garrabou J., Zabala M. (2001). Growth dynamics in four mediterranean demosponges. Estuar. Coast. Shelf Sci. 52, 293–303. doi: 10.1006/ecss.2000.0699
Giangrande A., Pierri C., Arduini D., Borghese J., Licciano M., Trani R., et al. (2020). An innovative IMTA system: Polychaetes, sponges and macroalgae co-cultured in a Southern Italian in-shore mariculture plant (Ionian Sea). J. Mar. Sci. Eng. 8, 733. doi: 10.3390/jmse8100733
Gökalp M., Kooistra T., Rocha M. S., Silva T. H., Osinga R., Murk A. J., et al. (2020). The effect of depth on the morphology, bacterial clearance, and respiration of the mediterranean sponge chondrosia reniformis (Nardo 1847). Mar. Drugs 18, 358. doi: 10.3390/md18070358
Gökalp M., Mes D., Nederlof M., Zhao H., Merijn de Goeij J., Osinga R. (2021). The potential roles of sponges in integrated mariculture. Rev. Aquac. 13, 1159–1171. doi: 10.1111/raq.12516
Gökalp M., Wijgerde T., Sarà A., De Goeij J. M., Osinga R. (2019). Development of an integrated mariculture for the collagen-rich sponge chondrosia reniformis. Mar. Drugs 17, 29. doi: 10.3390/md17010029
Goren L., Idan T., Shefer S., Ilan M. (2021). Sponge-associated polychaetes: not a random assemblage. Front. Mar. Sci. 8. doi: 10.3389/fmars.2021.695163
Handley S., Kelly S., Kelly M. (2003). Non-destructive video image analysis method for measuring growth in sponge farming: Preliminary results from the New Zealand bath-sponge Spongia (Heterofibria) manipulatus. N. Z. J. Mar. Freshw. Res. 37, 613–621. doi: 10.1080/00288330.2003.9517192
Hansen I. V., Weeks J. M., Depledge M. H. (1995). Accumulation of copper, zinc, cadmium and chromium by the marine sponge Halichondria panicea Pallas and the implications for biomonitoring. Mar. pollut. Bull. 31, 133–138. doi: 10.1016/0025-326X(94)00228-2
Herrnkind W. F., Butler M. J. IV, Hunt J. H., Childress M. (1997). Role of physical refugia: implications from a mass sponge die-off in a lobster nursery in Florida. Mar. Freshw. Res. 48, 759–770. doi: 10.1071/MF97193
Hoppe W. F. (1988). Growth, regeneration and predation in three species of large coral reef sponges. Mar. Ecol. Prog. Ser. Oldendorf 50, 117–125. doi: 10.3354/meps050117
Indraningrat A., Smidt H., Sipkema D. (2016). Bioprospecting sponge-associated microbes for antimicrobial compounds. Mar. Drugs 14, 87. doi: 10.3390/md14050087
Jesionowski T., Norman M., Żółtowska-Aksamitowska S., Petrenko I., Joseph Y., Ehrlich H. (2018). Marine spongin: naturally prefabricated 3D scaffold-based biomaterial. Mar. Drugs 16, 88. doi: 10.3390/md16030088
Kelly M., Handley S., Page M., Butterfield P., Hartill B., Kelly S. (2004). Aquaculture trials of the New Zealand bath-sponge Spongia (Heterofibria) manipulatus using lanterns. N. Z. J. Mar. Freshw. Res. 38, 231–241. doi: 10.1080/00288330.2004.9517233
Lenth R. V. (2023). emmeans: Estimated Marginal Means, aka Least-Squares Means. Available online at: https://CRAN.R-project.org/package=emmeans.
Leong W., Pawlik J. R. (2010). Fragments or propagules? Reproductive tradeoffs among Callyspongia spp. from Florida coral reefs. Oikos 119, 1417–1422. doi: 10.1111/j.1600-0706.2010.18092.x
Li Z., Xia G., Ou H., Chen M., You Y., Chen J. (2023). Large-scale mariculture of the sponge Haliclona simulans on floating rafts in Zhao’an Bay, Fujian Province, China. Aquaculture 563, 738893. doi: 10.1016/j.aquaculture.2022.738893
Lira A. L., de O., Craveiro N., Paresque K., Fukuda M. V., Rosa Filho J. S. (2024). Ecological review of the Syllidae (Annelida) associated with sponges (Porifera), including the description of a new species from northeastern Brazil. Ocean Coast. Res. 72, e24039. doi: 10.1590/2675-2824072.23117
Longo C., Cardone F., Corriero G., Licciano M., Pierri C., Stabili L. (2016). The co-occurrence of the demosponge Hymeniacidon perlevis and the edible mussel Mytilus galloprovincialis as a new tool for bacterial load mitigation in aquaculture. Environ. Sci. pollut. Res. 23, 3736–3746. doi: 10.1007/s11356-015-5587-z
Longo C., Scrascia M., Trani R., Pierri C., Cariglia A., Cariglia F., et al. (2020). “Assesment of sponge mariculture potential in polyculture system in Manfredonia Gulf toward the IMTA implementation,” in Proceedings of the Aquafarm Novelfarm, Pordenone, Italy, 19–20 February 2020.
Louden D., Inderbitzin S., Peng Z., De Nys R. (2007). Development of a new protocol for testing bath sponge quality. Aquaculture 271, 275–285. doi: 10.1016/j.aquaculture.2007.06.010
Maldonado M., Aguilar R., Bannister R. J., Bell J. J., Conway K. W., Dayton P. K., et al. (2017). “Sponge grounds as key marine habitats: A synthetic review of types, structure, functional roles, and conservation concerns,” in Marine Animal Forests: The Ecology of Benthic Biodiversity Hotspots. Eds. Rossi S., Bramanti L., Gori A., Orejas Saco del Valle C. (Springer International Publishing, Cham), 1–39. doi: 10.1007/978-3-319-17001-5_24-1
Maldonado M., Ribes M., Van Duyl F. C. (2012). “Nutrient fluxes through sponges,” in Advances in Marine Biology (Elsevier), 113–182. doi: 10.1016/B978-0-12-394283-8.00003-5
Maldonado M., Sánchez-Tocino L., Navarro C. (2010). Recurrent disease outbreaks in corneous demosponges of the genus Ircinia: epidemic incidence and defense mechanisms. Mar. Biol. 157, 1577–1590. doi: 10.1007/s00227-010-1431-7
Martin D., Britayev T. A. (2018). “Symbiotic polychaetes revisited: an update of the known species and relationships, (1998–2017),” in Oceanography and marine Biology (Boca Raton, USA: CRC Press), 371–447. doi: 10.1201/9780429454455-6
Maslin M., Gaertner-Mazouni N., Debitus C., Joy N., Ho R. (2021). Marine sponge aquaculture towards drug development: An ongoing history of technical, ecological, chemical considerations and challenges. Aquac. Rep. 21, 100813. doi: 10.1016/j.aqrep.2021.100813
Milanese M., Chelossi E., Manconi R., Sara A., Sidri M., Pronzato R. (2003). The marine sponge Chondrilla nucula Schmidt 1862 as an elective candidate for bioremediation in integrated aquaculture. Biomol. Eng. 20, 363–368. doi: 10.1016/s1389-0344(03)00052-2
Mohite S., Mahakal B., Bhatkar H., Patil S. (2020). Culture of marine sponges under controlled conditions. J. Exp. Zool. India. 23, 753–757.
Olesen T., Weeks J. (1994). Accumulation of Cd by the marine sponge Halichondria panicea Pallas: effects upon filtration rate and its relevance for biomonitoring. Bull. Environ. Contam. Toxicol. 52, 722–728. doi: 10.1007/BF00195494
Orani A. M., Barats A., Zitte W., Morrow C., Thomas O. P. (2018). Comparative study on the bioaccumulation and biotransformation of arsenic by some northeastern Atlantic and northwestern Mediterranean sponges. Chemosphere 201, 826–839. doi: 10.1016/j.chemosphere.2018.03.078
Oronti A., Danylchuk A. J., Elmore C. E., Auriemma R., Pesle G. (2012). Assessing the feasibility of sponge aquaculture as a sustainable industry in The Bahamas. Aquac. Int. 20, 295–303. doi: 10.1007/s10499-011-9457-5
Osinga R. (2010). Diet Composition of Two Temperate Calcareous Sponges: Leucosolenia eChinata and Leucetta sp. from the Wellington South Coast, New Zealand. Open Mar. Biol. J. 4, 74–81. doi: 10.2174/1874450801004010074
Osinga R., Kleijn R., Groenendijk E., Niesink P., Tramper J., Wijffels R. H. (2001). Development of In Vivo Sponge Cultures: Particle Feeding by the Tropical Sponge Pseudosuberites aff. andrewsi. Mar. Biotechnol. 3, 0544–0554. doi: 10.1007/s1012601-0078-2
Osinga R., Sidri M., Cerig E., Gokalp S. Z., Gokalp M. (2010). Sponge aquaculture trials in the East-Mediterranean Sea: new approaches to earlier ideas. Open Mar. Biol. J. 4, 74–81. doi: 10.2174/1874450801004010074
Osinga R., Tramper J., Wijffels R. H. (1999). Cultivation of marine sponges. Mar. Biotechnol. 1, 509–532. doi: 10.1007/PL00011807
Ou H., Zhai J., Wang D., Zhao J., Chen M., Ding S., et al. (2020). Cultivation of sponge Haliclona simulans juveniles in a floating sea raft. Aquaculture 529, 735660. doi: 10.1016/j.aquaculture.2020.735660
Padiglia A., Ledda F. D., Padedda B. M., Pronzato R., Manconi R. (2018). Long-term experimental in situ farming of Crambe crambe (Demospongiae: Poecilosclerida). PeerJ 6, e4964. doi: 10.7717/peerj.4964
Page M. J., Handley S. J., Northcote P. T., Cairney D., Willan R. C. (2011). Successes and pitfalls of the aquaculture of the sponge Mycale hentscheli. Aquaculture 312, 52–61. doi: 10.1016/j.aquaculture.2010.12.006
Page M. J., Northcote P. T., Webb V. L., Mackey S., Handley S. J. (2005). Aquaculture trials for the production of biologically active metabolites in the New Zealand sponge Mycale hentscheli (Demospongiae: Poecilosclerida). Aquaculture 250, 256–269. doi: 10.1016/j.aquaculture.2005.04.069
Patel B., Balani M., Patel S. (1985). Sponge ‘sentinel’of heavy metals. Sci. Total Environ. 41, 143–152. doi: 10.1016/0048-9697(85)90184-6
Pawlik J. R. (2011). The chemical ecology of sponges on Caribbean reefs: natural products shape natural systems. BioScience 61, 888–898. doi: 10.1525/bio.2011.61.11.8
Pawlik J. R., McMurray S. E. (2020). The emerging ecological and biogeochemical importance of sponges on coral reefs. Annu. Rev. Mar. Sci. 12, 315–337. doi: 10.1146/annurev-marine-010419-010807
Perez T., Longet D., Schembri T., Rebouillon P., Vacelet J. (2005). Effects of 12 years’ operation of a sewage treatment plant on trace metal occurrence within a Mediterranean commercial sponge (Spongia officinalis, Demospongiae). Mar. pollut. Bull. 50, 301–309. doi: 10.1016/j.marpolbul.2004.11.001
Perez T., Sarrazin L., Rebouillon P., Vacelet J. (2002). First evidences of surfactant biodegradation by marine sponges (Porifera): an experimental study with a linear alkylbenzenesulfonate. Hydrobiologia 489, 225–233. doi: 10.1023/A:1023217218585
Perez T., Wafo E., Fourt M., Vacelet J. (2003). Marine sponges as biomonitor of polychlorobiphenyl contamination: concentration and fate of 24 congeners. Environ. Sci. Technol. 37, 2152–2158. doi: 10.1021/es026234v
Pérez-Portela R., Noyer C., Becerro M. (2014). Genetic structure and diversity of the endangered bath sponge Spongia lamella. Aquat. Conserv. Mar. Freshw. Ecosyst. 25, 365–379. doi: 10.1002/aqc2423
Philp R. (1999). Cadmium content of the marine sponge Microciona prolifera, other sponges, water and sediment from the eastern Florida panhandle: possible effects on Microciona cell aggregation and potential roles of low pH and low salinity. Comp. Biochem. Physiol. C Pharmacol. Toxicol. Endocrinol. 124, 41–49. doi: 10.1016/S0742-8413(99)00046-8
Pronzato R. (2003). Mediterranean Sponge Fauna: a biological, historical and cultural heritage. Biogeogr. – J. Integr. Biogeogr. 24, 91–99. doi: 10.21426/B6110118
Pronzato R., Manconi R. (2008). Mediterranean commercial sponges: over 5000 years of natural history and cultural heritage. Mar. Ecol. 29, 146–166. doi: 10.1111/j.1439-0485.2008.00235.x
R Core Team (2021). R: A Language and Environment for Statistical Computing (Vienna, Austria: R Foundation for Statistical Computing). Available at: https://www.R-project.org/.
Reiswig H. M. (1971). Particle feeding in natural populations of three marine demosponges. Biol. Bull. 141, 568–591. doi: 10.2307/1540270
Santiago V. S., Manzano G. G., Yu C. C., Aliño P. M., Salvador-Reyes L. A. (2019). Mariculture potential of renieramycin-producing Philippine blue sponge Xestospongia sp. (Porifera: Haplosclerida). Aquaculture 502, 356–364. doi: 10.1016/j.aquaculture.2018.12.059
Santos-Gandelman J. F., Cruz K., Crane S., Muricy G., Giambiagi-deMarval M., Barkay T., et al. (2014). Potential application in mercury bioremediation of a marine sponge-isolated Bacillus cereus strain Pj1. Curr. Microbiol. 69, 374–380. doi: 10.1007/s00284-014-0597-5
Schippers K. J., Sipkema D., Osinga R., Smidt H., Pomponi S. A., Martens D. E., et al. (2012). Cultivation of sponges, sponge cells and symbionts: achievements and future prospects. Adv. Mar. Biol. 62, 273–337. doi: 10.1016/B978-0-12-394283-8.00006-0
Sella M. (1912). La pesca delle Spugne nella Libia Vol. 13 (Venezia: Memorie del Regio Comitato Talassografico Italiano), 1–153.
Storr J. F. (1957). The sponge industry of Florida (Miami, USA: State of Florida, Board of Conservation). Educational Series No. 9.
Storr J. F. (1964). Ecology of the Gulf of Mexico commercial sponges and its relation to the fishery (United States Fish and Wildlife Service). Special Scientific Report-Fisheries No. 466.
Tang J., Wu W., Yang F., Liu L., Yang Z., Liu L., et al. (2018). Marine sponge-derived smenospongine preferentially eliminates breast cancer stem-like cells via p38/AMPK α pathways. Cancer Med. 7, 3965–3976. doi: 10.1002/cam4.1640
Tang W.-Z., Zhao H.-M., Tian Y., Dai S.-W., Zhang A., Lin H.-W., et al. (2022). Merosesquiterpenes from the marine sponge Spongia pertusa Esper and their antifungal activities. Tetrahedron Lett. 93, 153690. doi: 10.1016/j.tetlet.2022.153690
Tian X.-H., Hong L.-L., Jiao W.-H., Lin H.-W. (2023). Natural sesquiterpene quinone/quinols: Chemistry, biological activity, and synthesis. Nat. Prod. Rep. 40, 718–749. doi: 10.1039/D2NP00045H
Verdenal B. (1990). Sponge culture on vertical ropes in the Northwestern Mediterranean Sea. New Perspect. Sponge Biol. 1, 416–424.
Verdenal B., Verdenal M. (1987). Evaluation de l’intérêt economique de la culture d’eponges commerciales sur les côtes méditerranéennes françaises. Aquaculture 64, 9–29. doi: 10.1016/0044-8486(87)90202-X
Webster N. S. (2007). Sponge disease: a global threat? Environ. Microbiol. 9, 1363–1375. doi: 10.1111/j.1462-2920.2007.01303.x
Westinga E., Hoetjes P. (1981). The intrasponge fauna of Spheciospongia vesparia (Porifera, Demospongiae) at Curaçao and Bonaire. Mar. Biol. 62, 139–150. doi: 10.1007/BF00388176
Wilkinson C. R., Vacelet J. (1979). Transplantation of marine sponges to different conditions of light and current. J. Exp. Mar. Biol. Ecol. 37, 91–104. doi: 10.1016/0022-0981(79)90028-5
Wörheide G., Dohrmann M., Erpenbeck D., Larroux C., Maldonado M., Voigt O., et al. (2012). “Chapter one - deep phylogeny and evolution of sponges (Phylum porifera),” in Advances in Sponge Science: Phylogeny, Systematics, Ecology. Eds. Becerro M. A., Uriz M. J., Maldonado M., Turon X. (Academic Press), 1–78. doi: 10.1016/B978-0-12-387787-1.00007-6
Wulff J. (2006). Ecological interactions of marine sponges. Can. J. Zool. 84, 146–166. doi: 10.1139/Z06-019
Wulff J. (2012). “Chapter four - ecological interactions and the distribution, abundance, and diversity of sponges,” in Advances in Sponge Science: Phylogeny, Systematics, Ecology. Eds. Becerro M. A., Uriz M. J., Maldonado M., Turon X. (Academic Press), 273–344. doi: 10.1016/B978-0-12-387787-1.00003-9
Yi Q., Wei Z., Hua L., Xingju Y., Meifang J. (2005). Cultivation of marine sponges. Chin. J. Oceanol. Limnol. 23, 194–198. doi: 10.1007/BF02894238
Keywords: Spongia anclotea, Spongia pertusa, sponge farming, aquaculture, growth, survival, Bahamas
Citation: Motta G, Auriemma R, Brooks A, Novak M, Pesle E, Terlizzi A and Avian M (2025) A cheap and efficient system for Spongia anclotea farming in the Bahamas. Front. Mar. Sci. 12:1519832. doi: 10.3389/fmars.2025.1519832
Received: 30 October 2024; Accepted: 21 February 2025;
Published: 11 March 2025.
Edited by:
Keshuai Li, BioMar (Norway), NorwayReviewed by:
Zhiyong Li, Shanghai Jiao Tong University, ChinaCopyright © 2025 Motta, Auriemma, Brooks, Novak, Pesle, Terlizzi and Avian. This is an open-access article distributed under the terms of the Creative Commons Attribution License (CC BY). The use, distribution or reproduction in other forums is permitted, provided the original author(s) and the copyright owner(s) are credited and that the original publication in this journal is cited, in accordance with accepted academic practice. No use, distribution or reproduction is permitted which does not comply with these terms.
*Correspondence: Gregorio Motta, Z3JlZ29yaW8ubW90dGFAcGhkLnVuaXRzLml0
Disclaimer: All claims expressed in this article are solely those of the authors and do not necessarily represent those of their affiliated organizations, or those of the publisher, the editors and the reviewers. Any product that may be evaluated in this article or claim that may be made by its manufacturer is not guaranteed or endorsed by the publisher.
Research integrity at Frontiers
Learn more about the work of our research integrity team to safeguard the quality of each article we publish.