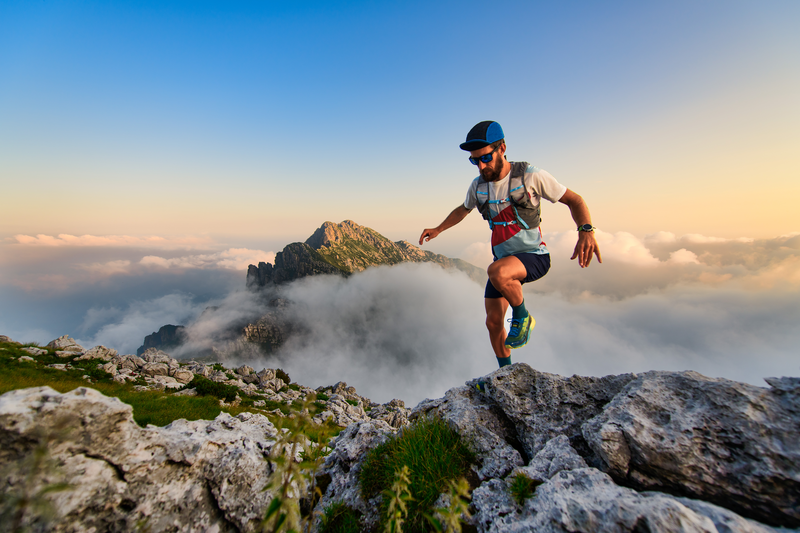
94% of researchers rate our articles as excellent or good
Learn more about the work of our research integrity team to safeguard the quality of each article we publish.
Find out more
METHODS article
Front. Mar. Sci.
Sec. Marine Conservation and Sustainability
Volume 12 - 2025 | doi: 10.3389/fmars.2025.1518562
The final, formatted version of the article will be published soon.
You have multiple emails registered with Frontiers:
Please enter your email address:
If you already have an account, please login
You don't have a Frontiers account ? You can register here
The decline in eel resources, coupled with the challenges of morphological identification and the rise of illegal trade, highlights the urgent need for accurate species identification techniques. To address this, a multiplex PCR assay was developed, targeting the mitochondrial Cytochrome b gene. Speciesspecific primers were designed and their efficacy validated through single PCR. The multiplex PCR conditions were then optimized to enable the simultaneous amplification of five major eel species. This assay exhibited high accuracy, specificity, and sensitivity, successfully identifying all five species even when DNA concentrations were low. This multiplex PCR assay offers a rapid and cost-effective solution for eel species identification, with the potential to significantly bolster eel conservation efforts. By enabling accurate species identification, it can help combat illegal trade and support the sustainable management of eel resources.
Keywords: Anguilla, multiplex PCR, Species identification, cytochrome b, Species-specific markers
Received: 28 Oct 2024; Accepted: 03 Mar 2025.
Copyright: © 2025 Noh, Dong, Park, Kim, Jung, Kong and Kim. This is an open-access article distributed under the terms of the Creative Commons Attribution License (CC BY). The use, distribution or reproduction in other forums is permitted, provided the original author(s) or licensor are credited and that the original publication in this journal is cited, in accordance with accepted academic practice. No use, distribution or reproduction is permitted which does not comply with these terms.
* Correspondence:
Eun Soo Noh, National Institute of Fisheries Science (NIFS), Busan, Republic of Korea
Disclaimer: All claims expressed in this article are solely those of the authors and do not necessarily represent those of their affiliated organizations, or those of the publisher, the editors and the reviewers. Any product that may be evaluated in this article or claim that may be made by its manufacturer is not guaranteed or endorsed by the publisher.
Research integrity at Frontiers
Learn more about the work of our research integrity team to safeguard the quality of each article we publish.