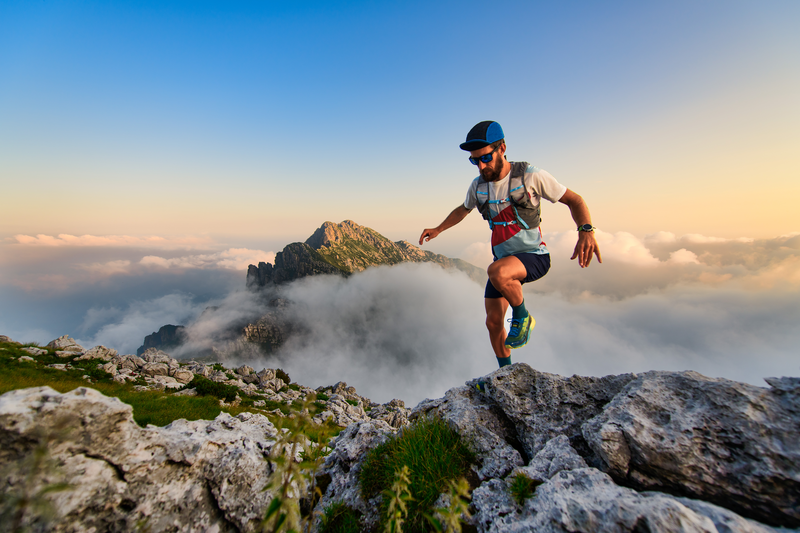
95% of researchers rate our articles as excellent or good
Learn more about the work of our research integrity team to safeguard the quality of each article we publish.
Find out more
ORIGINAL RESEARCH article
Front. Mar. Sci. , 24 March 2025
Sec. Marine Ecosystem Ecology
Volume 12 - 2025 | https://doi.org/10.3389/fmars.2025.1511921
This article is part of the Research Topic Bridging Knowledge Gaps in Marine Biological Invasions View all 7 articles
Introduction: The growing blue economy and rapid development and urbanisation of coastal areas drive an increase in marine infrastructures. These structures are built with artificial materials and the submerged parts thereof become substrates for colonisation by fouling biota which are often dominated by invasive alien species. However, knowledge on the ecological implications of artificial substrate material for marine biota remains limited, with a notable research gap with respect to Africa.
Methods: This field study assessed how artificial substrate material type might influence sessile fouling communities along the South African east coast. Fiberglass, High-Density Polyethylene (HDPE) and Polyvinyl Chloride (PVC) panels were used as artificial substrates for biofouling community settlement over a period of three months.
Results: Differences in artificial substrate material type influenced sessile fouling community structure, with fiberglass panels showing a dominant effect than the other two types of materials. Fiberglass panels also supported higher species diversity and percentage cover than the HDPE and PVC panels. Notably, no significant effect of species status (invasive and native) was detected on overall percentage cover.
Discussion: These results highlight the dominant influence of fiberglass materials on sessile assemblages. Overall, this study suggests that the type of material used in artificial marine structures may have ecological implications and therefore, should be included as an important consideration in material selection criteria.
The growing blue economy, urbanisation and increasing human population in coastal areas drive the proliferation of man-made infrastructures in marine environments (Dafforn et al., 2015; Firth et al., 2016a; Dodds et al., 2022). These structures can include seawalls, docks or jetties, buoys, marinas, ports, and/or ripraps or breakwaters made of artificial materials such as concrete, plastics or metals (Sempere-Valverde et al., 2018). With the growing population and related activities, artificial infrastructure is rapidly destroying and fragmenting natural habitats (Bishop et al., 2017), but also providing surfaces for colonisation by distinct marine assemblages (Mayer-Pinto et al., 2017). These changes could alter the connectivity of the seascape at local to regional scales, and ultimately, impair the structure and functioning of marine ecosystems (Bulleri and Chapman, 2010; Mayer-Pinto et al., 2018; Porter et al., 2018).
The literature on the ecological implications of artificial and natural structures show that benthic communities that recruit and develop on artificial substrata are different from those on nearby natural reefs, often displaying relatively lower coverage, species richness and diversity (Moschella et al., 2005; Tyrrell and Byers, 2007; Pister, 2009; Bulleri and Chapman, 2010; Sedano et al., 2019; Dodds et al., 2022). This is because substrates built from artificial materials commonly differ from natural substrata in terms of colour (Lathlean and Minchinton, 2012; Dobretsov et al., 2013; Ells et al., 2016), surface chemistry (Anderson, 1996; Sella and Perkol Finkel, 2015), chemical composition and physical properties such as microtexture and porosity (Bulleri and Chapman, 2010; Terlizzi and Faimali, 2010; Loke and Todd, 2016). For example, artificial substrate materials made from concrete, depending on its composition, could have a higher pH than seawater (Perkol-Finkel and Sella, 2014). Certain concrete formulations can leach heavy metals into the surrounding water column (McManus et al., 2018). Moreover, concrete substrate types are less heterogenous in microtexture than wood and natural rock (Coombes et al., 2015). All these factors could have implications for colonising marine biota by influencing species richness and abundance and ultimately, altering community structure (Hsiung et al., 2020; Dodds et al., 2022; Grasselli et al., 2024). Recently, there has been considerable interest in biocompatible concretes, which aim to reduce environmental impact and enhance sustainability (Gowell et al., 2015; Chlayon et al., 2018; Pioch et al., 2018; Hayek et al., 2022). This area is growing rapidly and represents a significant commercial and research focus (Gowell et al., 2015; Chlayon et al., 2018; Pioch et al., 2018; Hayek et al., 2022). Besides concrete, artificial structures as substrate types are generally less likely to offer refuge from adverse abiotic and biotic conditions compared to natural substrates (Firth et al., 2013; Loke et al., 2015), especially in intertidal environments (Ostalé-Valriberas et al., 2018). Therefore, there is a need to comprehensively investigate and understand the implications of structures made from artificial materials for marine biota to contribute data towards exploring avenues for adopting infrastructures with less ecological impacts.
Studies use different types of man-made materials to investigate the influence of artificial substrata on marine biota. These often include acrylic, High-Density Polyethylene (HDPE), Polyvinyl Chloride (PVC), fiberglass, steel, rubber, metal and concrete (Dodds et al., 2022; Grasselli et al., 2024). While most of these materials are used to construct marine infrastructures, concrete is by far the most ubiquitous (Bhattacharyya and Deb, 2022). Moreover, fiberglass and steel are also commonly used to construct small boats and recreational yachts (Sakinah et al., 2023; Ciocan et al., 2024) while concrete, acrylic, HDPE and PVC are widely used as artificial substrates in biofouling studies (Tyrrell and Byers, 2007; Dobretsov, 2015; Chase et al., 2016; Pinochet et al., 2020; Loureiro et al., 2021a; Dodds et al., 2022; Jewett et al., 2022; Grasselli et al., 2024).
Because artificial substrates are constructed from different types of materials as highlighted above, they exhibit differences in physico-chemical properties, which influence marine biota. Varying substratum characteristics include differences in material type, roughness, wettability (water adsorption to surface), thermal capacity or colour (Harlin and Lindbergh, 1977; Jones and Boulding, 1999; Osborn, 2005; Finlay et al., 2008; Coombes, 2011; Firth et al., 2016b; Sempere-Valverde et al., 2018; Grasselli et al., 2024). In terms of artificial material type, composite substrates such as fiberglass and concrete have higher wettability which could allow higher settlement of biota (Dugan et al., 2012; Dyer, 2014; Hsiung et al., 2020; Rubino et al., 2020) when compared to hydrophobic polymeric materials with low wettability including HDPE and PVC (Encinas et al., 2010; Dhandapani et al., 2024). Complex micro-topographies in rough artificial substrates enhance the turbulence of water near the surface, which in turn increases larvae transport to the substratum (Koehl, 2007). This results in higher spore and larval settlement on rough than smooth substrata (Koehl, 2007; Sempere-Valverde et al., 2018). Moreover, elevated surface water retention due to holes and crevices on rough substrates provide cool temperature conditions, lessening the likelihood of desiccation during low tides (Jones and Boulding, 1999; Coombes, 2011; Firth et al., 2016b). Finally, some studies on artificial substratum colour have shown a dominant effect of brightness on ascidian settlement densities (Ells et al., 2016), while others have reported higher densities of biofouling on black than white panels (Swain et al., 2006; Dobretsov et al., 2013; Nall et al., 2022) or no effect of colour on marine invertebrate larval settlement (Schaefer et al., 2024), thereby highlighting context dependency in terms of the effect of colour.
Of marine biota, sessile fouling communities, including algae and basibionts, and often dominated by invasive alien species, are particularly more likely to be influenced by varying artificial substrate types than vagile taxa (Grasselli et al., 2024). This is because the selection of microhabitat by sessile organisms is almost irreversible after metamorphosis, which influences post-settlement community composition (Osborn, 2003). Indeed, studies show that differences in artificial substrate types and overall substratum characteristics can alter fouling community structure by affecting diversity and species abundance (Anderson and Underwood, 1994; Tyrrell and Byers, 2007; Albano and Obenat, 2019; Muthukrishnan et al., 2019) but see Hartanto et al. (2022) who found no effect on macrofaunal species richness, total abundance and community composition. Effects can occur from the initial colonisation of fouling organisms to the development of communities over time (Anderson and Underwood, 1994; Albano and Obenat, 2019). As such, the measure of the density of artificial structures, coupled with their properties, over a gradient of human coastal modification can be used as a predictor for the abundance of species and fouling community diversity (Susick et al., 2020). However, in most regions of the world information about the effects of variable artificial substrates on fouling communities remains limited, particularly in Africa.
In South Africa, the distribution and abundance of fouling taxa along the coast have been assessed, with communities dominated by invasive alien species in harbours and marinas (Peters et al., 2014, 2017, 2019; Loureiro et al., 2021a). These fouling communities are regulated by light and predation (Loureiro et al., 2021b) and are vulnerable to cooling temperatures and ocean acidification (Matikinca and Robinson, 2024a). Moreover, fouling taxa from this coast also show limited tolerance to decreasing pH at different life stages under cooling and restricted food supply conditions (Matikinca and Robinson, 2024b). On the substrate materials side, fiberglass is a dominant material in small boats and recreational yachts, which are important regional vectors for the transfer of alien fouling species in South Africa (Peters et al., 2017, 2019). On the other hand, HDPE is one of the most abundant plastic materials in South Africa’s coastal waters and its buoyancy is known to be affected by biofouling (Fazey and Ryan, 2016; Ryan, 2020). Lastly, PVC is a common artificial substrate that has been used by various studies on biofouling dynamics along the South African coast (Peters et al., 2014, 2017, 2019; Loureiro et al., 2021a; Matikinca and Robinson, 2024a). However, notwithstanding the above, no research has considered how these different artificial substrates might simultaneously influence sessile fouling communities along this coast. Previous studies in South Africa have only used PVC panels to collect or monitor biofouling communities. Whether and how assemblages on these panels might compare with those on other artificial substrates, such as fiberglass and HDPE, remains unexplored.
This field study experimentally investigated the implications of artificial substrate material type for sessile fouling community dynamics along the South African east coast. Using fiberglass, HDPE and PVC panels, we tested the hypothesis that differences in artificial substrate material type would influence sessile fouling assemblages by altering community structure and species diversity. Due to the differences in physico-chemical properties of the three substrate material types, we predicted that fiberglass panels might show a dominant influence on sessile fouling community composition than HDPE and PVC panels.
The study was conducted at Point Yacht Club (29°51’48” S, 31°01’27” E) on the east coast of South Africa (Figure 1). During the austral Spring of 2023, nine experimental arrays with a total of 27 panels were deployed from floating jetties for three months (September – November) in the marina to collect fouling assemblages. Each array was assembled by fixing three panels (15 x 15 x 0.25 cm) (one fiberglass, one HDPE, and one PVC) perpendicular to a rope and suspended at a depth of 2 m below the water surface (Figure 2). This depth was chosen such that the distance between the panels and water surface was unaffected by tides (Schaefer et al., 2024) and is within the depth range covered by biofouling studies along the South African coast (Peters et al., 2017; Robinson et al., 2017; Loureiro et al., 2021a, b). As the jetties were floating structures, depth remained constant (Loureiro et al., 2021b). Moreover, all arrays were weighted to stabilise the panels and prevent them from posing a safety risk to vessels (Loureiro et al., 2021a). In South Africa, sessile fouling organisms grow facing downward on the underside of the panels (Peters et al., 2017; Robinson et al., 2017; Loureiro et al., 2021a, b). Therefore, the orientation of the panels used in this study followed the same methods as previous studies in this region (Peters et al., 2017; Robinson et al., 2017; Loureiro et al., 2021a, b). This orientation has also been used by studies from other regions such that the experimental surface faces downward parallel to the seafloor to mimic floating jetties (Crooks et al., 2011; Marraffini et al., 2017; Jewett et al., 2022). The panels were randomly attached to a Tremnet/plastic net on each array (Chase et al., 2015) to ensure that they remained in the same position relative to one another. A distance of at least 2 m was maintained between the arrays. Before the experiment, the panels were sanded and soaked in distilled water for 10 days to allow chemicals to leach (Loureiro et al., 2021a).
Figure 1. Map of the study site along the South African east coast where experimental arrays were deployed to monitor fouling communities. The map was adopted and edited with permission from Loureiro et al. (2021a).
Figure 2. Schematic illustration of the experimental setup to monitor fouling biota using fiberglass (FB), High-Density Polyethylene (HDPE) and Polyvinyl Chloride (PVC) panels. not drawn to scale.
The underside of the panels was photographed using a Canon PowerShot G7 X Mark III camera at the end of the experiment. The identification of specimens was made from the photographs. Specimens whose identification was uncertain were preserved and returned to the laboratory for microscopic analysis. Identifications were carried out to the lowest taxonomic level possible using Darwin (1854); Day (1967); Monniot et al. (2001); Smith and Gordon (2011) and Rocha et al. (2012). Community composition of sessile assemblages was quantified in terms of percentage cover and assessed using 100-point counts generated by overlaying a standardized grid onto the photographs.
A PERMANOVA was conducted to assess the influence of substrate material type on sessile fouling community structure. A square root transformation was applied to the non-standardized data to decrease the influence of the dominant species (Clarke and Warwick, 2001) and all multivariate analyses were underpinned by Bray-Curtis similarities (Bray and Curtis, 1957; Clarke and Warwick, 2001). PERMANOVA routines were based on 9999 permutations and a type III sum of squares. PERMDISP, based on the average distance from the centroid, was used to assess dispersion among the panels within treatments. A SIMPER analysis (with a cut-off of 90%) was performed to identify the species responsible for dissimilarities detected in community structure. All multivariate community analyses were conducted using PRIMER 6.0 (Clarke and Gorley, 2006; Anderson et al., 2008).
All univariate analyses were conducted using R version 4.4.0 (R Core Team, 2021). Before the analyses, the data were assessed for normality and homogeneity of variances using Shapiro-Wilk and Levene’s tests, respectively (Levene, 1960; Shapiro and Wilk, 1965). A Kruskal-Wallis test was used to assess the influence of substrate material type on the abundance of species responsible for the dissimilarities in community structure (Kruskal and Wallis, 1952). The Shannon-Wiener diversity index (H’) which includes both species richness and evenness (Clarke and Warwick, 1994), was used to assess the effect of substrate material type on diversity through the Kruskal-Wallis test. Likewise, the influence of substrate material type on species richness was also evaluated using the Kruskal-Wallis test. All pairwise comparisons for the Kruskal-Wallis analyses were conducted using Dunn’s test based on the Bonferroni adjustment method (Dunn, 1961, 1964). Finally, generalized least squares models (GLS) were conducted using the nlme package (Pinheiro and Bates, 2000) to assess the effect of substrate material type and species status (i.e. invasive and native) on the overall percentage cover.
A total of seven sessile taxa were identified on the settlement panels (Table 1). The sessile assemblage composition of fouling significantly differed according to the substrate material type (PERMANOVA: F = 7.40, P < 0.001; Figure 3). Sessile assemblages on High-Density Polyethylene (HDPE) and Polyvinyl Chloride (PVC) panels differed from those on fiberglass panels (HDPE vs fiberglass: t = 3.27, P < 0.001; PVC vs fiberglass: t = 3.42, P < 0.001). No significant differences were detected in community structure between HDPE and PVC panels (t = 1.00, P = 0.40). The differences observed between assemblages on the different materials were not caused by differences in dispersion (PERMDISP: F = 1.78, P = 0.19).
Table 1. Species recorded at Point Yacht Club on High-Density Polyethylene (HDPE), Polyvinyl Chloride (PVC), and fiberglass panels.
Figure 3. Two-dimensional multidimensional scaling plot based on standardised square root-transformed percentage cover of fouling communities at Point Yacht Club on the east coast of South Africa. The different symbols represent artificial substrate material types including High-Density Polyethylene (HDPE), Polyvinyl Chloride (PVC) and fiberglass (FB) panels.
The SIMPER analysis showed that the differences detected between the types of substrate material were driven by the invasive ascidians Diplosoma listerianum, Styela plicata, the invasive bryozoans Watersipora subtorquata, Bugula neritina, the native bryozoan Menipea triseriata, the native barnacle Amphibalanus amphitrite, and the native polychaete Spirorbis sp. (Table 2). These species contributed 42% dissimilarity between PVC and fiberglass communities, 38% between HDPE and fiberglass, and 29% between PVC and HDPE. All these species differed significantly in percentage cover among the different types of substrate material except for W. subtorquata (Figure 4; Tables 3, 4). They displayed higher abundance on fiberglass panels than on the other two material types (P < 0.05). No significant differences in percentage cover were detected between HDPE and PVC panels for any of the species except for S. plicata, which showed a significantly higher abundance on PVC than HDPE panels (P < 0.05).
Table 2. Results of SIMPER analyses based on Bray–Curtis dissimilarity of square root transformed species percentage cover (%C) among artificial substrate material types including High-Density Polyethylene (HDPE), Polyvinyl Chloride (PVC) and fiberglass panels.
Figure 4. Mean (+ SD) square root transformed percentage cover of species most responsible for the dissimilarity in community composition among artificial substrate types. All species but Watersipora subtorquata showed higher abundance on fiberglass (FB) than High-Density Polyethylene (HDPE) and Polyvinyl Chloride (PVC) panels. Line above W. subtorquata indicates no significant effect of treatment.
Table 3. Results of the Kruskal-Wallis test considering the effect of artificial substrate material type on the percentage cover of species responsible for the dissimilarities between treatments.
Table 4. Results of the Dunn’s test considering the pairwise comparisons for the percentage cover of species between the different types of substrate materials including High-Density Polyethylene (HDPE), Polyvinyl Chloride (PVC) and fiberglass panels.
The overall percentage cover was influenced by substrate material type only, with no significant effect of species status (invasive and native) (Figure 5; Table 5). Fiberglass panels supported higher overall percentage cover than HDPE and PVC panels (fiberglass vs HDPE: P = 0.005; fiberglass vs PVC: P = 0.010). No significant differences in percentage cover were observed between HDPE and PVC panels (P = 0.828). Notably, no significant interactions were found between substrate material type and species status.
Figure 5. Mean (+ SD) overall square root transformed percentage cover considering the effect of substrate material type including High-Density Polyethylene (HDPE), Polyvinyl Chloride (PVC) and fiberglass (FB) panels and species status (invasive and native).
Table 5. Results of Generalized Least Squares Models considering the effect of substrate material type and species status on overall percentage cover.
While no effect of treatment was detected on species richness, species diversity (Shannon-Wiener index) differed significantly between the different types of substrate material (Figure 6; Table 6). Fiberglass panels supported higher species diversity than the other two types of materials (fiberglass vs HDPE: P = 0.002; fiberglass vs PVC: P = 0.022). No significant differences in diversity were detected between HDPE and PVC panels (P = 1.00).
Figure 6. Shannon-Wiener diversity index (H’) of fouling communities on fiberglass (FB), High-Density Polyethylene (HDPE) and Polyvinyl Chloride (PVC) panels after three months. Treatments identified by different letters differed significantly (P < 0.05).
Table 6. Results of the Kruskal-Wallis test comparing the Shannon-Weiner diversity index (H’) and species richness among the different types of artificial substrate materials.
Although fouling communities are well-studied and commonly used as model systems in community ecology (Needles and Wendt, 2013; Robinson et al., 2017), the understanding of how they might be influenced by substrate material remains limited. This study suggests that substrate material type has an influence on the sessile assemblage of fouling communities. Overall, fiberglass appears to have a dominant influence on these assemblages than hydrophobic substrate material types.
As hypothesised, artificial substrate material type influenced community structure and altered diversity in sessile fouling assemblages. At the community level, these changes can be attributed to the differential responses of species within the community to different substrate material types with varying physico-chemical properties. For example, in the present study Watersipora subtorquata, Bugula neritina, Spirorbis and Diplosoma listerianum showed high abundance across the experimental panels while the low occurrence of Styela plicata and Amphibalanus amphitrite was noted. These results align with previous research which shows that benthic invertebrate communities are influenced by the type of substrate material they settle on (Tyrrell and Byers, 2007; Dobretsov, 2015; Giangrande et al., 2021; Dodds et al., 2022; Grasselli et al., 2024). For example, recent meta-analyses reported differences in biofouling between artificial and natural substrate materials (Dodds et al., 2022; Grasselli et al., 2024). In Muscat, Sultanate of Oman, biofouling communities structurally differed on different artificial substrates in two marinas (Dobretsov, 2015; Muthukrishnan et al., 2019). In Port Pendennis Marina, Falmouth, surface heterogeneity, orientation and chemical composition of subtidal artificial substrates were found to influence benthic biological community recruitment patterns across temporal scales (Hanlon et al., 2018). Overall, the above studies, together with the findings of the present study, suggest that the type of material used in constructing artificial marine structures and their ecological implications should be included as important aspects of material selection criteria.
Following biofouling studies from South Africa (Peters et al., 2017; Robinson et al., 2017; Loureiro et al., 2021a, 2021b) and other regions (Crooks et al., 2011; Marraffini et al., 2017; Jewett et al., 2022), the present study mimicked floating jetties by deploying the substrate panels in a horizontal orientation with the experimental surface facing downward parallel to the seafloor. With this orientation, organic matter collects on the top side of the panels. Therefore, since we analysed the underside of the panels where fouling organisms grow in South Africa (Peters et al., 2017; Robinson et al., 2017; Loureiro et al., 2021a, b), the influence of organic matter on the colonisation of biota was likely negligible. However, much more work is needed to develop a holistic understanding of the implications of different substrate orientations (i.e. horizontal vs vertical) and deposition of organic matter for the colonisation of biofouling communities along the South African coast.
Fiberglass panels, more than High-Density Polyethylene (HDPE) and Polyvinyl Chloride (PVC) panels, supported higher species diversity and percentage cover. This may be due to differences in structural properties such as the physico-chemical composition of these artificial substrates (Wieczorek and Todd, 1998). Fiberglass is a composite substrate material with higher wettability than HDPE and PVC which are hydrophobic polymeric materials (Encinas et al., 2010). Hydrophilic substrates, more than their hydrophobic counterparts, favour the settlement and attachment of fouling organisms (Roberts et al., 1991; Becker, 1993; Sudhakar et al., 2008; Kumar et al., 2014; Muthukrishnan et al., 2019), which explains the higher values of species diversity and percentage cover on fiberglass panels observed in the present study. On the other hand, hydrophobic substrates have a less potential to be colonised because they exhibit low surface energy, which reduces adhesion strength of fouling organisms, making them easily removable from the surface (Callow and Fletcher, 1994; Liu et al., 2022; Romeu and Mergulhão, 2023). Our findings align with previous work which has also found that hydrophobic polymeric substrates generally support lower abundances of fouling organisms than hydrophilic surfaces (Muthukrishnan et al., 2019; Dodds et al., 2022). For example, surface hydrophobicity has been found to exhibit an antifouling effect, which delays the development of biota in the initial stages of biofouling (Cho et al., 2013). Studies have reported an increase in the settlement and attachment of fouling biota such as barnacles and polychaetes on wood and steel, which are hydrophilic, than on polyethylene terephthalate (PET) and polyethylene (PE) surfaces, which are hydrophobic substrates (Roberts et al., 1991; Becker, 1993; Sudhakar et al., 2008; Kumar et al., 2014). A similar pattern was observed for micro-and macrofouling organisms on the same set of substrates (steel, wood, PET and PE) in two locations in Muscat, Oman (Muthukrishnan et al., 2019). It is, however, important to also highlight that some biota are still able to settle on substrate materials with low wettability and may benefit from reduced competition for space (Rittschof and Costlow, 1989; Dodds et al., 2022). For example, a laboratory study on the influence of substrate material on ascidian larval settlement showed that Ciona intestinalis (Linnaeus, 1767) and Botrylloides violaceus (Oka, 1927) exhibit species-specific settlement preferences, with more individuals settling on HDPE than PVC panels in the absence of competition (Chase et al., 2016). Similarly, another study reported preference of Bugulina flabellata (Thompson in Gray, 1848) and B. neritina larvae for plastic substrate materials with low wettability rather than settling on wood or concrete substrates (Pinochet et al., 2020). These contrasting findings may be due to differential species-specific responses to substrate material type, different life stages (e.g. larvae, juveniles and adults), different community stages (e.g. micro-and macrofouling communities) and different study designs (e.g. laboratory vs field experiments). This highlights context dependency of biofouling colonisation on substrate material types, which precludes the use of a generic model in understanding fouling dynamics on artificial marine structures. Therefore, there is a need to expand foundational studies on the influence of substrate material on the colonisation of fouling biota as the present knowledge does not enable generalizations to be drawn.
Species richness did not significantly differ between substrate material types. This could be due to the low number of identified species on the panels. Probably, these species can colonise substrates regardless of the material, and/or adapt to the different features of each material. Moreover, these species could be displaying a great colonisation potential, allowing them to displace other competitors during the colonisation process over the three months-period covered in this study. Indeed, previous work has shown that fouling communities that have developed over a relatively short period, such as those in the present study, are generally dominated by few opportunistic species that may be outcompeted over longer time periods and sometimes replaced with more species-rich communities (Hanlon et al., 2018; Dodds et al., 2022). Our findings align with previous studies that show that substrate material type does not influence the number of species in sessile fouling communities (Tyrrell and Byers, 2007; Dodds et al., 2022; Hartanto et al., 2022; Kosová et al., 2023). For example, a study in Australia found that fiberglass and aluminium panels had a similar number of species after 4 months of submersion (Anderson and Underwood, 1994). Similarly, multiple studies comparing the effect of rock and concrete have reported no significant differences in species richness among the two types of substrates (Cacabelos et al., 2016; Hartanto et al., 2022; Kosová et al., 2023). In Wells, Maine USA, natural substrate types (i.e. shell, marble, slate, and wood) and artificial substrates (i.e. aluminium sheet metal, Styrofoam, PVC, and rubber) also showed no significant differences in the number of species throughout the study from May to October (Tyrrell and Byers, 2007). Finally, a recent meta-analysis reported a similar pattern of no significant differences in species richness among natural substrates (i.e. wood, biogenic, and rock) and artificial substrates (i.e. concrete, clay, polymers, and metals) (Dodds et al., 2022). Overall, considering the results of the present study and those from the previous work, it can be concluded that species richness does not vary as a function of substrate material type, which suggests that other factors might be more significant or affect different ecological metrices such as abundance rather than richness (Dennis et al., 2018; Kosová et al., 2023). It is important to note, however, that the present study and the previous ones highlighted above were conducted over a relatively short period of time. Going forward, future studies on the effect of substrate material types on biofouling assemblages should consider covering longer time frames to see if species richness remains similar among substrates and to potentially capture species that recruit in different seasons.
While the present study has contributed important insights into how artificial substrate material type might influence fouling assemblages, it is not without limitations. This study was a field-based experiment, with assemblages exposed to the full spectrum of biotic interactions and abiotic variability that they experience in the field. By the nature of the study design, recruitment was accounted for. However, we could not control for predatory interactions at this level. To understand the implications of predator-prey interactions for fouling community dynamics, knowledge of the predators that feed on these communities need to be held first (Loureiro et al., 2021b; Matikinca and Robinson, 2024a). Such knowledge is currently lacking in the South African context (Loureiro et al., 2021b). Additionally, following previous studies, we relied on photographic and microscopic taxonomic identification of specimens on the panels (Peters et al., 2014, 2017, 2019; Loureiro et al., 2021a; Matikinca and Robinson, 2024a). We acknowledge the probability of misidentifying specimens without DNA analysis. Financial constraints associated with DNA analysis for species identifications are acknowledged below. Finally, the present study was conducted over a relatively short term in one season and may have not captured species that are likely to recruit in other seasons. As such, additional studies that track the development of biofouling assemblages at multiple stages over longer periods of time are needed to investigate the long-term influence of substrate material type. Insightful for such studies, particularly in the South African context, will be considering the potential influence of varying substratum characteristics such as colour, roughness, orientation (i.e. horizontal vs vertical) and thermal capacity over longer time frames. While such studies would generate insightful data, the logistical constraints associated with long-term monitoring biofouling research are acknowledged (Egoh et al., 2020; Loureiro et al., 2021a). These include costs associated with taxonomy workshops with expert taxonomists, buying microscopes, DNA analysis for species identifications, travel time, labour, materials for constructing experimental arrays, preservation of specimens, photography equipment and image analysis (Loureiro et al., 2021a). When scrape samples need to be taken, in addition to using experimental arrays, costs include a dive boat, dive team with their equipment, collection equipment and specimen preservation (Loureiro et al., 2021a).
Notwithstanding the above limitations, this study, the first of its kind in South Africa, provides valuable insights into the influence of substrate material type on biofouling communities in an understudied region. Our findings contribute to a broader understanding of how substrate material preferences of sessile fouling biota manifest in the context of marine biological invasions. By examining biofouling communities in this specific region, we offer data that can contribute to predictive modelling and inform management strategies beyond local scales. As such, the significance of the present study extends beyond the South African or African context, as biofouling processes and invasive species dynamics are globally relevant concerns. The increasing movement of marine vessels and the expansion of coastal infrastructure all contribute to the spread of fouling biota, dominated by non-native species (Peters et al., 2014; Mayer-Pinto et al., 2017; Dodds et al., 2022). Understanding the role of substrate type in shaping biofouling communities provides a foundation for predicting invasion risks in harbours, marinas, and artificial structures worldwide. Our findings can be compared with similar studies in other regions (i.e. Tyrrell and Byers (2007); Dobretsov (2015); Giangrande et al. (2021); Dodds et al. (2022); Grasselli et al. (2024)) to refine patterns of biofouling community responses to different substrate types. Data from the present study, together with those from the previous work, can inform biosecurity and monitoring programs in other regions, particularly in temperate and subtropical coastal environments where invasion pressures are intensifying due to biofouling. Moreover, this research underscores the importance of investigating biofouling in regions where baseline data are scarce, ultimately contributing to a more comprehensive global perspective on marine biological invasions.
This study expands the knowledge of biofouling community composition in the context of varying substrate material type along the South African east coast, an understudied region when it comes to the dynamics of alien fouling biota. The study demonstrated that artificial substrate material type affects sessile fouling community structure along this region. Specifically, this study found that fiberglass panels supported higher species diversity and percentage cover than HDPE and PVC panels, highlighting differences in the ecological implications of hydrophobic and hydrophilic substrates for sessile fouling assemblages. These community-level changes likely stem from a range of responses across different species within community assemblages. Importantly, the differences in material preferences and differential responses among species will preclude the use of a generic model for anticipating how fouling biota may respond to the rapid development of marine infrastructure. Overall, our findings suggest that there is a need to consider the ecological implications of the material used in artificial marine structures during material selection criteria. To understand such implications, ecological studies investigating biofouling dynamics on marine infrastructure should account for the influence of different physico-chemical properties of various substrates on biota. Such properties include differences in orientation, surface microtexture, colour, material type, surface chemistry and wettability. Importantly, studies, particularly in understudied regions with data scarcity, will need to consider these effects over longer time periods across different spatial scales to capture biota that recruit in different seasons. Studies will also need to include comparisons with mature fouling communities on adjacent substrates that have been exposed for a longer duration, paying particular attention to species status (native and invasive alien species). This comparison could help us discern whether the differences observed over a relatively short period of time can be attributed to material type only or whether they simply reflect the natural progression of fouling community development over time.
The raw data supporting the conclusions of this article will be made available by the authors, without undue reservation.
The animal study was approved by School of Life Sciences, University of KwaZulu-Natal and written permission was obtained to conduct the research at the Point Yacht Club in Durban. The study was conducted in accordance with the local legislation and institutional requirements.
PM: Conceptualization, Data curation, Formal analysis, Methodology, Project administration, Resources, Supervision, Validation, Visualization, Writing – original draft, Writing – review & editing. VZ: Data curation, Formal analysis, Investigation, Writing – original draft, Writing – review & editing.
The author(s) declare that financial support was received for the research, authorship, and/or publication of this article. VZ received the BSc Honours program funding through the School of Life Sciences at University of KwaZulu-Natal.
Merushri Pillay and Liam Ferguson are thanked for their assistance during field work. Sithembiso Msabala is also thanked for his assistance when preparing the panels. We are grateful to Point Yacht Club for allowing us access to their walk-ons. The three reviewers are thanked for their insightful comments that served to strengthen this work.
The authors declare that the research was conducted in the absence of any commercial or financial relationships that could be construed as a potential conflict of interest.
The author(s) declare that no Generative AI was used in the creation of this manuscript.
All claims expressed in this article are solely those of the authors and do not necessarily represent those of their affiliated organizations, or those of the publisher, the editors and the reviewers. Any product that may be evaluated in this article, or claim that may be made by its manufacturer, is not guaranteed or endorsed by the publisher.
Albano M. J., Obenat S. M. (2019). Fouling assemblages of native, non-indigenous and cryptogenic species on artificial structures, depths and temporal variation. J. Sea Res. 144, 1–15. doi: 10.1016/j.seares.2018.10.002
Anderson M. J. (1996). A chemical cue induces settlement of Sydney rock oysters, Saccostrea commercialis, in the laboratory and in the field. Biol. Bull. 190, 350–358. doi: 10.2307/1543027
Anderson M., Gorley R., Clarke K. (2008). PERMANOVA+ for PRIMER: Guide to Software and Statistical Methods (Plymouth, UK: PRIMER-E).
Anderson M. J., Underwood A. J. (1994). Effects of substratum on the recruitment and development of an intertidal estuarine fouling assemblage. J. Exp. Mar. Bio. Ecol. 184, 217–236. doi: 10.1016/0022-0981(94)90006-X
Becker K. (1993). Attachment strength and colonization patterns of two macrofouling species on substrata with different surface tension (in situ studies). Mar. Biol. 117, 301–309. doi: 10.1007/BF00345675
Bhattacharyya S. K., Deb A. (2022). “Use and prospects of concrete as a cementitious material,” in Future landscape of structural materials in India (Springer Nature Singapore, Singapore), 293–307. doi: 10.1007/978-981-16-8523-1_12
Bishop M. J., Mayer-Pinto M., Airoldi L., Firth L. B., Morris R. L., Loke L. H. L., et al. (2017). Effects of ocean sprawl on ecological connectivity: impacts and solutions. J. Exp. Mar. Bio. Ecol. 492, 7–30. doi: 10.1016/j.jembe.2017.01.021
Bray J. R., Curtis J. T. (1957). An ordination of the upland forest communities of southern Wisconsin. Ecol. Monogr. 27, 326–349. doi: 10.2307/1942268
Bulleri F., Chapman M. G. (2010). The introduction of coastal infrastructure as a driver of change in marine environments. J. Appl. Ecol. 47, 26–35. doi: 10.1111/j.1365-2664.2009.01751.x
Busk G. (1852). “Catalogue of marine polyzoa in the collection of the british museum,” in I. cheilostomata (London, UK: Trustees of the British Museum).
Cacabelos E., Martins G. M., Thompson R., Prestes A. C. L., Azevedo J. M. N., Neto A. I. (2016). Material type and roughness influence structure of inter-tidal communities on coastal defenses. Mar. Ecol. 37, 801–812. doi: 10.1111/maec.12354
Callow M. E., Fletcher R. L. (1994). The influence of low surface energy materials on bioadhesion - a review. Int. Biodeterior. Biodegrad. 34, 333–348. doi: 10.1016/0964-8305(94)90092-2
Chase A. L., Dijkstra J. A., Harris L. G. (2016). The influence of substrate material on ascidian larval settlement. Mar. pollut. Bull. 106, 35–42. doi: 10.1016/j.marpolbul.2016.03.049
Chase A. L., Dijkstra J. A., Harris L. G. (2015). Effects of substrate material on marine fouling community composition and ascidian larval settlement (New Hampshire, United States: University of New Hampshire).
Chlayon T., Iwanami M., Chijiwa N. (2018). Combined protective action of barnacles and biofilm on concrete surface in intertidal areas. Constr. Build. Mater. 179, 477–487. doi: 10.1016/j.conbuildmat.2018.05.223
Cho S. H., Ryu S. N., Hwang W. B., Yoon B. S. (2013). Anti-fouling property of hydrophobic surfaces in sea water. J. Korean Soc Mar. Environ. Energy 16, 82–87. doi: 10.7846/jkosmee.2013.16.2.82
Ciocan C., Annels C., Fitzpatrick M., Couceiro F., Steyl I., Bray S. (2024). Glass reinforced plastic (GRP) boats and the impact on coastal environment – Evidence of fibreglass ingestion by marine bivalves from natural populations. J. Hazard. Mater. 472, 134619. doi: 10.1016/j.jhazmat.2024.134619
Clarke K., Warwick R. (1994). Change in marine communities: an approach to statistical analysis and interpretation. (Plymouth, UK: PRIMER-E Ltd).
Clarke K. R., Warwick R. (2001). Change in marine communities: an approach to statistical analysis and interpretation (Plymouth, UK: PRIMER-E Ltd).
Coombes M. (2011). Biogeomorphology of Coastal Structures: Understanding interactions between hard substrata and colonising organisms as a tool for ecological enhancement (England, UK: University of Exeter).
Coombes M. A., La Marca E. C., Naylor L. A., Thompson R. C. (2015). Getting into the groove: Opportunities to enhance the ecological value of hard coastal infrastructure using fine-scale surface textures. Ecol. Eng. 77, 314–323. doi: 10.1016/j.ecoleng.2015.01.032
Crooks J. A., Chang A. L., Ruiz G. M. (2011). Aquatic pollution increases the relative success of invasive species. Biol. Invasions 13, 165–176. doi: 10.1007/s10530-010-9799-3
Dafforn K. A., Glasby T. M., Airoldi L., Rivero N. K., Mayer-Pinto M., Johnston E. L. (2015). Marine urbanization: An ecological framework for designing multifunctional artificial structures. Front. Ecol. Environ. 13, 82–90. doi: 10.1890/140050
Darwin C. (1854). A Monograph on the sub-class Cirripedia with figures of all the species (London, UK: Ray Society Publication).
Daudin F. M. (1800). Receuil de mémoires et de notes sur des espèces inédites ou peu connues de mollusques, de vers et de zoophytes (Paris, France: Fuchs & Treuttel et Wurtz).
Day J. (1967). A monograph on the Polychaeta of southern Africa. Vol. 1 & 2 (London, UK: British Museum of Natural History).
Dennis H. D., Evans A. J., Banner A. J., Moore P. J. (2018). Reefcrete: Reducing the environmental footprint of concretes for eco-engineering marine structures. Ecol. Eng. 120, 668–678. doi: 10.1016/j.ecoleng.2017.05.031
Dhandapani A., Krishnasamy S., Thiagamani S. M. K., Periasamy D., Muthukumar C., Sundaresan T. K., et al. (2024). Evolution, prospects, and predicaments of polymers in marine applications: A potential successor to traditional materials. Recycling 9, 1–20. doi: 10.3390/recycling9010008
Dobretsov S. (2015). Biofouling on artificial substrata in Muscat waters. J. Agric. Mar. Sci. 91, 24–29. doi: 10.24200/jams.vol20iss0pp24-29
Dobretsov S., Abed R. M. M., Voolstra C. R. (2013). The effect of surface colour on the formation of marine micro and macrofouling communities. Biofouling 29, 617–627. doi: 10.1080/08927014.2013.784279
Dodds K. C., Schaefer N., Bishop M. J., Nakagawa S., Brooks P. R., Knights A. M., et al. (2022). Material type influences the abundance but not richness of colonising organisms on marine structures. J. Environ. Manage. 307, 114549. doi: 10.1016/j.jenvman.2022.114549
Dugan J. E., Airoldi L., Chapman M. G., Walker S. J., Schlacher T. (2012). “Estuarine and coastal structures: Environmental effects, a focus on shore and nearshore structures,” in Treatise on Estuarine and Coastal Science. Eds. Wolanski E., McLusky D. (Academic Press, London). doi: 10.1016/B978-0-12-374711-2.00802-0
Dunn O. J. (1961). Multiple comparisons among means. J. Am. Stat. Assoc. 56, 52–64. doi: 10.1080/01621459.1961.10482090
Dunn O. J. (1964). Multiple comparisons using rank sums. Technometrics 6, 241–252. doi: 10.1080/00401706.1964.10490181
Egoh B. N., Ntshotsho P., Maoela M. A., Blanchard R., Ayompe L. M., Rahlao S. (2020). Setting the scene for achievable post-2020 convention on biological diversity targets: A review of the impacts of invasive alien species on ecosystem services in Africa. J. Environ. Manage. 261, 110171. doi: 10.1016/j.jenvman.2020.110171
Ells V., Filip N., Bishop C. D., DeMont M. E., Smith-Palmer T., Wyeth R. C. (2016). A true test of colour effects on marine invertebrate larval settlement. J. Exp. Mar. Bio. Ecol. 483, 156–161. doi: 10.1016/j.jembe.2016.07.011
Encinas N., Pantoja M., Abenojar J., Martínez M. A. (2010). Control of wettability of polymers by surface roughness modification. J. Adhes. Sci. Technol. 24, 1869–1883. doi: 10.1163/016942410X511042
Fazey F. M. C., Ryan P. G. (2016). Biofouling on buoyant marine plastics: An experimental study into the effect of size on surface longevity. Environ. Pollut. 210, 354–360. doi: 10.1016/j.envpol.2016.01.026
Finlay J. A., Fletcher B. R., Callow M. E., Callow J. A. (2008). Effect of background colour on growth and adhesion strength of Ulva sporelings. Biofouling 24, 219–225. doi: 10.1080/08927010802040693
Firth L. B., Knights A. M., Bridger D., Evans A. J., Mieszkowska N., Moore P. J., et al. (2016a). Ocean sprawl: Challenges and opportunities for biodiversity management in a changing world. Oceanogr. Mar. Biol. Annu. Rev. 54, 193–269. doi: 10.1201/9781315368597
Firth L. B., Thompson R. C., White F. J., Schofield M., Skov M. W., Hoggart S. P. G., et al. (2013). The importance of water-retaining features for biodiversity on artificial intertidal coastal defence structures. Divers. Distrib. 19, 1275–1283. doi: 10.1111/ddi.12079
Firth L. B., White F. J., Schofield M., Hanley M. E., Burrows M. T., Thompson R. C., et al. (2016b). Facing the future: The importance of substratum features for ecological engineering of artificial habitats in the rocky intertidal. Mar. Freshw. Res. 67, 131–143. doi: 10.1071/MF14163
Giangrande A., Arduini D., Borghese J., Del Pasqua M., Lezzi M., Petrocelli A., et al. (2021). Macrobenthic success of non-indigenous species related to substrate features in the mar grande of taranto, Italy (Mediterranean sea). BioInvasions Rec. 10, 238–256. doi: 10.3391/bir.2021.10.2.02
Gowell M. R., Coombes M. A., Viles H. A. (2015). Rock-protecting seaweed? Experimental evidence of bioprotection in the intertidal zone. Earth Surf. Process Landforms 40, 1364–1370. doi: 10.1002/esp.3736
Grasselli F., Strain E. M. A., Airoldi L. (2024). Material type and origin influences the abundances of key taxa on artificial structures. Coast. Eng. 187, 104419. doi: 10.1016/j.coastaleng.2023.104419
Gray J. E. (1848). “List of the specimens of british animals in the collection of the british museum. part 1,” in Centroniae or radiated animals (London, UK: Trustees of the British Museum).
Hanlon N., Firth L. B., Knights A. M. (2018). Time-dependent effects of orientation, heterogeneity and composition determines benthic biological community recruitment patterns on subtidal artificial structures. Ecol. Eng. 122, 219–228. doi: 10.1016/j.ecoleng.2018.08.013
Harlin M. M., Lindbergh J. M. (1977). Selection of substrata by seaweeds: Optimal surface relief. Mar. Biol. 40, 33–40. doi: 10.1007/BF00390625
Hartanto R. S., Loke L. H. L., Heery E. C., Hsiung A. R., Goh M. W. X., Pek Y. S., et al. (2022). Material type weakly affects algal colonisation but not macrofaunal community in an artificial intertidal habitat. Ecol. Eng. 176, 106514. doi: 10.1016/j.ecoleng.2021.106514
Hayek M., Salgues M., Souche J. C., Weerdt K. D., Pioch S. (2022). From concretes to bioreceptive concretes, influence of concrete properties on the biological colonization of marine artificial structures. IOP Conf. Ser. Mater. Sci. Eng. 1245, 12008. doi: 10.1088/1757-899x/1245/1/012008
Hsiung A. R., Tan W. T., Loke L. H. L., Firth L. B., Heery E. C., Ducker J., et al. (2020). Little evidence that lowering the pH of concrete supports greater biodiversity on tropical and temperate seawalls. Mar. Ecol. Prog. Ser. 656, 193–205. doi: 10.3354/meps13365
Jewett E. B., Lawson K. N., Larson K. J., Tracy B. M., Altman S., Chang A. L., et al. (2022). Differences in fouling community composition and space occupation across broad spatial and temporal scales. Front. Mar. Sci. 9. doi: 10.3389/fmars.2022.933405
Jones K. M. M., Boulding E. G. (1999). State-dependent habitat selection by an intertidal snail: The costs of selecting a physically stressful microhabitat. J. Exp. Mar. Bio. Ecol. 242, 149–177. doi: 10.1016/S0022-0981(99)00090-8
Koehl M. R. A. (2007). Mini review: Hydrodynamics of larval settlement into fouling communities. Biofouling 23, 357–368. doi: 10.1080/08927010701492250
Kosová E., James K., MacArthur M., Vovides A., Peters J., Metcalfe D., et al. (2023). The BioGeo Ecotile: Improving biodiversity on coastal defences using a multiscale, multispecies eco-engineering design. Ecol. Eng. 188, 106881. doi: 10.1016/j.ecoleng.2022.106881
Kruskal W. H., Wallis W. A. (1952). Use of ranks in one-criterion variance analysis. J. Am. Stat. Assoc. 47, 583–621. doi: 10.1080/01621459.1952.10483441
Kumar N., Venkatesan R., Doble M. (2014). “Macrofouling and bioadhesion of organisms on polymers,” in Polymers in a marine environment. Eds. Doble R., Venkatesan R., Kumar N. (Shropshire, UK: Smithers Rapra Technology Limited), 101–120.
Lathlean J. A., Minchinton T. E. (2012). Manipulating thermal stress on rocky shores to predict patterns of recruitment of marine invertebrates under a changing climate. Mar. Ecol. Prog. Ser. 467, 121–136. doi: 10.3354/meps09996
Lesueur C. A. (1823). Descriptions of several new species of ascidia. J. Acad. Nat. Sci. Philadelphia 3, 2–8.
Levene H. (1960). “Robust tests for equality of variances,” in Contributions to probability and statistics: Essays in Honor of Harold Hotelling. Eds. Olkin I., Hotelling H. (Stanford, USA: Stanford University Press), 278–292.
Liu Z., Zheng X., Zhang H., Li W., Jiang R., Zhou X. (2022). Review on formation of biofouling in the marine environment and functionalization of new marine antifouling coatings. J. Mater. Sci. 57, 18221–18242. doi: 10.1007/s10853-022-07791-8
Loke L. H. L., Ladle R. J., Bouma T. J., Todd P. A. (2015). Creating complex habitats for restoration and reconciliation. Ecol. Eng. 77, 307–313. doi: 10.1016/j.ecoleng.2015.01.037
Loke L. H. L., Todd P. A. (2016). Structural complexity and component type increase intertidal biodiversity independently of area. Ecology 97, 383–393. doi: 10.1890/15-0257.1
Loureiro T. G., Peters K., Robinson T. B. (2021a). Dropping plates to pick up aliens: towards a standardised approach for monitoring alien fouling species. Afr. J. Mar. Sci. 43, 1–15. doi: 10.2989/1814232X.2021.1989488
Loureiro T. G., Peters K., Robinson T. B. (2021b). Light, shade and predation: who wins and who loses in sessile fouling communities? Mar. Biodivers. 51, 94. doi: 10.1007/s12526-021-01241-5
Marraffini M. L., Ashton G. V., Brown C. W., Chang A. L., Ruiz G. M. (2017). Settlement plates as monitoring devices for non-indigenous species in marine fouling communities. Manage. Biol. Invasions 8, 559–566. doi: 10.3391/mbi.2017.8.4.11
Matikinca P., Robinson T. B. (2024a). Fouling communities from the South African west coast are vulnerable to cooling and ocean acidification. Mar. Biodivers. 54, 27. doi: 10.1007/s12526-024-01420-0
Matikinca P., Robinson T. B. (2024b). Altered temperature and restricted food supply reduce pH tolerance in both young and adult ascidians. Mar. Biol. Res. 20, 278–287. doi: 10.1080/17451000.2024.2390528
Mayer-Pinto M., Cole V. J., Johnston E. L., Bugnot A., Hurst H., Airoldi L., et al. (2018). Functional and structural responses to marine urbanisation. Environ. Res. Lett. 13, 014009. doi: 10.1088/1748-9326/aa98a5
Mayer-Pinto M., Johnston E. L., Bugnot A. B., Glasby T. M., Airoldi L., Mitchell A., et al. (2017). Building ‘blue’: An eco-engineering framework for foreshore developments. J. Environ. Manage. 189, 109–114. doi: 10.1016/j.jenvman.2016.12.039
McManus R. S., Archibald N., Comber S., Knights A. M., Thompson R. C., Firth L. B. (2018). Partial replacement of cement for waste aggregates in concrete coastal and marine infrastructure: A foundation for ecological enhancement? Ecol. Eng. 120, 655–667. doi: 10.1016/j.ecoleng.2017.06.062
Milne Edwards H. (1841). Observations sur les ascidies composées des côtes de la manche (Paris, France: Chez Fortin-Masson et Cie).
Monniot C., Monniot F., Griffiths C., Schleyer M. (2001). South African ascidians. Ann. South Afr. Museum. 108, 1–141. doi: biostor.org/reference/113425
Moschella P. S., Abbiati M., Åberg P., Airoldi L., Anderson J. M., Bacchiocchi F., et al. (2005). Low-crested coastal defence structures as artificial habitats for marine life: Using ecological criteria in design. Coast. Eng. 52, 1053–1071. doi: 10.1016/j.coastaleng.2005.09.014
Muthukrishnan T., Al Khaburi M., Abed R. M. M. (2019). Fouling microbial communities on plastics compared with wood and steel: Are they substrate- or location-specific? Microb. Ecol. 78, 361–374. doi: 10.1007/s00248-018-1303-0
Nall C. R., Schläppy M. L., Cottier-Cook E. J., Guerin A. J. (2022). Influence of coating type, colour, and deployment timing on biofouling by native and non-native species in a marine renewable energy context. Biofouling 38, 729–745. doi: 10.1080/08927014.2022.2121209
Needles L. A., Wendt D. E. (2013). Big changes to a small bay: Introduced species and long-term compositional shifts to the fouling community of Morro Bay (CA). Biol. Invasions 15, 1231–1251. doi: 10.1007/s10530-012-0362-2
Oka A. (1927). Zur kenntnis der japanishen botryllidae (Vorlaufige mitteilung). Proc. Imp. Acad. 3, 607–609.
Osborn D. A. (2003). “The effects of armoring the coastline on intertidal flora and fauna,” in In Oceans 2003. Celebrating the Past … Teaming Toward the Future. Eds. IEEE. (San Diego, USA: IEEE). doi: 10.1109/OCEANS.2003.178540
Osborn D. A. (2005). Rocky Intertidal Community Structure on Different Substrates. [PhD dissertation]. (Santa Cruz, California, USA: University of California).
Ostalé-Valriberas E., Sempere-Valverde J., Coppa S., García-Gómez J. C., Espinosa F. (2018). Creation of microhabitats (tidepools) in ripraps with climax communities as a way to mitigate negative effects of artificial substrate on marine biodiversity. Ecol. Eng. 120, 522–531. doi: 10.1016/j.ecoleng.2018.06.023
Perkol-Finkel S., Sella I. (2014). “Ecologically active concrete for coastal and marine infrastructure: Innovative matrices and designs,” in From Sea to Shore - Meeting the Challenges of the Sea. Eds Allsop W., Burgess K.. (London, UK: ICE Publishing).
Peters K., Griffiths C., Robinson T. B. (2014). Patterns and drivers of marine bioinvasions in eight Western Cape harbours, South Africa. Afr. J. Mar. Sci. 36, 49–57. doi: 10.2989/1814232X.2014.890669
Peters K., Sink K., Robinson T. B. (2017). Raising the flag on marine alien fouling species. Manage. Biol. Invasions 8, 1–11. doi: 10.3391/mbi.2017.8.1.01
Peters K., Sink K., Robinson T. B. (2019). Aliens cruising in: Explaining alien fouling macro-invertebrate species numbers on recreational yachts. Ocean Coast. Manage. 182, 104986. doi: 10.1016/j.ocecoaman.2019.104986
Pinochet J., Urbina M. A., Lagos M. E. (2020). Marine invertebrate larvae love plastics: Habitat selection and settlement on artificial substrates. Environ. pollut. 257, 113571. doi: 10.1016/j.envpol.2019.113571
Pioch S., Relini G., Souche J. C., Stive M. J. F., De Monbrison D., Nassif S., et al. (2018). Enhancing eco-engineering of coastal infrastructure with eco-design: Moving from mitigation to integration. Ecol. Eng. 120, 574–584. doi: 10.1016/j.ecoleng.2018.05.034
Pister B. (2009). Urban marine ecology in southern California: The ability of riprap structures to serve as rocky intertidal habitat. Mar. Biol. 156, 861–873. doi: 10.1007/s00227-009-1130-4
Porter A. G., Ferrari R. L., Kelaher B. P., Smith S. D. A., Coleman R. A., Byrne M., et al. (2018). Marine infrastructure supports abundant, diverse fish assemblages at the expense of beta diversity. Mar. Biol. 165, 112. doi: 10.1007/s00227-018-3369-0
R Core Team (2021). R: a language and environment for statistical computing. Available online at: https://www.r-project.org (Accessed March 1, 2022).
Rittschof D., Costlow J. (1989). Bryozoan and barnacle settlement in relation to initial surface wettability: a comparison of laboratory and field studies. Sci. Mar. 53, 411–416.
Roberts D., Rittschof D., Holm E., Schmidt A. R. (1991). Factors influencing initial larval settlement: temporal, spatial and surface molecular components. J. Exp. Mar. Bio. Ecol. 150, 203–221. doi: 10.1016/0022-0981(91)90068-8
Robinson T. B., Alexander M. E., Simon C. A., Griffiths C. L., Peters K., Sibanda S., et al. (2016). Lost in translation? Standardising the terminology used in marine invasion biology and updating South African alien species lists. Afr. J. Mar. Sci. 2338, 1–12. doi: 10.2989/1814232X.2016.1163292
Robinson T. B., Havenga B., van der Merwe M., Jackson S. (2017). Mind the gap - context dependency in invasive species impacts: A case study of the ascidian Ciona robusta. NeoBiota 32, 127–141. doi: 10.3897/neobiota.32.9373
Rocha R. M., Zanata T. B., Moreno T. R. (2012). Keys for the identification of families and genera of Atlantic shallow water ascidians. Biota Neotrop. 12, 269–303. doi: 10.1590/s1676-06032012000100022
Romeu M. J., Mergulhão F. (2023). Development of antifouling strategies for marine applications. Microorganisms 11, 1568. doi: 10.3390/microorganisms11061568
Rubino F., Nisticò A., Tucci F., Carlone P. (2020). Marine application of fiber reinforced composites: A review. J. Mar. Sci. Eng. 8, 1–28. doi: 10.3390/JMSE8010026
Ryan P. G. (2020). The transport and fate of marine plastics in South Africa and adjacent oceans. S. Afr. J. Sci. 116, 1–9. doi: 10.17159/sajs.2020/7677
Sakinah W., Kusnadi R. F., Prasetiaji D. B., Aji P. P., Widityo R. P. G., Kristianta F. X. (2023). The effect of shipbuilding material type on biofouling growth at Boom Marina, Banyuwangi, East Java, Indonesia. J. Kelaut. Indones. J. Mar. Sci. Technol. 16, 44–51. doi: 10.21107/jk.v16i1.17964
Schaefer N., Bishop M. J., Bugnot A. B., Herbert B., Hoey A. S., Mayer-Pinto M., et al. (2024). Variable effects of substrate colour and microtexture on sessile marine taxa in Australian estuaries. Biofouling 40, 223–234. doi: 10.1080/08927014.2024.2332710
Sedano F., Florido M., Rallis I., Espinosa F., Gerovasileiou V. (2019). Comparing sessile benthos on shallow artificial versus natural hard substrates in the Eastern Mediterranean Sea. Mediterr. Mar. Sci. 20, 688–702. doi: 10.12681/mms.17897
Sella I., Perkol Finkel S. (2015). Blue is the new green - Ecological enhancement of concrete based coastal and marine infrastructure. Ecol. Eng. 84, 260–272. doi: 10.1016/j.ecoleng.2015.09.016
Sempere-Valverde J., Ostalé-Valriberas E., Farfán G. M., Espinosa F. (2018). Substratum type affects recruitment and development of marine assemblages over artificial substrata: A case study in the Alboran Sea. Estuar. Coast. Shelf Sci. 204, 56–65. doi: 10.1016/j.ecss.2018.02.017
Shapiro S. S., Wilk M. B. (1965). An analysis of variance test for normality (Complete samples). Biometrika 52, 591–611. doi: 10.2307/2333709
Smith A., Gordon D. (2011). Bryozoans of southern New Zealand: a field identification guide. New Zealand Aquatic Environment and Biodiversity Report No. 75 (Wellington, New Zealand: Ministry of Fisheries Wellington).
Sudhakar M., Doble M., Murthy P. S., Venkatesan R. (2008). Marine microbe-mediated biodegradation of low- and high-density polyethylenes. Int. Biodeterior. Biodegrad. 61, 203–213. doi: 10.1016/j.ibiod.2007.07.011
Susick K., Scianni C., Mackie J. A. (2020). Artificial structure density predicts fouling community diversity on settlement panels. Biol. Invasions 22, 271–292. doi: 10.1007/s10530-019-02088-5
Swain G., Herpe S., Ralston E., Tribou M. (2006). Short-term testing of antifouling surfaces: The importance of colour. Biofouling 22, 425–429. doi: 10.1080/08927010601037163
Terlizzi A., Faimali M. (2010). “Fouling on artificial substrata,” in Biofouling. Eds. Dürr S., Thomason J. (Wiley-Blackwell, Oxford, UK), 170–184. doi: 10.1002/9781444315462.ch12
Tyrrell M. C., Byers J. E. (2007). Do artificial substrates favor nonindigenous fouling species over native species? J. Exp. Mar. Bio. Ecol. 342, 54–60. doi: 10.1016/j.jembe.2006.10.014
Keywords: biofouling, artificial substrate material, community ecology, marine invertebrates, marine structures, South Africa
Citation: Matikinca P and Zondi V (2025) The implications of artificial substrate material type for sessile fouling communities along the South African east coast. Front. Mar. Sci. 12:1511921. doi: 10.3389/fmars.2025.1511921
Received: 15 October 2024; Accepted: 17 February 2025;
Published: 24 March 2025.
Edited by:
Koebraa Peters, Cape Peninsula University of Technology, South AfricaReviewed by:
Kathleen Sullivan Sealey, University of Miami, United StatesCopyright © 2025 Matikinca and Zondi. This is an open-access article distributed under the terms of the Creative Commons Attribution License (CC BY). The use, distribution or reproduction in other forums is permitted, provided the original author(s) and the copyright owner(s) are credited and that the original publication in this journal is cited, in accordance with accepted academic practice. No use, distribution or reproduction is permitted which does not comply with these terms.
*Correspondence: Phikolomzi Matikinca, cGhpa29sb216aS5tYXRpa2luY2FAdWN0LmFjLnph
Disclaimer: All claims expressed in this article are solely those of the authors and do not necessarily represent those of their affiliated organizations, or those of the publisher, the editors and the reviewers. Any product that may be evaluated in this article or claim that may be made by its manufacturer is not guaranteed or endorsed by the publisher.
Research integrity at Frontiers
Learn more about the work of our research integrity team to safeguard the quality of each article we publish.