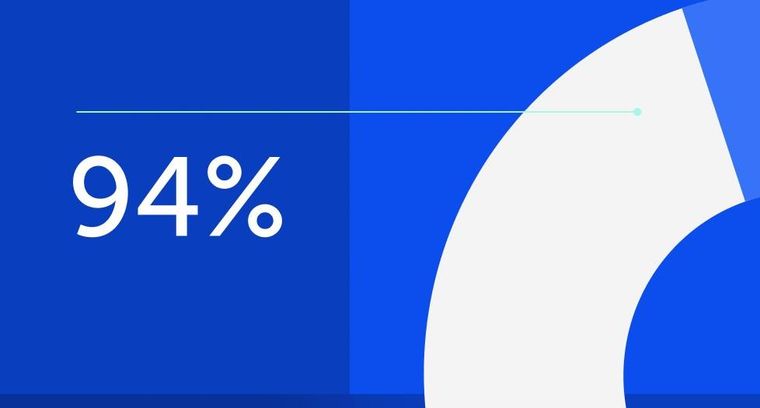
94% of researchers rate our articles as excellent or good
Learn more about the work of our research integrity team to safeguard the quality of each article we publish.
Find out more
ORIGINAL RESEARCH article
Front. Mar. Sci., 28 January 2025
Sec. Marine Fisheries, Aquaculture and Living Resources
Volume 12 - 2025 | https://doi.org/10.3389/fmars.2025.1510574
Introduction: Like pelagic fishes, cephalopods represent fishery resources with high worldwide potential and an important evolutionary node animal group. Thus, exploring the spatial and seasonal variations in cephalopod distribution and their associations with environmental factors is important for elucidating cephalopod diversity and the distribution of cephalopods under changing environmental conditions and for developing reasonable resource management and conservation measures.
Methods: On the basis of trawl survey data from 123 stations in the coastal waters of Zhejiang, China, in the spring and autumn of 2021, the relationships among the cephalopod species composition and diversity characteristics and the spatial and seasonal changes and environmental factors were investigated via the relative importance index (IRI), the alpha diversity index, and the GAM.
Results: The results revealed that the cephalopods in the coastal waters of Zhejiang belonged to three orders, six families, and 10 genera and that the dominant species were gradually replaced by species of minor economic importance and endemic small-scale fishery species. In spring, Uroteuthis duvaucelii was the dominant species, the resource density in the southern coastal waters of Zhejiang was relatively high, and the overall distribution increased from northeast to southwest. In autumn, U. duvaucelii and Abralia multihamata were the dominant species, and the coastal waters of Zhejiang presented two high-density distribution areas in the northern and central waters, with lower resource density in the southern waters. Analysis of the alpha diversity index revealed relatively low cephalopod community diversity, with significant differences in spatial and seasonal distributions. The GAM revealed that, in spring, surface dissolved oxygen and sea bottom temperature led to significant changes in cephalopod resource density (p<0.05), and water depth significantly affected cephalopod resource density (p<0.01); in autumn, only water depth significantly affected cephalopod resource density (p<0.01).
Discussion: The cephalopod resources in the coastal waters of Zhejiang are under the dual stresses of fishing and environmental changes. The protection and restoration of traditional economic cephalopod resources are needed to ensure their sustainable development and utilization.
Cephalopods play important ecological roles in marine trophic networks and are usually referred to as “key” species (Boavida-Portugal et al., 2022). The are an important component of fishery resources whose importance has risen sharply since 1950 and continues to grow. World-wide, cephalopod fisheries have rapidly expanded over the last 50 years, with the catch was approximately 374,200 tons in 2020 (Ainsworth et al., 2023). In the context of global climate change and the overfishing of fishery resources, cephalopods can actively respond to environmental change due to their rapid growth, short life cycle, and strong plasticity of the life cycle, as they can achieve both “active migration” and “passive utilization”. However, when environmental conditions exceed the physiological tolerance of an individual species or when key ecological species are affected, these changes may affect primary productivity, species distribution, and the community and food web structure and may even have direct and indirect impacts on marine ecosystems (Doubleday et al., 2016; Schickele et al., 2021; Boavida-Portugal et al., 2022).
Currently, coastal ecosystems are being affected by climate change at a faster rate than other ecosystems are, and coastal species are facing various pressures (Pang et al., 2022). The coastal waters of Zhejiang, China, belong to the East China Sea and are influenced by the multiple influences of the estuary of the Yangtze River, the Kuroshio current, and the East Asian monsoon. It is rich in nutrients and a suitable habitat for various marine organisms, is the most important fishery area in China, and is rich in cephalopod resources (Zheng et al., 2023). For many years, fishing intensity in the coastal waters of China has not been effectively controlled, which has directly led to the overfishing of fishery resources and the destruction of habitats. Historically, the dominant cephalopod in the East China Sea was Sepiella japonica, with a maximum annual output of 70,000 tons. However, owing to unreasonable development methods, resources began to decline in the early 1980s and were almost functionally extinct in the early 21st century. With the development of otter trawl and squid fishing operations, the composition of the cephalopod community and the dominant species in the coastal waters of Zhejiang have also changed multiple times. Since the implementation of fishery restoration and revitalization action in Zhejiang Province in 2013, fishery resources in the coastal waters of Zhejiang Province have been recovering, and the cephalopod community has also remained relatively stable in recent years (Chen et al., 2020). Based on the cephalopod survey data obtained from the Zhejiang coastal trawl in the spring and autumn of 2021, the spatial and seasonal changes in the species and community diversity of cephalopods and their correlations with the environment were analysed. The results of this study can provide a basis for the sustainable development and utilization of cephalopod resources in the coastal waters of Zhejiang.
The data were obtained from trawl survey of fishery resources in spring (April) and autumn (November) in 2021 in the coastal waters of Zhejiang. The survey area is 27°00′N~31°00′N, 120°50′E~123°75′E. The survey stations completely cover the coastal area of Zhejiang Province, and the stations are arranged eastwards to the line of the area closed to trawl fishery, with the stations at 0.5° intervals. A total of 123 sampling stations (Figure 1) were established to cover the coastal area of Zhejiang Province, arranged eastwards to the line of the area closed to trawl fishery, with the stations at 0.5° intervals. The coastal seas of Zhejiang were divided into the northern area (30°–31°N, with 46 stations), the central area (28.5°–30°N, with 41 stations), and the southern area (27°–28.5°N, 36 stations). The gear and net type of fishing vessel in the survey were single boat bottom otter trawls. The main engine power of the fishing vessel was 220 kW, the mesh size of the otter trawls was 20 mm, the circumference of the net opening was 50 m, and the average hauling speed of the fishing vessel during operation was 3.0 kn. The bit dragging time was set to 1 h. The environmental data were simultaneously acquired at sea via a multifunctional water quality meter (AAQ171). The parameters included water depth, seawater temperature, seawater salinity, chlorophyll a, and dissolved oxygen.
The cephalopods were identified to the species level on the basis of the “Checklist of Marine Biota of China Seas” (Liu, 2008). The total number and total weight of the specimen catch, were recorded. We also analysed the relationships among species composition, dominant species, diversity, and environmental factors. The analysis methods were as follows:
The survey data were normalized to the recorded catches, towing speed, and towing time for each species. With a towing speed of 3 kn and a towing time of 1 h as the standards, the resource density per unit time Y (ind./h) was calculated on the basis of the survey catch.
The index of relative importance (IRI) was used to calculate the dominant species of cephalopods in the coastal waters of Zhejiang Province (Pinkas et al., 1971):
where N is the percentage (%) of the number of individuals of a certain species in the total number of individuals caught; W is the percentage (%) of the body weight of a certain species in the total catch mass; and F is the percentage (%) of the number of stations where a certain species occurred to the total number of survey stations. The determination of dominant species was based on the division criteria of Wang et al. (2010): species with IRI>1000 were defined as the dominant species, those with 1000>IRI>100 as important species, those with 100>IRI>10 as common species, those with 10>IRI>1 as general species, and those with IRI<1 as rare species.
The index of Margalef index (D), Shannon–Weiner index (H'), and Pielou index (J') were used to analyse cephalopod diversity (Pielou, 1966; Spellerberg and Fedor, 2003; Magurran, 2021).
Margalef:
Shannon–Weiner:
Pielou:
where S is the total number of cephalopod species; N is the total number of cephalopods; and Pi is the proportion of the i-th type of cephalopod in the total number.
A generalized additive model (GAM) can explore the nonlinear relationship between the response variable and explanatory variables and determine the level of response for each explanatory variable (Hastie and Tibshirani, 1990; Walsh and Kleiber, 2001). Before modelling, the variance inflation factor (VIF) was first used to conduct a collinearity test on the explanatory variables for spring and autumn to remove the explanatory variables with multicollinearity (factors with VIF values greater than 10) (Cui et al., 2023). Then, the screened environmental factors were superimposed into the multivariate GAM, a smooth curve was fitted by a natural spline function, and a p value test was used to test the importance of the explanatory variables.
The above processes of diversity, model construction, and verification and assessment were performed via R4.2.2 software; the diversity was calculated via the “vegan” package; and the GAM was constructed and fitted via the “mgcv” package.
A total of 20 species of cephalopods belonging to three orders, six families, and 10 genera were captured in the spring and autumn of 2021. There were two genera and six species in one family in the Myopsida Orbigny of Teuthida, two genera and two species in one family in the Oegopsida of Teuthida, two genera and six species in two families of the Octopoda, and four genera and six species in two families of the Sepiida. Among them, 16 species were caught in spring, and 13 species were caught in autumn (Figure 2). In spring, Uroteuthis duvaucelii had the highest relative importance index at 1288.49, followed by S. japonica and Abralia multihamata. In autumn, the relative importance index of A. multihamata was the highest at 3723.09, followed by that of U. duvaucelii (1220.13). U. duvaucelii was the dominant species in both spring and autumn, whereas A. multihamata was the dominant species only in autumn (Table 1).
Figure 2. Temporal and spatial distribution of cephalopod species composition in Zhejiang coastal waters.
From the perspective of the spatial distribution of cephalopod resource density across the entire survey area, the resource density in the southern waters of Zhejiang was relatively high in spring, and the overall distribution increased from northeast to southwest. In autumn, there were two high-density distribution areas in the coastal waters of Zhejiang, i.e., the northern area and the central area, while the resource density in the southern area was lower. The time distribution of cephalopod resource density across the entire survey area revealed 86 stations where cephalopods appeared in spring, accounting for 69.92% of the total survey stations, and the average cephalopod resource density was 223 ind./h. In autumn, cephalopods appeared at 69 stations, accounting for 56.10% of the total survey stations, and the average cephalopod resource density was 543 ind./h (Figure 3).
Figure 3. Spatial and temporal distribution of cephalopod resource density in Zhejiang coastal waters.
The mean value of the Margalef index (D) for the cephalopods at each station in spring in the coastal waters of Zhejiang was 0.34, with a range of 0–1.44; the Shannon-Weiner index (H') was 0.46, with a range of 0–1.77; the Pielou index (J') was 0.37, with a range of 0–1. The mean Margalef index (D) value for the cephalopods at each station in autumn was 0.17, with a range of 0—0.94; the Shannon-Weiner index (H') was 0.26, with a range of 0—1.43; and the Pielou index (J') was 0.23, with a range of 0—1 (Table 2). The diversity indices of the three species mentioned above were all low, and the diversity of the cephalopod community in the coastal waters of Zhejiang was low overall.
From the perspective of spatial scale, the three diversity indices all tended to increase from the coastal waters to the outer seas. In spring, the three diversity indices were relatively uniform and concentrated in the survey sea area, with the highest level in the central area, followed by the northern area. In autumn, the distributions of the three diversity indices in the surveyed sea area showed obvious patch-like dispersion characteristics, and the Pielou index (J') had significant spatial distribution characteristics among the three diversity indices, with the central and southern areas having higher values than the northern areas did. The Margalef index (D) and Shannon–Weiner index (H') of the distribution characteristics were not obvious, with small maximum values existing only at sporadic stations in the northern and central areas (Figure 4).
Figure 4. Spatial distribution of Margalef index, Shannon-Weiner index and Pielou index in Zhejiang coastal waters in different seasons.
The variance inflation factor (VIF) can characterize the degree of correlation between the explanatory variables. In general, a variance inflation factor greater than 10 indicates a strong collinearity problem and that the variable should be discarded before modelling. After testing, the VIF values of each explanatory variable in spring were all less than 10, and the VIF values in autumn, except for that of the BSS, were all less than 10 (Table 3), indicating that there was no serious collinearity problem; therefore, these variables were all included in the model.
Table 3. Multicollinearity test between the distribution of cephalopods and environmental factors in Zhejiang coastal waters.
Based on the results of the VIF test and GAM, the optimal factors were screened to construct the GAM. In spring, sea bottom temperature had significant effects on cephalopod resource density (p<0.05), and water depth and surface dissolved oxygen had significantly affected cephalopod resource density (p<0.01, Table 4). Figure 5 shows the effect diagram of each environmental factor on the cephalopod resource density. The results revealed that the relationships between water depth and surface dissolved oxygen and cephalopod resource density were nonlinear. At a water depth in the range of 10–20 m, the cephalopod resource density was hardly affected by the change in water depth; at a water depth greater than 20 m, the cephalopod resource density increased (Figure 5A). Combined with the interaction of spatial factors and latitude, the sea area south of 29°N was the main cephalopod distribution area, and the resource density increased with increasing water depth (Figure 5B). The suitable range of dissolved oxygen for cephalopods is 6~10 mg/L. When the dissolved oxygen concentration was higher than 7 mg/L, the resource density of the cephalopods decreased rapidly. When the dissolved oxygen concentration was in the range of 8~9.5 mg/L, the resource density of the cephalopods decreased rapidly. The class resource density was minimally affected by changes in dissolved oxygen (Figure 5C). Combined with the interaction of spatial factors and latitude, the high-density area of cephalopod resources was mainly concentrated in the area between 28°N and 27°N, and the dissolved oxygen concentration was between 6 and 7 mg/L (Figure 5D). There was a significant positive linear correlation between sea bottom temperature and cephalopod resource density (Figure 5E). When the sea bottom temperature was between 16 and 20°C, the density of cephalopod resources increased with decreasing latitude (Figure 5F). In autumn, only water depth had a significant effect on the changes in cephalopod resource density (p<0.01), while the remaining indicators did not pass the significance test (Table 4). When the water depth was 10–20 m, the cephalopod resource density was almost unaffected by the change in water depth; when the water depth was 20–40 m or greater than 50 m, the cephalopod resource density increased with increasing water depth (Figure 5G). The combining these factors with the interaction of the spatial factor of latitude indicated that differences in latitude had no significant effect on the cephalopod resource density (Figure 5H).
Figure 5. Response of environmental factors to cephalopod resource density. Spring: (A) cephalopod resource density and water depth, (B) water depth and latitude interaction; (C) Cephalopod resource density and Surface Dissolved oxygen, (D) interaction between Surface Dissolved oxygen and latitude; (E) Cephalopod resource density and bottom seawater temperature, (F) Interaction between bottom seawater temperature and latitude. Autumn: (G) Cephalopod resource density and water depth, (H) water depth and latitude interaction.
The East China Sea, located in the western Pacific Ocean, is rich in Cephalopod species. As reported by Dong in 1978, there were approximately 35 species in the coastal waters, including 29 species from the waters south of the Yangtze River estuary to the waters near Pingtan Island, Fujian (i.e., the current study area). As reported by Zheng in 1994, there were approximately 31 species in the coastal waters, With the passage of time, due to environmental changes and changes in fishing methods, the types of catches are also changing. A total of 20 species of cephalopods belonging to three orders, seven families, and 10 genera were captured in this survey. The number of species has decreased by 31% compared with the historical data which is partly attributable to differences in gear, survey time, and survey area but partly a sign of the crisis in cephalopod species composition. In terms of spatiotemporal variations in cephalopod resource density, the occurrence rate at survey stations in spring (April) was higher than that in autumn (November), but the resource density was significantly lower than that in autumn, and the spatial distribution of cephalopod resource density in spring and autumn was significantly different. On the one hand, most cephalopods are mostly annual molluscs. The population structure is discontinuous and the spawning population is made up of supplementary populations, some species have different breeding groups in different seasons (Song et al., 2009). They are therefore characterised by short life cycles, rapid growth, rapid resource renewal and strong resilience. As reported by Song in 2008, the seasonal variation in the distribution of cephalopods is significant; the peak period of catches is autumn, followed by winter, and the level is low in spring. This feature was consistent with the results of the present study. In spring, there are mostly spawning stocks, which are characterized by small group sizes and larger individual sizes, and the parents are scattered in a radial manner in various coastal fisheries (Changjiangkou fisheries, Zhoushan fisheries, Yushan fisheries, Wentai fisheries, etc.) for breeding and spawning, the spawning season of spawning stocks ends around mid-June (Chen et al., 2013b). From July through August, juvenile creatures embark on a journey from the south to the north, heading towards the foraging areas to seek out food sources. As the season transitions from September to December, these individuals mature into the subadult phase, coinciding with the height of the cephalopod fishing season. Consequently, autumn serves as a critical period for the annual replenishment of the population, marked by a denser concentration of resources and a more aggregated distribution across the region (Chen et al., 2013b; Vidal and Shea, 2023). On the other hand, the migration habits of cephalopods and the marine environment are directly related to the distribution of resource density. Environmental factors such as salinity and temperature play absolute roles in the reproductive and overwintering migration of cephalopods. In a complex and changeable marine environment, spawning broodstock and recruitment stocks seek suitable spawning grounds for migration, which is also the result of long-term natural selection (Chen et al., 2013a).
The replacement of dominant species is also an important factor affecting the changes in distribution and species composition of cephalopod resources. Historically, the East China Sea has been rich in S. japonica, which has the largest number of cephalopods and the largest output in the East China Sea, in the mid-to-late 1990s, its resources were exhausted because of irrational development methods (Chen et al., 2013b). In 1993, with the development of otter trawl operations and squid fishing operations in Zhejiang Province, the operating fisheries expanded to the open seas, and the composition of cephalopod catches also experienced significant changes. Todarodes pacificus, Uroteuthis edulis, Sepia esculenta, Sepia kobiensis, Amphioctopus fangsiao, and Octopus minor have been the main species (Song et al., 2008). Chen et al. (2020) summarized and analysed the changes in the cephalopod communities in the East China Sea on the basis of historical research and survey data. From 1994 to 2000, U. edulis and T. pacificus were the representative dominant species of Loliginidae and Ommastrephidae, respectively. From 2000–2002, S. esculenta replaced S. japonica as the representative dominant species of the Sepiidae family. From 2003 to 2005, A. fangsiao and O. minor became the new dominant species. After 2006, the change in the dominant cephalopod species was more pronounced; the dominant species were members of multiple families, but few species were absolutely dominant. From 2008 to 2009, the dominant species were S. kobiensis, Sepiola birostrata, A. fangsiao, and U. duvaucelii; from 2014 to 2016, the dominant species were A. multihamata, Loligo beka, U. duvaucelii, U. edulis, S. birostrata, and O. minor. In this study, U. duvaucelii was the dominant species in spring and autumn, and A. multihamata was the dominant species in autumn. Compared with those of the historical surveys, the species composition of the cephalopod resources generally remained stable, but the variation in the dominant species was greater, and the number of dominant species was relatively small. Since 2014, A. multihamata has been regarded as the dominant species among endemic small-scale fishery species in China in recent surveys and plays an important role in fishery ecosystems as important bait species for marine fishes (Shi et al., 2008). The dominant species were replaced by species of minor economic importance, indicating that the dominant species are miniaturized and of low value. Traditional economically important species are still overexploited, which further demonstrates the significance of protecting and restoring traditional and economically important cephalopod resources.
Notably, the survey in spring revealed that S. japonica has a certain degree of dominance in the cephalopod community as important species in both spring and autumn; its relative importance index in spring is even greater than that of A. multihamata. S. japonica was once a traditionally dominant species of economic importance in the East China Sea, but its resources have severely declined since the late 1980s, and it has since been used only as a bycatch. Subsequently, S. esculenta, S. kobiensis, and Sepia pharaonis replaced S. japonica as the main capture targets, with S. esculenta and S. kobiensis as the dominant species. These findings suggest that different species of the same category compete directly for similar niches and that the amount of resources also ebbs and flows (Chen et al., 2020). In recent years, S. japonica has been regarded as one of the five most important species for fishing ground restoration and revitalization in Zhejiang Province. The recovery of its resources has benefited from ecological restoration and release and the creation of spawning grounds (Wang et al., 2016). Research has shown that human intervention is helpful for the recovery of cephalopod resources. However, further research is needed on whether resource restoration via human intervention affects other species in the same category or other species with highly similar niches.
The results of this study revealed that the three alpha diversity indices of the cephalopod communities in the coastal waters of Zhejiang were low, mainly due to the high-density clustering of individual dominant species (e.g., A. multihamata) leading to a decrease in cephalopod diversity in the study waters and the fact that A. multihamata migrates only short distances. The migration range of octopods is even smaller, and O. minor only moves between the upper and lower intertidal zones (Li et al., 2006; Chen et al., 2013a). Moreover, there are fewer dominant species in the cephalopod community, and the degree of dominance is higher than that of other species in the community, which is also one reason for the low community biodiversity index (Ryder et al., 1981; Lu et al., 2018).
The spatial and seasonal differences in the cephalopod community were significant. From the perspective of the spatial distribution of the diversity index according to the distance from the coastline, the three alpha diversity indices were characterized by significant differences, uneven distributions, and high values at local stations. Judging from the seasonal variation in the diversity index, the spatial distribution trends were generally similar, which may be attributed to the replacement of some cephalopod species (squids, cuttlefish and octopuses) by other cephalopod species from different areas due to the living habits and dispersal ability (migration) of cephalopods. Most cephalopods clearly migrated. Migration is an important part of the life cycle of cephalopods and an adaptation to the continuation and reproduction of the species (Chen et al., 2013b). The seasonal migration of cephalopods includes reproductive migration, overwintering migration, and feeding migration. Water temperature is the main external factor that influences the reproductive migration and community diversity distribution of cephalopods. When the water temperature increases, gonad maturation accelerates, and reproductive migration gradually occurs. Overwintering migration is also closely related to water temperature; the speed at which the water temperature decreases directly affects the timing of overwintering migration (Semmens et al., 2007). For example, April to June is the breeding season of S. japonica, and the overwintering populations undergo reproductive migration from the outer sea area to the shallow sea area. At the end of autumn and the beginning of winter, the new generation migrates from the shallow sea area to the outer sea area for wintering (Xu et al., 2024). U. edulis breeds every year. In the spring, as the coastal sea temperature increases, it moves from the wintering ground outside the continental shelf of the East China Sea to the northwestern coastal area for breeding and feeding migration. From June to August, due to the action of high-frequency easterly and southeasterly winds, the Taiwan Warm Current increases in strength, and U. edulis continues to move westwards, intensively concentrating in the coastal waters of the East China Sea for breeding and feeding migration. In autumn, as the water temperature gradually decreases, U. edulis begins to gradually move south and overwinters in the East China Sea in batches (Chen et al., 2013b).
Under different environmental conditions, due to different species compositions and proportions, species exhibit different habitat specializations or preferences (Keast, 1978; Rivas-Salvador et al., 2019). Most cephalopods are ecological opportunists. The annual life cycle and flexible reproductive strategy enable these animals to respond rapidly to environmental changes (Rocha et al., 2001; Rodhouse, 2010). Cephalopods are highly sensitive to changes in environmental conditions and various spatiotemporal scales. Under favourable environmental conditions, populations can expand rapidly, but unfavourable environments can cause population decline. Therefore, the distribution and resource fluctuations of cephalopod populations are variable on both spatial and temporal scales (Pierce et al., 2008). Wang et al. (2023) reported that latitude is a very important environmental factor affecting the diversity and distribution of 18 cephalopods in the northwestern Pacific Ocean. The GAM is currently the most commonly used model to study the relationships between the marine environment and fish distribution. Compared with traditional models, the GAM can explain the nonlinear relationships between the dependent and independent variables well. For example, Xiang et al. (2024) used the GAM to study the relationships between the cephalopod community and environmental factors on the continental slope of the Bering Sea. Puerta et al. (2015) used the GAM to reveal the influences of environmental and nutritional driving factors in the western Mediterranean Sea on the distribution of cephalopods. Pang et al. (2022) used the GAM to analyse the effects of environmental factors in Haizhou Bay of the Yellow Sea on the distribution and abundance of cephalopods. In this study, the GAM was used to investigate the effects of single-factor environmental factors and spatial interactions (latitude) on cephalopods. In spring, the distribution of cephalopod communities was affected mainly by water depth, surface dissolved oxygen, and sea bottom temperature, whereas in autumn, the distribution of cephalopod communities was affected mainly by a single factor, water depth. As an important factor affecting the distribution of cephalopod communities, water depth affects the habitat, reproduction, and migration of cephalopods. Most cephalopods migrate from deep water to shallow water to spawn and are concentrated in reefs and sandy seas with a water depth of 20~25 m, shallow seas overgrown with corals and seaweed. After October, owing to the cold air from the north moving south, the spawning of most cephalopods ends, and the broodstock dies in succession. The individuals who did not spawn and the recruitment stocks begin to swim to the deep-water area of the open sea. Moreover, as the mantle length increases during cephalopod development, the habitat water layer gradually decreases, which further increases the probability of being trawled (the main fishery production area is the area outside the line of the area closed to trawl fishery) (Ryder et al., 1981).
Insufficient or excessive dissolved oxygen concentrations in the water can both slow the growth of organisms and induce a stress response. For example, fish cannot survive for a long period of time in water with dissolved oxygen concentrations lower than 5 mg/L (Zhao and Shen, 2024). Owing to their short life cycle, cephalopods have high growth rates and food conversion rates, and their predation, feeding, and reproduction all require large amounts of oxygen; therefore, dissolved oxygen has a great effect on the distribution of cephalopods (Rodhouse et al., 2014). Figures 5C and 5D show that the relationship between the dissolved oxygen concentration and cephalopod resource distribution was nonlinear. When the dissolved oxygen concentration was higher than 7 mg/L, the cephalopod resource density decreased rapidly. Cephalopods have strong environmental adaptability and can optimize energy accumulation and metabolic distribution during growth and reproduction (Lin et al., 2017; Wu et al., 2024). Since spring is the cephalopod spawning season, compared with those in the larval and adult stages, the energy intake level and individual metabolic rate are lower in the spawning stock as a type of optimization and adaptation to low dissolved oxygen levels. For example, the optimum dissolved oxygen concentration for the spawning stock of the O. minor is 6~7 mg/L, and the optimum range of dissolved oxygen for the spawning stock of the S. japonica is 6~9 mg/L (Chen et al., 2019, 2021; Wu et al., 2023).
Temperature is critical to all key life stages of cephalopods, including egg laying, hatching, growth, and development, and to population dynamics and migration (Pierce et al., 2008; Schickele et al., 2021). Cephalopods are sensitive to water temperature changes. For example, the spatial distribution of the resource density of Loligo spp. in the North Sea was positively correlated with both sea bottom temperature (SBT) and sea surface temperature (SST), and the resource density in summer was negatively correlated with SST (Pierce et al., 2008). In April each year, the coastal water temperature of the East China Sea gradually increases, and the isothermal line is roughly distributed along the coastline. A strong temperature front is formed between the Taiwan Warm Current and coastal waters, with a high general distribution pattern in the southern region and low in the northern region. Water temperature changes drive the seasonal migration of cephalopods. Studies have shown that the relationships among cephalopod abundance and migration and the sea temperature may be related to large-scale climate change (Leporati et al., 2007). Seasonal temperature changes have significant effects on the growth of cephalopods and is the main factor affecting the distribution of cephalopods. The climate-driven warming of the coastal waters of China has already exceeded the rising global average temperature. Although a small increase in temperature may be conducive to the growth and reproduction of some species, changes in the marine environment caused by climate warming are irreversible, and a rise in temperature accelerates the growth rate of cephalopods, further affecting the body shape and age structure, spawning dynamics, and larval survival rates of cephalopods.
The original contributions presented in the study are included in the article/supplementary material. Further inquiries can be directed to the corresponding authors.
JL: Conceptualization, Data curation, Formal analysis, Project administration, Writing – original draft, Writing – review & editing. YDZ: Conceptualization, Data curation, Project administration, Writing – original draft, Writing – review & editing. TW: Conceptualization, Formal analysis, Visualization, Writing – original draft, Writing – review & editing. FC: Methodology, Resources, Writing – original draft, Writing – review & editing. KX: Formal analysis, Methodology, Writing – original draft, Writing – review & editing. GF: Formal analysis, Methodology, Visualization, Writing – original draft, Writing – review & editing. YZZ: Investigation, Methodology, Visualization, Writing – original draft, Writing – review & editing.
The author(s) declare financial support was received for the research, authorship, and/or publication of this article. This study was supported by National Key R & D project of China (2023YFD2401905, 2019YFD0901205) and National Natural Science Foundation of China (31702346).
The authors express their gratitude to the staff of the Zhejiang Marine Fisheries Research Institute.
The authors declare that the research was conducted in the absence of any commercial or financial relationships that could be construed as a potential conflict of interest.
The author(s) declare that no Generative AI was used in the creation of this manuscript.
All claims expressed in this article are solely those of the authors and do not necessarily represent those of their affiliated organizations, or those of the publisher, the editors and the reviewers. Any product that may be evaluated in this article, or claim that may be made by its manufacturer, is not guaranteed or endorsed by the publisher.
Ainsworth G. B., Pita P., Garcia Rodrigues J., Pita C., Roumbedakis K., Fonseca T., et al. (2023). Disentangling global market drivers for cephalopods to foster transformations towards sustainable seafood systems. People Nature. 5, 508–528. doi: 10.1002/pan3.10442
Boavida-Portugal J., Guilhaumon F., Rosa R., Araújo M. B. (2022). Global patterns of coastal cephalopod diversity under climate change. Front. Mar. Science. 8. doi: 10.3389/fmars.2021.740781
Chen F., Li N., Fang Z., Liang J., Zhang H. L., Zhou Y. D., et al. (2021). Habitat distribution change pattern of Uroteuthis edulis during spring and summer in the coastal waters of Zhejiang Province. J. Shanghai Ocean Univers. 30, 847–855. doi: 10.12024/jsou.20201203245
Chen F., Li N., Fang Z., Shen F. Y., Zhang H. L., Zhou Y. D. (2019). Relationship between spatial-temporal distribution of long-arm octopus Octopus minor and oceanographic environmental factors along north coast of Zhejiang province during spring and summer. J. Shanghai Ocean Univers. 28, 438–447. doi: 10.12024/jsou.20181202486
Chen F., Qu J. Y., Fang Z., Zhang H. L., Zhou Y. D., Liang J. (2020). Variation of community structure of Cephalopods in spring and autumn along the coast of Zhejiang Province. J. fish. China. 44, 1317–1328. doi: 10.11964/jfc.20190211664
Chen X. J., Han B. P., Liu B. L., Lu H. J. (2013a). World cephalopod resources and fisheries (Beijing: Ocean Press), 1–74.
Chen X. J., Wang Y. G., Qian W. G. (2013b). Important Cephalopod resources and fisheries in the coast of China (Beijing: Science Press), 1–147.
Cui Y. H., Liu S. D., Xu B. D., Ji Y. P., Zhang C. L., Ren Y. P., et al. (2023). Potential Effects of Climate Warming on Spatial Distribution of Octopus ocellatus Inhabiting Haizhou Bay in Spring. (Periodical of Ocean University of China). 53, 42–48. doi: 10.16441/j.cnki.hdxb.20220255
Dong Z. Z. (1978). On the geographical distribution of the cephalopods in the Chinese waters. Oceanol. Limnol. Sinica. 9, 108–116. doi: CNKI:SUN:HYFZ.0.1978-01-013
Doubleday Z. A., Prowse T. A., Arkhipkin A. I., Pierce G. J., Semmens J. M., Steer M. A., et al. (2016). Global proliferation of cephalopods. Curr. Biol. 26, R406–R407. doi: 10.1016/j.cub.2016.04.002
Hastie T. J., Tibshirani R. J. (1990). Generalized additive models. London: Chapman Hall. 1-40. doi: 10.1201/9780203753781
Keast A. J. (1978). Trophic and spatial interrelationships in the fish species of an Ontario temperate lake. Environ. Biol. Fishes. 3, 7–31. doi: 10.1007/BF00006306
Leporati S. C., Pecl G. T., Semmens J. M. (2007). Cephalopod hatchling growth: the effects of initial size and seasonal temperatures. Mar. Biol. 151, 1375–1383. doi: 10.1007/S00227-006-0575-Y
Li S. F., Yan L. P., Li H. Y., Li J. S., Chen J. H. (2006). Spatial distribution of cephalopod assemblages in the Region of the East China Sea. J. Fish. Sci. China. 13, 936–944. doi: 10.3321/j.issn:1005-8737.2006.06.011
Lin D., Chen X., Wei Y., Chen Y. (2017). The energy accumulation of somatic tissue and reproductive organs in post-recruit female Illex argentinus and the relationship with sea surface oceanography. Fish. Res. 185, 102–114. doi: 10.1016/J.FISHRES.2016.09.023
Lu Z. H., Zhu W. B., Xu K. D., Zhou Y. D., Dai Q., Lu K. E. (2018). Community Structure of Snails and its Relationship with Environmental Factors along Zhejiang Coast in Spring. Acta Hydrobiol. Sinica. 42, 606–615. doi: 10.7541/2018.076
Magurran A. E. (2021). Measuring biological diversity. Curr. Biol. 31, R1174–R1177. doi: 10.1016/j.cub.2021.07.049
Pang Y., Tian Y., Ju P., Sun P., Ye Z., Liu Y., et al. (2022). Change in cephalopod species composition in the overexploited coastal China seas with a closer look on Haizhou Bay, Yellow Sea. Region. Stud. Mar. Science. 53, 102419. doi: 10.1016/j.rsma.2022.102419
Pielou E. C. (1966). The measurement of diversity in different types of biological collections. J. Theor. Biol. 13, 131–144. doi: 10.1016/0022-5193(66)90013-0
Pierce G. J., Valavanis V. D., Guerra Á., Jereb P., Orsi-Relini L., Bellido J. M., et al. (2008). A review of cephalopod–environment interactions in European Seas. Hydrobiologia 612, 49–70. doi: 10.1007/s10750-008-9489-7
Pinkas L., Oliphant M. S., Iverson I. L. (1971). Food habits of albacore, bluefin tuna, and bonito in California waters. (State of California: Dept. of Fish and Game).
Puerta P., Hunsicker M. E., Quetglas A., Álvarez-Berastegui D., Esteban A., González M., et al. (2015). Spatially explicit modeling reveals cephalopod distributions match contrasting trophic pathways in the Western Mediterranean sea. PloS One 10, e0133439. doi: 10.1371/journal.pone.0133439
Rivas-Salvador J., Hořák D., Reif J. (2019). Spatial patterns in habitat specialization of European bird communities. Ecol. Indic. 105, 57–69. doi: 10.1016/J.ECOLIND.2019.05.063
Rocha F., Guerra Á., González Á.F. (2001). A review of reproductive strategies in cephalopods. Biol. Rev. 76, 291–304. doi: 10.1017/S1464793101005681
Rodhouse P. G. (2010). Effects of environmental variability and change on cephalopod populations: an Introduction to the CIAC '09 Symposium special issue. Ices J. Mar. Science. 67, 1311–1313. doi: 10.1093/ICESJMS/FSQ096
Rodhouse P. G., Pierce G. J., Nichols O. C., Sauer W. H., Arkhipkin A. I., Laptikhovsky V. V., et al. (2014). Environmental effects on cephalopod population dynamics: implications for management of fisheries. Adv. Mar. Biol. 67, 99–233. doi: 10.1016/B978-0-12-800287-2.00002-0
Ryder R. A., Kerr S. R., Taylor W. W., Larkin P. A. (1981). Community consequences of fish stock diversity. Can. J. Fish. Aquat. Sci. 38, 1856–1866. doi: 10.1139/F81-231
Schickele A., Francour P., Raybaud V. (2021). European cephalopods distribution under climate-change scenarios. Sci. Rep. 11, 3930. doi: 10.1038/s41598-021-83457-w
Semmens J. M., Pecl G. T., Gillanders B. M., Waluda C. M., Shea E. K., Jouffre D., et al. (2007). Approaches to resolving cephalopod movement and migration patterns. Rev. Fish Biol. Fish. 17, 401–423. doi: 10.1007/s11160-007-9048-8
Shi H. X., Jiao H. F., You Z. J. (2008). Research progress in Cephalopods’ reproductive biology. Water Fish. 28, 5–8. doi: 10.15928/j.1674-3075.2008.01.038
Song H. T., Ding T. M., Xu K. D. (2008). The quantity distribution and sustainable use of cephalopod in the East China sea. Period. Ocean Univ. China. 38, 911–915. doi: 10.16441/j.cnki.hdxb.2008.06.009
Song H. T., Ding T. M., Xu K. D. (2009). Commercial Cephalopod Resources in East China Sea (Beijing: Ocean Press), 1–13.
Spellerberg I. F., Fedor P. J. (2003). A tribute to Claude Shannon, (1916-2001) and a plea for more rigorous use of species richness, species diversity and the 'Shannon-Wiener' Index. Global Ecol. Biogeogr. 12, 177–179. doi: 10.1046/j.1466-822X.2003.00015.x
Vidal E. A., Shea E. K. (2023). Cephalopod ontogeny and life cycle patterns. Front. Mar. Science. 10. doi: 10.3389/fmars.2023.1162735
Walsh W. A., Kleiber P. (2001). Generalized additive model and regression tree analyses of blue shark (Prionace glauca) catch rates by the Hawaii-based commercial longline fishery. Fish. Res. 53, 115–131. doi: 10.1016/S0165-7836(00)00306-4
Wang R., Zhang R., Song P., Liu S., Li Y., Li H. (2023). Diversity and distribution of 18 cephalopod species, and their link with some environmental factors in the NW Pacific. Diversity 15, 694. doi: 10.3390/d15050694
Wang W. D., Liang J., Bi Y. X., Feng M. P., Zhou S. S., Yu B. C. (2016). Status and prospects of marine ranching construction in Zhejiang Province. J. Zhejiang Ocean Univ. (Natural Sci. Edition). 35, 181–185. doi: 10.3969/j.issn.1008-830X.2016.03.001
Wang X. H., Du F. Y., Qiu Y. S., Li C. H., Sun D. R., Jia X. P. (2010). Variations of fish species diversity, faunal assemblage, and abundances in Daya Bay in 1980-2007. Chin. J. Appl. Ecol. 21, 2403–2410. doi: 10.13287/j.1001-9332.2010.0350
Wu H. Z., Chen Z. K., Lin D. M. (2024). Effects of marine environment on fecundity characteristics of female argentine shortfin squids. South China Fish. Science. 20, 130–140. doi: 10.12131/20230161
Wu T., Liang J., Zhou Y. D., Xuan W. D., Fang G. J., Zhang Y. Z., et al. (2023). The factors driving the spatial variation in the selection of spawning grounds for Sepiella japonica in offshore Zhejiang Province, China. Fishes 9, 20. doi: 10.3390/fishes9010020
Xiang S. Y., Yu W., Jin P. C., Sheng Y. L., Dai Y. L., Li W. X. (2024). Cephalopod community structure and its relationship with environmental factors in the Bering Sea slope. J. Shanghai Ocean Univers. 33, 480–492. doi: 10.12024/jsou.20230404138
Xu M., Liu S., Zhang H., Li Z., Song X., Yang L., et al. (2024). Seasonal Analysis of Spatial Distribution Patterns and Characteristics of Sepiella maindroni and Sepia kobiensis in the East China Sea Region. Animals 14, 2716. doi: 10.3390/ani14182716
Zhao F. S., Shen Y. (2024). A review: research progress on effects of hypoxia on marine animals. Chin. J. Fish. 37, 109–117. doi: 1005-3832(2024)04-0109-09
Zheng Y. J. (1994). Development and utilization of situation and rational utilization with cephalopod resources in the East China Sea. Mar. Fish. 16, 199–203.
Keywords: cephalopods, spatial pattern, seasonal pattern, zhejiang coastal waters, environmental factors
Citation: Liang J, Zhou Y, Wu T, Chen F, Xu K, Fang G and Zhang Y (2025) Spatiotemporal distribution patterns of cephalopods and their responses to changes in the marine environment in the coastal waters of Zhejiang, China. Front. Mar. Sci. 12:1510574. doi: 10.3389/fmars.2025.1510574
Received: 13 October 2024; Accepted: 10 January 2025;
Published: 28 January 2025.
Edited by:
Wei Huang, Ministry of Natural Resources, ChinaReviewed by:
Francisco Javier Rocha, University of Vigo, SpainCopyright © 2025 Liang, Zhou, Wu, Chen, Xu, Fang and Zhang. This is an open-access article distributed under the terms of the Creative Commons Attribution License (CC BY). The use, distribution or reproduction in other forums is permitted, provided the original author(s) and the copyright owner(s) are credited and that the original publication in this journal is cited, in accordance with accepted academic practice. No use, distribution or reproduction is permitted which does not comply with these terms.
*Correspondence: Yongdong Zhou, enlkNTExQDEyNi5jb20=; Tian Wu, d3V0aWFuNTMwQDEyNi5jb20=
Disclaimer: All claims expressed in this article are solely those of the authors and do not necessarily represent those of their affiliated organizations, or those of the publisher, the editors and the reviewers. Any product that may be evaluated in this article or claim that may be made by its manufacturer is not guaranteed or endorsed by the publisher.
Research integrity at Frontiers
Learn more about the work of our research integrity team to safeguard the quality of each article we publish.