- 1Department of Environmental Science and Engineering, Fudan University, Shanghai, China
- 2College of Biological and Environmental Engineering, Zhejiang Shuren University, Hangzhou, China
Introduction: Coastal wetlands play an important role in the global ecosystem, yet they are increasingly facing ecological degradation due to pollution and the spread of invasive Spartina alterniflora (S. alterniflora). Among the environmental challenges, microplastics (MPs) contamination in S. alterniflora-invaded coastal wetlands is of growing concern. However, the effects of MPs pollution in S. alterniflora-invaded coastal wetlands are not clear.
Methods: This study investigated the effects of S. altemiflora invasion on the distribution of MPs and the effects of MPs pollution on the physicochemical properties of sediments and the structure of microbial communities in the Tiaozini wetland of Jiangsu Province, China.
Results and discussion: The total MPs abundance of the S. altemiflora single-plant area was the highest (16.338 x103 items/kg d.w.). Polyurethane and Chlorinated polyisoprene are typical MPs types in the study area. Polyurethane exhibits high abundance at all sampling sites, while Chlorinated polyisoprene is one of the dominant MPs types in the single-plant and sub-mature area of S. alterniflora. The results showed that the invasion of S. alterniflora promoted the accumulation of MPs in the sediments of the coastal wetland. This accumulation increased the total organic carbon (TOC), available phosphorus (AP) and total nitrogen (TN) contents. Moreover, MPs pollution increased the ALP and SUC enzyme activities of sediment. In addition, MPs contamination increased the abundance of Proteobacteria and Bacteroidota in the sediments. This study highlights the complex interactions between MPs pollution and S. alterniflora invasion, providing the foundation for understanding the synergistic impacts of these stressors on coastal wetland ecosystems.
1 Introduction
As a globally important environmental type, coastal wetlands have complex and diverse ecosystems (Li et al., 2021; Wang et al., 2023). The coastal wetlands play an important role in various material cycles (Wu et al., 2022). Due to the proximity of coastal wetlands to the “ocean”, coastal wetlands may experience more serious pollutant aggregation than other wetlands (Adomako et al., 2024). The invasion of Spartina alterniflora (S. alterniflora) and the accumulation of pollutants such as microplastics (MPs) are becoming increasingly prominent.
S. alterniflora is native to the Atlantic coast of North and South America. Due to its strong adaptability to environmental factors such as salinity and climate (Cao et al., 2021; Lin Y. et al., 2022), S. alterniflora has invaded large areas globally. The invasion of S. alterniflora has seriously affected the physicochemical properties, microbial community structure, and enzyme activities of the soil. Bai et al. (2017) found that the invasion of S. alterniflora significantly reduced the soil nutrient content, and Zhang et al. (2021) found that organic carbon content in wetlands invaded by S. alterniflora significantly increased compared to wetlands dominated by native plants. Moreover, S. alterniflora invasion changed the microbial communities structure of wetlands (Cao et al., 2021). Lin et al. (2021) also found that S. alterniflora invasion significantly affected the composition, diversity, and specific functions of soil microorganisms in different seasons.
With the rapid development of human society, plastic products have been widely used because of their excellent properties such as low cost and stable property. However, this has led to a significant environmental issue. It is estimated that nearly 11 million tons of plastic waste enter the oceans annually (Geyer et al., 2017). By 2050, the total amount of plastic debris in the ocean is expected to exceed the total amount of fish (Gallo et al., 2018). Behind the massive use of plastic products, MPs have emerged as a “new pollutant”, appearing in various environments and organisms, such as water bodies (Turan et al., 2021), sediments (Cincinelli et al., 2021), birds (Tokunaga et al., 2023), and plants (Yin et al., 2021; Adomako et al., 2024). However, the impact of MPs pollution on the physicochemical properties, enzyme activities, and microbial communities of sediments at different levels of S. alterniflora invasion is still unclear.
This study focuses on the Tiaozini coastal wetland, which has been invaded by S. alterniflora, and aims to investigate: (1) the distribution characteristics and abundance of MPs; (2) the effects of MPs on the physicochemical properties and enzyme activities of the coastal wetland sediments; (3) the influence of MPs on the microbial communities structure in the coastal wetland invaded by S. alterniflora. The results will provide the data support for understanding how invasive species and pollution interact to affect coastal wetland.
2 Materials and Methods
2.1 Study area and sample collection
The research area is located in the Tiaozini World Natural Heritage Site in Dongtai City, Jiangsu Province, China, and the study area ranges from 32°49′~32°51′ N, 120°57′~120°59′ E (Figure 1). According to the types of plants and distribution of S. alterniflora, the sampling sites were mainly located in the Suaeda area (SA) and the S. alterniflora none-plant area (NP), the S. alterniflora single-plant area (SP), the S. alterniflora sub-mature area (SM), and the S. alterniflora mature area (MA). Three sampling sites were set up in different areas, and the distance between the adjacent sampling sites was about 1 km. Sediment samples were collected at the depth of 0-20 cm at each sampling site and stored in the dark at 4°C.
2.2 MPs extraction and analysis
Weigh 30 g of the sample and add 60 mL of 1.7-1.8 kg/L ZnCl2 (Guaranteed reagent), stirred thoroughly for 2min, and leave it for 12 h. After that, the suspension was transferred to another beaker and 60 mL of 30% H2O2 was added, stirred thoroughly, and after that, left to stand for 24 h. The solution was vacuum pumped and filtered, and the filter membrane was immersed into ethanol solution for ultrasonication clearing the membrane with ethanol several times. The concentrated ethanol solution was dropped on the high reflective glass until the ethanol completely evaporated. Then the MPs were measured using a laser infrared imaging system (8700LDIR, Agilent, USA).
2.3 Identification of physical and chemical properties of soil
After the dried sediment samples were passed through a 2 mm sieve and impurities were removed, the physicochemical properties of the samples were determined, including total organic carbon (TOC) (Kong et al., 2015), total inorganic carbon (TIC), total nitrogen (TN) (Wang and Lin, 2023), ammoniacal nitrogen (NH4+-N) (Zhang et al., 2023), total phosphorus (TP), available phosphorus (AP) (Ding et al., 2023), total potassium (TK) (Yu et al., 2022), available potassium (AK) (Yu et al., 2022), and electrical conductivity (EC), and the activities of urease (URE), alkaline phosphatase (ALP) (Bai et al., 2017), catalase (CAT) (Chen et al., 2022), and sucrose (SUC) (Bai et al., 2017).
2.4 Analysis of microbial communities
In this study, we analyzed the abundance and structure of bacterial community composition in sediments by PCR amplification of 16S rDNA. 16S rDNA high-throughput sequencing was conducted by Shanghai Weipu Testing Technology Group Co., Ltd. (Shanghai, China). DNA was extracted from the sediment by 1% agar gel assay and amplified using a PCR instrument (GeneAmp® 9700, ABI, USA) and TransStart Fastpfu DNA polymerase (AP221-02, Allstyle Gold, China). The PCR products were quantified using a blue fluorescence quantification system (QuantiFluor™ -ST, Promega, USA). Then, Illumina libraries were constructed and Illumina sequencing was completed. After splicing the PE reads obtained from Illumina sequencing according to the overlap relationship, the OTU information of bacteria in the sediments was obtained.
2.5 Statistical analysis
The data were mainly organized and analyzed by Microsoft Excel 2019; the statistical analysis of data was realized by IBM SPSS 22.0; and the plotting of experimental results was completed by Origin 2023.
3 Results and discussion
3.1 Distribution characteristics of MPs pollution in coastal wetlands
The abundance of MPs in different areas is shown in Table 1. The MPs abundance in the mature area of S. alterniflora and the Suaeda growing area was 0.790 ×103-1.378 ×103 items/kg d.w. and 0.199 ×103-1.851 ×103 items/kg d.w., respectively, while the MPs abundance in the none-plant area (0.466 ×103-5.477 ×103 items/kg d.w.). The total MPs abundance is as follows: SP (16.338 ×103 items/kg d.w.)> SM (11.608 ×103 items/kg d.w.)> NP (7.491 ×103 items/kg d.w.) > SA (3.190 ×103 items/kg d.w.)> MA (3.145 ×103 items/kg d.w.). Tidal action may be an important reason for the seriousness of MPs pollution in non-plant areas compared with the mature area of S. alterniflora and Suaeda salsa (Wang et al., 2021). In comparison to the none-plant area, the mature area of S. alterniflora and Suaeda area are located farther from the coastline, potentially mitigating the influence of MPs originating from seawater on these areas. Furthermore, the S. alterniflora populations in the single-plant area and sub-mature area serve as the buffer, reducing the impact of MPs transported by seawater on the mature area of S. alterniflora and Suaeda area. The abundance of MPs in sediments increased significantly from 0.466 ×103-5.477 ×103 items/kg d.w. to 2.505 ×103-5.306 ×103 items/kg d.w. with the intensification of the invasion of S. alterniflora from the none-plant area to the sub-mature area of S. alterniflora, suggesting that the invasion of S. alterniflora exacerbated MPs contamination of the coastal wetland. Compared with the estuary of the Yangtze River (Peng et al., 2017) and the southern Yellow Sea, East China Sea (Zhang et al., 2019), the abundance of MPs was found to be significantly higher in this study area. This also indicates that coastal wetlands are more susceptible to MPs contamination than marine sediments. In addition, MPs contamination caused by the invasion of S. alterniflora has also exceeded the status of MPs contamination in sediments in many other countries (Rodrigues et al., 2018; Eo et al., 2019).
It was found that the MPs types varied among the S. alterniflora invasion area and the Suaeda area (Figure 2). The types of MPs were more diverse in the non-plant area, with 16 types, compared to the Suaeda area, which had 11 types. This phenomenon is closely related to the influence of tides (Anonymous, 2016). Tides continuously bring various types of MPs from the ocean, which accumulate in the wetland areas near the coastline as the tide rises and falls. As a result, area closer to the shore (non-plant area) exhibit a greater diversity of MPs types. In contrast, the Suaeda area, being farther from the ocean, is less affected by tidal-driven MPs influx, leading to a more limited variety of MPs. Tides not only serve as the primary pathway for the transport of MPs, but also play a key role in their diversification. The MPs types found gradually decreased from the non-plant area to the mature area of S. alterniflora growth (Figure 2). In the Suaeda growth area, Polyurethane, Fluororubber, and Polyethylene terephthalate were the dominant MPs types. In the non-plant area, Acrylate copolymer, Polyurethane, and Polybutadiene were the dominant MPs types. In the single-plant area, Polyurethane, Chlorinated polyisoprene, and Polytetrafluoroethylene were the dominant MPs types. Polyurethane, Polymethyl methacrylate, and Chlorinated polyisoprene were the dominant MPs types in the sub-mature area. Whereas, Polypropylene, Polyurethane, and Chlorinated polyethylene were the dominant MPs types in the mature area. Among them, Polyurethane was present in all sampling areas, and Chlorinated polyisoprene was common to both the single plant and sub-mature areas of S. alterniflora. Peng et al. (2024) detected various types of MPs in Yancheng coastal wetlands, including polypropylene and polyethylene terephthalate (Peng et al., 2024). Chen et al. found that polypropylene, polyethylene, and polyethylene terephthalate are the main types of MPs affecting coastal wetlands in the Pearl River Delta, China (Chen and Lee, 2021). Additionally, Rasta et al. (2020) discovered that polypropylene and polyester are the predominant types of MPs in Anzali Wetland (Rasta et al., 2020).
Therefore, in addition to the natural deposition of MPs in seawater (Xu et al., 2023b) and other factors, S. alterniflora invasion influenced the deposition process of MPs in coastal wetlands. This suggests that S. alterniflora invasion not only changes the abundance of MPs in coastal wetlands, but also changes the distribution of different types of MPs in coastal wetlands.
3.2 Impacts of MPs on the physicochemical properties of coastal wetland sediments
The effects of MPs pollution on the physicochemical properties of coastal wetland sediments are shown in Figure 3. The TOC content in the non-plant area was lower than that in the invasive single-plant and mature areas of S. alterniflora and the Suaeda area. This may be because the non-plant area is more affected by seawater, and the effects of tides and water-salt balance reduce the sediment TOC content (Kong et al., 2015). The higher TOC content in the single-plant area than in the non-plant area may result from MPs contamination exacerbated by the invasion of S. alterniflora. Unlike the single-plant area, the MPs content was significantly lower in the sub-mature area. This may be due to the greater soil disturbance by the root system of S. alterniflora in this area, which promotes the TOC exchange between sediment and tidal water, enhances sediment respiration, and thus reduces the sediment TOC content in this area (Xu et al., 2023a). As mentioned earlier, MPs content was lower and not significantly different between the mature and Suaeda areas. Therefore, the reason for the higher sediment TOC content in the mature and Suaeda areas may be due to the higher level of plant development in this region (Mao et al., 2009). The TIC concentration of sediments from different study areas had a similar trend to the TOC content. The TK and AK contents of sediments play an important role in physiological processes such as water regulation, photosynthesis, and stress tolerance in plants (Yu et al., 2022). However, there was no significant difference in the TK and AK contents in different study areas. AP content was significantly lower in the non-plant area, single-plant area, and sub-mature area. In agreement with the above studies, this may be due to the fact that the AP content in the non-plant, single-plant, and sub-mature areas was more affected by tides and root disturbance, among others. Whereas, the slight increase in AP content from the non-plant area to the sub-mature area may be due to MPs increasing the organic matter content of the sediments (Ding et al., 2023). The trend of TN in different study areas is similar to that of TOC, which may be due to the fact that TN content in sediments mainly depends on the bioaccumulation and hydrolysis of organic matter (Wang and Lin, 2023). Therefore, MPs pollution has an important effect on TOC, AP and TN content in coastal wetlands. Qin et al. (2015) also found that TOC and TN exhibit similar trends. The effect on NH4+-N content, on the other hand, maybe mainly influenced by the invasion of S. alterniflora. The invasion of S. alterniflora increased the oxygen content in the sediments, which in turn increased the conversion of NH4+-N (Zhang et al., 2023). In addition, it was found that MPs pollution resulted in differences in the EC between the Suaeda area and the S. alterniflora area.
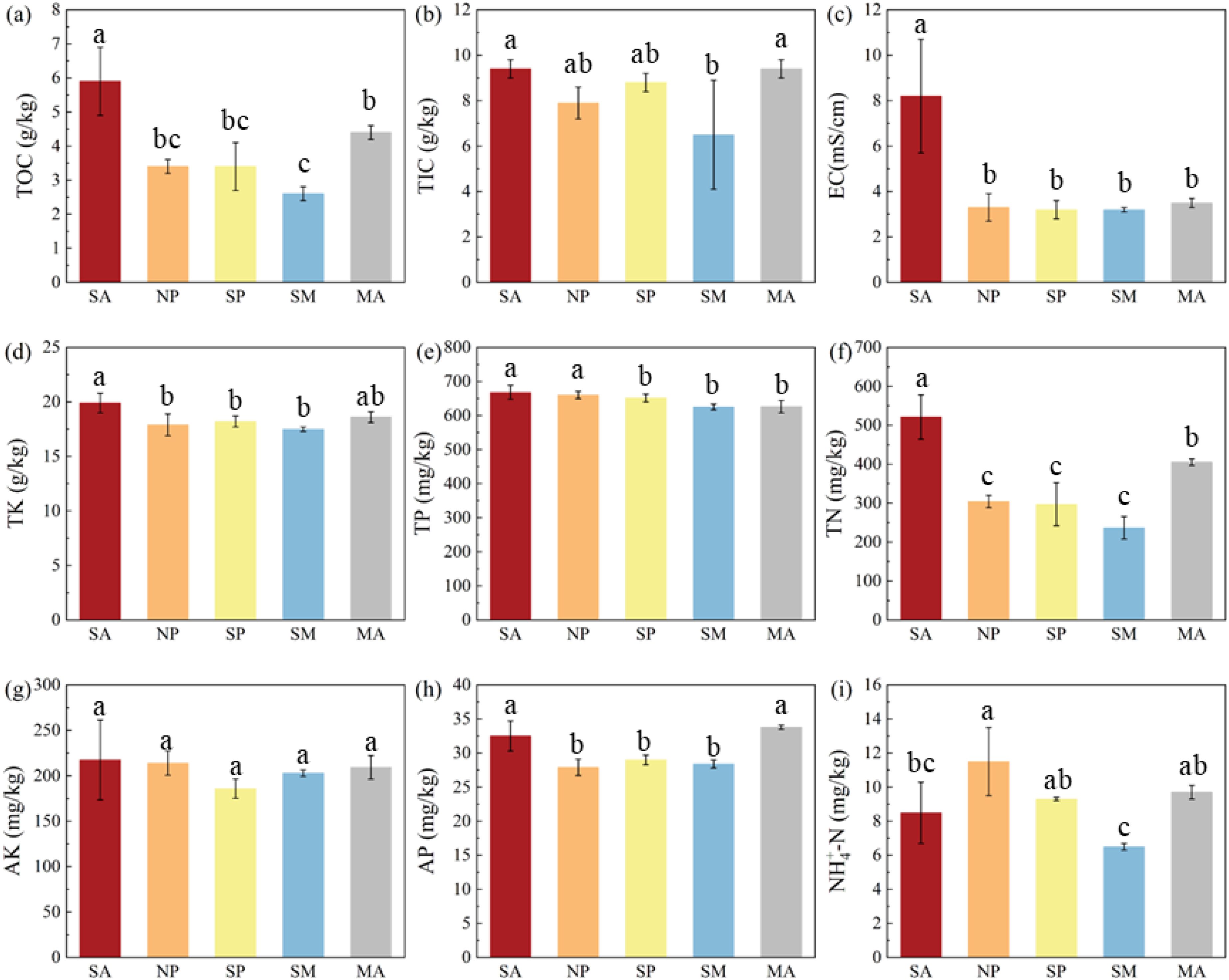
Figure 3. Effect of MPs contamination on the physicochemical properties of sediments from different areas. (A) TOC content; (B) TIC concentration; (C) EC value; (D) TK content; (E) TP content; (F) TN content; (G) AK content; (H) AP content; (I) NH4+‐N content. Different lowercase letters above bars indicate significant differences (p < 0.05) among treatment groups.
Sediment enzyme activities in the MPs-contaminated S. alterniflora invasion and Suaeda areas are shown in Table 2. A similar trend was observed between ALP and TOC in the MPs-contaminated area of S. alterniflora invasion. This suggests that the MPs in the sediments can be used as a substrate for the decomposition of ALP. Rocabruna et al. (2024) found that TOC has a positive effect on ALP activity. Additionally, Han et al. (2017) discovered a significant negative correlation between TOC content and SUC activity in their study of geographically isolated wetlands. Similarly, the activity of SUC in the sediments showed a significant correlation with the abundance of MPs, suggesting that MPs could also be one of the decomposition substrates for SUC. This may be because ALP and SUC can decompose organic matter in sediments (Bai et al., 2017). Therefore, some types of MPs can serve as decomposition substrates for ALP and SUC in sediments, which in turn can be converted into sediment nutrients.
3.3 Impact of MPs on microbial communities
The microbial abundance and community structure in different MPs contaminated areas are shown in Figure 4. The number of OTUs in different treatment groups were as follows: Suaeda area > S. alterniflora mature area > Single-plant area > Sub-mature area > None-plant area, with 3191 OTUs, 3116 OTUs, 2751 OTUs, 2443 OTUs, and 2362 OTUs, respectively (Figure 4A). The observed trend is similar to the variation in TOC content across different MPs contamination zones, which may be attributed to TOC being an important carbon source for microorganisms (Cao et al., 2023). This further suggests that MPs pollution has influenced the richness of microbial communities to some extent. The main bacterial phyla in the S. alterniflora invasion area and Suaeda area included Proteobacteria, Bacteroidota, Firmicutes, Actinobacteriota, Desulfobacterota, Chloroflexi, Campilobacterota, Gemmatimonadota, Bdellovibrionata, and Acidobacteriota. It was found that Proteobacteria and Bacteroidota were the most abundant microorganisms in the Suaeda area (22.37-38.94%; 13.53-21.64%), none-plant area (30.11-47.61%; 27.16-37.14%), single-plant area (33.67-46.01%; 23.18-32.48%), sub-mature area (36.94-38.40%; 29.39-37.16%), and mature area (39.28-48.93%; 28.35-32.77%) shared the major microbial types. In addition, Actinobacteriota was also the major microbial type in the Suaeda area (9.09-23.16%). Firmicutes were the main microbial type in none-plant areas (13.36-19.91%), single-plant areas (2.18-20.48%), sub-mature areas (14.52-23.10%), and mature areas (5.23-12.76%). Proteobacteria and Bacteroidota were the main dominant phyla. The abundance of these microorganisms was found to be related to organic matter and nutrients in the sediments (Larsbrink and Mckee, 2020; Huang and Lu, 2022). Hou et al. (2024) identified Proteobacteria, Actinobacteriota and Chloroflexi as the dominant phyla. Among these, Proteobacteria are known for their ability to effectively degrade complex organic matter (Lin S et al., 2022; Chen et al., 2015). The higher abundance of Actinobacteriota and Firmicutes may be because they are both salt-tolerant bacteria (Samaei et al., 2013).
4 Conclusion
The results showed that the total MPs abundance of the S. alterniflora single-plant area was the highest (16.338 ×103 items/kg d.w.), while the S. alterniflora mature area had the lowest MPs abundance (3.145 ×103 items/kg d.w.). The typical MPs types in the study area were Polyurethane and Chlorinated polyisoprene. MPs pollution increased TOC, AP and TN content. Moreover, MPs pollution increased the enzymatic activities of ALP and SUC in sediments. In addition, Proteobacteria and Bacteroidota were the major dominant phyla. These microorganisms were influenced by the physicochemical properties of the sediment. This study provides significant insights into the ecological impact of MPs pollution in coastal wetlands, particularly in areas invaded by S. alterniflora.
Data availability statement
The original contributions presented in the study are included in the article/supplementary material. Further inquiries can be directed to the corresponding authors.
Author contributions
GL: Conceptualization, Data curation, Investigation, Methodology, Writing – original draft. JR: Data curation, Visualization, Writing – review & editing. JD: Data curation, Investigation, Visualization, Writing – review & editing. KY: Data curation, Software, Validation, Writing – review & editing. JH: Conceptualization, Data curation, Supervision, Writing – review & editing. JS: Project administration, Resources, Writing – review & editing. YMZ: Data curation, Investigation, Validation, Writing – review & editing. JC: Conceptualization, Visualization, Writing – review & editing. YZ: Formal analysis, Visualization, Writing – review & editing. EZ: Conceptualization, Investigation, Visualization, Writing – review & editing. SH: Conceptualization, Formal analysis, Resources, Supervision, Writing – review & editing. ZZ: Conceptualization, Funding acquisition, Project administration, Supervision, Writing – review & editing.
Funding
The author(s) declare that financial support was received for the research, authorship, and/or publication of this article. This research was generously supported by funding from ABA Chemicals and the Yancheng Tiaozini Wetland Research Institute Co., Ltd. The funders were not involved in the study design, collection, analysis, interpretation of data, the writing of this article or the decision to submit it for publication.
Conflict of interest
The authors declare that the research was conducted in the absence of any commercial or financial relationships that could be construed as a potential conflict of interest.
Generative AI statement
The author(s) declare that no Generative AI was used in the creation of this manuscript.
Publisher’s note
All claims expressed in this article are solely those of the authors and do not necessarily represent those of their affiliated organizations, or those of the publisher, the editors and the reviewers. Any product that may be evaluated in this article, or claim that may be made by its manufacturer, is not guaranteed or endorsed by the publisher.
References
Adomako M. O., Wu J., Lu Y., Adu D., Seshie V. I., Yu F. H. (2024). Potential synergy of microplastics and nitrogen enrichment on plant holobionts in wetland ecosystems. Sci. Total Environ. 915, 14. doi: 10.1016/j.scitotenv.2024.170160
Anonymous (2016). Sources, fate and effects of microplastics in the marine environment: part 2 of a global assessment. GESAMP Rep. Stud. 93, 15–220.
Bai J., Yan J., He D., Cai J., Wang R., You W., et al. (2017). Effects of spartina alterniflora invasion in eastern fujian coastal wetland on the physicochemical properties and enzyme activities of mangrove soil. J. Beijing Forestry Univ. 39, 70–77.
Cao M. M., Cui L. N., Sun H. M., Zhang X. M., Zheng X., Jiang J. (2021). Effects of Spartina Alterniflora invasion on soil microbial community structure and ecological functions. Microorganisms 9, 16. doi: 10.3390/microorganisms9010138
Cao J. H., Li C. J., Gao X. D., Cai Y. H., Song X. L., Siddique K. H. M., et al. (2023). Agricultural soil plastic as a hidden carbon source stimulates microbial activity and increases carbon dioxide emissions. Resour. Conserv. Recycling 198, 9. doi: 10.1016/j.resconrec.2023.107151
Chen S. T., Gao D. Z., Zhang J. B., Müller C., Li X. F., Zheng Y. L., et al. (2022). Invasive Spartina alterniflora accelerates soil gross nitrogen transformations to optimize its nitrogen acquisition in an estuarine and coastal wetland of China. Soil Biol. Biochem. 174, 11. doi: 10.1016/j.soilbio.2022.108835
Chen Z. L., Lee S. Y. (2021). Contribution of microplastics to carbon storage in coastal wetland sediments. Environ. Sci. Technol. Lett. 8, 1045–1050. doi: 10.1021/acs.estlett.1c00784
Chen J. H., Liu X. Y., Li L. Q., Zheng J. W., Qu J. J., Zheng J. F., et al. (2015). Consistent increase in abundance and diversity but variable change in community composition of bacteria in topsoil of rice paddy under short term biochar treatment across three sites from South China. Appl. Soil Ecol. 91, 68–79. doi: 10.1016/j.apsoil.2015.02.012
Cincinelli A., Scopetani C., Chelazzi D., Martellini T., Pogojeva M., Slobodnik J. (2021). Microplastics in the black sea sediments. Sci. Total Environ. 760, 12. doi: 10.1016/j.scitotenv.2020.143898
Ding X. L., Wang W. Q., Wen J. H., Feng T. S., Peñuelas J., Sardans J., et al. (2023). Spartina alterniflora invasion differentially alters microbial residues and their contribution to soil organic C in coastal marsh and mangrove wetlands. Catena 230, 10. doi: 10.1016/j.catena.2023.107246
Eo S., Hong S. H., Song Y. K., Han G. M., Shim W. J. (2019). Spatiotemporal distribution and annual load of microplastics in the Nakdong River, South Korea. Water Res. 160, 228–237. doi: 10.1016/j.watres.2019.05.053
Gallo F., Fossi C., Weber R., Santillo D., Sousa J., Ingram I., et al. (2018). Marine litter plastics and microplastics and their toxic chemicals components: the need for urgent preventive measures. Environ. Sci. Europe 30, 14. doi: 10.1186/s12302-018-0139-z
Geyer R., Jambeck J. R., Law K. L. (2017). Production, use, and fate of all plastics ever made. Sci. Adv. 3, 5. doi: 10.1126/sciadv.1700782
Han L. L., Su D. R., Lv S. H., Luo Y., Li X. F., Jiao J., et al. (2017). Responses of biogeochemical characteristics and enzyme activities in sediment to climate warming under a simulation experiment in geographically isolated wetlands of the Hulunbuir Grassland, China. Int. J. Environ. Res. Public Health 14, 17. doi: 10.3390/ijerph14090968
Hou N., Zeng Q., Wang W., Zheng Y., Sardans J., Xue K., et al. (2024). Soil carbon pools and microbial network stability depletion associated with wetland conversion into aquaculture ponds in Southeast China. Sci. total Environ. 954, 176492. doi: 10.1016/j.scitotenv.2024.176492
Huang J., Lu Y. (2022). Decomposition of soil polymeric organic matter by Bacteroidetes and Clostridia:progress and perspectives. Microbiol. China 49, 1147–1157.
Huang B., Sun L. Y., Liu M. R., Huang H. Y., He H., Han F. X., et al. (2021). Abundance and distribution characteristics of microplastics in plateau cultivated land of Yunnan Province, China. Environ. Sci. pollut. Res. 28, 1675–1688. doi: 10.1007/s11356-020-10527-3
Kong F. L., Xi M., Li Y., Zhang W. H., Liu Y. (2015). Distribution characteristics of soil organic carbon in estuarine wetland of Qingdao dagu river. Appl. Mechanics Materials 737, 469–472. doi: 10.4028/www.scientific.net/amm.737.469
Larsbrink J., Mckee L. S. (2020). Advances in Applied Microbiology Vol. 110. Eds. Gadd G. M., Sariaslani S. (London: Academic Press Ltd-Elsevier Science Ltd), 63–98. doi: 10.1016/bs.aambs.2019.11.001
Li Q. G., Long Z. Q., Wang H. J., Zhang G. M. (2021). Functions of constructed wetland animals in water environment protection - A critical review. Sci. Total Environ. 760, 12. doi: 10.1016/j.scitotenv.2020.144038
Li Q. L., Wu J. T., Zhao X. P., Gu X. Y., Ji R. (2019). Separation and identification of microplastics from soil and sewage sludge. Environ. pollut. 254, 9. doi: 10.1016/j.envpol.2019.113076
Lin G. M., He Y. N., Lu J. G., Chen H., Feng J. X. (2021). Seasonal variations in soil physicochemical properties and microbial community structure influenced by Spartina alterniflora invasion and Kandelia obovata restoration. Sci. Total Environ. 797, 12. doi: 10.1016/j.scitotenv.2021.149213
Lin Y. X., Hu H. W., Yang P., Ye G. P. (2022). Spartina alterniflora invasion has a greater impact than non-native species, Phragmites australis and Kandelia obovata, on the bacterial community assemblages in an estuarine wetland. Sci. Total Environ. 822, 9. doi: 10.1016/j.scitotenv.2022.153517
Lin S. Y., Wang W. Q., Sardans J., Lan X. F., Fang Y. Y., Singh B. P., et al. (2022). Effects of slag and biochar amendments on microorganisms and fractions of soil organic carbon during flooding in a paddy field after two years in southeastern China. Sci. Total Environ. 824, 14. doi: 10.1016/j.scitotenv.2022.153783
Mao Z.-G., Wang G.-X., Liu J.-E., Ren L.-J. (2009). Influence of salt marsh vegetation on spatial distribution of soil carbon and nitrogen in Yancheng coastal wetland. Yingyong Shengtai Xuebao 20, 293–297.
Mu J. L., Qu L., Jin F., Zhang S. F., Fang C., Ma X. D., et al. (2019). Abundance and distribution of microplastics in the surface sediments from the northern Bering and Chukchi Seas. Environ. pollut. 245, 122–130. doi: 10.1016/j.envpol.2018.10.097
Peng X., Zhang G., Yan Y., Yao C., Liu Q., Tang Q., et al. (2024). Microplastics in surface sediments of Yancheng coastal wetlands of Jiangsu province, China: distribution characteristics and ecological risk assessment. Mar. Environ. Sci. 43, 320–328.
Peng G. Y., Zhu B. S., Yang D. Q., Su L., Shi H. H., Li D. J. (2017). Microplastics in sediments of the changjiang estuary, China. Environ. pollut. 225, 283–290. doi: 10.1016/j.envpol.2016.12.064
Qin X., Sun J., Wang H. (2015). Distribution of soil nutrients and response to main climatic factors in three river source. Ecol. Environ. Sci. 24, 1295–1301.
Rasta M., Sattari M., Taleshi M. S., Namin J. I. (2020). Identification and distribution of microplastics in the sediments and surface waters of Anzali Wetland in the Southwest Caspian Sea, Northern Iran. Mar. pollut. Bull. 160, 9. doi: 10.1016/j.marpolbul.2020.111541
Rocabruna P. C., Domene X., Matteazzi A., Figl U., Fundneider A., Fernández-Martínez M., et al. (2024). Effect of organic fertilisation on soil phosphatase activity, phosphorus availability and forage yield in mountain permanent meadows. Agric. Ecosyst. Environ. 368, 12. doi: 10.1016/j.agee.2024.109006
Rodrigues M. O., Abrantes N., Gonçalves F. J. M., Nogueira H., Marques J. C., Gonçalves A. M. M. (2018). Spatial and temporal distribution of microplastics in water and sediments of a freshwater system (Antua River, Portugal). Sci. Total Environ. 633, 1549–1559. doi: 10.1016/j.scitotenv.2018.03.233
Samaei M. R., Mortazavi S. B., Bakhshi B., Jafari A. J. (2013). Isolation, genetic identification, and degradation characteristics of n-hexadecane degrading bacteria from tropical areas in Iran. Fresenius Environ. Bull. 22, 1304–1312.
Tokunaga Y., Okochi H., Tani Y., Niida Y., Tachibana T., Saigawa K., et al. (2023). Airborne microplasticss detected in the lungs of wild birds in Japan. Chemosphere 321, 9. doi: 10.1016/j.chemosphere.2023.138032
Turan N. B., Erkan H. S., Engin G. O. (2021). Current status of studies on microplastics in the world’s marine environments. J. Cleaner Production 327, 12. doi: 10.1016/j.jclepro.2021.129394
Wang Y. Q., Bai J. H., Zhang L., Liu H. Z., Wang W., Liu Z., et al. (2023). Advances in studies on the plant rhizosphere microorganisms in wetlands: A visualization analysis based on CiteSpace. Chemosphere 317, 9. doi: 10.1016/j.chemosphere.2023.137860
Wang B., Lin X. B. (2023). Exotic Spartina alterniflora invasion enhances sediment N-loss while reducing N retention in mangrove wetland. Geoderma 431, 12. doi: 10.1016/j.geoderma.2023.116362
Wang C. H., Zhao J., Xing B. S. (2021). Environmental source, fate, and toxicity of microplastics. J. Hazardous Materials 407, 17. doi: 10.1016/j.jhazmat.2020.124357
Wu Y. M., Leng Z. R., Li J., Yan C. L., Wang X. H., Jia H., et al. (2022). Sulfur mediated heavy metal biogeochemical cycles in coastal wetlands: From sediments, rhizosphere to vegetation. Front. Environ. Sci. Eng. 16 (8), 18. doi: 10.1007/s11783-022-1523-x
Xu H., Zhang Y., Wang X., Huang X., Wang G. (2023a). Spatial distribution characteristics of soil organic carbon in Spartina alterniflora salt marsh in different invasive years along Beibu Gulf,Guangxi. Mar. Environ. Sci. 42, 405–409.
Xu N. H., Zhu Z. C., Gao W. L., Shao D. D., Li S. R., Zhu Q., et al. (2023b). Effects of waves, burial depth and material density on microplastics retention in coastal sediments. Sci. Total Environ. 864, 9. doi: 10.1016/j.scitotenv.2022.161093
Yin L. S., Wen X. F., Du C. Y., Jiang J., Wu L. X., Zhang Y., et al. (2020). Comparison of the abundance of microplastics between rural and urban areas: A case study from East Dongting Lake. Chemosphere 244, 7. doi: 10.1016/j.chemosphere.2019.125486
Yin L. S., Wen X. F., Huang D. L., Du C. Y., Deng R., Zhou Z. Y., et al. (2021). Interactions between microplastics/nanoplastics and vascular plants. Environ. pollut. 290, 11. doi: 10.1016/j.envpol.2021.117999
Yu C. F., Cao J. C., Du W., Zhu Z. Y., Xu M. (2022). Changes in the population and functional profile of bacteria and fungi in the rhizosphere of Suaeda salsa is driven by invasion of Spartina alterniflora. Ecol. Indic. 144, 9. doi: 10.1016/j.ecolind.2022.109516
Zhang G. L., Bai J. H., Zhao Q. Q., Jia J., Wang X., Wang W., et al. (2021). Soil carbon storage and carbon sources under different Spartina alterniflora invasion periods in a salt marsh ecosystem. Catena 196, 10. doi: 10.1016/j.catena.2020.104831
Zhang M., Schwarz C., Lin W. P., Naing H., Cai H. Y., Zhu Z. C. (2023). A new perspective on the impacts of Spartina alterniflora invasion on Chinese wetlands in the context of climate change: A case study of the Jiuduansha Shoals, Yangtze Estuary. Sci. Total Environ. 868, 14. doi: 10.1016/j.scitotenv.2023.161477
Zhang C. F., Zhou H. H., Cui Y. Z., Wang C. S., Li Y. H., Zhang D. D. (2019). Microplastics in offshore sediment in the Yellow Sea and East China Sea, China. Environ. pollut. 244, 827–833. doi: 10.1016/j.envpol.2018.10.102
Zheng Y. F., Li J. X., Cao W., Liu X. H., Jiang F. H., Ding J. F., et al. (2019). Distribution characteristics of microplastics in the seawater and sediment: A case study in Jiaozhou Bay, China. Sci. Total Environ. 674, 27–35. doi: 10.1016/j.scitotenv.2019.04.008
Zhou Y. F., Liu X. N., Wang J. (2019). Characterization of microplastics and the association of heavy metals with microplastics in suburban soil of central China. Sci. Total Environ. 694, 10. doi: 10.1016/j.scitotenv.2019.133798
Keywords: coastal wetlands, Spartina alterniflora, microplastics pollution, physicochemical properties, microbial communities
Citation: Li G, Ran J, Dong J, Yang K, Hu J, Shi J, Zhang Y, Chen J, Zhao Y, Zhu E, Huang S and Zheng Z (2024) Effects of microplastics pollution in sediments on physicochemical properties and microbial community distribution in coastal Spartina alterniflora-invaded wetlands. Front. Mar. Sci. 11:1535036. doi: 10.3389/fmars.2024.1535036
Received: 26 November 2024; Accepted: 09 December 2024;
Published: 23 December 2024.
Edited by:
Yanan Chen, Carnegie Mellon University, United StatesReviewed by:
Cheng Zhang, Zhejiang Agriculture and Forestry University, ChinaYali Huang, Chengdu University of Technology, China
Copyright © 2024 Li, Ran, Dong, Yang, Hu, Shi, Zhang, Chen, Zhao, Zhu, Huang and Zheng. This is an open-access article distributed under the terms of the Creative Commons Attribution License (CC BY). The use, distribution or reproduction in other forums is permitted, provided the original author(s) and the copyright owner(s) are credited and that the original publication in this journal is cited, in accordance with accepted academic practice. No use, distribution or reproduction is permitted which does not comply with these terms.
*Correspondence: Suzhen Huang, aHVhbmdzekBmdWRhbi5lZHUuY24=; Zheng Zheng, enpoZW5naGpAZnVkYW4uZWR1LmNu