- 1Yantai Center of Coastal Zone Geological Survey, China Geological Survey, Yantai, China
- 2Ministry of Natural Resources Observation and Research Station of Land-Sea Interaction Field in the Yellow River Estuary, Yantai, China
- 3Qingdao Institute of Marine Geology, China Geological Survey, Qingdao, China
The contamination of marine ecosystems with metal(loid)s is an increasing environmental concern, largely driven by anthropogenic activities, and poses a significant risk to the health of ecosystems and human well-being. Geochemical background values represent the typical concentrations of trace elements observed in the natural environment. The utilization of disparate background values gives rise to disparate evaluation outcomes. The objective of this study was to investigate the concentration profiles of metal(loid)s (Cu, Pb, Zn, Cr, Cd, As, and Hg) along a sediment core in order to obtain background values and assess the depositional processes and contamination levels in Laizhou Bay. With the exception of arsenic, the distribution patterns of the remaining metal(loid)s were similar and could be divided into four stages, which were primarily influenced by the mean grain size and sediment sources. The results of the analysis of multiple indicators indicated that there was no evidence of heavy metal enrichment or contamination in the core sediments. Furthermore, the data demonstrated that all metal(loid)s present were of natural origin. The historical changes in metal(loid)s in the core sediments were predominantly linked to the sedimentary environment, sediment sources, and mean grain size. The mean values of the metal(loid)s in the DU 4-2 unit, formed during the Early Holocene, may be regarded as reference values for background concentrations.
1 Introduction
Metal(loid)s are among the major chemical substances that cause environmental pollution (Gil and Olmedo, 2023; Thorat et al., 2023). Metal(loid)s have wide-ranging sources, long persistence, difficult degradation, difficult detection and recovery after pollution, and easy transfer and accumulation along the food chain (Briffa et al., 2020; Rehman et al., 2021). They can directly or indirectly affect the DNA of organisms, causing the abnormal development of marine organisms and even the extinction of sensitive species, resulting in irreversible impacts on ecosystems (Chen et al., 2016; Li et al., 2022). Heavy metal indicators in seawater and sediments are important components of marine environmental assessment (Onakpa et al., 2018; Sánchez et al., 2020; Strode et al., 2017).
Laizhou Bay is located northwest of the Shandong Peninsula and in the southern part of the Bohai Sea, covering an area of approximately 6000 km2 (Yuan et al., 2023). It is a typical semi-enclosed coastal bay and an important fishing area in China. Several rivers discharge into the sea, such as the Yellow, Xiaoqing, Bailang, Wei, and Jiaolai rivers, and carry large amounts of sediment pollutants every year (Duan et al., 2021). With the rapid economic development in the area, the combination of multiple human activities, such as land-based pollution, land reclamation projects, port shipping, and fishing, has led to prominent ecological and environmental issues in this sea area. Relevant studies have shown that human activity has increased the heavy metal content of sediments in the Bohai Sea (Wu et al., 2022). In addition, changes in land-based pollution and water dynamics in the bays and estuaries of the Bohai Sea have significantly influenced the deposition and distribution of metal(loid)s in sediments, potentially causing corresponding changes to the ecological environment.
Extensive research has been conducted on the characteristics of heavy metal pollution and ecological risks in the sediments of Laizhou Bay, based on large amounts of surface sediment (Li et al., 2013; Duan et al., 2021; Wu et al., 2022). However, there are some differences in the results of different studies, especially in the evaluation of heavy metal pollution and ecological risks, owing to the lack of unified background values. For example, when assessing heavy metal pollution in Laizhou Bay, as the background values, Xu et al. (2015) used the concentration of metal(loid)s in Bohai sediment, Duan et al. (2021) used the content of soil elements in Shandong Province, and Xu et al. (2021) selected the concentration of metal(loid)s in Bohai sediment and the concentration of metal(loid)s in adjacent land soil in Shandong Province, and then compared and analyzed the differences in the calculation results. Therefore, using multiple evaluation methods and combining them with historical changes in heavy metal content will provide a more objective and comprehensive understanding of the metal pollution status in Laizhou Bay. The historical trends of trace elements in the sediments of Laizhou Bay are poorly understood because of the lack of long drill holes. This study aimed to analyze historical variations in heavy metal content in Laizhou Bay using collected geological borehole data. Multiple evaluation methods were employed to comprehensively assess the status of heavy metal pollution and ecological risk. The goals were to gain a better understanding of heavy metal pollution in Laizhou Bay, identify characteristic pollutants, provide reference values for selecting background values of metal(loid)s in the study area, and provide a scientific basis for preventing and controlling heavy metal pollution in related marine areas and for the comprehensive management of the Bohai Sea.
2 Materials and methods
2.1 Sampling and analytical methods
The sediment core LZ01 (119.8413°E, 37.8316°N; Figure 1) was collected in 2013 using a rigging drill. The sediment core, measuring 70.1 meters in length, was obtained at a water depth of 17.82 meters. The average core recovery rate was 86.8%. The core was split lengthwise, described visually, and subsequently sub-sampled in the laboratory. A total of 261 subsamples were taken from the core LZ01 at 25–30 cm intervals for grain size and elemental analyses. The samples were treated and tested in accordance with the methodology described by Xu et al. (2015). The grain size properties were calculated in accordance with the formula proposed by Folk and Ward (1957). The quality of the elemental analysis was evaluated using parallel samples and the national sediment standard GBW07309 (GSD-9), which was analyzed for every 10 unknown samples. The relative error of the parallel samples was determined to be less than 5%.
The selection of gastropods and unbroken shells, including the peat layers, for accelerator mass spectrometry (AMS) 14C dating (Stuiver et al., 2020) is presented in Table 1. Nine samples from core LZ01 were collected for optically stimulated luminescence (OSL) dating of quartz, employing a Daybreak 2200 thermoluminescence/OSL reader (Table 2). The concentrations of K, U, and Th were determined through inductively coupled plasma mass spectrometry and subsequently converted into dose rates, with the requisite data sourced from Aitken (1998) and Marsh et al. (2002).
2.2 Assessment methods of heavy metal pollution
2.2.1 Enrichment factor
The enrichment factor (EF) is a quantitative indicator that is employed to evaluate the extent of heavy metal contamination in sediments and to ascertain its sources (Marmolejo-Rodríguez et al., 2017). By utilizing aluminum as a reference element, the elemental composition of the sample was normalized in order to eliminate the impact of variations in particle size (Zhou et al., 2022).
EF values were calculated using Equation 1:
where Mesample and Alsample are the trace element and Al concentrations in each sample, respectively, and Mebaseline and Albaseline are the reference element concentrations. According to Xu et al. (2015), the sediment in the study area was primarily from the material discharged by rivers along its western and southern coasts. Therefore, the elemental background concentrations in the soil of Shandong Province were adopted to calculate the EF values in this study (CNEMC, 1990). EF < 1.5 is indicative of metal(loid)s related to crustal sources, whereas EF > 1.5 implies anthropogenic sources (Zhang and Liu, 2002).
2.2.2 Geoaccumulation index
The geoaccumulation index (Igeo) is used to eliminate the influence of natural geological contributions, and is calculated using Equation 2:
In accordance with the findings of Muller (1981), seven categories of geoaccumulation indices were identified, spanning from class 0 (Igeo ≤ 0, unpolluted) to class 7 (Igeo > 5, extremely polluted).
2.2.3 Potential ecological risk index
The potential ecological risk index (ER) evaluates the degree of heavy metal contamination in sediments based on the potential toxicity of metal(loid)s and the environmental response. ER was calculated as follows:
The potential ecological risk coefficient for each heavy metal was calculated as follows: 1 for Zn, 2 for Cr, 5 for Cu and Pb, 10 for As, 30 for Cd, and 40 for Hg (Hakanson, 1980). The comprehensive potential ecological risk index for multiple metal(loid)s is represented by ER. Each heavy metal was classified into one of five categories based on its potential ecological risk (): low (less than 40), moderate (40-79), considerable (80-159), high (160-319), and very high (equal to or greater than 320) ecological risk. The ER was classified into four levels: low ecological risk (ER < 105), moderate ecological risk (105 ≤ ER < 210), significant ecological risk (210 ≤ ER < 420), and very high ecological risk (ER ≥ 420) (Xu et al., 2021).
3 Results
3.1 Sedimentary structure of the core
The general stratigraphic framework of core LZ01 was identified through a process of correlation involving the application of AMS14C and OSL ages, sedimentary structures, sedimentary facies, grain size, and comparison with other well-studied cores from adjacent areas. The core was subdivided into four units, designated DU1–DU4, in descending order (Figures 2, 3).
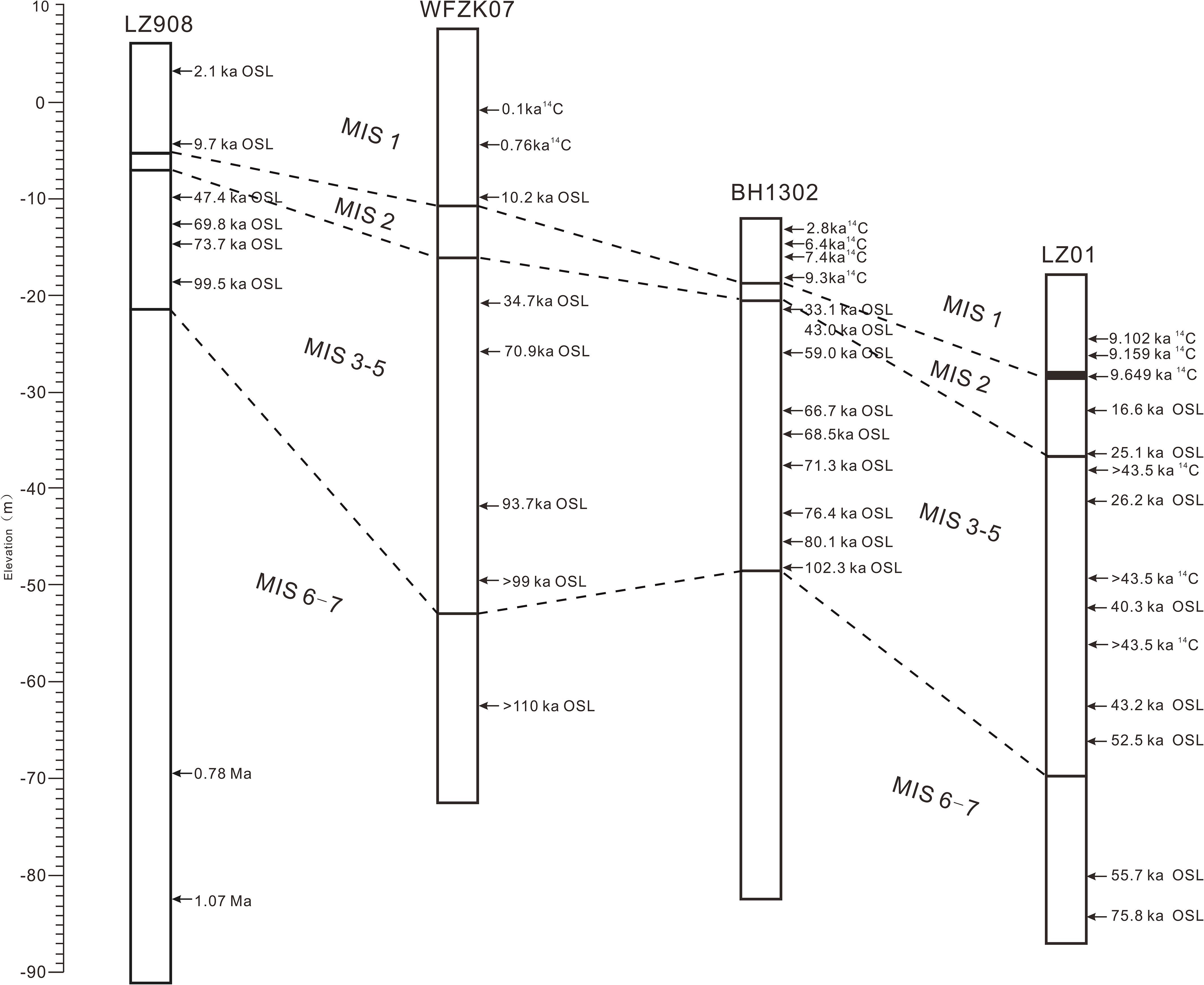
Figure 3. Comparison of late Quaternary sediment records from Laizhou Bay cores (BH1302, Yuan et al., 2023; LZ908, Yi et al., 2012; WFZK07, Guo et al., 2017).
DU 1 (70.10–51.87 m) was primarily composed of dark gray, dark yellow-gray, earthy yellow silt, and clayey silt, interspersed with clayey bands and silt lenses. Calcium nodules and brownish-yellow rust spots are visible, indicating moderate biological disturbance. Locally, there are gray-yellow to yellow-gray fine to medium sands, with average sorting. The sediment exhibits cross-bedding and contains minor clayey bands, along with occasional black spots and rust stains. The sediment composition is predominantly silt, with an average content of 54.86%, followed by sand at an average of 32.92%, with a maximum content reaching 94.23%. Clay content is the least, ranging from 0% to 37.85%, with an average of 12.21%. The average grain size varies significantly, ranging from 3.05 to 7.55 Φ, indicating considerable changes in the sedimentary environment, with an average of 5.17 Φ. The OSL dating for this unit yields relatively young and unreliable ages. Comparisons with surrounding boreholes suggest that the sedimentary environment corresponds to a coastal-marine transitional setting prior to the Late Pleistocene, which maybe formed during marine isotope stage (MIS) 6-7.
DU 2 (51.87–19.03 m) consists mainly of dark gray, dark yellow-gray, dark gray, and earthy yellow clayey silt and silt, with locally dense interlayers and irregular silt to fine sand lenses. A few brown rust spots are visible, along with intact small gastropods. The central area features yellow-gray fine to medium sand, with average sorting and cross-bedding, interspersed with irregular clayey bands. Shell layers are observed at depths of approximately 31.6 m, 33.6 m, 40.8 m, and around 51.8 m, containing abundant shell fragments and intact small gastropods. The sediments are primarily silt and sand, with average contents of 46.59% and 44.16%, respectively, while clay content is minimal, ranging from 0% to 35.63%, with an average of 9.25%. The average grain size also exhibits significant variability, ranging from 3.05 to 7.51 Φ, indicating substantial changes in the sedimentary environment, with an average of 4.69 Φ. The AMS 14C dating of four intact gastropods from the top to the bottom of this unit yields ages greater than 43,500 years. The OSL dating data is similarly young and unreliable. Comparisons with surrounding boreholes indicate that the OSL ages for the corresponding segment of borehole WFZK07 range from 34.7 ± ka to greater than 110 ka. The sedimentary environment of this unit is interpreted as a coastal to shallow marine setting, corresponding to the sedimentary layers formed during the Cangzhou and Xianxian transgressions, which formed during MIS 3-5.
The DU 3 unit (19.03–10.66 m) primarily consisted of dark gray, dark yellow-gray, yellow-gray, and ochre-colored silt to fine to medium sand, with generally poor sorting. It exhibits no distinct layering, interspersed with clay-rich bands, and occasionally contains black carbon spots and a small amount of brown rust stains. The sediment composition is predominantly silt and sand, with silt content ranging from 15.60% to 62.68%, averaging 48.21%. Sand content varies between 18.48% and 81.70%, with an average of 39.95%. Clay content is minimal, averaging 11.84%. The average grain size ranges from 3.47 to 5.91 Φ, with a mean of 4.95 Φ. Three OSL dating results from the top to the bottom of this unit range from 16.6 ± 2 ka to 25.1 ± 3 ka. The sedimentary environment of this unit is interpreted as a terrestrial river deposition during the Last Glacial Maximum (MIS 2).
The DU 4 unit (10.66–0 m) was divided into two subunits DU 4-2 and DU 4-1. DU 4-2 (10.66-6.80 m) mainly comprised dark gray silt-fine sand, with clayey banding, shells, and gastropods. The bottom layers were characterized by peat and shell fragments, with AMS14C ages of 9649 cal yr BP and 9459 cal yr BP, respectively. The mean grain size ranged from 4.46 to 5.98 Φ, with an average of 4.94 Φ. This peat layer is a marker of the Holocene bottom of the Bohai Sea. The other two AMS14C ages of the gastropods were 9102 cal yr BP and 9159 cal yr BP. Therefore, this subunit was interpreted as a transgressive deposit formed during the Early Holocene. DU 4-1 (6.80-0 m) was characterized by dark gray clayey silt-silt, with a silt-fine sandy lens. The mean grain size ranged from 4.15 to 7.17 Φ, with an average of 5.90 Φ. No dating data were available for this subunit. From the sedimentary characteristics and stratigraphic correlation with cores BH1302 and WFZK07 in the adjacent area (Figure 3), DU 4-1 was interpreted as shallow marine deposits during the Middle-Late Holocene.
3.2 Concentration of metal(loid)s
The mean Cu, Pb, Zn, Cr, Cd, As, and Hg concentrations in the LZ01 core were 17.36, 19.94, 52.73, 51.41, 0.08, 7.24, and 0.008 mg/kg, respectively (Table 3). The average concentrations of all metal(loid)s were lower than those in the Yellow River estuary (Liu et al., 2023), Dongying coast (Wu et al., 2022), Laizhou Bay (Duan et al., 2021), Southern Shandong Peninsula (Liu et al., 2015), and Coastal Shandong Peninsula (Li et al., 2013). The average concentrations of all metal(loid)s were below the element baseline in Shandong Peninsula soil (CNEMC, 1990).
Figure 4 illustrates the vertical profiles of the major and trace elements and the mean grain size (Mz) in the LZ01 sediment core. In addition to arsenic, all other metal(loid)s exhibited comparable distribution patterns, which were predominantly influenced by mean grain size. All metal(loid)s demonstrated the highest average concentrations in unit DU 4, which formed during the Holocene (Table 3).
4 Discussion
4.1 Assessment of sediment contamination
The results of the study, expressed as enrichment factors (EFs), are presented in Figure 5. The EFs of Cu, Pb, Zn, Cr, Cd, As, and Hg ranged from 0.06 to 2.34, 0.47 to 1.35, 0.62 to 1.47, 0.51 to 1.38, 0.62 to 2.02, 0.29 to 2.47, and 0.11 to 1.27, with mean values of 0.83, 0.92, 0.97, 0.92, 1.10, 0.92, 0.49, respectively (Table 4). The EF values decreased in the following order: Cd > Zn > Pb = Cr = As > Cu > Hg. The mean EF for all metal(loid)s was found to be below 1.5, indicating that these metal(loid)s were not subject to anthropogenic enrichment. The EF values for Cu, Cd, and As exceeded 1.5 in 2.68%, 11.26%, and 8.70% of the samples, respectively (Figures 5, 6A).
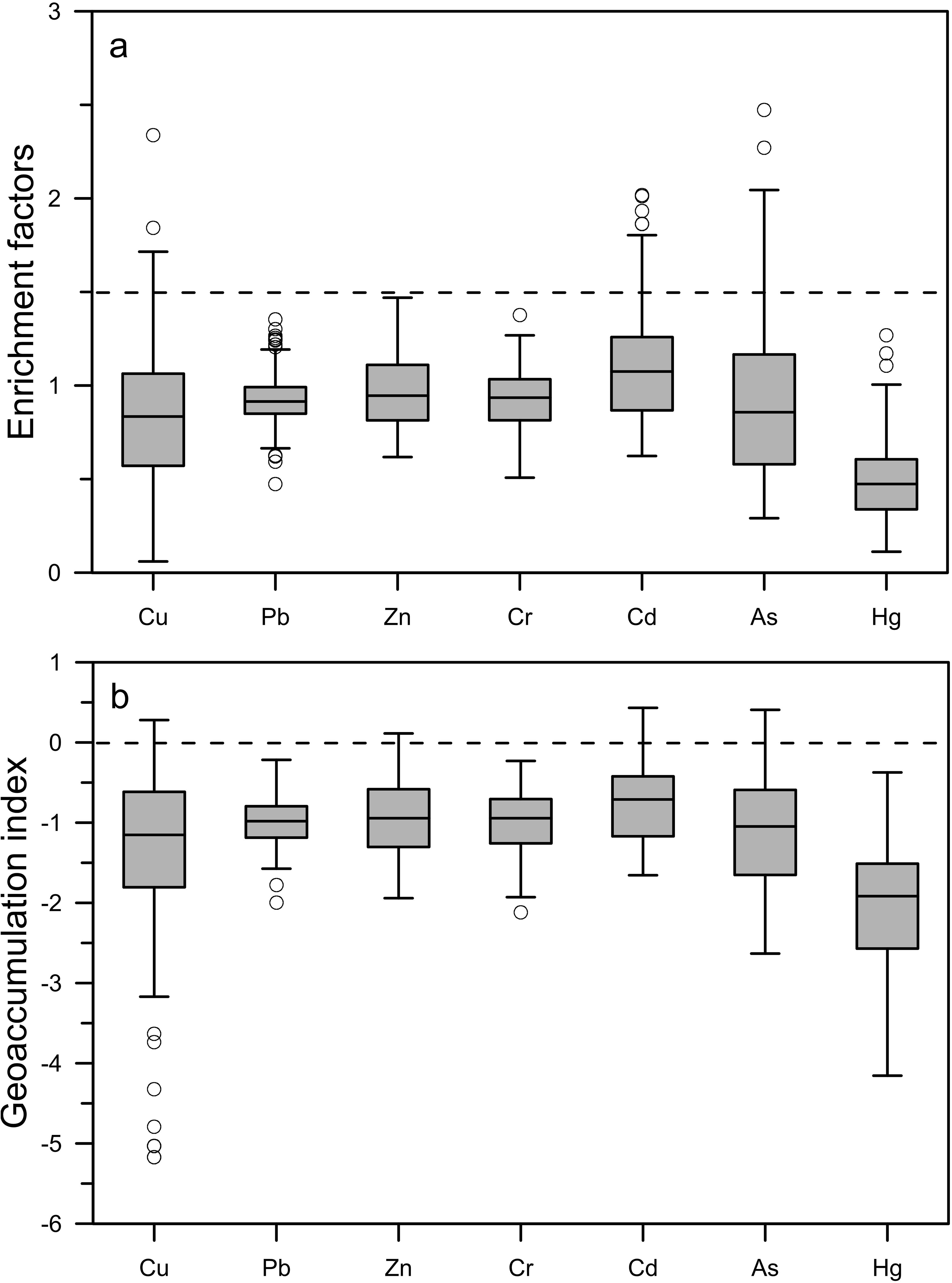
Figure 6. (A) Metal enrichment factors (EF) and (B) geoaccumulation indexes (Igeo) of metal(loid)s in the core sediments of the present study.
The Igeo values for Cu, Pb, Zn, Cr, Cd, As, and Hg in the sediments exhibited a range of -5.17 to 0.28, -2 to -0.22, -1.94 to 0.11, -2.12 to -0.23, -1.66 to 0.43, -2.63 to 0.41, and -4.15 to -0.37, with mean values of -1.33, -0.98, -0.93, -0.99, -0.76, -1.11, and -2.02, respectively (Table 4). The mean degree of pollution was highest for Cd, followed by Zn, Pb, Cr, As, Cu, and Hg. The mean Igeo values for all metal(loid)s were less than zero, indicating that the sediments were not contaminated by these metal(loid)s. The Igeo values for Cu, Zn, Cd, and As exceeded zero in 2.30%, 1.92%, 8.56%, and 5.93% of the samples, respectively (Figures 7, 6B).
The vertical distribution of was similar to that of Igeo (Figure 8). Except for Cd and Hg, the concentrations of all the other metal(loid)s were considered to present a low potential ecological risk ( < 40) in all units (Table 4). For Cd, the sediments from units DU4, DU2, and DU1 were at moderate potential risk in a certain layer. For Hg, the sediments from units DU2 and DU1 were at moderate potential risk occasionally. The results of the comprehensive potential ecological risk index (ER) showed that almost all metal(loid)s in the core sediments were at a low ecological risk (ER < 105) (Figure 8). Only a few layers in DU4, DU2, and DU1 showed a moderate ecological risk, appearing in MIS1, MIS3-5, and MIS 6-7. Overall, the cumulative concentrations and potential ecological risk coefficients of the metal(loid)s in Laizhou Bay were low.
4.2 Source of the metal(loid)s
The Pearson’s correlation coefficients between the concentrations of metal(loid)s (Cu, Pb, Zn, Cr, Cd, As, and Hg), selected major elements (Al, Ti, Mn and Fe), and Mz of the sediments are presented in Table 5. With the exception of As, Al demonstrated a strong correlation with the remaining elements (r = 0.709–0.939) and Mz (r = 0.861). Mz exhibited a moderate correlation with As and a positive correlation with the other elements. With the exception of As, all the remaining elements displayed a positive correlation with each other (1.0 > r ≥ 0.55).
In order to ascertain the sources of metal(loid)s in the environment, a principal components analysis (PCA) was conducted using the statistical software package SPSS. For the core sediments, the Kaiser-Meyer-Olkin (KMO) measure was 0.92, and the Bartlett’s test yielded a value of 0, indicating that the heavy metal data in this study are suitable for PCA analysis (Waykar and Petare, 2016). The loadings are presented in Table 6. Two principal components with an eigenvalue greater than 0.9 were extracted, accounting for 82.46% of the total variance (Figure 9). Principal component 1 (PC1) contributed 74.67% of the total variance and included all metal(loid)s and Mz, with high loadings for Al (0.92), Ti (0.88), Mn (0.90), Fe (0.97), Cu (0.88), Pb (0.74), Zn (0.97), Cr (0.94), Cd (0.87), Hg (0.78), and Mz (0.89). This indicates that these metal(loid)s have the same sources. The significant positive correlations between Mz (Al, Ti, Mn, and Fe) and the metal(loid)s listed in Table 5 indicate that metal(loid)s are predominantly associated with fine terrigenous sediments (particularly clay minerals) via preferential exchange and/or adsorption (Larrose et al., 2010). Aluminum oxide (Al2O3) is a product of continental differentiation and is relatively stable in the crust. Consequently, aluminum is regarded as a stable element in the transition from continents to oceans (Diagomanolin et al., 2004; Yuan et al., 2018), and is employed as an indicator of terrigenous components in the ocean and of terrigenous origin. The correlation between aluminum and metal(loid)s was found to be positive and moderate. In light of the combined results of EF and Igeo, it can be inferred that the metal(loid)s are derived primarily from crustal materials or natural weathering. Therefore, PC1 was identified as a natural source. PC2 accounted for 7.79% of the total variance, with a high loading for As (0.82). The EF and Igeo values of As exceeded 1.5 and zero in 8.70% and 5.93% of the samples, respectively. These occurrences were observed in a few layers within units DU 4, DU 2, and DU 1. In the context of historical times, it can be stated that there was no evidence of human activity. It can therefore be inferred that PC2 represents the change in sedimentary environment (Xiang et al., 2023).
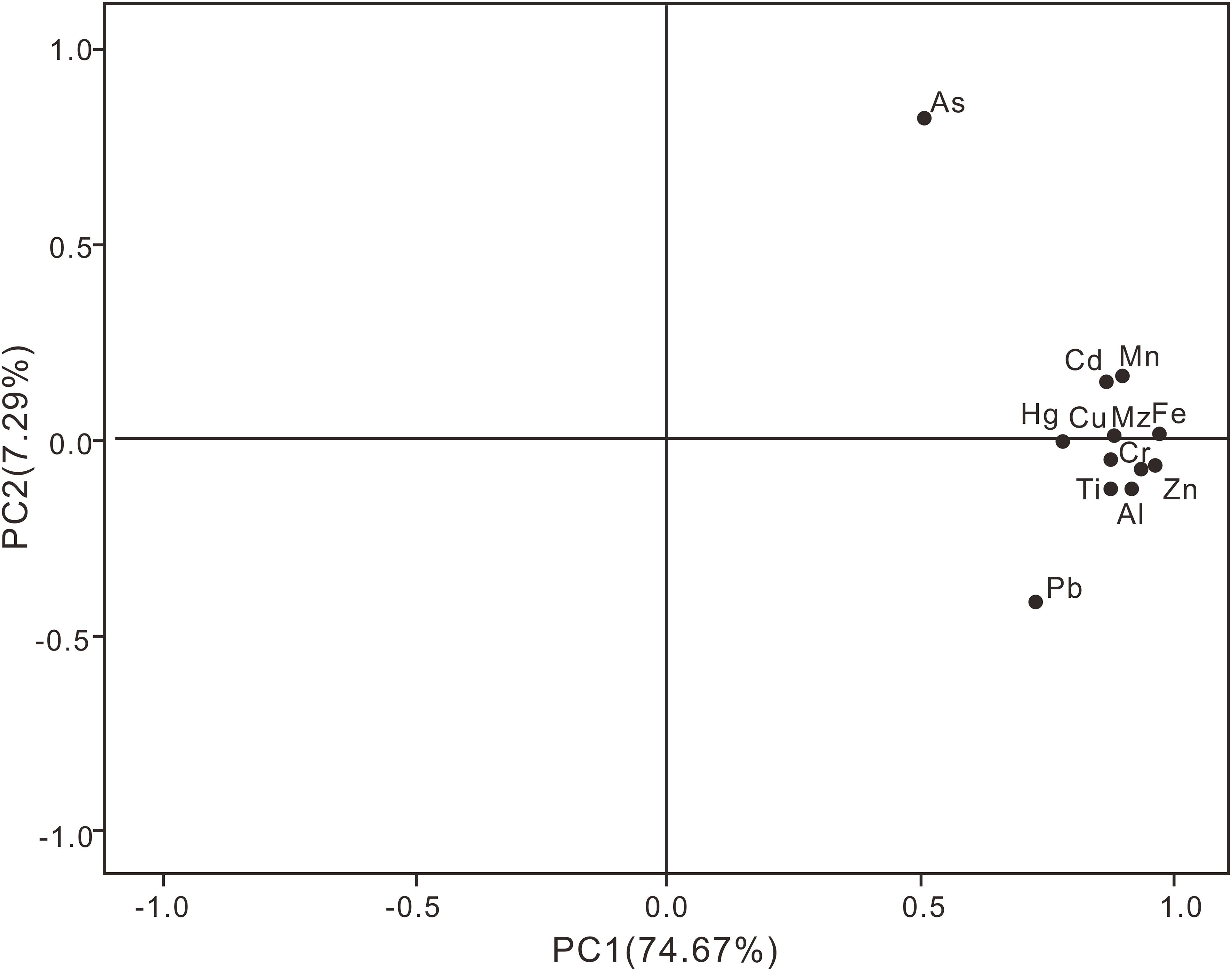
Figure 9. Principal component loading diagram for major and trace elements (Al, Ti, Mn, Fe, Cu, Pb, Zn, Cd, Cr, As, and Hg), and Mz in the core sediments.
4.3 Selection of elemental background values
The elemental background value refers to the content of chemical elements and compounds in soil that has not been influenced by human contamination. However, few accessible areas on Earth’s surface have not been affected by human activities, and determining appropriate sampling sites is difficult. Therefore, the use of drill core sediments from historical periods is a better approach for obtaining background elemental values. When assessing trace element pollution using surface sediments from the Laizhou Bay, the selection of different background values often leads to incomparable evaluation results (Xu et al., 2021; Zheng et al., 2022). In the present study, we used drill core sediments to determine the average content of trace elements in DU 4-2, including Cu (16.59 mg/kg), Pb (18.01 mg/kg), Zn (51.31 mg/kg), Cr (50.83 mg/kg), Cd (0.07 mg/kg), As (6.63 mg/kg), and Hg (0.01 mg/kg), which can be considered as background values for the Laizhou Bay region. The DU 4-2 unit was formed during the Early Holocene, when Laizhou Bay was submerged in seawater and the sea level was stable. The sediment source and depositional environment were similar to those of the present day, and no human activity occurred during this period. Therefore, the average values of trace elements in DU 4-2 can be considered as elemental background values.
4.4 Historical record of metal(loid)s
The sources and variations in heavy metal content in sediments are closely related to terrestrial inputs, sea-level changes, provenance shifts, and dynamic depositional environments (Wang, 2020). The DU 1 sedimentary unit formed during the MIS 6-7 period, which was a coastal-marine transitional setting. The rise of the Miao Island has impeded the exchange of water between the outer sea and the Bohai Sea. During periods of high sea level, the primary factors influencing the sedimentary environment in Laizhou Bay remain fluvial processes, with marine processes playing a secondary role. The study area is located at the terminal point of fluvial material transport, where sediment particles are relatively fine, likely dominated by sediments from the Yellow River, which exhibit higher concentrations of heavy metal elements. Entering Marine Isotope Stages (MIS) 3-5, the subsidence of the Miao Island uplift effectively ceased, resulting in a structural configuration of the Bohai Sea that is largely consistent with the present day. Sea level changes synchronized with the outer sea led to the formation of unit DU 2. The source of sediments in the study area may have shifted, now primarily consisting of near-source fluvial material from the southern shore of Laizhou Bay, characterized by coarser particle sizes and reduced concentrations of heavy metal elements in the sediments. The DU 3 sedimentary unit formed during the MIS2 period when the sea level was 120 m lower than it is today. The study area was exposed to the seabed and experienced fluvial deposition from small rivers and the Yellow River along the southern coast of Laizhou Bay (Yuan et al., 2023). The sediments were coarse grained. The heavy metal content in most of the sediments in the study area showed a strong correlation with grain size, indicating that metal(loid)s were mainly included/adsorbed into fine-grained sediments. Therefore, the heavy metal content of DU 3 was relatively low. During the Holocene, the sea level gradually increased and the study area was submerged by seawater. It was difficult for sediment from small rivers along the southern coast of Laizhou Bay to reach the study area, and the sediment in the borehole was mainly from the Yellow River with a fine grain size. The heavy metal content then began to increase.
5 Conclusion
The present study investigates the vertical distributions of major and trace elements and their pollution statuses in core sediments from Laizhou Bay, China. A general stratigraphic framework of the LZ01 core since the late Quaternary was established on the basis of sedimentary structures, sedimentary facies, grain size, and comparisons with other well-studied cores from adjacent areas. With the exception of arsenic, the distribution patterns of the remaining metal(loid)s were found to be similar and could be divided into four stages, which formed during the MIS 6-7, MIS 3-5, MIS 2, and MIS 1 periods. The highest average concentrations of metal(loid)s were observed in unit DU 4. The presence of multiple indicators indicated that there was no element enrichment or contamination in the core sediments, and that all metal(loid)s were of natural origin. The mean concentrations of the metal(loid)s in DU 4-2, which formed during the Early Holocene, can be used as reference values for background concentrations.
Data availability statement
The original contributions presented in the study are included in the article/supplementary material. Further inquiries can be directed to the corresponding authors.
Author contributions
LL: Conceptualization, Funding acquisition, Methodology, Writing – original draft. JG: Formal analysis, Investigation, Writing – original draft. SZ: Data curation, Resources, Writing – original draft. SL: Investigation, Software, Writing – original draft. DL: Investigation, Software, Writing – original draft. JZ: Validation, Visualization, Writing – original draft. XX: Software, Validation, Writing – original draft. BC: Conceptualization, Funding acquisition, Methodology, Writing – original draft. JQ: Formal analysis, Investigation, Writing – original draft.
Funding
The author(s) declare that no financial support was received for the research, authorship, and/or publication of this article.
Conflict of interest
The authors declare that the research was conducted in the absence of any commercial or financial relationships that could be construed as a potential conflict of interest.
Publisher’s note
All claims expressed in this article are solely those of the authors and do not necessarily represent those of their affiliated organizations, or those of the publisher, the editors and the reviewers. Any product that may be evaluated in this article, or claim that may be made by its manufacturer, is not guaranteed or endorsed by the publisher.
References
Aitken M. (1998). An Introduction to Optical Dating: the Dating of Quaternary Sediments by the Use of Photon-stimulated Luminescence (Oxford: Oxford University Press).
Briffa J., Sinagra E., Blundell R. (2020). Heavy metal pollution in the environment and their toxicological effects on humans. Heliyon. 6, e04691. doi: 10.1016/j.heliyon.2020.e04691
Chen B., Liu J., Qiu J., Zhang X., Wang S., Liu J. (2016). Spatio-temporal distribution and environmental risk of sedimentary heavy metals in the Yangtze river estuary and its adjacent areas. Mar. pollut. Bull. 116, 469–478. doi: 10.1016/j.marpolbul.2016.11.055
China National Environmental Monitoring Center (CNEMC) (1990). Chinese elemental background values for soils (Beijing: Chinese Environmental Science Press).
Diagomanolin V., Farhang M., Ghazi-Khansari M., Jafarzadeh N. (2004). Heavy metals (Ni, Cr, Cu) in the karoon waterway river, Iran. Toxicol. Lett. 151, 63–67. doi: 10.1016/j.toxlet.2004.02.018
Duan Y., Pei S., Liao M., Zhai S., Zhang H., Xu G., et al. (2021). Spatial distribution of heavy metals in the surface sediments of Laizhou Bay and their sources and pollution assessment. Mar. Geol. Quat. Geol. 41, 67–81. doi: 10.16562/j.cnki.0256-1492.2020112601
Folk R. L., Ward W. C. (1957). Brazos river bar: a study in the significance of grain size parameters. J. Sediment. Res. 27, 3–26. doi: 10.1306/74D70646-2B21-11D7-8648000102C1865D
Gil F., Olmedo P. (2023). “Food safety heavy metals and metalloids,” in Encyclopedia of Human Nutrition. Maryland, USA: Academic Press. doi: 10.1016/b978-0-12-821848-8.00041-x
Guo F., M. G., Hou G., Liu S., Wang J. (2017). Source tracing of rare earth elements: A case study of core 07 on the southern coast of Laizhou Bay. Cont. Shelf Res. 136, 29–38. doi: 10.1016/j.csr.2017.01.002
Hakanson L. (1980). An ecological risk index for aquatic pollution control.a sedimentological approach. Water Res. 14, 975–1001. doi: 10.1016/0043-1354(80)90143-8
Larrose A., Coynel A., Schäfer J., Blanc G., Masse L., Maneux E. (2010). Assessing the current state of the Gironde Estuary by mapping priority contaminant distribution and risk potential in surface sediment. Appl. Geochem. 25, 1912–1923. doi: 10.1016/j.apgeochem.2010.10.007
Li G., Hu B., Bi J., Leng Q., Xiao C., Yang Z. (2013). Heavy metals distribution and contamination in surface sediments of the coastal Shandong Peninsula (Yellow Sea). Mar. pollut. Bull. 76, 420–426. doi: 10.1016/j.marpolbul.2013.08.032
Li C., Wang H., Liao X., Xiao R., Liu K., Bai J., et al. (2022). Heavy metal pollution in coastal wetlands: A systematic review of studies globally over the past three decades. J. Hazard Mater. 424, 127312. doi: 10.1016/j.jhazmat.2021.127312
Liu R., Wang W., Kong L., Chen B. (2023). Records of trace metals since the Holocene in sediments of Laizhou Bay, Bohai Sea, China. Mar. pollut. Bull. 186, 114481. doi: 10.1016/j.marpolbul.2022.114481
Liu S., Zhang Y., Bi S., Zhang X., Li X., Lin M., et al. (2015). Heavy metals distribution and environmental quality assessment for sediments off the southern coast of the Shandong Peninsula, China. Mar. Pollu. Bull. 100, 483–485. doi: 10.1016/j.marpolbul.2015.09.028
Marmolejo-Rodríguez A. J., Morales-Blake A. R., González-Chavarín I., Hernández-Becerril D., Alonso-Rodríguez R., Rodríguez-Palacio M. C., et al. (2017). Trace elements from the Central Pacific Mexican Shelf: Geochemical associations and anthropogenic influences. Estuari. Coast. Shelf Sci. 198, 461–470. doi: 10.1016/j.ecss.2016.12.006
Marsh R., Prestwich W., Rink W., Brennan B. (2002). Monte Carlo eterminations of the beta dose rate to tooth enamel. Radiat. Meas. 35, 609–616. doi: 10.1016/S1350-4487(02)00065-3
Muller G. (1981). Die Schwermetallbelastung der sediment des Neckars und seiner Nebenflusse: eine Bestandsaufnahme. Chemiker Zeitung 105, 157–164.
Onakpa M. M., Njan A. A., Kalu O. C. (2018). A review of heavy metal contamination of food crops in Nigeria. Ann. Glob. Health. 84, 488–494. doi: 10.29024/aogh.2314
Rehman T., Naz S., Hussain R., Chatha A., Ahmad F., Yamin A., et al. (2021). Exposure to heavy metals causes histopathological changes and alters antioxidant enzymes in fresh water fish (Oreochromis niloticus). Asian J. Agricul. Biol, 1. doi: 10.35495/ajab.2020.03.143
Sánchez A., Gómez-León A., Pérez-Tribouillier H., Rey-Villiers N., Shumilin E. (2020). Vertical variability of benthic foraminifera and trace elements in a tropical coastal lagoon in the Gulf of California. Mar. pollut. Bull. 158, 111417. doi: 10.1016/j.marpolbul.2020.111417
Strode E., Jansons M., Purina I., Balode M., Berezina N. (2017). Sediment quality assessment using survival and embryo malformation tests in amphipod crustaceans: The Gulf of Riga, Baltic Sea AS case study. J. Mar. Syst. 172, 93–103. doi: 10.1016/j.jmarsys.2017.03.010
Stuiver M., Reimer P. J., Reimer R. W. (2020). CALIB 8.2 [WWW Program]. Available online at: http://calib.org (Accessed 2020-8-14).
Thorat B., Prasad P., Ram A. (2023). Heavy metal accumulation in a moderately polluted Ulhas estuary, Western India. Reg. Stud. Mar. Sci. 60, 102818. doi: 10.1016/j.rsma.2023.102818
Wang Y. (2020). Spatial distribution characteristics and source analysis of heavy metals in sediments of typical estuaries on the south coast of Laizhou Bay (Jinan, Shandong: Master Dissertation of Shandong Normal University).
Waykar B., Petare R. (2016). Studies on monitoring the heavy metal concentrations in the water, sediment and snail species in Latipada reservoir. J. Environ. Biol. 37, 585–589.
Wu Z., Dong Y., Liu R., Liu L., Gao J., Song W., et al. (2022). Assessment of heavy metal contamination in surface sediments off the Dongying coast, Bohai Sea. Mar. pollut. Bull. 180, 113826. doi: 10.1016/j.marpolbul.2022.113826
Xiang L., Jiang S., Cheng H., Wang Y., Liu Q., Yin Y., et al. (2023). Heavy metal concentration profiles and pollution assessment in the Jiangsu offshore area, China. Mar. pollut. Bull. 193, 115187. doi: 10.1016/j.marpolbul.2023.115187
Xu Y., Gao H., Wei X., Zhu J. (2021). Heavy metals and their ecological risk in the surface sediments of Laizhou Bay. Period. Ocean Uni. China 51, 74–85. doi: 10.16441/j.cnki.hdxb.20200326
Xu G., Liu J., Pei S., Gao M., Hu G., Kong X. (2015). Sediment properties and trace metal pollution assessment in surface sediments of the Laizhou Bay, China. Environ. Sci. pollut. Res. 22, 11634–11647. doi: 10.1007/s11356-015-4393-y
Yi L., Yu H. J., Ortiz J. D., Xu X., Chen S., Ge J., et al. (2012). Late Quaternary linkage of sedimentary records to three astronomical rhythms and the Asian monsoon, inferred from a coastal borehole in the south Bohai Sea, China. Palaeogeogr. Palaeoclimatol. Palaeoecol. 329, 101–117. doi: 10.1016/j.palaeo.2012.02.020
Yuan X., Hu R., Feng X., Qiu J., Wang N., Yao Z., et al. (2023). Sedimentary records and implications for the evolution of sedimentary environments inferred from BH1302 during the late Quaternary in the Bohai Sea, China. Mar. Geol. 456, 106986. doi: 10.1016/j.margeo.2022.106986
Yuan H., Zhao G., Li X., Ye S.. (2018). Regional distribution of heavy metals in surface sediments of Dafeng coastal wetlands in Yancheng, Jiangsu and its ecological implications. Mar. Geol. Front. 430, 51–59. doi: 10.16028/j.1009-2722.2018.09007
Zhang J., Liu C. L. (2002). Riverine composition and estuarine geochemistry of particulate metals in China-weathering features, anthropogenic impact and chemical fluxes. Estuar. Coast. Shelf Sci. 54, 1051–1070. doi: 10.1006/ecss.2001.0879
Zheng J., Wang Y., Zhao Y., Mo P., Wei A. (2022). Distribution characteristics and potential risks of major pollutants in sediments of fishing port economic zone and adjacent waters of Haizhou Bay. Mar. Envrion. Sci. 41, 731–737. doi: 10.13634/j.cnki.mes.2022.05.002
Keywords: metal(loid)s, core sediment, sediment contamination, late quaternary, Laizhou Bay (China)
Citation: Liu L, Gao J, Zhang S, Lin S, Lu D, Zhang J, Xie X, Chen B and Qiu J (2025) Depositional record of metal(loid)s since late quaternary in the Laizhou Bay, China. Front. Mar. Sci. 11:1526665. doi: 10.3389/fmars.2024.1526665
Received: 12 November 2024; Accepted: 17 December 2024;
Published: 08 January 2025.
Edited by:
Chaomeng Dai, Tongji University, ChinaReviewed by:
Alberto Sánchez-González, National Polytechnic Institute (IPN), MexicoJadran Faganeli, National Institute of Biology, Slovenia
Copyright © 2025 Liu, Gao, Zhang, Lin, Lu, Zhang, Xie, Chen and Qiu. This is an open-access article distributed under the terms of the Creative Commons Attribution License (CC BY). The use, distribution or reproduction in other forums is permitted, provided the original author(s) and the copyright owner(s) are credited and that the original publication in this journal is cited, in accordance with accepted academic practice. No use, distribution or reproduction is permitted which does not comply with these terms.
*Correspondence: Bin Chen, Y2hlbmJpbjEwMDdAaG90bWFpbC5jb20=; Jiandong Qiu, amlhbmRvbmdxaXVAMTYzLmNvbQ==