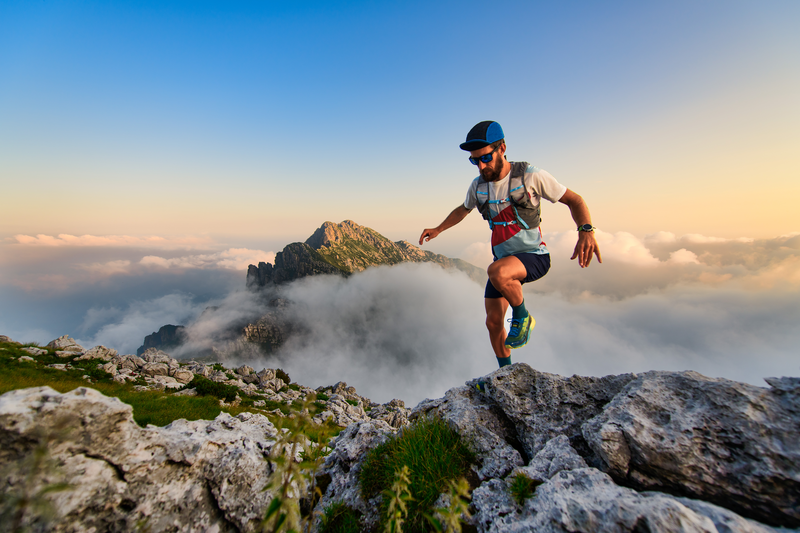
94% of researchers rate our articles as excellent or good
Learn more about the work of our research integrity team to safeguard the quality of each article we publish.
Find out more
METHODS article
Front. Mar. Sci. , 23 December 2024
Sec. Marine Megafauna
Volume 11 - 2024 | https://doi.org/10.3389/fmars.2024.1518710
Understanding the behaviour and ecological role of elasmobranchs is essential for their conservation, particularly in regions harbouring threatened and data-deficient species like the Inhambane coastline in southern Mozambique, southwestern Indian Ocean. This study employed long-life remote underwater video cameras (LL-RUV) to observe elasmobranch occurrence and behaviour at a rocky reef near Praia do Tofo known for frequent elasmobranch sightings. Between 2021 and 2024, 524 hours of LL-RUV data were collected, revealing the presence of 14 threatened and data-deficient elasmobranch species. Reef manta rays (Mobula alfredi), small eye stingrays (Megatrygon microps), and oceanic manta rays (Mobula birostris) were identified as the primary elasmobranch users of the studied cleaning stations (n = 81, n = 72 and n = 61 respectively), engaging in mutualistic interactions with cleaner fish and spending significant amounts of time at the studied reef. In contrast, spotted eagle rays (Aetobatus narinari) and blacktip sharks (Carcharhinus limbatus) were solely observed cruising over the reef without engaging in cleaning interactions (n = 40 and n = 27 respectively). In addition, this study provides evidence of intra- and inter-specific interactions between reef and oceanic manta rays, small eye stingrays and bowmouth guitarfish (Rhina ancylostoma) and co-occurrences of both manta ray species and smalleye stingrays with “hitchhiker” species including cobia (Rachycentron canadum) and remoras (Echeneis spp.). The use of LL-RUV cameras represents a significant advancement in marine research. These cameras enable continuous, non-intrusive, long-term monitoring of marine environments, capturing natural behaviours, while minimising disturbances caused by divers. As a result, more accurate observations of elasmobranch behaviour and cleaning station dynamics can be recorded, which might be missed during short-term surveys. This research highlights the potential of LL-RUV cameras as powerful tools for advancing our understanding of elasmobranch occurrence and behaviour and underscores their value in informing targeted and effective conservation strategies. Beyond elasmobranch research, LL-RUVs hold tremendous potential for studying a wide range of marine species and habitats, offering a versatile tool for ecological monitoring and conservation efforts across diverse marine ecosystems.
Elasmobranchs are essential to the health of marine ecosystems, often functioning as top predators or keystone species within their environments (Stevens, 2000; Ferretti et al., 2010; Heithaus et al., 2010). Their roles in controlling prey populations and facilitating ecosystem stability make them critical to sustaining or restoring marine biodiversity (Heupel et al., 2014; Barría et al., 2015; Roff et al., 2016; Shipley et al., 2023). Understanding the behaviour and ecological role of elasmobranchs is critical for their conservation and management, particularly in regions harbouring threatened and data-deficient species such as the Inhambane coastline in southern Mozambique (Heupel et al., 2007; Block et al., 2011; Speed et al., 2011; Pereira, 2021). Despite their ecological significance, numerous elasmobranch species are under threat from anthropogenic pressures (Couturier et al., 2012; Worm et al., 2013; Davidson et al., 2016; O’Malley et al., 2017). Dulvy et al. (2021) report that more than one third of chondrichthyans, which includes elasmobranchs, are threatened with extinction according to IUCN Red List criteria. In particular, overexploitation by fisheries and high rates of incidental bycatch are rapidly affecting marine biodiversity and reducing biomass, which is evidenced by the steep declines in shark and ray populations witnessed during the last decades (Myers and Worm, 2003; Rohner et al., 2013; McCauley et al., 2015; Dulvy et al., 2021). Beside deepwater and pelagic elasmobranchs, large-bodied, long-lived coastal elasmobranch species are vulnerable to such pressures, with five out of the seven most threatened families being rays (sawfishes, wedgefishes, sleeper rays, stingrays, guitarfishes) and two being sharks (angel sharks, thresher sharks) (Dulvy et al., 2014, 2021). The protracted reproductive cycles and low fecundity rates characteristic of elasmobranchs further exacerbate their vulnerability to overfishing and population declines (Marshall and Bennett, 2010; Musick et al., 2020). These traits limit population recovery, resilience, and the functional roles these species play in marine ecosystems. Combined with increasing anthropogenic pressures, this underscores the urgent need for comprehensive conservation strategies and sustainable management practices to mitigate ongoing declines and ensure their preservation.
The coastline of the Inhambane province in southern Mozambique is considered a hotspot for marine biodiversity (Rohner et al., 2013; O’Connor and Cullain, 2021; Pereira, 2021) and a growing destination for dive tourism due to the presence of flagship species such as whale sharks (Rhincodon typus) and manta rays (Mobula alfredi, Mobula birostris) (Tibiriçá et al., 2011; Venables et al., 2020). Mozambique harbours many threatened and data-deficient elasmobranch species, for which information on occurrence, population size and structure and distribution is currently lacking (O’Connor and Cullain, 2021; Pereira, 2021). As such, Mozambique can be assessed as a data-poor area. Among those species are mobulid rays (M. alfredi, M. birostris, Mobula kuhlii), stingrays (Megatrygon microps, Taeniurops meyeni, Pateobatis jenkinsii), eagle rays (Aetobatus narinari, Aetomylaeus vespertilio), guitarfish (Rhina ancylostoma), wedgefish (Rhynchobatus australiae, Rhynchobatus djiddensis), whale sharks (R. typus), requiem sharks (Carcharhinus amblyrhynchos, Carcharhinus leucas, Carcharhinus limbatus, Carcharhinus obscurus, Triaenodon obesus), leopard sharks (Stegostoma tigrinum) and hammerhead sharks (Sphyrna lewini) (Marshall, 2008; Guillaume and Séret, 2021; Keeping et al., 2021; Pereira, 2021; Venables et al., 2022). A comprehensive list of elasmobranchs present in the Inhambane province is provided in Table 1. Based on its diverse elasmobranch fauna and its importance for sharks and rays, the Southern Inhambane Province was recently recognised as an Important Shark and Ray Area (ISRA) by the IUCN, underscoring the ecological significance and high biodiversity of this area (IUCN SSC Shark Specialist Group, 2023). This designation highlights the need for targeted conservation efforts and supports prioritisation of the region in global initiatives, emphasising the importance of addressing data gaps to inform sustainable management and protect vulnerable species.
Table 1. Overview of elasmobranch species present in the southern Inhambane province of Mozambique (IUCN SSC Shark Specialist Group, 2023; Ross Newbigging, personal communication, 2024).
Understanding critical habitats for these species is fundamental in implementing targeted conservation strategies and delineating priority areas for conservation efforts. Cleaning stations in particular are of high interest for conservation as these sites are important ecological systems that facilitate mutualistic cleaning interactions between cleaner organisms and their clients (Grutter, 1997; Côté, 2000) and serve as key aggregation sites for many species including large-bodied elasmobranchs (Marshall and Bennett, 2010; Deakos et al., 2011; Oliver et al., 2011; Stevens, 2016; Caves, 2021). Cleaning stations play a vital role in the health of many marine species, as specialised cleaner fish remove ectoparasites, dead tissue and other unwanted materials from clients’ bodies (Youngbluth, 1968; Losey and Margules, 1974; Grutter, 1996; Cheney and Côté, 2001). Cleaning stations may also support wound healing processes in client species, as cleaners reduce injury redness associated with inflammation and potentially prevent infections from secondary pathogens (Vaughan, 2018). Investigating the significance of cleaning stations and their role in the behaviour of elasmobranchs offers valuable insights into the abundance and ecology of these species as well as conservation measures necessary for preserving these critical habitats and safeguarding the associated elasmobranch species.
Observing elasmobranch occurrence and behaviour has historically been challenging due to their elusive nature, constraints imposed by traditional research methods and the difficulties of conducting underwater investigations in the challenging marine environment, which also poses barriers to the study of marine fishes (Pratt and Carrier, 2001; Edgar et al., 2004). Biases introduced by the presence of human observers, the restricted duration of underwater observation and elasmobranchs’ high mobility complicate consistent monitoring (Edgar et al., 2004; Lee et al., 2016). Remote camera research, including baited remote underwater video systems (BRUVs), has increasingly been used in shark science in recent years, as it overcomes many of these challenges. BRUVs utilise bait to attract elasmobranchs and other marine species into the camera’s field of view, facilitating observations of occurrence, abundance and behaviour (Whitmarsh et al., 2017; Bouchet et al., 2018). However, the use of bait may also introduce biases by altering the animals’ natural behaviours and attracting species from outside the area of interest (Goetze et al., 2019). In contrast, non-baited remote underwater video (RUV) systems provide an opportunity to observe marine species in their natural behaviours without bait-induced biases (Oliver et al., 2011; Barr and Abelson, 2019; Peel et al., 2020). Previous studies primarily used action cameras and therefore faced limitations due to their short battery life and constrained recording duration (Oliver et al., 2011; Barr and Abelson, 2019; Zarco-Perello and Enríquez, 2019; Peel et al., 2020). The development of long-life remote underwater video cameras (LL-RUV), such as the Open Ocean Camera© (OOCAM) used in this study, marks a crucial advancement in the study of elusive marine species. With its extended battery life, the OOCAM allows for continuous, non-intrusive monitoring over extended time periods, overcoming the limitations of previous RUV systems. By providing continuous monitoring, the OOCAM allows for a more thorough assessment of reef activity and substantially enhances our understanding of elasmobranch behaviour. It also increases the likelihood of capturing rare or elusive species and behaviours that might be missed during short-term surveys or deterred by the presence of divers (Bilodeau et al., 2022; Cordier et al., 2022). Additionally, LL-RUVs can capture behaviours at dawn and dusk, which can be outside of usual SCUBA diving operator hours. The extended monitoring capacity by LL-RUVs also provides valuable insights into the dynamics of marine ecosystems, contributing to more effective conservation and management strategies. This study marks the first time an LL-RUV system like the OOCAM is used in a marine environment, offering a unique opportunity for long-term, non-intrusive observation of elusive marine species.
This research aims to investigate the efficacy of LL-RUV cameras in observing the behaviours of some of the rarest and most threatened marine megafauna species, without using bait (as with BRUVs) or disturbance by divers (as with DOVs). By strategically placing LL-RUV cameras at cleaning stations known for frequent elasmobranch sightings, this study seeks to assess the occurrence and behaviour of threatened and data-deficient elasmobranch species as well as intra- and inter-specific interactions.
Research was conducted in Praia do Tofo in the Inhambane province on the southern Mozambican coast (Figure 1) between July 2021 and March 2024. The study site, known as Giants Castle (-23.86°S, 35.55°E), lies within Tofo Bay and consists of a rocky reef wall at a depth of 26 – 35 metres below sea level, stretching approximately 120 metres. The reef wall runs from north to south with a steep slope descending along the eastern side and features several “cleaning station” regions, which are recognisable by their abundance of cleaner fish. The site experiences a predominant north-south current, and water visibility ranges from 1 to 25 metres depending on weather conditions, tidal cycles, and particulate matter in the water column. Giants Castle was chosen as the study site for this research due to the high frequency of elasmobranch sightings compared to other sites in the region (Marshall, 2008; Rohner et al., 2013; Venables et al., 2020; Keeping et al., 2021), which maximises the likelihood of capturing these species with the remote cameras. Giant’s Castle is also one of the more accessible diving areas from the boat launch site, allowing for frequent camera deployments.
Trial camera deployments were conducted to identify areas with the highest frequency of elasmobranch sightings. Two areas characterised by large schools of sea goldies (Pseudanthias squamipinnis) provided the most consistent sightings whilst facing east across the Giant Castle reef wall (hereafter cleaning station A and cleaning station B) (All Out Africa, unpubl. data) and were therefore selected for camera deployments in the present study (Figure 2).
Figure 2. Map of the studied reef, known as Giants Castle, adapted from Liquid Dive Adventures (2024). Three cleaning stations were identified at locations A, B and C (denoted by green circles) and camera stations were set up at cleaning station A and cleaning station B (denoted by camera icons). The OOCAM was consistently deployed in the same location at cleaning station B, whereas GoPro™ Hero 4 cameras were consistently deployed in the same location at cleaning station A.
An Open Ocean Camera© (OOCAM) (Clear Robotics Limited, 2021) was used to collect LL-RUV data for the study. The OOCAM was encased in an acrylic tube housing, equipped with temperature, pressure and light sensors, collecting environmental data every second during video recordings. It features a Sony IMX219 sensor with a resolution of 12.3 megapixels, a 400 Wh battery pack of approximately 148 hours recording capacity and a Raspberry Pi computer chip. The Raspberry Pi computer chip was set to have the camera record at three- to four-hour intervals between 06:00 and 18:00 at 1080p resolution, a frame rate of 15 frames per second (FPS) and a 90° field of view. The camera system was modified with a Nauticam vacuum check and leak detection system (Nauticam, 2023). To ensure camera stability and prevent equipment damage or loss from strong currents, the camera housing was attached to a metal frame and secured to a rock on the reef using ski rope (Figure 3A). While deployments in this study were conducted on rocky reef substrates, LL-RUV systems like the OOCAM can also be deployed on sandy or soft-bottom substrates if adequately weighed down to ensure stability. Care must be taken during reef deployments to avoid damaging coral structures. Researchers deployed the camera system at cleaning station B facing east across the narrow part of the reef using a lift bag. After each deployment, the camera was retrieved by the researchers and video data was downloaded for analysis.
Figure 3. Camera set-up at the two cleaning stations at Giants Castle. (A) Open Ocean Camera© at cleaning station B. The OOCAM was attached to metal frame, which was fixed to a rock on the reef using ski rope. (B) GoProTM Hero 4 camera in GoProTM underwater housing at cleaning station A. The camera station at cleaning station A consisted of a cement block into which the camera mount was placed before the cement dried. In addition, the cement block was tied to a rock on the reef using ski rope to prevent camera loss and damage to the reef.
In addition to the OOCAM, GoPro™ Hero 4 cameras were positioned at cleaning station A. GoPro™ cameras were mounted in a cement block, tied to a rock on the reef using ski rope to prevent camera loss (Figure 3B). GoPro™ cameras were set to record at 1080p resolution, 60 FPS and offered a 170° field of view. External battery packs were employed for the first three deployments in August 2022. Subsequent deployments used standard GoPro™ batteries. In the same manner as the OOCAM, GoPro™ cameras were deployed and retrieved by researchers. Camera deployments were conducted according to field conditions and the dive schedule of the commercial dive operator supporting this research.
Video footage was analysed using VLC Media Player Version 3.0.18 (VideoLan, 2006). Sightings of elasmobranchs were systematically recorded by trained citizen scientists and monitored by staff from the organisation All Out Africa (AOA). All contributing citizen scientists received standardised training on shark and ray identification via presentations by AOA staff. AOA staff administering training and monitoring data input had a minimum of M.Sc. degree in the field of marine science. Each sighting began when an elasmobranch individual entered the video frame for the first time and ended after it left the frame and did not return for more than five minutes (Oliver et al., 2011). For the purpose of this study, only sightings where animals could be identified to species level were included. Uniquely identifying markings such as spot patterns, predatory bite marks, injuries, or tail length were used to differentiate between individuals. Citizen scientists recorded the time the elasmobranch entered and exited the frame and the direction of travel. In addition, camera type (OOCAM or GoPro™), camera position on the reef (cleaning station A or cleaning station B), video file name and date were noted. A new data entry row was used for every new animal entering the video frame. All records of elasmobranch sightings on remote video cameras were confirmed by the researchers, who then also recorded additional information on displayed behaviours (Table 2), co-occurrence with other elasmobranchs and the presence of “hitchhiker” species (i.e. cobia (Rachycentron canadum) and remoras (Echeneis spp.)). Further assessment of elasmobranch behaviours was restricted to species with 25 or more sightings to ensure data reliability and reduce the risk of potential anomalies skewing the results of this study. A random subset of 10% of the video footage analysed by citizen scientists was reviewed by AOA staff for quality control purposes.
Table 2. Ethogram for sharks, and rays based on Klimley et al. (2023).
This research used remote underwater video, which is a non-invasive sampling method. No animal was captured, handled or removed from its natural environment for the purpose of this study. Research activities described in this paper were approved by the University of Gibraltar’s ethics committee under the ethics policy for research involving animals and their environment (ID 080/2023/UniGib). Fieldwork was conducted with the permission of the Museu de História Natural de Moçambique and adhered to the safety and scientific regimen of All Out Africa.
Between July 2021 and October 2023, the OOCAM was deployed six times, resulting in 442 hours of remote underwater video data. Recording lengths per deployment varied from 21 hours to 134 hours, with an average of 63 hours. 58 GoPro™ deployments between August 2022 and March 2024 attained 82 hours of video data. Each deployment varied from 0.3 to 5.5 hours with an average duration of 1.4 hours per deployment. External battery packs were employed for the first three GoPro™ deployments in August 2022 to prolong battery life. However, the external extension cables caused corrosion to the seals of the cameras’ underwater housings, resulting in camera flooding. Consequently, subsequent deployments used standard GoPro™ batteries. LL-RUV data is summarised in Table 3.
Table 3. Overview of LL-RUV data collected using the Open Ocean Camera© and GoPro™ Hero 4 cameras during the study period.
The LL-RUV recordings provide evidence of the occurrence of 14 elasmobranch species at the studied cleaning stations, all of which are classified as critically endangered, endangered, vulnerable or data deficient on the IUCN Red List (Table 4). The OOCAM recorded a total of 254 elasmobranch sightings during the study period. These were reef manta rays (n = 63), smalleye stingrays (n = 58), oceanic manta rays (n = 40), spotted eagle rays (n = 30), blotched fantail rays (n = 20), fevers of shortfin devil rays (n = 4, total of 15 individuals), bowmouth guitarfish (n = 10), blacktip sharks (n = 19), Jenkins whiprays (n = 5), pink whiprays (n = 1), scalloped hammerhead sharks (n = 1), grey reef sharks (n = 1), whale sharks (n = 1) and smoothnose wedgefish (n = 1). The GoPro™ camera station yielded 86 elasmobranch sightings: Oceanic manta rays (n = 21), reef manta rays (n = 18), smalleye stingrays (n = 14), spotted eagle rays (n = 10), bowmouth guitarfish (n = 8), blacktip sharks (n = 8), fevers of shortfin devil rays (n = 5, total of approximately 196 individuals), blotched fantail rays (n = 1) and scalloped hammerhead sharks (n = 1). In combination, reef manta rays (n = 81), smalleye stingrays (n = 72), oceanic manta rays (n = 61), spotted eagle rays (n = 40) and blacktip sharks (n = 27) were the most frequently recorded animals at the studied cleaning stations (Table 4).
Table 4. Overview of sightings per species for both OOCAM and GoProTM as well as total number of sightings per species.
Of the elasmobranch species recorded more than 25 times (reef manta ray, smalleye stingray, oceanic manta ray, spotted eagle ray and blacktip shark), each exhibited either cleaning or cruising behaviours. No feeding or courtship behaviours were observed (Figure 4). Out of the 81 sightings of reef manta rays, 78% displayed cleaning behaviour. The remaining 22% of sightings showed reef manta rays cruising over the reef with their cephalic lobes rolled and mouth closed. Oceanic manta rays also predominantly exhibited cleaning behaviour (72% of sightings), with the remaining 28% of sightings showing oceanic manta rays cruising over the reef. Smalleye stingrays were primarily observed cleaning (93% of sightings). The remaining observations of smalleye stingrays (7%) showed them cruising over the reef without slowing down or circling back into frame. Unlike manta rays and smalleye stingrays, spotted eagle rays were solely observed cruising in mid-water (n = 13) or close to the reef (n = 27), either individually (n = 13) or fevers of up to five individuals (n = 27). No cleaning behaviour or other behaviours were recorded by RUV cameras. Blacktip sharks, the only shark species recorded by LL-RUV cameras more than 25 times, were observed cruising either mid-water (n = 13) or close to the reef (n = 14). As was the case with spotted eagle rays, no other behaviours were observed for blacktip sharks.
Figure 4. Overview of observed behaviours for species with more than 25 sightings across camera types.
RUV cameras captured 14 instances of between two and five reef or oceanic manta ray individuals, passing in and out of the camera’s field of view, alternately circling the cleaning station. On three occasions, individuals of both species were observed alternately travelling over the cleaning station within approximately one minute of each other. On eight occasions, RUV cameras recorded two smalleye stingray individuals travelling over the cleaning station within less than eight minutes of each other, four of which were observed in frame together. Both reef and oceanic manta rays were recorded at the cleaning station within less than one minute of a smalleye stingray (n = 5), some even within three to six seconds of the smalleye stingray (n = 3). Reef manta rays were also observed circling the cleaning station alternately with bowmouth guitarfish (n = 3). Of these, there were two instances where both the reef manta ray and bowmouth guitarfish were captured in frame together. In one instance, both simultaneously turned and swam off in different directions (Supplementary Materials 1), in the other the bowmouth guitarfish passed behind the reef manta ray with no noticeable reaction by either animal. On one occasion, six reef manta ray individuals, two smalleye stingray individuals and one bowmouth guitarfish were observed alternately travelling over the cleaning station, moving in and out of frame repeatedly, for more than 90 minutes. Additionally, a smalleye stingray and a pink whipray were recorded once in frame together at the cleaning station, both cleaning and travelling in and out of frame together.
Both manta ray species and smalleye stingrays were observed travelling with “hitchhiker” species (Figure 5). Smalleye stingrays were accompanied by between one to twelve cobia in 63% of sightings, with an average of 4.2 cobia per sighting. In contrast, reef manta rays were observed with cobia in only 6% of sightings, with an average of 1.4 individuals. Oceanic manta rays were not observed in association with cobia. Both reef and oceanic manta rays were observed travelling with remoras, although the incidence and number of remoras were higher for oceanic manta rays (75% of sightings, with an average of 3.2 individuals and a maximum of 8 individuals) compared to reef manta rays (31% of sightings, with an average of 2.2 individuals and a maximum of 3 individuals). Smalleye stingrays were observed with remoras on three occasions (4% of sightings), with an average of one individual.
Figure 5. Percentage reef manta ray, oceanic ray, and smalleye stingray sightings where “hitchhikers” (remoras and / or cobia) were present.
This study has reported the first insights into using long-life, remote and unbaited cameras to observe the occurrence and behaviour of threatened and data-deficient elasmobranchs. The recorded species, in order of abundance of sightings, were reef manta rays, smalleye stingrays, oceanic manta rays, spotted eagle rays, blacktip sharks, bowmouth guitarfish, blotched fantail rays, Jenkins whiprays and scalloped hammerhead sharks. Pink whiprays, grey reef sharks, whale sharks and smoothnose wedgefish were only observed once. Shortfin devil rays were observed in fevers of more than 50 individuals on numerous occasions. In total, 14 species were observed (Table 2), compared to the 37 species known to occur in the region (Table 1). Several species listed in Table 1 were recorded only rarely, based on reports from local dive centres, including the great white shark (Carcharodon carcharias), Java shark (Carcharhinus amboinensis), shorttail nurse shark (Pseudoginglymostoma brevicaudatum), silvertip shark (Carcharhinus albimarginatus), tiger shark (Galeocerdo cuvier), bottlenose wedgefish, giant devil rays (Mobula mobular), ornate eagle rays, and cownose rays (Rhinoptera bonasus), and were not captured by the remote cameras. More than 20 observations of unidentified sharks, visible only as silhouettes and too far from the camera for clear identification, could correspond to undetected species such as bull sharks, silvertip sharks and dusky sharks. For research on pelagic sharks, BRUVs may be more effective, as bait attracts sharks to the camera, allowing for species identification. Additionally, bottom-dwelling ray species, such as bluespotted maskrays (Neotrygon caeruleopunctata), bluespotted stingrays (Taeniura lymma), and torpedo rays (Torpedo marmorata, Torpedo sinuspersici), were not detected by the camera system. These limitations are further discussed in section 4.4.
Through direct human observation techniques, such as dive and snorkel surveys and photo-identification, as well as acoustic and satellite telemetry, much is known about the presence of some of the more frequently observed species, such as manta rays, devil rays and whale sharks, along the Inhambane coastline. Such studies have addressed their population size and structure (Brooks et al., 2010; Marshall et al., 2011), trends in sightings (Rohner et al., 2013; Venables et al., 2024) and movement patterns (Rohner et al., 2018; Venables et al., 2020; Daly et al., 2023; Marshall et al., 2023). Much less is known about the lesser-seen elasmobranch species, such as smalleye stingrays, bowmouth guitarfish and other ray and wedgefish species. LL-RUV observations from the present study provide valuable insights that can inform conservation efforts, such as fisheries management, to protect habitats important to the livelihood of rare and threatened species.
Observed behaviours at the study site revealed distinct patterns among various elasmobranch species, shedding light on their utilisation of these critical habitats. Remote underwater video data demonstrated that reef manta rays, smalleye stingrays and oceanic manta rays are the most frequent elasmobranch users of the studied cleaning stations, engaging in mutualistic cleaning interactions with cleaner fish. Such cleaning interactions are vital for their health, as cleaner fish remove and ingest ectoparasites, dead tissue and other unwanted materials from clients’ bodies (Youngbluth, 1968; Losey and Margules, 1974; Grutter, 1996; Cheney and Côté, 2001), thereby improving the overall health of client species and potentially reducing the risk of infections (Grutter et al., 2003; Vaughan, 2018). Manta rays’ use of certain cleaning stations over others has previously been linked to the proximity to coastal feeding grounds (Rohner et al., 2013; Murie and Marshall, 2016). In the Praia do Tofo region, reef and oceanic manta rays can be observed feeding within a well-established year-round whale shark feeding aggregation area, located two kilometres south of the study site (Rohner et al., 2018). Such sightings support the theory of manta rays visiting cleaning stations in proximity to good feeding sites, which would explain the high number of manta ray sightings at the studied reef.
Cleaning behaviour by manta rays has previously been described as the ray reducing its swimming speed when approaching a cleaning station, hovering or circling above the reef and making repeated passes over a cleaning station while being inspected by cleaner fish (Marshall, 2008; Jaine et al., 2012; Kitchen-Wheeler, 2013). LL-RUV footage provides evidence of similar cleaning behaviours in smalleye stingrays. These rays were observed either hovering over the cleaning station while facing into the current or slowly moving their pectoral fins to maintain position, allowing cleaner fish to approach. After passing over the cleaning station, they would circle around and allow the current to carry them back to their initial position, before turning again and repeating the behaviour.
In contrast, spotted eagle rays and blacktip sharks were observed passing by the cleaning stations without actively engaging in interactions with cleaner fish. They were observed swimming in mid-water or cruising close to the reef but did not exhibit cleaning behaviour when in close proximity to the reef. This behavioural pattern raises questions about the cleaning behaviour of the two species, prompting further investigation into their preferred cleaning sites. In French Polynesia, ocellated eagle rays (Aetobatus ocellatus) were observed chafing on patches of sandy bottom, which was suggested as their primary cleaning behaviour or an addition to cleaning station visits (Berthe et al., 2016). Research by Oliver et al. (2011) on pelagic thresher sharks (Alopias pelagicus) in the Philippines provides valuable insights into shark cleaning behaviour at seamounts, suggesting potential avenues for exploring the observed differences in the utilisation of cleaning stations by various elasmobranch species. Seamounts and other offshore cleaning sites likely look fundamentally different from coastal cleaning stations in terms of cleaner fish assemblages, as different reefs may have co-evolved with different cleaning requirements and client species. Deploying camera systems strategically at different cleaning sites in different environments would help to illuminate the overall function of cleaning stations for different elasmobranch species and whether species that were not observed cleaning in the present study visit other reefs for cleaning purposes. Addressing questions regarding the use of cleaning station by different species requires a comprehensive assessment of reef topology and structure, cleaner fish abundance and composition, as well as considerations of currents and the risk of predation at the studied reef and other nearby cleaning stations. Further research into the cleaning ecology of elasmobranchs may yield valuable insights into the complex dynamics of cleaning interactions in marine ecosystems, thereby facilitating more effective conservation strategies for these vulnerable species.
The documented intra- and interspecific interactions observed at the studied reef provide novel insights into the complex social dynamics of rare and little-known elasmobranchs. The frequent presence of both species of manta ray and smalleye stingrays at the studied reef underscores the significance of the Praia do Tofo region for threatened elasmobranch populations in the Inhambane province, despite their dramatic documented decline in sightings (Venables et al., 2024). Observations of multiple individuals of the same species, as well as individuals from different species, utilising the same cleaning station concurrently, suggest a level of tolerance and cooperative behaviour among these marine organisms. The behavioural patterns exhibited by reef and oceanic manta rays, such as alternately swimming over the cleaning station, imply a degree of social organisation. Similar cooperative behaviours have been documented in other elasmobranch species including lemon sharks (Negaprion brevirostris), scalloped hammerhead sharks (Sphyrna lewini) and great white sharks (Carcharodon carcharias) (Klimley, 1983; Guttridge et al., 2011; Jacoby et al., 2012; Micarelli et al., 2023).
The behaviour observed between a reef manta ray and a bowmouth guitarfish, where they turned and swam away in different directions upon encountering each other at the cleaning station, raises questions about resource partitioning at cleaning stations. Further research into the ecological niches and behavioural repertoires of different elasmobranch species is warranted to elucidate the mechanisms underlying such interactions and their implications for community dynamics. Future research should focus on exploring how the presence of a combination of species simultaneously soliciting cleaning services affects the quality of cleaning received by each individual, whether there is a hierarchy among client species, or whether cleaner fish preferentially clean certain ray species over others.
The observation of a smalleye stingray and a pink whipray travelling together, with the pink whipray closely trailing the smalleye stingray in and out of frame, supports findings from the Great Barrier Reef in Australia, where pink whiprays have been observed piggybacking on smalleye stingrays (Meekan et al., 2016). This is the only such observation in this study, and further research is required to better understand this behaviour and its ecological significance.
The presence of “hitchhiker” species alongside smalleye stingrays and manta rays suggests potential symbiotic relationships or ecological strategies (Becerril-García et al., 2020; Nicholson-Jack et al., 2021; Pate et al., 2021). Oceanic manta rays, in particular, were frequently observed traveling with up to eight remoras attached to their ventral surface, while reef manta rays were also observed with remoras but less frequently and in lower numbers. These remoras, which feed primarily on zooplankton, attach themselves to manta rays and other megafauna species to be carried to feeding areas (Nicholson-Jack et al., 2021). In the process, they reduce the hydrodynamic swimming efficiency of their host, but it is possible that they also dislodge larger parasites (Nicholson-Jack et al., 2021). Smalleye stingrays and reef manta rays were also observed travelling with cobia on multiple occasions. The association of cobia especially with smalleye stingrays could indicate commensalism, where cobia benefit from protection or access to food resources provided by the larger rays (Michael, 1993). Further research is required to fully understand the nature and extent of these associations and their ecological implications.
The use of remote underwater video cameras has proven to be a non-invasive research method that holds significant potential for studying the undisturbed, unbaited, natural behaviours of some of the rarest and most threatened marine megafauna species. Remote underwater video cameras, especially LL-RUV cameras, offer a range of advantages over traditional research methods. RUV allows researchers to observe and document the occurrence and behaviour of marine species without the need for human interaction, thus minimising disturbance to the animals and reducing observational bias caused by animals altering their behaviour in the presence of divers (Becker et al., 2010; Dickens et al., 2011; Lee et al., 2016; Zarco-Perello and Enríquez, 2019). Previous RUV studies primarily used action cameras and therefore faced limitations due to their short battery life and constrained recording duration (Oliver et al., 2011; Barr and Abelson, 2019; Zarco-Perello and Enríquez, 2019; Peel et al., 2020).
This study demonstrates that LL-RUV cameras provide more comprehensive data on the occurrence and undisturbed behaviours of rare elasmobranch species than direct human observation techniques by offering uninterrupted video footage over extended periods (Bilodeau et al., 2022; Cordier et al., 2022). The extended recording duration of LL-RUV cameras also minimises disturbances caused by the presence of divers, their bubbles, or boat noise, which can deter elasmobranchs from cleaning stations, leading to them being missed during short-term surveys (de Vincenzi et al., 2021; Bilodeau et al., 2022; Cordier et al., 2022; Mickle and Higgs, 2022). Continuous monitoring has the potential to provide valuable insights into diurnal and seasonal variations in habitat use, undisturbed behaviours of various species, predator-prey interactions and other ecological dynamics, while also capturing activity during sunrise and sunset periods when divers are absent from the reefs. In contrast, the limited recording window of GoPro™ cameras means that video is only captured within 90 minutes of diver disturbance, which could lead to certain species avoiding the area, thus highlighting the advantage of using LL-RUV cameras for continuous, uninterrupted monitoring during all daylight hours.
Despite their numerous advantages, LL-RUV cameras have some limitations that should be acknowledged. The quality of video footage can be significantly affected in areas with low water visibility or high turbidity, such as near estuaries or river mouths (Figueroa-Pico et al., 2020; Jones et al., 2021). Under these conditions, animal sightings may be missed, particularly for species that do not approach the camera closely. While this issue also affects diver-based surveys, it highlights the importance of deploying LL-RUV cameras under favourable environmental conditions to avoid skewed results. For shark research, the absence of bait in LL-RUV setups can pose a challenge. BRUV systems are more effective for attracting sharks closer to the camera, enabling easier species identification and behavioural observations (Whitmarsh et al., 2017; Prat-Varela et al., 2023). By contrast, the LL-RUV camera used in this study is particularly well-suited to studying the natural cleaning behaviours of rays, such as manta rays and smalleye stingrays, which frequent cleaning stations. These species are less likely to respond to bait, making LL-RUVs a more appropriate tool for documenting their behaviour. Furthermore, baiting can introduce biases, as different species respond differently depending on bait type, and some, such as filter feeders, are entirely unaffected. The non-baited nature of LL-RUV systems allows for the observation of undisturbed, natural behaviours, providing a broader ecological perspective. Additionally, LL-RUVs avoid ecological disturbances caused by bait, which can disrupt local food webs or attract predators unnaturally. Unlike BRUVs, which often favour carnivorous or scavenging species, LL-RUVs capture a broader representation of marine communities, including herbivorous and filter-feeding species. The extended recording durations of LL-RUV cameras facilitate long-term, continuous monitoring, capturing behaviours and interactions across various times of the day, including periods when divers or baited systems are absent. Finally, by minimising diver presence, LL-RUV cameras reduce human-induced disturbance, making them an invaluable tool for studying sensitive habitats or species.
Although this study focused on the advantages of the LL-RUVs, it is important to also recognise the strengths of actions cameras like GoPro™ cameras in capturing high-resolution footage with a wider field of view. The superior video quality of GoPro™ cameras facilitated the identification of elasmobranch species, as their distinctive visual markings were visible more clearly, resulting in fewer unidentified sharks and rays in the dataset for GoPro™ recordings compared to the OOCAM. GoPro™ cameras were able to capture fevers of devil rays of up to 55 individuals in contrast to the OOCAM, which recorded only individual devil rays or fevers of up to six individuals. This disparity is likely due to the GoPro™ cameras’ wider field of vision, which enabled them to capture mid-water sightings as well as documenting cleaning stations dynamics. Depending on the research focus, four GoPro™ cameras spaced 90° apart can provide a more comprehensive view of reef activity and reduce the risk of missing rare species or behaviours. Alternatively, 360°cameras, which offer a comprehensive environmental view, could also be considered, though they share the same short battery life challenge as GoPro™ cameras (Auster and Giacalone, 2021; Hemery et al., 2022). Future studies should seek to combine the benefits of both camera systems, with LL-RUVs’ extended battery life and continuous monitoring capabilities complementing the high-resolution video and broader field of view of action cameras. This combination could provide an even more comprehensive approach to studying marine megafauna, enabling researchers to capture both cleaning behaviours and pelagic species occurrences while maximizing the strengths of each system in documenting diverse ecological dynamics.
Further analysis of temporal trends in sightings, ideally using LL-RUV cameras that capture all hours of the day, could provide additional insights into observed differences in species occurrence and behaviour. Elasmobranch visitations to the studied cleaning stations may be more frequent during the hours when SCUBA divers are present on the reefs (08:00 until 14:00), which is also when GoPro™ deployments are possible. A more continuous, non-invasive monitoring system like the OOCAM could help to enrich the data by capturing these temporal patterns over longer periods, providing a clearer understanding of species distribution and behaviour.
The main challenge of RUV-based research, especially long-term or continuous monitoring, is that data processing can be time-consuming, creating a bottleneck in data analysis (Erickson et al., 2023; Magneville et al., 2023). However, recent advancements in machine learning and computer vision algorithms have enabled automated species recognition, behaviour analysis and object tracking in large video datasets (Ditria et al., 2020, 2021; Marrable et al., 2022). These technological advances in artificial intelligence and machine learning have the potential to significantly reduce the time and effort required for data processing and analysis, thereby facilitating comprehensive, long-term monitoring of marine environments. Consequently, the use of RUV and associated technologies could open new avenues for efficient marine research, especially in the age of big data, leading to more timely development for conservation strategies. Future research should focus on developing automated video analysis and species recognition techniques to streamline the data analysis process, making it feasible to continuously monitor different reefs. This would enable comparisons across reefs and provide deeper insights into ecosystem dynamics. An example of this advancement is FishID, an AI-system that identifies, counts and measures aquatic animals and plants (FishID, 2024). Developed as part of The Global Wetlands Project at Griffith University, FishID exemplifies how technology can enhance marine conservation (FishID, 2024). Other organisations are also integrating AI solutions for environmental monitoring, showcasing the potential of technology to improve our understanding of marine ecosystems and conservation efforts.
In lieu of an automated data processing technique, contributions by trained citizen scientists in the analysis of LL-RUV footage were instrumental in this study. Previous research has shown that, if trained well, citizen scientists can acquire the knowledge and skills essential for contributing to scientific research (Norman et al., 2017; Keeping et al., 2021; Magson et al., 2022). Kosmala et al. (2016) and Chin and Pecl (2018) outline potential issues with the quality of data that can arise when using citizen scientists instead of trained scientists, but issues relating to the accuracy of data can be minimized with training and supervision of citizen scientists as well as expert validation of records (Kosmala et al., 2016; Keeping et al., 2021). In the present study, citizen scientists were trained on shark and ray identification through presentations and in-water training by AOA staff with a minimum of M.Sc. degree in a related field. All records of megafauna sightings on remote video cameras were confirmed by the researchers, who re-watched all video segment included in this study. In addition, a random subset of 10% of the video footage analysed by citizen scientists was reviewed by AOA staff to ensure sightings were recorded correctly.
This study demonstrates the effectiveness of long-life remote underwater video cameras in capturing undisturbed, unbaited, natural behaviours of elasmobranchs in southern Mozambique, a region known for harbouring many threatened and data-deficient species. The use of LL-RUV allowed for long-term monitoring of the studied site, providing a comprehensive overview of elasmobranch occurrence and behaviour. Video recordings provided evidence of 14 elasmobranch species, which are classified as critically endangered, endangered, vulnerable, or data-deficient by the IUCN, highlighting the importance of this reef and associated cleaning stations for these species (Table 4). Notably, reef manta rays, smalleye stingrays, and oceanic manta rays were found to be primary elasmobranch users of the studied cleaning stations, engaging in mutualistic interactions with cleaner fish and spending significant amounts of time at the studied reef, whereas other species such as spotted eagle rays and blacktip sharks were solely observed cruising over the reef without engaging in cleaning interactions. Understanding the natural behaviours and habitat use of these elusive species can inform targeted conservation strategies, helping to delineate priority areas for protection. The data gathered for this contributes to filling knowledge gaps about some of the rarest and most threatened marine megafauna species in Mozambique, offering valuable insights to inform future conservation strategies and management plans for these species and their habitats.
The use of long-life remote underwater video cameras marks a significant advancement in marine research. These cameras enable continuous, long-term monitoring of marine environments, capturing diurnal and seasonal variations and providing uninterrupted video footage over extended periods. This capability increases the likelihood of encountering rare and elusive species and minimises disturbance to natural behaviours, thereby enhancing the quality and reliability of the data collected. Integrating machine learning and artificial intelligence with RUV technology further streamlines data processing and analysis, making comprehensive, long-term monitoring more feasible and effective. The successful application of the OOCAM in this study demonstrates the potential of long-life remote underwater video cameras as powerful tools for advancing our understanding of elasmobranch occurrence and behaviour, thereby informing conservation efforts and marine management.
The original contributions presented in the study are included in the article/Supplementary Material. Further inquiries can be directed to the corresponding author.
The animal study was approved by Museu de História Natural de Moçambique. The study was conducted in accordance with the local legislation and institutional requirements.
JB: Conceptualization, Data curation, Formal analysis, Funding acquisition, Methodology, Writing – original draft, Writing – review & editing. KR: Conceptualization, Funding acquisition, Resources, Writing – review & editing. JD: Supervision, Writing – review & editing. AD: Supervision, Writing – review & editing. JK: Conceptualization, Data curation, Funding acquisition, Methodology, Supervision, Writing – review & editing.
The author(s) declare financial support was received for the research, authorship, and/or publication of this article. Funding for the research was provided by the Rufford Foundation (grant number 32205-1 and 37616-2), the All Out Africa Foundation and the University of Gibraltar. The funders were not involved in the study design, collection, analysis, interpretation of data, the writing of this article or the decision to submit it for publication.
Fieldwork was conducted with the assistance of Tofo Scuba dive centre. The All Out Africa Foundation and the University of Gibraltar provided support for analysis and write-up. Special thanks to Steven Scagnelli for his expertise in camera repairs, Rene Cote for donating one of the LL-RUV cameras, and Ross Newbigging for lending his expertise in shark and ray species identification and for helping to create a list of elasmobranch species previously recorded in this area. We also acknowledge the All Out Africa volunteers for their support in reviewing camera footage. The authors are grateful to the reviewers for their valuable feedback, which greatly improved the manuscript.
The authors declare that the research was conducted in the absence of any commercial or financial relationships that could be construed as a potential conflict of interest.
The author(s) declare that no Generative AI was used in the creation of this manuscript.
All claims expressed in this article are solely those of the authors and do not necessarily represent those of their affiliated organizations, or those of the publisher, the editors and the reviewers. Any product that may be evaluated in this article, or claim that may be made by its manufacturer, is not guaranteed or endorsed by the publisher.
The Supplementary Material for this article can be found online at: https://www.frontiersin.org/articles/10.3389/fmars.2024.1518710/full#supplementary-material
Auster P. J., Giacalone L. (2021). Virtual reality camera technology facilitates sampling of interactions between reef piscivores and prey. Mar. Technol. Soc. J. 55, 54–63. doi: 10.4031/MTSJ.55.2.1
Barr Y., Abelson A. (2019). Feeding - cleaning trade-off: Manta ray “Decision-Making” as a conservation tool. Front. Mar. Sci. 6. doi: 10.3389/fmars.2019.00088
Barría C., Coll M., Navarro J. (2015). Unravelling the ecological role and trophic relationships of uncommon and threatened elasmobranchs in the western Mediterranean Sea. Mar. Ecol. Prog. Ser. 539, 225–240. doi: 10.3354/meps11494
Becerril-García E., Gutiérrez-Ortiz M., Preciado-González P., Ayala-Bocos A. (2020). The presence of Remora remora on Mobula birostris in the largest Marine Protected Area of North America. Mar. Freshw. Res. 71, 414–417. doi: 10.13140/RG.2.2.29198.79687
Becker A., Cowley P. D., Whitfield A. K. (2010). Use of remote underwater video to record littoral habitat use by fish within a temporarily closed South African estuary. J. Exp. Mar. Biol. Ecol. 391, 161–168. doi: 10.1016/j.jembe.2010.06.028
Berthe C., Lecchini D., Mourier J. (2016). Chafing behaviour on a patch of sandy bottom by ocellated eagle ray (Aetobatus ocellatus). Mar. Biodiversity 47, 379–380. doi: 10.1007/s12526-016-0463-8
Bilodeau S. M., Schwartz A. W. H., Xu B., Paúl Pauca V., Silman M. R. (2022). A low-cost, long-term underwater camera trap network coupled with deep residual learning image analysis. PloS One 17, e0263377. doi: 10.1371/journal.pone.0263377
Block B. A., Jonsen I. D., Jorgensen S. J., Winship A. J., Shaffer S. A., Bograd S. J., et al. (2011). Tracking apex marine predator movements in a dynamic ocean. Nature 475, 86–90. doi: 10.1038/nature10082
Bouchet P., Meeuwig J., Huveneers C., Langlois T., Letessier T., Lowry M., et al. (2018). “Marine sampling field manual for pelagic stereo-BRUVS (Baited Remote Underwater Videos),” in In Field Manuals for Marine Sampling to Monitor Australian Waters. Eds. Przeslawski R., Foster S. (Tasmania, Australia: National Environmental Science Programme (NESP), 105–132.
Brooks K., Rowat D., Pierce S. J., Jouannet D., Vely M. (2010). Seeing spots: photo-identification as a regional tool for whale shark identification. Western Indian Ocean J. Mar. Sci. 9, 185–194. doi: 10.1111/brv.12770
Caves E. M. (2021). The behavioural ecology of marine cleaning mutualisms. Biol. Rev. 96, 2584–2601. doi: 10.1111/brv.12770
Cheney K. L., Côté I. M. (2001). Are caribbean cleaning symbioses mutualistic? Costs and benefits of visiting cleaning stations to longfin damselfish. Anim. Behav. 62, 927–933. doi: 10.1006/anbe.2001.1832
Chin A., Pecl G. (2018). “Citizen science in shark and ray research and conservation: strengths, opportunities, considerations and pitfalls,” in Shark Research: emerging technologies and applications for the field and laboratory. Eds. Carrier J. C., Heithaus M. R., Simpfendorfer C. A. (CRC Press, Boca Raton, FL, USA), 299–317.
Clear Robotics Limited (2021). Open ocean camera, clear robotics limited. (Hong Kong: University of Hong Kong).
Cordier C. P., Ehlers Smith D. A., Ehlers Smith Y., Downs C. T. (2022). Camera trap research in Africa: A systematic review to show trends in wildlife monitoring and its value as a research tool. Global Ecol. Conserv. 40, e02326. doi: 10.1016/j.gecco.2022.e02326
Côté I. M. (2000). Evolution and ecology of cleaning symbioses in the sea. Oceanography Mar. Biology: Annu. Rev. 38, 311–355.
Couturier L. I. E., Marshall A. D., Jaine F. R. A., Kashiwagi T., Pierce S. J., Townsend K. A., et al. (2012). Biology, ecology and conservation of the Mobulidae. J. Fish Biol. 80 (5), 1075–1119. doi: 10.1111/j.1095-8649.2012.03264.x
Daly R., Venables S., Rogers T., Filmalter J., Hempson T., Murray T., et al. (2023). Persistent transboundary movements of threatened sharks highlight the importance of cooperative management for effective conservation. Mar. Ecol. Prog. Ser. 720, 117–131. doi: 10.3354/meps14413
Davidson L. N. K., Krawchuk M. A., Dulvy N. K. (2016). Why have global shark and ray landings declined: improved management or overfishing? Fish Fisheries 17, 438–458. doi: 10.1111/faf.12119
Deakos M. H., Baker J. D., Bejder L. (2011). Characteristics of a manta ray Manta alfredi population off Maui, Hawaii, and implications for management. Mar. Ecol. Prog. Ser. 429, 245–260. doi: 10.3354/meps09085
de Vincenzi G., Micarelli P., Viola S., Buffa G., Sciacca V., Maccarrone V., et al. (2021). ‘Biological Sound vs. Anthropogenic Noise: Assessment of Behavioural Changes in Scyliorhinus canicula Exposed to Boats Noise’, Animals 11 (1), 174. doi: 10.3390/ani11010174
Dickens L. C., Goatley C. H. R., Tanner J. K., Bellwood D. R. (2011). Quantifying relative diver effects in underwater visual censuses. PloS One 6, e18965. doi: 10.1371/journal.pone.0018965
Ditria E. M., Connolly R. M., Jinks E. L., Lopez-Marcano S. (2020). Automating the analysis of fish abundance using object detection: optimizing animal ecology with deep learning. Front. Mar. Sci. 7. doi: 10.3389/fmars.2020.00429
Ditria E. M., Lopez-Marcano S., Sievers M., Jinks E. L., Brown C. J., Connolly R. M. (2021). Annotated video footage for automated identification and counting of fish in unconstrained seagrass habitats. Front. Mar. Sci. 8. doi: 10.3389/fmars.2021.629485
Dulvy N. K., Fowler S. L., Musick J. A., Cavanagh R. D., Kyne P. M., Harrison L. R., et al. (2014). Extinction risk and conservation of the world’s sharks and rays. eLife 3. doi: 10.7554/eLife.00590
Dulvy N. K., Pacoureau N., Rigby C. L., Pollom R. A., Jabado R. W., Ebert D. A., et al. (2021). Overfishing drives over one-third of all sharks and rays toward a global extinction crisis. Curr. Biol. 31, 4773–4787.e8. doi: 10.1016/j.cub.2021.08.062
Edgar G. J., Barrett N. S., Morton A. J. (2004). Biases associated with the use of underwater visual census techniques to quantify the density and size-structure of fish populations. J. Exp. Mar. Biol. Ecol. 308, 269–290. doi: 10.1016/j.jembe.2004.03.004
Erickson K. R., Bugnot A. B., Figueira W. F. (2023). Optimising sampling of fish assemblages on intertidal reefs using remote underwater video. PeerJ 11, e15426. doi: 10.7717/peerj.15426
Ferretti F., Worm B., Britten G. L., Heithaus M. R., Lotze H. K. (2010). Patterns and ecosystem consequences of shark declines in the ocean. Ecol. Lett. 13, 1055–1071. doi: 10.1111/j.1461-0248.2010.01489.x
Figueroa-Pico J., Carpio A. J., Tortosa F. S. (2020). Turbidity: A key factor in the estimation of fish species richness and abundance in the rocky reefs of Ecuador. Ecol. Indic. 111, 106021. doi: 10.1016/j.ecolind.2019.106021
FishID (2024). FishID - AI solutions for environmental monitoring. Available online at: https://fishid.org/what-is-fishid (Accessed 1 October 2024).
Germanov E. S., Marshall A. D., Hendrawan I. G., Admiraal R., Rohner C. A., Argeswara J., et al. (2019). Microplastics on the menu: plastics pollute Indonesian manta ray and whale shark feeding grounds. Front. Mar. Sci. 6. doi: 10.3389/fmars.2019.00679
Goetze J. S., Bond T., McLean D. L., Saunders B. J., Langlois T. J., Lindfield S., et al. (2019). A field and video analysis guide for diver operated stereo-video. Methods Ecol. Evol. 10, 1083–1090. doi: 10.1111/2041-210X.13189
Grutter A. (1996). Parasite removal rates by the cleaner wrasse Labroides dimidiatus. Mar. Ecol. Prog. Ser. 130, 61–70. doi: 10.3354/meps130061
Grutter A. S. (1997). Spatiotemporal variation and feeding selectivity in the diet of the cleaner fish labroides dimidiatus. Copeia. (2) 346–355. doi: 10.2307/1447754
Grutter A. S., Murphy J. M., Choat J. H. (2003). Cleaner fish drives local fish diversity on coral reefs. Curr. Biol. 13, 64–67. doi: 10.1016/S0960-9822(02)01393-3
Guillaume M. M. M., Séret B. (2021). Observations of sharks (Elasmobranchii) at Europa Island, a remote marine protected area important for shark conservation in the southern Mozambique Channel. PloS One 16, e0253867. doi: 10.1371/journal.pone.0253867
Guttridge T., Gruber S., DiBattista J., Feldheim K., Croft D., Krause S., et al. (2011). Assortative interactions and leadership in a free-ranging population of juvenile lemon shark Negaprion brevirostris. Mar. Ecol. Prog. Ser. 423, 235–245. doi: 10.3354/meps08929
Heithaus M., Frid A., Vaudo J., Worm B., Wirsing A. (2010). Unravelling the ecological importance of elasmobranchs. In Sharks and Their Relatives II: Biodiversity, adaptive physiology, and conservation. Carrier J. C., Musick J. A., Heithaus M. R. Eds. (CRC Press), 611–637. doi: 10.1201/9781420080483-c16
Hemery L. G., Mackereth K. F., Gunn C. M., Pablo E. B. (2022). Use of a 360-degree underwater camera to characterize artificial reef and fish aggregating effects around marine energy devices. J. Mar. Sci. Eng. 10, 555. doi: 10.3390/jmse10050555
Heupel M., Carlson J., Simpfendorfer C. (2007). Shark nursery areas: concepts, definition, characterization and assumptions. Mar. Ecol. Prog. Ser. 337, 287–297. doi: 10.3354/meps337287
Heupel M., Knip D., Simpfendorfer C., Dulvy N. (2014). Sizing up the ecological role of sharks as predators. Mar. Ecol. Prog. Ser. 495, 291–298. doi: 10.3354/meps10597
IUCN SSC Shark Specialist Group (2023). Southern Inhambane Province ISRA Factsheet (Gland, Switzerland: IUCN SSC Shark Specialist Group).
Jacoby D. M. P., Croft D. P., Sims D. W. (2012). Social behaviour in sharks and rays: analysis, patterns and implications for conservation. Fish Fisheries 13, 399–417. doi: 10.1111/j.1467-2979.2011.00436.x
Jaine F. R. A., Couturier L. I. E., Weeks S. J., Townsend K. A., Bennett M. B., Fiora K., et al. (2012). When Giants Turn Up: Sighting Trends, Environmental Influences and Habitat Use of the Manta Ray Manta alfredi at a Coral Reef. PloS One 7, e46170. doi: 10.1371/journal.pone.0046170
Jones R. E., Griffin R. A., Herbert R. J. H., Unsworth R. K. F. (2021). Consistency is critical for the effective use of baited remote video. Oceans 2, 215–232. doi: 10.3390/oceans2010013
Keeping J., Milligan R. J., Reeve-Arnold K., Bailey D. (2021). Trends in sightings of the stingrays of southern Mozambique. Mar. Freshw. Res. 72, 1824–1837. doi: 10.1071/MF21013
Kitchen-Wheeler A. (2013). The behaviour and ecology of Alfred mantas (Manta alfredi) in the Maldives (Newcastle, United Kingdom: Newcastle University).
Klimley A. (1983). “Social organization of schools of the Scalloped Hammerhead shark, Sphyrna lewini (Griffith and Smith),” in the Gulf of California. (San Diego, United States: University of California).
Klimley A. P., Porcher I. F., Clua E. E. G., Pratt H. L. (2023). A review of the behaviours of the Chondrichthyes: a multi-species ethogram for the chimaeras, sharks, and rays. Behaviour 160, 967–1080. doi: 10.1163/1568539X-bja10214
Kosmala M., Wiggins A., Swanson A., Simmons B. (2016). Assessing data quality in citizen science. Front. Ecol. Environ. 14, 551–560. doi: 10.1002/fee.1436
Lee C.-L., Huang Y.-H., Chen C.-H., Lin H.-J. (2016). Remote underwater video reveals grazing preferences and drift export in multispecies seagrass beds. J. Exp. Mar. Biol. Ecol. 476, 1–7. doi: 10.1016/j.jembe.2015.12.004
Liquid Dive Adventures (2024). Liquid dive adventures - dive sites. Available online at: https://www.liquiddiveadventures.com/dive-sites-tofo (Accessed 21 September 2024).
Losey G. S., Margules L. (1974). Cleaning symbiosis provides a positive reinforcer for fish. Science 184, 179–180. doi: 10.1126/science.184.4133.179
Magneville C., Leréec Le Bricquir M., Dailianis T., Skouradakis G., Claverie T., Villéger S. (2023). Long-duration remote underwater videos reveal that grazing by fishes is highly variable through time and dominated by non-indigenous species. Remote Sens. Ecol. Conserv. 9, 311–322. doi: 10.1002/rse2.311
Magson K., Monacella E., Scott C., Buffat N., Arunrugstichai S., Chuangcharoendee M., et al. (2022). Citizen science reveals the population structure and seasonal presence of whale sharks in the Gulf of Thailand. J. Fish Biol. 101, 540–549. doi: 10.1111/jfb.15121
Marrable D., Barker K., Tippaya S., Wyatt M., Bainbridge S., Stowar M., et al. (2022). Accelerating species recognition and labelling of fish from underwater video with machine-assisted deep learning. Front. Mar. Sci. 9. doi: 10.3389/fmars.2022.944582
Marshall A. (2008). Biology and population ecology of manta birostris in southern Mozambique. (Brisbane, Australia: The University of Queensland).
Marshall A. D., Bennett M. B. (2010). Reproductive ecology of the reef manta ray Manta alfredi in southern Mozambique. J. Fish Biol. 77, 169–190. doi: 10.1111/j.1095-8649.2010.02669.x
Marshall A. D., Dudgeon C. L., Bennett M. B. (2011). Size and structure of a photographically identified population of manta rays Manta alfredi in southern Mozambique. Mar. Biol. 158, 1111–1124. doi: 10.1007/s00227-011-1634-6
Marshall A. D., Flam A. L., Cullain N., Carpenter M., Conradie J., Venables S. K. (2023). Southward range extension and transboundary movements of reef manta rays Mobula alfredi along the east African coastline. J. Fish Biol. 102, 628–634. doi: 10.1111/jfb.15290
McCauley D. J., Pinsky M. L., Palumbi S. R., Estes J. A., Joyce F. H., Warner R. R. (2015). Marine defaunation: Animal loss in the global ocean. Science 347. doi: 10.1126/science.1255641
Meekan M. G., Trevitt L., Simpfendorfer C. A., White W. (2016). The piggybacking stingray. Coral Reefs 35, 1011–1011. doi: 10.1007/s00338-016-1429-9
Micarelli P., Reinero F. R., D’Agnese R., Pacifico A., Giglio G., Sperone E. (2023). Evidence of non-random social interactions between pairs of bait-attracted white sharks in gansbaai (South Africa). Diversity 15, 433. doi: 10.3390/d15030433
Michael S. (1993). Reef sharks and rays of the world. A guide to their identification, behaviour, and ecology. J. Mar. Biol. Assoc. United Kingdom 73, 987–987. doi: 10.1017/S0025315400034998
Mickle M. F., Higgs D. M. (2022). ‘Towards a new understanding of elasmobranch hearing’, Marine Biology 169 (1), 12. doi: 10.1007/s00227-021-03996-8
Murie C. J., Marshall A. D. (2016). Mobula kuhlii cleaning station identified at an inshore reef in southern Mozambique. PeerJ (Preprint). doi: 10.7287/peerj.preprints.1724v1
Musick J., Burgess G., Cailliet G., Camhi M., Fordham S. (2020). Management of sharks and their relatives (Elasmobranchii). Fisheries 25 (3), 9–13. doi: 10.1577/1548-8446(2000)025<0009:MOSATR>2.0.CO;2
Myers R. A., Worm B. (2003). Rapid worldwide depletion of predatory fish communities. Nature 423, 280–283. doi: 10.1038/nature01610
Nauticam (2023). Nauticam Vacuum Check And Leak Detection System (Fort Lauderdale, Florida, United States of America: nauticam).
Nicholson-Jack A. E., Harris J. L., Ballard K., Turner K. M. E., Stevens G. M. W. (2021). A hitchhiker guide to manta rays: Patterns of association between Mobula alfredi, M. birostris, their symbionts, and other fishes in the Maldives. PloS One 16. doi: 10.1371/journal.pone.0253704
Norman B. M., Holmberg J. A., Arzoumanian Z., Reynolds S. D., Wilson R. P., Rob D., et al. (2017). Undersea constellations: the global biology of an endangered marine megavertebrate further informed through citizen science. BioScience 67, 1029–1043. doi: 10.1093/biosci/bix127
O’Connor B., Cullain N. (2021). Distribution and community structure of at-risk and Data Deficient elasmobranchs in Zavora Bay, Mozambique. Afr. J. Mar. Sci. 43, 521–532. doi: 10.2989/1814232X.2021.1997814
O’Malley M., Townsend K. A., Hilton P., Heinrichs S., Stewart J. D. (2017). Characterization of the trade in manta and devil ray gill plates in China and South-east Asia through trader surveys. Aquat. Conservation: Mar. Freshw. Ecosyst. 27, 394–413. doi: 10.1002/aqc.2670
Oliver S. P., Hussey N. E., Turner J. R., Beckett A. J. (2011). Oceanic sharks clean at coastal seamount. PloS One 6, e14755. doi: 10.1371/journal.pone.0014755
Pate J. H., Macdonald C., Wester J. (2021). Surveys of recreational anglers reveal knowledge gaps and positive attitudes towards manta ray conservation in Florida. Aquat. Conservation: Mar. Freshw. Ecosyst. 31, 1410–1419. doi: 10.1002/aqc.3508
Peel L. R., Stevens G. M. W., Daly R., Keating Daly C. A., Collin S. P., Nogués J., et al. (2020). Regional movements of reef manta rays (Mobula alfredi) in Seychelles waters. Front. Mar. Sci. 7. doi: 10.3389/fmars.2020.00558
Pereira M. (2021). “Marine & Coastal areas under protection: Mozambique,” in Western Indian Ocean Marine Protected Areas Outlook: Towards achievement of the Global Biodiversity Framework Targets (United Nations Environment Programme (UNEP), Western Indian Ocean Marine Science Association (WIOMSA, Nairobi, Kenya), 119–132.
Pratt H. L., Carrier J. C. (2001). A review of elasmobranch reproductive behaviour with a case study on the nurse shark, ginglymostoma cirratum. Environ. Biol. Fishes 60, 157–188. doi: 10.1023/A:1007656126281
Prat-Varela A., Torres A., Cervantes D., Aquino-Baleytó M., Abril A.-M., Clua E. E. G. (2023). Improved baited remote underwater video (BRUV) for 24 h real-time monitoring of pelagic and demersal marine species from the epipelagic zone. J. Mar. Sci. Eng. 11, 1182. doi: 10.3390/jmse11061182
Ritter E. K., Amin R. W. (2016). Mouth Cleaning of Lemon Sharks, Negaprion brevirostris, by Sharksuckers, Echeneis naucrates. Copeia 104, 728–733. doi: 10.1643/CE-16-431
Roff G., Doropoulos C., Rogers A., Bozec Y.-M., Krueck N. C., Aurellado E., et al. (2016). The ecological role of sharks on coral reefs. Trends Ecol. Evol. 31, 395–407. doi: 10.1016/j.tree.2016.02.014
Rohner C. A., Pierce S. J., Marshall A. D., Weeks S. J., Bennett M. B., Richardson A. J. (2013). Trends in sightings and environmental influences on a coastal aggregation of manta rays and whale sharks. Mar. Ecol. Prog. Ser. 482, 153–168. doi: 10.3354/meps10290
Rohner C. A., Richardson A. J., Jaine F. R. A., Bennett M. B., Weeks S. J., Cliff G., et al. (2018). Satellite tagging highlights the importance of productive Mozambican coastal waters to the ecology and conservation of whale sharks. PeerJ 6, e4161. doi: 10.7717/peerj.4161
Sazima I., Moura R. (2000). Shark (Carcharhinus perezi), Cleaned by the Goby (Elacatinus randalli), at Fernando de Noronha Archipelago, Western South Atlantic. Copeia 1, 297–299. doi: 10.1643/0045-8511(2000)2000[0297:SCPCBT]2.0.CO;2
Shipley O. N., Matich P., Hussey N. E., Brooks A. M. L., Chapman D., Frisk M. G., et al. (2023). Energetic connectivity of diverse elasmobranch populations – implications for ecological resilience. Proc. R. Soc. B: Biol. Sci. 290. doi: 10.1098/rspb.2023.0262
Smith K., Scarpaci C., Louden B., Otway N. (2015). Behaviour of aggregated grey nurse sharks Carcharias taurus off eastern Australia: similarities and differences among life-history stages and sites. Endangered Species Res. 27, 69–85. doi: 10.3354/esr00652
Speed C., Meekan M., Field I., McMahon C., Stevens J., McGregor F., et al. (2011). Spatial and temporal movement patterns of a multi-species coastal reef shark aggregation. Mar. Ecol. Prog. Ser. 429, 261–275. doi: 10.3354/meps09080
Stevens J. (2000). The effects of fishing on sharks, rays, and chimaeras (chondrichthyans), and the implications for marine ecosystems. ICES J. Mar. Sci. 57, 476–494. doi: 10.1006/jmsc.2000.0724
Stevens G. (2016). Conservation and population ecology of manta rays in the Maldives. York, England: University of York.
Tibiriçá Y., Birtles A., Valentine P., Miller D. K. (2011). Diving tourism in Mozambique: an opportunity at risk? Tourism Mar. Environments 7, 141–151. doi: 10.3727/154427311X13195453162732
Vaughan D. (2018). Cleaner shrimp as biocontrols in aquaculture. Townsville City, Australia: James Cook University.
Venables S. K., Conradie J., Marshall A. D. (2022). First records of the ornate eagle ray Aetomylaeus vespertilio from the Inhambane Province, Mozambique. J. Mar. Biol. Assoc. United Kingdom 101, 1085–1088. doi: 10.1017/S0025315422000054
Venables S. K., van Duinkerken D. I., Rohner C. A., Marshall A. D. (2020). Habitat use and movement patterns of reef manta rays Mobula alfredi in southern Mozambique. Mar. Ecol. Prog. Ser. 634, 99–114. doi: 10.3354/meps13178
Venables S. K., Rohner C. A., Flam A. L., Pierce S. J., Marshall A. D. (2024). Persistent declines in sightings of manta and devil rays (Mobulidae) at a global hotspot in southern Mozambique. Environ. Biol. Fishes. doi: 10.1007/s10641-024-01576-5
Whitmarsh S. K., Fairweather P. G., Huveneers C. (2017). What is Big BRUVver up to? Methods and uses of baited underwater video. Rev. Fish Biol. Fisheries 27, 53–73. doi: 10.1007/s11160-016-9450-1
Worm B., Davis B., Kettemer L., Ward-Paige C. A., Chapman D., Heithaus M. R., et al. (2013). Global catches, exploitation rates, and rebuilding options for sharks. Mar. Policy 40, 194–204. doi: 10.1016/j.marpol.2012.12.034
Youngbluth M. J. (1968). Aspects of the ecology and ethology of the cleaning fish, labroides phthirophagus randall. Z. für Tierpsychologie 25, 915–932. doi: 10.1111/j.1439-0310.1968.tb00052.x
Keywords: long-life remote underwater video, RUV, elasmobranchs, Mozambique, cleaning station, hitchhiker, citizen science
Citation: Buschmann J, Roques KG, Davies JS, Dissanayake A and Keeping JA (2024) Novel approach to studying marine fauna: using long-life remote underwater video cameras to assess occurrence and behaviour of threatened and data-deficient elasmobranch species in southern Mozambique. Front. Mar. Sci. 11:1518710. doi: 10.3389/fmars.2024.1518710
Received: 28 October 2024; Accepted: 29 November 2024;
Published: 23 December 2024.
Edited by:
Xuelei Zhang, Ministry of Natural Resources, ChinaCopyright © 2024 Buschmann, Roques, Davies, Dissanayake and Keeping. This is an open-access article distributed under the terms of the Creative Commons Attribution License (CC BY). The use, distribution or reproduction in other forums is permitted, provided the original author(s) and the copyright owner(s) are credited and that the original publication in this journal is cited, in accordance with accepted academic practice. No use, distribution or reproduction is permitted which does not comply with these terms.
*Correspondence: Jule Buschmann, anVsZUBhbGxvdXRhZnJpY2Eub3Jn
Disclaimer: All claims expressed in this article are solely those of the authors and do not necessarily represent those of their affiliated organizations, or those of the publisher, the editors and the reviewers. Any product that may be evaluated in this article or claim that may be made by its manufacturer is not guaranteed or endorsed by the publisher.
Research integrity at Frontiers
Learn more about the work of our research integrity team to safeguard the quality of each article we publish.