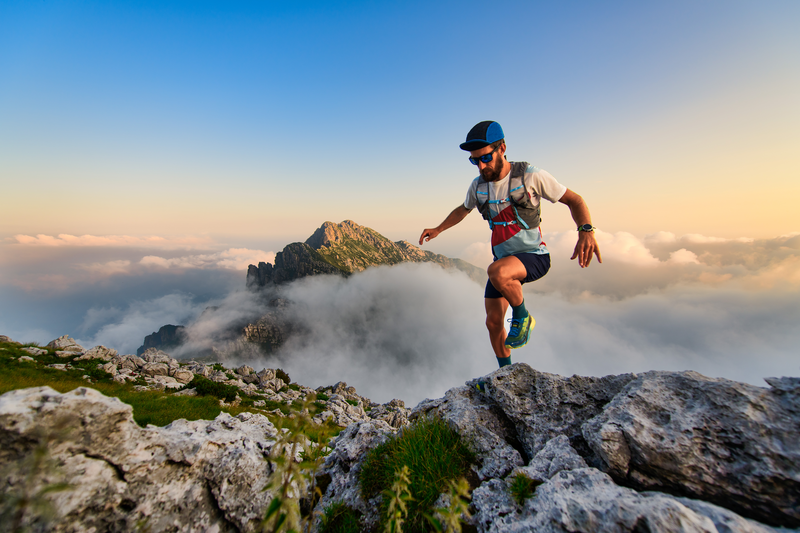
95% of researchers rate our articles as excellent or good
Learn more about the work of our research integrity team to safeguard the quality of each article we publish.
Find out more
ORIGINAL RESEARCH article
Front. Mar. Sci. , 08 January 2025
Sec. Marine Affairs and Policy
Volume 11 - 2024 | https://doi.org/10.3389/fmars.2024.1518235
This article is part of the Research Topic Challenges and Solutions in Forecasting and Decision-Making in Marine Economy and Management View all 22 articles
Introduction: The sustainable development of marine fisheries has been a major concern, with the carrying capacity of marine fishery resources becoming a focal point of research.
Methods: This study, utilizing remote sensing data, marine capture fisheries catch data, and fishing effort data from 2013 to 2020, aims to determine the maximum sustainable yield using a surplus production model and provide a comprehensive assessment of the status and potential of China’s marine fishery resources.
Results: The results indicate that China’s marine fishery resources exhibit significant regional variability, with the East China Sea contributing the largest share of catch and maintaining sustainability, while regions such as the South China Sea, Yellow Sea, and Bohai Sea have exceeded their ecological carrying capacities. Correlation analysis highlights that nutrient levels and water quality (e.g., chemical oxygen demand) are critical for resource stability, while the distribution and management of protected areas further influence carrying capacity.
Discussion: This study contributes to the development of more effective fishery policies, aiming to balance economic benefits with ecological health. By understanding these dynamics, policymakers can better address the challenges facing sustainable marine fisheries.
Sustainable management of marine fisheries is essential for maintaining ecological balance and supporting human livelihoods, particularly in regions where fishing plays a significant economic role (Pauly et al., 1998; FAO, 2020; Kemp et al., 2023). China’s coastal and offshore waters are among the most productive globally, supporting a rapidly expanding fishing industry that has developed significantly over the past few decades (Shen and Heino, 2014). However, increasing demand for fish products and the growing capacity of fishing fleets have placed unprecedented pressure on Chinese marine fisheries, raising concerns about resource depletion, ecosystem degradation, and the sustainability of fishery resources (Han, 2018; Tang et al., 2016; Szuwalski et al., 2017; Chen et al., 2018; Yin et al., 2024). In response, marine resource management practices in China have made some advances, including improved regulations and monitoring systems (Shen and Heino, 2014; Chen et al., 2023; Yue et al., 2023), but the challenges of sustainable fishery resource management, particularly in terms of accurately assessing and managing the carrying capacity of marine ecosystems, remain substantial.
Carrying capacity, in the context of marine ecosystems, refers to the maximum population of fish that an ecosystem can support without undergoing degradation (Odum, 1997; Wang et al., 2022). Estimating this capacity is essential for preventing overexploitation and ensuring the sustainability of fishery resources. A common approach to assess carrying capacity is through the use of surplus production models (SPMs), which estimate the maximum sustainable yield (MSY) —the highest catch that can be maintained over time without depleting the fishery (Hilborn and Walters, 1992). The MSY serves as a critical benchmark in fishery management, offering insights into the capacity of marine resources to support fishing activities. By providing a quantitative estimate of the MSY, SPMs allow researchers and policymakers to evaluate the sustainability of current fishing practices and help develop management strategies to maintain fish stocks within sustainable limits (Jacobson et al., 2002; Kokkalis et al., 2024). However, most studies using SPMs and MSY focus on single species or specific regions, often neglecting the broader, more complex interactions within entire marine ecosystems (Wang and Liu, 2013; Stäbler et al., 2016; Derhy et al., 2024; Sultana et al., 2023).
Recent studies have called for a more integrated approach that applies MSY not only at the species level but also across larger geographic scales, incorporating ecosystem dynamics to better understand the carrying capacity of marine fisheries on a broader level (Fulton et al., 2022; Sun et al., 2020; Demirel et al., 2020; Griffiths et al., 2024). Comprehensive assessments of fishery resource carrying capacity across entire marine regions remain scarce, particularly for large, productive areas such as China’s waters, where systematic evaluations are needed to inform sustainable management strategies.
This study applies the surplus production model as a key tool to estimate the MSY for marine fishery resources in Chinese waters. Using extensive data collected from statistical yearbooks, we analyze marine fishery catch trends over the past eight years. The estimated MSY is then employed to construct a carrying capacity evaluation model that assesses the current status of China’s marine fisheries, followed by an analysis of the factors influencing this status. The goal of this research is to provide a scientific basis for managing marine fishery resources in China, as well as to offer insights that may benefit global fisheries management.
The data used in this study primarily includes marine fishery catch data, fishing effort, environmental factors, aquaculture distribution data and protected area information from 2013 to 2020. The main sources of data are the China Fishery Statistical Yearbook, Global Fishing Watch (GFW), China Marine Ecological Early Warning Monitoring Surveys, and official reports from provincial government websites (Table 1).
Catch data used in this study were sourced from the China Fishery Statistical Yearbooks (Bureau of Fisheries of Ministry of Agriculture and Rural Affairs of China, 2014– 2021). The data are collected through standardized government surveys and compiled by provincial and national fishery bureaus. Although the accuracy of catch statistics available from these Yearbooks has been questioned in the past, with suggestions of over-reporting domestic fisheries production, it is also acknowledged that “a more effective statistical system for domestic fisheries has been developed” (Pauly et al., 2014). Indeed, there have been three major adjustments in the China Fishery Statistical Yearbooks throughout this period of years to reduce past inaccuracy and uncertainty in reported catches, accompanied by timely updating of China’s fisheries data based on these adjustments in the Food and Agriculture Organization of the United Nation’s (FAO) global capture production database. Detailed revision information about China’s fisheries data has been described by its Fisheries Bureau of the Ministry of Agriculture and Rural Affairs (1999).
The fishing effort data is represented by the total annual fishing hours of Chinese vessels. This data was derived by filtering the daily fishing vessel operation time provided by GFW to include only vessels registered in China (Global Fishing Watch, 2021). The Global Fishing Watch dataset is a globally recognized platform that utilizes Automatic Identification System (AIS) signals from fishing vessels, combined with advanced algorithms, to monitor fishing activities worldwide. This dataset provides detailed spatial resolution (10th-degree grids) and temporal resolution (daily) of fishing effort, including vessel locations, activity intensity, and fishing types. GFW collaborates with international organizations such as the Food and Agriculture Organization (FAO) and regional fishery management bodies, ensuring alignment with global fisheries management standards. Its data have been widely cited in peer-reviewed publications and used for policy-making in fisheries management.
Environmental monitoring data was collected from the 2013–2020 China Marine Ecological Early Warning Monitoring Surveys. China Marine Ecological Early Warning Monitoring Surveys provide comprehensive data on marine ecological changes, focusing on three aspects: trend monitoring of large-scale, long-term changes; thematic monitoring of specific regions or ecological issues; and research-oriented monitoring supporting restoration and disaster management. Key indicators include seawater quality (e.g., nutrients, pH), hydro-meteorological factors (e.g., temperature, salinity), and biological metrics (e.g., biodiversity, habitat conditions). This study used the data of sea surface temperature (SST), pH, dissolved inorganic nitrogen (DIN), phosphate (PO43-), dissolved oxygen (DO), chemical oxygen demand (COD), and chlorophyll-a (chl a) concentrations from 2,041 stations. The monitoring framework is guided by annual work plans and coordinated by the Ministry of Natural Resources, with coastal provinces and marine research institutions responsible for conducting the surveys. To ensure data consistency and reliability, all monitoring activities adhere to national protocols, such as the Specifications for Oceanographic Survey—Part 4: Survey of Chemical Parameters in Seawater (GB/T 12763.4-2007) and the Specification for Marine Monitoring—Part 4: Seawater Analysis (GB 17378.4-2007). A rigorous quality control system is in place, including regular training and multi-level review processes. After completing three levels of review—local, regional, and national—the data are submitted to the national data management agency for final validation and approval before being authorized for use.
Aquaculture distribution data used in this study were obtained from sea area ownership records. These records include detailed information on the spatial distribution of aquaculture activities, licensing, and usage rights for designated marine areas. The data collection process adheres to national standards and guidelines to ensure consistency and reliability, forming a robust basis for assessing aquaculture’s role in marine resource management.
Data on marine protected areas (MPAs) were obtained from official provincial reports, which document MPA designations, sizes, and management measures. Provincial natural resource authorities organize the designation and delineation of protected areas based on unified rules set by the National Forestry and Grassland Administration. Following expert evaluation and approval, the finalized information is submitted to the National Forestry and Grassland Administration for record-keeping. These records were used to assess spatial coverage and protection impacts.
The surplus production model of Schaefer (1954) was applied in this study to the per annum total catch in tons and standardized number of fishing units. This model considered the stock as one big unit of biomass.
The Schaefer model expresses the relationship between catch yield (Y) and fishing effort (E) using a quadratic function:
Where:
Y: the catch yield.
E: the fishing effort.
a and b are model parameters that are determined by fitting the data.
Using the Least Squares Method to estimate the optimal values for a and b, which minimizes the sum of squared errors between the predicted and actual values. Once the parameters a and b are determined, MSY and the corresponding fishing effort (Emsγ) can be calculated.
The fishing effort at Emsγ occurs when the yield is maximized. This can be found by taking the derivative of the Schaefer model with respect to E and setting it to zero:
MSY is then obtained by substituting Emsγ back into the original equation:
The catch per unit effort (CPUE) was used to analyze fishery productivity, and together with MSY and Emsγ, it provides a comprehensive understanding of the relationship between fishing effort, catch, and stock biomass.
The carrying capacity of marine fishery resources is represented by the ratio of the annual CPUE to the catch per unit effort corresponding to MSY. The formula is as follows:
Where:
B: carrying capacity of marine fishery resources.
According to the evaluation results of marine fishery carrying capacity B, a classification assessment is conducted based on Table 2, following the principle of maintaining stability by reasonably controlling offshore fishing intensity.
Since temperature and nutrients are influenced by both human activities and natural processes, “environmental” and “anthropogenic” factors were distinguished in this study to distinguish between direct and indirect anthropogenic stressors. For environmental factors, SST, pH, cholorophyII a, DIN, PO43-, DO and COD were included, which covered a range of physical and chemical indicators, as well as the pollution indicators that are important in a marine environment. For human activities aspect, mariculture and establishment of marine protected areas were selected.
To assess the relationship between fishery resource status and environmental factors, we employed the grey correlation analysis (Tosun, 2006). The fishery resource status was represented by CPUE, which provides a standardized measure of fishery resources accounting for fishing effort. Environmental factors, including sea surface temperature, salinity, dissolved oxygen, and nutrient levels, were used as independent variables. Data were log-transformed where necessary to ensure normality. The steps is :
First, the original data were normalized by the mean method.
where and xj(k) are normalized, and and Xj (k) are original.
Secondly, the correlation coefficient ϵ0i (k) was calculated as follows:
where , denotes the reference sesequence, and denotes the comparability sequence. is distinguishing or identification coefficient which is between 0 and 1 (taking 0.5). is the minimum values of .
Finally, the grey correlation degree was calculated as the average value of the grey correlation coefficient, which was defined as follows:
where is the grey relational grade which represents the level of correlation between the reference sequence and the comparability sequence. If a particular comparability sequence is more important than the other comparability sequences to the reference sequence, then the grey correlation degree for that comparability sequence and reference sequence will be higher than others.
China’s marine fishery catch exhibited a clear trend from 2013 to 2020, first increasing and then gradually decreasing (Figure 1). From 2013 to 2015, the catch yield grew steadily, reaching a peak of 13.14 million tons in 2015. And between 2015 and 2020, the catch volume declined steadily.
The marine fishery catch yield also exhibited considerable variability across different sea regions. Among the four major sea areas—namely the East China Sea, the South China Sea, the Bohai Sea, and the Yellow Sea—the East China Sea consistently contributed the largest share to the overall national catch. This region’s relatively higher productivity can be attributed to a combination of favorable environmental factors, such as nutrient-rich waters, and its geographic proximity to key fishing grounds. The South China Sea, while also significant, contributed slightly less, followed by the Bohai Sea and Yellow Sea, which produced comparatively smaller portions of the total catch volume. These differences reflect not only regional ecological conditions but also varying levels of fishing effort and resource management strategies across these zones. The annual variation in catch volume within these regions generally aligned with the national trends. For instance, the peak in 2015 and subsequent decline between 2015 and 2020 were observed across all four sea areas. This consistency suggests that the factors influencing marine fishery catch volume—such as overfishing, environmental changes, and possible resource depletion—were not isolated to one region but were instead part of broader, nationwide trends affecting China’s marine fisheries.
In terms of species composition, fish constituted the majority of the catch, representing approximately 68.48%, followed by crustaceans at 19.11% (Figure 2). The dominant fish species between 2013 and 2020 included Trichiurus, Engraulis, Caranx, Scomberomorus, Pampus, Nemipterus, Muraenesox, Larimichthys, Collichthys, Sparus, Thamnaconus and Sphyraenus, with Trichiurus and Engraulis being the most prevalent. Crustacean catches primarily consisted of Portunus, Scylla, Paramamosain, Acetes, Penaeus, Trachypenaeus, with Portunus and Acetes comprising the majority. Thus, Trichiurus, Engraulis, Portunus and Acetes emerged as the key species in China’s marine fishery, with Trichiurus being the most dominant. The remaining catch was comprised of mollusks, algae, and cephalopods, all of which, though less dominant, still played an important role in the overall marine ecosystem and fisheries economy.
The fishing effort in China’s offshore waters was assessed based on the duration of fishing operations by vessels registered in China. The results indicated a fluctuating trend in fishing effort between 2013 and 2020, with an initial increase followed by a decline (Figure 3). From 2013 to 2018, the fishing effort showed a gradual upward trend, peaking at 9,650 hours in 2018. A slight decrease was observed between 2019 and 2020, though the overall effort remained higher than pre-2018 levels. Comparing these results with catch data from 2015 to 2020 revealed that, despite no significant reduction in fishing effort, the total catch gradually declined. This may indicate that overfishing has led to a depletion of fishery resources.
Spatial analysis of fishing effort across the four major sea regions—Bohai Sea, Yellow Sea, East China Sea, and South China Sea—revealed significant regional variation. The Bohai Sea exhibited the lowest fishing effort, likely due to its smaller geographic area and possibly lower fishery productivity. The East China Sea, known for its historically high levels of fishing activity, showed a fluctuating downward trend in effort. The Yellow Sea and South China Sea exhibited a pattern of rising fishing effort followed by a decrease, mirroring the national trend. These regions may have benefited from the initial expansion in fishing capacity but are now experiencing the consequences of sustained pressure on fishery resources. The synchronization of these trends with the national pattern suggests that broader economic and regulatory factors, such as fishing subsidies, technological advancements, or nationwide policy interventions, may be influencing fishing behavior across regions.
MSY and Emsγ values were computed for each study region using the Schaefer surplus production model. Table 3 presents a detailed summary of the computed values for each region, showcasing the potential yield of marine fishery resources under optimal fishing efforts.
The results indicate that the overall MSY for Chinese marine fishery resources was 12,165,299.60 tons, achieved at an effort of 6,328.88 hours. Upon further analysis, it was observed that the MSY and Emsγ values differed significantly across the four major seas of China, reflecting the variability in marine productivity and fishing pressure in each region. In the Bohai Sea, the MSY was calculated at 1,017,054.40 tons, with a corresponding Emsγ of 603.89 hours. This relatively smaller effort suggests that the Bohai Sea has a lower productivity compared to other regions, likely due to its limited water exchange and greater influence of anthropogenic activities. For the Yellow Sea, the MSY was 3,038,229.16 tons, with an Emsγ of 2,968.01 hours. This sea, with a larger surface area and significant water exchange compared to the Bohai Sea, can sustain higher fishing efforts, reflecting a relatively higher productivity. In the East China Sea, the MSY reached 5,389,914.82 tons, at an Emsγ of 2,422.17 hours. The East China Sea, benefiting from both the Kuroshio current and continental runoff, presents high biological productivity, contributing to its high MSY. However, the increasing fishing intensity in this region requires careful management to avoid overexploitation. Lastly, the MSY in the South China Sea was 4,407,318.90 tons, with a corresponding Emsγ of 2,841.84 hours. This region, characterized by its deep waters and diverse marine ecosystems, holds considerable fishery potential, yet its relatively large fishing effort indicates a need for stricter controls to maintain sustainability.
Between 2013 and 2016, the overall fishery resources in China’s seas remained within sustainable limits, as indicated by CPUE/(MSY/Emsγ) ratios consistently above 1, which suggest that despite total catch volumes approaching or slightly exceeding MSY, the ecosystems’ carrying capacities were largely intact. However, beginning in 2017, a marked decline in the CPUE/(MSY/Emsγ) ratio was observed, dropping below 1, indicating a shift toward overexploitation. By 2018, the ratio had fallen to unsustainable levels, further corroborating the negative impact of increased fishing pressure on the ecosystems’ ability to regenerate (Table 4).
In the Bohai Sea, the CPUE/(MSY/Emsγ) ratio was 0.90 in 2013, reflecting a state of critical overexploitation, where the fishing effort slightly exceeded the ecosystem’s sustainable limits. However, significant improvements were observed in 2014 and 2015, with CPUE/(MSY/Emsγ) ratios rising sharply to 2.29 and 5.66, respectively, while fishing time reduced significantly. These changes suggest that resource use was highly efficient during these years. Nevertheless, by 2018, the CPUE/(MSY/Emsγ) ratio had dramatically declined to 0.46, coupled with a fishing effort that surpassed sustainable thresholds. This indicates a return to overexploitation and significant stress on the Bohai Sea’s fishery resources (Table 5).
From 2013 to 2015, the Yellow Sea’s fishery remained within sustainable limits, with CPUE/(MSY/Emsγ) ratios consistently above 1. However, starting in 2016, the carrying capacity declined significantly, with the CPUE/(MSY/Emsγ) ratio dropping from 1.34 to 0.66 by 2017. This rapid decline suggests that increased fishing pressure during this period outpaced the region’s resource replenishment capacity. Despite a reduction in fishing effort in 2018, the CPUE saw only a modest recovery, indicating that the region’s fishery resources had not yet fully returned to sustainable levels (Table 6).
The East China Sea showed signs of overexploitation between 2013 and 2015, with CPUE/(MSY/Emsγ) ratios below 1. However, a notable recovery occurred in 2016, when the ratio surged to over 2.0, and fishing effort significantly decreased. This marked a transition toward more sustainable resource management, with the CPUE/(MSY/Emsγ) ratio remaining above 1 in the following years. By 2020, the ratio stood at 1.21, indicating that the East China Sea had largely recovered from its previous overexploitation and was operating within sustainable limits (Table 7).
Table 7. The assessment results of carrying capacity of marine fishing resources in the East China Sea.
From 2013 to 2017, the South China Sea maintained relatively high CPUE/(MSY/Emsγ) ratios, indicating that fishing activities remained sustainable. However, 2018 marked a drastic shift, with the CPUE/(MSY/Emsγ) ratio plummeting to 0.40, and fishing effort exceeding 173% of the Emsγ. This sharp decline reflects severe overexploitation, with fish stocks being depleted at unsustainable rates. Although fishing effort was slightly reduced in 2019 and 2020, the CPUE remained low, around 0.60, indicating that the South China Sea’s resources had not yet recovered from the prior overexploitation (Table 8).
Table 8. The assessment results of carrying capacity of marine fishing resources in the South China Sea.
The grey relational analysis results (Figure 4) reveal the degree of association between environmental factors and CPUE across the Bohai Sea, Yellow Sea, East China Sea, and South China Sea. The following findings illustrate the key factors influencing the fishery resource carrying capacity in each sea area.
In the Bohai Sea, most environmental factors exhibit moderate to high correlations with the CPUE, with values generally exceeding 0.6. The highest correlations are observed with PO43- (0.64), COD (0.64), and area of protected areas (0.63), suggesting that nutrient levels (PO43-) and COD, a proxy for water pollution, significantly impact fishery resource sustainability. Additionally, the influence of protected areas highlights the importance of marine spatial management in maintaining fishery resources in this region.
In the Yellow Sea, Chl a (0.73), COD (0.72), DIN (0.70), and area of protected areas (0.75) exhibit the strongest correlations with CPUE. This indicates that nutrient availability (Chl a, DIN) and water quality (COD) are key drivers of the fishery resource carrying capacity. The high correlation with protected areas suggests that expanding and optimizing marine protected zones could contribute positively to the sustainable management of fishery resources in this sea.
The East China Sea shows notably high correlation values for DO (0.80), COD (0.85), and area of protected areas (0.79), indicating that DO and COD are critical factors affecting the fishery resource carrying capacity. These factors suggest that water quality, particularly regarding oxygen levels and organic pollution, plays a significant role in sustaining the fishery resources in this region. Additionally, protected areas are shown to have a substantial influence on resource maintenance.
The correlations between environmental factors and CPUE in the South China Sea are generally lower compared to other regions, with the highest correlations seen for PO43- (0.50), DIN (0.49), and area of protected areas (0.53). While these factors have moderate correlations, it is evident that nutrient levels (PO43-, DIN) and protected areas still play an essential role in influencing the fishery resource carrying capacity, although the effects are less pronounced compared to other sea regions.
During the study period (2013–2020), China’s marine fishery resources were predominantly within sustainable limits prior to 2017. However, after 2017, a clear shift toward overexploitation was observed, with declining CPUE/(MSY/Emsγ) ratios across all sea regions. The transition to overexploitation after 2017 aligns with studies highlighting the escalating fishing pressures and environmental degradation in China’s seas (Liu, 2019; Sun et al., 2023). This overall pattern is consistent with global fisheries, where overfishing and poor management policies often result in a rapid decline in resource availability (Andersen et al., 2024; Kang, 2006; Ritchie and Roser, 2023). While the overall trend is clear, the conditions in each sea area differ considerably.
The fisheries carrying capacity in the Bohai Sea is relatively low, primarily due to its semi-enclosed geography and limited water exchange capacity, which result in the accumulation of nutrients and pollutants within the water column (Zou et al., 2024). Key factors affecting the resource status in this region include PO43- and COD, with correlation coefficients of 0.64. Phosphate serves as a limiting nutrient in the Bohai Sea, where increased concentrations enhance phytoplankton productivity, thereby improving fishery resource conditions. Previous studies have shown that phosphate levels in the confined environment of the Bohai Sea directly impact marine primary productivity (Xu et al., 2010; Zhang et al., 2023). Additionally, rising COD levels, associated with organic pollutant accumulation, exacerbate hypoxia, further stressing fishery resources (Zhao et al., 2011).
In contrast, the Yellow Sea exhibits a relatively high resource carrying capacity. Although coastal development in the Yellow Sea is intensive, its open connection with larger bodies of water facilitates strong water exchange, which provides stable nutrient input to support fishery productivity (Yang et al., 2022). Despite this higher carrying capacity, the Yellow Sea’s fishery resources remain overexploited. Chl a, COD, DIN, and protected area coverage show high correlations with CPUE, with protected area size being the most critical factor. This finding underscores the role of protected areas in mitigating fishing pressure and promoting resource recovery. Furthermore, fluctuations in DIN directly affect phytoplankton growth, with both excess and deficiency disrupting ecological balance, destabilizing food supply chains for fish populations, and impacting the sustainable productivity of fishery resources (Zhang et al., 2019; Jin et al., 2021).
The East China Sea has a high resource carrying capacity, largely due to favorable water exchange conditions and abundant nutrient inputs. The interaction between the Kuroshio Current and river runoff provides substantial nutrients and oxygen, supporting high fishery productivity (Wang et al., 2020; Luo et al., 2023). The resource status in the East China Sea is generally sustainable, with DO, Chl-a, and COD identified as key influencing factors. However, eutrophication and hypoxia have been found to limit the recovery of fishery resources in certain areas (Chang et al., 2012; Xu et al., 2024).
The South China Sea also demonstrates a relatively high carrying capacity for fishery resources, aided by its deep-water environment and diverse ecosystems that offer favorable habitats. Sufficient nutrient supply and effective water circulation naturally support fishery productivity. Despite this, fishery resources in the South China Sea face overexploitation, with PO43- levels and protected area coverage being pivotal factors (Zhang, 2024). Given the relatively low nutrient concentrations in the South China Sea, increased phosphate can directly stimulate phytoplankton productivity, supporting the recovery of fishery resources (Yi et al., 2014; Song et al., 2019). While existing protected areas have shown some effectiveness in alleviating fishing pressure, incomplete management and enforcement limit their ability to control overfishing in certain areas (Teh et al., 2017).
Given the diversity of marine environments in China, a more diversified set of management measures is necessary. Different regions face distinct challenges: the Bohai Sea suffers from severe pollution and habitat degradation, while the South China Sea is subject to intense fishing pressure. Research recommends implementing Total Allowable Catch systems tailored to the specific conditions of each region. Pilot projects in areas like Maoming have shown that the introduction of catch quotas and Total Allowable Catch systems has successfully improved sustainability by reducing overfishing and optimizing resource allocation (Lyu et al., 2022). Expanding these programs to include economically significant species in China’s marine fisheries can strengthen population management and reduce resource depletion (Zhu et al., 2021). Furthermore, refining Total Allowable Catch programs can balance ecological sustainability with social equity, especially in regions with severe overfishing (Ding et al., 2022).
Tailoring the duration and timing of fishing bans to the biological characteristics of local species and the carrying capacity of fishery resources is essential for maximizing the effectiveness of these bans. For instance, Yan and Lu (2020) argue that implementing an earlier fishing ban in the South Yellow Sea would significantly enhance the protection of spawning cycles, based on their analysis of local fish population dynamics and the critical timing of spawning events. Studies have shown that region-specific management strategies have successfully reduced overfishing in other contexts as well (Zhao and Jia, 2020). This approach can further promote the recovery of fish populations across various marine regions (Chen et al., 2020).
Expanding Marine Protected Areas (MPAs) is crucial for the recovery and sustainability of fishery resources, particularly in overfished regions (McDonald et al., 2024). MPAs allow fish populations to regenerate by restricting fishing activities, and studies have shown that this leads to increased abundance, size, and diversity of species within the protected zones. Additionally, spillover effects from MPAs, where fish migrate to adjacent areas, help boost catches in nearby fisheries, benefiting both conservation and economic goals (Kerwath et al., 2013). MPAs also protect breeding grounds and promote biodiversity, leading to higher resilience and growth rates in fish populations (Marshall et al., 2019; Franceschini et al., 2024). Combining MPA expansion with habitat restoration efforts, such as the creation of artificial reefs, further enhances fishery recovery, as seen in Daya Bay where artificial reefs increased species diversity and biomass (Yu et al., 2015). This integrated approach ensures both ecological and economic benefits, promoting long-term sustainability of fishery resources.
One limitation of this study is the reliance on relatively coarse estimates of marine fishery resources from the “China Fisheries Statistical Yearbook”, which may not fully capture the spatial and temporal variations in fishery dynamics. To address this, we utilized data from Global Fishing Watch to represent fishing effort more accurately. This dataset provides more granular and comprehensive information on fishing activity, allowing for a better understanding of regional and global patterns in fishing pressure. However, integrating such global datasets with local data sources could further improve the accuracy of fishery resource assessments.
Another limitation of this study is the absence of data from 2021 to 2023. The COVID-19 pandemic disrupted global fishing operations, data collection, and reporting, leading to gaps and inconsistencies in the available records. Additionally, the fishing effort data used in this study is sourced from Global Fishing Watch, which requires substantial time for processing, integration, and validation to ensure high reliability. As a result, the most recent validated dataset extends only to 2020. While the data from 2013 to 2020 provide a robust baseline for analysis, future studies incorporating post-2020 data would further validate and refine the findings presented here.
Additionally, this study uses CPUE as the primary indicator of fishery health. While CPUE is widely used to reflect the abundance and availability of fish stocks, it does have limitations, such as being influenced by changes in fishing technology or effort rather than actual stock health. Future studies could incorporate more direct biological indicators, such as spawning biomass and recruitment rates, to provide a more holistic view of fishery sustainability and resource dynamics.
Based on the findings of this study, several key conclusions can be drawn. Between 2013 and 2020, China’s marine fishery resources underwent notable changes, with catch yields showing an initial increase followed by a gradual decline. There were clear regional differences in fishery yields across major sea areas, with the East China Sea consistently contributing the largest share to total catch. Additionally, the composition of species in the catch highlights the biodiversity of China’s coastal fisheries, with species such as Trichiurus and Engraulis playing a significant role.
Fishing effort trends varied across regions, with the East China Sea showing fluctuating and slightly decreasing fishing intensity, while the South China Sea and Yellow Sea initially increased, followed by a gradual decline. The Bohai Sea, however, maintained consistently low fishing effort. These differences illustrate diverse fishing strategies and resource pressures across regions.
Regarding resource carrying capacity, there were significant differences in MSY among the sea regions. The East China Sea had the highest MSY, supported by the nutrient influx from the Kuroshio Current and land runoff, resulting in higher biological productivity. The South China Sea followed, benefiting from a rich marine ecosystem. The Yellow Sea’s MSY was also substantial due to frequent water exchange, while the Bohai Sea’s MSY was relatively low due to geographical constraints and anthropogenic impacts. These variations underscore the need for tailored management strategies that align with each region’s ecological conditions and resource management requirements. Notably, only the East China Sea remains within sustainable limits, whereas the South China Sea, Yellow Sea, and Bohai Sea have surpassed their ecological carrying capacities, indicating evident overexploitation. Enhanced management in these regions is essential to mitigate the ecological pressures of overfishing.
The results of the grey relational analysis further indicate that factors influencing resource sustainability vary across regions. Nutrient levels and water quality (e.g., chemical oxygen demand) are critical determinants of fishery sustainability, while the distribution and management of protected areas significantly contribute to resource stability.
The original contributions presented in the study are included in the article. Further inquiries can be directed to the corresponding author.
ZL: Conceptualization, Methodology, Project administration, Writing – review & editing. WL: Methodology, Supervision, Validation, Writing – review & editing. TW: Data curation, Formal analysis, Funding acquisition, Methodology, Writing – original draft. YZ: Methodology, Writing – original draft. LH: Methodology, Writing – original draft. LY: Conceptualization, Methodology, Software, Writing – original draft. LD: Methodology, Resources, Writing – original draft.
The author(s) declare financial support was received for the research, authorship, and/or publication of this article. This work was supported by 2024 National Statistical Science Fund of China (2024LZ024).
The authors declare that the research was conducted in the absence of any commercial or financial relationships that could be construed as a potential conflict of interest.
The author(s) declare that no Generative AI was used in the creation of this manuscript.
All claims expressed in this article are solely those of the authors and do not necessarily represent those of their affiliated organizations, or those of the publisher, the editors and the reviewers. Any product that may be evaluated in this article, or claim that may be made by its manufacturer, is not guaranteed or endorsed by the publisher.
Andersen N. F., Cavan E. L., Cheung W. W., Martin A. H., Saba G. K., Sumaila U. R. (2024). Good fisheries management is good carbon management. NPJ Ocean. Sustainabil. 3, 17. doi: 10.1038/s44183-024-00053-x
Bureau of Fisheries of Ministry of Agriculture and Rural Affairs of China (2014-2021). 2014-2021 China Fishery Statistical Yearbook (Beijing: China Agriculture Press).
Chang N., Shiao J., Gong G. (2012). Diversity of demersal fish in the East China Sea: Implication of eutrophication and fishery. Continental. Shelf. Res. 47, 42–54. doi: 10.1016/J.CSR.2012.06.011
Chen J., Chen H., Smith T. F., Rangel-Buitrago N. (2023). Analyzing the impact and evolution of ocean & coastal management: 30 years in retrospect. Ocean. Coast. Manage. 242, 106697. doi: 10.1016/j.ocecoaman.2023.106697
Chen J., Gao J., Liu Y., Chen C., Chen H. (2018). Evolution path of marine fishery management concepts. Mar. Econ. 8, 7.
Chen Y., Shan X., Wang N., Jin X., Guan L., Gorfine H., et al. (2020). Assessment of fish vulnerability to climate change in the Yellow Sea and Bohai Sea. Mar. Freshw. Res. 71, 729–736. doi: 10.1071/MF19101
Demirel N., Zengin M., Ulman A. (2020). First large-scale eastern Mediterranean and Black Sea stock assessment reveals a dramatic decline. Front. Mar. Sci. 7, 103. doi: 10.3389/fmars.2020.00103
Derhy G., Elkalay K., Rincón M. M., Khalil K. (2024). Stochastic surplus production and length-based assessment models to compare Maximum Sustainable Yield advice for the Moroccan Atlantic chub mackerel. Front. Mar. Sci. 11, 1386541. doi: 10.3389/fmars.2024.1386541
Ding Q., Shan X., Jin X., Gorfine H. (2022). Achieving greater equity in allocation of catch shares: A case study in China. Front. Mar. Sci. 9. doi: 10.3389/fmars.2022.1049893
FAO (2020). The state of world fisheries and aquaculture 2020 (Rome: Food and Agriculture Organization of the United Nations).
Fisheries Bureau of the Ministry of Agriculture and Rural Affairs (1999). China fisheries statistical yearbook 1999 (Beijing: China Agriculture Press).
Franceschini S., Lynham J., Madin E. M. (2024). A global test of MPA spillover benefits to recreational fisheries. Sci. Adv. 10, eado9783. doi: 10.1126/sciadv.ado9783
Fulton E. A., Sainsbury K., Noranarttragoon P., Leadbitter D., Staples D. J., Porobic J., et al. (2022). Shifting baselines and deciding on the desirable form of multispecies maximum sustainable yield. ICES. J. Mar. Sci. 79, 2138–2154. doi: 10.1093/icesjms/fsac150
Global Fishing Watch (2021). Fishing Effort. Available online at: https://globalfishingwatch.org/data-download/datasets/public-fishing-effort (Accessed 1 March 2024).
Griffiths C. A., Winker H., Bartolino V., Wennhage H., Orio A., Cardinale M. (2024). Including older fish in fisheries management: A new age-based indicator and reference point for exploited fish stocks. Fish. Fish. 25, 18–37. doi: 10.1111/faf.12789
Han Y. (2018). Marine fishery resources management and policy adjustment in China since 1949. China Rural Econ. 9, 14–28.
Hilborn R., Walters C. J. (1992). Quantitative Fisheries Stock Assessment: Choices, Dynamics and Uncertainty (New York: Chapman and Hall), 570 pp.
Jacobson L. D., Cadrin S. X., Weinberg J. R. (2002). Tools for estimating surplus production and F MSY in any stock assessment model. North Am. J. Fish. Manage. 22, 326–338. doi: 10.1577/1548-8675(2002)022<0326:TFESPA>2.0.CO;2
Jin C. J., Zhang C. S., Wang L. S., Shi X. Y., Han X. R., Tang H. J. (2021). Spatial-temporal variation of dissolved inorganic nitrogen (DIN) and impacts of water mass on the reserves estimation of the total DIN content in the Yellow Sea. China Environ. Sci. 41, 13.
Kang J. (2006). Analysis on the development trends of capture fisheries in North-East Asia and the policy and management implications for regional co-operation. Ocean. Coast. Manage. 49, 42–67. doi: 10.1016/j.ocecoaman.2005.10.002
Kemp P. S., Subbiah G., Barnes R., Boerder K., O’Leary B. C., Stewart B. D., et al. (2023). The future of marine fisheries management and conservation in the United Kingdom: Lessons learnt from over 100 years of biased policy. Mar. Policy 147, 105075. doi: 10.1016/j.marpol.2022.105075
Kerwath S., Winker H., Götz A., Attwood C. (2013). Marine protected area improves yield without disadvantaging fishers. Nat. Commun. 4. doi: 10.1038/ncomms3347
Kokkalis A., Berg C. W., Kapur M. S., Winker H., Jacobsen N. S., Taylor M. H., et al. (2024). Good practices for surplus production models. Fish. Res. 275, 107010. doi: 10.1016/j.fishres.2024.107010
Liu Z. (2019). Research on the status, causes and governance of marine fishing ground desertification. Issues Agric. Econ. 6, 105–116. doi: 10.1029/2022JC019216
Luo Y., Shi J., Guo X., Mao X., Yao P., Zhao B., et al. (2023). Yearly variations in nutrient supply in the East China Sea due to the Zhejiang coastal upwelling and Kuroshio intrusion. J. Geophys. Res.: Oceans. 128, e2022JC019216. doi: 10.1029/2022JC019216
Lyu S., Deng S., Lin K., Zeng J., Wang X. (2022). Designing and the pilot trial of bivalve molluscan fishing quotas on maoming coastal waters of China, northern south China Sea. Front. Mar. Sci. 9, 863376. doi: 10.3389/fmars.2022.863376
Marshall D., Gaines S., Warner R., Barneche D., Bode M. (2019). Underestimating the benefits of marine protected areas for the replenishment of fished populations. Front. Ecol. Environ. 17, 407–413. doi: 10.1002/FEE.2075
McDonald G., Bone J., Costello C., Englander G., Raynor J. (2024). Global expansion of marine protected areas and the redistribution of fishing effort. Proc. Natl. Acad. Sci. 121, e2400592121. doi: 10.1073/pnas.2400592121
Pauly D., Belhabib D., Blomeyer R., Cheung W. W., Cisneros-Montemayor A. M., Copeland D., et al. (2014). China’s distant-water fisheries in the 21st century. Fish. Fish. 15, 474–488. doi: 10.1111/faf.2014.15.issue-3
Pauly D. V., Christensen J., Dalsgaard R., Froese Jr., Torres F. C. (1998). Fishing down marine food webs. Science 279, 860–863. doi: 10.1126/science.279.5352.860
Ritchie H., Roser M. (2023). Fish and overfishing. Our World in Data (United Kingdom: University of Oxford). Available at: https://ourworldindata.org/fish-and-overfishing.
Schaefer M. B. (1954). Some aspects of the dynamic of population important to the management of the commercial marine fisheries. Bull. Inter-American. Trop. Tuna. Commission. 1, 27–56.
Shen G., Heino M. (2014). An overview of marine fisheries management in China. Mar. Policy 44, 265–272. doi: 10.1016/j.marpol.2013.09.012
Song X., Tan M., Xu G., Su X., Liu J., Ni G., et al. (2019). Is phosphorus a limiting factor to regulate the growth of phytoplankton in Daya Bay, northern South China Sea: a mesocosm experiment. Ecotoxicology 28, 559–568. doi: 10.1007/s10646-019-02049-7
Stäbler M., Kempf A., Mackinson S., Poos J. J., Garcia C., Temming A. (2016). Combining efforts to make maximum sustainable yields and good environmental status match in a food-web model of the southern North Sea. Ecol. Model. 331, 17–30. doi: 10.1016/j.ecolmodel.2016.01.020
Sultana R., Liu Q., Al-Mamun M. A., Barman P. P., Shamsuzzaman M. M., Barua S. (2023). Estimating stock status and biological reference points of the sardine fishery using the surplus production model from the bay of Bengal, Bangladesh. J. Mar. Sci. Eng. 11, 944. doi: 10.3390/jmse11050944
Sun M., Li Y., Chen Y. (2023). Unveiling unselective fishing in China: a nationwide meta-analysis of multispecies fisheries. Fish. Fish. 24, 142–158. doi: 10.1111/faf.12715
Sun M., Li Y., Zhang C., Xu B., Ren Y., Chen Y. (2020). Management of data-limited fisheries: identifying informative data to achieve sustainable fisheries. North Am. J. Fish. Manage. 40, 733–751. doi: 10.1002/nafm.10438
Szuwalski C. S., Burgess M. G., Costello C., Gaines S. D. (2017). High fishery catches through trophic cascades in China. Proc. Natl. Acad. Sci. U.S.A. 114, 717–721. doi: 10.1073/pnas.1612722114
Tang Q., Ying Y., Wu Q. (2016). The biomass yields and management challenges for the Yellow Sea large marine ecosystem. Environ. Dev. 17, 175–181. doi: 10.1016/j.envdev.2015.06.012
Teh L., Witter A., Cheung W., Sumaila U., Yin X. (2017). What is at stake? Status and threats to South China Sea marine fisheries. Ambio 46, 57–72. doi: 10.1007/s13280-016-0819-0
Tosun N. (2006). Determination of optimum parameters for multi-performance characteristics in drilling by using grey relational analysis. Int. J. Adv. Manufacturing. Technol. 28, 450–455. doi: 10.1007/s00170-004-2386-y
Wang X., Lu G., Zhao L., Du X., Gao T. (2020). Assessment of fishery resources using environmental DNA: The large yellow croaker (Larimichthys crocea) in the East China Sea. Fish. Res. 235, 105813. doi: 10.1016/j.fishres.2020.105813
Wang Y., Liu Q. (2013). Application of CEDA and ASPIC computer packages to the hairtail (Trichiurus japonicus) fishery in the East China Sea. Chin. J. Oceanol. Limnol. 31, 92–96. doi: 10.1007/s00343-013-2073-7
Wang Z., Feng J., Lozano-Montes H. M., Loneragan N. R., Zhang X., Tian T., et al. (2022). Estimating ecological carrying capacity for stock enhancement in marine ranching ecosystems of Northern China. Front. Mar. Sci. 9. doi: 10.3389/fmars.2022.936028
Xu S., Song J., Li X., Yuan H., Li N., Duan L., et al. (2010). Changes in nitrogen and phosphorus and their effects on phytoplankton in the Bohai Sea. Chin. J. Oceanol. Limnol. 28, 945–952. doi: 10.1007/s00343-010-0005-3
Xu Z., Sun Q., Miao Y., Li H., Wang B., Jin H., et al. (2024). Ecosystem dynamics and hypoxia control in the East China Sea: A bottom-up and top-down perspective. Sci. Total. Environ. 918, 170729. doi: 10.1016/j.scitotenv.2024.170729
Yan Y. Y., Lu C. C. (2020). Suggestions on adjusting the summer fishing moratorium. J. Zhejiang. Ocean. Univ. (Natural. Science). 39, 163–166, 179.
Yang H., Peng D., Liu H., Mu Y., Kim D. (2022). Towards sustainable development of fisheries in the yellow and east China seas shared by South Korea and China. SSRN. Electronic. J. doi: 10.2139/ssrn.4096908
Yi R., Tan Y., Wang S., Shen P., Ke Z., Huang L., et al. (2014). Cell size dependent responses of phytoplankton assemblages to nitrate and phosphate additions in surface waters of the Northern South China sea. Open J. Mar. Sci. 4, 61–67. doi: 10.4236/ojms.2014.42008
Yin J., Xue Y., Xu B., Ji Y., Zhang C., Ren Y., et al. (2024). Potential impacts of ocean warming on energy flow and fisheries production in an overexploited ecosystem: Implication for effective fisheries management. Ecol. Indic. 158, 111433. doi: 10.1016/j.ecolind.2023.111433
Yu J., Pimao C., Tang D., Qin C. (2015). Ecological effects of artificial reefs in Daya Bay of China observed from satellite and in situ measurements. Adv. Space. Res. 55, 2315–2324. doi: 10.1016/j.asr.2015.02.001
Yue W., Hou B., Ye G., Wang Z. (2023). China’s land-sea coordination practice in territorial spatial planning. Ocean. Coast. Manage. 237, 106545. doi: 10.1016/j.ocecoaman.2023.106545
Zhang C., Seo Y., Kang H., Lim J. (2019). Exploitable carrying capacity and potential biomass yield of sectors in the East China Sea, Yellow Sea, and East Sea/Sea of Japan large marine ecosystems. Deep. Sea. Res. Part II.: Topical. Stud. Oceanogr. 162, 77–88. doi: 10.1016/J.DSR2.2018.11.016
Zhang H. (2024). The South China Sea fishing crisis: The overlooked role of Chinese subnational governments. The Pacific Review. 37, 1–30.
Zhang K., Zhao X., Xue J., Mo D., Zhang D., Xiao Z., et al. (2023). The temporal and spatial variation of chlorophyll a concentration in the China Seas and its impact on marine fisheries. Front. Mar. Sci. 10, 1212992. doi: 10.3389/fmars.2023.1212992
Zhao X., Jia P. (2020). Towards sustainable small-scale fisheries in China: A case study of Hainan. Mar. Policy 121, 103935. doi: 10.1016/j.marpol.2020.103935
Zhao X., Wang X., Shi X., Li K., Ding D. (2011). Environmental capacity of chemical oxygen demand in the Bohai Sea: modeling and calculation. Chin. J. Oceanol. Limnol. 29, 46–52. doi: 10.1007/S00343-011-9080-3
Zhu W., Lu Z., Dai Q., Lu K., Li Z., Zhou Y., et al. (2021). Transition to timely and accurate reporting: An evaluation of monitoring programs for China’s first Total Allowable Catch (TAC) pilot fishery. Mar. Policy. 131, 104503. doi: 10.1016/J.MARPOL.2021.104503
Keywords: marine fishery resources, fishing effort, surplus production models, carrying capacity, correlation analysis, sustainable marine fisheries
Citation: Liu Z, Lu W, Wang T, Zhang Y, He L, Yang L and Deng L (2025) The assessment of carrying capacity of marine fishery resources in China. Front. Mar. Sci. 11:1518235. doi: 10.3389/fmars.2024.1518235
Received: 28 October 2024; Accepted: 16 December 2024;
Published: 08 January 2025.
Edited by:
Song Ding, Zhejiang University of Finance and Economics, ChinaReviewed by:
Zhaoqun Liu, Hainan University, ChinaCopyright © 2025 Liu, Lu, Wang, Zhang, He, Yang and Deng. This is an open-access article distributed under the terms of the Creative Commons Attribution License (CC BY). The use, distribution or reproduction in other forums is permitted, provided the original author(s) and the copyright owner(s) are credited and that the original publication in this journal is cited, in accordance with accepted academic practice. No use, distribution or reproduction is permitted which does not comply with these terms.
*Correspondence: Lu Yang, NDU1MDY4NDE1QHFxLmNvbQ==
†These authors have contributed equally to this work and share first authorship
Disclaimer: All claims expressed in this article are solely those of the authors and do not necessarily represent those of their affiliated organizations, or those of the publisher, the editors and the reviewers. Any product that may be evaluated in this article or claim that may be made by its manufacturer is not guaranteed or endorsed by the publisher.
Research integrity at Frontiers
Learn more about the work of our research integrity team to safeguard the quality of each article we publish.