- Integrative Science Center of Germplasm Creation in Western China (Chongqing) Science City, Key Laboratory of Freshwater Fish Reproduction and Development Ministry of Education, College of Fisheries, Southwest University, Chongqing, China
Cortisol is the major glucocorticoid, which is considered to be a key factor linking the environmental stress and sex differentiation in teleosts. In the present study, we explored the possible role of cortisol in sex differentiation in a protogynous hermaphroditic swamp eel, Monopterus albus. We cloned and characterized the full-length cDNA sequences of glucocorticoid receptor genes, gr1 and gr2. A novel alternative splicing variant (gr1b) was also discovered, which lacked a 27-bp nucleotide insertion in gr1, resulting in a nine-amino-acid deletion in GR1. Real-time PCR indicated that swamp eel gr1a mainly expressed in the brain; gr1b mainly in the spleen, head kidney, kidney and muscle; gr2 mainly in the muscle. During female-to-male sex reversal, the expression levels of gr1a and gr1b decreased significantly at the stage of ovotestis III, and gr2 showed a trend of increase, and increased significantly in the testis. In the brain, acute heat stress up-regulated significantly the expression of gr1a and gr1b, while had no significant effect on gr2. However, in the ovary, heat stress only up-regulated significantly the expression of gr1b at 12 h. Serum cortisol concentration increased significantly by heat stress at 1 h, and returned to normal levels at 6 and 12 h. Cortisol injection up-regulated significantly the expression levels of gr1b and gr2, while had no significant effect on gr1a in the ovary. At the same time, cortisol injection down-regulated significantly the expression of cyp19a1a, and only decreased significantly the expression of foxl2 at 6 and 12 h. Cortisol injection up-regulated significantly the expression of dmrt1a at 6, 24 and 48 h, while only increased the expression of gsdf at 6 h. The results indicate that cortisol and GRs may play roles in sex differentiation by the regulation of gonadal differentiation-related gene expression in swamp eel.
1 Introduction
Corticosteroid hormones, including glucocorticoids and mineralocorticoids, are involved in a large number of physiological processes, such as stress response, growth, reproduction, energy balance, immune modulation, and so on (Mommsen et al., 1999; Charmandari et al., 2005; Faught and Vijayan, 2018). Glucocorticoids are synthesized in the cortex from the adrenal glad, and play an important role in stress response, which is regulated by hypothalamic-pituitary-adrenal (HPA) axis in mammals (Whirledge and Cidlowski, 2013). However, glucocorticoids, including cortisol and corticosterone, are synthesized in interrenal cells from the head kidney in teleosts, which are mediated by the hypothalamic-pituitary-interrenal (HPI) axis (Khansari et al., 2019). Cortisol is the main endogenous glucocorticoid, which is mediated by glucocorticoid receptor (GR) and mineralocorticoid receptor (MR) (Kino and Chrousos, 2004). So far, GR is considered to be the mainly receptor for cortisol in teleosts (Cruz et al., 2013).
GR is a ligand-dependent transcription factor, which belongs to the member of nuclear receptor superfamily (Bury, 2017). The structure of GR is relatively conservative, which contains an N-terminal activation function-1 (AF-1), a DNA-binding domain (DBD), a hinge region, and a C-terminal ligand-binding domain (LBD) (Kim et al., 2011). The DBD region contains two highly-conserved zinc finger motifs, which is involved in DNA binding, homo-dimerization, and interactions with other proteins (He et al., 2022). GR was first discovered in rat, and then more GRs were discovered in other vertebrates (Härfstrand et al., 1986). Due to whole genome duplication, two types of GRs, GR1 and GR2, were discovered in most teleosts, which were encoded by gr1 and gr2, respectively (Li et al., 2012; Bury, 2017). In teleosts, GR was first discovered in rainbow trout (Oncorhynchus mykiss) in 1995, and eight years later, a second type of GR was also identified, providing the evidence that two distinct GRs were existed in the same fish (Ducouret et al., 1995; Bury et al., 2003). So far, GRs have been discovered in many teleosts, such as zebrafish (Danio rerio) (Schaaf et al., 2008), sea bass (Dicentrarchus labrax) (Vazzana et al., 2010), medaka (Oryzias dancena) (Kim et al., 2011), channel catfish (Ictalurus punctatus) (Small and Quiniou, 2018), yellow clownfish (Amphiprion clarkii) (Zhang et al., 2020), large yellow croaker (Larimichthys crocea) (He et al., 2022), and so on. In the past, the nomenclature of the two teleost GRs was confused, resulting in the inconvenient to functional research. Based on the rule of nomenclature used in rainbow trout, it is accepted that GR1 has a nine-amino-acid insert between two zinc fingers in the DBD region, and GR2 does not (Vallejos-Vidal et al., 2022a). In some teleosts, due to alternative splicing, it is also found that GR1 has two types, GR1a and GR1b (Takeo et al., 1996; Kim et al., 2011). Compared to GR1a, GR1b only lacks nine amino acids (aa) in the DBD region, resulting in the change on ligand-induced transcriptional activites (Takeo et al., 1996).
The expression of gr1 and gr2 can be detected in various tissues in teleosts, indicating they may have multiple functions. Cortisol, stress and other environmental factors have effects on the expression of grs. In sea bass, long-term exposure to crowding stress resulted in the increase of blood cortisol levels, while down-regulated the expression of gr in the liver (Terova et al., 2005). Cortisol implantation stimulated the increase of plasma cortisol, and up-regulated the expression of gr in gilthead sea bream (Sparus aurata) (Teles et al., 2013a). In large yellow croaker, acute low-salinity stress increased the concentration of plasma cortisol, and up-regulated the expression of gr in some body tissues (He et al., 2022). Recent studies demonstrated that cortisol played an important role in sex differentiation and sex change in teleosts (Hattori et al., 2009; Yamaguchi et al., 2010; Kitano et al., 2012; Goikoetxea et al., 2017). In the brain of medaka, the expression of gr1 was greater in female than that in male, exhibiting sexually dimorphic expression (Kikuchi et al., 2015). Cortisol injection caused the degeneration of oocytes, and the increase of serum 11-ketotestosterone (11-KT) levels, resulting in the sex change in female orange-spotted grouper (Epinephelus coioides) (Chen et al., 2020).
The swamp eel (Monopterus albus), belonging to the family of Synbranchidae of the order Synbranchiformes, was the first discovered protogynous hermaphrodite fish (Zhou and Gui, 2016). It exhibits a similar shape to other living eels, such as American eel, European eel, and Japanese eel, but they belong to the order Anguilliformes (Qu, 2018). In recent years, the total annual production of swamp eel is about 300, 000 tonnes, becoming an important freshwater economic fish in China. So far, the regulatory mechanism of sex reversal is still limited in swamp eel. In this study, we first cloned the full-length cDNA sequences of grs, and detected the expression patterns in various tissues and gonads at different developmental stages of sex reversal in swamp eel. Meanwhile, we detected the effect of acute high-temperature stress on the expression of grs and the concentration of serum cortisol in female swamp eel. And we also evaluated the effect of cortisol on the expression of grs and gonadal differentiation-related genes. These results indicated that cortisol and GRs may be involved in the regulation of gonadal differentiation and sex reversal in swamp eel.
2 Materials and methods
2.1 Animals and sampling
The swamp eels used in this study were purchased from a local farmer’s market in Beibei, Chongqing Province, China. The fish were monitored in an indoor plastic aquarium (80 L) at room temperature (26°C) at the Southwest University Aquaculture Research Base. The fish were fed with tubificidae (3% of body weight) during temporary rearing. One third of the water was replaced once a day. Prior sampling, all the fish were anesthetized with tricaine methanesulphonate (MS-222, 350 mg/L). All experiments were carried out in accordance with the guidelines of the Institutional Animal Care and Use Committees (IACUC) of Southwest University, Chongqing, China (Approval No: IACUC-20230822-01).
To obtain the cDNA sequence of grs, total RNA was isolated from the liver of swamp eel. For tissue distribution analysis, 10 tissues were isolated from five female fish (BL = 37.70 ± 1.16 cm), including heart, liver, spleen, head kidney, kidney, intestine, muscle, brain, pituitary, and ovary. All the tissues were immersed in liquid nitrogen, and then stored at -80°C until total RNA extraction. To obtain the gonads at different developmental stages of sex reversal, the phenotypic sex was confirmed by histological observation under a light microscope. According to the previous classification (Zhang et al., 2008), five sexual phases were chosen for analysis, including ovary (O), ovotestis I (OT1), ovotestis II (OT2), ovotestis III (OT3) and testis (T). And five fish were sampled at each developmental stage.
2.2 RNA isolation and cDNA synthesis
Total RNA was extracted using the RNAiso Plus (Takara, Beijing, China), according to the standard method. Subsequently, the purity and concentration were measured using a Nanodrop 2000 spectrophotometer (Thermo Scientific, Waltham, USA), and the integrity was assessed by agarose gel electrophoresis. Based on the manufacturer’s guidelines, the synthesis of the first-strand cDNA was carried out using the PrimeScript™ RT Reagent Kit with gDNA Eraser (Takara, Beijing, China).
2.3 Cloning of full-length cDNA
To obtained the partial cDNA sequence of grs, two pairs of primers (gr1-F1/gr1-R1, gr2-F1/gr2-R1) were designed according to the predicted gr sequences in the swamp eel genome (GenBank accession number: gr1, XM_020599717; gr2, XM_020619247). The 20 µL PCR mixture contained 10 µL Premix Taq™ (LA Taq™ version 2.0 plus dye) (Takara, Beijing, China), 0.6 µL each of forward and reverse primers (10 mM), 7.8 µL ddH2O and 1.0 µL cDNA. PCR program was as follows: initial denaturation for 1 min at 98°C, followed by 35 cycles of 10 s at 98°C, 30 s at 60°C, and 1.5 min at 72°C, and a final extension for 5 min at 72°C. In order to generate the full-length cDNA sequences of gr1 and gr2, rapid amplification of cDNA ends (RACE) was performed according to the classical method (Sambrook and Russell, 2001). The PCR products were purified from agarose gel using a gel recovery kit (Sangon, Shanghai, China), and cloned into the pMD19-T vector (Takara, Beijing, China). Following transfection into Escherichia coli DH5α competent cells (Takara, Beijing, China), recombinants were confirmed by DNA sequencing. In this study, all the primers were designed using the software of Primer Premier 5.0 (Table 1). Both primer synthesis and DNA sequencing were performed by Sangon Biotech Company in Shanghai, China.
2.4 Bioinformatic analysis of sequences
The full-length cDNA sequences of gr1 and gr2 were generated by the assemblage of the sequences of partial cDNA fragment, 3’RACE and 5’RACE. The open reading frame (ORF) was predicted by an online tool on the NCBI website (https://www.ncbi.nlm.nih.gov/orffinder/). Multiple sequence alignment was performed using the Clustal X 2.0 software. A phylogenetic tree based on the amino acid sequences was constructed by the MEGA 7.0 software using neighbor-joining (NJ) method.
2.5 Quantitative real-time PCR
The qRT-PCR was performed using CFX96™ Real-Time PCR Detection System (BioRad, Hercules, USA). The 20 µL PCR mixture contained 10 µL TB Green® Premix Ex Taq™ II (Tli RNase H Plus) (Takara, Beijing, China), 0.65 µL each primer (10 mM), 6.7 µL ddH2O and 2 µL 5-fold diluted cDNA template. PCR program was as follows: 95°C for 30 s for denaturation, followed by 40 cycles at 95°C for 5 s, 60°C for 30 s. Primer specificity was detected by a melt curve analysis from 65°C to 95°C. Samples were analyzed in triplicate, and a negative control without cDNA was included in each assay. Because of the relatively stable expression, elongation factor-1-α (ef1α) was suitable as reference gene in swamp eel (Hu et al., 2014). The expression levels of the target genes were evaluated using the 2−ΔΔCt method (Livak and Schmittgen, 2001).
2.6 Acute heat stress
To evaluate the effect of acute heat stress, we designed an experiment of high-temperature treatment. After acclimatization for one week, 60 female fish were evenly assigned to six plastic tanks. The plastic tanks were randomly divided into two groups, including control group at ambient temperature (26°C), and the acute heat stress group at high temperature (33°C). The water temperature was controlled and maintained using an electric heating rod. When the temperature increased to 33°C, the time was recorded as 0 h. Samples were collected from five fish at each of the following time points: 1, 6 and 12 h. Blood samples were stored at 4°C overnight, and centrifuged at 5000 r/min for 20 min at 4°C. Serum was collected from supernatant and stored at -20°C until further analysis. Serum cortisol levels were determined using enzyme immunoassay kit (Cayman Chemical, USA) according to the manufacturer’s instructions. Brain and ovary were isolated and stored at -80°C for total RNA extraction.
2.7 Intraperitoneal injection of cortisol
Hydrocortisone (cortisol) was purchased from Macklin Biochemical Technology Company (Shanghai, China), and was dissolved in 0.1% dimethyl sulfoxide (DMSO). Based on previous study, two different doses (1 and 10 mg/kg body weight) were chosen for intraperitoneal (IP) injection (Chen et al., 2020). Two control groups were performed, one of which was only injected with 0.6% physiological saline, and the other was 0.1% DMSO. The IP injection was carried out according to our previous study (Feng et al., 2022), and the injection volume was 10 µL/g body weight. Briefly, after acclimatization for one week, the female swamp eel were chosen for IP injection. Brain and ovary were isolated for total RNA extraction at each of the following time points: 6, 12, 24 and 48 h. Five fish were sampled at each group and time point.
2.8 Statistical analysis
All data were shown as mean ± standard error (SE). Data were analyzed using Statistica version 10.0 software (Statsoft Inc., Tulsa, USA). One-way analysis of variance (ANOVA) was performed to determine any significant differences of mRNA expression levels in various tissues and during sex reversal. One-way ANOVA was also chosen for analyze the difference of gene expression after IP injection at the same sampled point. Data normality and variance homogeneity were tested before the analysis. Significant differences were determined by Duncan’s multiple rang tests. Independent-sample t-test was performed to determine the differences between the control and acute heat stress groups. For all the tests, a p value less than 0.05 was considered statistically significant. All plots were drawn using GraphPad Prism version 8.0.2 (GraphPad Software Inc., CA, USA).
3 Results
3.1 Molecular cloning and sequence analysis
Based on the predicted sequences of grs in the published genome of swamp eel, two different types of grs were obtained, including gr1 and gr2. And gr1 had two different transcripts, gr1a and gr1b, both of which only had a 27-bp difference in the coding region, resulting in the lack of 9 aa in the DBD region of GR1b (Figure 1A). The full-length cDNA sequence of gr1a was 3776 bp (GenBank accession number: PP215140), including a 5’-untranslated region (UTR) of 475 bp, a 3’UTR of 883 bp, and a complete open reading frame (ORF) of 2418 bp, encoding a precursor protein of 805 aa. The full-length cDNA sequence of gr1b was 3749 bp (GenBank accession number: PP215141), including a complete ORF of 2391 bp, which encoded a precursor protein of 796 aa. The full-length cDNA sequence of gr2 was 3237 bp (GenBank accession number: PP215142), including a 5’UTR of 294 bp, a 3’UTR of 618 bp, and a complete ORF of 2325 bp, encoding a precursor protein of 774 aa.
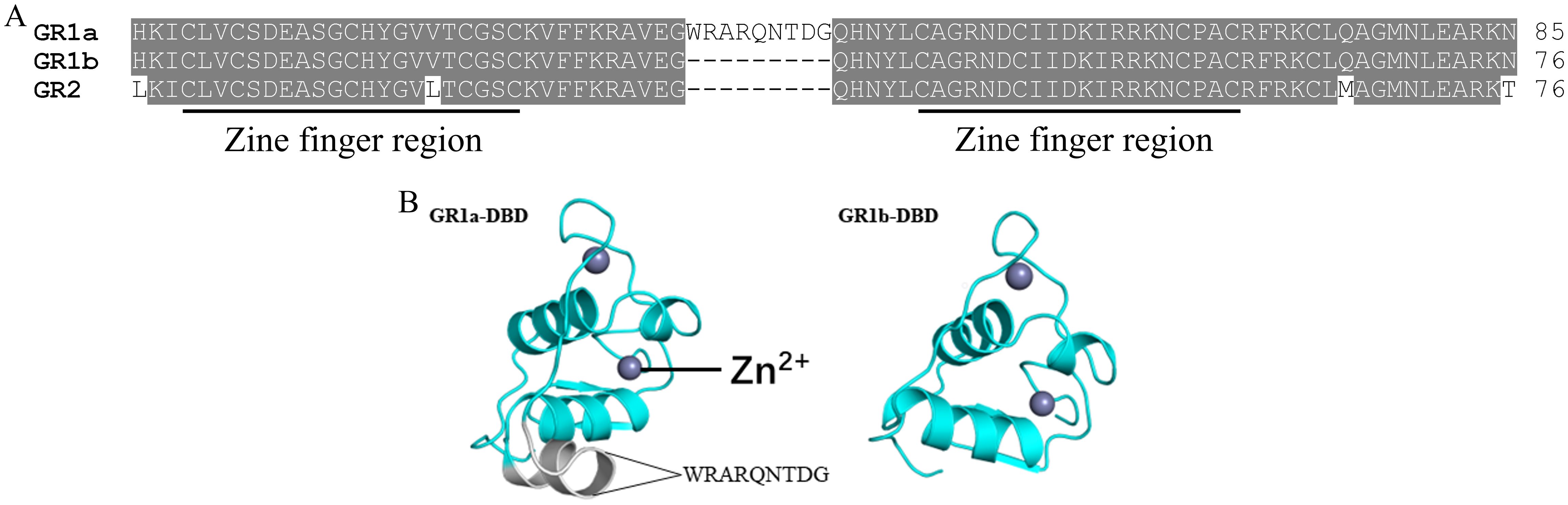
Figure 1. Multiple sequence alignments of swamp eel GR proteins (A) and predicted 3D structures of DBD regions of GR1a and GR1b (B). The two zine finger regions in the DBD region are underlined.
There were two putative zine finger regions in the DBD region (Figure 1A). Sequence alignment of the DBD region indicated that only 4 aa were different between swamp eel GR1 and GR2, indicating it was highly conserved (Figure 1A). Compared to the predicted three dimensional protein structure of GR1b DBD, GR1a DBD obtained an additional helix because of the insertion of the 9 aa, WRARQNTDG (Figure 1B).
A phylogenetic tree was constructed based on the protein sequences of GRs in vertebrates, and swamp eel MR was taken as outgroup (Figure 2). All the vertebrate GRs were separated from swamp eel MR clearly. Teleostean GRs were divided into two big branches, GR1 and GR2, and were separated from tetrapod GRs. Swamp eel GR1 was clustered with the GR1 of black rockfish (Sebastes schlegelii) and European flounder (Platichthys flesus). Swamp eel GR2 was clustered with the GR2 of yellow clownfish, gilthead seabream, European perch (Perca fluviatilis) and black rockfish.
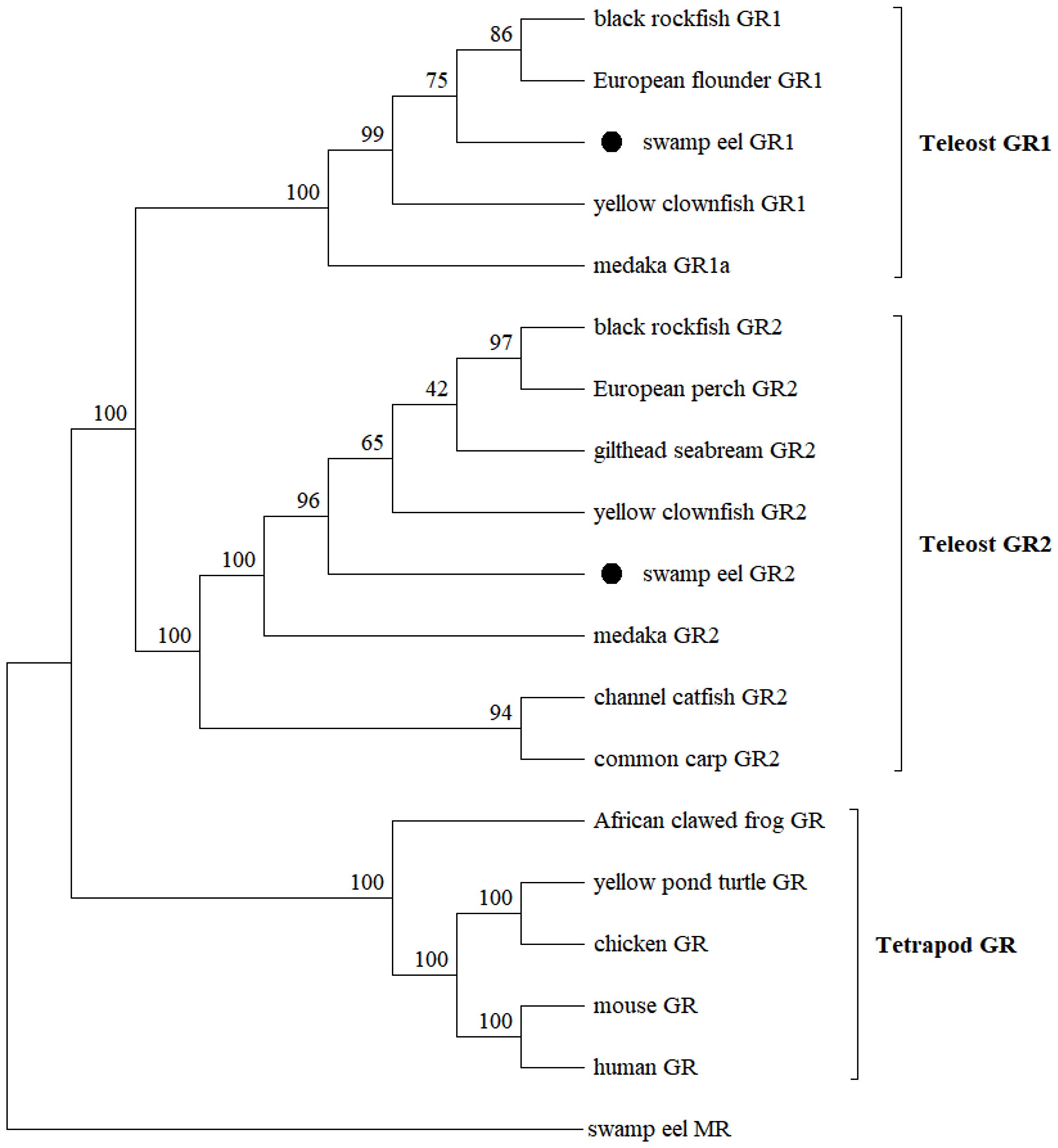
Figure 2. Molecular phylogenetic tree of GRs. GenBank accession numbers are as follows: black rockfish, Sebastes schlegelii (GR1: UPO71093, GR2: UPO71094); European flounder, Platichthys flesus (GR1: AEX56588); yellowtail clownfish, Amphiprion clarkii (GR1: QNJ34169, GR2: QNJ34170); medaka, Oryzias dancena (GR1a: ADZ24979, GR2: ADZ24980); European perch, Perca fluviatilis (GR2: AGR03715); gilthead seabream, Sparus aurata (GR2: ABF30967); channel catfish, Ictalurus punctatus (GR2: AUB30299); common carp, Cyprinus carpio (GR2: CAJ70650); African clawed frog, Xenopus laevis (GR1: NP_001081531); yellowpond turtle, Mauremys mutica (GR: XP_044883169); chicken, Gallus gallus (GR: ABB05045); mouse, Mus musculus (GR: ABF57998); human, Homo sapiens (GR: CAJ65924); swamp eel, Monopterus albus (MR: XP_020447412).
3.2 Tissue distribution of grs in swamp eel
The expression pattern of grs in various tissues was detected using qRT-PCR (Figure 3). The relative expression levels of gr1a were the highest in the brain, higher in the kidney, pituitary, spleen, muscle and head kidney, and lower in ovary, heart, liver and intestine (Figure 3A). The expression levels of gr1b were the highest in the spleen, head kidney, kidney, muscle, followed by brain, ovary, and heart, and the lowest in the liver, intestine and pituitary (Figure 3B). The expression levels of gr2 were the highest in the muscle, followed by heart, spleen, head kidney, kidney, intestine, and brain, and lowest in ovary, liver and pituitary (Figure 3C).
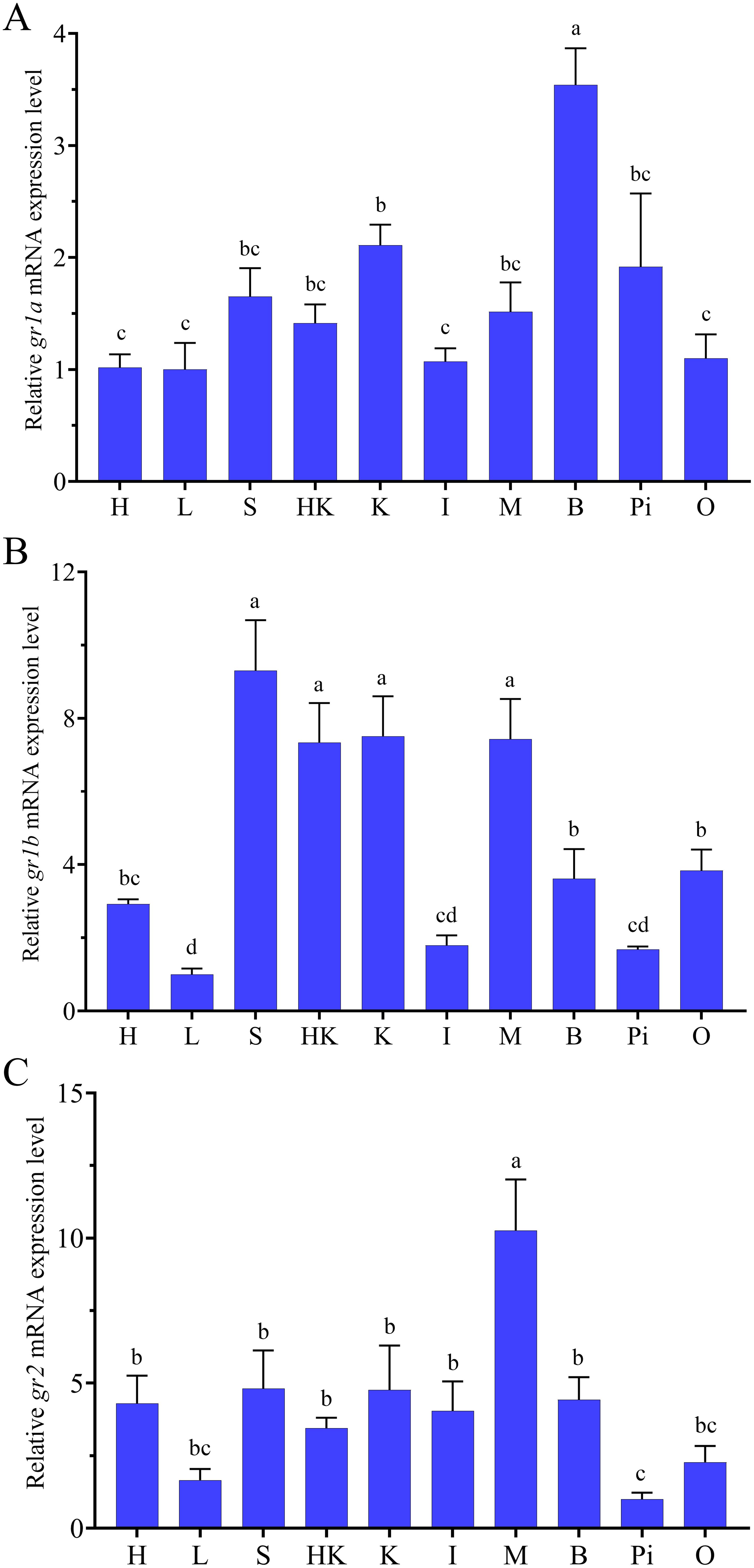
Figure 3. Tissue distribution of gr1a (A), gr1b (B) and gr2 (C) in swamp eel (n=5). H, Heart; L, liver; S, spleen; HK, head kidney; K, kidney; I, intestine; M, muscle; B, brain; Pi, pituitary; O, ovary. Values with different superscript denote significantly difference (P < 0.05).
3.3 The expression changes of grs during sex reversal
During natural sex reversal, compared to the expression in the ovary, the levels of gr1a decreased in the ovotestis and testis, but only decreased significantly in ovotestis III (Figure 4A). The expression levels of gr1b showed a trend of increase followed by decrease, and the increase was not significant in ovotestis I, but decrease significantly in ovotestis II and ovotestis III (Figure 4B). The expression levels of gr2 showed a trend of increase during natural sex reversal, and only increased significantly in the testis (Figure 4C).
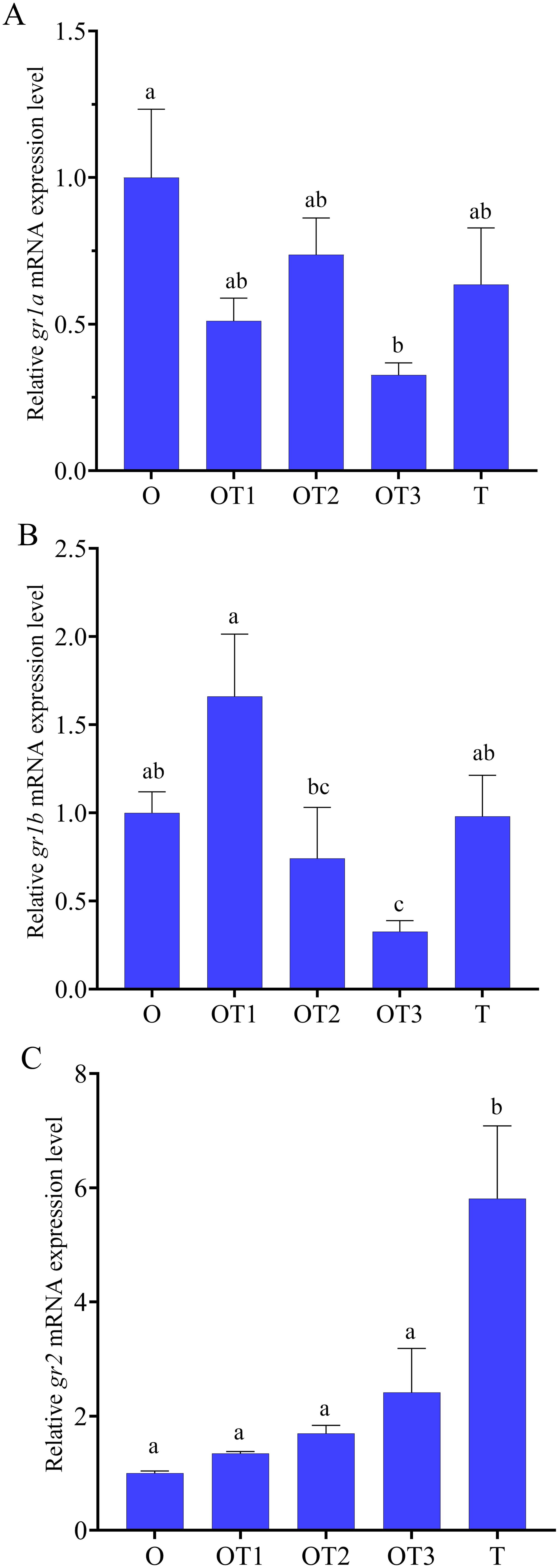
Figure 4. Relative expression levels of gr1a (A), gr1b (B) and gr2 (C) in gonads at different stages of in swamp eel (n=5). Ovary (O), ovotestis I (OT1), ovotestis II (OT2), ovotestis III (OT3), testis (T). Values with different superscript denote significantly difference (P < 0.05).
3.4 Effect of acute heat stress on the expression of grs and serum cortisol levels
In the brain, heat stress significantly down-regulated the expression of gr1a at 1 h, while had no significant change at 6 and 12 h (Figure 5A). Heat stress significant up-regulated the expression levels of gr1b at all the three time points: 1, 6 and 12 h (Figure 5B). Compared to the control group, heat stress up-regulated the expression levels of gr2, but the difference was not significant (Figure 5C). In the ovary, heat stress had no significant effect on the expression of gr1a and gr2, and only significantly up-regulated the expression levels of gr1b at 12 h (Figures 5D–F). Compared to the control group, heat stress stimulated a significant increase of serum cortisol concentration at 1 h, while had no significant effects at 6 and 12 h (Figure 5G).
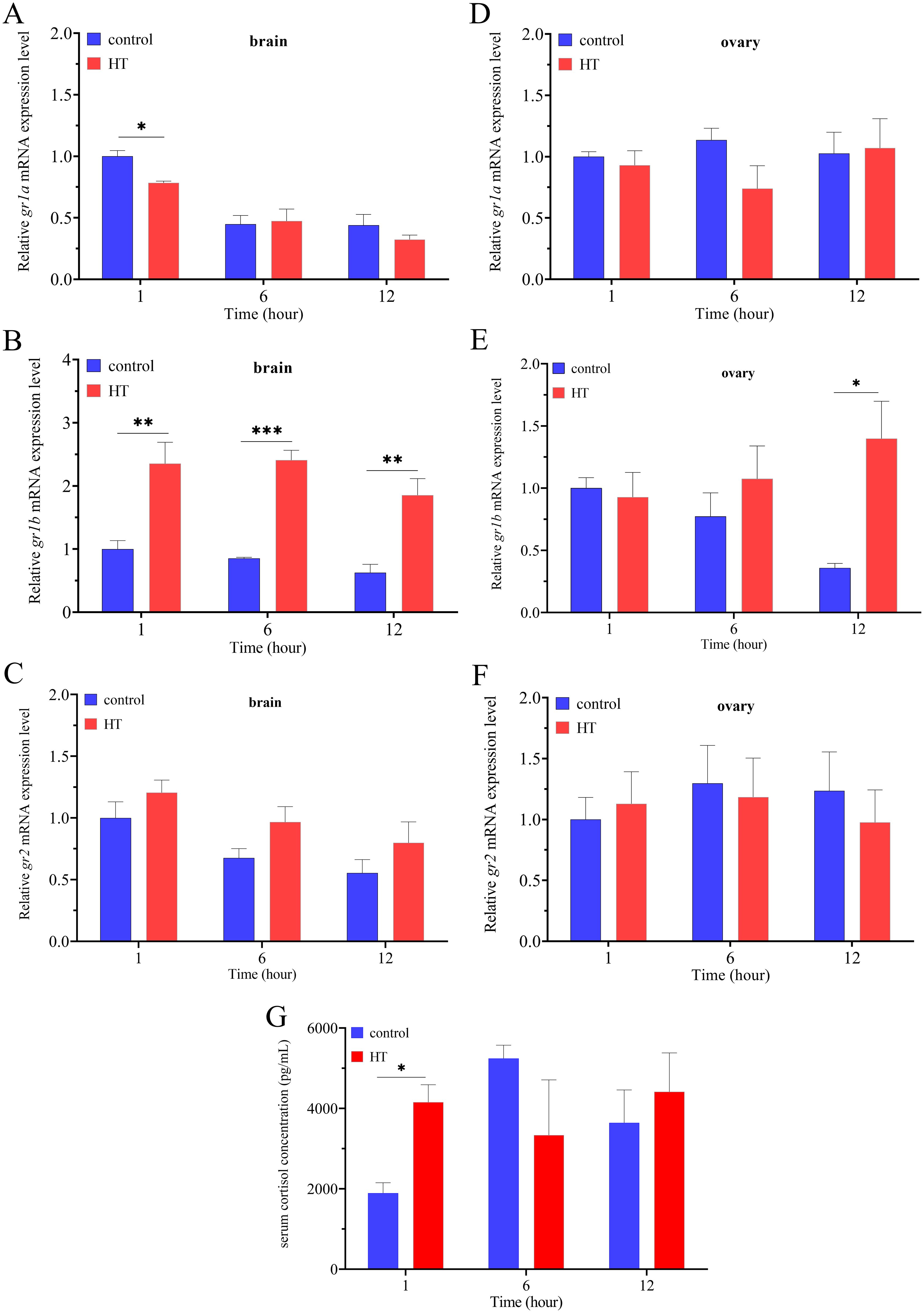
Figure 5. The changes of serum cortisol concentration and relative expression levels of grs in the brain and ovary under acute high temperature stress (n=5). The expression levels of gr1a (A), gr1b (B) and gr2 (C) in the brain under high temperature stress. The expression levels of gr1a (D), gr1b (E) and gr2 (F) in the ovary under high temperature stress. The concentration of serum cortisol under the high temperature stress (G). The significant difference was indicated by an asterisk (P < 0.05), two asterisks (P < 0.01) or three asterisks (P < 0.001).
3.5 Effects of cortisol injection on the expression of grs in the ovary
Compared to the control group injected with 0.6% physiological saline, the injection of 0.1% DMSO had no significant effects on the expression of gr1a, gr1b and gr2 in the ovary (Figure 6). No matter at the low dose of 1 μg/g or high dose of 10 μg/g, cortisol injection had no significant effects on the expression of gr1a in the ovary at all the four time point: 6, 12, 24 and 48 h (Figure 6A). Compared to the control group, the expression levels of gr1b were only significantly up-regulated at the high dose of 10 μg/g at 6, 24 and 48 h, while had no significant change at 12 h (Figure 6B). Cortisol injection only up-regulated the expression levels of gr2 at the high dose of 10 μg/g at 6 h, and had no significant change at the low dose and other time points (Figure 6C).
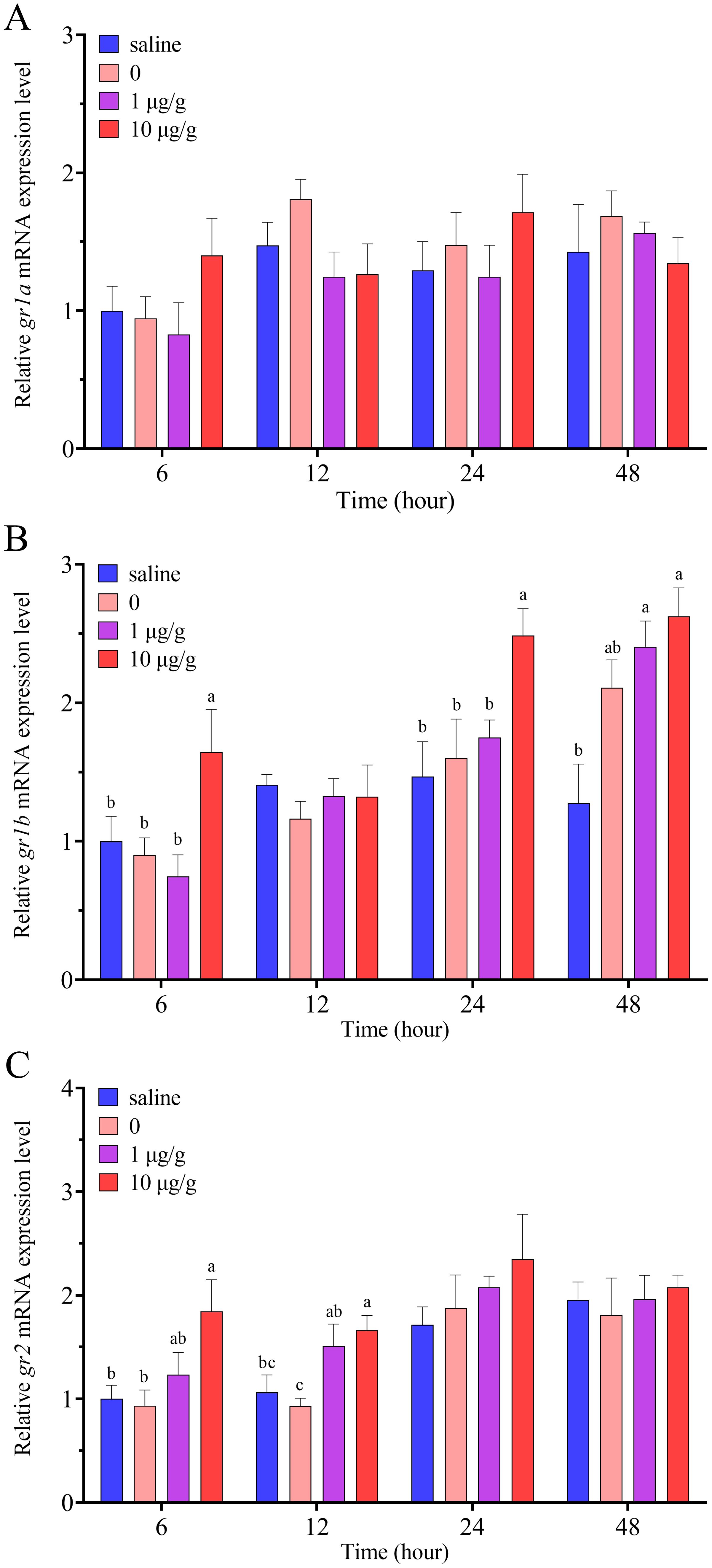
Figure 6. Relative expression levels of gr1a (A), gr1b (B) and gr2 (C) in the ovary after IP injection of cortisol in swamp eel (n=5). The group injected only with 0.1% DMSO was designated as 0. Values with different superscript denote significantly difference at the same sampling time (P < 0.05).
3.6 Effects of cortisol injection on the expression of gonadal differentiation-related genes
Compared to the control group, cortisol injection down-regulated significantly the expression levels of cyp19a1a at all the four time point: 6, 12, 24 and 48 h (Figure 7A). After IP injection of cortisol, the expression levels of foxl2 only decreased significantly at 6 and 12 h, while there was no significant change at 24 and 48 h (Figure 7B). After IP injection of cortisol, the expression levels of dmrt1a were up-regulated significantly at 6, 24 and 48 h, while had no significant change at 12 h (Figure 7C). The expression levels of gsdf were only up-regulated significantly by cortisol at 6 h, while the up-regulation was not significant at 24 and 48 h (Figure 7D).
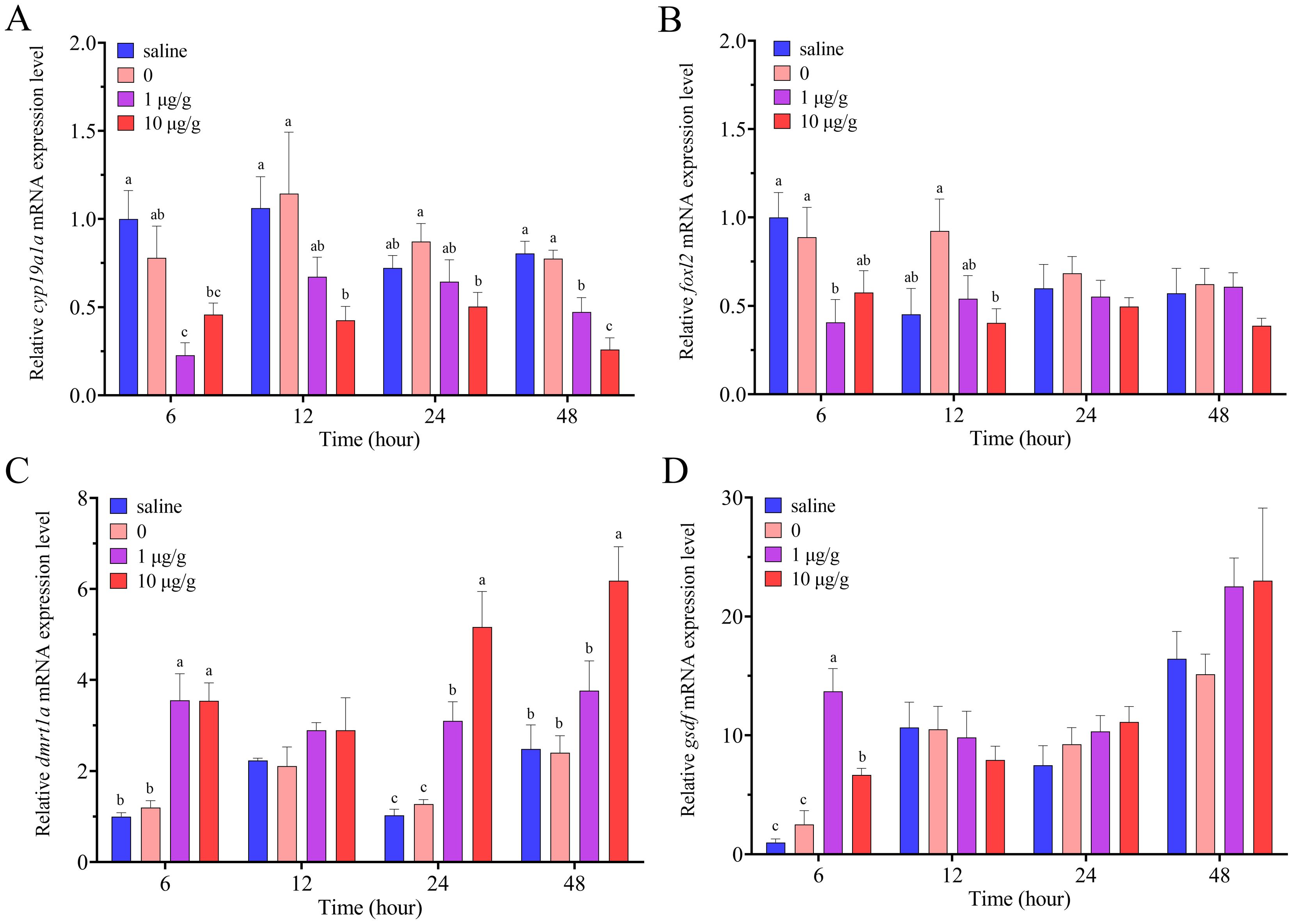
Figure 7. Relative expression levels of cyp19a1a (A), foxl2 (B), dmrt1a (C) and gsdf (D) in the ovary after IP injection of cortisol in swamp eel (n=5). The group injected only with 0.1% DMSO was designated as 0. Values with different superscript denote significantly difference at the same sampling time (P < 0.05).
4 Discussion
Due to the third round of genome duplication, two types of GRs have been identified in most teleosts, such as rainbow trout (Bury et al., 2003), channel catfish (Small and Quiniou, 2018) and large yellow croaker (He et al., 2022). However, only one type of GR has been found in zebrafish (Schaaf et al., 2009). In this study, we obtained two types of grs in swamp eel, gr1 and gr2. And another novel transcript of gr1 (gr1b), was also discovered in swamp eel, which was the same as gr1a except for the lack of the 27-bp insert between the two zine fingers in the DBD region. It is likely the result of alternative splicing, and the same phenomenon has been found in some teleosts, such as rainbow trout (Takeo et al., 1996) and medaka (Kim et al., 2011). The 9-aa insert altered the three dimensional structure of the DBD of GR, resulting in the change of transactivation activity (Greenwood et al., 2003). In previous studies, it had found that GR1a exhibited a higher responsiveness to the agonists than GR1b in rainbow trout (Takeo et al., 1996) and common carp (Cyprinus carpio L.) (Stolte et al., 2008).
Just as most teleost GRs, the sequence of the 9-aa insert was WRARQNTDG in swamp eel. However, in common carp (Stolte et al., 2009), sterlet (Acipenser ruthenus) (Li et al., 2012) and channel catfish (Small and Quiniou, 2018), the sequences of this insert were WRARQNMDG, WRARQNADG and WRARQNTHG, respectively. The results indicate that the insert is not the same, but the sequence of the first 6 aa is relatively conserved. It still needs further study to explore the effects of the difference of the insertion sequences on the functional significance. The phylogenetic tree analysis suggested that swamp eel GR1 and GR2 were clustered into teleost GR1 and GR2, respectively, indicating they divided in the early evolution.
Cortisol is a multifunctional hormone, which plays the role by the mediation of GRs. Therefore, the expression of grs is ubiquitous, but the levels are different in various tissues. In the present study, the tissue expression patterns of gr1a, gr1b and gr2 were different in swamp eel. High expression levels of gr1a were detected in the brain, indicating it may be the main transcript involved in stress response. The expression of gr1b was mainly in the spleen, head kidney, kidney and muscle, indicating it may be involved in metabolism and immune regulation. At the same time, gr2 mainly expressed in the muscle, indicating it may be involved in the growth. In channel catfish, the expression levels of gr1 were significantly higher than those of gr2 in gills, intestine, head kidney and heart, indicating two types of GRs have distinct biological functions (Small and Quiniou, 2018). In previous studies, it also found that gr2 had the highest expression levels in the muscle of ayu (Plecoglossus altivelis) (Ning et al., 2019) and female yellowtail clownfish (Zhang et al., 2020). So far, there is no much research on the discovery and expression analysis of the novel splice variant of gr1. In rainbow trout, gr1b was only isolated in the testis (Takeo et al., 1996). In Haplochromis burtoni, the normal transcript of gr1 mainly expressed in the gill and liver, while the short one mainly expressed in the kidney and spleen (Greenwood et al., 2003). These results indicated that the short transcript may be involved in some novel biological processes.
It is interesting to find that gr1a, gr1b and gr2 had moderate expression levels in the ovary in swamp eel. In previous studies, gr1 and gr2 could also be detected in the gonad of yellowtail clownfish (Zhang et al., 2020) and large yellow croaker (He et al., 2022). In black rockfish, grs were expressed in oocytes, the follicle layer and the ovarian wall (Wang et al., 2022). These results suggested that GRs may be involved in the regulation of gonadal differentiation, development and reproduction. So far, there is no much research focuses on the expression change of grs during sex reversal. In the highly social fish, Neolamprologus pulcher, the expression level of liver gr2 was higher in males than that in females (O’Connor et al., 2013). In protandrous hermaphroditic yellowtail clownfish, the expression level of gr1 in the ovary was significantly higher than that in the testis (Zhang et al., 2020). In our present study, the expression levels of gr1a and gr1b changed in the gonads at different stages of sex reversal, while gr2 increased during this process in swamp eel. The results indicated that GRs were involved in the regulation of sex reversal, and GR2 may play a more important role during this process.
Cortisol is the main corticosteroid hormone in response to environmental stress, which is mediated by GRs in teleosts (Das et al., 2018). The cortisol concentration and expression levels of grs were changed for stress adaptation. In sea bass, high rearing density stress significantly increased the blood cortisol levels, while significantly reduced the expression levels of gr (Terova et al., 2005). In large yellow croaker, acute low-salinity stress significantly increased plasma cortisol concentration, and up-regulated the expression levels of gr2 in the brain, liver and gill tissues (He et al., 2022). In gilthead sea bream, acute air-exposure stress increased the cortisol levels in plasma and skin mucus, and up-regulated the expression levels of gr1 in the skin, gills and anterior gut (Vallejos-Vidal et al., 2022b). Fish are poikilothermic animal, which are easily affected by the environmental water temperature. In medaka larvae, high water temperature treatment significantly stimulated the increase of whole-body levels of cortisol (Hayashi et al., 2010). Acute low temperature stress significantly increased the levels of plasma cortisol and gr mRNA expression in the brain and head kidney in large yellow croaker (Liu et al., 2021). In our study, acute high water temperature treatment sharply increased serum cortisol concentration at 1 h, and significantly up-regulated the expression levels of gr1b in the brain and ovary in swamp eel. The results indicated that acute high temperature adaptation may be mainly mediated by GR1b in swamp eel. Similar results have also been found that acute stress mainly changed the expression levels of gr1 but not gr2 in channel catfish (Small and Quiniou, 2018) and gilthead sea bream (Tsalafouta et al., 2018).
Cortisol activates the specific intracellular responses by GRs, and forms the hormone-receptor complex for the regulation of transactivation or transrepression (Cole, 2006). So far, the regulation of cortisol on the expression of grs exhibits species- and tissue-specific. In mammals, cortisol treatment down-regulated the expression of gr in human and rat cell lines (Rosewicz et al., 1988). In rainbow trout, cortisol implantation significantly up-regulated the expression of gr in the liver (Vijayan et al., 2003). In another similar study, cortisol implantation changed the expression of gr1 and gr2, exhibiting the differentiation with the time and tissue in rainbow trout (Teles et al., 2013b). The expression levels of gr were increased by the treatment of cortisol implantation, but decreased by single IP injection in the intestinal in Mozambique tilapia (Oreochromis mossambicus) (Takahashi et al., 2006). In the present study, cortisol injection stimulated the expression of gr1b and gr2, indicating there existed a mode of autoregulation of gr expression by cortisol in the ovary. And it also suggested that GRs were regulated by cortisol, playing roles in the ovary in swamp eel.
Sex determination and differentiation is complex, which is affected by genetic and environmental factors. As lower vertebrates, the sex of fish is easily affected by environmental factors, such as temperature, rearing density, pH and steroid hormones (Rajendiran et al., 2021). In recent years, it has been founded that cortisol is a mediator involved in the environment-induced sex differentiation and sex change in teleosts (Goikoetxea et al., 2017). Several studies have demonstrated that cortisol is involved in high temperature-induced masculinization in teleosts, such as medaka (Hayashi et al., 2010), Japanese flounder (Oreochromis mossambicus) (Yamaguchi et al., 2010) and pejerry (Odontesthes bonariensis) (Hattori et al., 2009). In Japanese flounder, cortisol incubation suppressed the expression of cyp19a1a by the activation of cAMP signal pathway (Yamaguchi et al., 2010). In female medaka larvae, cortisol exposure inhibited the expression of cyp19a1, and induced the expression of gsdf, resulting in female-to-male sex reversal (Kitano et al., 2012). In protogynous orange-spotted grouper, IP injection of cortisol increased serum 11-KT levels, and the expression of sex-related genes, resulting in the degeneration of oocytes and sex change (Chen et al., 2020). In our study, IP injection of cortisol inhibited the expression of cyp19a1a and foxl2, and stimulated the expression of dmrt1a and gsdf in the ovary in swamp eel. The results suggested that cortisol also exhibited masculinization by the regulation of sex-related genes in protogynous swamp eel. However, it still needs further study to explore the possible regulatory mechanism of cortisol on sex differentiation.
5 Conclusions
In summary, we cloned the full-length cDNA sequences of gr1 and gr2, and discovered that gr1 contained two transcripts, gr1a and gr1b, in swamp eel. The distribution of grs in various tissues suggested they exhibited extensive and different expression. For the first time, we detected the expression of grs in the gonads at different stages of sex reversal, indicating GRs may be involved in gonadal differentiation and development. Acute high temperature stress increased the concentration of serum cortisol and stimulated the expression of gr1b in the brain and ovary. IP injection of cortisol stimulated the expression of gr1b and gr2, and up-regulated the expression of male sex-related genes, while down-regulated female sex-related genes. The results suggested that cortisol and GRs may be involved in the regulation of gonadal differentiation and sex change in swamp eel.
Data availability statement
The datasets presented in this study can be found in online repositories. The names of the repository/repositories and accession number(s) can be found in the article/supplementary material.
Ethics statement
The animal study was approved by Institutional Animal Care and Use Committees (IACUC) of Southwest University, Chongqing, China (Approval No: IACUC-20230822-01). The study was conducted in accordance with the local legislation and institutional requirements.
Author contributions
JS: Data curation, Formal analysis, Methodology, Software, Writing – original draft. LS: Formal analysis, Methodology, Writing – original draft. JY: Methodology, Writing – original draft. WY: Resources, Supervision, Writing – review & editing. HX: Resources, Supervision, Writing – review & editing. KF: Conceptualization, Formal analysis, Funding acquisition, Supervision, Writing – original draft, Writing – review & editing.
Funding
The author(s) declare financial support was received for the research, authorship, and/or publication of this article. This research was supported by the Fundamental Research Funds for the Central Universities (Grant No. SWU-KT23003).
Conflict of interest
The authors declare that the research was conducted in the absence of any commercial or financial relationships that could be constructed as a potential conflict of interest.
Generative AI statement
The author(s) declare that no Generative AI was used in the creation of this manuscript.
Publisher’s note
All claims expressed in this article are solely those of the authors and do not necessarily represent those of their affiliated organizations, or those of the publisher, the editors and the reviewers. Any product that may be evaluated in this article, or claim that may be made by its manufacturer, is not guaranteed or endorsed by the publisher.
References
Bury N. R. (2017). The evolution, structure and function of the ray finned fish (Actinopterygii) glucocorticoid receptors. Gen. Comp. Endocrinol. 251, 4–11. doi: 10.1016/j.ygcen.2016.06.030
Bury N. R., Sturm A., Le Rouzic P., Lethimonier C., Ducouret B., Guiguen Y., et al. (2003). Evidence for two distinct functional glucocorticoid receptors in teleost fish. J. Mol. Endocrinol. 31, 141–156. doi: 10.1677/jme.0.0310141
Charmandari E., Tsigos C., Chrousos G. (2005). Endocrinology of the stress response. Annu. Rev. Physiol. 67, 259–284. doi: 10.1146/annurev.physiol.67.040403.120816
Chen J. X., Peng C., Yu Z. S., Xiao L., Yu Q., Li S. S., et al. (2020). The administration of cortisol induces female-to-male sex change in the protogynous orange-spotted grouper, Epinephelus coioides. Front. Endocrinol. 11, 12. doi: 10.3389/fendo.2020.00012
Cole T. J. (2006). Glucocorticoid action and the development of selective glucocorticoid receptor ligands. Biotechnol. Annu. Rev. 12, 269–300. doi: 10.1016/S1387-2656(06)12008-6
Cruz S. A., Lin C. H., Chao P. L., Hwang P. P. (2013). Glucocorticoid receptor, but not mineralocorticoid receptor, mediates cortisol regulation of epidermal ionocyte development and ion transport in zebrafish (Danio rerio). PloS One 8, e77997. doi: 10.1371/journal.pone.0077997
Das C., Thraya M., Vijayan M. M. (2018). Nongenomic cortisol signaling in fish. Gen. Comp. Endocrinol. 265, 121–127. doi: 10.1016/j.ygcen.2018.04.019
Ducouret B., Tujague M., Ashraf J., Mouchel N., Servel N., Valotaire Y., et al. (1995). Cloning of a teleost fish glucocorticoid receptor shows that it contains a deoxyribonucleic acid-binding domain different from that of mammals. Endocrinology 136, 3774–3783. doi: 10.1210/en.136.9.3774
Faught E., Vijayan M. M. (2018). Maternal stress and fish reproduction: the role of cortisol revisited. Fish Fish. 19, 1016–1030. doi: 10.1111/faf.12309
Feng K., Su J. L., Wu Z. L., Su S. Q., Yao W. Z. (2022). Molecular cloning and expression analysis of thyrotropin-releasing hormone, and its possible role in gonadal differentiation in rice field eel Monopterus albus. Animals 12, 1691. doi: 10.3390/ani12131691
Goikoetxea A., Todd E. V., Gemmell N. J. (2017). Stress and sex: does cortisol mediate sex change in fish? Reproduction 154, 149–160. doi: 10.1530/REP-17-0408
Greenwood A. K., Butler P. C., White R. B., DeMarco U., Pearce D., Fernald R. D. (2003). Multiple corticosteroid receptors in a teleost fish: distinct sequences, expression patterns, and transcriptional activities. Endocrinology 144, 4226–4236. doi: 10.1210/en.2003-0566
Härfstrand A., Fuxe K., Cintra A., Agnati L. F., Zini I., Wikström A. C., et al. (1986). Glucocorticoid receptor immunoreactivity in monoaminergic neurons of rat brain. Proc. Natl. Acad. Sci. U.S.A. 83, 9779–9783. doi: 10.1073/pnas.83.24.9779
Hattori R. S., Fernandino J. I., Kishii A. I., Kimura H., Kinno T., Oura M., et al. (2009). Cortisol-induced masculinization: does thermal stress affect gonadal fate in pejerrey, a teleost fish with temperature-dependent sex determination? PloS One 4, e6548. doi: 10.1371/journal.pone.0006548
Hayashi Y., Kobira H., Yamaguchi T., Shiraishi E., Yazawa T., Hirai T., et al. (2010). High temperature causes masculinization of genetically female medaka by elevation of cortisol. Mol. Reprod. Dev. 77, 679–686. doi: 10.1002/mrd.21203
He L. Y., Shi X. L., Zeng X. Y., Zhou F. F., Lan T. Z., Chen M. S., et al. (2022). Characterization of the glucocorticoid receptor of large yellow croaker (Larimichthys crocea) and its expression in response to salinity and immune stressors. Comp. Biochem. Physsiol. A Mol. Integra. Physiol. 265, 111124. doi: 10.1016/j.cbpa.2021.111124
Hu Q., Guo W., Gao Y., Tang R., Li D. P. (2014). Reference gene selection for real-time RT-PCR normalization in rice field eel (Monopterus albus) during gonad development. Fish Physiol. Biochem. 40, 1721–1730. doi: 10.1007/s10695-014-9962-3
Khansari A. R., Balasch J. C., Vallejos-Vidal E., Teles M., Fierro-Castro C., Tort L., et al. (2019). Comparative study of stress and immune-related transcript outcomes triggered by vibrio Anguillarum bacterin and air exposure stress in liver and spleen of gilthead seabream (Sparus aurata), zebrafish (Danio rerio) and rainbow trout (Oncorhynchus mykiss). Fish Shellfish Immunol. 86, 436–448. doi: 10.1016/j.fsi.2018.11.063
Kikuchi Y., Hosono K., Yamashita J., Kawabata Y., Okubo K. (2015). Glucocorticoid receptor exhibits sexually dimorphic expression in the medaka brain. Gen. Comp. Endocrinol. 223, 47–53. doi: 10.1016/j.ygcen.2015.09.031
Kim M. A., Kim D. S., Sohn Y. C. (2011). Characterization of two functional glucocorticoid receptors in the marine medaka Oryzias dancena. Gen. Comp. Endocrinol. 171, 341–349. doi: 10.1016/j.ygcen.2011.02.015
Kino T., Chrousos G. P. (2004). Glucocorticoid and mineralocorticoid receptors and associated diseases. Essays Biochem. 40, 137–155. doi: 10.1042/bse0400137
Kitano T., Hayashi Y., Shiraishi E., Kamei Y. (2012). Estrogen rescues masculinization of genetically female medaka by exposure to cortisol or high temperature. Mol. Reprod. Dev. 79, 719–726. doi: 10.1002/mrd.22080
Li Y., Sturm A., Cunningham P., Bury N. R. (2012). Evidence for a divergence in function between two glucocorticoid receptors from a basal teleost. BMC Evol. Biol. 12, 1–12. doi: 10.1186/1471-2148-12-137
Liu C., Ding J., Gao X. M., Du C., Hou C. C., Wu X. F., et al. (2021). Effects of acute low temperature stress on the hormones and gene expression of glucocorticoid receptor of large yellow croaker Larimichthys crocea. J. Therm. Biol. 99, 103018. doi: 10.1016/j.jtherbio.2021.103018
Livak K. J., Schmittgen T. D. (2001). Analysis of relative gene expression data using real-time quantitative PCR and the 2-ΔΔCT method. Methods 25, 402–408. doi: 10.1006/meth.2001.1262
Mommsen T. P., Vijayan M. M., Moon T. W. (1999). Cortisol in teleosts: dynamics, mechanisms of action, and metabolic regulation. Rev. Fish Biol. Fisher. 9, 211–268. doi: 10.1023/A:1008924418720
Ning Y. J., Chen S. Y., Lu X. J., Lu J. F., Chen J. (2019). Glucocorticoid receptor in ayu (Plecoglossus altivelis): genomic and non-genomic effects on monocytes/macrophages function. Fish Shellfish Immunol. 86, 1151–1161. doi: 10.1016/j.fsi.2018.12.065
O’Connor C. M., Rodela T. M., Mileva V. R., Balshine S., Gilmour K. M. (2013). Corticosteroid receptor gene expression is related to sex and social behaviour in a social fish. Comp. Biochem. Physsiol. A Mol. Integra. Physiol. 164, 438–446. doi: 10.1016/j.cbpa.2012.12.003
Qu X. C. (2018). Sex determination and control in eels. Sex control aquaculture. 11, 775–792. doi: 10.1002/9781119127291.ch40
Rajendiran P., Jaafar F., Kar S., Sudhakumari C., Senthilkumaran B., Parhar I. S. (2021). Sex determination and differentiation in teleost: roles of genetics, environment, and brain. Biology 10, 973. doi: 10.3390/biology10100973
Rosewicz S., McDonald A. R., Maddux B. A., Goldfine I. D., Miesfeld R. L., Logsdon C. D. (1988). Mechanism of glucocorticoid receptor down-regulation by glucocorticoids. J. Biol. Chem. 263, 2581–2584. doi: 10.1016/S0021-9258(18)69104-5
Sambrook J., Russell D. (2001). Molecular cloning: a laboratory manual. 3rd ed (New York: Cold Spring Harbor Laboratory Press).
Schaaf M. J. M., Champagne D., van Laanen I. H., van Wijk D. C. W. A., Meijer A. H., Meijer O. C., et al. (2008). Discovery of a functional glucocorticoid receptor β-isoform in zebrafish. Endocrinology 149, 1591–1599. doi: 10.1210/en.2007-1364
Schaaf M. J. M., Chatzopoulou A., Spaink H. P. (2009). The zebrafish as a model system for glucocorticoid receptor research. Comp. Biochem. Physsiol. A Mol. Integra. Physiol. 153, 75–82. doi: 10.1016/j.cbpa.2008.12.014
Small B. C., Quiniou S. M. A. (2018). Characterization of two channel catfish, Ictalurus punctatus, glucocorticoid receptors and expression following an acute stressor. Comp. Biochem. Physsiol. A Mol. Integra. Physiol. 216, 42–51. doi: 10.1016/j.cbpa.2017.11.011
Stolte E. H., Chadzinska M., Przybylska D., Flik G., Savelkoul H. F., Verburg-van Kemenade B. L. (2009). The immune response differentially regulates Hsp70 and glucocorticoid receptor expression in vitro and in vivo in common carp (Cyprinus carpio L.). Fish Shellfish Immunol. 27, 9–16. doi: 10.1016/j.fsi.2008.11.003
Stolte E. H., Nabuurs S. B., Bury N. R., Sturm A., Flik G., Savelkoul H. F., et al. (2008). Stress and innate immunity in carp: corticosteroid receptors and pro-inflammatory cytokines. Mol. Immunol. 46, 70–79. doi: 10.1016/j.molimm.2008.07.022
Takahashi H., Sakamoto T., Hyodo S., Shepherd B. S., Kaneko T., Grau E. G. (2006). Expression of glucocorticoid receptor in the intestine of a euryhaline teleost, the Mozambique tilapia (Oreochromis mossambicus): effect of seawater exposure and cortisol treatment. Life Sci. 78, 2329–2335. doi: 10.1016/j.lfs.2005.09.050
Takeo J., Hata J. I., Segawa C., Toyohara H., Yamashita S. (1996). Fish glucocorticoid receptor with splicing variants in the DNA binding domain. FEBS Lett. 389, 244–248. doi: 10.1016/0014-5793(96)00596-0
Teles M., Boltaña S., Reyes-López F., Santos M. A., Mackenzie S., Tort L. (2013a). Effects of chronic cortisol administration on global expression of GR and the liver transcriptome in Sparus aurata. Mar. Biotechnol. 15, 104–114. doi: 10.1007/s10126-012-9467-y
Teles M., Tridico R., Callol A., Fierro-Castro C., Tort L. (2013b). Differential expression of the corticosteroid receptors GR1, GR2 and MR in rainbow trout organs with slow release cortisol implants. Comp. Biochem. Physsiol. A Mol. Integra. Physiol. 164, 506–511. doi: 10.1016/j.cbpa.2012.12.018
Terova G., Gornati R., Rimoldi S., Bernardini G., Saroglia M. (2005). Quantification of a glucocorticoid receptor in sea bass (Dicentrarchus labrax, L.) reared at high stocking density. Gene 357, 144–151. doi: 10.1016/j.gene.2005.06.016
Tsalafouta A., Sarropoulou E., Papandroulakis N., Pavlidis M. (2018). Characterization and expression dynamics of key genes involved in the gilthead sea bream (Sparus aurata) cortisol stress response during early ontogeny. Mar. Biotechnol. 20, 611–622. doi: 10.1007/s10126-018-9833-5
Vallejos-Vidal E., Khansari A. R., Teles M., Reyes-Cerpa S., Mancera J. M., Tort L., et al. (2022a). The insertion/deletion in the DNA-binding region allows the discrimination and subsequent identification of the glucocorticoid receptor 1 (gr1) and gr2 nucleotide sequences in gilthead sea bream (Sparus aurata): standardizing the gr nomenclature for a better understanding of the stress response in teleost fish species. Front. Mar. Sci. 9. doi: 10.3389/fmars.2022.1021046
Vallejos-Vidal E., Sanz-Milian B., Teles M., Reyes-Cerpa S., Mancera J. M., Tort L., et al. (2022b). The gene expression profile of the glucocorticoid receptor 1 (gr1) but not gr2 is modulated in mucosal tissues of gilthead sea bream (Sparus aurata) exposed to acute air-exposure stress. Front. Mar. Sci. 9. doi: 10.3389/fmars.2022.977719
Vazzana M., Vizzini A., Sanfratello M. A., Celi M., Salerno G., Parrinello N. (2010). Differential expression of two glucocorticoid receptors in sea bass (teleost fish) head kidney after exogeneous cortisol inoculation. Comp. Biochem. Physsiol. A Mol. Integra. Physiol. 157, 49–54. doi: 10.1016/j.cbpa.2010.05.003
Vijayan M. M., Raptis S., Sathiyaa R. (2003). Cortisol treatment affects glucocorticoid receptor and glucocorticoid-responsive genes in the liver of rainbow trout. Gen. Comp. Endocrinol. 132, 256–263. doi: 10.1016/S0016-6480(03)00092-3
Wang X. J., Meng W., Qi X., Li Y., Li J. F., Lyu L. K., et al. (2022). Molecular characterization and expression patterns of glucocorticoid receptors in the viviparous black rockfish Sebastes schlegelii. Gen. Comp. Endocrinol. 316, 113947. doi: 10.1016/j.ygcen.2021.113947
Whirledge S., Cidlowski J. A. (2013). A role for glucocorticoids in stress-impaired reproduction: beyond the hypothalamus and pituitary. Endocrinology 154, 4450–4468. doi: 10.1210/en.2013-1652
Yamaguchi T., Yoshinaga N., Yazawa T., Gen K., Kitano T. (2010). Cortisol is involved in temperature-dependent sex determination in the Japanese flounder. Endocrinology 151, 3900–3908. doi: 10.1210/en.2010-0228
Zhang Y. Y., Zhang H., Wang J., Zhang X., Bu S. Y., Liu X. C., et al. (2020). Molecular characterization and expression patterns of glucocorticoid receptor (GR) genes in protandrous hermaphroditic yellowtail clownfish, Amphiprion clarkii. Gene 745, 144651. doi: 10.1016/j.gene.2020.144651
Zhang Y., Zhang W. M., Yang H. Y., Zhou W. L., Hu C. Q., Zhang L. H. (2008). Two cytochrome P450 aromatase genes in the hermaphrodite rice field eel Monopterus albus: mRNA expression during ovarian development and sex change. J. Endocrinol. 199, 317–331. doi: 10.1677/JOE-08-0303
Keywords: cDNA cloning, qRT-PCR, gene expression, sex differentiation, cortisol, glucocorticoid receptor, swamp eel
Citation: Su J, Sun L, Yang J, Yao W, Xu H and Feng K (2025) Cloning and expression analysis of the glucocorticoid receptors and the effects of cortisol in sex differentiation in protogynous hermaphroditic swamp eel, Monopterus albus. Front. Mar. Sci. 11:1518033. doi: 10.3389/fmars.2024.1518033
Received: 27 October 2024; Accepted: 17 December 2024;
Published: 07 January 2025.
Edited by:
Yafei Duan, South China Sea Fisheries Research Institute, ChinaReviewed by:
Basanta Kumar Das, Central Inland Fisheries Research Institute (ICAR), IndiaJorge Aedo, Catholic University of the Maule, Chile
Sofia Priyadarsani Das, National Taiwan Ocean University, Taiwan
Qiaomu Hu, Chinese Academy of Fishery Sciences (CAFS), China
Copyright © 2025 Su, Sun, Yang, Yao, Xu and Feng. This is an open-access article distributed under the terms of the Creative Commons Attribution License (CC BY). The use, distribution or reproduction in other forums is permitted, provided the original author(s) and the copyright owner(s) are credited and that the original publication in this journal is cited, in accordance with accepted academic practice. No use, distribution or reproduction is permitted which does not comply with these terms.
*Correspondence: Ke Feng, ZmVuZ2tlMTYzQHN3dS5lZHUuY24=