- Haikou Marine Geological Survey Center, China Geological Survey, Haikou, China
Surface sediment samples were collected from the surrounding waters of the two largest tourist islands in Sanya, China, to compare and evaluate the sources, distribution, and ecological risks of 21 organochlorine pesticides (OCPs). The total concentration of OCPs ranged from 1.35 to 5.0 ng/g. Among the OCPs, ΣDDTs accounted for the largest proportion, followed by ΣHCHs. The concentrations of HCHs and DDTs from the west side of West Island were significantly higher than those from the east side, and fine-grained sediments exhibited a stronger adsorption effect on OCPs. Source analysis indicated that the area experienced new inputs of HCH pollutants, while historical residues of HCHs remained high. Residual OCPs are still widely present in the environment, transported mainly by river runoff, with a smaller portion originating from atmospheric deposition and ship paints. Ecological risk assessment results showed that factors occasionally causing adverse biological effects include Heptachlor epoxide, 4,4'-DDE, ΣDDT, Dieldrin, Endrin, and γ-HCH, while other factors rarely caused negative biological effects. Potential ecological effect evaluations indicated that stations SY03, SY04, SY06, and SY09 were classified as having moderate ecological effect levels, while other stations were classified as having no ecological effects. Strengthened investigation, monitoring, and control of pollutant sources in ecologically impacted areas are necessary. This study fills a data gap for the region and provides an academic foundation for environmental protection and the sustainable development of tourism resources.
1 Introduction
The persistence of synthetic chemicals in ecosystems represents one of the most critical environmental challenges facing both terrestrial and aquatic environments. Among these chemicals, organochlorine pesticides (OCPs) have been banned for decades in many countries; however, they continue to pose significant risks to ecosystems due to their resistance to degradation (Huang et al., 2019a; Guo et al., 2021). OCPs are a group of synthetically produced, broad-spectrum insecticides characterized by low toxicity and long-lasting residual effects. They were primarily used in pest control during the production of fruits, vegetables, and cereal crops (Wang et al., 2017) and were once the most widely produced and utilized pesticides globally (Chopra et al., 2011). Eight of the twelve persistent organic pollutants (POPs) listed in the Stockholm Convention on Persistent Organic Pollutants are organochlorine pesticides. Despite the prohibition of OCP use in most countries, including China, since the 1980s (Cheng et al., 2021), environmental legacy issues persist, with residues still detected in soils, water bodies, and marine ecosystems (Gai et al., 2014; Xu et al., 2020; Kang et al., 2022). The hazards associated with OCPs have garnered global attention (Lyall et al., 2017; Lan et al., 2019).
From 1952 to 1983, China widely used technical-grade DDTs and HCHs (Zhou et al., 2006). During transport, these substances were subjected to deposition and river inputs, leading to the continued movement of residues, which is a key factor in their persistence within environmental systems (Hu et al., 2011). Due to their volatility and hydrophobic characteristics, organochlorine pesticides exhibit long-range transport, transboundary migration, and multi-media environmental transfer, with deposition occurring in sediments under the influence of gravity (Wania and Mackay, 1996; Kuranchie-Mensah et al., 2012). Oceans, as the ultimate sink for OCPs, receive these pollutants through river runoff and atmospheric deposition, with marine sediments serving as the natural repository for most HCHs and DDTs entering the sea (Liu et al., 2016).
Sanya, located in the tropical monsoon climate zone in southern China, is both an agricultural hub and a major tourist destination, making it particularly vulnerable to the long-term effects of environmental pollutants like OCPs. Given the rapid urbanization and ongoing agricultural activities in the region, the risk of OCP residues in coastal sediments remains a concern. Investigating the levels and distribution of OCPs in the coastal sediments of Sanya is crucial not only for assessing ecological risks but also for ensuring seafood safety, protecting marine biodiversity, and supporting the sustainable development of the tourism industry. This study aims to quantify the levels and spatial distribution of OCPs in Sanya’s coastal sediments, identify their potential sources, and assess the associated ecological risks. These insights are vital for formulating evidence-based environmental management strategies to mitigate pollution and ensure the ecological and economic sustainability of the region.
2 Sample collection and analytical methods
2.1 Sampling locations
2.1.1 Sample collection and analysis
Sample collection for this study was conducted in July 2022. Surface sediment samples from the nearshore areas of a tourist island were collected at ten stations using a surface grab sampler. According to the pacifications for oceanographic survey – Part 8: Marine geology and geophysics survey, the sampling depth was set at 0-5 cm. Upon collection, the samples were thoroughly homogenized, frozen, and transported to the laboratory for further analysis upon the vessel’s return to shore. Sampling locations are illustrated in Figure 1. The analysis of organochlorine pesticides (OCPs) in the collected samples was performed using a gas chromatograph (Agilent 7890, Agilent Technologies, USA). Based on geographical divisions, the study area was categorized into two bays: Sanya Bay and Haitang Bay. Sanya Bay primarily consists of muddy and silty sediments, whereas Haitang Bay is dominated by sandy and gravelly sediments. Land use in the Sanya Bay area is predominantly residential, tourism, and agricultural, while Haitang Bay is characterized by tourism and modern agricultural activities. The main rivers in the study area are the Sanya River and the Tengqiao River.
The sampling coordinates, water depth, and sediment type information are provided in Table 1.
2.2 Analytical methods
Foreign objects (e.g., stones, leaves) were removed from each sample, and two aliquots of approximately 10 g (accurate to 0.01 g) were weighed. One aliquot was used to determine the dry matter content, while the other was air-dried, treated with an appropriate amount of anhydrous sodium sulfate, and then ground and homogenized into a flowable, sand-like dehydrated state. The dehydrated sample was transferred to an extraction vessel, and 30 mL of acetone-n-hexane mixed solvent I was added. Extraction was conducted at 110°C using microwave extraction for 10 minutes. This method improves the extraction efficiency and selectivity by rapidly and uniformly heating the sample, precisely controlling temperature and time, while standardizing the operation, significantly enhancing the efficiency and accuracy of organochlorine pesticide analysis.
The extract was collected by centrifugation or filtration. A layer of glass wool or a glass fiber filter membrane was placed in a glass funnel, onto which approximately 5 g of anhydrous sodium sulfate was added. The extract was filtered directly into a concentration device through the funnel, and the container holding the extract was thoroughly rinsed with 5–10 mL of acetone-n-hexane mixed solvent I, with the rinse solution also filtered into the concentration device. The dehydrated extract was concentrated to 1 mL below 45°C for purification. Approximately 8 mL of n-hexane was used to rinse the magnesium silicate solid-phase extraction column, ensuring the adsorbent surface remained wetted. The concentrated extract was transferred to the magnesium silicate solid-phase extraction column using a pipette, allowed to stand for 1 minute, and the effluent was discarded. Subsequently, 2 mL of acetone-n-hexane mixed solvent II was added and allowed to stand for 1 minute, after which the eluent was collected in a 10 mL mini-concentrate tube. The column was further rinsed with acetone-n-hexane mixed solvent II until the eluent volume reached 10 mL. The purified eluent was concentrated to a final volume of 1.0 mL and then transferred to a 2 mL sample vial for analysis. A total of twenty-one indicators were tested, including ΣHCHs (α-HCH, β-HCH, γ-HCH, δ-HCH), ΣDDTs (4,4’-DDD, 4,4’-DDE, 4,4’-DDT, 2,4’-DDT, methoxychlor [Metox]), ΣCHLs (heptachlor, heptachlor epoxide [isomer B], α-chlordane, γ-chlordane), ΣDRINs (aldrin, dieldrin, endrin, endrin aldehyde, endrin ketone), and ΣENDs (endosulfan I, endosulfan II, endosulfan sulfate). The detection limits for individual OCPs are presented in Table 2.
2.3 Quality control
To ensure the accuracy of organochlorine pesticide (OCP) detection, both a blank experiment and a standard solution at the midpoint concentration of the calibration curve were included in the analysis. The relative deviation between the measured results and the initial calibration curve at this point remained within ±20%. Additionally, a parallel sample and a matrix spike sample were analyzed, with the relative deviation of the parallel sample within 20% and the recovery rate of the spiked sample ranging from 60% to 120%. These results indicate that the analytical procedure met all standard requirements. Waste liquids and residues generated during the experiment were collected and disposed of following proper classification procedures.
Quantitative analysis of OCPs was performed using a gas chromatograph with an electron capture detector (GC-ECD, Agilent Technologies 7890A, USA). The chromatographic column utilized was 30 m in length, with an inner diameter of 0.32 mm and a film thickness of 0.25 µm, featuring a stationary phase of 5% diphenylpolysiloxane and 95% dimethylpolysiloxane. The column temperature program was set as follows: an initial temperature of 100°C, increased at a rate of 15°C/min to 220°C, held for 5 minutes, further increased at 15°C/min to 260°C, and held for 20 minutes. The injector and detector temperatures were maintained at 220°C and 280°C, respectively. GC peaks were identified by comparing retention times to individual authentic standards within a margin of ±0.3%. The standard solution for composite OCPs included 21 congeners, purchased from AccuStandard, Inc., USA. All results for sediment samples in this study were reported on a dry-weight basis.
2.4 Data processing
Residual flow velocity vector maps and sampling station distribution maps were created using ArcGIS mapping software. Classified stacked charts of OCP concentrations and percentage composition charts of OCP homologues for each chlorinated band were produced using Excel. Correlation analysis was visualized using the Genescloud platform, a free online data analysis tool (https://www.genescloud.cn). The Kruskal-Wallis non-parametric test and Dunn’s test were employed to assess significant differences. Potential sources and principal components of OCPs were analyzed using the PCA-multiple linear regression (PCA-MLR) method, with Principal Component Analysis (PCA) conducted and visualized in R 4.2.2. If the concentration of a compound was below the limit of detection (LOD) and reported as not detected (n.d.), the value was replaced by LOD divided by two for statistical analysis.
2.5 Ecological risk assessment calculation
Sediments serve as both reservoirs for pollutants and potential sources of their release into ecosystems. Over the past three decades, numerous Sediment Quality Guidelines (SQGs) have been developed and are widely used for health and environmental risk assessments by regulatory agencies to estimate sediment pollution (Burton, 2002). Four primary assessment methods were employed:(1)CCME Guidelines: Proposed by the Canadian Council of Ministers of the Environment (CCME (Canadian Council of Ministers of the Environment), 2002) to protect aquatic life in marine (including estuarine) environments.(2)NOAA Guidelines: Developed by the National Oceanic and Atmospheric Administration (NOAA (National Oceanic and Atmospheric Administration), 1999), providing sediment quality standards.(3)Freshwater Ecosystems Sediment Quality Guidelines (FESQ): Proposed by MacDonald et al. (2000), focusing on freshwater ecosystems. Each method provides two threshold levels:(1)CCME: Threshold Effect Level (TEL) and Probable Effect Level (PEL).(2)NOAA: Effects Range Low (ERL) and Effects Range Median (ERM).(3)FESQ: Threshold Effect Concentration (TEC) and Probable Effect Concentration (PEC). Table 3 presents the ERL, ERM, TEL, PEL, TEC, and PEC values calculated using these sediment quality guidelines. If the detected concentration of a target compound is below the TEL, ERL, or TEC, it indicates that negative biological effects are unlikely (<25% probability). If the detected concentration exceeds the PEL, ERM, or PEC, it suggests that negative biological effects are likely (>50% probability). Concentrations between the TEL-PEL, ERL-ERM, or TEC-PEC thresholds indicate that occasional negative biological effects may occur.(4)Potential Adverse Biological Effects Evaluation: This method (MacDonald et al., 2000; Tian et al., 2013) assesses the potential adverse effects of five OCP isomers (γ-HCH, dieldrin, p,p’-DDD, p,p’-DDE, p,p’-DDT) using the Sediment Quality Guideline Quotient (SQGQ) (Tian et al., 2013). First, the PEL quotient (PELQ) is calculated using the formula PELQi = Ci/PEL, where PEL is the probable effect level for pollutant i, and Ci is the measured concentration of the same pollutant. The SQGQ is then calculated using the formula SQGQ = ΣPELQ/n, where n is the number of OCPs analyzed with available PEL values. The SQGQ can be classified into three categories: (1) No effect (SQGQ < 0.1); (2) Moderate effect (0.1 ≤ SQGQ < 1); and (3) High adverse biological effect (SQGQ ≥ 1). The potential ecological risk of OCPs in the study area was evaluated using these assessment methods.
3 Results and analysis
3.1 OCP contamination levels
The average concentration of organochlorine pesticides (OCPs) in the study area was 2.96 ng/g dry weight (dw), with mean concentrations of 3.51 ng/g dw in Sanya Bay and 2.13 ng/g dw in Haitang Bay (Figure 2). The overall detection rate for individual OCPs was 32.4%, with γ-HCH, 4,4-DDD, and dieldrin detected in 100% of the samples. The detection rates for 4,4-DDE and methoxychlor were 90% and 70%, respectively. Detection rates for other compounds were 50% or lower, and α-HCH, δ-HCH, 2,4-DDT, α-chlordane, aldrin, as well as endosulfan I and II were not detected in any samples. This absence indicates that these seven compounds exhibit minimal to no accumulation in the environment. Overall, the detection rates for individual OCPs were relatively low.
Hydrodynamic simulations conducted by Hu et al. (2022) for Sanya Bay covered the period from June 1 to July 26, 2019, which coincided with the sampling period of this study. The tidal current characteristics of Sanya Bay were as follows: the flood tide flowed west-northward (WN), while the ebb tide flowed primarily east-southward (ES). Residual currents, representing the net flow after periodic tidal movements, exhibited unidirectional patterns that provided key insights into the transport of pollutants and sediments. The residual current vector map of the study area indicates that water from the Sanya River initially flowed northwestward past Dongdao, then eastward toward West Island (first passing station SY14), where it bifurcated into north and south flows along the coast of West Island (subsequent stations SY09 and SY06). On the western side of West Island, the residual current flowed east-southward and converged at station SY04, while the north-south residual currents moved away from the island at stations SY03 and SY13. The variation in residual current direction corresponded closely with the distribution of OCP concentrations in bottom sediments, exhibiting higher concentrations in areas reached earlier by river runoff and gradually decreasing concentrations downstream. A similar pattern was observed in Haitang Bay, where station HT09, closest to the river mouth and influenced by east-west tidal currents, exhibited a circular flow around Wuzhizhou Island. Consequently, OCP concentrations decreased progressively from station HT09 to HT07, HT05, and HT04.
OCP concentrations in the study area ranged from 1.35 to 5.0 ng/g dry weight (dw). Comparative analysis with OCP contamination levels in other estuarine, bay, and coastal regions (detailed in Table 4) revealed that OCP levels in the study area are lower than those reported in developed countries and densely populated regions, such as Hangzhou Bay, western Egypt, coastal areas of Hainan Island, and South Korean river networks. Notably, although the coastal areas of Hainan Island have lower population density and reduced industrial and agricultural activities, the high density of tourists induces significant disturbances in nearshore waters. This results in sediment resuspension and the release of OCPs, leading to slightly elevated concentrations and indicating that potential risks should not be overlooked. The OCP levels observed in this study are comparable to those reported in most other nearshore sediments globally, including the East China Sea, the southern coast of the Mediterranean, the Seto Inland Sea, and the coral reefs of Sanya Bay. However, they are slightly higher than concentrations found in regions such as the Yellow Sea, the coral reefs of the Xisha Islands, the southwestern coast of India, and Gorgan Bay in Iran.
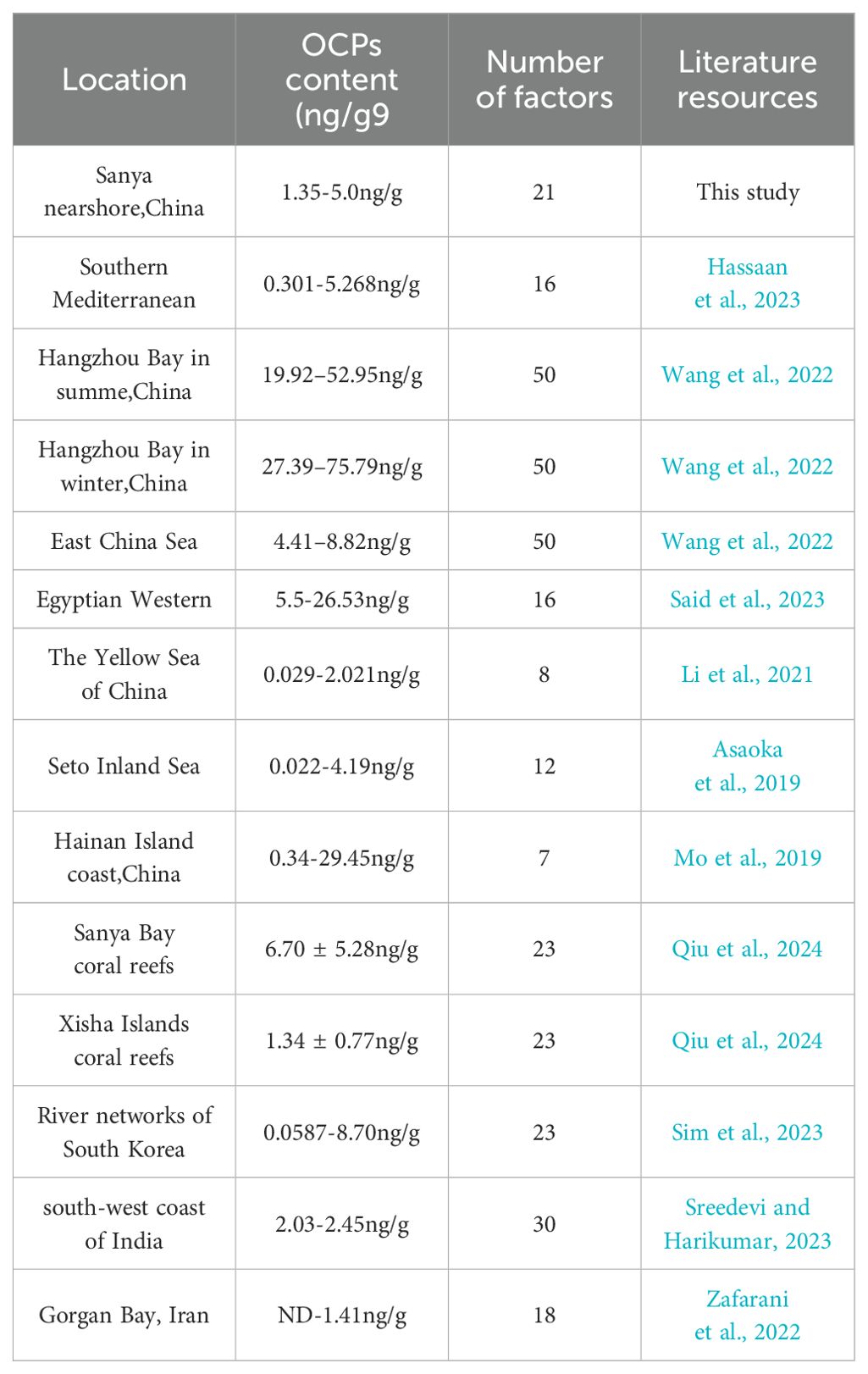
Table 4. Comparison of OCP concentrations in estuarine and coastal sediments between China and other countries.
3.2 Compositional characteristics of OCPs
To elucidate the compositional characteristics of organochlorine pesticides (OCPs) in the collected surface sediment samples, a pie chart illustrating the percentage composition of five OCP groups was created (Figure 3). The results revealed that ΣDDTs constituted the largest proportion (47.58%), followed by ΣHCHs (30.55%), ΣDRINs (14.39%), ΣCHLs (7.09%), and ΣENDs (0.38%), which had the smallest share. Historically utilized in industrial and agricultural applications, DDTs degrade into p,p′-Dichlorodiphenyldichloroethane (DDD) under anaerobic conditions through reductive dichlorination and into p,p′-Dichlorodiphenyldichloroethene (DDE) under aerobic conditions via dehydrogenation oxidation processes (Sun et al., 2010; Alonso-Hernandez et al., 2014). Consequently, the detection rates of DDD and DDE were notably high, at 100% and 90%, respectively. In contrast, the detection rate of 4,4′-DDT was low, observed only at stations SY03, SY04, SY06, and SY09, with no detections at other stations. These specific stations, influenced primarily by the residual currents from the western waters of West Island, indicate a continued input of 4,4′-DDT in the western area. Conversely, the eastern region, mainly affected by the Sanya River, showed no detection of 4,4′-DDT, suggesting that DDT use in Sanya City is of historical origin. In aerobic environments, historical DDT has converted to 4,4′-DDE, resulting in its widespread presence in the eastern region. This distribution correlates with higher organic matter content and enhanced microbial degradation in the area.
The average concentration of β-HCH was 0.35 ng/g dry weight (dw). β-HCH is the most stable of the HCH isomers, rendering it resistant to degradation in sediments (Wacławek et al., 2019). Both α-HCH and γ-HCH can transform into β-HCH, making β-HCH the predominant HCH pollutant. β-HCH was not detected at stations SY03, SY04, SY06, and SY09, whereas γ-HCH was present at high levels at these locations. This pattern aligns with the residual current flow, indicating a continuous input of β-HCH from the western waters of West Island. At other stations, γ-HCH levels were lower than those of β-HCH, reflecting restricted usage of γ-HCH and its subsequent transformation into β-HCH. These stations, primarily influenced by the Sanya River watershed, suggest historical pesticide usage and indicate that the sources of γ-HCH and 4,4′-DDT are similar.
In the study area, dieldrin was more prevalent than its parent compound, aldrin. Aldrin was not detected in any samples, while dieldrin was present in 100% of the samples. Since aldrin and dieldrin were never produced or used in China, their presence indicates global pollutant migration, with atmospheric transport and precipitation serving as the primary sources. At station SY14, 57% of ΣCHLs was observed, with heptachlor being the only compound above the detection limit. Heptachlor rapidly binds to suspended particles in seawater and deposits after being introduced into the ocean via rivers (Guo et al., 2021). Therefore, the elevated concentration of heptachlor at SY14 suggests that the Sanya River is the primary source of this compound.
3.3 Spatial distribution characteristics of OCPs
Since the majority of organochlorine pesticides (OCPs) in nearshore regions are introduced through riverine runoff (Li et al., 2023), the distribution of sampling stations and the OCP concentration profiles presented in Figure 1 indicate that the highest concentration stations in both Sanya Bay and Haitang Bay are located closest to their respective estuaries. In contrast, OCP concentrations at other stations are primarily influenced by the directions of tidal currents and residual flows. Analysis of the compositional characteristics of OCPs revealed that pollutants at stations SY03, SY04, SY06, and SY09 originated not only from the Sanya River but were also transported from the western waters of West Island via residual currents. To better compare the differences between these two sources, a spatial analysis of OCP data was conducted based on defined spatial regions. Figure 4 illustrates the overall differences between the two regions as determined by the Kruskal-Wallis non-parametric test, along with the significance levels of pairwise comparisons using Dunn’s test, reflecting the spatial variation characteristics of OCP concentrations across different regions.
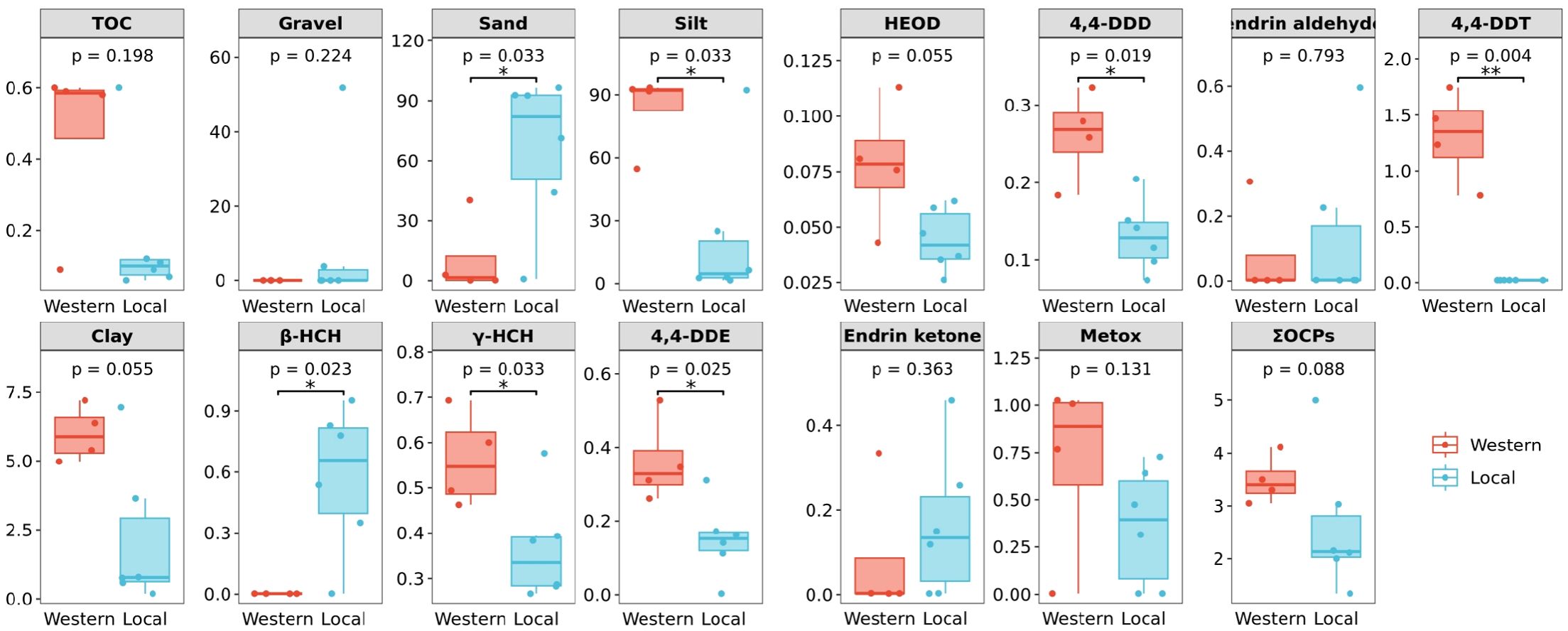
Figure 4. Kruskal-Wallis non-parametric test results for OCP concentration differences in the study area (Significance Levels: *, P<0.05; **, P<0.01; ***, P<0.001).
When comparing geographic regions (Sanya Bay and Haitang Bay), only the concentration of 4,4’-DDD in Sanya Bay was significantly higher than in Haitang Bay (P < 0.05), with no significant differences observed for other OCPs. Given that 4,4’-DDD is a degradation product of DDT, geographic regionalization was deemed unsuitable for this study, and source-based regional characteristics were more appropriate. By categorizing the sources by substance, stations SY03, SY04, SY06, and SY09 were classified as originating from the western side of West Island, while stations SY13, SY14, HT04, HT05, HT07, and HT09 were classified as originating from the eastern side. For OCPs, γ-HCH, 4,4’-DDE, 4,4’-DDD, and 4,4’-DDT were significantly higher on the western side of West Island than on the eastern side. Ratio analysis indicated that lindane is still in use in the study area, while DDE and DDD are degradation products of DDT; the higher concentration of DDT results in significantly elevated levels of these degradation products. Conversely, β-HCH was significantly higher on the eastern side of West Island than on the western side, suggesting less historical use of HCHs on the western side and greater historical usage in the eastern region. These findings suggest that source-based pollution characteristics provide better differentiation than geographic regionalization. Additionally, grain size distribution varied between the two areas, with silt content on the western side of West Island being significantly higher than on the eastern side (P < 0.05), while sand content was significantly higher on the eastern side compared to the western side (P < 0.05).
3.4 Correlation analysis of OCP concentrations with sediment characteristics
To elucidate the factors influencing the transport and migration of organochlorine pesticides (OCPs), we conducted Pearson correlation analysis between OCP concentrations, total organic carbon (TOC), and grain size, using a significance level of P = 0.05. Additionally, hierarchical clustering via hclust was performed, and the results are presented in Figure 5. The analysis revealed that the total OCPs (ΣOCPs) were positively correlated with γ-HCH and 4,4-DDD, indicating a significant influence from these compounds. ΣOCPs also showed positive correlations with TOC, silt, and clay, and a negative correlation with sand, suggesting that hydrophobic OCPs preferentially adsorb onto finer particles. This highlights the crucial role of sediment characteristics in controlling the distribution of organic pollutants (Chen et al., 2006). Consistent with previous studies (Oliveira et al., 2016), OCPs were more likely to adsorb onto fine-grained sediments and deposit nearshore. DDE was positively correlated with TOC, indicating that aerobic microbial degradation of DDT is associated with higher organic carbon content. Fine-grained sediments exhibited strong positive correlations with organic carbon and DDTs, clustering with γ-HCH and dieldrin, which suggests that these compounds share similar adsorption characteristics and transport behaviors. Notably, 4,4-DDT and β-HCH were negatively correlated, reflecting their distinct spatial distribution: 4,4-DDT was primarily sourced recently from the western side of West Island, whereas β-HCH originated historically from the eastern side. This disparity was evident at stations SY03, SY04, SY06, and SY09, leading to their negative correlation. Furthermore, endrin aldehyde, endrin ketone, and β-HCH showed no significant correlations with other factors but clustered with gravel. These compounds possess high persistence and low degradability, enabling their migration across extensive environmental areas, which explains their association with sand.
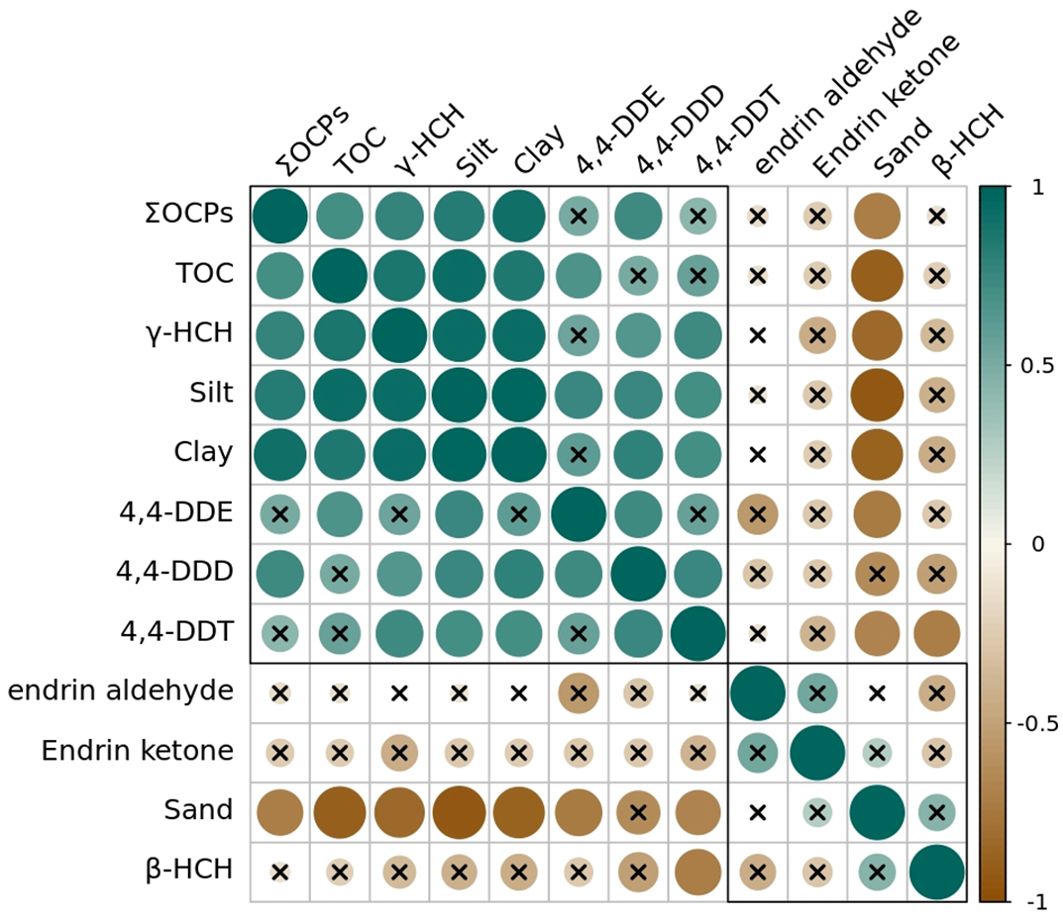
Figure 5. Correlation analysis between PCB concentration and organic carbon content, grain size in the study area.
4 Source analysis and ecological risk of OCPs
The primary sources of organochlorine pesticide (OCP) pollution include wastewater and exhaust emissions from Persistent Organic Pollutant (POP) production, wastewater and exhaust from chlorination processes in chemical manufacturing, and the widespread application of pesticides, including insecticides, for pest control in specific areas. Although DDT and HCH have been banned for many years, they persist in the environment (Zeng et al., 2017). Residual OCPs enter the marine environment primarily through surface runoff, atmospheric deposition, industrial wastewater discharges, riverine inputs from urban waste, soil leaching, and maritime activities (Xu et al., 2020). This study identified relatively high concentrations and detection frequencies of DDT and HCH, underscoring the need for further investigation into the sources of OCP pollutants.
4.1 Determining the sources of OCPs using ratio analysis
Isomeric ratios of HCHs and DDTs are instrumental in identifying potential pollution sources (Nyihirani et al., 2022). HCHs are commercially produced in two formulations: technical HCHs and agricultural HCHs (Li et al., 2015). Technical HCHs in China typically consist of four main isomers: 60–70% α-HCH, 5–12% β-HCH, 10–15% γ-HCH, and 6–10% δ-HCH, with α-HCH being the most abundant. Agricultural HCHs, known as lindane, are composed of over 99% γ-HCH (Sun et al., 2010). The environmental stability of these isomers follows the order γ-HCH < α-HCH < δ-HCH < β-HCH, with γ-HCH being the least stable (Hu and Tao, 2023). β-HCH is the most persistent isomer due to its lower vapor pressure and degradability (Buser and Mueller, 1995). Both α-HCH and γ-HCH can transform into β-HCH in the environment, increasing the proportion of β-HCH over time. A ratio of (δ-HCH + β-HCH)/(α-HCH + γ-HCH) > 0.5 indicates historical HCH residues, while a ratio close to 0.5 suggests recent pollutant inputs. In the study area, ratios at stations SY03, SY04, SY06, SY09, and HT09 were all below 0.013, significantly less than 0.5, indicating new pollutant inputs. These first four stations are on the western side of West Island, and HT09 is near a river mouth, suggesting ongoing agricultural use of lindane in these areas. At other stations, the ratios exceeded 1, indicating substantial historical HCH residues.
The α-HCH/γ-HCH ratio is another commonly used indicator to identify potential HCH sources. A ratio > 7 suggests long-range transport or long-term degradation; a ratio between 3 and 7 indicates industrial-grade HCH sources; and a ratio < 3 suggests the use of lindane in the environment (Qiu et al., 2019). According to He et al. (2013), the α-HCH/γ-HCH ratio for lindane still in use is below 0.1. In the study area, the α-HCH/γ-HCH ratio was less than 0.1, indicating that lindane is still in use in Sanya City.
By calculating the ratios of DDT metabolites, the duration of DDT usage or introduction into the environment can be assessed, revealing source and transport information (Shi et al., 2016). When the (DDD + DDE)/DDTs ratio exceeds 0.5, it indicates long-term degradation of DDT into DDD and DDE; a ratio below 0.5 reflects recent DDT inputs (Ma et al., 2016). In this study, the (DDD + DDE)/DDTs ratio at stations SY03, SY04, SY06, and SY09 was below 0.5, indicating recent DDT inputs on the western side of West Island, whereas other stations had ratios exceeding 0.5, suggesting long-term degradation sources.
Depending on aerobic or anaerobic conditions, DDTs can be degraded by microorganisms into DDE or DDD, respectively. According to Strandberg et al. (1998), the DDT/DDE ratio is a useful indicator for determining whether DDTs at a specific site are recent or historical inputs and can also indicate aerobic or anaerobic conditions (Peng et al., 2020). When the DDD/DDE ratio < 1, it indicates that DDTs are primarily metabolized aerobically, resulting in DDE; if DDD/DDE > 1, it indicates anaerobic metabolism, leading to DDD formation. In the study area, only stations SY13 and HT09 had ratios greater than 1. These stations also exhibited lower organic matter content, sand content greater than 70%, and the lowest total nitrogen content, indicating anaerobic conditions with higher consumption of organic matter and nutrients. Other stations had DDD/DDE ratios less than 1, indicating aerobic metabolism and a higher proportion of DDE in DDTs.
In China, technical-grade DDTs consist of 65%–85% 4,4′-DDT and 15%–21% 2,4′-DDT, while dicofol, synthesized from technical-grade DDTs, contains approximately 11.4% 2,4′-DDT and 1.7% 4,4′-DDT (Qiu et al., 2005). Due to the significant compositional differences between these two formulations, the ratio of 2,4′-DDT to 4,4′-DDT is widely used to distinguish whether DDTs originate from technical-grade DDTs or dicofol, with a ratio below 0.3 indicating technical-grade DDTs and a ratio above 0.3 indicating dicofol (Qiu et al., 2005). In this study, stations SY03, SY04, SY06, and SY09 exhibited 2,4′-DDT/4,4′-DDT ratios below 0.3, indicating that these stations are likely influenced by technical-grade DDTs. Other stations, where 4,4′-DDT was not detected, are not discussed.
The α-chlordane/γ-chlordane ratio can be used to assess the age of chlordane in the environment. However, since γ-chlordane was only detected at station HT05, the discussion is limited to this station. Chlordane degrades slowly in the environment, and the α-chlordane/γ-chlordane ratio at station HT05 was far less than 1, indicating recent chlordane release into the environment. Since its introduction in the 1940s, chlordane has been widely used in residential and agricultural applications (Hidayati et al., 2021). Station HT05 is located closer to the shore compared to other stations, suggesting that the chlordane detected may have originated from point source emissions from nearby residential areas.
In summary, based on the (δ-HCH + β-HCH)/(α-HCH + γ-HCH) and α-HCH/γ-HCH ratios, it can be inferred that stations SY03, SY04, SY06, SY09, and HT09 have new HCH pollutant inputs alongside significant historical HCH residues. The DDTs at stations SY03, SY04, SY06, and SY09 are derived from technical-grade DDTs. The chlordane input at station HT05 is likely due to point source emissions from land-based sources.
4.2 Principal component analysis
To further identify the primary sources and contribution rates of organochlorine pesticides (OCPs) in the study area, a principal component analysis (PCA) was conducted. The concentrations of OCPs were first standardized, and PCA was performed using SPSS 26 for Windows. Initially, the dataset underwent the Kaiser-Meyer-Olkin (KMO) test and Bartlett’s test of sphericity (KMO = 0.51, Bartlett’s test p < 0.05). Since the KMO value exceeded 0.5, PCA was carried out, and principal components were extracted based on the criterion of eigenvalues greater than 1, resulting in a principal component coefficient plot.
The PCA results, depicted in Figure 6, illustrate the factors for each OCP and their loadings. Two principal components (PCs) were identified, accounting for 61.9% and 17.1% of the total variance, respectively. PC1 exhibited high positive loadings for γ-HCH, 4,4-DDE, 4,4-DDD, 4,4-DDT, silt, and clay, primarily representing HCHs and DDTs. Medium loadings were observed for dieldrin and methoxychlor, which are likely associated with runoff sources, as these pesticides are carried by sediment into the ocean, showing positive correlations with silt and clay. The factors associated with PC1 suggest the presence of related pesticides in these areas, as HCHs, DDTs, and dieldrin were extensively used in the past and continue to persist in the environment. Methoxychlor, a replacement for DDT, is still in use (Huang et al., 2019b), indicating ongoing movement of organochlorine pesticides in the environment. OCPs in sediments are primarily derived from terrestrial inputs (Wurl and Obbard, 2005), mainly through river runoff. Riverine sources can be further categorized into non-point source pollution, construction-related pollution, industrial pollution, and agricultural pollution, all of which converge into the ocean via rivers.
The differing correlations between dieldrin and DDT with PC1 are related to their historical usage periods. Although DDT and dieldrin were used during overlapping periods, their peak usage times and subsequent bans differed, with DDT peaking in the 1950s and 1960s and still permitted for certain public health applications, while dieldrin peaked in the 1950s to 1970s before being banned. This resulted in variations in their correlation levels. Methoxychlor, as a substitute, is also present in the environment; however, it’s easier conversion leads to a lower correlation. The medium negative loading for β-HCH in PC1 is attributed to its derivation from γ-HCH degradation, indicating a historical source. Since the western side of West Island shows minimal historical sources, β-HCH exhibits a negative correlation in this region.
PC2 exhibited medium positive loadings for β-HCH and high negative loadings for methoxychlor. Ratio analysis provides evidence of new HCH pollution, as β-HCH is derived from the degradation of γ-HCH. The longer HCH persists in the environment, the higher the proportion of β-HCH, indicating that PC2 represents a historical degradation source. Methoxychlor, as a DDT substitute (Huang et al., 2019), has low toxicity to humans and animals, does not bioaccumulate, and is easily broken down by multifunctional oxidases into water-soluble, non-toxic excretions. It remains widely used and has not been extensively degraded, which explains its strong negative correlation with PC2.
Although PC3 had an eigenvalue less than 1 and accounted for only 8.7% of the variance, it was not considered a principal component. However, it exhibited medium loadings for 4,4-DDE and 4,4-DDD, and a negative loading for dieldrin. DDE and DDD originate from the same pollution source (DDT) and share similar environmental behaviors and degradation pathways. PC3 is likely related to atmospheric deposition sources, as dieldrin, DDT, and its degradation products are volatile and capable of long-range atmospheric transport. Although dieldrin also enters the environment through atmospheric deposition, it may degrade more rapidly under certain conditions, resulting in less accumulation compared to DDE and DDD. This explains the negative loading of dieldrin in PC3. Another potential source is from ships, as DDT is not only used for controlling agricultural pests and malaria but also as an active ingredient in antifouling paints for fishing vessels in some developing countries, including China (Peng et al., 2020). This would account for the positive correlation with DDE and DDD and the negative correlation with dieldrin.
In summary, based on the (δ-HCH + β-HCH)/(α-HCH + γ-HCH) and α-HCH/γ-HCH ratios, it can be inferred that stations SY03, SY04, SY06, SY09, and HT09 have new HCH pollutant inputs alongside significant historical HCH residues. The DDTs at stations SY03, SY04, SY06, and SY09 are derived from technical-grade DDTs, while the chlordane input at station HT05 is likely due to point source emissions from land-based sources.
4.3 Ecological risk assessment
The ecological risk assessment results are summarized in Table 5. The analysis indicates that no stations or factors consistently cause adverse biological effects. However, certain pollutants occasionally pose risks to biological health, including Heptachlor epoxide, 4,4’-DDE, ΣDDT, Dieldrin, Endrin, and γ-HCH. Using the CCME evaluation method, only 4,4’-DDT and γ-HCH were identified as occasional threats. The NOAA evaluation method identified five pollutants—Heptachlor epoxide, 4,4’-DDE, ΣDDT, Dieldrin, and Endrin—as occasional causes of adverse biological effects. In contrast, the FESQ method assessed all pollutants as rarely causing adverse biological effects.
From a station-specific perspective, the primary locations associated with adverse biological effects are SY03, SY04, SY06, SY09, HT07, and HT09. The four SY stations are primarily influenced by the western side of West Island, where pollutant concentrations are generally higher, resulting in occasional adverse biological effects. This necessitates attention to specific pollution sources and transport mechanisms. Station HT09, located near the estuary of Houhai Bay, exhibited pollutants including Heptachlor epoxide, Dieldrin, Endrin, and γ-HCH. These OCPs have been banned, indicating significant historical usage, yet historical residues continue to be transported from land to sea. The pollutants that occasionally cause negative biological effects were banned between the 1970s and 1980s, with γ-HCH specifically banned in 2009 under the Stockholm Convention (Handl, 2012). Despite their bans, these compounds have half-lives of approximately 5–10 years in soil and sediments, leading to their persistence and slow degradation rates. Consequently, they continue to pose occasional negative biological effects. Regarding future ecological risk predictions, pollutant concentrations are expected to gradually decrease over the medium term (10–30 years), although some ecosystems, particularly those with a history of severe pollution, may continue to experience ecological pressures. In the long term (over 30 years), most pollutant concentrations are anticipated to diminish, thereby reducing ecological risks. However, certain persistent pollutants may remain in sediments for extended periods, potentially continuing to affect ecosystems in specific regions.
Based on the three sediment quality assessment methods (SQGs) utilized in this study, we determined that the CCME method is more suitable for estuarine and nearshore marine areas, the NOAA method is more appropriate for evaluating sediment quality in terrestrial regions, and the FESQ method is best suited for riverine areas where pollutants are more concentrated and present in higher quantities.
The ecological risk assessment results, presented in Table 5, indicate that stations SY03, SY04, SY06, and SY09 have Sediment Quality Guideline Quotient (SQGQ) values within the range of 0.1 ≤ SQGQ < 1. This categorization signifies that these four stations are areas with moderate adverse biological effects. In contrast, the SQGQ values at all other stations are below 0.1, suggesting that adverse biological effects are unlikely in those areas. Consequently, both the sediment quality standards evaluation and the ecological risk assessment identify SY03, SY04, SY06, and SY09 as critical areas of concern. This highlights that the primary source of pollution in the study area originates from the western side of West Island, which has already exerted a negative ecological impact on the region. Therefore, there is an urgent need to enhance the investigation and monitoring of pollution sources on the western side of West Island and implement targeted control measures. This will be the main focus of our next phase of work and research.
Based on the spatial distribution characteristics and source analysis of organochlorine pesticides (OCPs), it is evident that OCPs persist in the environment, accumulating from rivers into nearshore areas. In Sanya City, pollution sources are primarily divided between the western and eastern sides of West Island. The eastern side is mainly influenced by inputs from the Sanya River and the Haitang Bay River, whereas the specific source on the western side remains uncertain. It is hypothesized that the source may be the Ningyuan River, located 40 km from the Sanya River, or possibly the Beibu Gulf, which has a more complex origin potentially involving contributions from Guangxi Province in China, the Qiongzhou Strait, or Vietnam. Consequently, subsequent research will focus on tracing the western pollution sources of West Island, beginning with monitoring the Ningyuan River and extending to broader sampling across the western marine region to further clarify the specific sources affecting the western side of West Island. The objective is to obtain more comprehensive data on the distribution and sources of OCP pollutants, thereby enabling the formulation of effective environmental protection and monitoring strategies, ultimately reducing pesticide usage and minimizing ecological toxicity and pollution.
5 Conclusion
This study of organochlorine pesticides (OCPs) in the marine areas surrounding the two largest tourist islands in Sanya City demonstrates that OCPs persist in the surface sediments of coastal waters, with concentrations ranging from 1.35 to 5.0 ng/g dry weight (dw) and an average concentration of 2.96 ng/g dw. The distribution of OCP concentrations closely follows the patterns of residual currents, indicating that these currents significantly influence pollutant distribution in sediments. Among the detected OCPs, γ-HCH, 4,4’-DDD, and dieldrin were present in 100% of the samples, with ΣDDTs (47.58%) being the most predominant group, followed by ΣHCHs (30.55%). Based on the residual current characteristics in the study area, pollutant sources were categorized into western and eastern origins of West Island. Concentrations of β-HCH, γ-HCH, 4,4’-DDE, 4,4’-DDD, and 4,4’-DDT were significantly higher on the western side. Additionally, γ-HCH and DDTs exhibited strong adsorption to fine-grained sediments, showing a positive correlation. The primary sources of OCPs in the study area are historical residues, with minor contributions from atmospheric deposition and ship coatings. Furthermore, new inputs of HCHs have been identified. The potential ecological impact of OCPs on the western side of West Island is assessed as moderate, highlighting the need for enhanced tracing and monitoring of pollution sources in this region. Developing more effective environmental protection and management strategies will be the focus of our future research efforts.
Data availability statement
The raw data supporting the conclusions of this article will be made available by the authors, without undue reservation.
Author contributions
HW: Validation, Visualization, Writing – original draft. LZ: Writing – review & editing. FY: Supervision, Writing – review & editing. LY: Resources, Writing – original draft. CL: Investigation, Writing – original draft. CS: Methodology, Writing – original draft.
Funding
The author(s) declare financial support was received for the research, authorship, and/or publication of this article. We appreciate the financial support of the China Geological Survey Haicheng wen coastal natural resources comprehensive survey project (DD20230414); Hainan Haitang Bay-Yazhou Bay Island (reef) comprehensive geological survey(No.DD20220992). Comprehensive geological survey of Xisha Xuande Atoll(DD20242841); 1:250,000 Marine Regional Geological Survey of the Qizhou Archipelago Waters(No.DD20230413); Haikou Key Laboratory of Marine Contaminants Monitoring Innovation and Application; and Innovation Base for Island Reef Spatial Resource Investigation, Monitoring, and Technology Utilization.
Conflict of interest
The authors declare that the research was conducted in the absence of any commercial or financial relationships that could be construed as a potential conflict of interest.
Generative AI statement
The author(s) declare that no Generative AI was used in the creation of this manuscript.
Publisher’s note
All claims expressed in this article are solely those of the authors and do not necessarily represent those of their affiliated organizations, or those of the publisher, the editors and the reviewers. Any product that may be evaluated in this article, or claim that may be made by its manufacturer, is not guaranteed or endorsed by the publisher.
References
Alonso-Hernandez C. M., Mesa-Albernas M., Tolosa I. (2014). Organochlorine pesticides (OCPs) and polychlorinated biphenyls (PCBs) in sediments from the Gulf of Batabanó, Cuba. Chemosphere 94, 36–41. doi: 10.1016/j.chemosphere.2013.09.007
Asaoka S., Umehara A., Haga Y., Matsumura C., Yoshiki R., Takeda K. (2019). Persistent organic pollutants are still present in surface marine sediments from the Seto Inland Sea, Japan. Mar. Pollut. Bull. 149, 110543. doi: 10.1016/j.marpolbul.2019.110543
Burton J. R. G. (2002). Sediment quality criteria in use around the world. Limnology 3, 65–76. doi: 10.1007/s102010200008
Buser H. R., Mueller M. D. (1995). Isomer and enantioselective degradation of hexachlorocyclohexane isomers in sewage sludge under anaerobic conditions. Environ. Sci. Technol. 29, 664–672. doi: 10.1021/es00003a013
CCME (Canadian Council of Ministers of the Environment) (2002). “Canadian sediment quality guidelines for the protection of aquatic life,” in Canadian environmental quality guidelines (Canadian Council of Ministers of the Environment, Winnipeg, MB).
Chen S. J., Gao X. J., Mai B. X., Chen Z. M., Luo X. J., Sheng G. Y., et al. (2006). Polybrominated diphenyl ethers in surface sediments of the Yangtze River Delta: levels, distribution and potential hydrodynamic influence. Environ. pollut. 144, 951–957. doi: 10.1016/j.envpol.2006.01.044
Cheng C., Hu T., Liu W., Mao Y., Shi M., Xu A., et al. (2021). Modern lake sedimentary record of PAHs and OCPs in a typical karst wetland, south China: response to human activities and environmental changes. Environ. pollut. 291, 118173. doi: 10.1016/j.envpol.2021.118173
Chopra A. K., Sharma M. K., Chamoli S. (2011). Bioaccumulation of organochlorine pesticides in aquatic system—an overview. Environ. Monit. Assess. 173, 905–916. doi: 10.1007/s10661-010-1433-4
Gai N., Pan J., Tang H., Tan K. Y., Chen D. Z., Zhu X. H., et al. (2014). Selected organochlorine pesticides and polychlorinated biphenyls in atmosphere at Ruoergai high altitude prairie in eastern edge of Qinghai-Tibet Plateau and their source identifications. Atmospheric Environ. 95, 89–95. doi: 10.1016/j.atmosenv.2014.06.005
Guo F., Yin S., Wang H., Zhang J., Liu Y., Aamir M., et al. (2021). Polychlorinated biphenyls (PCBs) in the colostrum samples from the Yangtze River Region: Exposure profile and risk assessment. Environ. pollut. 285, 117253. doi: 10.1016/j.envpol.2021.117253
Handl G. (2012). Declaration of the United Nations conference on the human environment (Stockholm Declaration), 1972 and the Rio Declaration on Environment and Developmen. United Nations Audiovisual Library Int. Law 11, 1–11.
Hassaan M. A., Elkatory M. R., Ragab S., El Nemr A. (2023). Polychlorinated biphenyls (PCBs) and organochlorine pesticides (OCPs) in water-sediment system of southern Mediterranean: Concentration, source and ecological risk assessment. Mar. pollut. Bull. 196, 115692. doi: 10.1016/j.marpolbul.2023.115692
He S., Cao L., Wang Y., Dong C., Lin C. (2013). Distribution and sources of organochlorine pesticides in the surface soils of the central Jianghan Plain. Environ. Sci. Technol. 36, 75–79. doi: 10.3969/j.issn.1003-6504.2013.02.016
Hidayati N. V., Asia L., Khabouchi I., Torre F., Widowati I., Sabdono A., et al. (2021). Ecological risk assessment of persistent organic pollutants (POPs) in surface sediments from aquaculture system. Chemosphere 263, 128372. doi: 10.1016/j.chemosphere.2020.128372
Hu C., Tao Y. (2023). Spatial–temporal occurrence and sources of organochlorine pesticides in the sediments of the largest deep lake (Lake Fuxian) in China. Environ. Sci. pollut. Res. 30, 31157–31170. doi: 10.1007/s11356-022-24394-7
Hu L. M., Lin T., Shi X. F., Yang Z. S., Wang H. J., Zhang G., et al. (2011). The role of shelf mud depositional process and large river inputs on the fate of organochlorine pesticides in sediments of the Yellow and East China seas. Geophysical Res. Lett. 38. doi: 10.1029/2010GL045723
Hu M., Cui Z., Wu Z., Liu W., Jia L. (2022). Spatial differences and formation conditions of beach mud blackening in Sanya Bay. Mar. Geology Front. 07, 23–30. doi: 10.16028/j.1009-2722.2021.078
Huang W., He Y., Xiao J., Huang Y., Li A., He M., et al. (2019b). Risk of breast cancer and adipose tissue concentrations of polychlorinated biphenyls and organochlorine pesticides: a hospital-based case-control study in Chinese women. Environ. Sci. Pollut. Res. 26, 32128–32136. doi: 10.1007/s11356-019-06404-3
Huang Y., Li J., Yang Y., Yuan H., Wei Q., Liu X., et al. (2019a). Characterization of enzyme-immobilized catalytic support and its exploitation for the degradation of methoxychlor in simulated polluted soils. Environ. Sci. Pollut. Res. 26, 28328–28340. doi: 10.1007/s11356-019-05937-x
Kang Y., Zhang R., Yu K., Han M., Pei J., Chen Z., et al. (2022). Organochlorine pesticides (OCPs) in corals and plankton from a coastal coral reef ecosystem, south China sea. Environ. Res. 214, 114060. doi: 10.1016/j.envres.2022.114060
Kuranchie-Mensah H., Atiemo S. M., Palm L. M. N. D., Blankson-Arthur S., Tutu A. O., Fosu P. (2012). Determination of organochlorine pesticide residue in sediment and water from the Densu river basin, Ghana. Chemosphere 86, 286–292. doi: 10.1016/j.chemosphere.2011.10.031
Lan J., Jia J., Liu A., Yu Z., Zhao Z. (2019). Pollution levels of banned and non-banned pesticides in surface sediments from the East China Sea. Mar. pollut. Bull. 139, 332–338. doi: 10.1016/j.marpolbul.2019.01.006
Li H., Jiang W., Pan Y., Li F., Wang C., Tian H. (2021). Occurrence and partition of organochlorine pesticides (OCPs) in water, sediment, and organisms from the eastern sea area of Shandong Peninsula, Yellow Sea, China. Mar. pollut. Bull. 162, 111906. doi: 10.1016/j.marpolbul.2020.111906
Li X., Jiang S., Zheng H., Shi Y., Cai M., Cai Y. (2023). Organophosphorus pesticides in southeastern China marginal seas: Land-based export and ocean currents redistribution. Sci. Total Environ. 858, 160011. doi: 10.1016/j.scitotenv.2022.160011
Li J., Li F., Liu Q. (2015). Sources, concentrations and risk factors of organochlorine pesticides in soil, water and sediment in the Yellow River estuary. Mar. pollut. Bull. 100, 516–522. doi: 10.1016/j.marpolbul.2015.09.003
Liu J., Qi S., Yao J., Yang D., Xing X., Liu H., et al. (2016). Contamination characteristics of organochlorine pesticides in multimatrix sampling of the Hanjiang River Basin, southeast China. Chemosphere 163, 35–43. doi: 10.1016/j.chemosphere.2016.07.040
Lyall K., Croen L. A., Sjödin A., Yoshida C. K., Zerbo O., Kharrazi M., et al. (2017). Polychlorinated biphenyl and organochlorine pesticide concentrations in maternal mid-pregnancy serum samples: association with autism spectrum disorder and intellectual disability. Environ. Health Perspect. 125, 474–480. doi: 10.1289/EHP277
Ma J., Pan L. B., Yang X. Y., Liu X. L., Tao S. Y., Zhao L., et al. (2016). DDT, DDD, and DDE in soil of Xiangfen County, China: residues, sources, spatial distribution, and health risks. Chemosphere 163, 578–583. doi: 10.1016/j.chemosphere.2016.08.050
MacDonald D. D., Ingersoll C. G., Berger T. A. (2000). Development and evaluation of consensus-based sediment quality guidelines for freshwater ecosystems. Arch. Environ. contamination Toxicol. 39, 20–31. doi: 10.1007/s002440010075
Mo L., Zheng J., Wang T., Shi Y. G., Chen B. J., Liu B., et al. (2019). Legacy and emerging contaminants in coastal surface sediments around Hainan Island in South China. Chemosphere 215, 133–141. doi: 10.1016/j.chemosphere.2018.10.022
NOAA (National Oceanic and Atmospheric Administration) (1999). Screening quick reference tables (SquiRTs). Available online at: http://response.restoration.noaa.gov/cpr/sediment/squirt/squirt.html.
Nyihirani F., Qu C., Yuan Z., Zhang Y., Mbululo Y., Janneh M., et al. (2022). Level, source, and distribution of organochlorine pesticides (OCPs) in agricultural soils of Tanzania. Environ. Monit. Assess. 194, 1–20. doi: 10.1007/s10661-021-09631-9
Oliveira A. H., Cavalcante R. M., Duaví W. C., Fernandes G. M., Nascimento R. F., Queiroz M. E., et al. (2016). The legacy of organochlorine pesticide usage in a tropical semi-arid region (Jaguaribe River, Ceará, Brazil): implications of the influence of sediment parameters on occurrence, distribution and fate. Sci. Total Environ. 542, 254–263. doi: 10.1016/j.scitotenv.2015.10.058
Peng S., Kong D., Li L., Zou C., Chen F., Li M., et al. (2020). Distribution and sources of DDT and its metabolites in porewater and sediment from a typical tropical bay in the South China Sea. Environ. pollut. 267, 115492. doi: 10.1016/j.envpol.2020.115492
Qiu Y. W., Li J., Zhao M. X., Yu K. F., Zhang G. (2024). The emerging and legacy persistent organic contaminants in corals of the South China Sea. Chemosphere 359, 142324. doi: 10.1016/j.chemosphere.2024.142324
Qiu Y. W., Qiu H. L., Zhang G., Li J. (2019). Bioaccumulation and cycling of organochlorine pesticides (OCPs) and polychlorinated biphenyls (PCBs) in three mangrove reserves of south China. Chemosphere 217, 195–203. doi: 10.1016/j.chemosphere.2018.10.188
Qiu X., Zhu T., Yao B., Hu J., Hu S. (2005). Contribution of dicofol to the current DDT pollution in China. Environ. Sci. Technol. 39, 4385–4390. doi: 10.1021/es050342a
Said T. O., Ragab S., El Sikaily A., Hassaan M. A., Arshad M., El Nemr A. (2023). Chlorinated pesticides and PCB residues in the Egyptian Western Desert oases sediments. Mar. pollut. Bull. 193, 115236. doi: 10.1016/j.marpolbul.2023.115236
Shi J., Li P., Li Y., Liu W., Zheng G. J. S., Xiang L., et al. (2016). Polychlorinated biphenyls and organochlorine pesticides in surface sediments from Shantou Bay, China: sources, seasonal variations and inventories. Mar. pollut. Bull. 113, 585–591. doi: 10.1016/j.marpolbul.2016.09.006
Sim W., Nam A., Lee M., Oh J. E. (2023). Polychlorinated biphenyls and organochlorine pesticides in surface sediments from river networks, South Korea: Spatial distribution, source identification, and ecological risks. Environ. Sci. pollut. Res. 30, 94371–94385. doi: 10.1007/s11356-023-28973-0
Sreedevi M. A., Harikumar P. S. (2023). Occurrence, distribution, and ecological risk of heavy metals and persistent organic pollutants (OCPs, PCBs, and PAHs) in surface sediments of the Ashtamudi wetland, south-west coast of India. Regional Stud. Mar. Sci. 64, 103044. doi: 10.1016/j.rsma.2023.103044
Strandberg B. O., van Bavel B., Bergqvist P. A., Broman D., Ishaq R., Näf C., et al. (1998). Occurrence, sedimentation, and spatial variations of organochlorine contaminants in settling particulate matter and sediments in the northern part of the Baltic Sea. Environ. Sci. Technol. 32, 1754–1759. doi: 10.1021/es970789m
Sun J., Feng J., Liu Q., Li Q. (2010). Distribution and sources of organochlorine pesticides (OCPs) in sediments from upper reach of Huaihe River, East China. J. Hazardous Materials 184, 141–146. doi: 10.1016/j.jhazmat.2010.08.016
Tian Y. Z., Li W. H., Shi G. L., Feng Y. C., Wang Y. Q. (2013). Relationships between PAHs and PCBs, and quantitative source apportionment of PAHs toxicity in sediments from Fenhe reservoir and watershed. J. Hazardous Materials 248, 89–96. doi: 10.1016/j.jhazmat.2012.12.054
Wacławek S., Silvestri D., Hrabák P., Padil V. V., Torres-Mendieta R., Wacławek M., et al. (2019). Chemical oxidation and reduction of hexachlorocyclohexanes: A review. Water Res. 162, 302–319. doi: 10.1016/j.watres.2019.06.072
Wang W., Bai J., Zhang G., Wang X., Jia J., Cui B., et al. (2017). Depth-distribution, possible sources, and toxic risk assessment of organochlorine pesticides (OCPs) in different river sediment cores affected by urbanization and reclamation in a Chinese delta. Environ. pollut. 230, 1062–1072. doi: 10.1016/j.envpol.2017.06.068
Wang X., Zhang Z., Zhang R., Huang W., Dou W., You J., et al. (2022). Occurrence, source, and ecological risk assessment of organochlorine pesticides and polychlorinated biphenyls in the water–sediment system of Hangzhou Bay and East China Sea. Mar. pollut. Bull. 179, 113735. doi: 10.1016/j.marpolbul.2022.113735
Wania F., Mackay D. (1996). Peer reviewed: tracking the distribution of persistent organic pollutants. Environ. Sci. Technol. 30, 390A–396A. doi: 10.1021/es962399q
Wurl O., Obbard J. P. (2005). Organochlorine pesticides, polychlorinated biphenyls and polybrominated diphenyl ethers in Singapore’s coastal marine sediments. Chemosphere 58, 925–933. doi: 10.1016/j.chemosphere.2004.09.054
Xu L., Granger C., Dong H., Mao Y., Duan S., Li J., et al. (2020). Occurrences of 29 pesticides in the Huangpu River, China: Highest ecological risk identified in Shanghai metropolitan area. Chemosphere 251, 126411. doi: 10.1016/j.chemosphere.2020.126411
Zafarani G. G., Karbalaei S., Golshani R., Pustokhina I., Walker T. R. (2022). Baseline occurrence, distribution and sources of PAHs, TPH, and OCPs in surface sediments in Gorgan Bay, Iran. Mar. pollut. Bull. 175, 113346. doi: 10.1016/j.marpolbul.2022.113346
Zeng F., Yang D., Xing X., Qi S. (2017). Evaluation of Bayesian approaches to identify DDT source contributions to soils in Southeast China. Chemosphere 176, 32–38. doi: 10.1016/j.chemosphere.2017.02.049
Keywords: contamination characteristics, tourist city, surface sediments, source analysis, ecological risk
Citation: Wang H, Zhang L, Yang F, Yan L, Lin C and Shen C (2025) Characteristics, source analysis, and risk assessment of organochlorine pesticide contamination in nearshore surface sediments of a tropical tourist island. Front. Mar. Sci. 11:1513515. doi: 10.3389/fmars.2024.1513515
Received: 18 October 2024; Accepted: 11 December 2024;
Published: 09 January 2025.
Edited by:
Lixin Pei, The University of Melbourne, AustraliaReviewed by:
Edison Barbieri, Agência de Agronegócio e Tecnologia de São Paulo (APTA), BrazilWenjing Lin, Chinese Academy of Geological Sciences, China
Copyright © 2025 Wang, Zhang, Yang, Yan, Lin and Shen. This is an open-access article distributed under the terms of the Creative Commons Attribution License (CC BY). The use, distribution or reproduction in other forums is permitted, provided the original author(s) and the copyright owner(s) are credited and that the original publication in this journal is cited, in accordance with accepted academic practice. No use, distribution or reproduction is permitted which does not comply with these terms.
*Correspondence: Lin Zhang, emxpbl9hQG1haWwuY2dzLmdvdi5jbg==; Feng Yang, bGlrZXlhbmdmZkAxMjYuY29t