- 1Chesapeake Biological Laboratory, University of Maryland Center for Environmental Science, Solomons, MD, United States
- 2Risk Assessment Research Center, Korea Institute of Ocean Science and Technology, Geoje, Republic of Korea
- 3Department of Ocean Science, University of Science and Technology, Daejeon, Republic of Korea
The widespread use of polymers across various industries has led to significant microplastic pollution in marine environments, with millions of tons of microplastics being released annually. This study examines the contribution of coatings particles released from commercial ships, to marine microplastic pollution. Key sources of these microplastics include the weathering and abrasion of coatings during ship maintenance activities. Marine coatings, which are rich in polymers such as polyurethanes and epoxies, are released into the ocean through processes like normal wear and tear, damage, in-water cleaning, and removal of old paint layers. Our research indicates that the global shipping sector could be a substantial contributor to microplastic pollution, potentially releasing thousands of tons annually. Predictive modeling identifies bulk carriers as the largest contributors, followed by tankers, containerships, and cargo vessels. This study also finds that manual biofouling cleaning by divers generates more microplastics than cleaning using mechanized in-water cleaning (IWC) systems with debris capture. Mitigation strategies, such as alternative cleaning methods and improved waste capture and processing, are proposed, but their effectiveness remains uncertain due to implementation challenges. A multidisciplinary approach and coordinated global efforts are essential to develop effective strategies for reducing microplastic pollution from ship coatings and protecting marine ecosystems.
1 Introduction
Current global estimates suggest that millions of tons of microplastics are released into marine ecosystems annually (Boucher and Friot, 2017; Paruta et al., 2022), posing significant threats to biodiversity and ecosystem health. These primary sources of ocean microplastics include pellets or particles from: (a) manufacturing, transport, and recycling processes; (b) synthetic textiles shed by laundry abrasion; (c) tire wear and abrasion; (d) weathered road markings and coatings; (e) residues from personal care products; and (f) urban dust generated by various processes (Boucher and Friot, 2017). While terrestrial sources are commonly regarded as the major contributors, ocean-based activities such as shipping, aquaculture, and fisheries also significantly contribute to direct ocean microplastic release (Peng et al., 2022). Among these, microplastics associated with marine coatings can range from a few hundred to millions of tons per year (Verschoor et al., 2016; Boucher and Friot, 2017; Hahn et al., 2018; Galafassi et al., 2019; Paruta et al., 2022; Kim et al., 2024b).
Paint or coating particles are significant contributors to microplastic pollution due to their polymer backbones, which serve as binding agents. These polymers include alkyls, epoxies, polyurethanes (PU), polyesters, polyacrylates, and polystyrenes (PS) (Gaylarde et al., 2021). Microplastics from marine coatings are released through various pathways, including normal wear, unintended paint damage, removal of old paint layers during maintenance activities like sanding and abrasion, and rinsing of painting tools (Tamburri et al., 2022). Furthermore, recent studies suggest that additives in coatings, such as antifouling metals, booster biocides, pigments, and corrosion inhibitors, increase the persistence and environmental impacts of paint particles, posing a threat to marine life and food webs (Singh and Turner, 2009; Gade et al., 2012; Soroldoni et al., 2017, 2020; Soon et al., 2021a; Soon et al., 2021b; Soon et al., 2023). Although microplastic release from ship coatings has been previously considered (e.g., Tamburri et al., 2022), an assessment of possible microplastic loads associated with common ship maintenance activities has not been conducted.
Biofouling on ship surfaces negatively impacts vessel performance and fuel efficiency, and directly contributes to increased exhaust emissions and the spread of non-indigenous species (NIS) (e.g., Tamburri et al., 2020). Anti-fouling coatings, the primary strategy for managing biofouling, typically last about five years but do not prevent biofouling on all surfaces, particularly as the coatings age, during prolonged stationary periods, or on surfaces unsuitable for coating (Tamburri et al., 2021; ACT/MERC, 2022). To address these limitations, many commercial ships employ in-water cleaning (IWC) to actively remove biofouling, thereby improving operational efficiency and reducing the risk of transporting NIS (Scianni and Georgiades, 2019; Tamburri et al., 2020; Scianni et al., 2023). While IWC is essential for maintaining vessel efficiency and performance, it can inadvertently release coating particles into the surrounding water (Tamburri et al., 2021; Lim et al., 2023). The abrasive action of cleaning tools, such as brushes and water jets, can dislodge small fragments of paint (USEPA, 2011; Tamburri et al., 2021). These small particles can remain suspended in the water and may be transported over long distances by currents, amplifying their impact on marine ecosystems (Muller-Karanassos et al., 2019; Turner et al., 2022).
Though IWC has been known to abrade antifouling coatings, releasing contaminants into the marine environment (e.g., Valkirs et al., 2003; Inglis et al., 2012; Morrisey et al., 2013; Earley et al., 2014), its contribution to ocean microplastic pollution remains poorly understood (Tamburri et al., 2022). Studies indicate that IWC can release substantial quantities of particles into coastal and open ocean waters, ranging from 23.8 to 267.3 mg/L of suspended solids (Soon et al., 2021b, 2023). These particles predominantly consist of coating fragments dislodged during the cleaning process. The amount of particles released during IWC is influenced by several factors, including the frequency of cleaning, the type and condition of antifouling paints, and the specific techniques used (Krutwa et al., 2019). The mechanical removal of biofouling can generate particles ranging from nanometers to several millimeters in size. Moreover, the accumulation of coating layers on ship surfaces over time, due to continuous painting without proper removal, can lead to higher release rates during cleaning, as aged and degraded paint layers are more easily detached (Oliveira and Granhag, 2020). These polymer-rich particles can persist in the environment, posing risks to marine life through direct exposure and ingestion (Soroldoni et al., 2020). Additionally, IWC systems themselves may serve as a source of microplastic, with the most obvious being the wearing of nylon brushes (Tamburri et al., 2021).
This study aims to better understand the multifaceted dynamics of microplastic release from IWC of ship coatings. Through initial examinations of IWC processes, this study helps to elucidate the intricate pathways through which ship coatings contribute to microplastic pollution and provides preliminary estimates of microplastic release from ships, thereby laying a foundation for targeted mitigation strategies.
2 Method
In this study, data from previous research were collected and analyzed to estimate the total release of plastics resulting from IWC activities on ships. The dataset comprised three primary components: total suspended solids (TSS) release rates from Soon et al. (2021b, 2023), the estimated number of ships from the United Nations Conference on Trade and Development (UNCTAD, 2023), and the mean wetted surface area (WSA) by ship type, as reported by Moser et al. (2016).
The release rate refers to the release of materials from the specific unit area of a ship’s hull during IWC, which was captured as wastewater during sample collection. These data were utilized to calculate the total plastic release associated with IWC activities.
The microplastic release rate was determined using the following formula:
a: Proportion of paint particle. This formula assumes that the majority of total suspended solids (TSS) in wastewater is coating particles released during IWC, given the type of biofouling that was removed (i.e., soft biofilms and occasional light macroalgae). Other sources of TSS (i.e., ambient water particulates) will be present, but their contribution is likely relatively small in this context. Therefore, release estimates were generated using two coating particles proportion of TSS: (a) 50% at the likely lower end, and (b) 100% as a worst-case scenario. This formula is also based on the definition of microplastic put forward by Tamburri et al. (2022), which states that coating-associated microplastics are any particle containing ≥10% polymer content (i.e., all current marine coatings, which have polymer concentrations ranging from 10-90%), with the entire mass of the particle considered a microplastic. This is also consistent with Kim et al. (2024b) who found that paint particles produced during hull cleaning consist of 97.9% acrylate.
The total plastic release due to IWC was then calculated using the equation:
3 Results – case studies
Analyses of particle release from IWC activities can provide valuable insights into microplastic pollution resulting from ship biofouling management. While several new proactive and reactive IWC systems (both with and without debris capture options) have become available in recent years (e.g., Scianni and Georgiades, 2019; Tamburri et al., 2021), the great majority of ship IWC currently being carried out around the world is still done by divers with handheld cleaning devices. Previous studies have sampled and analyzed particulate matter from two types of IWC processes: (a)manual handheld cleaning by a diver using a brush (bristles made of nylon, 25 mm × 1 mm Ø, 30 bristles/cm2), and (b) commercially available mechanized remotely operated vehicles (ROV) equipped with rotating brushes and a suction debris collection and filtration system. In the diver-based manual cleaning process, quadrats (50 cm × 50 cm) were used to estimate the cleaned area, and samples of released debris were collected by a hose and pumped to shore. The TSS release rate from the study ranged from 12.9 to 37.5 g/m2. The ROV-based IWC system involved a suction debris capture approach where wastewater passed through a filtration system to remove particles of various sizes. Samples were collected both before and after the physical separation of particles on the ROV-based IWC system, with TSS release rates ranging from 1.64 to 3.29 g/m2 and 0.38 to 0.84 g/m2, respectively. Detailed sample collection methodology is available in Soon et al. (2021a, 2023).
Despite efforts to minimize particle emissions from ship biofouling IWC, studies consistently found particulate matter in all effluent samples, with red paint particles being the predominant component (Soon et al., 2021a, 2023). An analysis of total suspended solids (TSS) in wastewater from IWC trials revealed higher TSS release rates during diver-based scrubbing (ranging from 12.9 to 37.5 g/m2) compared to ROV-based cleaning (ranging from 1.64 to 3.29 g/m2) (Table 1). This difference is likely due to the more intense cleaning performed by divers compared to the broader, less concentrated cleaning by ROVs. ROV-based cleaning with a debris capture and processing system demonstrated even lower TSS release rates, ranging from 0.38 to 0.84 g/m2 (Table 2).
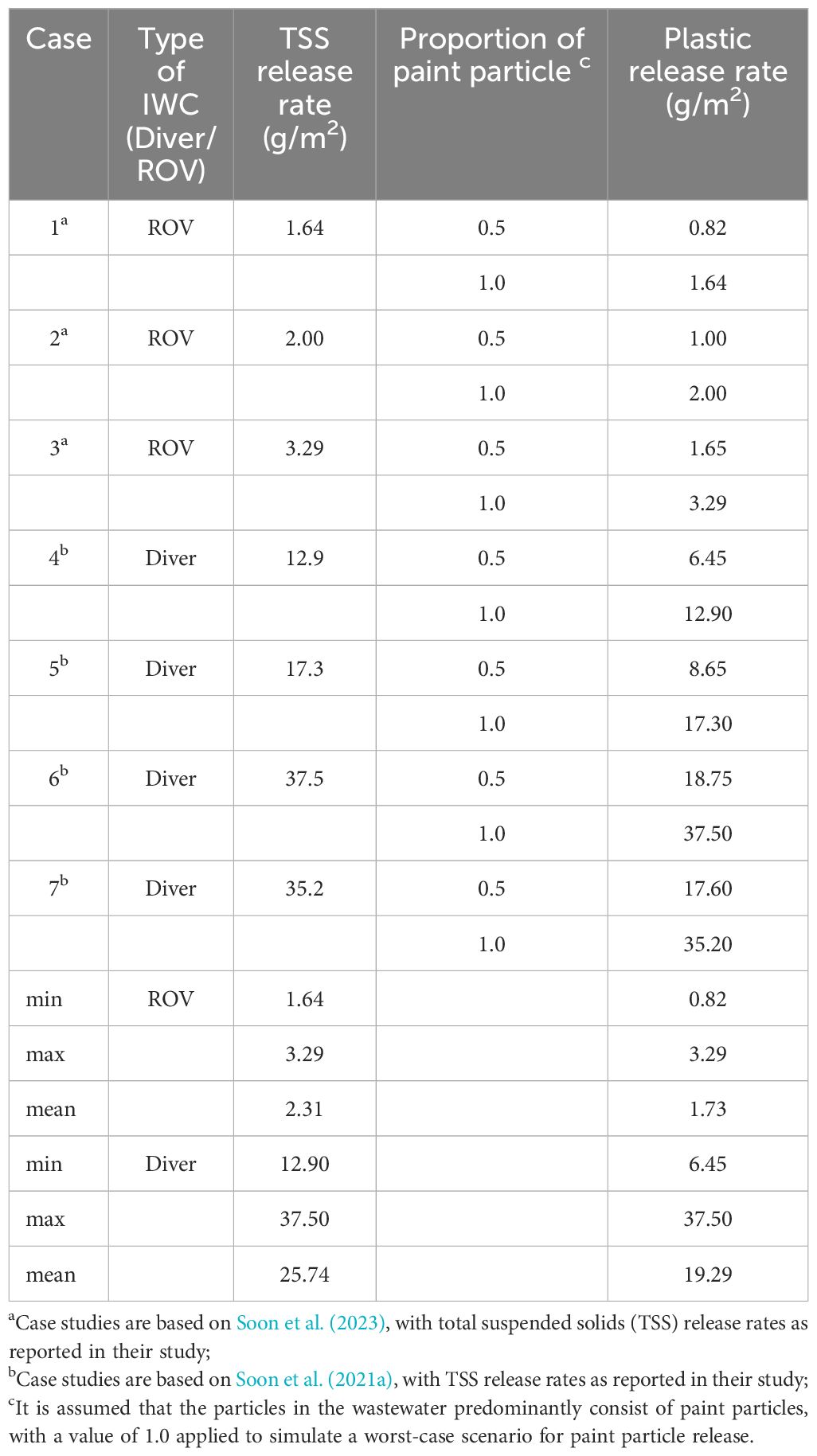
Table 1. Release rates of plastics from ship paint during in-water cleaning (IWC) without effluent treatments.
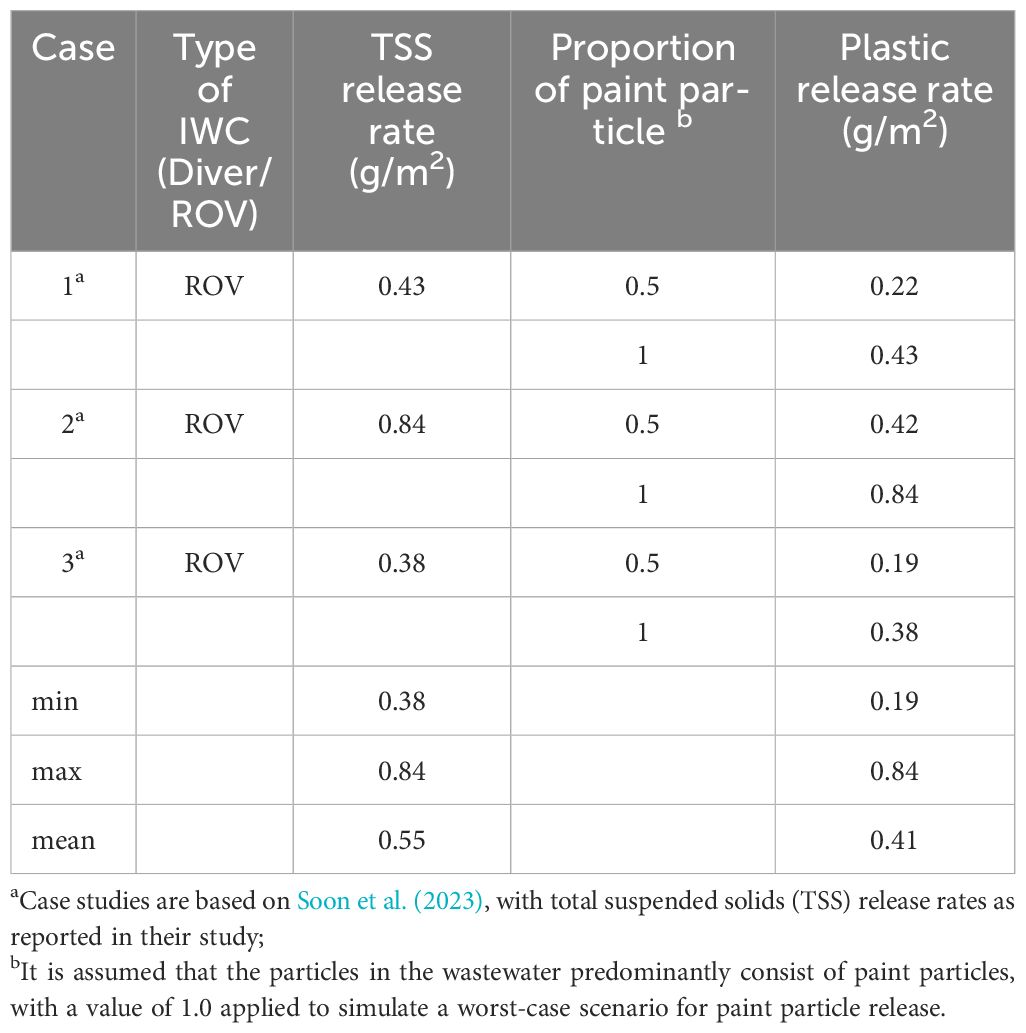
Table 2. Release rates of plastics from ship paint during in-water cleaning (IWC) with effluent treatments.
Visual examination of samples indicated distinct red-colored coating particles mixed with seaweed in influent IWC samples, while effluent samples primarily contained red paint particles (Figure 1). Given the prevalence of red paint particles in effluent solids, the release rate of these particles can be quantified based on the TSS release rates. However, TSS also often includes particulate matter other than paint particles, such as plankton, detritus, and fine sediments. To refine the estimates of paint particles, we adjusted for organic matter contributions using a conversion factor for coating particles: 50% of TSS for the lower-end estimate and 100% for the worst-case scenario (Tables 1, 2). We then estimated the plastic release rate (g/m2) using the formula outlined above in the method section. This approach allows us to approximate the release rates of coating-derived microplastics from each cleaning event, including those with debris capture and treatment systems, as shown in Tables 1 and 2.
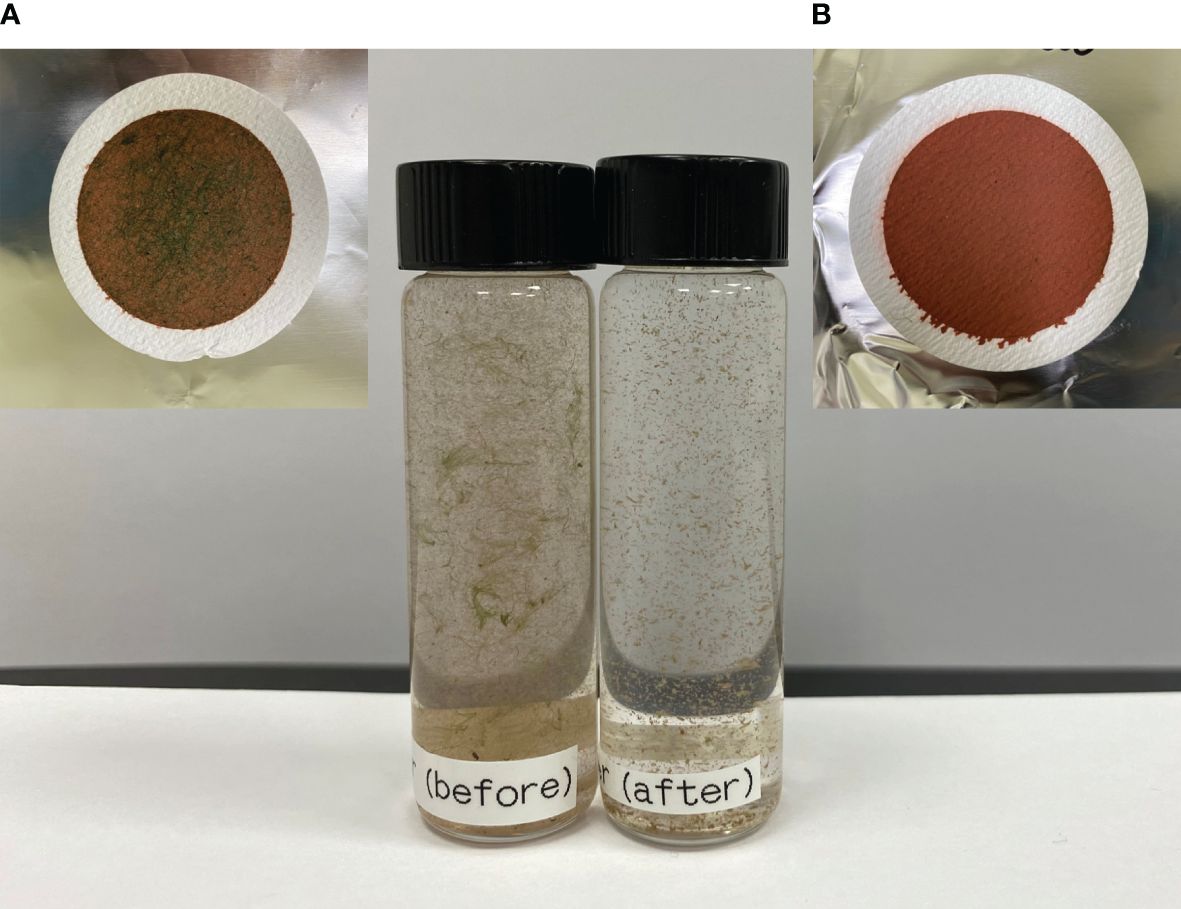
Figure 1. In-water cleaning (IWC) effluent samples before (A) and after (B) debris treatment. The pre-treatment sample (left) shows dispersed red paint particles mixed with seaweed, while the post-treatment sample (right) predominantly contains red paint particles.
Based on our predictive modeling, the estimated minimum microplastic release rates from IWC conducted by ROV (with rotating brush and debris capture) and by diver (scrubbing with a handheld brush) are 0.82 g/m2 and 6.45 g/m2, respectively. However, in worst-case scenarios, the release rates from IWC by ROV and diver could reach 3.29 g/m2 and 37.50 g/m2, respectively, with rates stabilizing at 0.84 g/m2 after effluent treatment. Not surprisingly, on average, IWC conducted by divers shows a higher mean release rate of 19.29 g/m2 compared to ROV-based cleaning, which averages 1.73 g/m2. After the IWC system’s physical separation of particles, these average release rates decrease substantially to 0.41 g/m2.
These estimated release rates enable predictions of total plastic emissions from various ship types, using data from vessels registered with the United Nations Conference on Trade and Development (UNCTAD, 2023). According to UNCTAD (2023), the global fleet consists of 13,149 bulkers, 11,778 tankers, 5,823 containerships, 20,397 general cargo vessels, and 54,248 other vessels. By applying the proposed wetted surface area (WSA) for each ship type, as reported by Moser et al. (2016), we calculated the total WSA of all vessels and, consequently, the potential global emission of ship paint-derived microplastics from IWC, assuming one IWC operation per vessel per year (Table 3). Although this simple multiplication may not accurately represent actual annual emissions, these estimates provide insights into the potential contribution of IWC to marine microplastic pollution and the impact of different cleaning and capture methods.
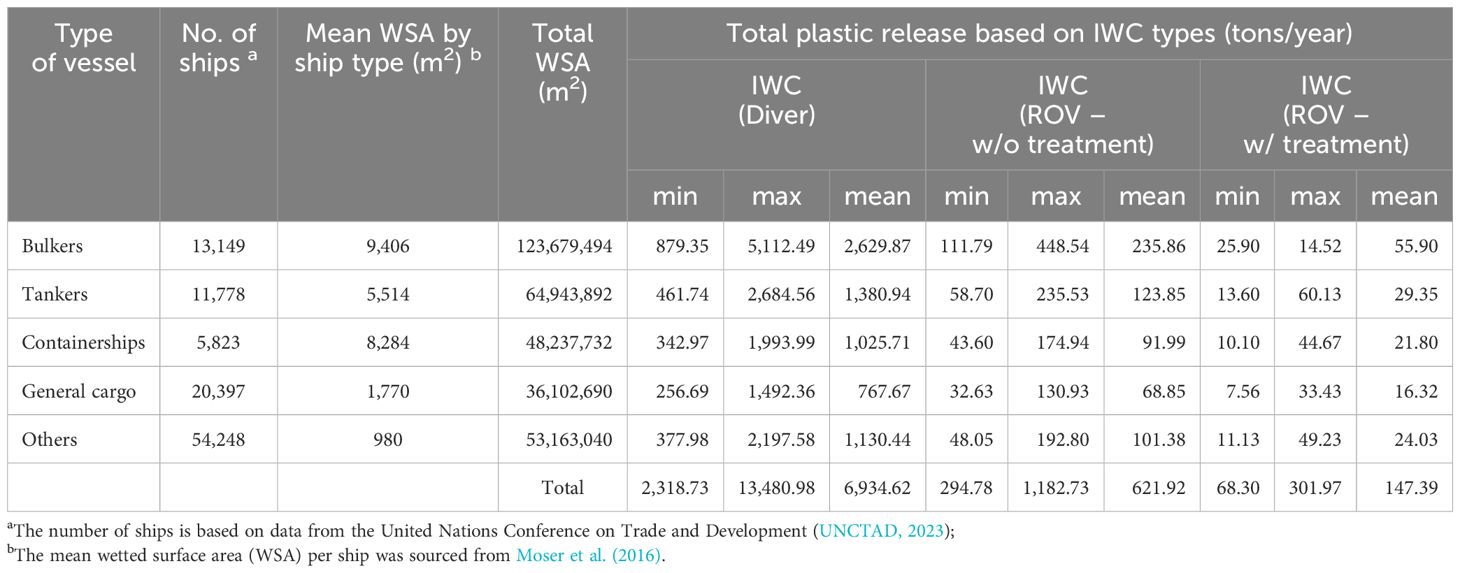
Table 3. Estimated total plastic release from in-water cleaning (IWC) of all vessels worldwide per year, based on representative wetted surface areas (WSA) and the number of vessels by type.
The total plastic release from IWC of all vessels worldwide – conducted manually by divers, using an ROV-based system without capture, and with an ROV-based system with capture and debris processing – ranges from approximately 2,319 to 13,481 tons/yr, 295 to 1,183 tons/yr, and 68 to 302 tons/yr, respectively. Notably, bulkers would contribute the most to plastic release due to their larger WSA compared to other vessel types, with manual IWC conducted by divers resulting in the highest average release of approximately 2,630 tons/yr. Tankers would follow closely behind, with an average release of 1,381 tons/yr if cleaned by divers. While transitioning to more mechanized and automated cleaning with capture approaches could reduce the average release by more than 90%, the total plastic release could still be substantial at around 623 tons/yr, with further reductions after debris processing, dropping to approximately 147 tons/yr. These estimates underscore the potential for microplastic release from ship IWC and are valuable for developing targeted strategies to mitigate ocean microplastic pollution. However, effective mitigation must also consider factors beyond coating release during cleaning.
4 Discussion
4.1 Microplastic release by coatings and cleanings
To effectively control biofouling on ship surfaces, a comprehensive antifouling strategy integrates both chemical and physical approaches to optimize performance (Wezenbeek et al., 2018; Xie et al., 2019; Culver et al., 2021). Different types of ships employ various antifouling coatings and hull husbandry practices to prevent or mitigate biofouling accumulation. However, these methods can sometimes reduce antifouling effectiveness and increase environmental risks. For instance, IWC of ships may release propagules and viable fragments of biofouling organisms (Hopkins and Forrest, 2008; Kim et al., 2024a) and increase the leaching of coating material (Schiff et al., 2004; Turner, 2010; Ralston, 2023) or antifouling paint particles (APPs), which can continue to release biocides into the environment (Almeida et al., 2023). Although some assessments of IWC capture efficiency exist, published results are scarce and limited and no capture system would be 100% effective at preventing the release of removed debris (Davidson et al., 2008; Hopkins et al., 2010; Tamburri et al., 2020).
Marine coatings contain 10-90% polymers (e.g., polyurethanes, epoxies, acrylics), potentially introducing microplastics during hull cleaning (IMO, 2019; Tamburri et al., 2022; Kim et al., 2024b). Factors such as ship operations, environmental conditions, the condition and age of the paint layers, and the type of coatings, can all influence the magnitude of microplastic release. Older, weathered, degraded, or damaged coatings are more likely to shed microplastic particles compared to newer coatings (Tamburri et al., 2022). Additionally, the adhesion of biofouling organisms like barnacles and macroalgae can contribute to increased microplastic release by adding mechanical forces during cleaning (Oliveira and Granhag, 2016).
4.2 Contribution of IWC brush wearing
Brushes are the most common approach used for IWC of ship biofouling, typically crafted from synthetic materials such as nylon or polypropylene, and have the potential to shed small plastic fibers during the cleaning process. Moreover, wire brushes used for severe hard-fouling, add complexity as they are more aggressive and can wear down both the bristles and hull coatings, potentially releasing larger coating chips as microplastics. The size, strength, and flexibility of the bristles play a critical role in how effectively and safely the brushes can remove biofouling without causing excessive damage to the hull or producing harmful debris.
As these brush bristles come into contact with the hull, friction and abrasion occur, leading to the detachment of minute fragments, especially at vulnerable points such as the tips. Additionally, prolonged exposure to environmental factors like sunlight and saltwater can further degrade the integrity of the brush fibers, increasing the likelihood of their fragmentation into microplastic particles over time. This degradation not only affects the brushes’ performance but also raises environmental concerns due to the potential release of microplastics into coastal marine ecosystems.
As highlighted by Tamburri et al. (2022), it is important to address the issue of brush-associated microplastics by implementing effective capture and disposal systems during IWC operations. Research into alternative materials, such as biodegradable or recycled options, could also help reduce microplastic shedding. Furthermore, the establishment of regulations and industry standards governing brush materials and cleaning practices can help mitigate the release of microplastics into marine environments. For instance, implementing periodic replacement schedules for brushes can minimize wear and tear, thereby reducing the potential for microplastic emissions. Further research is needed to quantify the amount of microplastics released from IWC brushes and to develop mitigation strategies.
4.3 Impact of IWC frequency/rate and environmental factors
The frequency of IWC activities for ships at a given location or on specific vessels significantly influences microplastic release and its environmental impacts. Globally, an increase in IWC operations can lead to higher microplastic emissions, particularly in major shipping routes and ports. At the port level, the impact of IWC on microplastic release varies depending on factors such as ship traffic volume, port infrastructure, and environmental conditions. Ports with high levels of maritime activity and poor water circulation would be more susceptible to microplastic pollution.
The amount of microplastics released from IWC on individual ships depend on a complex set of interacting variables, including (a) the type, age, and condition of coating; (b) the type, extent, and level of biofouling; (c) the method of IWC employed (e.g., proactive or reactive IWC, with or without debris capture, and the approach to debris processing); (d) the total area cleaned; and (e) the frequency of cleaning. Thus, a large bulk carrier may not necessarily release more microplastics than a small ferry if different IWC approaches are used. Even with identical coatings, a smaller vessel might release more microplastics if it undergoes more frequent cleaning.
However, microplastic coating particles found in ports do not always originate solely from local sources, as discussed by Brannstrom et al. (2023). Wind and water currents can transport particles, resulting in microplastics appearing in ports that may not directly reflect local emissions. For example, research by Vogy-Vincent et al. (2023) estimated that a significant portion of debris found on beaches in Seychelles and other remote islands in the western Indian Ocean originates from other countries. Additionally, locations with seasonal variability and diverse climate conditions may face complex challenges in addressing microplastic pollution. For instance, a recent report by Diaz-Jaramillo et al. (2023) found that increased rainfall and runoff during the wet season may transport more microplastics into ports, while calm conditions during the dry season may lead to slower dispersal of pollutants.
Regions can also differ in the types of coatings used, IWC practices, environmental awareness, and ship maintenance standards, which will further complicate the issue of microplastic pollution. Moreover, ports located near urban areas, industrial sites, or busy shipping lanes often have higher concentrations of microplastics. As noted by Diaz-Jaramillo et al. (2023), pollution from urban runoff, atmospheric deposition, or direct discharge into port waters can significantly impact the coastal environment, in terms of plastic debris loads and transport.
4.4 Assumptions, uncertainties, and limitations
The methods used to estimate microplastic emissions from IWC might not accurately reflect real-world conditions, as they rely on complex models with assumptions that introduce uncertainties. Factors such as cleaning efficiency, coating degradation, and environmental dynamics might be overlooked in these estimations. Additionally, the interaction between microplastics and other environmental contaminants or organisms (e.g., microbes) could influence their fate and behavior, further complicating efforts to quantify emissions accurately (He et al., 2022). Acknowledging these uncertainties and limitations is critical for accurately interpreting the results and guiding future research efforts.
Moreover, the current data represent only the particulate matter collected, not the total amount produced during the cleaning processes. Quantifying IWC system capture efficiencies or rates under diverse and variable real-world conditions is challenging. It is known that far less than 100% of the material removed from submerged ship surfaces is collected and disposed of (Tamburri et al., 2021). Consequently, the estimates likely underrepresent the potential contribution of marine coatings to microplastic contamination in coastal waters, underscoring the need to address specific sources of pollution to mitigate their adverse effects on marine ecosystems.
Another source of uncertainty is the variability in paint compositions across different vessels and regions, which affects microplastic release estimates (Tribou and Swain, 2015). This variability introduces uncertainty into the estimation of microplastic releases, as different paint formulations may release varying amounts and types of microplastics during IWC. Without comprehensive data on the exact composition of paints used on individual vessels, it is challenging to accurately quantify the contribution of coatings to microplastic pollution.
While mitigation strategies, such as alternative brush materials and enhanced capture efficacy and debris processing, have been proposed to reduce the release of coating-derived microplastics into the environment, their effectiveness in practice remains uncertain. Challenges related to implementation, cost-effectiveness, and potential unintended consequences could impact the success of these strategies. Additionally, the long-term durability and performance of alternative materials or treatment systems under real-world conditions are not fully understood, leading to uncertainty about their ability to effectively mitigate microplastic pollution.
Regulatory and policy constraints also pose limitations on the effectiveness of mitigation strategies. Inconsistent regulations across jurisdictions, coupled with a lack of enforcement mechanisms and competing interests among stakeholders, can impede the implementation of measures aimed at reducing microplastic pollution from ship IWC. For instance, data collected from IWC operations could be a valuable resource for companies, but it is often not made publicly available, limiting transparency and accountability (Soon et al., 2023). Overcoming these barriers necessitates coordinated action at international, national, and local levels, which may be challenging due to divergent priorities and governance structures.
Addressing these uncertainties requires integrating multiple lines of evidence and considering the complexities of the marine environment. Detailed field studies, controlled experiments, and validation efforts are necessary to refine assumptions and enhance our understanding of microplastic pollution. Transparency about uncertainties enhances scientific rigor and credibility in addressing the global challenge of microplastic pollution.
5 Conclusion
This study highlights the potential role of IWC of ship biofouling in contributing to microplastic pollution, primarily from ship coatings. Although we can estimate microplastic release from IWC activities, further research is needed to accurately quantify the microplastic emissions from ship coatings and brushes, as well as to understand the impacts of cleaning frequency and environmental factors. Achieving this will require a multidisciplinary approach, incorporating field studies, controlled experiments, and advanced modeling. Overcoming regulatory constraints is also crucial for effective mitigation. Coordinated efforts at international, national, and local levels are essential to reduce microplastic emissions from ship hull cleaning and protect marine ecosystems.
Data availability statement
The original contributions presented in the study are included in the article/supplementary material. Further inquiries can be directed to the corresponding author.
Author contributions
ZS: Formal Analysis, Investigation, Methodology, Writing – original draft. MT: Funding acquisition, Supervision, Writing – review & editing. TK: Investigation, Methodology, Writing – original draft. MK: Conceptualization, Project administration, Writing – review & editing.
Funding
The author(s) declare financial support was received for the research, authorship, and/or publication of this article. This research was supported by Korea Institute of Marine Science & Technology Promotion (KIMST) funded by the Ministry of Oceans and Fisheries (‘Techniques development for management and evaluation of biofouling on ship hulls (RS-2021-KS211530)’ and ‘Land/sea-based input and fate of microplastics in the marine environment (RS-2022-KS221604)’). Additional support was provided by the US Department of Transportation’s Maritime Administration for ‘Investigating the Fate, Behavior, and Bioavailability of Microplastics Released from Ship Coatings’ (2023-693JF72150003).
Conflict of interest
The authors declare that the research was conducted in the absence of any commercial or financial relationships that could be construed as a potential conflict of interest.
Generative AI statement
The author(s) declare that no Generative AI was used in the creation of this manuscript.
Publisher’s note
All claims expressed in this article are solely those of the authors and do not necessarily represent those of their affiliated organizations, or those of the publisher, the editors and the reviewers. Any product that may be evaluated in this article, or claim that may be made by its manufacturer, is not guaranteed or endorsed by the publisher.
References
ACT/MERC (Alliance for Coastal Technologies/Maritime Environmental Resource Center) (2022). Guidelines for testing ship biofouling in-water cleaning systems. Available online at: https://www.maritime-enviro.org/Downloads/ACT_MERC_IWC_Syste_Testing_Guideline_2022.pdf (Accessed July, 2024). TS-788-22, CBL/UMCES 2023-017.
Almeida J. C., Castro I. B., Nunes B. Z., Zanardi-Lamardo E. (2023). Antifouling booster biocides in Latin America and the Caribbean: A 20-year review. Mar. pollut. Bull. 189, 114718. doi: 10.1016/j.marpolbul.2023.114718
Boucher J., Friot D. (2017). Primary Microplastics in the Oceans: A Global Evaluation of Sources (Gland, Switzerland: IUCN. International Union for Conservation of Nature and Natural Resources (IUCN). Available online at: https://portals.iucn.org/library/sites/library/files/documents/2017–002-En.pdf (Accessed July, 2024).
Brannstrom S., Rosengren H., Wrange A.-L., Olshammar M. (2023). Microplastic emissions from paint (Sweden: Swedish Meteorological and Hydrological Institute). Available online at: https://naturvardsverket.diva-portal.org/smash/get/diva2:1811470/FULLTEXT01.pdf (Accessed July, 2024).
Culver C. S., Johnson L. T., Page H. M., Dugan J. E., Santschi C. A. (2021). Integrated pest management for fouling organisms on boat hulls. Special Sect.: Integr. Pest Manage. 41, 301–328. doi: 10.1002/nafm.10360
Davidson I. C., McCann L. D., Sytsma M. D., Ruiz G. M. (2008). Interrupting a multispecies bioinvasion vector: the efficacy of in-water cleaning for removing biofouling on obsolete vessels. Mar. pollut. Bull. 56, 1538–1544. doi: 10.1016/j.marpolbul.2008.05.024
Diaz-Jaramillo M., Gonzalez M., Tomba J. P., Silva L. I., Islas M. S. (2023). Occurrence and dynamics of microplastics and emerging concern microparticles in coastal sediments: Impact of stormwater upgrade and port-associated facilities. Sci. Total. Environ. 899, 165724. doi: 10.1016/j.scitotenv.2023.165724
Earley P. J., Swope B. L., Barbeau K., Bundy R., McDonald J. A., Rivera-Duarte I. (2014). Life cycle contributions of copper from vessel painting and maintenance activities. Biofouling 30, 51–68. doi: 10.1080/08927014.2013.841891
Gade A. L., Heiaas H., Lillicrap A., Hylland K. (2012). Ecotoxicity of paint mixtures: Comparison between measured and calculated toxicity. Sci. Total. Environ. 435-436, 526–540. doi: 10.1016/j.scitotenv.2012.07.011
Galafassi S., Nizzetto L., Volta P. (2019). Plastic sources: A survey across scientific and grey literature of their inventory and relative contribution to microplastics pollution in natural environments, with an emphasis on surface water. Sci. Total. Environ. 693, 133499. doi: 10.1016/j.scitotenv.2019.07.305
Gaylarde C. C., Neto J. A. B., da Fonseca E. M. (2021). Paint fragments as polluting microplastics: A brief review. Mar. pollut. Bull. 162, 111847. doi: 10.1016/j.marpolbul.2020.111847
Hahn S., Kershaw P., Sherrington C., Bapasola A., Jamieson O., Cole G., et al. (2018). Investigating options for reducing releases in the aquatic environment of microplastics emitted by (but not intentionally added in) products. Report for DG Environment of the European Commission (Eunomia report, 23rd February 2018). Available online at: https://bmbf-plastik.de/sites/default/files/2018-04/microplastics_final_report_v5_full.pdf (Accessed July, 2024).
He S., Jia M., Xiang Y., Song B., Xiong W., Cao J., et al. (2022). Biofilm on microplastics in aqueous environment: Physicochemical properties and environmental implications. J. Hazard. Mater. 424, 127286. doi: 10.1016/j.jhazmat.2021.127286
Hopkins G. A., Forrest B. M. (2008). Management options for vessel hull fouling: An overview of risks posed by in-water cleaning. ICES J. Mar. Sci. 65, 811–815. doi: 10.1093/icesjms/fsn026
Hopkins G. A., Forrest B. M., Coutts A. D. M. (2010). The effectiveness of rotating brush devices for management of vessel hull fouling. Biofouling 26, 555–566. doi: 10.1080/08927014.2010.494330
IMO (International Maritime Organization) (2019). Hull scrapings and marine coatings as a source of microplastics (United Kingdom: London). Available online at: https://wwwcdn.imo.org/localresources/en/OurWork/Environment/Documents/Hull%20Scrapings%20final%20report.pdf (Accessed July, 2024).
Inglis G., Floerl O., Woods C. (2012). Scenarios of vessel biofouling risk and their management: An evaluation of options (Wellington: New Zealand: Ministry of Agriculture and Forestry). Available online at: https://mpi.govt.nz/dmsdocument/4029-scenarios-of-vessel-biofouling-risk-and-their-management (Accessed July, 2024).
Kim D.-H., Alayande A. B., Lee J.-M., Jang J.-H., Jo S.-M., Jae M.-R., et al. (2024a). Emerging marine environmental pollution and ecosystem disturbance in ship hull cleaning for biofouling removal. Sci. Total. Environ. 906, 167459. doi: 10.1016/j.scitotenv.2023.167459
Kim T., Eo S., Shim W. J., Kim M. (2024b). Qualitative and quantitative assessment of microplastics derived from antifouling paint in effluent from ship hull hydroblasting and their emission into the marine environment. J. Hazard. Mater. 477, 135258. doi: 10.1016/j.jhazmat.2024.135258
Krutwa A., Klemola E., Broeg K. (2019). In-water cleaning (IWC) of boats and ships in the Baltic Sea region-Current procedures and future needs (Hamburg, Germany: Federal Maritime and Hydrographic Agency (BSH). Available online at: https://www.balticcomplete.com/attachments/article/312/COMPLETE%20in-water%20cleaning%20workshop%20report.pdf (Accessed July, 2024). Workshop Report.
Lim Y. K., Kim M., Yoon J. Y., Soon Z. Y., Shin K., Baek S. H. (2023). Effect of wastewater from in-water cleaning of ship hulls on attached and unattached microalgae. Mar. pollut. Bull. 194, 115273. doi: 10.1016/j.marpolbul.2023.115273
Morrisey D., Gadd J., Page M., Floerl O., Woods C., Lewis J., et al. (2013). In-water cleaning of vessels: Biosecurity and chemical contamination risks. MPI Technical Paper No: 2013/11 (New Zealand Government – Ministry for Primary Industries). Available online at: https://www.mpi.govt.nz/dmsdocument/4092-in-water-cleaning-of-vessels-biosecurity-and-chemical-contamination-risks (Accessed July, 2024).
Moser C. S., Wier T. P., Grant J. F., First M. R., Tamburri M. N., Ruiz G. M., et al. (2016). Quantifying the total wetted surface area of the world fleet: a first step in determining the potential extent of ships’ biofouling. Biol. Invasions. 18, 265–277. doi: 10.1007/s10530-015-1007-z
Muller-Karanassos C., Turner A., Arundel W., Vance T., Lindeque P. K., Cole M. (2019). Antifouling paint particles in intertidal estuarine sediments from southwest England and their ingestion by the harbour ragworm, Hediste diversicolor. Environ. pollut. 249, 163–170. doi: 10.1016/j.envpol.2019.03.009
Oliveira D., Granhag L. (2016). Matching forces applied in underwater hull cleaning with adhesion strength of marine organisms. J. Mar. Sci. Eng. 4(4), 66. doi: 10.3390/jmse4040066
Oliveira D. R., Granhag L. (2020). Ship hull in-water cleaning and its effects on fouling-control coatings. Biofouling 36, 332–350. doi: 10.1080/08927014.2020.1762079
Paruta P., Pucino M., Boucher J. (2022). Plastic paints the environment, EA- environmental action (EA - Environmental Action). Available online at: https://www.e-a.earth/wp-content/uploads/2023/07/plastic-paint-the-environment.pdf (Accessed July, 2024).
Peng G., Xu B., Li D. (2022). Gray Water from ships: A significant sea-based source of microplastics? Environ. Sci. Technol. 56, 4–7. doi: 10.1021/acs.est.1c05446
Ralston E. (2023). The effect of husbandry and original location on the fouling of transplanted panels. J. Mar. Sci. Eng. 11, 478. doi: 10.3390/jmse11030478
Schiff K., Diehl D., Valkirs A. (2004). Copper emissions from antifouling paint on recreational vessels. Mar. pollut. Bull. 48, 371–377. doi: 10.1016/j.marpolbul.2003.08.016
Scianni C., Georgiades E. (2019). Vessel in-water cleaning or treatment: Identification of environmental risks and science needs for evidence-based decision making. Front. Mar. Sci. 6, 467. doi: 10.3389/fmars.2019.00467
Scianni C., Georgiades E., Mihaylova R., Tamburri M.N. (2023). Balancing the consequences of in-water cleaning of biofouling to improve ship efficiency and reduce biosecurity risk. Front. Mar. Sci. 10, 1239723. doi: 10.3389/fmars.2023.1239723
Singh N., Turner A. (2009). Trace metals in antifouling paint particles and their heterogeneous contamination of coastal sediments. Mar. pollut. Bull. 58, 559–564. doi: 10.1016/j.marpolbul.2008.11.014
Soon Z. Y., Jung J.-H., Loh A., Yoon C., Shin D., Kim M. (2021a). Seawater contamination associated with in-water cleaning of ship hulls and the potential risk to the marine environment. Mar. pollut. Bull. 171, 112694. doi: 10.1016/j.marpolbul.2021.112694
Soon Z. Y., Jung J. H., Yoon C., Kang J. H., Kim M. (2021b). Characterization of hazards and environmental risks of wastewater effluents from ship hull cleaning by hydroblasting. J. Hazard. Mater. 403, 123708. doi: 10.1016/j.jhazmat.2020.123708
Soon Z. Y., Kim T., Jung J. H., Kim M. (2023). Metals and suspended solids in the effluents from in-water hull cleaning by remotely operated vehicle (ROV): Concentrations and release rates into the marine environment. J. Hazard. Mater. 460, 132456. doi: 10.1016/j.jhazmat.2023.132456
Soroldoni S., Abreu F., Castro I. B., Duarte F. A., Pinho G. L. L. (2017). Are antifouling paint particles a continuous source of toxic chemicals to the marine environment? J. Hazard. Mater. 330, 76–82. doi: 10.1016/j.jhazmat.2017.02.001
Soroldoni S., Da Silva S. V., Castro I. B., Martins C. M. G., Pinho G. L. L. (2020). Antifouling paint particles cause toxicity to benthic organisms: Effects on two species with different feeding modes. Chemosphere 238, 124610. doi: 10.1016/j.chemosphere.2019.124610
Tamburri M. N., Davidson I. C., First M. R., Scianni C., Newcomer K., Inglis G. J., et al. (2020). In-water cleaning and capture to remove ship biofouling: An initial evaluation of efficacy and environmental safety. Front. Mar. Sci. 7, 437. doi: 10.3389/fmars.2020.00437
Tamburri M. N., Georgiades E. T., Scianni C., First M. R., Ruiz G. M., Junemann C. E. (2021). Technical considerations for development of policy and approvals for in-water cleaning of ship biofouling. Front. Mar. Sci. 8, 804766. doi: 10.3389/fmars.2021.804766
Tamburri M. N., Soon Z. Y., Scianni C., Øpstad C. L., Oxtoby N. S., Doran S., et al. (2022). Understanding the potential release of microplastics from coatings used on commercial ships. Front. Mar. Sci. 9, 1074654. doi: 10.3389/fmars.2022.1074654
Tribou M., Swain G. (2015). Grooming using rotating brushes as a proactive method to control ship hull fouling. Biofouling 31, 309–319. doi: 10.1080/08927014.2015.1041021
Turner A. (2010). Marine pollution from antifouling paint particles. Mar. pollut. Bull. 60, 159–171. doi: 10.1016/j.marpolbul.2009.12.004
Turner A., Ostle C., Wootton M. (2022). Occurrence and chemical characteristics of microplastic paint flakes in the North Atlantic Ocean. Sci. Total. Environ. 806, 150375. doi: 10.1016/j.scitotenv.2021.150375
UNCTAD (United Nations Conference of Trade and Development) (2023). Merchant fleet by flag of registration and by type of ship, annual. Available online at: https://unctadstat.unctad.org/datacentre/dataviewer/US.MerchantFleet (Accessed July, 2024).
USEPA (United States Environmental Protection Agency) (2011). Underwater ship husbandry discharges (Washington, DC 20460: United States Environmental Protection Agency, Office of Wastewater Management). Available online at: https://www3.epa.gov/npdes/pubs/vgp_hull_husbandry.pdf (Accessed July, 2024).
Valkirs A. O., Seligman P. F., Haslbeck E., Caso J. S. (2003). Measurement of copper release rates from antifouling paint under laboratory and in-situ conditions. Mar. pollut. Bull. 46, 763–779. doi: 10.1016/S0025-326X(03)00044-4
Verschoor A., De Poorter L., Dröge R., Kuenen J., de Valk E. (2016). Emission of microplastics and potential mitigation measures: Abrasive cleaning agents, paints and tyre wear (Netherlands: National Institute for Public Health and the Environment). Available online at: https://www.rivm.nl/bibliotheek/rapporten/2016-0026.pdf (Accessed July, 2024).
Vogy-Vincent N. S., Burt A. J., Kaplan D. M., Mitarai S., Turnbull L. A., Johnson H. L. (2023). Sources of marine debris for Seychelles and other remote islands in the western Indian Ocean. Mar. pollut. Bull. 187, 114497. doi: 10.1016/j.marpolbul.2022.114497
Wezenbeek J. M., Moermond C. T. A., Smit C. E. (2018). Antifouling systems for pleasure boats. RIVM report 2018-008. Available online at: https://www.rivm.nl/bibliotheek/rapporten/2018-0086.pdf (Accessed July, 2024).
Keywords: marine coatings, microplastics, in-water cleaning, antifouling paint particles, environmental impact
Citation: Soon ZY, Tamburri MN, Kim T and Kim M (2024) Estimating total microplastic loads to the marine environment as a result of ship biofouling in-water cleaning. Front. Mar. Sci. 11:1502000. doi: 10.3389/fmars.2024.1502000
Received: 26 September 2024; Accepted: 12 November 2024;
Published: 28 November 2024.
Edited by:
Matteo Oliva, Centro Interuniversitario di Biologia Marina ed Ecologia Applicata “G. Bacci” di Livorno (CIBM), ItalyReviewed by:
Nüket Sivri, Istanbul University-Cerrahpasa, TürkiyeEric Holm, Naval Surface Warfare Center Carderock Division, United States
Copyright © 2024 Soon, Tamburri, Kim and Kim. This is an open-access article distributed under the terms of the Creative Commons Attribution License (CC BY). The use, distribution or reproduction in other forums is permitted, provided the original author(s) and the copyright owner(s) are credited and that the original publication in this journal is cited, in accordance with accepted academic practice. No use, distribution or reproduction is permitted which does not comply with these terms.
*Correspondence: Moonkoo Kim, bWtpbUBraW9zdC5hYy5rcg==