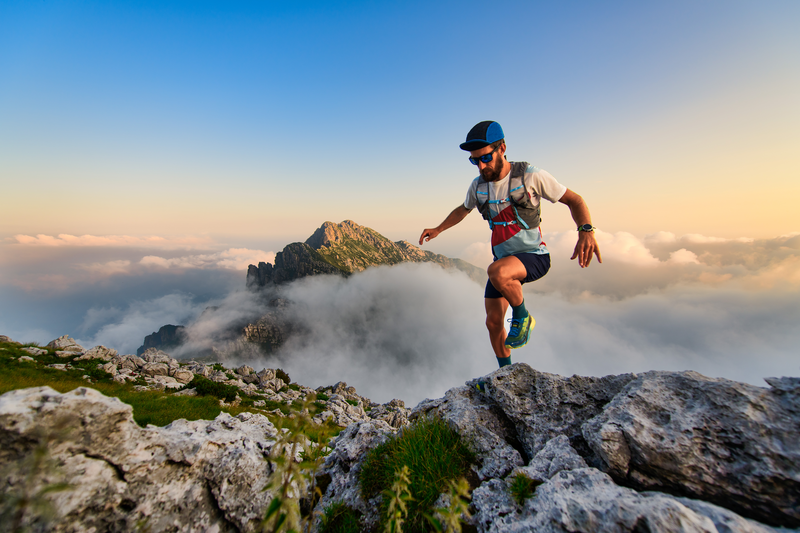
94% of researchers rate our articles as excellent or good
Learn more about the work of our research integrity team to safeguard the quality of each article we publish.
Find out more
REVIEW article
Front. Mar. Sci. , 10 December 2024
Sec. Marine Fisheries, Aquaculture and Living Resources
Volume 11 - 2024 | https://doi.org/10.3389/fmars.2024.1499228
This article is part of the Research Topic Towards Sustainable Marine Aquaculture: Innovations and Eco-Friendly Practices View all 8 articles
This review delves into the increasing interest in probiotics and paraprobiotics as a viable alternative to antibiotics in aquaculture, highlighting their potential to enhance fish health and prevent diseases. As the aquaculture industry continues its global expansion, addressing the challenges associated with disease outbreaks in high-density fish populations becomes imperative. The review underscores the promising role of probiotics and paraprobiotics as a sustainable strategy to mitigate these challenges. The diverse positive impacts of various probiotic strains such as Arthrobacter, Bacillus, Lactobacillus, Bifidobacterium, Clostridium, and others emphasize their roles in enhancing growth, resistance to diseases (including bacterial, viral, and parasitic infections), stress reduction, water quality management, and environmental sustainability. Challenges such as stability, host specificity, and regulatory considerations must be addressed to optimize the use of probiotics in aquaculture. Additionally, paraprobiotics, or non-viable microbial cells, present a safer alternative to the criticized antibiotics and even live probiotics in environments where microbial viability poses a risk. These inactivated cells retain the ability to modulate the immune system and improve gut health, offering a promising complementary approach to fish disease prevention. The review advocates for a systematic approach combining research, innovation, and collaboration to effectively integrate probiotics and paraprobiotics into fish farming practices. Furthermore, the mechanisms by which probiotics and paraprobiotics modulate gut microbiota, produce antimicrobial compounds, and strengthen fish’s immune system have been elucidated. Moreover, the practical applications of probiotics in fish farming, including optimal administration methods and the challenges and limitations faced by the industry, have been discussed. Emphasis on the importance of continued research to explore new probiotic and paraprobiotic strains and develop innovative delivery systems to ensure the sustainability of aquaculture has been discussed. By enhancing fish health, reducing the need for antibiotics, and improving water quality, probiotics, and paraprobiotics contribute to more sustainable and environmentally responsible aquaculture operations.
Aquaculture stands as the swiftest expanding sector in animal production globally, trading around 160 million tons of farmed fish valued at US$80 billion annually (Loh et al., 2020). This trend accelerates, notably with high-value demersal fish species such as grouper, barramundi, snapper, and pompano (FAO, 2014). The aquaculture industry faces a significant setback due to disease outbreaks among aquatic species, resulting from the necessity of high stocking densities to meet the escalating fish demand (Abarike et al., 2018). Antibiotics, vaccines, and other prophylactic mechanisms have been widely employed for disease management in aquaculture. The widespread use of antibiotics in treating infections creates selective pressure for antibiotic resistance, a trait that could be transferred to other bacteria. It is widely acknowledged that administering antibiotics to finfish and shellfish potentially causes adverse effects on the host organisms and humans. This understanding led the European Union to prohibit antibiotic use in 2003. As an alternative approach to using antibiotics, vaccines, and chemicals in treating diseases, probiotics and paraprobiotics have emerged due to their eco-friendly and immune-based prevention attributes in aquaculture (Ringø, 2020). As antibiotics face limitations as growth promoters in the livestock and aquatic industries across many countries, the utilization of probiotics is rising, emerging as a viable alternative (Kwoji et al., 2021).
Probiotics are recognized for their positive impacts on health and have become established as dietary supplements, well-known for their multitude of health benefits (Mishra et al., 2015). The World Health Organization (WHO) and Food and Agricultural Organization (FAO) defines probiotics as “live microorganisms that, when taken in sufficient quantities, provide health advantages to the host (Wang et al., 2019). Over time, a range of probiotic species from the bacteria genus Arthrobacter, Bacillus, Enterococcus, Lactobacillus, Lactococcus, Micrococcus, Pediococcus, Aeromonas, Burkholderia, Enterobacter, Pseudomonas, Rhodopseudomonas, Roseobacter, Shewanella, and Clostridium butyricum have been identified and applied to boost the growth and immune capabilities of various aquaculture species (Kuebutornye et al., 2019; Tran et al., 2020a). Probiotics encompass nonpathogenic species like those from the Saccharomyces, Streptococcus, and Lactococcus classes. Their beneficial effects on the host can be either direct or indirect, involving improved barrier function, modulation of the mucosal immune system, synthesis of antimicrobial substances, bolstered food digestion and absorption, and changes to the intestinal microflora (Hemaiswarya et al., 2013). Meanwhile, microbial organisms employed as probiotics or under assessment as potential probiotics in Chinese aquaculture hail from diverse taxonomic divisions, encompassing Actinobacteria, Bacteroidetes, Firmicutes, Proteobacteria, and yeast (Wang et al., 2019). Probiotics have shown an ardent ability to improve fish’s health and welfare by aiding disease resistance, enhancing growth, reducing stress, managing water quality, and improving reproduction (Indriyani Nur, 2019) (Figure 1). The effectiveness of probiotics in fish can vary based on factors such as the specific probiotic strain used, fish species, environmental conditions, dosage, and method of administration (Nayak, 2010).
Probiotics’ beneficial effects are not limited to their living cells. Alongside probiotics, paraprobiotics (also known as ghost probiotics) are non-viable microbial cells that confer health benefits to the host (Taverniti and Guglielmetti, 2011; Monteiro et al., 2023). They have recently gained much attention due to their potential role in aquaculture. Paraprobiotics are considered a safer alternative to live probiotics, especially in environments where the viability of microorganisms may pose a risk. They retain the ability to modulate the immune system, produce bioactive compounds, and improve gut health, similar to live probiotics (Taverniti and Guglielmetti, 2011; Vallejo-Cordoba et al., 2020; Monteiro et al., 2023). The use of paraprobiotics can be particularly advantageous in aquaculture settings where the stability and safety of microbial supplements have critical concerns (Nayak, 2010). Paraprobiotics are defined as “inactivated (non-viable) microbial cells, which, when administered in sufficient amounts, confer benefit to consumers.” This description resembles that of probiotics defined by the FAO/WHO with slight adjustments (Aguilar-Toalá et al., 2018; Siciliano et al., 2021). Numerous studies have demonstrated that dead probiotic cells can also elicit various biological responses. Research has consistently shown that products containing both viable and non-viable cells can produce beneficial biological responses (Thakur et al., 2016; Bajpai et al., 2018). Paraprobiotics, derived from heat-inactivated cells, can be utilized to boost immune responses and exhibit immunomodulatory activities. The components of dead cells have been found to exhibit anti-inflammatory properties in the gastrointestinal tract (GIT). Interestingly, both live and dead probiotics have been shown to exert specific actions (Adams, 2010; de Almada et al., 2016; Akter et al., 2020). Probiotic bacteria can be rendered inactive using various techniques, including sonication, heat, chemicals, and gamma or ultraviolet (UV) radiation. The most commonly used technique among them is heat inactivation. In some circumstances, the heat-inactivation method may be safer than other inactivation techniques like UV-inactivation. These techniques kill microorganisms, and each one has a unique inactivation mechanism. The probiotic microorganisms’ beneficial qualities ought to be preserved in paraprobiotics through the inactivation process (Choudhury and Kamilya, 2019; Tran et al., 2022). Paraprobiotics’ health-promoting benefits are illustrated in Figure 2.
Probiotics and paraprobiotics stand out as safe supplements that enhance host health by boosting growth, offering nutrition, regulating microbial presence, fortifying immune responses, optimizing feed utilization, bolstering digestive enzyme activity and efficiency, reducing stress, refining water quality, and managing diseases (Selim and Reda, 2015) (Figure 1). Both probiotics and paraprobiotics offer sustainable, environmentally friendly solutions for enhancing fish health and disease resistance, making them essential components in modern aquaculture practices (Indriyani Nur, 2019).
The application of probiogenomics in aquaculture represents a cutting-edge approach to enhance the effectiveness of both probiotic and paraprobiotic strategies in fish health management. This emerging field combines genomic technologies with probiotic and paraprobiotic research to elucidate the molecular mechanisms underlying probiotic-host interactions, facilitating the development of more potent and targeted probiotic strains (Pérez-Sánchez et al., 2014). Probiogenomics enables researchers to identify and screen potential probiotic candidates more efficiently, focusing on strains with specific genetic traits that confer beneficial effects on fish health and growth (Ringø et al., 2018). This approach has also revolutionized the development of commercial probiotics/paraprobiotics by allowing for the selection of strains with enhanced stability, functionality, and host-specificity. Then, probiogenomics provides valuable insights into optimizing probiotic dosages and formulations for different fish species, as the efficacy of probiotics in disease prevention can vary significantly depending on the concentration and the target species (Hoseinifar et al., 2018). By leveraging probiogenomic approaches, aquaculturists can develop more effective, species-specific probiotic/paraprobiotic solutions to improve fish health, growth performance, and disease resistance in aquaculture systems.
This review aims to explore the protective effects of probiotics and paraprobiotics in fish, outline some of the main functions performed by these beneficial microorganisms in aquaculture, and discuss the advancements in probiogenomics that are shaping the future of biotic applications in the aquaculture industry.
Probiotic candidates in fish are typically isolated from the fish’s digestive system, rearing water, sediments in culture tanks, or other sources. These candidates are then evaluated for their probiotic properties via in vitro (such as antimicrobial activity, acid and bile tolerance, and adhesion ability) and in vivo functional assays (Ringø et al., 2018). The selection of probiotics varies greatly from one fish species to another to properly maintain the good-to-bad ratio of bacteria in the gut mucosal surface (Han et al., 2015). Unlike live probiotics, paraprobiotics do not pose the same risks associated with live bacteria, such as the potential for gene transfer or environmental persistence. Paraprobiotics have been shown to elicit similar beneficial effects as probiotics, including immune modulation, enhancement of barrier function, and production of bioactive compounds (Taverniti and Guglielmetti, 2011). These properties make paraprobiotics a viable alternative, especially in conditions where the stability and safety of live microorganisms might be compromised. Several bacterial candidates have been tested for probiotic and paraprobiotic potential, including Bacillus sp., Micrococcus sp., Enterococcus sp., Phaeobacter sp., Shewanella sp., Lactobacillus sp., and Pseudomonas sp (Lobo et al., 2014). These microorganisms have been shown to improve fish health, growth performance, and survival rates, as well as enhance the expression of several immunological factors and reduce the pathogen load to the gut mucus layer (Banerjee and Ray, 2017). They can also contribute to nutrient enhancement in the host, such as increasing crude lipid, total protein, and body weight in Nile tilapia (Oreochromis niloticus) fed with the probiotic strain of Lactobacillus sp (Hamdan et al., 2016). These microorganisms, whether viable or non-viable, have beneficial effects on the gut of aquatic animals in the digestion of dietary nutrients as well as in the production of energy (Ringø et al., 2018). The integration of paraprobiotics into aquaculture is still emerging, but their potential to control different physiological activities of aquatic organisms and provide similar benefits to live probiotics is promising. Several probiotics, such as Aeromonas media (e.g., strain A199), B. subtilis, Lactobacillus helveticus, Enterococcus faecium, Carnobacterium inhibens, are considered to be significantly effective at present (Lakshmi et al., 2013). Apart from these discussed laboratory-based probiotics, various experimentally approved commercial probiotics, and paraprobiotics are also available on the market that is also effective in aquaculture (Table 1).
Table 1. Commercial probiotics and paraprobiotics available on the market for aquaculture production.
Screening probiotics for use in fish aquaculture is a crucial process involving multiple steps to ensure the safety and efficacy of the potential probiotic strains. The initial phase typically begins with isolating microorganisms from various sources, including the GIT of healthy fish, aquatic environments, and fermented products (Amoah et al., 2024; Merrifield et al., 2010). These isolates are then subjected to a series of in vitro tests to evaluate their potential probiotic properties. Common screening criteria include the ability to survive and grow under conditions similar to those found in the fish gut, such as low pH and the presence of bile salts (Vine et al., 2004). Additionally, potential probiotics and paraprobiotics should exhibit antagonistic activity against known fish pathogens, which is often assessed through methods like agar well diffusion or co-culture assays (Newaj-Fyzul et al., 2014). Screening in vitro involves evaluating the adherence capacity of the potential probiotics to fish intestinal mucus or cell lines, as this property is considered essential for colonization and persistence in the host gut (Balcázar et al., 2008). In the case of paraprobiotics, the focus is on their ability to modulate immune responses and produce bioactive compounds even in their non-viable state (Adams, 2010). The production of beneficial compounds, such as digestive enzymes, vitamins, or antimicrobial substances, is also assessed during this stage. Safety considerations are paramount, and potential probiotics are screened for antibiotic resistance to ensure they do not contribute to the spread of antibiotic-resistance genes in aquatic environments (Pérez-Sánchez et al., 2014). The absence of virulence factors and toxin production is verified to guarantee the safety of the probiotic candidates for the host fish and human consumers.
Following successful in vitro screening, promising probiotic and paraprobiotic candidates undergo in vivo evaluation in target fish species. These trials assess their effects on fish growth performance, feed utilization, immune response, and disease resistance (Ringø et al., 2018). Growth parameters such as weight gain, specific growth rate (SGR), and feed conversion ratio (FCR) are commonly measured. Immune parameters, including lysozyme activity, phagocytic activity, and expression of immune-related genes, are evaluated to determine their immunomodulatory effects (Hoseinifar et al., 2015b). Challenge tests against common fish pathogens are conducted to assess the protective effects of the probiotic treatment. Additionally, the impact of the probiotic on gut microbiota composition and intestinal morphology is often examined using molecular techniques and histological analysis (Carnevali et al., 2017).
The final stages of probiotic screening involve assessing the technological properties of the selected strains, such as their ability to survive processing conditions, storage stability, and ease of administration in aquaculture settings (Merrifield et al., 2010). Factors like temperature tolerance, oxygen requirements, and compatibility with feed ingredients are considered to ensure the practical application of the probiotic in fish farming. It is important to note that the efficacy of probiotics can vary depending on factors such as fish species, developmental stage, and environmental conditions. Comprehensive evaluations under different scenarios are necessary to determine the most suitable probiotic strains for specific applications in aquaculture (Dawood et al., 2018). Some studies have demonstrated that the heterogeneous expression of probiotic bacteria in fish can increase intraepithelial lymphocytes, acidophilic granulocytes, and bactericidal activity specific to fish. As research advances, new screening methodologies, including high-throughput sequencing and omics approaches, are being incorporated to provide a more comprehensive understanding of probiotic-host interactions and identify novel probiotic candidates with enhanced benefits for fish health and aquaculture production. The strategy for selecting probiotics for commercial use is shown in Figure 3. A list of probiotics and paraprobiotics currently available on the market has been provided in Table 1.
Gut microbiota modulation refers to the alteration or management of the microorganisms residing in the GIT to achieve a desired outcome. This modulation can be achieved through various means, including supplementing probiotics and paraprobiotics. The supplementation of Bacillus cereus and Geotrichum candidum probiotics in Labeo rohita fish resulted in the detection of a lower relative abundance of Trichosporon and Cryptococcus and even an absence of opportunistic pathogenic strains (Staphylococcus saprophyticus and Sporobolomyces lactosus) in the gut as compared to the control group (Ghori et al., 2022). Using paraprobiotics as a food carrier in a carbohydrate matrix can be a far more promising way to enhance gut modulation than incorporating them directly into foods or protein-rich diets. Adding paraprobiotics increased the prevalence of beneficial bacterial genera, including Lactobacillus, Bifidobacterium, and Ruminococcus, which are known for their positive roles in gut health. In contrast, the genera Corynebacterium and Plesiomonas, which are less associated with gut health benefits, were less prevalent after paraprobiotic supplementation (Yolmeh et al., 2024).
Fish share a notably intimate connection with their surrounding environment. Contrasts between land and water species are evident in how their intestinal microbiota interacts with their surroundings. Different fish species also have a wide range of GIT morphology differences (Denev et al., 2009). Aquatic animals regularly encounter potential pathogens during osmoregulation and feeding. Unlike terrestrial animals, the bacterial makeup in the GIT of aquatic animals spurred the development of the probiotic concept. While humans and land-based livestock undergo embryonic development protected within an amnion, fish and shellfish release their larvae into the external environment early in their life cycle. These vulnerable larvae, despite their underdeveloped digestive tracts, begin feeding at an earlier age, exposing them to a heightened risk of gastrointestinal microbiota-related issues (Lara-flores, 2011). An imbalanced fish gut microbiota with heightened bad bacteria can result in decreased metabolism, stunted growth, stress, and the onset of diseases. The influence of gut microbiota on fish physiology heavily relies on the specific composition of microbial communities within the gut, largely shaped by their diet (Ghori et al., 2022). In tilapia, alterations in the gastrointestinal microbiota corresponded with the stimulation of the endocrine system, leading to intensified expression of insulin-like growth factor system genes, showcasing the microbiota’s influence on fish development by regulating gene expression and organ function in intestinal epithelial cells (Haque et al., 2022; Luan et al., 2023). Understanding the intricate bacterial makeup through advanced sequencing techniques is pivotal in precisely modifying these communities and enhancing fish health and productivity. Manipulating fish gut microbiota using prebiotics, antibiotics, and probiotics offers a further exploration of their role. Emerging dietary elements, particularly prebiotics, and synbiotics as supplementary feeds aside from probiotics and paraprobiotics, regulate fish physiology, growth, and overall health. Researchers are increasingly focusing on these additives due to their direct impact on the gut microbiome in fish (Ghori et al., 2022).
Some researchers have suggested that combining probiotics and paraprobiotics might offer synergistic benefits to animals, including fish. For example, a recent work by Yuhana et al. (2024) explored the effects of euryhaline probiotic and paraprobiotic B. cereus BR2 on African catfish. The experiment, which involved the supplementation of 1% (w/w) dose of probiotics and paraprobiotics at a cell density of 108 and 1010 CFU/mL revealed a significant enhancement in the growth performance (survival rate, final weight, FCR, and SGR), digestive enzyme (protease, amylase, and lipase) activities, immune-related genes (IL-1β and MHC-2β expression), and resistance to Edwardsiella tarda ETS1.1 in the treated group contrast to the results obtained in the control group. Another study by Meng et al. (2023) showed that combining live and inactivated Lactobacillus rhamnosus enhanced gut microbiota diversity and nutrient absorption in common carp (Cyprinus carpio).
Probiotics actively colonize the gut and interact with the host’s microbiome and immune system, whereas paraprobiotics modulate the gut environment through their cellular components, such as peptidoglycans, lipoteichoic acids, and metabolites, without colonizing the gut.
In general, microbial populations release substances that can kill or slow the growth of other microbes, shaping how they compete for resources like chemicals and energy (Verschuere et al., 2000). These substances in the host’s gut, surfaces, or environment create a defense against opportunistic pathogens. The antibacterial effect of bacteria is attributed to several factors, including the production of antibiotics (Williams and Vickers, 1986), siderophores (Sugita et al., 2012), bacteriocins (Vandenbergh, 1993), and changes in pH through the release of organic acids (Sugita et al., 1997). For example, according to Sugita et al. (2012), probiotic strains such as Photobacterium leiognathi, Vibrio scophthalmi, and Enterovibrio norvegicus were noted to produce siderophore inhibitory substances against some pathogenic bacteria. Again, Banerjee and Ray (2017) also reported that Bacillus subtilis strain LR1 produces bacteriocins that inhibit pathogens, including Aeromonas hydrophila, Aeromonas salmonicida, Bacillus mycoides, and Pseudomonas fluorescens. Also, Feliatra et al. (2018) reported that Bacillus thuringiensis as a probiotic strain H4, produces bacteriocins that inhibit pathogens, including Pseudomonas stutzeri (Feliatra et al., 2015). As shown in laboratory studies, bacteriocins (Table 2) can inhibit pathogen replication using small-molecule compounds (Cavera et al., 2015; Heilbronner et al., 2021; Soltani et al., 2021; Retnaningrum, 2024). Leading this category are short-chain fatty acids (SCFAs) like lactic acid, alongside hydrogen peroxide, showing similar inhibitory effects. Within Lactobacilli, both low-molecular-weight bacteriocins (LMWB) and high-molecular-weight bacteriocins (class III) are produced. LMWB, classified as antimicrobial peptides, fall into three categories: class I lantibiotics (featuring posttranslationally modified peptides with unique amino acids like lanthionine), class II (heat-stable non-lantibiotics), and class IV (cyclic antimicrobial peptides) (Maqueda et al., 2008; Oelschlaeger, 2010). Acetic and lactic acid, among other organic acids, exhibit potent inhibition against gram-negative bacteria, making them key antimicrobial components in probiotics. These acids penetrate bacterial cells in their undissociated form, where they later dissociate within the cytoplasm. This process may cause a decrease in intracellular pH or accumulation of the acid’s ionized form, potentially resulting in pathogen death (Bermudez-Brito et al., 2012). Several bacterial species including Blautia spp., Bifidobacterium spp., and Clostridium spp. (Clostridium butyricum and Clostridium lactatifermentans) are reported to be involved in SCFA production (organic acids such as propionic and butyric acid), significant in enhancing the growth performance, intestinal health (enhancing the morphology (intestinal mucosal thickness, goblet cell counts, villi length), and gut microbial community and diversity), immune responses, and antioxidant activities (such as increase in total-superoxide dismutase, superoxide dismutase, glutathione peroxidase, and catalase) (Tran et al., 2020b; 2023).
Lactic acid bacteria, known for producing bacteriocins, often inhibit the growth of other microorganisms, primarily gram-positive ones (Timothy et al., 2021; Fernandes and Jobby, 2022). While most pathogens associated with aquaculture are gram-negative, research has explored the potential role of lactic acid bacteria as probiotics in aquaculture (Vandenbergh, 1993). So, considering lactic acid bacteria’s minimal presence in fish gut microbiota and their typically nonpathogenic nature, it’s uncertain if their inhibition of related species significantly contributes to the overall health of the host organism (Verschuere et al., 2000).
While paraprobiotics do not produce active antimicrobial compounds like live probiotics, they can still contribute to antimicrobial effects through various mechanisms. First, the cell wall components of paraprobiotics, such as peptidoglycan and lipoteichoic acids, can stimulate the host’s immune system, indirectly contributing to antimicrobial defense (de Almada et al., 2016). Additionally, some paraprobiotics may contain preformed antimicrobial compounds, such as bacteriocins or organic acids, that were produced before inactivation, which can still exert their effects despite the cells being non-viable (Nataraj et al., 2020). Although they are not alive, paraprobiotics can occupy space in the gut, potentially preventing the adhesion of pathogenic bacteria to the intestinal epithelium, a mechanism known as competitive exclusion (Zommiti et al., 2018). Certain studies have demonstrated that paraprobiotics can disrupt existing biofilms formed by pathogenic bacteria, indirectly contributing to antimicrobial effects (Sharma et al., 2020). Lastly, they are known to modulate the composition of the gut microbiota, potentially favoring the growth of beneficial bacteria that produce antimicrobial compounds, thus supporting overall gut health (Chuah et al., 2019). These mechanisms illustrate the multifaceted role of paraprobiotics in enhancing host defenses against pathogens, even in the absence of live cells.
While the mechanisms of action differ between probiotics and paraprobiotics, both can contribute to antimicrobial effects in the host. Probiotics primarily act through the direct production of antimicrobial compounds, while paraprobiotics exert their effects through preformed compounds, immune modulation, and physical interactions with the gut environment.
Probiotics, those beneficial microorganisms found in various supplements or naturally in the environment, play a crucial role in bolstering a fish’s immune system against diseases. A recent review by Shija et al. (2023) showed that Bacillus probiotics offer a key advantage by regulating mucosal and systemic immunity, leading to decreased inflammation and heightened infection-fighting capabilities. They play a significant role in enhancing the absorption of essential vitamins and minerals from food, aiding in the breakdown of proteins, carbohydrates, and fats, and ensuring that the body receives vital nutrients for optimal growth and health (Peng et al., 2020). When administered to fish, probiotics interact with the fish’s gut microbiota, fostering a balanced and healthy environment within the GIT. Selecting the appropriate probiotics is critical, as incorrect choices can detrimentally affect nutrient metabolism, immune regulation, resistance against colonization, and defense against pathogens (Butt and Volkoff, 2019). Serum immunoglobulins play a vital role in the humoral immune system of fish and higher vertebrates, contributing significantly to disease resistance (Sahoo et al., 2021). B lymphocytes produce antibodies that attach to encountered antigens, effectively blocking disease-causing agents from entering the body (El-Ezabi et al., 2011). Other immune parameters, including lysozyme, acid phosphatase (ACP), alkaline phosphatase (AKP), and catalase (CAT), play influential roles in enhancing the immune response of fish. Various Bacillus probiotics, including B. amyloliquefaciens, B. coagulans ATCC 7050, B. licheniformis ATCC 11946, B. tequilensis GPSAK2, B. velezensis TPS3N, B. velezensis GPSAK4, P. polymyxa ATCC 842, B. subtilis TPS4, B. subtilis GPSAK9, and B. amyloliquefaciens TPS1, whether used independently or in combination, demonstrate the ability to elevate immunoglobulin levels, bolster leukocyte counts, and enhance lysozyme, ACP, AKP, and CAT levels (Amoah et al., 2021, 2023; Shija et al., 2023).
Probiotics play a significant role in improving nutrient utilization in fish. Producing digestive enzymes is one of the primary mechanisms by which probiotics enhance nutrient utilization. Several probiotic strains can synthesize enzymes such as amylases, proteases, and lipases, complementing the host’s digestive capabilities (Merrifield et al., 2010). For example, a report by Mohapatra et al. (2012) found that rohu (L. rohita) fingerlings fed with a multi-strain probiotic supplement showed significantly higher intestinal amylase, protease, and lipase activities compared to the control group. This enhanced enzymatic activity can lead to improved digestion and absorption of nutrients from the feed, resulting in better growth performance and feed conversion ratios. Probiotics and paraprobiotics also contribute to nutrient utilization by modifying the gut microbiota composition and metabolism. Beneficial bacteria can create a more favorable gut environment for nutrient absorption and even synthesize certain beneficial nutrients for the host. For example, some probiotic strains have been shown to produce SCFAs through fermentation of non-digestible carbohydrates. These SCFAs serve as energy sources for intestinal epithelial cells and have various beneficial effects on fish health (Hoseinifar et al., 2018). So, by improving nutrient utilization, probiotics and paraprobiotics not only promote better growth and health outcomes for fish but also contribute to more efficient and sustainable aquaculture practices. Table 3 shows examples of the beneficial effects of probiotics and paraprobiotics on the immune system and nutrient utilization in various fish species.
Table 3. Effects of Probiotics and Paraprobiotics on Immune System and Nutrient Utilization in Various Fish Species.
Probiotics and paraprobiotics deal with pathogens through competitive exclusion, a mechanism where beneficial microorganisms outcompete pathogens for resources and attachment sites. This process involves the production of antimicrobial compounds, competition for nutrients, and colonization of sites on the host’s mucosal surfaces (Rahman et al., 2021). Similarly, heat-killed Lactobacillus plantarum (a paraprobiotic) has demonstrated the ability to adhere to intestinal mucus, potentially blocking pathogen attachment sites in fish (Soltani et al., 2024). The competitive exclusion effect has also been observed with probiotic yeasts, such as Saccharomyces cerevisiae, which can reduce the colonization of A. hydrophila in the intestines of Nile tilapia (de Moraes et al., 2022). Multi-strain probiotic formulations have also shown enhanced competitive exclusion effects compared to single-strain probiotics in various aquaculture species (Dawood et al., 2018).
Probiotics and paraprobiotics play a crucial role in modulating the immune system of aquatic organisms, enhancing their resistance to pathogens. These beneficial microorganisms stimulate innate and adaptive immune responses, increasing immune cell production, antibodies, and cytokines (Bruce and Brown, 2017). For example, dietary supplementation with B. amyloliquefaciens has been shown to upregulate the expression of immune-related genes in the liver and intestine of Nile tilapia, improving their resistance to Streptococcus agalactiae infection (Van Doan et al., 2021). Paraprobiotics, such as heat-killed Enterococcus faecalis, have demonstrated the ability to enhance the activity of phagocytes and increase the production of lysozyme in rainbow trout, leading to improved survival rates when challenged with Aeromonas salmonicida (Rodriguez-Estrada et al., 2013). Probiotic mixtures containing Lactobacillus and Bacillus species have been reported to stimulate the production of pro-inflammatory cytokines and enhance the activity of natural killer cells in various fish species, contributing to improved disease resistance (Van Doan et al., 2021). Additionally, the immunomodulatory effects of probiotics and paraprobiotics have been noted to extend beyond direct pathogen interactions, influencing aquaculture species’ overall health and stress resistance (Dawood et al., 2020).
One of the key mechanisms by which probiotics and paraprobiotics fight against disease pathogens is through the production of antimicrobial compounds. These substances include organic acids, hydrogen peroxide, bacteriocins, and biosurfactants, which can directly inhibit or kill pathogenic microorganisms (Banerjee and Ray, 2017). For example, B. subtilis isolated from a healthy fish gut produced various antimicrobial peptides that effectively inhibited the growth of V. anguillarum and A. hydrophila (Kuebutornye et al., 2019). Lactic acid bacteria, such as Lactobacillus plantarum, produced organic acids that lowered the pH of the intestine, creating unfavorable conditions for pathogen growth (Giri et al., 2018). Even in their non-viable form, paraprobiotics can retain the ability to produce or release antimicrobial compounds. Heat-killed L. acidophilus has been found to maintain its antibacterial activity against fish pathogens due to the presence of stable antimicrobial peptides (Ringø et al., 2018). The synergistic effects of multiple antimicrobial compounds produced by probiotic consortia have shown enhanced pathogen inhibition compared to single-strain applications in various aquaculture systems (Puvanasundram et al., 2021).
Probiotics and paraprobiotics deal with pathogens through the formation of beneficial biofilms and the disruption of pathogenic biofilms. Biofilms are complex communities of microorganisms attached to surfaces, and their formation can significantly impact the colonization and persistence of both beneficial and pathogenic bacteria in aquaculture environments (Cai and Arias, 2017; Muhammad et al., 2020). Bacillus species have been shown to form protective biofilms on fish skin and intestinal surfaces, creating a barrier against pathogen colonization (Benhamed et al., 2014; Elsadek et al., 2023). These beneficial biofilms can also produce extracellular polymeric substances (EPS) that have antimicrobial properties and enhance the host’s immune response (Watters et al., 2016; Singh et al., 2021). Conversely, probiotics and paraprobiotics can disrupt existing pathogenic biofilms. For example, cell-free supernatants from L. plantarum have demonstrated the ability to inhibit and disperse biofilms formed by V. parahaemolyticus (Knipe et al., 2021; Shangguan et al., 2021). Heat-killed B. subtilis paraprobiotic strain has also shown the capacity to interfere with quorum sensing systems of pathogens, thereby reducing their biofilm formation capabilities (Rajesh and Rai, 2016; Mayer and Kronstad, 2017; Arjes et al., 2022). The interaction between probiotics, paraprobiotics, and biofilms represents a complex and dynamic process that plays a crucial role in pathogen control in aquaculture systems (Cai and Arias, 2017; Arunkumar et al., 2020).
Probiotics and paraprobiotics significantly tackle disease pathogens by modulating the host microbiome, which plays a crucial role in maintaining aquatic organisms’ health and disease resistance. By introducing beneficial microorganisms or their components, these supplements can alter the composition and diversity of the gut microbiota, creating an environment less favorable for pathogen colonization (Choudhury and Kamilya, 2019; Vargas-Albores et al., 2021). For example, dietary supplementation with L. rhamnosus has been shown to increase the abundance of beneficial bacteria while reducing potentially pathogenic species in the intestines of zebrafish, leading to improved resistance against A. hydrophila infection (Zhong et al., 2022; Zhou et al., 2022). Heat-killed Enterococcus faecium paraprobiotics have demonstrated the ability to modulate the gut microbiota of rainbow trout, promoting the growth of lactic acid bacteria and inhibiting potential pathogens (Rodriguez-Estrada et al., 2013; Ringø et al., 2018; Zhong et al., 2022; Zhou et al., 2022). The modulation of the host microbiome by probiotics and paraprobiotics extends beyond the gut, influencing the microbial communities on the skin and gills of aquatic animals, which serve as important barriers against pathogen invasion (Yeşilyurt et al., 2021; Goh et al., 2023; Szydłowska and Sionek, 2023). Consequently, the interaction between probiotics, paraprobiotics, and the host microbiome has been shown to influence metabolic processes and nutrient absorption, indirectly improving the host’s ability to resist pathogen infections (Karthika Parvathy et al., 2022; Zawistowska-Rojek and Tyski, 2022).
Probiotics and paraprobiotics deal with pathogens via the interference with quorum sensing (QS) systems, which are cell-to-cell communication mechanisms used by many pathogenic bacteria to coordinate virulence factor production and biofilm formation (Lazar et al., 2023; Rawal and Ali, 2023; Che et al., 2024). By disrupting these communication systems, probiotics and paraprobiotics can effectively reduce pathogens’ virulence and colonization ability in aquaculture environments. For example, certain strains of B. subtilis have been found to produce enzymes that degrade acyl-homoserine lactones (AHLs), key signaling molecules in the QS systems of many Gram-negative pathogens like Vibrio species (Garg et al., 2014; Singh, 2015; Shaheer et al., 2021; Monzón-Atienza et al., 2024). Probiotic Lactobacillus strains have demonstrated the ability to inhibit the QS-regulated production of virulence factors in A. hydrophila, a common fish pathogen (Lu et al., 2023; Zhang et al., 2023). Heat-killed Lactobacillus plantarum paraprobiotics have shown the capacity to interfere with QS systems even in their non-viable state, suggesting that cell components or metabolites play a role in this interaction (Vallejo-Cordoba et al., 2020; Siciliano et al., 2021). The QS interference mechanisms of probiotics and paraprobiotics extend beyond direct pathogen interactions, influencing the overall microbial ecology of aquaculture systems and potentially reducing the spread of antibiotic resistance genes (Hernández, 2021; Srirengaraj et al., 2023; Che et al., 2024). So, combining QS interference with other probiotic mechanisms, such as competitive exclusion and immunomodulation, has shown synergistic effects in pathogen control, highlighting the multifaceted nature of probiotic and paraprobiotic interactions in aquaculture.
The protective benefits of probiotics on fish health have gained significant attention. These beneficial bacteria enhance fish immunity to diseases, promote growth, and help reduce stress. Below is an illustrative table demonstrating the protective impacts of probiotics on fish health (Table 4).
Probiotics are vital in aquaculture, fostering enhanced growth, increased feed efficiency, and overall health across diverse fish species (El-Saadony et al., 2021). Probiotics also significantly enhance growth performance in aquaculture by influencing several key mechanisms. One primary mechanism is the improvement of nutrient digestion and absorption. Probiotics can enhance the digestive enzyme activity in the fish gut, leading to a more efficient breakdown of feed components and increased nutrient availability (Singh et al., 2011). This efficiency in nutrient utilization translates into improved growth rates. Another crucial mechanism is the modulation of gut microbiota. By promoting a balanced microbial community, probiotics reduce the prevalence of harmful bacteria that can compete with fish for nutrients (Dawood et al., 2019). This balance helps maintain a healthier gut environment, which supports better growth. A balanced microbiota can also improve immune function, bolster disease resistance, and promote overall health, consequently fostering improved growth and performance (Tan et al., 2019; Wang et al., 2020; Han et al., 2024).
Probiotics also contribute to improved feed conversion ratios by optimizing nutrient absorption and reducing waste. This enhanced feed utilization reduces feed conversion ratios and lowers production costs (Hasan et al., 2023). Additionally, probiotics may mitigate stress responses by improving gut health, which helps the fish allocate more energy toward growth rather than coping with stress (Rueda-Robles et al., 2022).
Probiotics play a multifaceted role: they foster a healthier gut environment, mitigating the impact of environmental stressors on fish health and growth. This support frequently enhances growth rates and overall performance (Ntakirutimana et al., 2023). Improving digestion and absorption, probiotics can reduce excess nutrient release into the water, thus aiding in the reduction of environmental pollution associated with aquaculture practices (Nathanailides et al., 2021). Overall, the enhanced growth performance observed with probiotic use can be attributed to their role in optimizing digestion, maintaining a balanced gut microbiota, and improving feed conversion efficiency.
Environmental changes or handling stress can significantly affect fish’s health and overall well-being (Andrews et al., 2021; Menon et al., 2023). Investigating the potential of probiotics in mitigating these stress responses is a progressive approach. Probiotics, known for their beneficial effects on gut health and immunity, have shown promising results in various animal studies, including fish, in reducing stress and enhancing resilience to environmental stressors (Mohapatra et al., 2014). In fish, stress responses often manifest through behavior, physiology, and immune function changes. Probiotics might help modulate these responses by promoting a healthy gut microbiota, which is crucial to the fish’s overall health and stress tolerance (Luan et al., 2023).
Research indicates that the administration of specific probiotic strains may enhance stress tolerance in fish through various mechanisms, such as the regulation of stress hormones (Schreck and Tort, 2016). In fish, the hypothalamic-pituitary-interrenal (HPI) axis is the primary mediator of stress as it controls the release of cortisol, a major stress hormone (Zhang et al., 2015). According to Chowdhury et al. (2020), probiotics have been demonstrated to affect this endocrine axis by modifying the release and regulation of cortisol, which helps to promote a more balanced and under-control stress response. For example, by improving gut-brain axis communication, some probiotic strains may lower cortisol levels, which in turn may lessen the activation of the HPI axis during stressful situations (Herrera et al., 2019). Studies have previously highlighted probiotics’ immunity boost mechanisms (Ashaolu, 2020) and enhanced gut health mechanisms (Hasan and Banerjee, 2020). A healthy gut microbiome can improve the immune system, potentially making fish more resistant to immunosuppression due to stress, and can help nutrient absorption and reduce inflammation, which can indirectly reduce stress levels in fish. As probiotics can potentially alleviate stress-induced alterations in fish behavior by fostering a healthier internal environment, the mitigation of behavioral change mechanisms is significant (Naiel et al., 2022).
Despite promising initial results, further research is essential to comprehend the specific mechanisms, identify the most effective probiotic strains for different fish species, determine appropriate dosages, and establish optimal administration methods (Cai et al., 2022). Environmental stressors vary widely, so investigating probiotics’ efficacy across different stressors (temperature changes, pollutants, handling stress) would be crucial to determining their broader applicability (Bajagai et al., 2016). The potential use of probiotics to reduce stress responses in fish holds significant promise for aquaculture, fisheries, and conservation efforts (Vine et al., 2006). It could lead to more sustainable practices and healthier fish populations, ultimately benefiting the industry and the environment.
Research on probiotics and paraprobiotics in fish has shown promising results in resistance to various diseases, including bacterial, viral, and parasitic infections. In a review by Pandiyan et al. (2013), the effects of probiotics in preventing common bacterial infections in farmed fish were detailed. It was demonstrated that specific probiotic strains, when added to the fish diet, reduced the incidence of bacterial infections (Table 5) by promoting a healthy gut microbiota, thus enhancing fish’s immune response. Also, another research conducted by Kuebutornye et al. (2019) provided important information on using probiotics to fight bacterial infections in different fish species. The findings highlighted that certain probiotic supplementation effectively suppressed the growth of pathogenic bacteria in the fish gut, thereby lowering infection rates. Infections caused by parasites, although very limited regarding their information to researchers, have caused tremendous damage to the aquaculture sector. Abdel-Aziz et al. (2020) research on the impact of probiotics on parasitic infections in fish highlighted that certain probiotic supplements altered the gut environment, making it less favorable for parasite survival and development, leading to a decrease in parasite infestation and improved overall fish health. Mohapatra et al. (2013) also concluded that by modulating the host’s immune response and gut microbiota, probiotics played a crucial role in preventing and managing parasitic diseases among different fish species. Besides that, several viral infections have been recorded in aquaculture, stampeding the growth and development of the sector. In the works of Chattaraj et al. (2022), where the role of probiotics in preventing viral infections in aquaculture was elucidated, they observed that probiotics containing specific strains enhanced antiviral immunity in fish, reducing the susceptibility to viral pathogens and lessening the severity of infections. Mondal et al. (2022) also explained the efficacy of probiotics in controlling viral outbreaks in fish farms. Their work indicated that regular administration of probiotics reduced viral loads and enhanced the immune defense mechanisms in the fish, lowering viral infection rates significantly. Table 5 displays some other examples of the function of probiotics in preventing bacterial, parasitic, and viral infection in fish.
These studies collectively emphasize the potential of probiotics as a preventive measure against common diseases in fish, demonstrating their effectiveness in reducing the incidence and severity of bacterial, viral, and parasitic infections through their beneficial effects on the fish’s immune system and gut health.
Probiotics have emerged as a promising area of aquaculture research, particularly in their role in promoting reproduction in fish. These beneficial microorganisms have been found to influence various aspects of fish reproduction, from gamete quality to larval survival. Applying probiotics in fish reproduction is a broader trend towards more sustainable and environmentally friendly aquaculture practices.
One of the primary ways probiotics promote fish reproduction is by improving broodstock’s overall health and immune function. Gioacchini et al. (2010) demonstrated that probiotic supplementation in the zebrafish (Danio rerio) diet increased fecundity and egg quality. The researchers observed higher fertilization and hatching rates in eggs from probiotic-fed females compared to the control group. They attributed these improvements to the probiotics’ ability to modulate the expression of genes related to reproduction and metabolism. Probiotics have also been shown to enhance sperm quality in male fish. A study by Hoseinifar et al. (2015b) on common carp (Cyprinus carpio) found that dietary supplementation with Lactobacillus plantarum significantly improved sperm motility, viability, and concentration. The researchers suggested that these improvements were likely due to the probiotics’ antioxidant properties and ability to enhance nutrient absorption.
Beyond gamete quality, probiotics have been found to impact larval development and survival positively. Research by Carnevali et al. (2017) on European sea bass (Dicentrarchus labrax) showed that probiotic treatment of larvae resulted in higher survival rates, which could be linked to enhanced reproductive success in later stages. The study also noted accelerated immune system development in probiotic-treated larvae, which could benefit fish health and reproduction in the long term. The mechanisms by which probiotics influence fish reproduction are multifaceted. One key pathway is through the modulation of the gut-brain axis. Falcinelli et al. (2016) demonstrated that probiotic administration in zebrafish altered gene expression in appetite control and reproduction in the brain, suggesting probiotics’ ability to influence reproductive processes through neuroendocrine pathways.
Probiotics also play a role in enhancing the nutritional status of fish, which indirectly supports reproductive processes. A study by Mohammadian et al. (2019) on rainbow trout (Oncorhynchus mykiss) found that probiotic supplementation improved protein digestibility and amino acid absorption. This enhanced nutritional status could improve gamete production and overall reproductive performance. The impact of probiotics on fish reproduction extends to stress reduction, which is crucial for optimal reproductive performance. Stress can negatively affect reproductive processes in fish, leading to reduced gamete quality and spawning success. Research by Dawood et al. (2020) on Nile tilapia (O. niloticus) showed that probiotic supplementation reduced cortisol levels and oxidative stress markers, potentially creating a more favorable physiological state for reproduction.
Probiotics have emerged as a promising tool in aquaculture for improving water quality via the reduction of harmful nitrogenous compounds accumulation in aquatic environments. According to Zhou et al. (2009), a mixture of Bacillus sp. has the capacity of reducing the total nitrogen and phosphorus in grass carp culture water. Verschuere et al. (2000) reviewed probiotics in aquaculture and highlighted their potential to improve water quality by competing with harmful bacteria for nutrients and space. Lalloo et al. (2007) showed that multi-species probiotics could effectively reduce ammonia, nitrite, and nitrate levels in ornamental fish systems. As reviewed by Martínez Cruz et al. (2012), probiotics maintain water quality through the decomposition of undesired organic substances. Gatesoupe (1999), in his discussion on probiotics, also emphasized that they have the capacity to alter the microbial composition in both the water and the digestive tract of cultured aquatic species. Kesarcodi-Watson et al. (2008) reviewed the use of probiotics in aquaculture and highlighted their potential to inhibit the growth of pathogenic bacteria, thereby improving water quality. Xie et al. (2013) found that a probiotic mixture could significantly reduce the abundance of potential pathogens in the water of grass carp culture systems. Thus, the ability of probiotics to modulate microbial communities in aquatic environments significantly contributes to improving water quality.
Soil probiotics have emerged as a promising approach to improve water quality in aquaculture systems, indirectly benefiting fish health and productivity (Flegel, 1998). These beneficial microorganisms, typically applied to the sediment or water column, can significantly impact water quality parameters crucial for fish welfare. One of the primary ways soil probiotics improve water quality is by reducing harmful nitrogenous compounds. Bacillus species are noted to reduce ammonia and nitrite levels in aquaculture ponds effectively. The researchers found that these probiotics enhanced the nitrogen cycle by promoting the growth of nitrifying bacteria, thereby improving water quality and creating a healthier environment for fish (Zhou et al., 2009). Similarly, Kumar et al. (2016) reported that soil probiotics containing B. subtilis significantly reduced ammonia levels in carp ponds, improving fish growth and survival rates. Using probiotics in aquaculture and highlighting their potential to break down organic waste, minimize sludge accumulation, and improve overall water clarity (Verschuere et al., 2000). This process enhances water quality and helps maintain optimal dissolved oxygen levels, which are critical for fish health. A group of Bacillus species applied to pond soil was noted to effectively reduce organic matter and improve water transparency in tilapia culture systems (Lalloo et al., 2007). Soil probiotics were noted to maintain a balanced microbial community in aquaculture environments, suppressing the growth of pathogenic organisms that can deteriorate water quality. Probiotics added to soil indirectly improved water quality by reducing the bacterial load contributing to poor water conditions (Martínez Cruz et al., 2012).
Probiotics have been shown to enhance the innate immune response in various fish species. A study by Nayak (2010) demonstrated that probiotic administration could stimulate the production of antimicrobial peptides and increase phagocytic activity in fish. Pérez-Sánchez et al. (2011) found that dietary supplementation with Shewanella putrefaciens improved the innate immune response in gilthead seabream. Giri et al. (2013) reported that B. subtilis supplementation could enhance lysozyme and respiratory burst activity in rohu fish (L. rohita). Hoseinifar et al. (2015a) observed increased complement and lysozyme activities in beluga (Huso huso) juveniles fed with L. acidophilus. The adaptive immune system of fish can also be modulated by probiotic supplementation. Research by Panigrahi et al. (2007) showed that dietary L. rhamnosus supplementation could enhance antibody production and expression of cytokine genes in rainbow trout. Abid et al. (2013) found that a multi-strain probiotic mixture increased serum immunoglobulin levels in tilapia. Salinas et al. (2008) demonstrated that L. delbrueckii enhanced T-cell mediated immunity in gilthead seabream. Again, Standen et al. (2013) reported that probiotic Pediococcus acidilactici stimulated intestinal T-cell proliferation and modulated cytokine expression in tilapia.
There are several incidences recorded where probiotics improved disease resistance in fish after enhancing their immune status. Newaj-Fyzul et al. (2007) found that rainbow trout fed with B. subtilis showed increased resistance against A. hydrophila infection. Aly et al. (2008b) demonstrated that a mixture of B. subtilis and L. acidophilus improved survival rates of tilapia challenged with A. hydrophila and Pseudomonas fluorescens. Nikoskelainen et al. (2003) reported enhanced resistance against A. salmonicida in rainbow trout fed with L. rhamnosus. Zorriehzahra et al. (2016) reviewed the immunomodulatory effects of probiotics in fish and shellfish, highlighting their potential for disease prevention. The positive effects on disease resistance was achieved via the probiotic’s ability to enhance fish immunity. The impact of probiotics on fish immune systems extends to stress tolerance and overall health. Taoka et al. (2006) showed that probiotic-fed tilapia exhibited lower cortisol levels and improved stress resistance. Carnevali et al. (2006) found that L. delbrueckii supplementation enhanced growth and reduced stress-related gene expression in sea bass larvae. Mohapatra et al. (2012) demonstrated that probiotic supplementation improved rohu’s growth performance and immunological parameters. Lastly, Merrifield et al. (2010) reviewed the application of probiotics in aquaculture, emphasizing their role in enhancing fish health and immune function.
Paraprobiotics, as highlighted earlier, are nonviable microbial cells, and using these bacteria has several benefits over using live microorganisms (de Almada et al., 2018). The application of paraprobiotics has not gained the same traction as the well-established usage of probiotics in aquaculture (Choudhury and Kamilya, 2019). Many paraprobiotic preparations have been tested in fish and shellfish following the work of Villamil et al. (2002), who reported the immunostimulatory potential of heat-killed Lactococcus lactis in turbot. These investigations mainly aimed to discover how paraprobiotics affect immunological responses, growth, and disease resistance. According to numerous research studies, paraprobiotics can modulate immunity and provide disease resistance just as well as their viable counterparts (Dash et al., 2015; Dawood et al., 2015a). Conversely, compared to their non-viable counterparts, viable microorganisms have been shown to offer greater health advantages (Muñoz-Atienza et al., 2015; Choudhury and Kamilya, 2019). Paraprobiotics are derived from probiotics that have been inactivated through methods such as heat, pressure, or radiation (Lee et al., 2023). Although these cells are no longer viable, they still offer important health benefits, including resistance to antibiotic-resistant pathogens, promoting immune health, and ensuring safety in industrial applications (Abd El-Ghany, 2020; Siciliano et al., 2021). With stability and effectiveness similar to live probiotics, paraprobiotics have a wide range of uses in the food and pharmaceutical industries (Cuevas-González et al., 2020; Vallejo-Cordoba et al., 2020) (See Figure 4).
The Indigenous microbiota is thought to play a crucial role in maintaining the metabolic functions of the digestive tract in aquatic animals (Choudhury and Kamilya, 2019). Feed probiotics, which are viable beneficial microbes, have been shown to improve the appetite of aquatic animals by enhancing digestibility through various mechanisms. For example, they produce enzymes that break down complex nutrients, making them more accessible to the animal’s digestive system, leading to better nutrient absorption and utilization and subsequently stimulating appetite (Dawood et al., 2015a). Paraprobiotics have been found to enhance aquatic animal’s growth performance and feed utilization. Several studies have demonstrated the positive impact of paraprobiotics on growth parameters in fish, including improved growth rate, feed efficiency, weight gain, SGR, feed grain, and protein efficiency ratio (Rodriguez-Estrada et al., 2013; Dawood et al., 2015a, 2015b; Yan et al., 2016). However, not all studies have reported positive results, as heat-killed probiotics (B. subtilis, L. lactis, and S. cerevisiae) added to the diet of rohu did not have a significant impact on growth, protein efficiency ratio, nutrient retention, digestibility, FCR, or gut colonization (Mohapatra et al., 2012). While paraprobiotics have been shown to positively impact various growth parameters in fish, the underlying mechanisms behind this beneficial effect remain unclear and require further investigation.
Paraprobiotics are beneficial to the host because they increase the host’s resistance to pathogenic infections. Although the exact mechanisms by which paraprobiotics inhibit pathogens are unclear, it is generally accepted that immunostimulation is the main mechanism through which the host resists pathogenic microorganisms (Fura et al., 2016; de Almada et al., 2018). Several studies have demonstrated the ability of paraprobiotics to enhance disease resistance in aquatic animals. For example, Villamil et al. (2002) found that heat-killed lactic acid bacteria inhibited the growth of pathogenic V. anguillarum in vitro. Feeding rainbow trout with formalin-killed paraprobiotics reduced mortality after A. salmonicida infection (Irianto and Austin, 2002; Rodriguez-Estrada et al., 2013). Other studies have shown that paraprobiotics can improve resistance to various pathogens, including Edwardsiella tarda in Nile tilapia (Taoka et al., 2006), Aeromonas sp. in rainbow trout (Newaj-Fyzul et al., 2007), V. anguillarum and A. hydrophila in Chinese Drum (Leong, 2008; Pan et al., 2008), V. harveyi in Japanese pufferfish (Biswas et al., 2013b), Flavobacterium psychrophilum in rainbow trout (LaPatra et al., 2014), and A. hydrophila in M. rosenbergii (Dash et al., 2015). Most of these studies also found that paraprobiotics not only enhanced disease resistance but also stimulated the immune system, implying that the immunostimulatory effects of paraprobiotics contribute to their ability to protect against disease.
The function of paraprobiotics in boosting the immune system has been thoroughly studied, especially in models of higher vertebrates (Taverniti and Guglielmetti, 2011; de Almada et al., 2016). Studies conducted in vitro have consistently shown how effective paraprobiotics are at boosting immune responses. For example, it has been demonstrated that exposure to paraprobiotic preparations dramatically increases the myeloperoxidase concentration, phagocytic activity, nitric oxide production, and respiratory burst activity in fish head-kidney leukocytes (Villamil et al., 2002; Kamilya et al., 2015; Choudhury and Kamilya, 2019). In response to paraprobiotic stimulation, the expression of several immune-related genes has been markedly up-regulated. This involves the overexpression of COX-2 and pro-inflammatory cytokines such as IL-1, IL-6, IL-8, IL-17A/F-3, TNF-α, and TNF-β. Furthermore, there has been an upregulation of TGF-β1, IL-2, IL-7, IL-15, IL-21, IL-10, and other regulatory cytokines, as well as cell-mediated immune regulators such as IL-12p35, IL-12p40, and IL-18, and antiviral cytokines such as IFN-α and IFN-γ. There has been a notable up-regulation of defense and antibacterial genes, including granzyme A/K, g-type lysozyme, catalase, phospholipid-hydroperoxide glutathione peroxidase, non-specific cytotoxic cell receptor protein-1, and BPI/LBP, indicating a strong immune response to paraprobiotic stimulation (Caipang et al., 2010; Lazado et al., 2010; Biswas et al., 2013a, 2013b; Román et al., 2013; Sun et al., 2014; Giri et al., 2016). The immune-stimulatory effects of paraprobiotics have been consistently demonstrated across various studies. The up-regulation of immune-related genes and defense genes suggests that paraprobiotics play a crucial role in enhancing the immune system’s response to pathogens. Furthermore, the significant increase in respiratory burst activity, myeloperoxidase content, phagocytic activity, and nitric oxide production in fish head-kidney leukocytes exposed to paraprobiotic preparations in vitro highlights the potential of paraprobiotics as a therapeutic agent in disease prevention and treatment (Choudhury and Kamilya, 2019).
Paraprobiotics can cause a variety of humoral and cellular immunological responses in different fish species, according to in vivo research. Increased levels of serum and gut lysozyme activity, peroxidase content, oxygen radical production, myeloperoxidase activity, alkaline phosphatase activity, natural hemolytic complement activity, a1-antiprotease, immunoglobulin levels, and total serum protein are among these reactions (Taoka et al., 2006; Dash et al., 2015; Dawood et al., 2015a, 2015b; Singh et al., 2017; Choudhury and Kamilya, 2019). Besides that, studies have demonstrated that dietary paraprobiotics improve neutrophil migration, plasma bactericidal activity, phagocytic activity, respiratory burst activity, and cytotoxic activity. They also increase the number of macrophages, lymphocyte populations, acidophilic granulocytes, and gut IgM+ cells (Taoka et al., 2006; Pan et al., 2008; Salinas et al., 2008; Choudhury and Kamilya, 2019). It has been discovered that paraprobiotics stimulate the expression of several immune-relevant genes, such as TLR2, C3, and iNOS genes, as well as pro-inflammatory cytokines, cell-mediated immune regulators, antiviral cytokines, and other regulatory cytokines (Biswas et al., 2013a, 2013b; Yan et al., 2016; Singh et al., 2017). Studies have shown that paraprobiotics can considerably improve immunological parameters in aquatic animals. These results imply that paraprobiotics may be advantageous for immunostimulation on a molecular and cellular level. Several structural elements of the bacterial cell, which have been demonstrated in earlier research to elicit immunological responses, may be responsible for the immunostimulatory qualities of paraprobiotics (Kataria et al., 2009; Adams, 2010; Taverniti and Guglielmetti, 2011; de Almada et al., 2016).
Probiogenomics, a term coined to describe the application of genomic technologies to probiotic research, has emerged as a powerful approach in aquaculture to enhance our understanding of probiotic mechanisms and to develop more effective probiotic strains. This field combines genomics, transcriptomics, proteomics, and metabolomics to elucidate the molecular basis of probiotic effects in aquatic organisms by enhancing host health (Pérez-Sánchez et al., 2014).
One of the primary applications of probiogenomics in aquaculture is the genomic characterization of potential probiotic strains. Whole-genome sequencing of probiotic candidates provides valuable insights into their metabolic capabilities, stress resistance mechanisms, and potential for producing bioactive compounds (Elshaghabee et al., 2017). For instance, a report by Pérez-Sánchez et al. (2011) utilized comparative genomics to identify genes related to adhesion factors, bacteriocin production, and immunomodulatory compounds in L. plantarum strains isolated from fish. This genomic information not only aids in enhancing our understanding of the molecular basis of probiotic effects but also facilitates the development of genetic markers for rapid screening and identification of promising probiotic strains in aquaculture settings.
Probiogenomics also enables the study of host-microbe interactions at a molecular level, providing insights into how probiotics influence fish health and physiology. Transcriptomic analyses of fish intestinal tissue following probiotic administration have revealed modulation of genes involved in immune response, metabolism, and stress tolerance (Hoseinifar et al., 2018). For example, a report by Giri et al. (2018) employed RNA-seq technology to investigate the effects of dietary B. subtilis on the intestinal transcriptome of rohu (L. rohita), identifying significant changes in the expression of genes related to innate immunity and lipid metabolism. Such studies contribute to understanding the mechanisms underlying probiotic benefits and help optimize probiotic applications in aquaculture.
The integration of multi-omics approaches in probiogenomics research is paving the way for a systems biology perspective on probiotic function in aquaculture. Combining genomics with proteomics and metabolomics allows for a comprehensive assessment of probiotic effects on host metabolism and the gut microbiome (Chauhan and Singh, 2019). For instance, Xia et al. (2018) employed a multi-omics approach to investigate L. plantarum’s effects on tilapia’s intestinal health, revealing coordinated changes in microbial community structure, host gene expression, and metabolite profiles. The field of probiogenomics is an emerging field, and its relevance in aquaculture is yet to be established (Lazado and Caipang, 2014). As probiogenomics continues to advance, it promises to revolutionize aquaculture research by enabling next-generation probiotics with enhanced specificity and efficacy, ultimately contributing to improved fish health and growth, and the application of probiogenomics is poised to become a cornerstone in advancing the aquaculture industry. For example, studies have shown that probiotics such as Lactobacillus and Bacillus strains improve immune response and survival rates in fish like rainbow trout, shellfish and other finfish species, which transcends to enhancing their disease resistance (Pérez-Sánchez et al., 2014; Ringø et al., 2020). These advancements provide robust evidence of probiotically enhanced diets benefiting aquaculture (Hai, 2015).
Using probiotics and paraprobiotics in aquaculture requires effective administration methods to ensure they achieve the desired outcomes. Various administration methods have been developed, including incorporating probiotics into fish feed for dosage control (Brunt and Austin, 2005), dissolving probiotics in water (Rakhfid et al., 2020), immersion or bath treatments for specific applications (Hang, 2021), injection (LaPatra et al., 2014), coating probiotics on fish feed pellets for controlled delivery (Zulhisyam et al., 2020), and encapsulation for prolonged release (Huang et al., 2021).
Incorporation into feed whis is one of the most common and practical ways to administer probiotics in fish feed, allows for controlled dosing and ensures that fish receive the probiotics throughout the feeding process. Feed-based administration has been shown to improve growth performance, enhance gut health, and increase disease resistance (Liao and Nyachoti, 2017; Salaheen et al., 2017; Amit et al., 2022). Recent advancements include microencapsulation techniques that protect probiotics during feed processing and ensure their survival in the GIT (Liu et al., 2019; Yao et al., 2020). Microencapsulation also enables a more controlled release of the probiotic organisms, optimizing their effects over time (Sarao and Arora, 2017; Frakolaki et al., 2021; Kowalska et al., 2022). Then, dissolving probiotics into the water or using immersion/bath treatments is particularly effective for larval or juvenile stages of fish, where feeding routines may not be well established (Luz and Favero, 2021; Vargas-González et al., 2024). Recent studies suggest that water-based probiotic administration can enhance immune responses and reduce pathogen load in fish-rearing systems (Yazhiniprabha et al., 2022; Yaslikan et al., 2023). Immersion methods are often used during the early stages of fish development to prevent disease outbreaks (Mohd-Aris et al., 2019; Mondal and Thomas, 2022; Oliveira et al., 2022).
Paraprobiotics, being inactivated microbial cells or cell fractions, often leverage similar administration routes but with specific considerations. Recent studies have shown promising results with heat-killed probiotics incorporated into feed, demonstrating improvements in growth performance, immune function, and disease resistance in various fish species (Dawood et al., 2018; Van Doan et al., 2020). Novel techniques such as spray-drying have been employed to create stable, powder forms of paraprobiotics for easy incorporation into feed or water (Talpur et al., 2014). Importantly, research has begun to explore the co-administration of probiotics and paraprobiotics, revealing potential synergistic effects that could enhance overall fish health and productivity (Azimirad et al., 2016). As the field evolves, there is a growing focus on optimizing dosages, developing targeted delivery systems, and conducting long-term studies to fully understand the impacts of these microbial-based interventions on fish health, growth, and environmental sustainability in aquaculture settings (Hoseinifar et al., 2018).
Nonetheless, the effectiveness of each administration method can be influenced by factors such as the probiotic strain used, fish species, water quality, and environmental conditions (Sayes et al., 2018). So, further research is needed to determine the most appropriate and efficient administration method for a particular aquaculture operation. Several studies have shown that different probiotic administration methods can affect fish’s immune response. For example, a study conducted by Simón et al. (2021) showed that administering probiotics through injection can improve the immune response of fish better than administering probiotics through feed. On the other hand, Rodrigues et al. (2020) showed that encapsulation of probiotics can improve fish survival and reduce stress. In recent years, several new technologies for probiotic administration in aquaculture have been developed, such as using nanoparticles (Qiao et al., 2022) and microencapsulation (Gyawali et al., 2023). Nanoparticles, for example, allow for better bioavailability of probiotics and can target specific areas of the GIT, improving the overall health of fish (Gómez-Guillén and Montero, 2021; Nasr-Eldahan et al., 2021; Vijayaram et al., 2024). Another promising technology is the use of biofilms, where probiotics are embedded into biofilm matrices, allowing them to colonize surfaces in aquaculture systems and provide continuous probiotic effects (Ashrafudoulla et al., 2021; Li et al., 2022). These technologies can improve the effectiveness of probiotic administration and reduce production costs. Thus, further research is necessary to identify the most suitable and efficient probiotic administration methods for specific aquaculture operations, as well as to develop new technologies that can enhance the effectiveness of probiotic administration.
Overcoming challenges in applying probiotics and paraprobiotics in fish farming involves addressing various issues, including stability (Terpou et al., 2019), host specificity, response, and regulatory considerations. Several challenges are associated with the application of probiotics in fish farming, each requiring distinct solutions (Ishthiaq et al., 2021).
First, ensuring probiotic and paraprobiotics stability is critical for their effectiveness, with factors like temperature, moisture, and oxygen sensitivity impacting their viability (Dinkçi et al., 2019). Formulating probiotics with stabilizers, employing encapsulation techniques, and storing them under optimal conditions, such as through microencapsulation, can enhance stability, safeguarding their viability until administration (Sun et al., 2023).
Another challenge lies in the host specificity of probiotics, where the efficacy of a particular strain in one fish species may not be replicated in others (Fontana et al., 2013). Research efforts are needed to identify probiotic strains with broad-spectrum benefits or species-specific formulations, tailoring applications to the unique microbiota of different fish species in aquaculture systems (Das et al., 2022). Variations in individual fish responses pose another challenge, stemming from differences in microbiota, genetics, and environmental conditions within a species (Adamovsky et al., 2018). Thorough trials and individual fish monitoring can help identify these variations. Customizing probiotic formulations or dosages based on individual or population-specific needs can enhance overall efficacy (Lowe et al., 2020).
The regulatory landscape presents challenges as the use of probiotics and paraprobiotics in fish farming is subject to varying frameworks across regions (Lulijwa et al., 2020). Engaging with regulatory authorities, staying informed about guidelines, and conducting research to provide scientific evidence on safety and efficacy are essential steps for navigating these challenges and obtaining regulatory approval. Concerns about the environmental impact of releasing probiotics into aquatic environments, including ecological impacts and resistance development, present challenges. Mitigating risks involves implementing best management practices, such as targeted delivery methods and environmental impact monitoring. Responsible probiotic use, coupled with adherence to environmental regulations, is crucial in addressing these concerns (Hancz, 2022). While paraprobiotics may pose fewer environmental risks due to their inactivated nature, their long-term effects on aquatic ecosystems still need to be studied.
Optimizing dosage and application methods is another challenge, considering factors like feed conversion, water quality, and fish behavior (Yildiz et al., 2017). Conducting controlled studies to determine optimal dosage and application methods based on specific aquaculture conditions is crucial (Bregnballe, 2022). Tailoring probiotic administration to suit the feeding habits and environment of the fish species in question enhances overall efficacy (Wuertz et al., 2021). Addressing these challenges collectively will contribute to the sustainable and effective integration of probiotics in fish farming practices. By addressing these challenges systematically through scientific research, innovation in formulation and application methods, and collaboration with regulatory bodies, the effective integration of probiotics in fish farming can be realized, promoting sustainable and healthy aquaculture practices.
Research on the application of probiotics in fish has undergone significant advancements, with a notable evolution in approaches over the years. A thorough examination of published research on probiotics reveals several key trends: firstly, the use of probiotics has emerged as a viable and sustainable strategy for disease control; secondly, there is an ongoing quest to discover new probiotic candidates; and thirdly, the applications of probiotics have expanded beyond disease control, demonstrating a broader range of benefits (Lazado and Caipang, 2014). In certain situations when probiotics are harmed and/or rendered inert during processing and/or shelf life, the creation of paraprobiotics as supplements and their incorporation into meals and beverages provide a significant substitute. Paraprobiotics will, therefore, have several uses in situations where adding probiotics ought to solve a technological problem. Parapobiotic products, as opposed to live probiotic goods, do enable the creation of safer and more stable products. Consequently, the use of paraprobiotics in food, medicine, supplements, and fodder is rapidly growing, and soon, their use will be widespread. Recent research has indicated that the biogenic and paraprobiotic properties of dead cells, microbial fractions, or cell lysates can preserve the host’s health.
Envisioning the future of probiotics in fisheries necessitates comprehensively exploring research gaps, novel strains, and innovative delivery systems (Vieira et al., 2021). Addressing research gaps is pivotal in understanding fish microbiomes to identify bacterial strains beneficial for different species. The focus should extend to characterizing the gut microbiota of various fish and comprehending how probiotics interact with existing microbial communities over time (Legrand et al., 2020). Investigating the long-term effects of probiotic administration is equally crucial, delving into sustained benefits, potential host microbiota adaptation, and any unintended consequences.
Exploring new strains is vital for enhancing the spectrum of benefits offered by probiotics. This involves isolating unique microbial strains with superior properties, including stress tolerance, antimicrobial activity, and positive effects on growth and immune responses (Kwoji et al., 2021). Customizing probiotic formulations for different fish species is essential, requiring research to understand species-specific requirements and preferences.
Innovative delivery systems play a key role in shaping the future of probiotics. Advancements in microencapsulation techniques can enhance stability and targeted delivery, with research exploring new materials and methods to improve probiotic survival in the digestive system (Vivek et al., 2023). Exploring nanotechnology applications offers innovative possibilities, as well as investigating nanoencapsulation methods to improve bioavailability and controlled release (Chadha, 2021). Developing precision delivery systems, such as smart feed formulations or site-specific administration, can optimize distribution, minimize environmental impact, and enhance overall effectiveness (Karunathilake et al., 2023).
Ecosystem-level studies are paramount for sustainable aquaculture practices. Assessing the broader ecological impact of probiotics on aquatic ecosystems is vital in understanding interactions with non-target species and environmental factors (Ibrahem, 2013). Investigating the potential for antibiotic resistance and horizontal gene transfer among probiotic strains and native microorganisms ensures responsible probiotic use (Rossi et al., 2014).
Integrating omics technologies (Kwoji et al., 2023), including genomics, metagenomics, and proteomics, provides comprehensive insights into the genetic makeup of probiotic strains, host responses, and microbial interactions. This integrative approach enhances our understanding of the mechanisms underlying probiotic functionality, paving the way for informed and sustainable advancements in probiotics within fisheries.
To summarize, probiotics and paraprobiotics are increasingly recognized as valuable alternatives to antibiotics in aquaculture, offering numerous benefits for fish health and disease prevention. These beneficial microorganisms help modulate the gut microbiota, enhancing digestion, nutrient absorption, and immune responses in fish. Probiotics, which are live microorganisms, produce antimicrobial compounds such as bacteriocins and organic acids that inhibit the growth of pathogens. At the same time, paraprobiotics, composed of inactivated cells, provide similar benefits through their bioactive components. Both probiotics and paraprobiotics strengthen the immune system, improving disease resistance against bacterial, viral, and parasitic infections. Additionally, probiogenomics enables the development of more targeted and effective probiotic strains tailored to specific fish species and environmental conditions. Commercially available products utilizing these microorganisms already show promising results in improving fish growth, survival rates, and water quality, making them essential components in sustainable aquaculture practices.
MF: Conceptualization, Data curation, Methodology, Validation, Writing – original draft. KA: Conceptualization, Data curation, Funding acquisition, Methodology, Writing – original draft, Writing – review & editing. YH: Methodology, Supervision, Writing – review & editing. JC: Project administration, Supervision, Writing – review & editing. AA: Writing - review & editing, Formal analysis. CN: Formal analysis, Writing – review & editing. VS: Formal analysis, Writing – review & editing. XJ: Formal analysis, Writing – review & editing. FB: Formal analysis, Writing – review & editing. HC: Conceptualization, Funding acquisition, Methodology, Resources, Supervision, Writing – review & editing.
The author(s) declare financial support was received for the research, authorship, and/or publication of this article. The research leading to these results was financially supported by the National Natural Science Foundation of China (32273131), the Program for Scientific Research Start-up Funds of Guangdong Ocean University (060302022310), the Key Research and Development Program of Guangdong (2021B02020200020), the Science and Technology Plan of Guangdong Province (2023B0202010016), the Youth Science and Technology Innovation Talent of Guangdong TeZhi plan talent (2023TQ07A888), the Research on breeding technology of candidate species for Guangdong modern marine ranching (2024-MRB-00-001) and the Science and Technology Plan of Zhanjiang City (2024E03007).
The authors declare that the research was conducted in the absence of any commercial or financial relationships that could be construed as a potential conflict of interest.
All claims expressed in this article are solely those of the authors and do not necessarily represent those of their affiliated organizations, or those of the publisher, the editors and the reviewers. Any product that may be evaluated in this article, or claim that may be made by its manufacturer, is not guaranteed or endorsed by the publisher.
Abarike E. D., Cai J., Lu Y., Yu H., Chen L., Jian J., et al. (2018). Effects of a commercial probiotic BS containing Bacillus subtilis and Bacillus licheniformis on growth, immune response and disease resistance in Nile tilapia, Oreochromis niloticus. Fish Shellfish Immunol. 82, 229–238. doi: 10.1016/j.fsi.2018.08.037
Abdel-Aziz M., Bessat M., Fadel A., Elblehi S. (2020). Responses of dietary supplementation of probiotic effective microorganisms (EMs) in Oreochromis niloticus on growth, hematological, intestinal histopathological, and antiparasitic activities. Aquac. Int. 28. doi: 10.1007/s10499-019-00505-z
Abd El-Ghany W. A. (2020). Paraprobiotics and postbiotics: Contemporary and promising natural antibiotics alternatives and their applications in the poultry field. Open Vet. J. 10 (3), 323–330. doi: 10.4314/ovj.v10i3.11
Abdulmawjood A., Herrmann J., Riede S., Jimenez G., Becker A., Breves G. (2019). Evaluation of enterotoxin gene expression and enterotoxin production capacity of the probiotic strain Bacillus toyonensis BCT-7112T. PloS One 14. doi: 10.1371/journal.pone.0214536
Abid A., Davies S. J., Waines P., Emery M., Castex M., Gioacchini G., et al. (2013). Dietary synbiotic application modulates Atlantic salmon (Salmo salar) intestinal microbial communities and intestinal immunity. Fish Shellfish Immunol. 35. doi: 10.1016/j.fsi.2013.09.039
Abou-El-Atta M. E., Abdel-Tawwab M., Abdel-Razek N., Abdelhakim T. M. N. (2019). Effects of dietary probiotic Lactobacillus plantarum and whey protein concentrate on the productive parameters, immunity response and susceptibility of Nile tilapia, Oreochromis niloticus (L.), to Aeromonas sobria infection. Aquac. Nutr. 25. doi: 10.1111/anu.12957
Adamovsky O., Buerger A. N., Wormington A. M., Ector N., Griffitt R. J., Bisesi J. H., et al. (2018). The gut microbiome and aquatic toxicology: An emerging concept for environmental health. Environ. Toxicol. Chem. 37, 2758–2775. doi: 10.1002/etc.4249
Adams C. A. (2010). The probiotic paradox: Live and dead cells are biological response modifiers. Nutr. Res. Rev. 23. doi: 10.1017/S0954422410000090
Adorian T. J., Jamali H., Farsani H. G., Darvishi P., Hasanpour S., Bagheri T., et al. (2019). Effects of probiotic bacteria Bacillus on growth performance, digestive enzyme activity, and hematological parameters of asian sea bass, Lates calcarifer (Bloch). Probiotics Antimicrob. Proteins 11. doi: 10.1007/s12602-018-9393-z
Aguilar-Toalá J. E., Garcia-Varela R., Garcia H. S., Mata-Haro V., González-Córdova A. F., Vallejo-Cordoba B., et al. (2018). Postbiotics: An evolving term within the functional foods field. Trends Food Sci. Technol. 75. doi: 10.1016/j.tifs.2018.03.009
Akter S., Park J. H., Jung H. K. (2020). Potential Health-promoting benefits of paraprobiotics, inactivated probiotic cells. J. Microbiol. Biotechnol. 30. doi: 10.4014/JMB.1911.11019
Al-Dohail M. A., Hashim R., Aliyu-Paiko M. (2011). Evaluating the use of Lactobacillus acidophilus as a biocontrol agent against common pathogenic bacteria and the effects on the haematology parameters and histopathology in African catfish Clarias gariepinus juveniles. Aquac. Res. 42. doi: 10.1111/j.1365-2109.2010.02606.x
Aly S. M., Abdel-Galil Ahmed Y., Abdel-Aziz Ghareeb A., Mohamed M. F. (2008b). Studies on Bacillus subtilis and Lactobacillus acidophilus, as potential probiotics, on the immune response and resistance of Tilapia nilotica (Oreochromis niloticus) to challenge infections. Fish Shellfish Immunol. 25. doi: 10.1016/j.fsi.2008.03.013
Aly S. M., Abd-El-Rahman A. M., John G., Mohamed M. F. (2008a). Characterization of Some Bacteria Isolated from Oreochromis niloticus and their Potential Use as Probiotics. Aquaculture 277. doi: 10.1016/j.aquaculture.2008.02.021
Amit, Pandey A., Tyagi A., Khairnar S. O. (2022). Oral feed-based administration of Lactobacillus plantarum enhances growth, haematological and immunological responses in Cyprinus carpio. Emerg. Anim. Species 3. doi: 10.1016/j.eas.2022.100003
Amoah K., Cai J., Huang Y., Wang B., Shija V. M., Wang Z., et al. (2024). Identification and characterization of four Bacillus species from the intestine of hybrid grouper (Epinephelus fuscoguttatus♀ × E. lanceolatus♂), their antagonistic role on common pathogenic bacteria, and effects on intestinal health. Fish Shellfish Immunol. 152, 109795. doi: 10.1016/j.fsi.2024.109795
Amoah K., Dong X., Tan B., Zhang S., Chi S., Yang Q., et al. (2021). Effects of three probiotic strains (Bacillus coagulans, B. licheniformis and Paenibacillus polymyxa) on growth, immune response, gut morphology and microbiota, and resistance against Vibrio harveyi of northern whitings, Sillago sihama Forsská. Anim. Feed Sci. Technol. 277, 114958. doi: 10.1016/j.anifeedsci.2021.114958
Amoah K., Tan B., Zhang S., Chi S., Yang Q., Liu H., et al. (2023). Host gut-derived Bacillus probiotics supplementation improves growth performance, serum and liver immunity, gut health, and resistive capacity against Vibrio harveyi infection in hybrid grouper (♀Epinephelus fuscoguttatus × ♂Epinephelus lanceolatus). Anim. Nutr. 14, 163–184. doi: 10.1016/j.aninu.2023.05.005
Andrews N., Bennett N. J., Le Billon P., Green S. J., Cisneros-Montemayor A. M., Amongin S., et al. (2021). Oil, fisheries and coastal communities: A review of impacts on the environment, livelihoods, space and governance. Energy Res. Soc Sci. 75. doi: 10.1016/j.erss.2021.102009
Araújo C., Muñoz-Atienza E., Hernández P. E., Herranz C., Cintas L. M., Igrejas G., et al. (2015). Evaluation of Enterococcus spp. from rainbow trout (Oncorhynchus mykiss, Walbaum), feed, and rearing environment against fish pathogens. Foodborne Pathog. Dis. 12. doi: 10.1089/fpd.2014.1906
Arjes H. A., Gui H., Porter R., Atolia E., Peters J. M., Gross C., et al. (2022). Fatty acid synthesis knockdown promotes biofilm wrinkling and inhibits sporulation in bacillus subtilis. MBio 13. doi: 10.1128/mbio.01388-22
Arunkumar M., LewisOscar F., Thajuddin N., Pugazhendhi A., Nithya C. (2020). In vitro and in vivo biofilm forming Vibrio spp: A significant threat in aquaculture. Process Biochem. 94. doi: 10.1016/j.procbio.2020.04.029
Ashaolu T. J. (2020). Immune boosting functional foods and their mechanisms: A critical evaluation of probiotics and prebiotics. Biomed. Pharmacother. 130, 110625. doi: 10.1016/j.biopha.2020.110625
Ashrafudoulla M., Mizan M. F. R., Park S. H., Ha S. D. (2021). Current and future perspectives for controlling Vibrio biofilms in the seafood industry: a comprehensive review. Crit. Rev. Food Sci. Nutr. 61. doi: 10.1080/10408398.2020.1767031
Azimirad M., Meshkini S., Ahmadifard N., Hoseinifar S. H. (2016). The effects of feeding with synbiotic (Pediococcus acidilactici and fructooligo saccharide) enriched adult Artemia on skin mucus immune responses, stress resistance, intestinal microbiota and performance of angelfish (Pterophyllum scalare). Fish Shellfish Immunol. 54. doi: 10.1016/j.fsi.2016.05.001
Bajagai Y. S., Klieve A. V., Dart P. J., Bryden W. L. (2016). Probiotics in animal nutrition – Production, impact and regulation (Rome, Italy: Food & Agriculture Organization of the United Nations).
Bajpai V. K., Chandra V., Kim N. H., Rai R., Kumar P., Kim K., et al. (2018). Ghost probiotics with a combined regimen: a novel therapeutic approach against the Zika virus, an emerging world threat. Crit. Rev. Biotechnol. 38. doi: 10.1080/07388551.2017.1368445
Balcázar J. L., de Blas I., Ruiz-Zarzuela I., Cunningham D., Vendrell D., Múzquiz J. L. (2006). The role of probiotics in aquaculture. Vet. Microbiol. 114, 173–186. doi: 10.1016/j.vetmic.2006.01.009
Balcázar J. L., Vendrell D., de Blas I., Ruiz-Zarzuela I., Muzquiz J. L., Girones O. (2008). Characterization of probiotic properties of lactic acid bacteria isolated from intestinal microbiota of fish. Aquaculture 278. doi: 10.1016/j.aquaculture.2008.03.014
Banerjee G., Ray A. K. (2017). The advancement of probiotics research and its application in fish farming industries. Res. Vet. Sci. 115. doi: 10.1016/j.rvsc.2017.01.016
Barui K., Choudhury T. G., Kamilya D., Devi A. A., Monsang S. J., Rathore G., et al. (2024). Paraprobiotic supplementation to fish feed: effects on the immune support system and control of Aeromonas hydrophila infection in Labeo rohita. Aquac. Int. 32, 1–15. doi: 10.1007/s10499-023-01372-5
Beck B. R., Kim D., Jeon J., Lee S. M., Kim H. K., Kim O. J., et al. (2015). The effects of combined dietary probiotics Lactococcus lactis BFE920 and Lactobacillus plantarum FGL0001 on innate immunity and disease resistance in olive flounder (Paralichthys olivaceus). Fish Shellfish Immunol. 42. doi: 10.1016/j.fsi.2014.10.035
Benhamed S., Guardiola F. A., Mars M., Esteban M.Á. (2014). Pathogen bacteria adhesion to skin mucus of fishes. Vet. Microbiol. 171. doi: 10.1016/j.vetmic.2014.03.008
Bermudez-Brito M., Plaza-Díaz J., Muñoz-Quezada S., Gómez-Llorente C., Gil A. (2012). Probiotic mechanisms of action. Ann. Nutr. Metab. 61, 160–174. doi: 10.1159/000342079
Biswas G., Korenaga H., Nagamine R., Kawahara S., Takeda S., Kikuchi Y., et al. (2013a). Cytokine mediated immune responses in the Japanese pufferfish (Takifugu rubripes) administered with heat-killed Lactobacillus paracasei spp. paracasei (06TCa22) isolated from the Mongolian dairy product. Int. Immunopharmacol. 17, 358–365. doi: 10.1016/j.intimp.2013.06.030
Biswas G., Korenaga H., Nagamine R., Takayama H., Kawahara S., Takeda S., et al. (2013b). Cytokine responses in the Japanese pufferfish (Takifugu rubripes) head kidney cells induced with heat-killed probiotics isolated from the Mongolian dairy products. Fish Shellfish Immunol. 34. doi: 10.1016/j.fsi.2013.01.024
Bregnballe J. (2022). A guide to recirculation aquaculture: an introduction to the new environmentally friendly and highly productive closed fish farming systems. Rome, Italy: Food & Agriculture Organization. doi: 10.4060/cc2390en
Bruce T. J., Brown M. L. (2017). A review of immune system components, cytokines, and immunostimulants in cultured finfish species. Open J. Anim. Sci. 07. doi: 10.4236/ojas.2017.73021
Brunt J., Austin B. (2005). Use of a probiotic to control lactococcosis and streptococcosis in rainbow trout, Oncorhynchus mykiss (Walbaum). J. Fish Dis. 28, 693–701. doi: 10.1111/j.1365-2761.2005.00672.x
Brunt J., Newaj-Fyzul A., Austin B. (2007). The development of probiotics for the control of multiple bacterial diseases of rainbow trout, Oncorhynchus mykiss (Walbaum). J. Fish Dis. 30. doi: 10.1111/j.1365-2761.2007.00836.x
Butt R. L., Volkoff H. (2019). Gut microbiota and energy homeostasis in fish. Front. Endocrinol. (Lausanne). 10. doi: 10.3389/fendo.2019.00009
Cai W., Arias C. R. (2017). Biofilm formation on aquaculture substrates by selected bacterial fish pathogens. J. Aquat. Anim. Health 29. doi: 10.1080/08997659.2017.1290711
Cai X., Wen J., Long H., Ren W., Zhang X., Huang A., et al. (2022). The probiotic effects, dose, and duration of lactic acid bacteria on disease resistance in Litopenaeus vannamei. Aquac. Rep. 26, 101299. doi: 10.1016/j.aqrep.2022.101299
Caipang C. M. A., Brinchmann M. F., Kiron V. (2010). Antagonistic activity of bacterial isolates from intestinal microbiota of Atlantic cod, Gadus morhua, and an investigation of their immunomodulatory capabilities. Aquac. Res. 41. doi: 10.1111/j.1365-2109.2009.02327.x
Cámara-Ruiz M., Balebona M. C., Esteban M.Á., Moriñigo M.Á. (2020). Probiotic Shewanella putrefaciens (SPPDP11) as a fish health modulator: A review. Microorganisms 8, 1–19. doi: 10.3390/microorganisms8121990
Carnevali O., de Vivo L., Sulpizio R., Gioacchini G., Olivotto I., Silvi S., et al. (2006). Growth improvement by probiotic in European sea bass juveniles (Dicentrarchus labrax, L.), with particular attention to IGF-1, myostatin and cortisol gene expression. Aquaculture 258. doi: 10.1016/j.aquaculture.2006.04.025
Carnevali O., Maradonna F., Gioacchini G. (2017). Integrated control of fish metabolism, wellbeing and reproduction: The role of probiotic. Aquaculture 472. doi: 10.1016/j.aquaculture.2016.03.037
Casillas-Hernández R., Gonzalez-Galaviz J. R., Rodriguez-Anaya L. Z., Gil-Núñez J. C., Rodríguez-Jaramillo M.d. C. (2023). Dietary Use of Methionine Sources and Bacillus amyloliquefaciens CECT 5940 Influences Growth Performance, Hepatopancreatic Histology, Digestion, Immunity, and Digestive Microbiota of Litopenaeus vannamei Fed Reduced Fishmeal Diets. Animals 13. doi: 10.3390/ani13010043
Cavera V. L., Arthur T. D., Kashtanov D., Chikindas M. L. (2015). Bacteriocins and their position in the next wave of conventional antibiotics. Int. J. Antimicrob. Agents 46. doi: 10.1016/j.ijantimicag.2015.07.011
Chadha S. (2021). “Recent advances in nano-encapsulation technologies for controlled release of biostimulants and antimicrobial agents,” in Advances in nanofertilizers and nano-pesticides in agriculture: A smart delivery system for crop improvement. (Amsterdam, Netherlands: Elsevier). pp. 29–55. doi: 10.1016/B978-0-12-820092-6.00002-1
Chattaraj S., Ganguly A., Mandal A., Das Mohapatra P. K. (2022). A review of the role of probiotics for the control of viral diseases in aquaculture. Aquac. Int. 30, 2513–2539. doi: 10.1007/s10499-022-00915-6
Chauhan A., Singh R. (2019). Probiotics in aquaculture: a promising emerging alternative approach. Symbiosis 77. doi: 10.1007/s13199-018-0580-1
Che J., Shi J., Fang C., Zeng X., Wu Z., Du Q., et al. (2024). Elimination of pathogen biofilms via postbiotics from lactic acid bacteria: A promising method in food and biomedicine. Microorganisms 12. doi: 10.3390/microorganisms12040704
Chi C., Jiang B., Yu X. B., Liu T. Q., Xia L., Wang G. X. (2014). Effects of three strains of intestinal autochthonous bacteria and their extracellular products on the immune response and disease resistance of common carp, Cyprinus carpio. Fish Shellfish Immunol. 36. doi: 10.1016/j.fsi.2013.10.003
Choudhury T. G., Kamilya D. (2019). Paraprobiotics: an aquaculture perspective. Rev. Aquac. 11. doi: 10.1111/raq.12290
Chowdhury G., Hossain M. S., Dey T., Akhtar S., Jinia M. A., Das B., et al. (2020). Effects of dietary probiotics on the growth, blood chemistry and stress response of Pabda catfish (Ompok pabda) juveniles. AACL Bioflux 13(3), 1595–1605.
Chuah L. O., Foo H. L., Loh T. C., Mohammed Alitheen N. B., Yeap S. K., Abdul Mutalib N. E., et al. (2019). Postbiotic metabolites produced by Lactobacillus plantarum strains exert selective cytotoxicity effects on cancer cells. BMC Complement. Altern. Med. 19. doi: 10.1186/s12906-019-2528-2
Cuevas-González P. F., Liceaga A. M., Aguilar-Toalá J. E. (2020). Postbiotics and paraprobiotics: From concepts to applications. Food Res. Int. 136. doi: 10.1016/j.foodres.2020.109502
Das T. K., Pradhan S., Chakrabarti S., Mondal K. C., Ghosh K. (2022). Current status of probiotic and related health benefits. Appl. Food Res. 2, 100185. doi: 10.1016/j.afres.2022.100185
Dash G., Raman R. P., Pani Prasad K., Makesh M., Pradeep M. A., Sen S. (2015). Evaluation of paraprobiotic applicability of Lactobacillus plantarum in improving the immune response and disease protection in giant freshwater prawn, Macrobrachium rosenbergii (de Man 1879). Fish Shellfish Immunol. 43. doi: 10.1016/j.fsi.2014.12.007
Dawood M. A. O., Abo-Al-Ela H. G., Hasan M. T. (2020). Modulation of transcriptomic profile in aquatic animals: Probiotics, prebiotics and synbiotics scenarios. Fish Shellfish Immunol. 97. doi: 10.1016/j.fsi.2019.12.054
Dawood M. A. O., Koshio S., Abdel-Daim M. M., Van Doan H. (2019). Probiotic application for sustainable aquaculture. Rev. Aquac. 11, 907–924. doi: 10.1111/raq.12272
Dawood M. A. O., Koshio S., Esteban M.Á. (2018). Beneficial roles of feed additives as immunostimulants in aquaculture: a review. Rev. Aquac. 10. doi: 10.1111/raq.12209
Dawood M. A. O., Koshio S., Ishikawa M., Yokoyama S. (2015a). Effects of heat killed Lactobacillus plantarum (LP20) supplemental diets on growth performance, stress resistance and immune response of red sea bream, pagrus major. Aquaculture 442. doi: 10.1016/j.aquaculture.2015.02.005
Dawood M. A. O., Koshio S., Ishikawa M., Yokoyama S. (2015b). Effects of partial substitution of fish meal by soybean meal with or without heat-killed Lactobacillus plantarum (LP20) on growth performance, digestibility, and immune response of amberjack, Seriola dumerili juveniles. BioMed. Res. Int. 2015. doi: 10.1155/2015/514196
De D., Ghoshal T. K., Ananda Raja R., Kumar S. (2015). Growth performance, nutrient digestibility and digestive enzyme activity in Asian seabass, Lates calcarifer juveniles fed diets supplemented with cellulolytic and amylolytic gut bacteria isolated from brackishwater fish. Aquac. Res. 46, 1688–1698. doi: 10.1111/are.12325
de Almada C. N., Almada C. N., Martinez R. C. R., Sant’Ana A. S. (2016). Paraprobiotics: Evidences on their ability to modify biological responses, inactivation methods and perspectives on their application in foods. Trends Food Sci. Technol. 58. doi: 10.1016/j.tifs.2016.09.011
de Almada C. N., de Almada C. N., de Sant’Ana A. S. (2018). “Paraprobiotics as potential agents for improving animal health,” in Probiotics and prebiotics in animal health and food safety. Cambridge, MA: Academic Press. pp. 247–268. doi: 10.1007/978-3-319-71950-4_10
de Moraes A. V., Owatari M. S., da Silva E., de Oliveira Pereira M., Piola M., Ramos C., et al. (2022). Effects of microencapsulated probiotics-supplemented diet on growth, non-specific immunity, intestinal health and resistance of juvenile Nile tilapia challenged with Aeromonas hydrophila. Anim. Feed Sci. Technol. 287, 115286. doi: 10.1016/j.anifeedsci.2022.115286
Denev S., Staykov Y., Moutafchieva R., Beev G. (2009). International Aquatic Research Microbial ecology of the gastrointestinal tract of fish and the potential application of probiotics and prebiotics in finfish aquaculture. Int. Aquat. Res. Int. Aquat Res. 1, 1–29.
Dinkçi N., Akdeniz V., Akalin A. S. (2019). Survival of probiotics in functional foods during shelf life. In Food quality and shelf life (San Diego, CA: Elsevier Inc). pp. 201–233. doi: 10.1016/B978-0-12-817190-5.00006-9
Dubois J. Y. F., Kouwen T. R. H. M., Schurich A. K. C., Reis C. R., Ensing H. T., Trip E. N., et al. (2009). Immunity to the bacteriocin sublancin 168 is determined by the SunI (YolF) protein of Bacillus subtilis. Antimicrob. Agents Chemother. 53. doi: 10.1128/AAC.01189-08
El-Ezabi M., El- Serafy S., Essa M., Daboor S., Esmael N. (2011). The viability of probiotics as a factor influencing the immune response in the Nile tilapia, Oreochromis niloticus. Egypt. J. Aquat. Biol. Fish. 15. doi: 10.21608/ejabf.2011.2081
El-Haroun E. R., Goda A. M. A. S., Kabir Chowdhury M. A. (2006). Effect of dietary probiotic Biogen® supplementation as a growth promoter on growth performance and feed utilization of Nile tilapia Oreochromis niloticus (L.). Aquac. Res. 37. doi: 10.1111/j.1365-2109.2006.01584.x
El-Saadony M. T., Alagawany M., Patra A. K., Kar I., Tiwari R., Dawood M. A. O., et al. (2021). The functionality of probiotics in aquaculture: An overview. Fish Shellfish Immunol. 117. doi: 10.1016/j.fsi.2021.07.007
Elsadek M. M., Wang S., Wu Z., Wang J., Wang X., Zhang Y., et al. (2023). Characterization of Bacillus spp. isolated from the intestines of Rhynchocypris lagowskii as a potential probiotic and their effects on fish pathogens. Microb. Pathog. 180, 106163. doi: 10.1016/j.micpath.2023.106163
Elshaghabee F. M. F., Rokana N., Gulhane R. D., Sharma C., Panwar H. (2017). Bacillus as potential probiotics: Status, concerns, and future perspectives. Front. Microbiol. 8. doi: 10.3389/fmicb.2017.01490
Endres J. R., Clewell A., Jade K. A., Farber T., Hauswirth J., Schauss A. G. (2009). Safety assessment of a proprietary preparation of a novel Probiotic, Bacillus coagulans, as a food ingredient. Food Chem. Toxicol. 47. doi: 10.1016/j.fct.2009.02.018
Falcinelli S., Rodiles A., Unniappan S., Picchietti S., Gioacchini G., Merrifield D. L., et al. (2016). Probiotic treatment reduces appetite and glucose level in the zebrafish model. Sci. Rep. 6. doi: 10.1038/srep18061
FAO (2014). The state of world fisheries and aquaculture: Challenges and Opportunities. Rome, Italy: Food and Agriculture Organization of the United Nations.
Feliatra F., Muchlisin Z. A., Teruna H. Y., Utamy W. R., Nursyirwani N., Dahliaty A. (2018). Potential of bacteriocins produced by probiotic bacteria isolated from tiger shrimp and prawns as antibacterial to Vibrio, Pseudomonas, and Aeromonas species on fish. F1000Research 7. doi: 10.12688/F1000RESEARCH.13958.1
Feliatra, Yoswaty D., Lukystyowati I., Nugroho T. T., Hasyimi W. (2015). The potential of the isolated probiotics bacterial from giant prawns’ digestive tract (Macrobrachium rosenbergii, de Man) with 16s Rdna Sequencing Technique. Int. J. Ocean. Oceanogr. 9. doi: 10.21534/ai.v15i2.33
Fernandes A., Jobby R. (2022). Bacteriocins from lactic acid bacteria and their potential clinical applications. Appl. Biochem. Biotechnol. 194, 1–15. doi: 10.1007/s12010-022-03870-3
Flegel T. W., Pasharawipas T. (1998). “Advances in shrimp biotechnology,” in Proceedings of the National Centre for Genetic Engineering and Biotechnology Thailand. Bangkok, Thailand: National Centre for Genetic Engineering and Biotechnology. pp. 245–250.
Fontana L., Bermudez-Brito M., Plaza-Diaz J., Muñoz-Quezada S., Gil A. (2013). Sources, isolation, characterisation and evaluation of probiotics. Br. J. Nutr. 109. doi: 10.1017/S0007114512004011
Frakolaki G., Giannou V., Kekos D., Tzia C. (2021). A review of the microencapsulation techniques for the incorporation of probiotic bacteria in functional foods. Crit. Rev. Food Sci. Nutr. 61. doi: 10.1080/10408398.2020.1761773
Fura J., Sarkar S., Pidgeon S., Pires M. (2016). Combatting bacterial pathogens with immunomodulation and infection tolerance strategies. Curr. Top. Med. Chem. 17, 1–15. doi: 10.2174/1568026616666160829160707
Galvez A., Maqueda M., Valdivia E., Quesada A., Montoya E. (1986). Characterization and partial purification of a broad spectrum antibiotic AS-48 produced by Streptococcus faecalis. Can. J. Microbiol. 32. doi: 10.1139/m86-141
Garg N., Manchanda G., Kumar A. (2014). Bacterial quorum sensing: Circuits and applications. Antonie van Leeuwenhoek. Int. J. Gen. Mol. Microbiol. 105. doi: 10.1007/s10482-013-0082-3
Gatesoupe F. (1999). The use of probiotics in aquaculture. Aquaculture 180, 147–165. doi: 10.1016/S0044-8486(99)00187-8
Ghori I., Tubassam M., Ahmad T., Zuberi A., Imran M. (2022). Gut microbiome modulation mediated by probiotics: Positive impact on growth and health status of Labeo rohita. Front. Physiol. 13. doi: 10.3389/fphys.2022.949559
Gioacchini G., Maradonna F., Lombardo F., Bizzaro D., Olivotto I., Carnevali O. (2010). Increase of fecundity by probiotic administration in zebrafish (Danio rerio). Reproduction 140. doi: 10.1530/REP-10-0145
Giri S. S., Sen S. S., Jun J. W., Park S. C., Sukumaran V. (2016). Heat-killed whole-cell products of the probiotic Pseudomonas aeruginosa VSG2 strain affect in vitro cytokine expression in head kidney macrophages of Labeo rohita. Fish Shellfish Immunol. 50. doi: 10.1016/j.fsi.2016.02.007
Giri S. S., Sen S. S., Saha S., Sukumaran V., Park S. C. (2018). Use of a potential probiotic, Lactobacillus plantarum L7, for the preparation of a rice-based fermented beverage. Front. Microbiol. 9. doi: 10.3389/fmicb.2018.00473
Giri S. S., Sukumaran V., Oviya M. (2013). Potential probiotic Lactobacillus plantarum VSG3 improves the growth, immunity, and disease resistance of tropical freshwater fish, Labeo rohita. Fish Shellfish Immunol. 34. doi: 10.1016/j.fsi.2012.12.008
Goh J. X. H., Tan L. T. H., Law J. W. F., Khaw K. Y., Zengin G., Chan K. G., et al. (2023). Probiotics: comprehensive exploration of the growth promotion mechanisms in shrimps. Prog. Microbes Mol. Biol. 6. doi: 10.36877/pmmb.a0000324
Gómez-Guillén M. C., Montero M. P. (2021). Enhancement of oral bioavailability of natural compounds and probiotics by mucoadhesive tailored biopolymer-based nanoparticles: A review. Food Hydrocoll. 118. doi: 10.1016/j.foodhyd.2021.106772
Gram L., Melchiorsen J., Spanggaard B., Huber I., Nielsen T. F. (1999). Inhibition of Vibrio Anguillarum by Pseudomonas fluorescens AH2, a possible probiotic treatment of fish. Appl. Environ. Microbiol. 65. doi: 10.1128/aem.65.3.969-973.1999
Gray E. J., Di Falco M., Souleimanov A., Smith D. L. (2006). Proteomic analysis of the bacteriocin thuricin 17 produced by Bacillus thuringiensis NEB17. FEMS Microbiol. Lett. 255. doi: 10.1111/j.1574-6968.2005.00054.x
Gyawali I., Zhou G., Xu G., Li G., Wang Y., Zeng Y., et al. (2023). Supplementation of microencapsulated probiotics modulates gut health and intestinal microbiota. Food Sci. Nutr. 11. doi: 10.1002/fsn3.3414
Hai N. V. (2015). The use of probiotics in aquaculture. J. Appl. Microbiol. 119, 917–935. doi: 10.1111/jam.12886
Hajirezaee S., Khanjani M. H. (2023). Rosmarinic acid alone or in combination with Lactobacillus rhamnosus ameliorated resistance to ammonia stress in the rainbow trout, Oncorhynchus mykiss: Growth, immunity, antioxidant defense and liver functions. Ann. Anim. Sci. 23. doi: 10.2478/aoas-2023-0024
Hamdan A. M., El-Sayed A. F. M., Mahmoud M. M. (2016). Effects of a novel marine probiotic, Lactobacillus plantarum AH 78, on growth performance and immune response of Nile tilapia (Oreochromis niloticus). J. Appl. Microbiol. 120. doi: 10.1111/jam.13081
Han B., Long W. Q., He J. Y., Liu Y. J., Si Y. Q., Tian L. X. (2015). Effects of dietary Bacillus licheniformis on growth performance, immunological parameters, intestinal morphology and resistance of juvenile Nile tilapia (Oreochromis niloticus) to challenge infections. Fish Shellfish Immunol. 46. doi: 10.1016/j.fsi.2015.06.018
Han C., Song S., Cui C., Cai Y., Zhou Y., Wang J., et al (2024). Strain-specific benefits of bacillus probiotics in hybrid grouper: growth enhancement, metabolic health, immune modulation, and vibrio harveyi resistance. Animals 14 (7), 1062. doi: 10.3390/ani14071062
Hancz C. (2022). Application of probiotics for environmentally friendly and sustainable aquaculture: A review. Sustain. 14. doi: 10.3390/su142215479
Hang M. (2021). The probiotic potential of lactic acid bacteria (LAB) isolated from naniura (a traditional Batak food). Canrea J. Food Technol. Nutr. Culin. J. 4, 68–74. doi: 10.20956/canrea.v4i1.444
Haque R., Das I. I., Sawant P. B., Chadha N. K., Sahoo L., Kumar R., et al. (2022). Tenets in microbial endocrinology: A new vista in teleost reproduction. Front. Physiol. 13. doi: 10.3389/fphys.2022.871045
Harikrishnan R., Kim M. C., Kim J. S., Balasundaram C., Heo M. S. (2011). Protective effect of herbal and probiotics enriched diet on haematological and immunity status of Oplegnathus fasciatus (Temminck & Schlegel) against Edwardsiella tarda. Fish Shellfish Immunol. 30. doi: 10.1016/j.fsi.2011.01.013
Hasan I., Rimoldi S., Saroglia G., Terova G. (2023). Sustainable fish feeds with insects and probiotics positively affect freshwater and marine fish gut microbiota. Animals 13. doi: 10.3390/ani13101633
Hasan K. N., Banerjee G. (2020). Recent studies on probiotics as beneficial mediators in aquaculture: A review. J. Basic Appl. Zool. 81, 1–16. doi: doi 10.1186/s41936-020-00190-y
Hauville M. R., Zambonino-Infante J. L., Gordon Bell J., Migaud H., Main K. L. (2016). Effects of a mix of Bacillus sp. as a potential probiotic for Florida pompano, common snook and red drum larvae performances and digestive enzyme activities. Aquac. Nutr. 22. doi: 10.1111/anu.12226
Heilbronner S., Krismer B., Brötz-Oesterhelt H., Peschel A. (2021). The microbiome-shaping roles of bacteriocins. Nat. Rev. Microbiol. 19, 1–15. doi: 10.1038/s41579-021-00569-w
Hemaiswarya S., Raja R., Ravikumar R., Carvalho I. S. (2013). Mechanism of action of probiotics. Braz. Arch. Biol. Technol. 56, 113–119. doi: 10.1590/S1516-89132013000100015
Herrera M., Mancera J. M., Costas B. (2019). The use of dietary additives in fish stress mitigation: Comparative endocrine and physiological responses. Front. Endocrinol. (Lausanne). 10. doi: 10.3389/fendo.2019.00447
Hoseinifar S. H., Esteban M.Á., Cuesta A., Sun Y. Z. (2015a). Prebiotics and fish immune response: A review of current knowledge and future perspectives. Rev. Fish. Sci. Aquac 23. doi: 10.1080/23308249.2015.1052365
Hoseinifar S. H., Mirvaghefi A., Amoozegar M. A., Sharifian M., Esteban M.Á. (2015b). Modulation of innate immune response, mucosal parameters and disease resistance in rainbow trout (Oncorhynchus mykiss) upon synbiotic feeding. Fish Shellfish Immunol. 45. doi: 10.1016/j.fsi.2015.03.029
Hoseinifar S. H., Sun Y. Z., Wang A., Zhou Z. (2018). Probiotics as means of diseases control in aquaculture, a review of current knowledge and future perspectives. Front. Microbiol. 9. doi: 10.3389/fmicb.2018.02429
Huang R. M., Feng K., Li S. F., Zong M. H., Wu H., Han S. Y. (2021). Enhanced survival of probiotics in the electrosprayed microcapsule by addition of fish oil. J. Food Eng. 307, 110650. doi: 10.1016/j.jfoodeng.2021.110650
Ibrahem M. D. (2013). Evolution of probiotics in aquatic world: Potential effects, the current status in Egypt and recent prospectives. J. Adv. Res. 6, 765–791. doi: 10.1016/j.jare.2013.12.004
Indriyani Nur (2019). Penyakit Ikan. Penyakit Ikan Edisi Revi. Yogyakarta, Indonesia: Deepublish. Available online at: https://deepublishstore.com/produk/buku-ajar-penyakit-ikan/
Irianto A., Austin B. (2002). Irianto_et_al-2002-journal_of_Fish_Diseases.pdf. Fish Dis. 25, 333–342. doi: 10.1046/j.1365-2761.2002.00375.x
Ishthiaq I. B., Ahmed J., Ramalingam K. (2021). Probiotics in brackish water fish farming: A special focus on encapsulated probiotics. Biointerface Res. Appl. Chem. 11, 14697–14708. doi: 10.33263/BRIAC116.1469714708
James G., Das B. C., Jose S., Rejish R. K. (2021). Bacillus as an aquaculture friendly microbe. Aquac. Int. 29. doi: 10.1007/s10499-020-00630-0
Kalmokoff M. L., Teather R. M. (1997). Isolation and characterization of a bacteriocin (Butyrivibriocin AR10) from the ruminal anaerobe Butyrivibrio fibrisolvens AR10: Evidence in support of the widespread occurrence of bacteriocin-like activity among ruminal isolates of B. fibrisolvens. Appl. Environ. Microbiol. 63. doi: 10.1128/aem.63.2.394-402.1997
Kamilya D., Baruah A., Sangma T., Chowdhury S., Pal P. (2015). Inactivated probiotic bacteria stimulate cellular immune responses of catla, Catla catla (Hamilton) in vitro. Probiotics Antimicrob. Proteins 7. doi: 10.1007/s12602-015-9191-9
Karthika Parvathy K. R., Mallick B., Unpaprom Y., Maniam G. P., Govindan N., Balasubramanian P. (2022). “Microbe–Host Metabolic Interaction: Probiotic Approach,” in Microbial Engineering for Therapeutics. (Singapore: Springer Nature Singapore). pp. 201–230. doi: 10.1007/978-981-19-3979-2_9
Karunathilake E. M. B. M., Le A. T., Heo S., Chung Y. S., Mansoor S. (2023). The path to smart farming: innovations and opportunities in precision agriculture. Agric. 13, 1–26. doi: 10.3390/agriculture13081593
Kataria J., Li N., Wynn J. L., Neu J. (2009). Probiotic microbes: Do they need to be alive to be beneficial? Nutr. Rev. 67. doi: 10.1111/j.1753-4887.2009.00226.x
Kawai Y., Saito T., Kitazawa H., Itoh T. (1998). Gassericin A; an uncommon cyclic bacteriocin produced by Lactobacillus gasseri LA39 linked at N- and C-terminal ends. Biosci. Biotechnol. Biochem. 62. doi: 10.1271/bbb.62.2438
Kawulka K., Sprules T., McKay R. T., Mercier P., Diaper C. M., Zuber P., et al. (2003). Structure of subtilosin A, an antimicrobial peptide from Bacillus subtilis with unusual posttranslational modifications linking cysteine sulfurs to α-carbons of phenylalanine and threonine. J. Am. Chem. Soc 125. doi: 10.1021/ja029654t
Kemperman R., Kuipers A., Karsens H., Nauta A., Kuipers O., Kok J. (2003). Identification and characterization of two novel clostridial bacteriocins, circularin A and closticin 574. Appl. Environ. Microbiol. 69. doi: 10.1128/AEM.69.3.1589-1597.2003
Kesarcodi-Watson A., Kaspar H., Lategan M. J., Gibson L. (2008). Probiotics in aquaculture: The need, principles and mechanisms of action and screening processes. Aquaculture 274 (1), 1–14. doi: 10.1016/j.aquaculture.2007.11.019
Kim D. H., Austin B. (2006). Cytokine expression in leucocytes and gut cells of rainbow trout, Oncorhynchus mykiss Walbaum, induced by probiotics. Vet. Immunol. Immunopathol. 114. doi: 10.1016/j.vetimm.2006.08.015
Kim D. H., Austin B. (2008). Characterization of probiotic cyanobacteria isolated from rainbow trout (Oncorhynchus mykiss) intestine. Lett. Appl. Microbiol. 47. doi: 10.1111/j.1472-765X.2008.02401.x
Kiss A., Balikó G., Csorba A., Chuluunbaatar T., Medzihradszky K. F., Alföldi L. (2008). Cloning and characterization of the DNA region responsible for megacin A-216 production in Bacillus megaterium 216. J. Bacteriol 190. doi: 10.1128/JB.00557-08
Knipe H., Temperton B., Lange A., Bass D., Tyler C. R. (2021). Probiotics and competitive exclusion of pathogens in shrimp aquaculture. Rev. Aquac. 13. doi: 10.1111/raq.12477
Kowalska E., Ziarno M., Ekielski A., Żelaziński T. (2022). Materials used for the microencapsulation of probiotic bacteria in the food industry. Molecules 27. doi: 10.3390/molecules27103321
Kuebutornye F. K. A., Abarike E. D., Lu Y. (2019). A review on the application of Bacillus as probiotics in aquaculture. Fish Shellfish Immunol. 87, 820–828. doi: 10.1016/j.fsi.2019.02.010
Kumar R., Mukherjee S. C., Prasad K. P., Pal A. K. (2006). Evaluation of Bacillus subtilis as a probiotic to Indian major carp Labeo rohita (Ham.). Aquac. Res. 37. doi: 10.1111/j.1365-2109.2006.01551.x
Kumar V., Roy S., Meena D. K., Sarkar U. K. (2016). Application of probiotics in shrimp aquaculture: importance, mechanisms of action, and methods of administration. Rev. Fish. Sci. Aquac. 24. doi: 10.1080/23308249.2016.1193841
Kwoji I. D., Aiyegoro O. A., Okpeku M., Adeleke M. A. (2021). Multi-strain probiotics: Synergy among isolates enhances biological activities. Biol. (Basel). 10, 1–20. doi: 10.3390/biology10040322
Kwoji I. D., Aiyegoro O. A., Okpeku M., Adeleke M. A. (2023). Multi-omics’ data integration: applications in probiotics studies. Sci. Food 7, 1–10. doi: 10.1038/s41538-023-00199-x
Lakshmi B., Viswanath B., Sai Gopal D. V. R. (2013). Probiotics as antiviral agents in shrimp aquaculture. J. Pathog. 2013. doi: 10.1155/2013/424123
Lalloo R., Ramchuran S., Ramduth D., Görgens J., Gardiner N. (2007). Isolation and selection of Bacillus spp. as potential biological agents for enhancement of water quality in culture of ornamental fish. J. Appl. Microbiol. 103. doi: 10.1111/j.1365-2672.2007.03360.x
LaPatra S. E., Fehringer T. R., Cain K. D. (2014). A probiotic Enterobacter sp. provides significant protection against Flavobacterium psychrophilum in rainbow trout (Oncorhynchus mykiss) after injection by two different routes. Aquaculture 433, 361–366. doi: 10.1016/j.aquaculture.2014.06.022
Lara-flores M. (2011). The use of probiotic in aquaculture : an overview. Int. Res. J. Microbiol. 2, 471–478. doi: 10.14303/irjm.2011.103
Lawton E. M., Cotter P. D., Hill C., Ross R. P. (2007). Identification of a novel two-peptide lantibiotic, Haloduracin, produced by the alkaliphile Bacillus halodurans C-125. FEMS Microbiol. Lett. 267. doi: 10.1111/j.1574-6968.2006.00539.x
Lazado C. C., Caipang C. M. A. (2014). Mucosal immunity and probiotics in fish. Fish Shellfish Immunol. 39. doi: 10.1016/j.fsi.2014.04.015
Lazado C. C., Caipang C. M. A., Gallage S., Brinchmann M. F., Kiron V. (2010). Expression profiles of genes associated with immune response and oxidative stress in Atlantic cod, Gadus morhua head kidney leukocytes modulated by live and heat-inactivated intestinal bacteria. Comp. Biochem. Physiol. - B Biochem. Mol. Biol. 155. doi: 10.1016/j.cbpb.2009.11.006
Lazar V., Oprea E., Ditu L. M. (2023). Resistance, tolerance, virulence and bacterial pathogen fitness—Current state and envisioned solutions for the near future. Pathogens 12. doi: 10.3390/pathogens12050746
Lee N.−K, Park Y. S., Kang D. K., Paik H. D. (2023). Paraprobiotics: definition, manufacturing methods, and functionality. Food Sci. Biotechnol. 32, 1–15. doi: 10.1007/s10068-023-01378-y
Leer R. J., van der Vossen J. M. B. M., Van Giezen M., Van Noort J. M., Pouwels P. H. (1995). Genetic analysis of acidocin B, a novel bacteriocin produced by Lactobacillus acidophilus. Microbiology 141. doi: 10.1099/13500872-141-7-1629
Legrand T. P. R. A., Wynne J. W., Weyrich L. S., Oxley A. P. A. (2020). A microbial sea of possibilities: current knowledge and prospects for an improved understanding of the fish microbiome. Rev. Aquac. 12, 1101–1134. doi: 10.1111/raq.12375
Le Marrec C., Hyronimus B., Bressollier P., Verneuil B., Urdaci M. C. (2000). Biochemical and genetic characterization of coagulin, a new antilisterial bacteriocin in the pediocin family of bacteriocins, produced by Bacillus coagulans I4. Appl. Environ. Microbiol. 66. doi: 10.1128/AEM.66.12.5213-5220.2000
Lensch A., Lindfors H. A., Duwenig E., Fleischmann T., Hjort C., Kärenlampi S. O., et al. (2024). Safety aspects of microorganisms deliberately released into the environment. EFB Bioeconomy J. 4, 100061. doi: 10.1016/j.bioeco.2023.100061
Leong J. (2008). “Fish viruses,” in Encyclopedia of Virology (pp. 227). doi: 10.1016/B978-012374410-4.00400-3
Li Z., Wang X., Wang J., Yuan X., Jiang X., Wang Y., et al. (2022). Bacterial biofilms as platforms engineered for diverse applications. Biotechnol. Adv. 57, 107932. doi: 10.1016/j.bioteChadv.2022.107932
Liao S. F., Nyachoti M. (2017). Using probiotics to improve swine gut health and nutrient utilization. Anim. Nutr. 3. doi: 10.1016/j.aninu.2017.06.007
Lievin Moal V. (2016). A gastrointestinal anti-infectious biotherapeutic agent: The heat-treated Lactobacillus LB. Therap. Adv. Gastroenterol. 9. doi: 10.1177/1756283X15602831
Liu H., Cui S. W., Chen M., li Y., Liang R., Xu F., et al. (2019). Protective approaches and mechanisms of microencapsulation to the survival of probiotic bacteria during processing, storage and gastrointestinal digestion: A review. Crit. Rev. Food Sci. Nutr. 59. doi: 10.1080/10408398.2017.1377684
Liu Z. Y., Yang H. L., Hu L. H., Yang W., Ai C. X., Sun Y. Z. (2021). Autochthonous probiotics alleviate the adverse effects of dietary histamine in juvenile grouper (Epinephelus coioides). Front. Microbiol. 12. doi: 10.3389/fmicb.2021.792718
Lobo C., Moreno-Ventas X., Tapia-Paniagua S., Rodríguez C., Moriñigo M. A., de la Banda I. G. (2014). Dietary probiotic supplementation (Shewanella putrefaciens Pdp11) modulates gut microbiota and promotes growth and condition in Senegalese sole larviculture. Fish Physiol. Biochem. 40. doi: 10.1007/s10695-013-9844-0
Loh J. Y., Chan H. K., Yam H. C., In L. L. A., Lim C. S. Y. (2020). An overview of the immunomodulatory effects exerted by probiotics and prebiotics in grouper fish. Aquac. Int. 28, 729–750. doi: 10.1007/s10499-019-00491-2
Lowe J. R., Briggs A. M., Whittle S., Stephenson M. D. (2020). A systematic review of the effects of probiotic administration in inflammatory arthritis. Complement. Ther. Clin. Pract. 40. doi: 10.1016/j.ctcp.2020.101207
Lu L., Wang J., Qin T., Chen K., Xie J., Xi B. (2023). Carvacrol inhibits quorum sensing in opportunistic bacterium Aeromonas hydrophila. Microorganisms 11. doi: 10.3390/microorganisms11082027
Luan Y., Li M., Zhou W., Yao Y., Yang Y., Zhang Z., et al. (2023). The fish microbiota: research progress and potential applications. Engineering. 29, 137–146. doi: 10.1016/j.eng.2022.12.011
Lulijwa R., Rupia E. J., Alfaro A. C. (2020). Antibiotic use in aquaculture, policies and regulation, health and environmental risks: a review of the top 15 major producers. Rev. Aquac. 12, 640–663. doi: 10.1111/raq.12344
Luz R. K., Favero G. C. (2023). Tilapia larviculture. Biology and Aquaculture of Tilapia. (Boca Raton, FL: CRC Press). pp. 196–220. doi: 10.1201/9781003004134-11
Maqueda M., Sánchez-Hidalgo M., Fernández M., Montalbán-López M., Valdivia E., Martínez-Bueno M. (2008). Genetic features of circular bacteriocins produced by Gram-positive bacteria. FEMS Microbiol. Rev. 32, 2–22. doi: 10.1111/j.1574-6976.2007.00087.x
Martínez Cruz P., Ibáñez A. L., Monroy Hermosillo O. A., Ramírez Saad H. C. (2012). Use of probiotics in aquaculture. ISRN Microbiol. 2012, 1–13. doi: 10.5402/2012/916845
Marzouk M. S., Moustafa M. M., Mohamed N. (2008). “The influence of some probiotics on the growth performance and intestinal microbial flora of O. niloticus,” in Proceedings of the 8th International Symposium on Tilapia in Aquaculture. pp. 1059–1071.
Mayer F. L., Kronstad J. W. (2017). Disarming fungal pathogens: Bacillus safensis inhibits virulence factor production and biofilm formation by Cryptococcus neoformans and Candida albicans. MBio 8. doi: 10.1128/mBio.01537-17
Mehrim A. I. (2009). Effect of dietary supplementation of Biogen® (commercial probiotic) on mono-sex nile tilapia Oreochromis niloticus under different stocking densities. J. Fish. Aquat. Sci. 4. doi: 10.3923/jfas.2009.261.273
Meng D., Hao Q., Zhang Q., Yu Z., Liu S., Yang Y., et al. (2023). A compound of paraprobiotic and postbiotic derived from autochthonous microorganisms improved growth performance, epidermal mucus, liver and gut health and gut microbiota of common carp (Cyprinus carpio). Aquaculture 570, 739378. doi: 10.1016/j.aquaculture.2023.739378
Menon S. V., Kumar A., Middha S. K., Paital B., Mathur S., Johnson R., et al. (2023). Water physicochemical factors and oxidative stress physiology in fish, a review. Front. Environ. Sci. 11. doi: 10.3389/fenvs.2023.1240813
Merrifield D. L., Dimitroglou A., Foey A., Davies S. J., Baker R. T. M., Bøgwald J., et al. (2010). The current status and future focus of probiotic and prebiotic applications for salmonids. Aquaculture 302. doi: 10.1016/j.aquaculture.2010.02.007
Mishra V., Shah C., Mokashe N., Chavan R., Yadav H., Prajapati J. (2015). Probiotics as potential antioxidants: A systematic review. J. Agric. Food Chem. 63, 3615–3626. doi: 10.1021/jf506326t
Mohamed K., Megahed M. E., Ali M. A. M. (2017). Effect of dietary supplementation of Agrimos® on growth performance, feed utilization and immunological parameters of Macrobrachium rosenbergii juveniles. Aquac. Int. 25. doi: 10.1007/s10499-017-0123-4
Mohammadi N., Tukmechi A. (2015). The effects of iron nanoparticles in combination with Lactobacillus casei on growth parameters and probiotic counts in rainbow trout (Oncorhynchus mykiss) intestine. J. Vet. Res. 70. doi: 10.22059/jvr.2015.52968
Mohammadian T., Nasirpour M., Tabandeh M. R., Heidary A. A., Ghanei-Motlagh R., Hosseini S. S. (2019). Administrations of autochthonous probiotics altered juvenile rainbow trout Oncorhynchus mykiss health status, growth performance and resistance to Lactococcus garvieae, an experimental infection. Fish Shellfish Immunol. 86. doi: 10.1016/j.fsi.2018.11.052
Mohapatra S., Chakraborty T., Kumar V., Deboeck G., Mohanta K. N. (2013). Aquaculture and stress management: A review of probiotic intervention. J. Anim. Physiol. Anim. Nutr. (Berl). 97, 405–430. doi: 10.1111/j.1439-0396.2012.01301.x
Mohapatra S., Chakraborty T., Prusty A. K., Das P., Paniprasad K., Mohanta K. N. (2012). Use of different microbial probiotics in the diet of rohu, Labeo rohita fingerlings: Effects on growth, nutrient digestibility and retention, digestive enzyme activities and intestinal microflora. Aquac. Nutr. 18. doi: 10.1111/j.1365-2095.2011.00866.x
Mohapatra S., Chakraborty T., Prusty A. K., PaniPrasad K., Mohanta K. N. (2014). Beneficial effects of dietary probiotics mixture on hemato-immunology and cell apoptosis of Labeo rohita fingerlings reared at higher water temperatures. PloS One 9. doi: 10.1371/journal.pone.0100929
Mohd-Aris A., Muhamad-Sofie M. H. N., Zamri-Saad M., Daud H. M., Yasin Ina-Salwany M. (2019). Live vaccines against bacterial fish diseases: A review. Vet. World 12. doi: 10.14202/vetworld.2019.1806-1815
Mondal H., Chandrasekaran N., Mukherjee A., Thomas J. (2022). Viral infections in cultured fish and shrimps: current status and treatment methods. Aquac. Int. 30, 1–15. doi: 10.1007/s10499-021-00795-2
Mondal H., Thomas J. (2022). A review on the recent advances and application of vaccines against fish pathogens in aquaculture. Aquac. Int. 30. doi: 10.1007/s10499-022-00884-w
Monteiro S. S., Schnorr C. E., Pasquali M.A.de B. (2023). Paraprobiotics and postbiotics—Current state of scientific research and future trends toward the development of functional foods. Foods 12. doi: 10.3390/foods12122394
Monzón-Atienza L., Bravo J., Torrecillas S., Gómez-Mercader A., Montero D., Ramos-Vivas J., et al. (2024). An in-depth study on the inhibition of quorum sensing by Bacillus velezensis D-18: its significant impact on Vibrio biofilm formation in aquaculture. Microorganisms 12. doi: 10.3390/microorganisms12050890
Muhammad M. H., Idris A. L., Fan X., Guo Y., Yu Y., Jin X., et al. (2020). Beyond risk: bacterial biofilms and their regulating approaches. Front. Microbiol. 11. doi: 10.3389/fmicb.2020.00928
Muñoz-Atienza E., Araújo C., Lluch N., Hernández P. E., Herranz C., Cintas L. M., et al. (2015). Different impact of heat-inactivated and viable lactic acid bacteria of aquatic origin on turbot (Scophthalmus maximus L.) head-kidney leucocytes. Fish Shellfish Immunol. 44. doi: 10.1016/j.fsi.2015.02.021
Murata M., Kondo J., Iwabuchi N., Takahashi S., Yamauchi K., Abe F., et al. (2018). Effects of paraprobiotic Lactobacillus paracasei MCC1849 supplementation on symptoms of the common cold and mood states in healthy adults. Benef. Microbes 9. doi: 10.3920/BM2017.0197
Naiel M. A. E., Shehata A. M., El-Kholy A. I., El-Naggar K., Farag M. R., Alagawany M. (2022). The mitigating role of probiotics against the adverse effects of suboptimal temperature in farmed fish: A review. Aquaculture. 550, 737877. doi: 10.1016/j.aquaculture.2021.737877
Nasr-Eldahan S., Nabil-Adam A., Shreadah M. A., Maher A. M., El-Sayed Ali T. (2021). A review article on nanotechnology in aquaculture sustainability as a novel tool in fish disease control. Aquac. Int. 29. doi: 10.1007/s10499-021-00677-7
Nataraj B. H., Ali S. A., Behare P. V., Yadav H. (2020). Postbiotics-parabiotics: The new horizons in microbial biotherapy and functional foods. Microb. Cell Fact. 19. doi: 10.1186/s12934-020-01426-w
Nathanailides C., Kolygas M., Choremi K., Mavraganis T., Gouva E., Vidalis K., et al. (2021). Probiotics have the potential to significantly mitigate the environmental impact of freshwater fish farms. Fishes 6, 1–15. doi: 10.3390/fishes6040076
Nayak S. K. (2010). Probiotics and immunity: A fish perspective. Fish Shellfish Immunol. 29, 2–14. doi: 10.1016/j.fsi.2010.02.017
Nayak S. K. (2021). Multifaceted applications of probiotic Bacillus species in aquaculture with special reference to Bacillus subtilis. Rev. Aquac. 13. doi: 10.1111/raq.12503
Newaj-Fyzul A., Adesiyun A. A., Mutani A., Ramsubhag A., Brunt J., Austin B. (2007). Bacillus subtilis AB1 controls Aeromonas infection in rainbow trout (Oncorhynchus mykiss, Walbaum). J. Appl. Microbiol. 103. doi: 10.1111/j.1365-2672.2007.03402.x
Newaj-Fyzul A., Al-Harbi A. H., Austin B. (2014). Review: Developments in the use of probiotics for disease control in aquaculture. Aquaculture 431. doi: 10.1016/j.aquaculture.2013.08.026
Ng W. K., Kim Y. C., Romano N., Koh C. B., Yang S. Y. (2014). Effects of Dietary Probiotics on the Growth and Feeding Efficiency of Red Hybrid Tilapia, Oreochromis sp., and Subsequent Resistance to Streptococcus agalactiae. J. Appl. Aquac 26. doi: 10.1080/10454438.2013.874961
Nguyen T. L., Park C. I. L., Kim D. H. (2017). Improved growth rate and disease resistance in olive flounder, Paralichthys olivaceus, by probiotic Lactococcus lactis WFLU12 isolated from wild marine fish. Aquaculture 471. doi: 10.1016/j.aquaculture.2017.01.008
Nikoskelainen S., Ouwehand A. C., Bylund G., Salminen S., Lilius E. M. (2003). Immune enhancement in rainbow trout (Oncorhynchus mykiss) by potential probiotic bacteria (Lactobacillus rhamnosus). Fish Shellfish Immunol. 15. doi: 10.1016/S1050-4648(03)00023-8
Ntakirutimana R., Syanya F. J., Mwangi P. (2023). Exploring the impact of probiotics on the gut ecosystem and morpho-histology in fish: current knowledge of tilapia. Asian J. Fish. Aquat. Res. 25, 93–112. doi: 10.9734/ajfar/2023/v25i3670
Oelschlaeger T. A. (2010). Mechanisms of probiotic actions - A review. Int. J. Med. Microbiol. 300, 57–62. doi: 10.1016/j.ijmm.2009.08.005
Oliveira T. F., Quieróz G. A., Leibowitz M. P., Gomes Leal C. A. (2022). Development of an inactivated whole cell vaccine through immersion for immunoprophylaxis of Francisella orientalis infections in Nile tilapia (Oreochromis niloticus L.) fingerlings and juveniles. Fish Shellfish Immunol. 127, 405–411. doi: 10.1016/j.fsi.2022.06.040
Omar A. A., Gado M. S., Kandel H. E., Farrag F. A., Shukry M. (2024). Probiotic Efficacy in Aquaculture: The Role of Technospore® (Bacillus coagulans) in Improving Nile Tilapia (Oreochromis niloticus) Performance and Disease Resistance: a Study on Gut Health, Immunological Response, and Gene Expression. Probiotics and Antimicrob. Proteins, 1-18. doi: 10.1007/s12602-024-10279-3
Oscáriz J. C., Pisabarro A. G. (2000). Characterization and mechanism of action of cerein 7, a bacteriocin produced by Bacillus cereus Bc7. J. Appl. Microbiol. 89. doi: 10.1046/j.1365-2672.2000.01123.x
Pan X., Wu T., Song Z., Tang H., Zhao Z. (2008). Immune responses and enhanced disease resistance in Chinese drum, Miichthys miiuy (Basilewsky), after oral administration of live or dead cells of Clostridium butyrium CB2. J. Fish Dis. 31. doi: 10.1111/j.1365-2761.2008.00955.x
Pandiyan P., Balaraman D., Thirunavukkarasu R., George E. G. J., Subaramaniyan K., Manikkam S., et al. (2013). Probiotics in aquaculture. Drug Invent. Today 5, 55–59. doi: 10.1016/j.dit.2013.03.003
Panigrahi A., Kiron V., Satoh S., Hirono I., Kobayashi T., Sugita H., et al. (2007). Immune modulation and expression of cytokine genes in rainbow trout Oncorhynchus mykiss upon probiotic feeding. Dev. Comp. Immunol. 31. doi: 10.1016/j.dci.2006.07.004
Parisot J., Carey S., Breukink E., Chan W. C., Narbad A., Bonev B. (2008). Molecular mechanism of target recognition by subtilin, a class I lanthionine antibiotic. Antimicrob. Agents Chemother. 52. doi: 10.1128/AAC.00836-07
Pavadi P., Murthy H. S., Honnananda B. R., Choudhary B. K. (2018). Enhancement of Growth, Immunity, Resistance and Survival of Freshwater Prawn, Macrobrachium rosenbergii against White Muscle Disease (WMD) Due to Dietary Administration of Probiotic and Biogut. Int. J. Curr. Microbiol. Appl. Sci. 7. doi: 10.20546/ijcmas.2018.704.067
Peng M., Tabashsum Z., Anderson M., Truong A., Houser A. K., Padilla J., et al. (2020). Effectiveness of probiotics, prebiotics, and prebiotic-like components in common functional foods. Compr. Rev. Food Sci. Food Saf. 19. doi: 10.1111/1541-4337.12565
Pérez-Sánchez T., Balcázar J. L., Merrifield D. L., Carnevali O., Gioacchini G., de Blas I., et al. (2011). Expression of immune-related genes in rainbow trout (Oncorhynchus mykiss) induced by probiotic bacteria during Lactococcus garvieae infection. Fish Shellfish Immunol. 31. doi: 10.1016/j.fsi.2011.05.005
Pérez-Sánchez T., Ruiz-Zarzuela I., de Blas I., Balcázar J. L. (2014). Probiotics in aquaculture: A current assessment. Rev. Aquac. 6. doi: 10.1111/raq.12033
Puvanasundram P., Chong C. M., Sabri S., Yusoff M. S., Karim M. (2021). Multi-strain probiotics: Functions, effectiveness and formulations for aquaculture applications. Aquac. Rep. 21. doi: 10.1016/j.aqrep.2021.100905
Qiao L., Dou X., Song X., Xu C. (2022). Green synthesis of nanoparticles by probiotics and their application. Adv. Appl. Microbiol. 119, 83–128. doi: 10.1016/bs.aambs.2022.05.003
Rahman A., Hossain S., Shefat T., Chowdhury M. A., Khan S. U. (2021). Effects of probiotic Bacillus on fish growth performance, immune response and disease resistance in aquaculture. J. Aquac. Res. Dev. 12. doi: 10.20944/preprints202103.0075.v1
Raida M. K., Buchmann K. (2009). Innate immune response in rainbow trout (Oncorhynchus mykiss) against primary and secondary infections with Yersinia ruckeri O1. Dev. Comp. Immunol. 33. doi: 10.1016/j.dci.2008.07.001
Rajesh P. S., Rai V. R. (2016). Inhibition of QS-regulated virulence factors in Pseudomonas aeruginosa PAO1 and Pectobacterium carotovorum by AHL-lactonase of endophytic bacterium Bacillus cereus VT96. Biocatal. Agric. Biotechnol. 7. doi: 10.1016/j.bcab.2016.06.003
Rakhfid A., Kulsum W. O. N., Fendi F., Mosriula M., Bakri M., Karyawati K., et al. (2020). The use of probiotic for growth and survival of milkfish (Chanos chanos Forskal). Akuatikisle J. Akuakultur Pesisir dan Pulau-Pulau Kecil 4, 83–89. doi: 10.29239/j.akuatikisle.4.2.83-89
Rawal S., Ali S. A. (2023). Probiotics and postbiotics play a role in maintaining dermal health. Food Funct. 14. doi: 10.1039/d3fo00152k
Retnaningrum E. (2024). Keragaman dan metabolisme serta aplikasi mikroorganisme ekstremofil dalam berbagai bidang untuk mendukung kesejahteraan manusia. Fak. Biol. Univ. Gadjah Mada.
Ridha M. T., Azad I. S. (2012). Preliminary evaluation of growth performance and immune response of Nile tilapia Oreochromis niloticus supplemented with two putative probiotic bacteria. Aquac. Res. 43. doi: 10.1111/j.1365-2109.2011.02899.x
Ringø E. (2020). Probiotics in shellfish aquaculture. Aquac. Fish. 5, 1–27. doi: 10.1016/j.aaf.2019.12.001
Ringø E., Hoseinifar S. H., Ghosh K., Van Doan H., Beck B. R., Song S. K. (2018). Lactic acid bacteria in finfish-An update. Front. Microbiol. 9. doi: 10.3389/fmicb.2018.01818
Ringø E., Van Doan H., Lee S. H., Soltani M., Hoseinifar S. H., Harikrishnan R., et al. (2020). Probiotics, lactic acid bacteria and bacilli: interesting supplementation for aquaculture. J. Appl. Microbiol. 129. doi: 10.1111/jam.14628
Rivki M., Bachtiar A. M., Informatika T., Teknik F., Indonesia U. K. (2021). Potential use of probiotics and micro/macro algae as additives and major ingredients in aquafeeds. In Proceedings of the International Conference on Aquaculture. (Jakarta, Indonesia: Universitas Kristen Indonesia), pp. 1755.
Rodrigues F. J., Cedran M. F., Bicas J. L., Sato H. H. (2020). Encapsulated probiotic cells: Relevant techniques, natural sources as encapsulating materials and food applications – A narrative review. Food Res.Int. 137, 109682. doi: 10.1016/j.foodres.2020.109682
Rodriguez-Estrada U., Satoh S., Haga Y., Fushimi H., Sweetman J. (2013). Effects of inactivated Enterococcus faecalis and Mannan oligosaccharide and their combination on growth, immunity, and disease protection in rainbow trout. N. Am. J. Aquac. 75. doi: 10.1080/15222055.2013.799620
Román L., Real F., Padilla D., El Aamri F., Déniz S., Grasso V., et al. (2013). Cytokine expression in head-kidney leucocytes of European sea bass (Dicentrarchus labrax L.) after incubation with the probiotic Vagococcus fluvialis L-21. Fish Shellfish Immunol. 35. doi: 10.1016/j.fsi.2013.07.036
Rossi F., Rizzotti L., Felis G. E., Torriani S. (2014). Horizontal gene transfer among microorganisms in food: Current knowledge and future perspectives. Food Microbiol. 42. doi: 10.1016/j.fm.2014.04.004
Rueda-Robles A., Rodríguez-Lara A., Meyers M. S., Sáez-Lara M. J., Álvarez-Mercado A. I. (2022). Effect of probiotics on host-microbiota in bacterial infections. Pathogens 11. doi: 10.3390/pathogens11090986
Sahoo S., Banu H., Prakash A., Tripathi G. (2021). “Immune system of fish: An evolutionary perspective,” in Antimicrobial Immune Response. (New Delhi, India: Springer), 1–10. doi: 10.5772/intechopen.99541
Salaheen S., Kim S. W., Haley B. J., Van Kessel J. A. S., Biswas D. (2017). Alternative growth promoters Modulate broiler gut microbiome and enhance body weight gain. Front. Microbiol. 8. doi: 10.3389/fmicb.2017.02088
Salinas I., Myklebust R., Esteban M. A., Olsen R. E., Meseguer J., Ringø E. (2008). In vitro studies of Lactobacillus delbrueckii subsp. lactis in Atlantic salmon (Salmo salar L.) foregut: Tissue responses and evidence of protection against Aeromonas salmonicida subsp. Salmonicida epithelial damage. Vet. Microbiol. 128. doi: 10.1016/j.vetmic.2007.10.011
Sarao L. K., Arora M. (2017). Probiotics, prebiotics, and microencapsulation: A review. Crit. Rev. Food Sci. Nutr. 57. doi: 10.1080/10408398.2014.887055
Sayes C., Leyton Y., Riquelme C. (2018). “Probiotic bacteria as a healthy alternative for fish aquaculture,” In Antibiotic Use in Animals. pp. 115–132. doi: 10.5772/intechopen.71206
Schreck C. B., Tort L. (2016). The concept of stress in fish. In Fish physiology 1st ed. (San Diego, CA: Elsevier Inc) 35, 1–34. doi: 10.1016/B978-0-12-802728-8.00001-1
Selim K. M., Reda R. M. (2015). Improvement of immunity and disease resistance in the Nile tilapia, Oreochromis niloticus, by dietary supplementation with Bacillus amyloliquefaciens. Fish Shellfish Immunol. 44. doi: 10.1016/j.fsi.2015.03.004
Shaheer P., Sreejith V. N., Joseph T. C., Murugadas V., Lalitha K. V. (2021). Quorum quenching Bacillus spp.: an alternative biocontrol agent for Vibrio harveyi infection in aquaculture. Dis. Aquat. Organ 146. doi: 10.3354/dao03619
Shangguan W., Xie T., Zhang R., Lu C., Han X., Zhong Q. (2021). Anti-biofilm potential of kefir-derived Lactobacillus paracasei L10 against Vibrio parahaemolyticus. Lett. Appl. Microbiol. 73. doi: 10.1111/lam.13568
Sharma M., Chandel D., Shukla G. (2020). Antigenotoxicity and cytotoxic potentials of metabiotics extracted from isolated probiotic, Lactobacillus rhamnosus MD 14 on caco-2 and HT-29 human colon cancer cells. Nutr. Cancer 72. doi: 10.1080/01635581.2019.1615514
Shija V. M., Amoah K., Cai J. (2023). Effect of Bacillus probiotics on the immunological responses of nile tilapia (Oreochromis niloticus): A review. Fishes 8, 1–16. doi: 10.3390/fishes8070366
Siciliano R. A., Reale A., Mazzeo M. F., Morandi S., Silvetti T., Brasca M. (2021). Paraprobiotics: A new perspective for functional foods and nutraceuticals. Nutrients 13. doi: 10.3390/nu13041225
Simón R., Docando F., Nuñez-Ortiz N., Tafalla C., Díaz-Rosales P. (2021). Mechanisms used by probiotics to confer pathogen resistance to teleost fish. Front. Immunol. 12. doi: 10.3389/fimmu.2021.653025
Singh R. P. (2015). Attenuation of quorum sensing-mediated virulence in Gram-negative pathogenic bacteria: Implications for the post-antibiotic era. Medchemcomm 6. doi: 10.1039/c4md00363b
Singh S., Datta S., Narayanan K. B., Rajnish K. N. (2021). Bacterial exo-polysaccharides in biofilms: role in antimicrobial resistance and treatments. J. Genet. Eng. Biotechnol. 19. doi: 10.1186/s43141-021-00242-y
Singh K., Kallali B., Kumar A., Thaker V. (2011). Probiotics: A review. Asian Pac. J. Trop. Biomed. 1, S287–S290. doi: 10.1016/S2221-1691(11)60174-3
Singh S. T., Kamilya D., Kheti B., Bordoloi B., Parhi J. (2017). Paraprobiotic preparation from Bacillus amyloliquefaciens FPTB16 modulates immune response and immune relevant gene expression in Catla catla (Hamilton 1822). Fish Shellfish Immunol. 66. doi: 10.1016/j.fsi.2017.05.005
Soltan M., El-L S. (2008). Effect of probiotics and some spices as feed additives on the performance and behavior of the Nile tilapia, Oreochromis niloticus. Egypt. J. Aquat. Biol. Fish. 12. doi: 10.21608/ejabf.2008.1992
Soltani M., Ghosh K., Dutta D., Ringø E. (2023). “Prebiotics and Probiotics as Effective Immunomodulators in Aquaculture,” in Immunomodulators in Aquaculture and Fish Health. (Boca Raton, FL: CRC Press), 136–168. doi: 10.1201/9781003361183-13
Soltani S., Hammami R., Cotter P. D., Rebuffat S., Ben Said L., Gaudreau H., et al. (2021). Bacteriocins as a new generation of antimicrobials: toxicity aspects and regulations. FEMS Microbiol. Rev. 45. doi: 10.1093/femsre/fuaa039
Srirengaraj V., Razafindralambo H. L., Rabetafika H. N., Nguyen H. T., Sun Y. Z. (2023). Synbiotic agents and their active components for sustainable aquaculture: concepts, action mechanisms, and applications. Biol. (Basel). 12. doi: 10.3390/biology12121498
Standen B. T., Rawling M. D., Davies S. J., Castex M., Foey A., Gioacchini G., et al. (2013). Probiotic Pediococcus acidilactici modulates both localized intestinal- and peripheral immunity in tilapia (Oreochromis niloticus). Fish Shellfish Immunol. 35. doi: 10.1016/j.fsi.2013.07.018
Sugita H., Matsuo N., Hirose Y., Iwato M., Deguchi Y. (1997). Vibrio sp. strain NM 10, isolated from the intestine of a Japanese coastal fish, has an inhibitory effect against Pasteurella piscicida. Appl. Environ. Microbiol. 63. doi: 10.1128/aem.63.12.4986-4989.1997
Sugita H., Mizuki H., Itoi S. (2012). Diversity of siderophore-producing bacteria isolated from the intestinal tracts of fish along the Japanese coast. Aquac. Res. 43. doi: 10.1111/j.1365-2109.2011.02851.x
Sun Y. Z., Xia H. Q., Yang H. L., Wang Y. L., Zou W. C. (2014). TLR2 signaling may play a key role in the probiotic modulation of intestinal microbiota in grouper Epinephelus coioides. Aquaculture 430. doi: 10.1016/j.aquaculture.2014.03.042
Sun Y. Z., Yang H. L., Ma R. L., Zhang C. X., Lin W. Y. (2011). Effect of dietary administration of Psychrobacter sp. On the growth, feed utilization, digestive enzymes and immune responses of grouper Epinephelus coioides. Aquac. Nutr. 17, e733–e740. doi: 10.1111/j.1365-2095.2010.00837.x
Sun Q., Yin S., He Y., Cao Y. (2023). Biomaterials and encapsulation techniques for probiotics: Current status and future prospects in biomedical applications. Nanomaterials. 13 (15), 2185. doi: 10.3390/nano13152185
Sutyak K. E., Wirawan R. E., Aroutcheva A. A., Chikindas M. L. (2008). Isolation of the Bacillus subtilis antimicrobial peptide subtilosin from the dairy product-derived Bacillus amyloliquefaciens. J. Appl. Microbiol. 104. doi: 10.1111/j.1365-2672.2007.03626.x
Szydłowska A., Sionek B. (2023). Probiotics and postbiotics as the functional food components affecting the immune response. Microorganisms 11. doi: 10.3390/microorganisms11010104
Taherpour M., Roomiani L., Islami H. R., Mehrgan M. S. (2023). Effect of dietary butyric acid, Bacillus licheniformis (probiotic), and their combination on hemato-biochemical indices, antioxidant enzymes, immunological parameters, and growth performance of Rainbow trout (Oncorhynchus mykiss). Aquac. Rep. 30. doi: 10.1016/j.aqrep.2023.101534
Talpur A. D., Munir M. B., Mary A., Hashim R. (2014). Dietary probiotics and prebiotics improved food acceptability, growth performance, haematology and immunological parameters and disease resistance against Aeromonas hydrophila in snakehead (Channa striata) fingerlings. Aquaculture 426, 14–20. doi: 10.1016/j.aquaculture.2014.01.013
Tan H. Y., Chen S. W., Hu S. Y. (2019). Improvements in the growth performance, immunity, disease resistance, and gut microbiota by the probiotic Rummeliibacillus stabekisii in Nile tilapia (Oreochromis niloticus). Fish Shellfish Immunol. 92, 265–275. doi: 10.1016/j.fsi.2019.06.027
Tang K. F., Bondad-Reantaso M. G., Surachetpong W., Dong H. T., Fejzic N., Wang Q., et al. (2021). Tilapia lake virus disease strategy manual. (Rome, Italy: Food and Agriculture Organization of the United Nations). p. 1220.
Taoka Y., Maeda H., Jo J. Y., Jeon M. J., Bai S. C., Lee W. J., et al. (2006). Growth, stress tolerance and non-specific immune response of Japanese flounder Paralichthys olivaceus to probiotics in a closed recirculating system. Fish. Sci. 72. doi: 10.1111/j.1444-2906.2006.01152.x
Taverniti V., Guglielmetti S. (2011). The immunomodulatory properties of probiotic microorganisms beyond their viability (ghost probiotics: Proposal of paraprobiotic concept). Genes Nutr. 6. doi: 10.1007/s12263-011-0218-x
Terpou A., Papadaki A., Lappa I. K., Kachrimanidou V., Bosnea L. A., Kopsahelis N. (2019). Probiotics in food systems: significance and emerging strategies towards improved viability and delivery of enhanced beneficial value. Nutrients 11. doi: 10.3390/nu11071591
Thakur B. K., Saha P., Banik G., Saha D. R., Grover S., Batish V. K., et al. (2016). Live and heat-killed probiotic Lactobacillus casei Lbs2 protects from experimental colitis through Toll-like receptor 2-dependent induction of T-regulatory response. Int. Immunopharmacol. 36. doi: 10.1016/j.intimp.2016.03.033
Timothy B., Iliyasu A. H., Anvikar A. R. (2021). Bacteriocins of lactic acid bacteria and their industrial application. Curr. Top. Lact. Acid Bact. Probiotics 7. doi: 10.35732/ctlabp.2021.7.1.1
Toba T., Samant S. K., Yoshioka E., Itoh T. (1991). Reutericin 6, a new bacteriocin produced by Lactobacillus reuteri LA 6. Lett. Appl. Microbiol. 13. doi: 10.1111/j.1472-765X.1991.tb00629.x
Tovar-Ramírez D., Mazurais D., Gatesoupe J. F., Quazuguel P., Cahu C. L., Zambonino-Infante J. L. (2010). Dietary probiotic live yeast modulates antioxidant enzyme activities and gene expression of sea bass (Dicentrarchus labrax) larvae. Aquaculture 300. doi: 10.1016/j.aquaculture.2009.12.015
Tran N. T., Li Z., Ma H., Zhang Y., Zheng H., Gong Y., et al. (2020a). Clostridium butyricum: a promising probiotic confers positive health benefits in aquatic animals. Rev. Aquac. 12. doi: 10.1111/raq.12459
Tran N. T., Li Z., Wang S., Zheng H., Aweya J. J., Wen X., et al. (2020b). Progress and perspectives of short-chain fatty acids in aquaculture. Rev. Aquac. 12. doi: 10.1111/raq.12317
Tran N. T., Liang H., Li J., Deng T., Zhang M., Li S. (2023). Health benefits of butyrate and its producing bacterium, Clostridium butyricum, on aquatic animals. Fish Shellfish Immunol. Rep. 4. doi: 10.1016/j.fsirep.2023.100088
Tran N. T., Yang W., Nguyen X. T., Zhang M., Ma H., Zheng H., et al. (2022). Application of heat-killed probiotics in aquaculture. Aquaculture 548. doi: 10.1016/j.aquaculture.2021.737700
Vallejo-Cordoba B., Castro-López C., García H. S., González-Córdova A. F., Hernández-Mendoza A. (2020). Postbiotics and paraprobiotics: A review of current evidence and emerging trends. Adv. Food Nutr. Res. 94, 1–34. doi: 10.1016/bs.afnr.2020.06.001
Vandenbergh P. A. (1993). Lactic acid bacteria, their metabolic products and interference with microbial growth. FEMS Microbiol. Rev. 12. doi: 10.1016/0168-6445(93)90065-H
Van Doan H., Hoseinifar S. H., Ringø E., Ángeles Esteban M., Dadar M., Dawood M. A. O., et al. (2020). Host-associated probiotics: A key factor in sustainable aquaculture. Rev. Fish. Sci. Aquac. 28. doi: 10.1080/23308249.2019.1643288
Van Doan H., Wangkahart E., Thaimuangphol W., Panase P., Sutthi N. (2021). Effects of Bacillus spp. Mixture on Growth, Immune Responses, Expression of Immune-Related Genes, and Resistance of Nile Tilapia Against Streptococcus agalactiae Infection. Probiotics Antimicrob. Proteins, 1–16. doi: 10.1007/s12602-021-09845-w
van Hai N., Fotedar R. (2010). A review of probiotics in shrimp aquaculture. J. Appl. Aquac. 22. doi: 10.1080/10454438.2010.500597
Vargas-Albores F., Martínez-Córdova L. R., Hernández-Mendoza A., Cicala F., Lago-Lestón A., Martínez-Porchas M. (2021). Therapeutic modulation of fish gut microbiota, a feasible strategy for aquaculture? Aquaculture 544. doi: 10.1016/j.aquaculture.2021.737050
Vargas-González A., Barajas M., Pérez-Sánchez T. (2024). Isolation of lactic acid bacteria (LAB) from salmonids for potential use as probiotics: in vitro assays and toxicity assessment of Salmo trutta embryonated eggs. Animals 14. doi: 10.3390/ani14020200
Vasanth G. K., Kiron V., Kulkarni A., Dahle D., Lokesh J., Kitani Y. (2015). A microbial feed additive abates intestinal inflammation in Atlantic salmon. Front. Immunol. 6. doi: 10.3389/fimmu.2015.00409
Verschuere L., Rombaut G., Sorgeloos P., Verstraete W. (2000). Probiotic bacteria as biological control agents in aquaculture. Microbiol. Mol. Biol. Rev. 64, 655–671. doi: 10.1128/mmbr.64.4.655-671.2000
Vieira M., Pittia P., Silva C. L., Dubois-Brissonnet F., Costa R., Chrysanthopoulou F. (2021). “Sustainable development goals in food systems: Challenges and opportunities for the future,” in Proceedings of the 6th International ISEKI-Food Conference 2021.
Vijayaram S., Razafindralambo H., Sun Y. Z., Piccione G., Multisanti C. R., Faggio C. (2024). Synergistic interaction of nanoparticles and probiotic delivery: A review. J. Fish Dis. 47. doi: 10.1111/jfd.13916
Villamil L., Tafalla C., Figueras A., Novoa B. (2002). Evaluation of immunomodulatory effects of lactic acid bacteria in turbot (Scophthalmus maximus). Clin. Diagn. Lab. Immunol. 9. doi: 10.1128/CDLI.9.6.1318-1323.2002
Vine N. G., Leukes W. D., Kaiser H. (2006). Probiotics in marine larviculture. FEMS Microbiol. Rev. 30, 404–427. doi: 10.1111/j.1574-6976.2006.00017.x
Vine N. G., Leukes W. D., Kaiser H., Daya S., Baxter J., Hecht T. (2004). Competition for attachment of aquaculture candidate probiotic and pathogenic bacteria on fish intestinal mucus. Journal of fish diseases 27(6), 319–326.
Vinoj G., Jayakumar R., Chen J. C., Withyachumnarnkul B., Shanthi S., Vaseeharan B. (2015). N-hexanoyl-L-homoserine lactone-degrading Pseudomonas aeruginosa PsDAHP1 protects zebrafish against Vibrio parahaemolyticus infection. Fish Shellfish Immunol. 42. doi: 10.1016/j.fsi.2014.10.033
Vivek K., Mishra S., Pradhan R. C., Nagarajan M., Kumar P. K., Singh S. S., et al. (2023). A comprehensive review on microencapsulation of probiotics: technology, carriers and current trends. Appl. Food Res. 3. doi: 10.1016/j.afres.2022.100248
Waiyamitra P., Zoral M. A., Saengtienchai A., Luengnaruemitchai A., Decamp O., Gorgoglione B., et al. (2020). Probiotics modulate tilapia resistance and immune response against tilapia lake virus infection. Pathogens 9. doi: 10.3390/pathogens9110919
Wang C., Chuprom J., Wang Y., Fu L. (2020). Beneficial bacteria for aquaculture: nutrition, bacteriostasis and immunoregulation. J. Appl. Microbiol. 128. doi: 10.1111/jam.14383
Wang A., Ran C., Wang Y., Zhang Z., Ding Q., Yang Y., et al. (2019). Use of probiotics in aquaculture of China—a review of the past decade. Fish Shellfish Immunol. 86, 734–755. doi: 10.1016/j.fsi.2018.12.026
Watters C., Fleming D., Bishop D., Rumbaugh K. P. (2016). Chapter seven – host responses to biofilm. Prog. Mol. Biol. Transl. Sci. 142, 193–239. doi: 10.1016/bs.pmbts.2016.05.007
Williams S. T., Vickers J. C. (1986). The ecology of antibiotic production. Microb. Ecol. 12. doi: 10.1007/BF02153221
Wirawan R. E., Swanson K. M., Kleffmann T., Jack R. W., Tagg J. R. (2007). Uberolysin: A novel cyclic bacteriocin produced by Streptococcus uberis. Microbiology 153. doi: 10.1099/mic.0.2006/005967-0
Wuertz S., Schroeder A., Wanka K. M. (2021). Probiotics in fish nutrition—long-standing household remedy or native nutraceuticals? Water (Switzerland) 13. doi: 10.3390/w13101348
Xia Y., Lu M., Chen G., Cao J., Gao F., Wang M., et al. (2018). Effects of dietary Lactobacillus rhamnosus JCM1136 and Lactococcus lactis subsp. lactis JCM5805 on the growth, intestinal microbiota, morphology, immune response and disease resistance of juvenile Nile tilapia, Oreochromis niloticus. Fish Shellfish Immunol. 76. doi: 10.1016/j.fsi.2018.03.020
Xie F., Zhu T., Zhang F., Zhou K., Zhao Y., Li Z. (2013). Using Bacillus amyloliquefaciens for remediation of aquaculture water. Springerplus 2. doi: 10.1186/2193-1801-2-119
Xu H. J., Jiang W. D., Feng L., Liu Y., Wu P., Jiang J., et al. (2016). Dietary vitamin C deficiency depressed the gill physical barriers and immune barriers referring to Nrf2, apoptosis, MLCK, NF-κB and TOR signaling in grass carp (Ctenopharyngodon idella) under infection of Flavobacterium columnare. Fish Shellfish Immunol. 58. doi: 10.1016/j.fsi.2016.09.029
Yan Y. Y., Xia H. Q., Yang H. L., Hoseinifar S. H., Sun Y. Z. (2016). Effects of dietary live or heat-inactivated autochthonous Bacillus pumilus SE5 on growth performance, immune responses and immune gene expression in grouper Epinephelus coioides. Aquac. Nutr. 22. doi: 10.1111/anu.12297
Yao M., Xie J., Du H., McClements D. J., Xiao H., Li L. (2020). Progress in microencapsulation of probiotics: A review. Compr. Rev. Food Sci. Food Saf. 19. doi: 10.1111/1541-4337.12532
Yaslikan N. M., Yaminudin J., Rasdi N. W., Karim M. (2023). Microfeed incorporated with probiotic for aquaculture: A review. World’s Vet. J. 13. doi: 10.54203/SCIL.2023.WVJ64
Yazhiniprabha M., Gopi N., Mahboob S., Al-Ghanim K. A., Al-Misned F., Ahmed Z., et al. (2022). The dietary supplementation of zinc oxide and selenium nanoparticles enhance the immune response in freshwater fish Oreochromis mossambicus against aquatic pathogen Aeromonas hydrophila. J. Trace Elem. Med. Biol. 69. doi: 10.1016/j.jtemb.2021.126878
Yeşilyurt N., Yılmaz B., Ağagündüz D., Capasso R. (2021). Involvement of probiotics and postbiotics in the immune system modulation. Biologics 1. doi: 10.3390/biologics1020006
Yildiz H. Y., Robaina L., Pirhonen J., Mente E., Domínguez D., Parisi G. (2017). Fish welfare in aquaponic systems: Its relation to water quality with an emphasis on feed and faeces-A review. Water (Switzerland) 9. doi: 10.3390/w9010013
Yolmeh M., Xavier-Santos D., Sant’Ana A. S. (2024). Modulating gut microbiota by paraprobiotics: Mechanisms, advantages, and challenges. Food Biosci. 60, 104305. doi: 10.1016/j.fbio.2024.104305
Yuhana M., Asvia S., Widanarni W., Afiff U. (2024). Improvement in growth, enzyme activity, and gene expression against Edwardsiellosis in African catfish (Clarias sp.) with dietary supplementation of euryhaline probiotic and paraprobiotic Bacillus cereus BR2. Aquac. Int. 32, 9303–9319. doi: 10.1007/s10499-024-01617-x
Zang L., Ma Y., Huang W., Ling Y., Sun L., Wang X., et al. (2019). Dietary Lactobacillus plantarum ST-III alleviates the toxic effects of triclosan on zebrafish (Danio rerio) via gut microbiota modulation. Fish Shellfish Immunol. 84. doi: 10.1016/j.fsi.2018.11.007
Zawistowska-Rojek A., Tyski S. (2022). How to improve health with biological agents—Narrative review. Nutrients 14. doi: 10.3390/nu14091700
Zhang H. P., Dong W. L., Chen L., Wang Y. M., Muhammad I., Ju A. Q., et al. (2020). Effects of dietary Lactobacillus plantarum C20015 on growth, immunity, and disease resistance in koi carp. Aquac. Int. 28. doi: 10.1007/s10499-020-00558-5
Zhang H., Zhang Z., Li J., Qin G. (2023). New strategies for biocontrol of bacterial toxins and virulence: focusing on quorum-sensing interference and biofilm inhibition. Toxins (Basel). 15. doi: 10.3390/toxins15090570
Zhang X., Zhong Y., Tian H., Wang W., Ru S. (2015). Impairment of the cortisol stress response mediated by the hypothalamus-pituitary-interrenal (HPI) axis in zebrafish (Danio rerio) exposed to monocrotophos pesticide. Comp. Biochem. Physiol. Part: C Toxicol. Pharmacol., 176–177. doi: 10.1016/j.cbpc.2015.07.003
Zhong X., Li J., Lu F., Zhang J., Guo L. (2022). Application of zebrafish in the study of the gut microbiome. Anim. Model. Exp. Med. 5. doi: 10.1002/ame2.12227
Zhou X. X., Wang Y. B., Li W. F. (2009). Effect of probiotic on larvae shrimp (Penaeus vannamei) based on water quality, survival rate and digestive enzyme activities. Aquaculture 287. doi: 10.1016/j.aquaculture.2008.10.046
Zhou W., Xie M., Xie Y., Liang H., Li M., Ran C., et al. (2022). The effect of dietary supplementation of Lactobacillus rhamnosus GCC-3 fermentation product on gut and liver health, and resistance against bacterial infection of the genetically improved farmed tilapia (GIFT, Oreochromis niloticus). Aquaculture. 558, 738326. doi: 10.1016/j.aquaculture.2022.738326
Zommiti M., Bouffartigues E., Maillot O., Barreau M., Szunerits S., Sebei K., et al. (2018). In vitro assessment of the probiotic properties and bacteriocinogenic potential of Pediococcus pentosaceus MZF16 isolated from artisanal Tunisian meat “dried ossban. Front. Microbiol. 9. doi: 10.3389/fmicb.2018.02607
Zorriehzahra M. J., Delshad S. T., Adel M., Tiwari R., Karthik K., Dhama K., et al. (2016). Probiotics as beneficial microbes in aquaculture: an update on their multiple modes of action: a review. Vet. Q. 36. doi: 10.1080/01652176.2016.1172132
Keywords: probiotics, paraprobiotics, bacteriocins, disease resistance, probiogenomics, sustainable aquaculture, gut microbiota modulation
Citation: Fachri M, Amoah K, Huang Y, Cai J, Alfatat A, Ndandala CB, Shija VM, Jin X, Bissih F and Chen H (2024) Probiotics and paraprobiotics in aquaculture: a sustainable strategy for enhancing fish growth, health and disease prevention-a review. Front. Mar. Sci. 11:1499228. doi: 10.3389/fmars.2024.1499228
Received: 20 September 2024; Accepted: 11 November 2024;
Published: 10 December 2024.
Edited by:
Kumbukani Mzengereza, Mzuzu University, MalawiReviewed by:
Ngoc Tuan Tran, Shantou University, ChinaCopyright © 2024 Fachri, Amoah, Huang, Cai, Alfatat, Ndandala, Shija, Jin, Bissih and Chen. This is an open-access article distributed under the terms of the Creative Commons Attribution License (CC BY). The use, distribution or reproduction in other forums is permitted, provided the original author(s) and the copyright owner(s) are credited and that the original publication in this journal is cited, in accordance with accepted academic practice. No use, distribution or reproduction is permitted which does not comply with these terms.
*Correspondence: Kwaku Amoah, YW1vYWhrMjAxMEB5YWhvby5jb20=; Huapu Chen, Y2hlbmhwQGdkb3UuZWR1LmNu
Disclaimer: All claims expressed in this article are solely those of the authors and do not necessarily represent those of their affiliated organizations, or those of the publisher, the editors and the reviewers. Any product that may be evaluated in this article or claim that may be made by its manufacturer is not guaranteed or endorsed by the publisher.
Research integrity at Frontiers
Learn more about the work of our research integrity team to safeguard the quality of each article we publish.