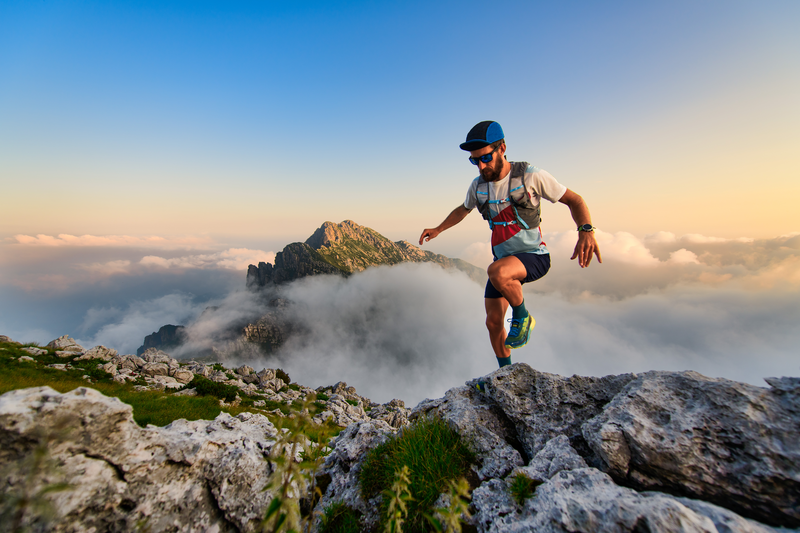
94% of researchers rate our articles as excellent or good
Learn more about the work of our research integrity team to safeguard the quality of each article we publish.
Find out more
ORIGINAL RESEARCH article
Front. Mar. Sci. , 08 November 2024
Sec. Aquatic Physiology
Volume 11 - 2024 | https://doi.org/10.3389/fmars.2024.1489436
This article is part of the Research Topic Nutritional Metabolism and Immunity of Aquatic Animals View all 8 articles
Introduction: The present study aimed to evaluate the effects of substituting fish meal with pork meal in feed on the growth performance, feed utilization, intestinal morphology, and immune function of Penaeus monodon.
Methods: A total of 600 uniformly sized juvenile shrimp with an initial weight of 1.20 ± 0.03 g were randomly assigned to four groups, with each group consisting of six replicates of 25 shrimp each. Fish meal protein was replaced with 0%, 5%, 10%, and 15% pork meal, and crystalline amino acids and fish oil were supplemented accordingly. The groups were designated as P0, P5, P10, and P15, and the study duration was 70 days.
Results: Results indicated no significant difference in specific growth rate (SGR) between the P5 and P10 groups compared to the P0 group. However, the SGR in the P15 group was significantly lower than that in the P5 and P10 groups. Feed conversion rates (FCRs) for the P5 and P10 groups were comparable to the P0 group, whereas the P15 group showed a significantly higher FCR than the other groups. There were no significant differences in whole body composition among all the groups. Apart from alanine, the amino acid composition of muscles did not differ significantly among all the groups. Results of enzyme activity in plasma revealed that the replacement of fish meal with pork meal reduced levels of aspartate aminotransferase (AST) and superoxide dismutase (SOD). Specifically, levels of AST in the P5 and P15 groups were significantly lower compared to the P0 and P10 groups. Histological examination of midgut tissues showed that villus height initially increased and then decreased with higher replacement ratios. The P5 group had the highest villus height, while the P15 group had the lowest villus height. Analysis of gene expression related to liver immune functions indicated that genes in TLR signaling pathway were significantly elevated in the P15 group compared to the other groups.
Discussion: In conclusion, even with the supplementation of crystalline amino acids and fish oil, pork meal should not replace more than 10% of fish meal in the feed of P. monodon to maintain optimal growth and feed efficiency.
In the shrimp aquaculture industry, feed costs constitute 50%–60% of the total expenses, with fish meal comprising 30%–40% of the feed (Li et al., 2023). Aquaculture expansion increases demand for fish meal. However, due to natural disasters and overfishing impacts, supplies of fish meal persistently decrease, with prices of fish meal escalating annually. This leads to rising feed costs for shrimp, significantly hindering the sustainable growth of aquaculture. Consequently, identifying new high-quality protein sources to replace fish meal has emerged as a focal point in shrimp feed nutrition research.
Penaeus monodon, popularly known as grass shrimp and black tiger shrimp, is distinguished by its large size, broad salt tolerance, resistance to high temperatures and low oxygen, and strong disease resistance among other traits (Liu et al., 2021). It is one of the three major shrimp species farmed globally. Currently, numerous studies have focused on replacing fish meal with various animal and plant protein sources in the diet of Litopenaeus vannamei (Cummins et al., 2017; Fang et al., 2016; Molina-Poveda et al., 2017; Sun et al., 2016; Valle et al., 2015; Toyes-Vargas et al., 2017). However, nutritional requirements of P. monodon are quite different from those of L. vannamei (Jiang et al., 2013; Zhang et al., 2011; Richard et al., 2011; Sriket et al., 2007). Consequently, a protein source that is appropriate for replacing fish meal in the feed of L. vannamei may not be suitable for P. monodon. Current research on alternative protein sources to fish meal in the feed of P. monodon mainly involves fermented copra meal (Apines-Amar et al., 2016), soybean meal (Lim and Dominy, 1990), microbial biomass products (Glencross et al., 2014), lupin meal (Sudaryono et al., 1999), and several seaweed meals (Simon et al., 2020; Nguyen Thi Ngoc et al., 2018). A substantial number of animal and plant protein sources have not been evaluated for the effectiveness in the feed of P. monodon.
Pork meal is a solid protein ingredient derived from fresh pork offal meat after oil extraction. It boasts high protein and lipid content, while it is affordable and has broad sourcing (Qiu et al., 2015). Common pork meal contains 70.0% crude protein and 8.0% ash, respectively. Under the same weight, the price of pork meal is only approximately 40% of that of fish meal. Due to the existence of homologous viruses and animal ethics, pork meal is forbidden to be added in pig diets, but it is not specified in other animals, so it could replace a certain proportion of fish meal in aquatic feed. The effectiveness of pork meal in feed of aquatic animals has been assessed in species such as L. vannamei (Qiu et al., 2015), Chinese mitten crab (Zhu et al., 2018), tilapia (Hernandez et al., 2010), and Furong crucian carp (Cheng et al., 2020), while its use in the feed of P. monodon has not been documented. Compared to fish meal, pork meal exhibits an imbalanced amino acid profile, which may reduce digestion and absorption rates of feed. Furthermore, due to its high lipid saturation and ash content, substituting a significant proportion of fish meal with pork meal can result in less palatability (Chang et al., 2005; Zhang et al., 2008). In addition, a high proportion of substitutions may compromise the liver and intestinal health of the cultured animals (Robaina et al., 1997). Consequently, it is essential to employ suitable nutritional strategies to mitigate the adverse effects associated with substituting fish meal with pork meal.
Thus, substituting fish meal with pork meal combined with targeted nutritional measures, may effectively mitigate the shortage of fish meal resources and cut down feed costs. The present study may offer a theoretical foundation for the development of efficient and environment-friendly formula feed for P. monodon, fostering the healthy and sustainable growth of its aquaculture industry.
Four isoproteic and isolipidic diets were formulated by replacing fish meal protein with 0%, 5%, 10%, and 15% pork meal (Table 1). According to the different substitution ratios of pork meal, four experimental groups were named P0, P5, P10, and P15. All raw materials were crushed and passed 300 μM grading screen; then, the ingredients, excluding fish oil, soybean oil, and soybean lecithin, were mixed according to the principle of gradual amplification, as outlined in the feed formula provided in Table 1. Finally, fish oil, soybean oil, and soybean lecithin were added for further mixing. After adding an appropriate amount of water, the mixture was pelleted by a granulator. The pelleted feed was dried in an oven at 45°C for 12 h. All feeds were stored in a −20°C refrigerator during the experiment.
Table 1. Composition of experimental diets for Penaeus monodon containing pork meal as a substitute for fish meal.
Juvenile P. monodon were provided by Hai xingnong Seed Co., Ltd. (Zhan Jiang, China). Before the experiment officially started, juvenile P. monodon were fed with commercial feed for 7 days. Subsequently, 600 healthy and vigorous juvenile shrimps with an initial weight of 1.20 ± 0.03 g were selected and randomly divided into four groups with six replicates in each group and 25 shrimps in each replicate. The present experiment was carried out in an indoor recirculating aquaculture system, consisting of 24 aquaculture drums. The breeding experiment lasted for 70 days. During the experiment, shrimps were fed three times daily (8:00, 12:00, and 16:00). The feeding situation was observed 1.5 h after feeding. The amount of feed in each replicate was adjusted according to the feeding situation. During the experiment, the dissolved oxygen content in the water was 4.5–5.3 mg/L, the ammonia nitrogen content was 0–0.2 mg/L, and the water temperature was 27°C–29°C.
After the breeding experiment, the survival prawns, total weight, and total feed amount of P. monodon in each barrel were counted for calculating survival rate (SR), weight gain rate (WGR), SGR, and FCR. Samples were taken after P. monodon was starved for 24 h. Three prawns were randomly selected from each barrel to measure their body length and weight for calculating condition factor (CF). A total of 12 prawns (two prawns per barrel) were randomly selected from each experimental group and stored at −20°C for body composition analysis. A total of 12 prawns (two prawns per barrel) were randomly sampled from each experimental group, and their muscle tissues were stripped and stored at −80°C for amino acid composition analysis. Six prawns (one prawns per barrel) were randomly selected from each experimental group, and the midgut was dissected and cut, fixed in Bonn’s solution, and used for preparing intestinal tissue sections. A total of 12 prawns (two prawns per barrel) were randomly selected from each experimental group; then, blood was collected with a 1-ml needle soaked in sodium heparin, after centrifuging at 3,000 rpm for 15 min at 4°C, and the supernatant serum was aspirated and stored at −80°C for detection of serum biochemical indicators. A total of 18 prawns (three prawns per barrel) were randomly selected from each experimental group, and the hepatopancreas was dissected and cut, and frozen at −80°C for gene expression detection.
The moisture, crude protein, lipid, and ash contents of P. monodon were analyzed by Association of Official Analytical Chemists (AOAC) method. The moisture content was determined by drying constant weight method (105°C, 72 h). The contents of crude protein and lipid were detected by Kjeldahl method and Soxhlet extraction method, respectively. The instruments used were a FOSS 2300 Protein automatic analyzer (FOSS, Sweden) and a Buchi 36680 fat extractor (Buchi, Switzerland). The crude ash content was determined in a box-type resistance furnace by 550°C burning method.
Amino acid content of muscles was measured according to the GB/T14965-1994 in China: the sample was hydrolyzed for 22 h at 110°C with 6M HCl in sealed glass tubes filled with nitrogen. The hydrolyzed samples were taken, and the amino acid concentration was diluted to 50nM with 0.2N sodium citrate buffer, pH 2.2. The pH-adjusted samples were analyzed by a Biochrom 20 Automatic Amino Acid Analyzer (Holliston, USA). The content of tryptophan was determined by the colorimetric method of Basha and Roberts (Basha and Roberts, 1977) after alkaline hydrolysis of each sample. All determinations were performed in triplicate.
The activities of alanine aminotransferase (ALT), AST, SOD, and ceruloplasmin (CP) in serum were detected using the ALT Assay Kit, AST Assay Kit, T-SOD assay kit (Hydroxylamine method) and CP assay kit respectively (Jiancheng, Nan Jing, China). The operation steps were carried out according to the instructions.
After the midgut tissue was fixed with Bonn’s solution, it was dehydrated, transparent, dewaxed, and embedded in alcohol step by step, and then sectioned with a tissue microtome (Leica, Wetzlar, Germany) and stained with HE staining method. The sections were observed under a Nikon eclipse ti-s microscope (Tokyo, Japan). The intestinal absorption surface area was analyzed by ImageJ software.
After liver tissues were triturated, total RNA extraction was performed using Trizol (Takara, Tokyo, Japan). Then, the absorbance values of RNA at 230 nm and 260 nm were detected using a Nanodrop 2000 (Eppendorf, Hamburg, Germany). Finally, cDNA was synthesized using Primescript™ RT Reagent Kit (Takara, Tokyo, Japan).
Real-time quantitative PCR (RT-qPCR) of target genes was performed by SYBR Green Real-Time PCR kit (Takara, Tokyo, Japan) in a fluorescence quantitative PCR machine (Bio-Rad, California, USA). In all treatments of the present experiment, no significant difference in 18s expression was found, suggesting that it could serve as a housekeeping gene. Quantitative results were analyzed by 2−ΔΔCT method (Livak and Schmittgen, 2001).
Statistical analyses were performed using SPSS 20.0. Data were presented as mean ± SEM. To determine significant differences among treatment groups, Tukey’s test was applied for post-hoc analysis following one-way ANOVA. A significance level of p< 0.05 was established for all tests. Assumptions of normality and homogeneity of variances were verified prior to analysis.
Results indicated that the SR remained unaffected among all groups, regardless of the substitution ratio of pork meal (Table 2). As the substitution ratio increased, the final weight, WGR, and SGR of P. monodon initially rose and then declined (Table 2). Substituting fish meal protein with 5% and 10% pork meal did not significantly affect these metrics in P. monodon but showed some improvement (Table 2). Conversely, substituting fish meal protein with 15% pork meal significantly reduced the final weight of P. monodon compared to the control group, with concurrent declines in the WGR and SGR, although these differences were not statistically significant. Regarding FCR, P5 and P10 groups showed no significant difference in feed coefficient from the P0 group, whereas the P15 group exhibited a significant increase. No significant differences were observed in CF of P. monodon among all groups (Table 2).
Table 2. Effects of replacing fish meal protein with pork meal and supplementing crystal amino acids and fish oil on growth performance and feed utilization of Penaeus monodon..
There were no significant differences in moisture, crude protein, crude lipid, and ash content of whole shrimp among the four groups (Table 3). In addition, the analysis of amino acid composition of the muscle revealed that there was no significant difference in the content of amino acids except alanine among four groups. As the substitution level increased from 0% to 10%, the alanine content in the muscle gradually rose, with the P10 group showing a significantly higher level than the P0 group. However, in the P15 group, the alanine content in the muscle significantly decreased (Table 4).
Table 3. Effects of replacing fish meal protein with pork meal and supplementing crystal amino acids and fish oil on body composition of Penaeus monodon.
Table 4. Effects of replacing fish meal protein with pork meal and supplementing with crystal amino acids and fish oil on the amino acid composition of in the muscle of Penaeus monodon.
Results revealed that substituting fish meal protein with 5%, 10%, and 15% pork meal did not significantly affect the ALT, SOD, and CP enzyme activities in the serum of P. monodon. However, SOD enzyme activity was lower in all three substitution groups compared to the P0 group. AST enzyme activity was also reduced in the substitution groups compared to the control, with significantly lower activity in the P5 and P15 group (Figure 1).
Figure 1. Effects of replacing fish meal protein with pork meal and supplementing with crystal amino acids and fish oil on the activity of hemolymph enzymes in Penaeus monodon. ALT, alanine aminotransferase; AST, aspartate aminotransferase; SOD, superoxide dismutase; CP, copper blue protein; P0, 0% pork meal replacement group; P5, 5% pork meal replacement group; P10, 10% pork meal replacement group; P15, 15% pork meal replacement group. The error line represents the standard error of the mean (n=6), and different letters indicate significant numerical differences (p<0.05).
Compared with the P0 group, the replacement of fish meal protein with 5%, 10%, and 15% pork meal changed the histomorphology of the midgut of P. monodon (Figure 2). The height of intestinal villi in the P5 group was the highest and significantly higher than that in the P0 and P15 groups. Although there was no significant difference in the height of intestinal villi between the P10 group and the P0 group, there was also an upward trend, and it was significantly higher than that in the P15 group. In contrast to the results of the P5 and P10 groups, the height of intestinal villi in the P15 group was the lowest among four groups and was significantly lower than that of the other three groups (Figure 3).
Figure 2. Effect of replacing fish meal protein with pork meal and supplementing with crystal amino acids and fish oil on the intestinal tissue morphology of Penaeus monodon. P0, 0% pork meal replacement group; P5, 5% pork meal replacement group; P10, 10% pork meal replacement group; P15, 15% pork meal replacement group.
Figure 3. Effect of replacing fish meal protein with pork meal and supplementing with crystal amino acids and fish oil on the intestinal villus height of Penaeus monodon. P0, 0% pork meal replacement group; P5, 5% pork meal replacement group; P10, 10% pork meal replacement group; P15, 15% pork meal replacement group. The error line represents the standard error of the mean (n=7), and different letters indicate significant numerical differences (p<0.05).
The results of gene expression revealed that the expression of Toll, myeloid differentiation primary response gene 88 (MyD88), tumor necrosis factor receptor associated factor 6 (TRAF6), and heat shock protein 70 (HSP70) remained stable initially and then increased with the rising substitution ratio, peaking in the P15 group. There was no significant difference in the expression of immune-related genes in the P5 and P10 groups compared with the P0 group. While the expression of myd88 and traf6 in the P15 group was significantly elevated compared with the P10 group. Although there was no significant difference in the expression of toll and hsp70 between the P15 group and the P0 group, a rising trend was found (Figure 4).
Figure 4. Effect of replacing fish meal protein with pork meal and supplementing with crystal amino acids and fish oil on the immune gene expression of hepatopancreas in Penaeus monodon. MyD88, myeloid differentiation primary response gene 88; TRAF6, tumor necrosis factor receptor associated factor 6; HSP70, heat shock protein 70. P0, 0% pork meal replacement group; P5, 5% pork meal replacement group; P10, 10% pork meal replacement group; P15, 15% pork meal replacement group. The error line represents the standard error of the mean (n=7), and different letters indicate significant numerical differences (p<0.05).
Since the mid-twentieth century, rendered animal protein meals have been used in animal feeds (Tacon, 2020). Pork meal is an animal protein source with a wide range of sources and relatively low price. To date, the effect of pork meal in the feed of P. monodon has not been evaluated.
Hernandez et al. found that while replacing up to 40% of fish meal protein with pork meal did not significantly inhibit the SGR of L. vannamei, overall growth performance still showed a downward trend as the replacement ratio increased (Hernandez et al., 2008). In contrast to this study, the present study demonstrated that a low proportion of pork meal substitution promoted the growth of P. monodon. Lim et al. indicated that pork meal is rich in protein and lipids, making it suitable for formulating feeds for certain carnivorous fish species (Lim et al., 2021). Given that P. monodon is primarily carnivorous, this may explain why pork meal is well-suited for this species. Additionally, the study of Hernandez et al. did not include the supplementation of fish oil and crystalline amino acids (Hernandez et al., 2008). In the present study, the inclusion of crystalline amino acids helped balance the amino acid profile, thereby enhancing protein absorption of feeds. A past study have suggested that the slow growth observed when replacing fish meal with alternative animal protein sources may be attributed to the reduced content of unsaturated fatty acids due to lower fish oil levels in the feed (Ma et al., 2020). The high levels of saturated fatty acids in pork meal have also been identified as a contributing factor to decreased growth performance (Fei, 2017). Therefore, the supplementation of fish oil in the present study, which provided polyunsaturated fatty acids such as EPA and DHA, may contributed to the observed growth promotion.
Similar to the findings of the present study, other studies have demonstrated that replacing fish meal with high proportions of pork meal can inhibit the growth of cultured animals. Huang et al. found that when pork meal replaced more than 30% of fish meal, the growth performance of Trachinotus ovatus was negatively affected (Huang et al., 2022). Hernandez et al. reported that substituting 40% of fish meal with pork meal reduced the growth rate of L. vannamei (Hernandez et al., 2008). Lee et al. observed that replacing more than 20% of fish meal with pork meal had a significant adverse impact on the growth and development of Sebastes schlegelii (Lee et al., 2024). The imbalance in amino acid composition and the low digestibility of pork meal are likely reasons why excessive replacement of fish meal negatively affects feed palatability. Additionally, in shrimp farming, the prolonged presence of feed in the water leads to the rapid dissolution of crystalline amino acids, making it difficult to maintain a balanced amino acid profile under high replacement levels.
Consistent with results about growth performance, in the present study, when the replacement level was <10%, there was no significant difference in the FCR of the feed compared to the control group, indicating that within this replacement range, the shrimp could effectively absorb and convert the nutrients. However, when the replacement level exceeded 10%, the FCR of the feed became significantly higher than that of the other groups. This phenomenon has also been observed in studies on Oreochromis niloticus (Hernandez et al., 2010), Furong crucian carp (Cheng et al., 2020). and Micropterus salmoides (Bian et al., 2022).
Regarding whole body composition of P. monodon, the present study found that different levels of pork meal substitution had no significant effect on the moisture, crude protein, and ash content of P. monodon. This result aligns with findings in L. vannamei (Qiu et al., 2015) and M. salmoides (Bian et al., 2022). Although there was no significant difference in crude lipid content across the four groups, the 15% substitution group exhibited slightly higher crude lipid levels compared to the other groups. This could be attributed to the higher lipid content in pork meal, which is rich in saturated fatty acids, potentially leading to excessive lipid accumulation due to less efficient lipid metabolism (Zhou et al., 2005).
Amino acid profiles in the muscle and other tissues are commonly used to assess the dietary amino acid utilization (Mambrini and Kaushik, 1995). Several studies on various species have shown that substituting FM with alternative protein sources, without amino acids supplementation, can lead to reduced levels of certain amino acids (Kari et al., 2022; Xu et al., 2022). In addition, the amino acid composition of the muscle, especially the level of flavor amino acids, can affect the flavor of the muscle of farmed animals (Espe, 2008), which then affect the economic benefits of breeding animals. In the present study, the muscle of P. monodon in the P10 group showed significantly higher alanine content compared to that in the P0 group. This increase can be explained by the higher alanine content in pork meal relative to fish meal (Hernandez et al., 2010), along with the fact that P. monodon in the P10 group did not show significant differences in FCR compared to the control group. However, in the P15 group, where FCR was significantly lower than that of the P0 group, the muscle alanine content was significantly reduced. In addition, the levels of umami amino acids such as glutamic acid, aspartic acid, phenylalanine, glycine, and tyrosine did not differ significantly among the four groups, suggesting that the substitution of fish meal with pork meal may not markedly affect the muscle flavor of P. monodon.
The intestine is the primary digestive organ in shrimp, and the height of intestinal villi is a key indicator of nutrient absorption capacity, as higher villi indicate a greater absorptive surface area (Mai et al., 2020). In the present study, when pork meal substitution was below 10%, the midgut villus height of P. monodon increased, which may partially explain why the low substitution groups did not show significant differences in growth performance and feed conversion efficiency compared to the control group. In contrast, in the P15 group, villus height was significantly reduced, which could be a contributing factor to the observed growth inhibition and increased feed conversion ratio in this group. Similar to these findings, Huang et al. reported that when pork meal replaced fish meal at a high level in the diet of T. ovatus (Huang et al., 2022), a decrease in villus height in the foregut and midgut was observed, indicating that excessive substitution level can alter the intestinal morphology, which consequently damage the digestive and absorptive capacity of the fish.
The levels of AST and ALT in the blood are primary indicators used to assess hepatopancreatic damage in shrimp (Zhang et al., 2019; Nyblom et al., 2004). These enzymes are predominantly located within the organelles of hepatocytes, and their elevated serum levels correspond with the extent of hepatocyte damage due to the excessive release of these active enzymes. In the present study, the levels of AST were significantly lower in all substitution groups compared to the control group, suggesting that substituting fish meal with pork meal reduced liver damage in P. monodon to some extent. Similar results were reported by Lee et al., where substituting fish meal with pork meal did not significantly affect serum ALT and AST levels in S. schlegelii. However, in that study, the levels of AST in all substitution groups were also lower than those in the control group (Lee et al., 2024).
The antioxidant capacity of the body reflects its ability to eliminate excess reactive oxygen species (ROS), which is critical for defending against oxidative stress and maintaining redox homeostasis. SOD is considered the primary defense against oxidative stress, as it catalyzes the conversion of superoxide anion (O2−) into oxygen (O2) and hydrogen peroxide (H2O2); the latter is subsequently converted into non-toxic water (H2O) by catalase (CAT) and glutathione peroxidase (GSH-Px) (Mai et al., 2020). In the present study, SOD activity in plasma decreased with increasing levels of pork meal substitution, indicating that excessive pork meal in the diet reduced the antioxidant capacity of P. monodon. This finding is consistent with that of Lee et al. (2024).
Previous studies have shown that replacing fish meal with a high proportion of pork meal can lead to immune and inflammatory responses in aquatic animals. As shown in the study of juvenile golden pompano, when more than 30% of fish meal in the feed was replaced by pork meal, Nf-κB signaling pathway was activated, significantly increasing the gene expression of pro-inflammatory cytokines IL-1β and IL-8 and reducing the expression of anti-inflammatory cytokines IL-10 and TGF-β (Huang et al., 2022). In the case of Macrobrachium rosenbergii, when the proportion of pork meal in the feed reaches 15%, the levels of immunoglobulin and lysozyme in the plasma were significantly reduced (Zhai et al., 2023). However, there has been no report on the effect of replacing fish meal with pork meal on the immune response of P. monodon. Therefore, the present study analyzed the impact of pork meal substitution for fish meal on the immune response of liver in P. monodon. Unlike vertebrates, which possess a well-developed immune system, invertebrates primarily rely on their innate immune system to defend against pathogens (Li and Xiang, 2013). The Toll-like receptors (TLRs) pathway plays a crucial role in innate immunity, activating the expression of inflammatory factors via MyD88-dependent and MyD88-independent pathways (Medzhitov et al., 1997). Studies have shown that nutrients like fatty acids can regulate the expression of toll and the myd88 (Ding et al., 2018; Zuo et al., 2012). In addition, recent work has provided evidence that endogenous HSPs, which are thought to be released from dying infected cells, can increase the activation of innate immune responses via interactions with Toll-like receptors (Osterloh et al., 2004; Asea et al., 2002; Akira et al., 2006). In addition, studies in L. vannamei showed that the gene expression of hsp70 was significantly upregulated in shrimp infected with Vibrio alginolyticus and Vibrio parahaemolyticus (Zhou et al., 2010; Junprung et al., 2019). In the present study, it was found that a 15% pork meal substitution for fish meal significantly upregulated the expression of myd88 and its downstream gene traf6, indicating an enhanced immune response in this group. The expression of hsp70 also increased in P15 group compared with P0 group. The results indicated that when the proportion of pork meal in the diet of P. monodon reached 15%, the innate immune response of shrimp was activated. This may be due to compromised digestion and absorption, leading to weakened shrimp and increased susceptibility to pathogens in the surrounding water environment.
In conclusion, the present study found that substituting up to 10% of fish meal with pork meal, along with the corresponding supplementation of fish oil and crystalline amino acids, did not adversely affect the growth performance, body composition, muscle amino acid composition, and liver and intestinal health of P. monodon. However, when the substitution level reached 15%, significant growth inhibition, increased feed conversion ratio, and adverse effects on intestinal morphology were observed.
The original contributions presented in the study are included in the article/supplementary material. Further inquiries can be directed to the corresponding author.
The animal study was approved by Animal ethics committee of Jimei University. The study was conducted in accordance with the local legislation and institutional requirements.
QC: Writing – original draft. CZ: Writing – review & editing. BY: Project administration, Supervision, Writing – review & editing. SW: Methodology, Supervision, Writing – review & editing. JM: Project administration, Writing – review & editing. XQ: Funding acquisition, Resources, Supervision, Writing – review & editing.
The author(s) declare that financial support was received for the research, authorship, and/or publication of this article. This research was supported by Panyu Innovation and Entrepreneurship Leading Team Project (2021-R01-4).
We thank Guijuan Yu, Yanan Shen, and Ruili Jiang for their help during the experiment.
Authors QC, BY, SW, JM, and XQ were employed by the company Guangdong Haid Group Co., Ltd.
The remaining author declares that the research was conducted in the absence of any commercial or financial relationships that could be construed as a potential conflict of interest.
All claims expressed in this article are solely those of the authors and do not necessarily represent those of their affiliated organizations, or those of the publisher, the editors and the reviewers. Any product that may be evaluated in this article, or claim that may be made by its manufacturer, is not guaranteed or endorsed by the publisher.
Akira S., Uematsu S., Takeuchi O. (2006). Pathogen recognition and innate immunity. Cell 124, 783–801. doi: 10.1016/j.cell.2006.02.015
Apines-Amar M. J. S., Andrino-Felarca K. G. S., Cadiz R. E., Corre V. L. Jr., Calpe A. T. (2016). Effects of partial replacement of fish meal by fermented copra meal on the growth and feed efficiency in black tiger shrimp, Penaeus monodon. Israeli J. Aquaculture-Bamidgeh 68, 1244. doi: 10.46989/001c.20837
Asea A., Rehli M., Kabingu E., Boch J. A., Baré O., Auron P. E., et al. (2002). Novel signal transduction pathway utilized by extracellular HSP70: role of Toll-like receptor (TLR) 2 and TLR4*. J. Biol. Chem. 277, 15028–15034. doi: 10.1074/jbc.M200497200
Basha S. M., Roberts R. M. (1977). A simple colorimetric method for the determination of tryptophan. Analytical Biochem. 77, 378–386. doi: 10.1016/0003-2697(77)90251-2
Bian Y., Yang H., Xu X., Lin X., Cao K., Li X., et al. (2022). Effects of replacing dietary fish meal with fermented soybean meal, meat and bone meal and their mixture on growth, serum biochemical indices and intestinal histology of largemouth bass (Micropterus salmoides). J. Fisheries China 46, 1813–1823.
Chang Q., Liang M., Wang J., Zhai Y. (2005). Apparent digestibility coefficients of various feed ingredients for Japanese sea bass (Lateolabrax japonicus). Acta Hydrobiologica Sin. 29, 172–176. doi: 10.3724/issn1000-3207-2005-2-175-r
Cheng X., Jiang G., Xiang J., Song R., Li S., Wu Y., et al. (2020). Effects of dietary fishmeal replacement with meat and bonemeal on the growth performance, blood physiological and biochemical indices, muscle chemical composition and texture characteristics in juvenile furong crucian carp (Furong carp ♀× red crucian carp ♂). Acta Hydrobiologica Sin. 44, 85–94.
Cummins V. C. Jr., Rawles S. D., Thompson K. R., Velasquez A., Kobayashi Y., Hager J., et al. (2017). Evaluation of black soldier fly (Hermetia illucens) larvaemeal as partial or total replacement of marine fish meal in practical diets for Pacific white shrimp (Litopenaeus vannamei). Aquaculture 473, 337–344. doi: 10.1016/j.aquaculture.2017.02.022
Ding Z., Zhou J., Kong Y., Zhang Y., Cao F., Luo N., et al. (2018). Dietary arachidonic acid promotes growth, improves immunity, and regulates the expression of immune-related signaling molecules in Macrobrachium nipponense (De Haan). Aquaculture 484, 112–119. doi: 10.1016/j.aquaculture.2017.11.010
Espe M. (2008). 9 - Understanding factors affecting flesh quality in farmed fish In Improving Farmed Fish Quality and Safety. Ed. Lie Ø. (Woodhead Publishing Series in Food Science, Technology and Nutrition), 241–264. doi: 10.1533/9781845694920.2.241
Fang X., Yu D., Buentello A., Zeng P., Davis D. A. (2016). Evaluation of new non- genetically modified soybean varieties as ingredients in practical diets for Litopenaeus vannamei. Aquaculture 451, 178–185. doi: 10.1016/j.aquaculture.2015.08.026
Fei G. (2017). Discriminant analysis of animal-derived feedstuffs of different species by spectroscopy based on lipid characteristics (Beijing, China: China Agricultural University). Doctor degree thesis dissertation.
Glencross B., Irvin S., Arnold S., Blyth D., Bourne N., Preston N. (2014). Effective use of microbial biomass products to facilitate the complete replacement of fishery resources in diets for the black tiger shrimp, Penaeus monodon. Aquaculture 431, 12–19. doi: 10.1016/j.aquaculture.2014.02.033
Hernandez C., Olvera-Novoa M. A., Aguilar-Vejar K., Gonzalez-Rodriguez B., Abdo de la Parra I. (2008). Partial replacement of fish meal by porcine meat meal in practical diets for Pacific white shrimp (Litopenaeus vannamei). Aquaculture 277, 244–250. doi: 10.1016/j.aquaculture.2008.02.016
Hernandez C., Olvera-Novoa M. A., Hardy R. W., Hermosillo A., Reyes C., Gonzalez B. (2010). Complete replacement of fish meal by porcine and poultry by-product meals in practical diets for fingerling Nile tilapia Oreochromis niloticus: digestibility and growth performance. Aquaculture Nutr. 16, 44–53.
Huang J., Zhou C., Xu F., Luo X., Huang X., Huang Z., et al. (2022). Effects of partial replacement of fish meal with porcine meat meal on growth performance, antioxidant status, intestinal morphology, gut microflora and immune response of juvenile golden pompano (Trachinotus ovatus). Aquaculture 561, 738646. doi: 10.1016/j.aquaculture.2022.738646
Jiang S., Huang J., Lin H., Yang Q., Zhou F., Qiu L., et al. (2013). Effects of dietary protein levels on the growth and survival of Penaeus monodon from different families. J. Shanghai Ocean Univ. 22, 349–356.
Junprung W., Supungul P., Tassanakajon A. (2019). Litopenaeus vannamei heat shock protein 70 (LvHSP70) enhances resistance to a strain of Vibrio parahaemolyticus, which can cause acute hepatopancreatic necrosis disease (AHPND), by activating shrimp immunity. Dev. Comp. Immunol. 90, 138–146. doi: 10.1016/j.dci.2018.09.011
Kari Z. A., Kabir M., Dawood M. A. O., Razab M. K. A. A., Ariff N. S. N. A., Sarkar T., et al. (2022). Effect of fish meal substitution with fermented soy pulp on growth performance, digestive enzyme, amino acid profile, and immune-related gene expression of African catfish (Clarias gariepinus). Aquaculture 546, 737418. doi: 10.1016/j.aquaculture.2021.737418
Lee T. H., Oh H. Y., Lee G. J., Kim H. S., Choi J. (2024). Effects of replacing fish meal with a blend of corn gluten meal and meat meal on the growth, biochemical profile, digestive enzyme activity, antioxidant status, and innate immunity of juvenile black rockfish (Sebastes schlegelii). Aquaculture 579, 740225. doi: 10.1016/j.aquaculture.2023.740225
Li F., Xiang J. (2013). Recent advances in researches on the innate immunity of shrimp in China. Dev. Comp. Immunol. 39, 11–26. doi: 10.1016/j.dci.2012.03.016
Li F., Xie S., Yun B., Qian X. (2023). Effects of dietary protein levels on growth performance, digestive enzyme activities and serum biochemical indices of Penaeus monodon. Feed industry 44, 76–82.
Lim C., Dominy W. (1990). Evaluation of soybean-meal as a replacement for marine animal protein in diets for shrimp (Penaeus vannamei). Aquaculture 87, 53–63. doi: 10.1016/0044-8486(90)90210-E
Lim M. T., Pan B. J., Toh D. W. K., Sutanto C. N., Kim J. E. (2021). Animal protein versus plant protein in supporting lean mass and muscle strength: A systematic review and meta-analysis of randomized controlled trials. Nutrients 13, 661. doi: 10.3390/nu13020661
Liu M., Zhang H., Sun G., Wang Z., Jiang W. (2021). Research progress on nutritional requirements and functional feed additives of Penaeus maculatus. Curr. Fisheries 46.
Livak K. J., Schmittgen T. D. (2001). Analysis of relative gene expression data using real-time quantitative PCR and the 2–ΔΔCT method. Methods 25, 402–408. doi: 10.1006/meth.2001.1262
Ma Y., Li Y., Xu C., Li M., Chen H., Ye R., et al. (2020). Diet with a high proportion replacement of fishmeal by terrestrial compound protein displayed better farming income and environmental benefits in the carnivorous marine teleost (Trachinotus ovatus). Aquaculture Rep. 18, 100449. doi: 10.1016/j.aqrep.2020.100449
Mai H., Guo X., Wang J., Chi S., Dong X., Yang Q., et al. (2020). Effects of dietary protein levels on intestinal tract histomorphology and microflora composition in juvenile pearl gentian grouper (Epinephelus lanceolatu ♂× E. fuscoguttatus ♀). J. Dailian Ocean Univ. 35, 63–70.
Mambrini M., Kaushik S. J. (1995). Indispensable amino acid requirements of fish: correspondence between quantitative data and amino acid profiles of tissue proteins. J. Appl. Ichthyology 11, 240–247. doi: 10.1111/j.1439-0426.1995.tb00024.x
Medzhitov R., PrestonHurlburt P., Janeway C. A. (1997). A human homologue of the Drosophila Toll protein signals activation of adaptive immunity. Nature 388, 394–397. doi: 10.1038/41131
Molina-Poveda C., Cardenas R., Jover M. (2017). Evaluation of amaranth (Amaranthus caudatus L.) and quinoa (Chenopodium quinoa) protein sources as partial substitutes for fish meal in Litopenaeus vannamei grow-out diets. Aquaculture Res. 48, 822–835. doi: 10.1111/are.2017.48.issue-3
Nguyen Thi Ngoc A., Tran Ngoc H., Tran Thi Thanh H. (2018). Effects of partial replacement of fishmeal protein with green seaweed (Cladophora spp.) protein in practical diets for the black tiger shrimp (Penaeus monodon) postlarvae. J. Appl. Phycology 30, 2649–2658. doi: 10.1007/s10811-018-1457-7
Nyblom H., Berggren U., Balldin J., Olsson R. (2004). High AST/ALT ratio may indicate advanced alcoholic liver disease rather than heavy drinking. Alcohol Alcoholism 39, 336–339. doi: 10.1093/alcalc/agh074
Osterloh A., Meier-Stiegen F., Veit A., Fleischer B., von Bonin A., Breloer M. (2004). Lipopolysaccharide-free heat shock protein 60 activates T cells*. J. Biol. Chem. 279, 47906–47911. doi: 10.1074/jbc.M408440200
Qiu H., Li Y., Hou Y., Zhou Q. (2015). Effect of partial replacement of fish meal by poultry by-product meal or pork meat powder on growth performance, feed utilization and serum biochemical indexes of pacific white shrimp (Litopenaeus vannamei). Chin. J. Anim. Nutr. 27, 2784–2792.
Richard L., Surget A., Rigolet V., Kaushik S. J., Geurden I. (2011). Availability of essential amino acids, nutrient utilisation and growth in juvenile black tiger shrimp, Penaeus monodon, following fishmeal replacement by plant protein. Aquaculture 322, 109–116. doi: 10.1016/j.aquaculture.2011.09.032
Robaina L., Moyano F. J., Izquierdo M. S., Socorro J., Vergara J. M., Montero D. (1997). Corn gluten and meat and bone meals as protein sources in diets for gilthead seabream (Sparus aurata): Nutritional and histological implications. Aquaculture 157, 347–359. doi: 10.1016/S0044-8486(97)00174-9
Simon C. J., Truong H. H., Noble T. H., Osborne S. A., Wynne J. W., Wade N. M. (2020). Microbial biomass, marine invertebrate meals and feed restriction influence the biological and gut microbiota response of shrimp Penaeus monodon. Aquaculture 520, 734679. doi: 10.1016/j.aquaculture.2019.734679
Sriket P., Benjakul S., Visessanguan W., Kijroongrojana K. (2007). Comparative studies on chemical composition and thermal properties of black tiger shrimp -: (Penaeus monodon) and white shrimp (Penaeus vannamei) meats. Food Chem. 103, 1199–1207. doi: 10.1016/j.foodchem.2006.10.039
Sudaryono A., Tsvetnenko E., Evans L. H. (1999). Replacement of soybean meal by lupin meal in practical diets for juvenile Penaeus monodon. J. World Aquaculture Soc. 30, 46–57. doi: 10.1111/j.1749-7345.1999.tb00316.x
Sun H., Tang J.-W., Yao X.-H., Wu Y.-F., Wang X., Liu Y. (2016). Effects of replacement of fish meal with fermented cottonseed meal on growth performance, body composition and haemolymph indexes of Pacific white shrimp, Litopenaeus vannamei Boon. Aquaculture Res. 47, 2623–2632. doi: 10.1111/are.2016.47.issue-8
Tacon A. G. J. (2020). Trends in global aquaculture and aquafeed production: 2000–2017. Rev. Fisheries Sci. Aquaculture 28, 43–56. doi: 10.1080/23308249.2019.1649634
Toyes-Vargas E., Maria Calderon-de la Barca A., Duran-Encinas Y., Palacios E., Civera-Cerecedo R. (2017). Marine co-product meals as a substitute of fishmeal in diets for white shrimp Litopenaeus vannamei improve growth, feed intake and muscle HUFA composition. Aquaculture Res. 48, 3782–3800. doi: 10.1111/are.13205
Valle B. C. S., Dantas E. M., Silva J. F. X., Bezerra R. S., Correia E. S., Peixoto S. R. M., et al. (2015). Replacement of fishmeal by fish protein hydrolysate and biofloc in the diets of Litopenaeus vannamei postlarvae. Aquaculture Nutr. 21, 105–112. doi: 10.1111/anu.2015.21.issue-1
Xu X., Yang H., Zhang C., Bian Y., Yao W., Xu Z., et al. (2022). Effects of replacing fishmeal with cottonseed protein concentrate on growth performance, flesh quality and gossypol deposition of largemouth bass (Micropterus salmoides). Aquaculture 548, 737551. doi: 10.1016/j.aquaculture.2021.737551
Zhai H., Chen M., Yun B., Qian X., Jiang D., Yuan R., et al. (2023). Effect of replacing fish meal by pork meal on growth performance, feed utilization and immune performance of Macrobrachium rosenbergii. Feed Res. 46, 55–58.
Zhang J., Huang Z., Lin H., Niu J., Lv G., Chen Y., et al. (2011). Effects of dietary protein content on growth performance and digestive emzyme activity of juvenile tiger shrimp, penaeus monodon. (Xiamen, Fujian, China: China Society of Fisheries).
Zhang S., ShouQi X., XiaoMing Z., Wu L., Dong H., YunXia Y. (2008). Effect of faecal collection interval and dietary meat and bone meal levels on digestibility of nutrients in gibel carp (Carassius auratus gibelio). Acta Hydrobiologica Sin. 32, 79–90.
Zhang Y., Chen P., Liang X. F., Han J., Wu X. F., Yang Y. H., et al. (2019). Metabolic disorder induces fatty liver in Japanese seabass, Lateolabrax japonicas fed a full plant protein diet and regulated by cAMP-JNK/NF-kB-caspase signal pathway. Fish Shellfish Immunol. 90, 223–234. doi: 10.1016/j.fsi.2019.04.060
Zhou J., Wang W.-N., He W.-Y., Zheng Y., Wang L., Xin Y., et al. (2010). Expression of HSP60 and HSP70 in white shrimp, Litopenaeus vannamei in response to bacterial challenge. J. Invertebrate Pathol. 103, 170–178. doi: 10.1016/j.jip.2009.12.006
Zhou Q., Mai K., Liu Y., Tan B. (2005). Advances in animal and plant protein sources in place of fish meal. J. Fisheries China 29, 404–410.
Zhu S., Long X., Xiang C., Zhang J., Deng D., Zhou Y., et al. (2018). Effects of dietary fishmeal replacement with protein mixtures on growth performance, physiological metabolism and biochemical composition of juvenile Chinese mitten crab (Eriocheir sinensis). Acta Hydrobiologica Sin. 44, 85–94.
Zuo R., Ai Q., Mai K., Xu W., Wang J., Xu H., et al. (2012). Effects of dietary n-3 highly unsaturated fatty acids on growth, nonspecific immunity, expression of some immune related genes and disease resistance of large yellow croaker (Larmichthys crocea) following natural infestation of parasites (Cryptocaryon irritans). Fish Shellfish Immunol. 32, 249–258. doi: 10.1016/j.fsi.2011.11.005
Keywords: fish meal, growth performance, immune response, intestinal morphology, nutrition regulation, Penaeus monodon, pork meal
Citation: Chen Q, Zhang C, Yun B, Wang S, Ma J and Qian X (2024) Effects of replacing fish meal with pork meal and supplementing with crystalline amino acids and fish oil on growth performance, body composition, enzyme activity, intestinal morphology, and immune response of Penaeus monodon. Front. Mar. Sci. 11:1489436. doi: 10.3389/fmars.2024.1489436
Received: 01 September 2024; Accepted: 10 October 2024;
Published: 08 November 2024.
Edited by:
Songlin Li, Shanghai Ocean University, ChinaReviewed by:
Sofia Priyadarsani Das, National Taiwan Ocean University, TaiwanCopyright © 2024 Chen, Zhang, Yun, Wang, Ma and Qian. This is an open-access article distributed under the terms of the Creative Commons Attribution License (CC BY). The use, distribution or reproduction in other forums is permitted, provided the original author(s) and the copyright owner(s) are credited and that the original publication in this journal is cited, in accordance with accepted academic practice. No use, distribution or reproduction is permitted which does not comply with these terms.
*Correspondence: Xueqiao Qian, cXhxQGhhaWQuY29tLmNu
Disclaimer: All claims expressed in this article are solely those of the authors and do not necessarily represent those of their affiliated organizations, or those of the publisher, the editors and the reviewers. Any product that may be evaluated in this article or claim that may be made by its manufacturer is not guaranteed or endorsed by the publisher.
Research integrity at Frontiers
Learn more about the work of our research integrity team to safeguard the quality of each article we publish.