- 1Università di Sassari, Dipartimento di Scienze Chimiche, Fisiche, Matematiche e Naturali, Sassari, Italy
- 2National Biodiversity Future Center, Palermo, Italy
- 3Università di Palermo, Dipartimento di Scienze della Terra e del Mare, Palermo, Italy
In this study, we explored the fish acoustic community at two coralligenous sites in Sardinia (Tavolara and Santa Teresa, western Mediterranean Sea) during the summer 2023. Our goal was to understand spatial and temporal patterns of fish acoustic activity on different temporal scales to offer insights for optimizing acoustic monitoring of this crucial ecosystem. We identified seventeen distinct sound types, revealing a diverse acoustic community. Tavolara had higher acoustic richness and abundance compared to Santa Teresa, which may be attributed to site-specific factors such as habitat structure, species composition, or levels of protection. Temporal analysis revealed clear diel patterns, with certain sounds associated with nocturnal or diurnal periods, reflecting the daily rhythms of different species. The study also examined how recording duration influenced acoustic richness, finding that longer recordings (15 minutes per hour and 15 minutes per hour at night) provided a more comprehensive detection of acoustic activity. Additionally, the number of recording days required to detect species richness varied depending on the site. While extended recordings improve the likelihood of detecting rare or sporadic sounds, they also present challenges in data management and equipment maintenance. The study underscores the importance of carefully planning sampling strategies to optimize acoustic monitoring and ensure effective and sustainable ecological research in coralligenous ecosystems.
1 Introduction
Fish produce and utilize sounds for mate attraction, social cohesion, nest and territorial defence, and orientation (Amorim, 2006), but they also generate unintentional sounds during movement, navigation and feeding. By monitoring changes in these biological sounds, it is possible to gain insights into the presence and activities of species (Sueur et al., 2008), potentially identifying crucial ecological behaviours. For this reason, in recent decades, passive acoustic monitoring of aquatic sounds has gained importance as a non-invasive method to evaluate species richness and assess the status of marine ecosystems (Sueur and Farina, 2015; Mooney et al., 2020). This approach involves identifying significant biological sounds and estimating their temporal and spatial patterns (Gibb et al., 2019; Mooney et al., 2020).
Coral reefs are among the most biodiverse ecosystems on the planet, in both tropical (Pandolfi et al., 2011) and temperate environments (Ballesteros, 2006; Teixidó et al., 2013; Martin et al., 2014). Healthy coral reefs exhibit a rich and complex soundscape, characterised by diverse sounds generated by fish, invertebrates, and other marine organisms. In contrast, degraded reefs show a significant reduction in acoustic diversity, reflecting a loss of biodiversity and ecological functions (Kaplan et al., 2015). Acoustic monitoring provides valuable insights into the presence and ecologically relevant behaviour of reef organisms, which otherwise are challenging to be observed directly, particularly in the intricate and dynamic environment of a coral reef. By capturing the sounds associated with these activities, it is possible to gain a deeper understanding of the ecological processes that sustain reef ecosystems. Furthermore, changes in the acoustic environment of coral reefs can reveal impacts of human activities and climate change (Lin et al., 2021), such as mass mortality events and shifts in species composition due to warming events (La Manna et al., 2024a; Mullet et al., 2024). Thus, acoustic monitoring not only can help tracking animal populations and behaviours, but it also allows detecting environmental changes and anthropogenic impacts, making it a complementary tool for conservation and ecological studies (Krause and Farina, 2016).
While acoustic monitoring of tropical coral reefs has progressively increased over the past few years, studies on temperate coral reefs remain limited (Di Iorio et al., 2021; La Manna et al., 2024a). In particular, the acoustic properties of Mediterranean coralligenous habitats have been poorly investigated (Di Iorio et al., 2021), despite the considerable efforts made in studying other key Mediterranean habitats, such as Posidonia oceanica meadows, rocky coastal reefs (e.g., Di Iorio et al., 2018, 2020; Parmentier et al., 2018; Desiderà et al., 2019; Di Iorio et al., 2020; Picciulin et al., 2021, 2022; La Manna et al., 2021; Bolgan et al., 2022; La Manna et al., 2024b) and even submarine canyon (Bolgan et al., 2020).
The coralligenous is defined as biogenic temperate reefs primarily formed by the accumulation of calcareous encrusting algae (e.g., crustose coralline algae and Peyssonneliales), along with animals such as bryozoans, anthozoans, and serpulids, typically found in the circalittoral zone (at depths between 20 and 120 meters), under sciaphilous condition (Ballesteros, 2006). Due to its high complexity and heterogeneity, the coralligenous ecosystem hosts several communities (La Rivière et al., 2021), supporting one of the highest biodiversity hotspots in the Mediterranean Sea (Ballesteros, 2006). Consequently, this ecosystem is protected under European regulations, including the 92/43/CE Habitat Directive and the 2008/56/EC Marine Strategy Framework Directive. However, these ecosystems face numerous threats from human-induced stressors (Piazzi et al., 2012), such as sediment re-suspension (Balata et al., 2005; Mateos-Molina et al., 2015), eutrophication (Piazzi et al., 2018), mechanical disturbances from fishing and anchoring (Bavestrello et al., 1997; Hinz, 2017), and water warming (Zunino et al., 2017). Particularly, elevated sea water temperature has led to species mass mortality events and a reduction in community structural complexity and resilience over the past few decades (Garrabou et al., 2009; Ponti et al., 2014; Garrabou et al., 2022).
Traditionally, the monitoring of coralligenous habitats has relied on visual methods, such as scuba diving surveys and underwater photography (Di Camillo et al., 2023). These techniques, while valuable, are limited by 1) the habitat depth range which poses significant logistical challenges, including limited dive times, the need for specialised equipment, and the inherent risks associated with deep diving and 2) the complex and heterogeneous habitat structure due to dense assemblages of calcareous algae, bryozoans, and anthozoans which provide numerous hiding spots for fishes complicating accurate fish populations assessment (due to the difficulties in their direct observation and species identification). Moreover, visual methods can only provide instant or temporary limited pictures of reef biodiversity (Jarriel et al., 2024) underestimating the fish community dynamics. Consequently, studying the fish communities associated with the coralligenous habitats has been particularly neglected. To fill these gaps in knowledge, passive acoustic monitoring (PAM) appears to be a crucial and non-invasive tool for providing continuous information over extended periods, allowing for the detection of the acoustic presence of fish and other marine organisms. This is particularly useful for studying nocturnal or cryptic species. In this context, Di Iorio et al. (2021) recently conducted the first investigation of the fish-related biophony of coralligenous reefs, sampling 27 sites across the north-western Mediterranean Sea using passive acoustic monitoring devices. Their aim was to analyse regional variation in acoustic fish composition and the ecological conditions influencing the acoustic biodiversity of these ecosystems. The study reported the highest acoustic biodiversity ever recorded in Mediterranean habitats studied so far, despite the recordings (in each site) lasted only one day. Despite this effort, the optimal recording duration for accurate and efficient estimates of coralligenous acoustic diversity - while also minimizing deployment time, battery consumption, data storage and management – remains unclear. In fact, the outcomes of bioacoustics surveys are likely to vary significantly based on species’ calling activity patterns, as already verified in some Mediterranean species (Picciulin et al., 2012; Kéver et al., 2016; Parmentier et al., 2018; Bolgan et al., 2022), and on the timing and duration of recordings (Sugai et al., 2019; Wood et al., 2021). Thus, in the present study, we investigated the fish acoustic community at two coralligenous sites in Sardinia (Western Mediterranean Sea) during the summer 2023 (from mid-June to mid-August). Our objective was to examine the temporal patterns of coralligenous fish acoustics on different temporal scale, identifying peak periods of acoustic activity. Additionally, in the absence of an established optimal acoustic survey design, we tackled this issue by comparing acoustic richness across various recording duration schemes providing valuable insights for optimizing acoustic monitoring of this critical ecosystem, thus enabling a better use of available economic and human resources.
2 Methods
2.1 Acoustic data collection and analysis
Acoustic data collection was conducted in the North-Western coast of Sardinia (Mediterranean Sea; Figure 1) at two sites: Tavolara Island and Santa Teresa Gallura (hereafter TA and ST). TA is located within the Marine Protected Area (MPA) of Tavolara Punta Coda Cavallo, established in 1997 and covering a total area of 15,280 hectares. The acoustic recorder was deployed at 35 m of depth, in the B zone (partial protection), where artisanal fishing is permitted while recreational fishing is prohibited. ST is within the Capo Testa Punta Falcone MPA, established in 2018 and spanning 5,000 hectares. Here, the acoustic recorder was placed within the C zone of the MPA, on a coralligenous cliff at a depth of 34 m, where both professional and recreational fishing are permitted. The site is also within the access corridor to the port, where navigation by ferry boats is allowed.
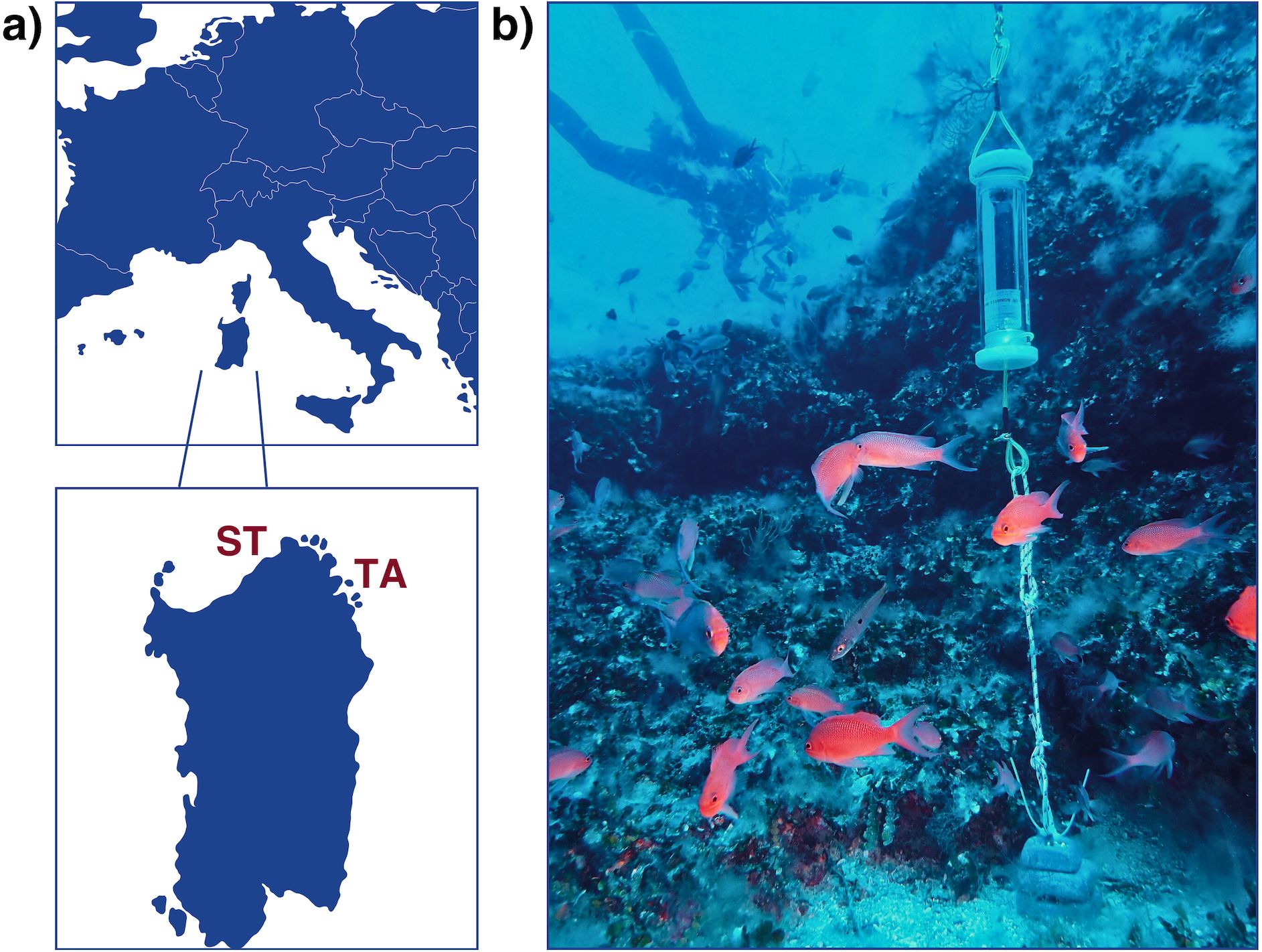
Figure 1. (A) Study sites (ST, Santa Teresa and TA, Tavolara) and (B) Underwater autonomous acoustic recorder.
At both sites, the coralligenous habitat is primarily characterised by coralline algae and to a lesser extent by green algae (e.g., Halimeda tuna and Flabellia petiolata) and brown algae (e.g., Dictyotales spp.). The habitat also includes encrusting and prostrate sponges, as well as Alcyonacea such as the red gorgonian Paramuricea clavata (Pinna et al., 2021).
In each site, an underwater autonomous acoustic recorder (RASP-URec384k-Nauta RCS) was deployed via SCUBA divers. Each RASP was featured with a Sensor Technology SQ26-05 pre-amplified hydrophone (Sensitivity -164 dB re: V/µPa, flat frequency response between 100 Hz and 30 kHz), a DODOTRONIC programmable recorder (gain 0 dB), battery set, and 400 Gb-SD memory card. The recorders were set to record 15 min every hour in the period between mid-June and mid-August, obtaining 6 hours of recordings per day at a sampling rate of 192 kHz (16-bit resolution). To our knowledge, no studies in the Mediterranean Sea have evaluated the effectiveness of different duty cycles for monitoring fish acoustic communities. Accordingly, the choice of the duty cycles was based only on maximizing recording duration within the limits of battery consumption allowed by the recording systems.
Each recording was visually and aurally inspected by Raven Pro 1.6 (Cornell University), displaying the spectrogram 180 s at a time with frequencies between 0 and 8.5 kHz (FFT length = 8,192; Hamming window, 50% overlap), since most fish make sounds below 5 kHz (Amorim, 2006; Popper et al., 2019). Each clearly distinguishable fish sound, identifiable in both the oscillogram and spectrogram, was selected using Raven software. Then, the following acoustic characteristics were measured: sound duration (in sec), number of pulses, pulse rate (the ratio between sound duration and number of pulses), peak-frequencies (the frequency at which the highest sound power occurs, in Hz), centre frequency (the frequency that divides the sound into two frequency intervals of equal energy, in Hz) and bandwidth (the difference between the 5% and 95% frequencies, in Hz). The number of pulses and the pulse rate were manually measured only for sounds consisting of pulse series, while peak frequencies, centre frequency, and bandwidth were automatically measured by Raven for all selected sounds. Fish sounds were classified based on known fish sounds from the Mediterranean Sea (Parmentier et al., 2010; Picciulin et al., 2012; Kéver et al., 2012, 2016; Bertucci et al., 2015; Parmentier et al., 2018; Di Iorio et al., 2018; Bolgan et al., 2019) and a methodological framework initially proposed by Desiderà et al. (2019), which was based on the acoustic characteristics of the sound types and later modified (Table 1). Five different sound types were classified as frequency modulated (sounds characterised by a changing frequency), namely “FM” and “SFM” (series of FM, emitted by unknown species), “DS” (down sweep frequency modulated sounds, emitted by unknown species; Desiderà et al., 2019), “DSS” (series of down sweep frequency modulated sounds, emitted by unknown species; Desiderà et al., 2019), “LDS” (slight-down sweep frequency modulated sound with peak frequency < 200 Hz, associated with Epinephelus marginatus; Bertucci et al., 2015). Seven sound types were composed of irregular pulse series, namely “LPS” (low frequency pulse series, associated with Epinephelus marginatus; Bertucci et al., 2015) and pulse series which were classified based on their acoustic characteristics applying a k-means clustering (MacQueen, 1967). This clustering method is a widely used unsupervised machine learning algorithm for dividing a dataset into k groups, or clusters, where k is a number specified by the analyst: the algorithm assigns objects to different clusters in a way that maximizes similarity within the same cluster (high intra-class similarity) and minimizes similarity between different clusters (low inter-class similarity), based on the Euclidean distance. Each cluster in k-means clustering is represented by its centre, or centroid, which is the mean of the points assigned to that cluster. The optimal number of clusters was estimated by gap statistic (Tibshirani et al., 2001) using the clusGap function from in R (Maechler et al., 2023). Based on the results (see Supplementary Materials, Supplementary Figure S1) six different pulse series were classified: “PS1” (long series of more than 20 pulses with peak frequency around 600 Hz, pulse rate of about 1 sec, emitted by unknown species), “PS2” (series of 5-7 pulses, with peak frequency around 300 Hz and duration shorter than 1 s, emitted by unknown species), “PS3” (series of 3 pulses, with peak frequency around 600-700 Hz and pulse rate of around 0.1 sec, emitted by unknown species), “PS4” (series of 6 pulses, with peak frequency between 600 and 800 Hz, and pulse rate around 1 sec, emitted by unknown species), “PS5” (series of 3-4 pulses with peak frequency around 250 Hz and duration of 0.4 sec, emitted by unknown species), and “PS6” (series of 3-4 pulses, with peak frequency around 600 Hz, longer than 2 sec, emitted by unknown species). Finally, three sound types were composed of regular pulses and have been associated with known species, namely RPS (regular pulse series associated with Sciaena umbra, Bonacito et al., 2001, 2002; Codarin et al., 2012; Picciulin et al., 2012, 2013, 2018, 2020, 2021), APPPS (accelerating pulse series with alternating pulse period associated with Ophidion rochei, Parmentier et al., 2010; Kéver et al., 2012, 2015, 2016; Picciulin et al., 2018), and UFPS (ultra-fast pulses series, associated with Scorpaena spp., Di Iorio et al., 2018; Bolgan et al., 2019). Two sound types were composed of a single pulse, namely “SB” (single boom, associated with Epinephelus marginatus; Bertucci et al., 2015) and “HSB” (a single boom with peak frequency higher than 135 Hz, emitted by unknown species).
2.2 Data analysis
The percentage of occurrence of each fish sound type was calculated as the abundance of the sound type over the total sound types counted daily. Acoustic richness was calculated as the number of different sound type per day of recording, acoustic abundance was calculated as the total number of fish sounds per day of recording, while acoustic composition was expressed by the acoustic abundance of each fish sound type per day of recording.
Acoustic richness and abundance were analysed through a permutational analysis of variance (PERMANOVA) based on Euclidean distance, using Site (two levels: TA and ST) and Period (two levels: early-summer and mid-summer, June 14–July 15 and July 16–August 16, respectively) in a crossed design. The sampling period was divided into early and mid-summer to account for the differing temperature conditions observed during these two periods (La Manna, pers. obs.). A non-metric multidimensional ordination (nMDS) was used to visually represent the similarity of fish acoustic composition between sites across periods. Values were fourth root transformed before calculating the Bray-Curtis similarity. Spatial and temporal differences in the structure of fish acoustic composition were analysed by a PERMANOVA based on Bray-Curtis resemblance matrix of fourth root transformed data, using the same model of univariate analysis. The Pair-wise test was used to discriminate between levels of significant factors.
To capture hourly trend in fish acoustic abundance, the percentage of occurrence of each sound type per hour was calculated and represented in several radar plots, distinguished per site.
To verify if the number of recording days was sufficient to represent the whole acoustic richness of each site, a species accumulation curve was calculated with the “speccacc” function of the Vegan package in R. To investigate the relationship between species richness and recording duration, we compared acoustic richness across different duty cycles by selecting various schemes from the 15 minutes of recording per hour: one minute per hour, five minutes per hour, fifteen minutes per hour, and fifteen minutes per hour exclusively during nighttime (from dusk to dawn). Thus, a Generalized Linear Model (GLM) with a Poisson distribution was applied to evaluate the influence of recording duration (a categorical variable with four levels: 1 min/hour, 5 min/hour, 15/hour min, and 15 min/hour at night) and its interaction with site (a categorical variable with two levels: TA and ST) on acoustic richness. For this analysis only the recordings of August, when the highest sound abundance was found, were used (see Results).
All statistical analyses were carried out in R (R Core Team, 2019) using the packages ‘vegan’ (Oksanen, 2017) and ‘MASS’ (Venables and Ripley, 2002).
3 Results
3.1 General results
Recordings were overall collected for 64 days at TA (from June 14 to August 16, 2023) and 62 days at ST (from June 16 to August 16, 2023), detecting a total of 18,886 and 9,889 fish sounds, respectively. The analysis of recordings has identified 17 distinct sound types (17 at TA and 12 at ST, Figure 2) with a frequency distribution very different between sites and temporal periods. The most frequently recorded sound type was the RPS (the pulse series associated with Sciaena umbra; occurrence percentage of 81% at St and 38% at TA), which was almost continuously present over the entire study period both in TA and ST (Supplementary Figure S2A). The second most common sound, the UFPS (the “/kwa/” sound associated with Scorpaena spp.), was recorded exclusively at ST, with an occurrence percentage of 15%, and an increasing abundance from the end of July up to the mid of August (Supplementary Figure S2B). The sound associated with Ophidion rochei (APPPS) was extremely rare (Figure 2) and was recorded mainly in TA and only in few days of June and August (Supplementary Figure S2C). The sounds related to Epinephelus marginatus (namely, SB, LPS and LDS) were recorded almost exclusively in TA (Figure 2) and during the whole period (Supplementary Figure S2D). The HSB, a single boom like sound, but with highest peak frequency than that described by Bertucci et al. (2015), showed the same daily presence of the SB emitted by Epinephelus marginatus (Supplementary Figure S2D). Following RPS and UFPS, the PS2, PS3, and PS5 sounds had the highest occurrence percentages (Figure 2). PS2 and PS5 were both more abundant in TA and in June-July compared to August (Supplementary Figure S2E). PS1, PS4 and PS6 were quite rare (Figure 2) and were recorded over few distinct days (Supplementary Figure S2E). The frequency modulated sounds (DS, DSS, FM and SFM) were recorded in less than 5% of the recordings at both sites (Figure 2). While DS and DSS were continuously present over the entire study period, FM and SFM were recorded only in few days of August (Supplementary Figure S2F).
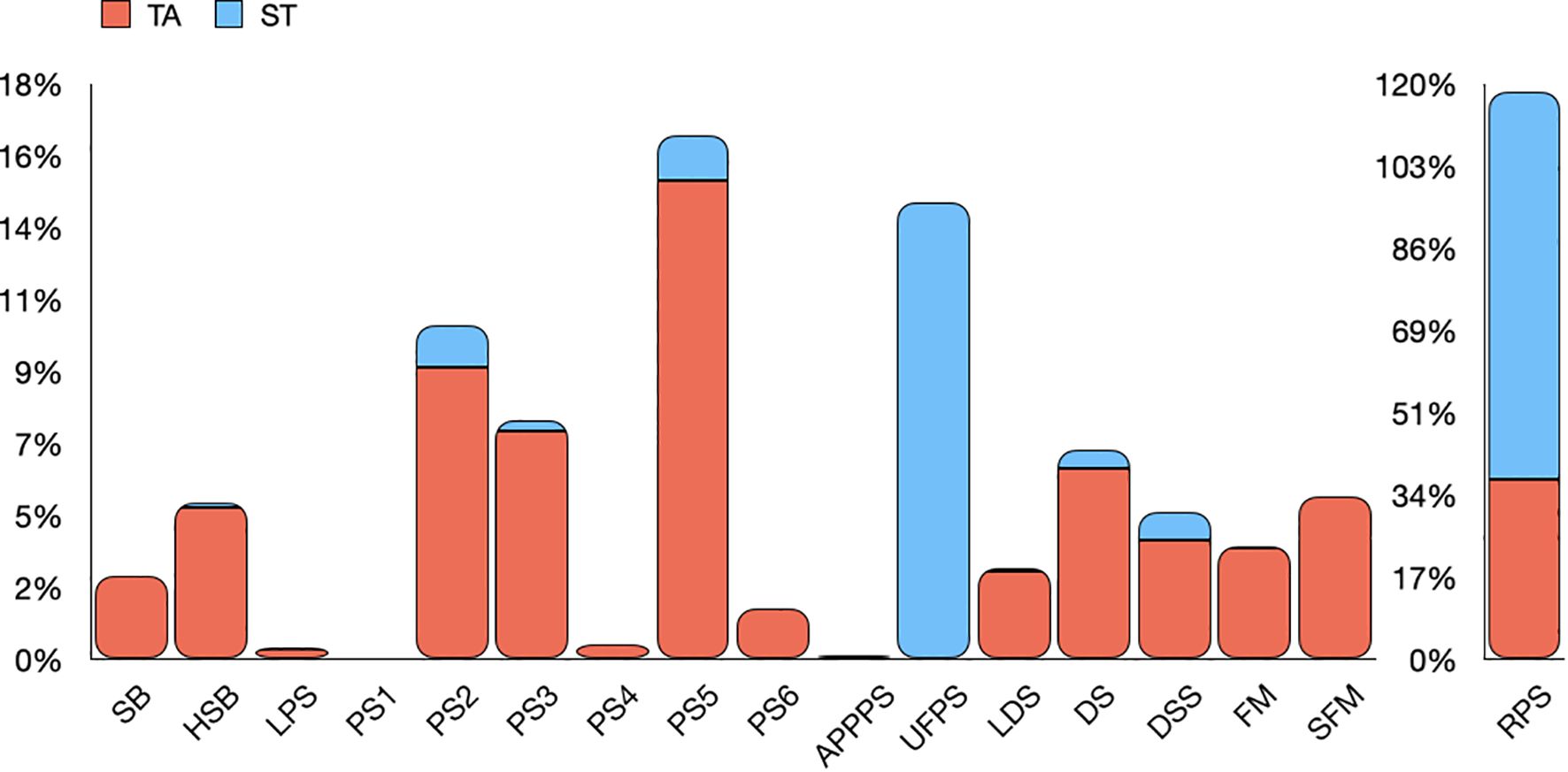
Figure 2. Percentage of occurrence of each identified sound at TA, Tavolara (orange bars) and ST, Santa Teresa (blue bars), calculated as the abundance of the sound type over the total sound types counted daily.
3.2 Spatial and temporal pattern
Acoustic richness was significantly higher at TA compared to ST but showed no significant variation across the periods (Table 2). PERMANOVA and nMDS found significant variations in both fish acoustic abundance (Figure 3) and composition (Figure 4) based on site and period interaction (Table 2). Specifically, post hoc pair wise comparisons on PERMANOVA identified a higher fish acoustic abundance in mid-summer compared to early summer, but only in ST, and that at TA the acoustic abundance was higher than in ST in both periods. Furthermore, pair wise comparisons also indicated that the fish acoustic composition at ST was much more heterogeneous between periods than at TA (Figure 4).
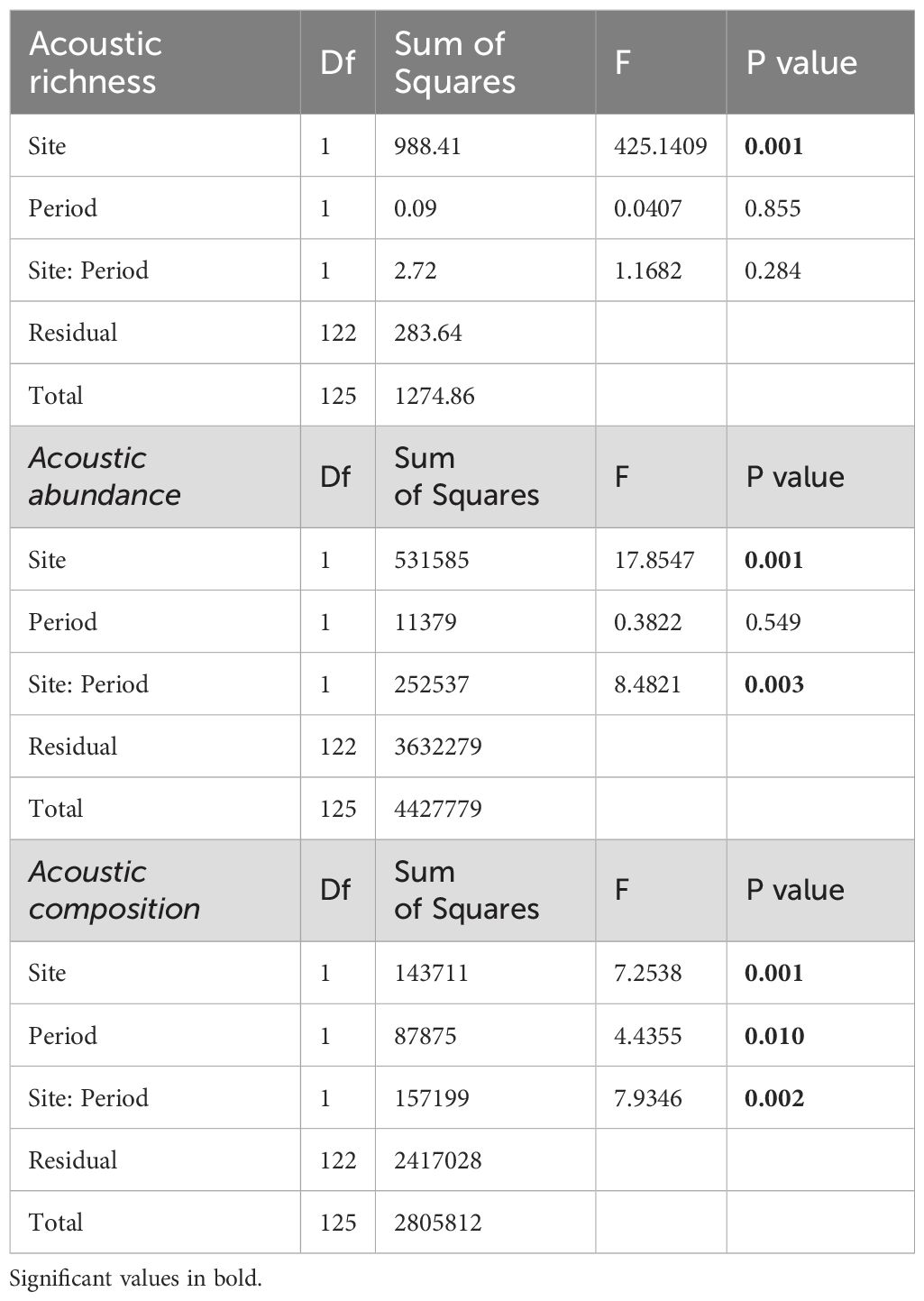
Table 2. PERMANOVA results of the effect of Site (ST and TA) and period (early and mid-summer) on fish acoustic richness, abundance, and fish acoustic composition.
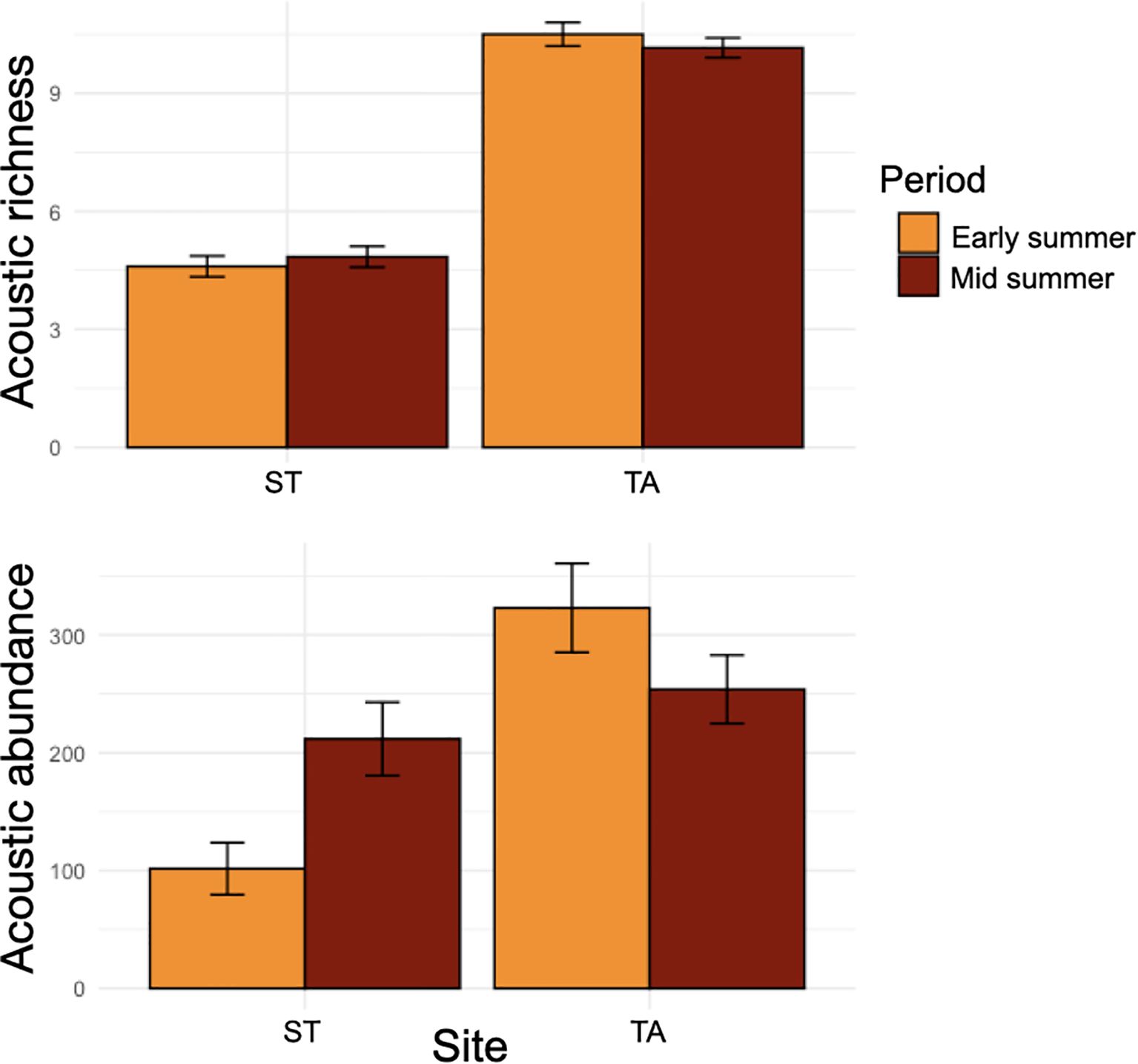
Figure 3. Mean ± se of acoustic richness and acoustic abundance per site and period. Acoustic richness was calculated as the number of different sound type per day of recording; acoustic abundance was calculated as the total number of fish sounds per day of recording.
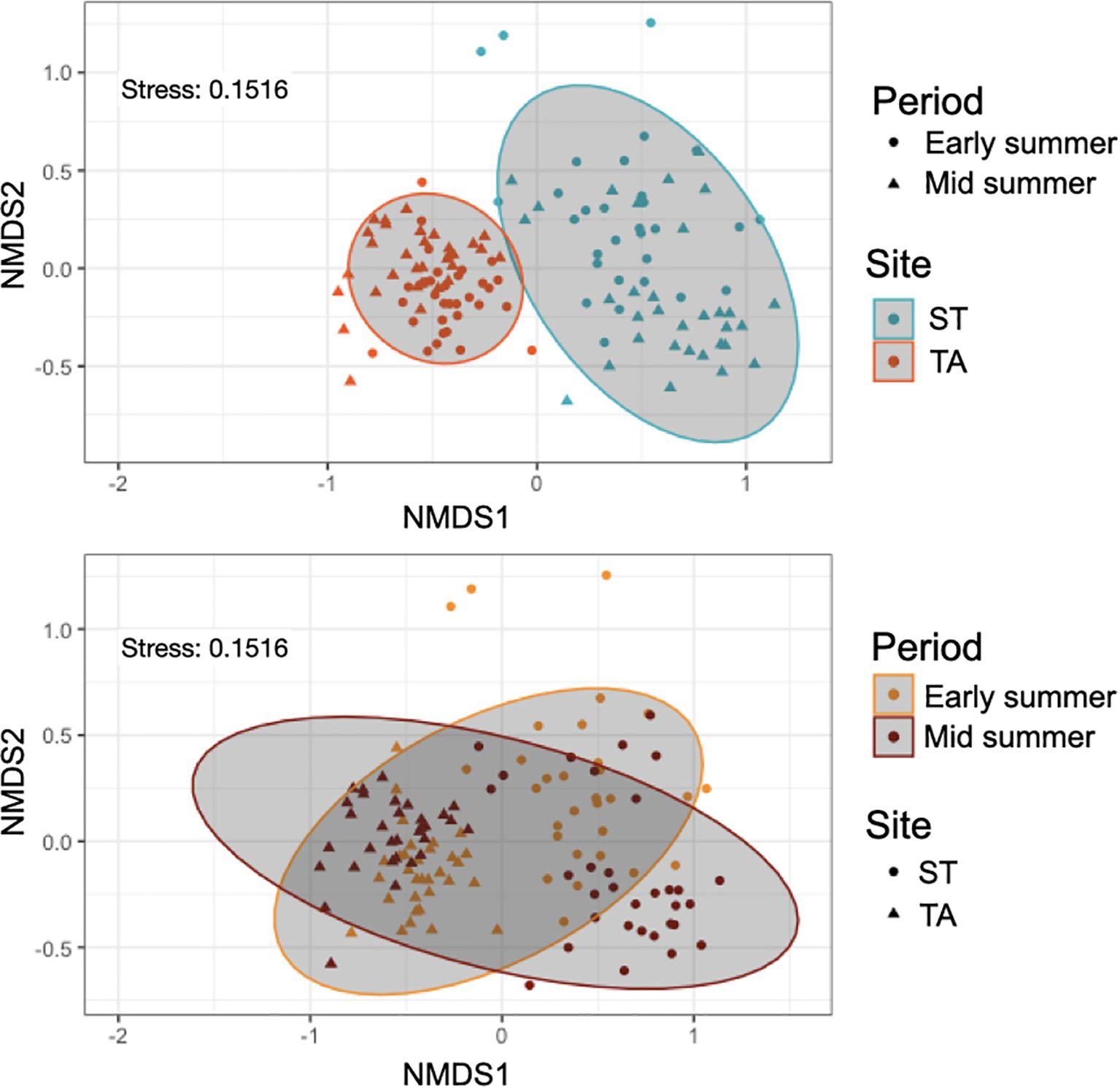
Figure 4. Multidimensional scaling plots showing the similarity of fish acoustic composition between sites across periods. Acoustic composition was expressed by the acoustic abundance of each fish sound type per day of recording.
Sound type occurrence was highly dependent on the hour of the day. Some sounds were exclusively recorded during specific nocturnal (e.g., RPS between 9 and 11 pm, PS2 between 10 and 11 pm, SB and HSB between 10 pm and 7 am) or diurnal hours (e.g., PS3 from 7 am to 8 pm) at both sites. A few sound types were recorded at different hours depending on the site (e.g. DS, Figures 5, 6).
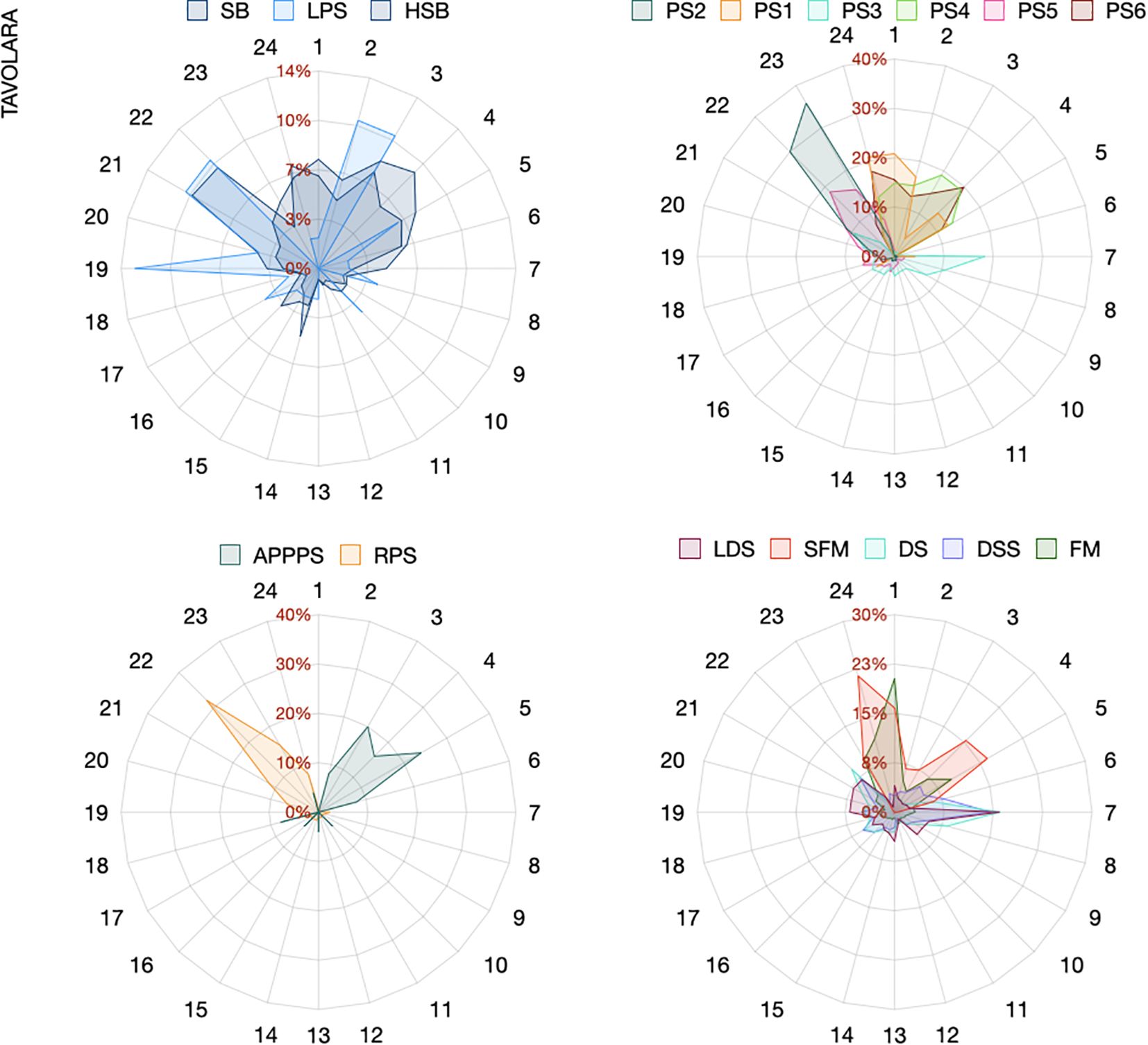
Figure 5. Radar plots showing the hourly occurrence (in percentage) of the main fish sounds (those with percentage of occurrence higher than 3%) recorded at Tavolara.
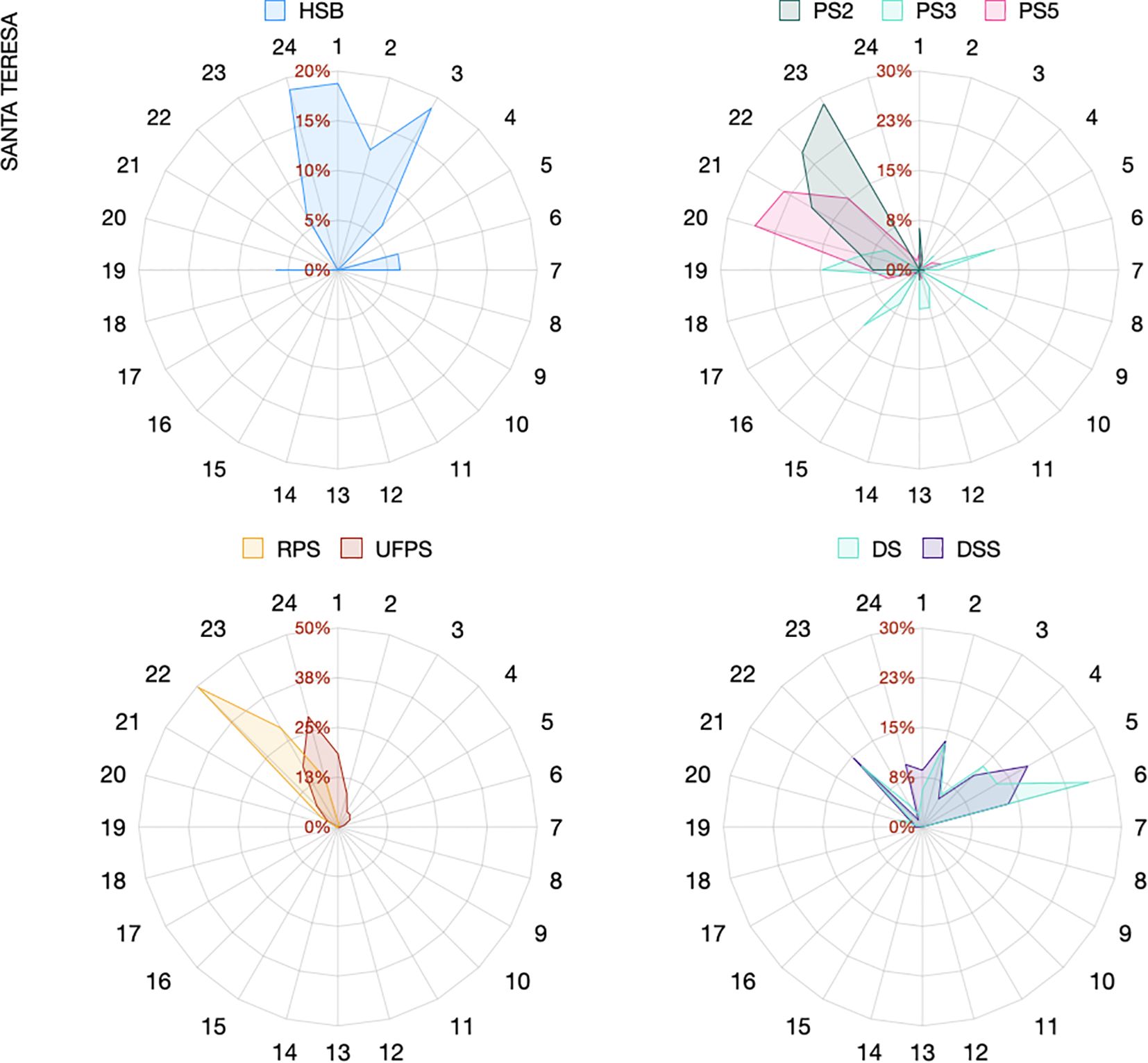
Figure 6. Radar plots showing the hourly occurrence (in percentage) of the main fish sounds (those with percentage of occurrence higher than 3%) recorded at Santa Teresa.
3.3 Optimal recording duration
To assess the adequate number of days of recording for capturing acoustic richness, a species accumulation curve was calculated for each site. At TA, 94% of the total species were identified after 11 days of recording, while at ST, the curve did not plateau, indicating the need for additional recording days to detect less abundant species (Figure 7).
The negative binomial GLM applied on the acoustic richness of August showed that the duration of recording influenced the ability to capture the species richness: namely longer duty cycle (15 min/hour and 15 min/hour at night) had the highest acoustic richness at both sites (Table 3, Figure 8).
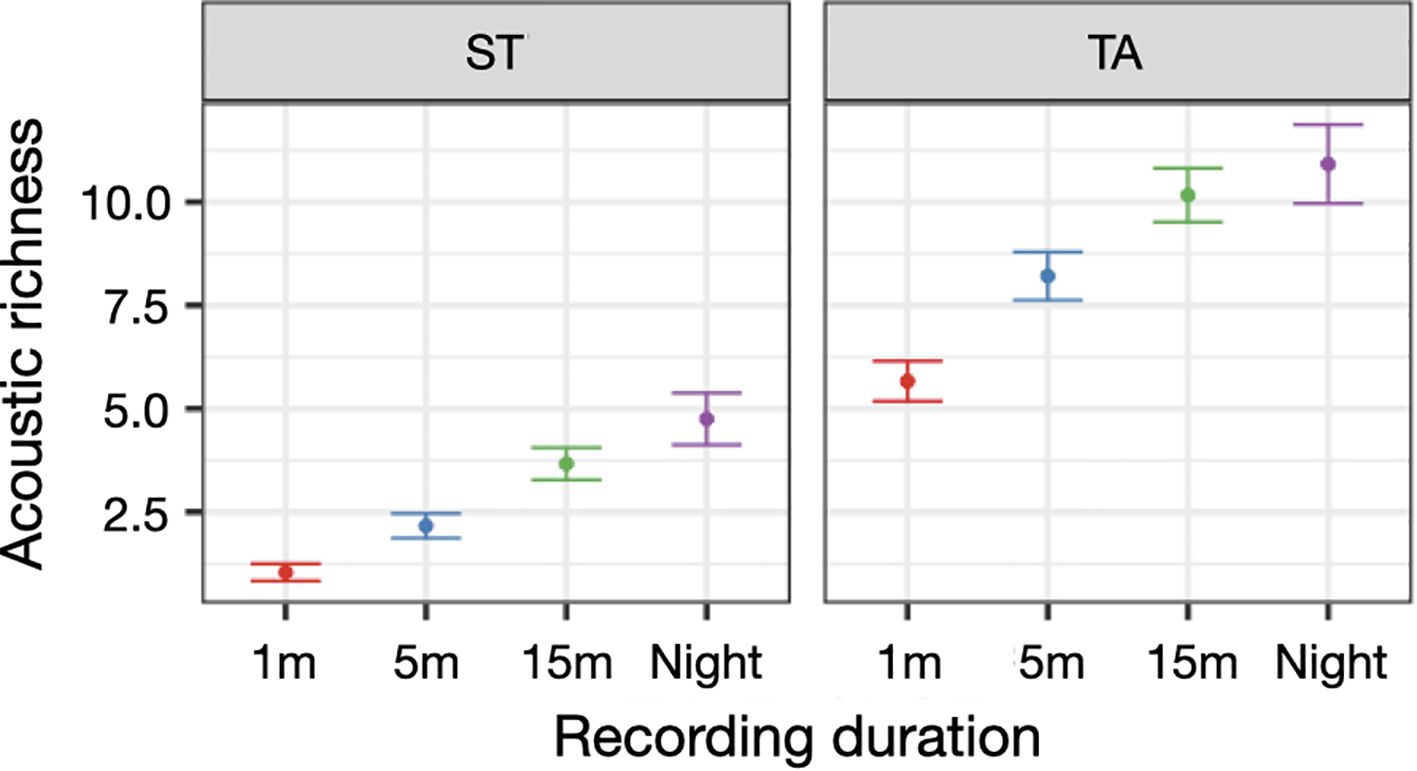
Figure 8. Acoustic richness predicted by the negative binomial GLM per recording duration [1 min/hour (1m), 5 min/hour (5m), 15 min/hour (15m) and 15 min/hour at night (night)] in the two site TA (Tavolara) and ST (Santa Teresa).
4 Discussion
In this study, we investigated the fish acoustic community at two coralligenous sites in Sardinia (Western Mediterranean Sea) over two summer months to examine the patterns of fish acoustics on different temporal scale and to provide practical insights for optimizing fish acoustic monitoring of this critical ecosystem. In fact, understanding the temporal patterns of fish acoustic activity is essential since different species tend to vocalize at specific times of day or night, often in response to activities like feeding, mating, and avoiding predators or to avoid species-specific sounds overlap each other (Krause, 1993; Bertucci et al., 2020). Thus, by analysing these patterns it is possible to detect peaks of acoustic activity, related to specific biological events, and species-interactions within the fish community.
4.1 Spatial and temporal patterns
Seventeen distinct sound types were identified, highlighting a diverse acoustic community within these coralligenous ecosystems. The significant difference in sound detection between the two sites suggests that TA had a more acoustically active or rich fish community during the study period compared to ST, which may be attributed to site-specific environmental factors, habitat structure, species composition or highest level of protection. The number of sound types recorded was lower than those observed in the coralligenous of Corsica and France (Di Iorio et al., 2021) and this discrepancy may be due to differences in regional fish assemblages and/or variations in the classification of fish sounds. In fact, in our study, single-pulse sounds were excluded, and pulse series were statistically classified (based on spectral and temporal acoustic characteristics), potentially accounting for the variation in the number of identified sound types.
The most frequently recorded sound type was the RPS, associated with Sciaena umbra, which was quite more prevalent at ST likely suggesting a difference in the species abundance between the two sites. The UFPS sound, linked to Scorpaena spp., was the second most common sound, and it was exclusively recorded at one site (ST), where it accounted for 15% of the total sounds. In contrast, other sound types such as PS2, PS3, and PS5 were more commonly recorded at the other site (TA). All other sound types were detected with low occurrence (<5%) at both sites. These findings suggest that a few dominant species significantly shape the local soundscapes at ST, where other soniferous species may either be present at very low densities or rely less on acoustic signals for communication (Rice et al., 2017). A similar site-specific variation in acoustic activity has been observed in other Mediterranean coralligenous reefs. Di Iorio et al. (2021) suggested that higher levels of sound production in the coralligenous reefs are associated with more complex habitats, likely due to the enhanced shelter and breeding opportunities habitat heterogeneity provides for fish species. This would suggest that habitat complexity may play a crucial role in driving acoustic activity, as already found in other habitats and ecosystems (Lyon et al., 2019; La Manna et al., 2024b), a hypothesis that warrants further investigation.
Furthermore, while acoustic richness did not vary significantly across periods, acoustic abundance was highest in mid-summer at ST. The nMDS and PERMANOVA analyses confirmed significant differences in fish acoustic community composition between the two sites across the periods: the higher R-value attributed to site (0.051) suggests that spatial differences had a more substantial impact on community composition than variations between periods (R-value=0.031). This could indicate that while the diversity of vocalizing species remained relatively stable, the abundance of acoustic signals increased towards the end of summer, at least in ST, likely due to seasonal peaks in reproductive or territorial behaviours (Staaterman et al., 2014; Parsons et al., 2016; McWilliam et al., 2017; Kaplan et al., 2018) or changes in environmental conditions. These insights are particularly important in the context of climate change and other environmental stressors (tourism, boat traffic, underwater noise), which can alter the natural rhythms of marine ecosystems (Sueur et al., 2019). Therefore, by monitoring these changes, we can detect early signs of ecological disruption and assess the resilience of coralligenous habitats (La Manna et al., 2024a).
The diversity of sound types was also highly dependent on the time of day, with some sounds being specific to nocturnal or diurnal hours. For example, RPS (associated to Sciaena umbra) and APPPS (associated to Ophidion rochei) were mostly recorded between 9 and 11 pm and between 2 am and 6 am, respectively, accordingly with the hourly pattern found for these species in other Mediterranean sites (e.g., Kéver et al., 2016; Parmentier et al., 2018; Desiderà et al., 2019; La Manna et al., 2021; Bolgan et al., 2022). In contrast, few other sound types (e.g. PS3) were more common during daytime hours. This temporal partitioning of acoustic activity likely reflects the daily rhythms of different species, with some being more active or vocal at night and others during the day. Diel periodicity in fish acoustic activity has been documented in various regions (McCauley and Cato, 2000; Luczkovich et al., 2008; Rice et al., 2017; Panicker and Stafford, 2023) including the Mediterranean Sea (e.g., Picciulin et al., 2013; Buscaino et al., 2016; Parmentier et al., 2018; La Manna et al., 2021; Bolgan et al., 2022). In many of these locations, fish calling activity and chorus typically start in the early evening, intensify around sunset, peak a few hours after sunset, and conclude by sunrise. Some other fish instead are more vocal during dawn and dusk, known as crepuscular peaks, which coincide with increased feeding activity and predator-prey interactions (Elise et al., 2019; Bertucci et al., 2020; Carriço et al., 2020). By capturing these daily rhythms, acoustic monitoring can provide a more comprehensive understanding of the ecological dynamics within coralligenous communities. High-resolution temporal data can help identify critical windows of activity (Bolgan et al., 2022), essential for the survival and reproduction of key species, that can be used for designing effective monitoring strategies or temporal restrictions of human activities.
4.2 Influence of recording duration on acoustic richness
Species accumulation curves revealed a wide inconsistency between sites suggesting that at TA a relatively short monitoring period would have been sufficient to capture most of the acoustic diversity, while at ST additional recording days (over 60) would have been necessary to detect possible less abundant or more elusive species. This difference could be either due to lower species abundance or a less uniform distribution of acoustic activity at ST, making it harder to detect all species within a limited timeframe.
Furthermore, longer duty cycle (15 min/hour and 15 min/per hour at night) captured the highest acoustic richness, consistently at both sites, indicating that extended recording periods are more effective in detecting the full range of species acoustic activity. However, it is important to emphasize that these results pertain to the maximum duty cycle investigated (15 minutes) and the maximum number of sampling days permitted by our recording systems. Even if further research is needed to explore different duty cycles and recording durations, our results are consistent with other studies which obtained a significantly increased in the detection of species richness and acoustic events by longer recording periods (Parsons et al., 2016; Wood et al., 2021). While longer recordings offer a more comprehensive view of the acoustic environment, increasing the chances of detecting rare or sporadic biological sounds, they also introduce significant challenges: extended recording times can quickly consume battery power, particularly in remote or underwater settings where regular maintenance is difficult. Moreover, longer recordings produce large volumes of data, which can be challenging to store, manage, and be analysed. These findings highlight the crucial need to balance extended recording durations with practical limitations, such as battery life and data processing demands. This underscores the importance of carefully planning sampling strategies to optimize the effectiveness of acoustic monitoring without overconsuming logistical resources. Achieving this balance is key to ensuring an efficient and sustainable use of passive acoustic monitoring in ecological research (Risch et al., 2014; Gibb et al., 2019; Sugai et al., 2019).
5 Conclusions
Given the recent decline in coralligenous reef biodiversity - particularly due to sea warming and thermal anomalies - it is essential to develop cost-effective monitoring methods that extend beyond the typical visual assessment of macroalgae and invertebrates. While passive acoustic monitoring has been used for decades for research and monitoring of tropical coral reefs, its application to Mediterranean coralligenous reefs has only recently been explored (Di Iorio et al., 2021; La Manna et al., 2024a). The present study improved the understanding of the temporal and spatial variability of fish acoustic communities in this critical ecosystem, even if further research is needed to investigate the factors driving site-specific differences and to identify which species produce most of the detected sound types.
The current findings highlight the significance of site-specific context in determining acoustic diversity and suggest that tailored monitoring strategies may be required to capture the full spectrum of acoustic activity at different sites. Adjusting monitoring efforts to align with peak periods of acoustic activity can improve both the efficiency and accuracy of data collection. Additionally, extended recording durations can provide a more comprehensive view of species richness, although balancing recording time with resource constraints (e.g., battery life and data management) remains a key consideration. The insights from this study can guide future monitoring efforts, deepen our understanding of these complex ecosystems, also to support the conservation of their rich biodiversity.
Data availability statement
The raw data supporting the conclusions of this article will be made available by the authors, without undue reservation.
Ethics statement
Ethical approval was not required for the study involving animals in accordance with the local legislation and institutional requirements because the study just involves passive acoustic recordings of freely swimming animals. No manipulative or laboratories experiments were conducted.
Author contributions
GLM: Writing – original draft, Supervision, Methodology, Investigation, Formal analysis, Data curation, Conceptualization. MM: Writing – original draft, Methodology, Formal analysis. RV: Writing – original draft, Methodology, Formal analysis. GM: Writing – original draft, Formal analysis. GS: Writing – original draft, Supervision, Funding acquisition. GC: Writing – original draft, Supervision, Investigation, Funding acquisition, Conceptualization.
Funding
The author(s) declare financial support was received for the research, authorship, and/or publication of this article. Support was received from the National Biodiversity Future Center funded by the Italian Ministry of University and Research, PNRR, Missione 4, Componente 2, “Dalla ricerca all’impresa”, Investimento 1.4 Project CN00000033.
Acknowledgments
GLM, GC, GM, and GS acknowledge the support of the National Biodiversity Future Center funded by the Italian Ministry of University and Research, PNRR, Missione 4, Componente 2, “Dalla ricerca all’impresa”, Investimento 1.4 Project CN00000033. This manuscript reflects only the authors’ views and opinions, neither the European Union nor the European Commission can be considered responsible for them. Special thanks to Patrizia Stipcich and Luigi Piazzi for their help with the fieldwork, and to Lauren Polimeno for fine-tuning the English.
Conflict of interest
The authors declare that the research was conducted in the absence of any commercial or financial relationships that could be construed as a potential conflict of interest.
Publisher’s note
All claims expressed in this article are solely those of the authors and do not necessarily represent those of their affiliated organizations, or those of the publisher, the editors and the reviewers. Any product that may be evaluated in this article, or claim that may be made by its manufacturer, is not guaranteed or endorsed by the publisher.
Supplementary material
The Supplementary Material for this article can be found online at: https://www.frontiersin.org/articles/10.3389/fmars.2024.1483661/full#supplementary-material
References
Balata D., Piazzi L., Cecchi E., Cinelli F. (2005). Variability of Mediterranean coralligenous assemblages subject to local variation in sediment deposition. Mar. Environ. Res. 60, 403–421. doi: 10.1016/j.marenvres.2004.12.005
Ballesteros E. (2006). Mediterranean coralligenous assemblages: A synthesis of present knowledge. Oceanogr. Mar. Biol. 44, 123–195.
Bavestrello G., Cerrano C., Zanzi D., Cattaneo Vietti R. (1997). Damage by fishing activities in the gorgonian coral Paramuricea clavata in the Ligurian Sea. Aquat. Conserv.: Mar. Freshw. Ecosyst. 7, 253–262.
Bertucci F., Lejeune P., Payrot J., Parmentier E. (2015). Sound production by dusky grouper Epinephelus marginatus at spawning aggregation sites. J. Fish Biol. 87, 400–421. doi: 10.1111/jfb.2015.87.issue-2
Bertucci F., Maratrat K., Berthe C., Besson M., Guerra A. S., Raick X., et al. (2020). Local sonic activity reveals potential partitioning in a coral reef fish community. Oecologia 193, 125–134. doi: 10.1007/s00442-020-04647-3
Bolgan M., Di Iorio L., Dailianis T., Catalan I. A., Lejeune P., Picciulin M., et al. (2022). Fish acoustic community structure in Neptune seagrass meadows across the Mediterranean basin. Aquat. Conserv.: Mar. Freshw. Ecosyst. 32, 329–347.
Bolgan M., Gervaise C., Di Iorio L., Lossent J., Lejeune P., Raick X., et al. (2020). Fish biophony in a Mediterranean submarine canyon. J. Acoust. Soc. America 147, 2466–2477. doi: 10.1121/10.0001101
Bolgan M., Soularde J., Di Iorio L., Gervaise C., Lejeune P., Gobert S., et al. (2019). Sea chordophones make the mysterious/Kwa/sound: identification of the emitter of the dominant fish sound in Mediterranean seagrass meadows. J. Exp. Biol. 222, 1. doi: 10.1242/jeb.196931
Bonacito C., Costantini M., Picciulin M., Ferrero E. A., Hawkins A. D. (2001). “Acoustical and temporal features of Sciaena umbra (Sciaenidae) in the Miramare marine reserve (Gulf of Trieste, Italy),” in Proceeding of the XVIII IBAC, International Bioacoustics Council Meeting, Cogne.
Bonacito C., Costantini M., Picciulin M., Ferrero E. A., Hawkins A. D. (2002). Passive hydrophone census of Sciaena umbra (Sciaenidae) in the Gulf of Trieste (Northern Adriatic sea, Italy). Bioacoustics 12, 292–294. doi: 10.1080/09524622.2002.9753726
Buscaino G., Ceraulo M., Pieretti N., Corrias V., Farina A., Filiciotto F., et al. (2016). Temporal patterns in the soundscape of the shallow waters of a Mediterranean marine protected area. Sci. Rep. 6, 34230. doi: 10.1038/srep34230
Carriço R., Silva M. A., Menezes G., Vieira M., Bolgan M., Fonseca P. J., et al. (2020). Temporal dynamics in diversity patterns of fish sound production in the Condor seamount (Azores, NE Atlantic). Deep-Sea Res. Part I: Oceanographic Research Papers. 164, 103357. doi: 10.1016/j.dsr.2020.103357
Codarin A., Picciulin M., Sebastianutto L., Calcagno G., Spoto M., Ferrero E. A. (2012). “Nocturnal acoustic activity in the shallow waters of WWF-Miramare Natural Marine Reserve (Trieste, Italy),” in In Effects of Noise on Aquatic Life, Advances in Experimental Medicine and Biology, vol. 730 . Eds. Popper A. N., Hawkins A. D. (Springer Science + Business Media, LLC, New York), 161–164.
Desiderà E., Guidetti P., Panzalis P., Navone A., Valentini-Poirrier C. A., Boissery P., et al. (2019). Acoustic fish communities: sound diversity of rocky habitats reflects fish species diversity. Mar. Ecol. Prog. Ser. 608, 183–197. doi: 10.3354/meps12812
Di Camillo C. G., Ponti M., Storari A., Scarpa C., Roveta C., Pulido Mantas T., et al. (2023). Review of the indexes to assess the ecological quality of coralligenous reefs: towards a unified approach. Front. Mar. Sci. 10, 1252969. doi: 10.3389/fmars.2023.1252969
Di Iorio L., Audax M., Deter J., et al. (2021). Biogeography of acoustic biodiversity of NW Mediterranean coralligenous reefs. Sci. Rep. 11, 16991. doi: 10.1038/s41598-021-96378-5
Di Iorio L., Bonhomme P., Michez N., Ferrari B., Gigou A., Panzalis P., et al. (2020). Spatio-temporal surveys of the brown meagre Sciaena umbra using passive acoustics for management and conservation. BioRxiv. doi: 10.1101/2020.06.03.131326
Di Iorio L., Raick X., Parmentier E., Boissery P., Valentini Poirier C. A., Gervaise C. (2018). Posidonia meadows calling: a ubiquitous fish sound with monitoring potential. Remote Sens. Ecol. Conserv. 4, 248–263. doi: 10.1002/rse2.2018.4.issue-3
Elise S., Bailly A., Urbina-Barreto I., Mou-Tham G., Chiroleu F., Vigliola L., et al. (2019). An optimised passive acoustic sampling scheme to discriminate among coral reefs’ ecological states. Ecol. Indic. 107, 105627. doi: 10.1016/j.ecolind.2019.105627
Garrabou J., Coma R., Bensoussan N., Bally M., Chevaldonné P., Cigliano M., et al. (2009). Mass mortality in Northwestern Mediterranean rocky benthic communities: effects of the 2003 heat wave. Glob. Change Biol. 15, 1090–1103. doi: 10.1111/j.1365-2486.2008.01823.x
Garrabou J., Gómez-Gras D., Medrano A., Cerrano C., Ponti M., Schlegel R., et al. (2022). Marine heatwaves drive recurrent mass mortalities in the Mediterranean Sea. Global Change Biol. 28, 5708–5725. doi: 10.1111/gcb.v28.19
Gibb R., Browning E., Glover-Kapfer P., Jones K. E. (2019). Emerging opportunities and challenges for passive acoustics in ecological assessment and monitoring. Methods Ecol. Evol. 10, 169–185. doi: 10.1111/mee3.2019.10.issue-2
Hinz H. (2017). “Impact of bottom fishing on animal forests: Science, conservation, and fisheries management,” in Marine animal forests: the ecology of benthic biodiversity hotspots. Eds. Rossi S., Bramanti L., Gori A., Orejas C. (Springer International Publishing, Cham, Switzerland), p 1041–p 1059.
Jarriel S. D., Formel N., Ferguson S. R., Jensen F. H., Apprill A., Mooney T. A. (2024). Unidentified fish sounds as indicators of coral reef health and comparison to other acoustic methods. Front. Remote Sens. 5, 1338586. doi: 10.3389/frsen.2024.1338586
Kaplan M., Mooney T., Partan J., Solow A. (2015). Coral reef species assemblages are associated with ambient soundscapes. Mar. Ecol. Prog. Ser. 533, 93–107. doi: 10.3354/meps11382
Kaplan M. B., Lammers M. O., Zang E., Aran Mooney T. (2018). Acoustic and biological trends on coral reefs off Maui, Hawaii. Coral Reefs 37, 121–133. doi: 10.1007/s00338-017-1638-x
Kéver L., Boyle K. S., Dragičević B., Dulčić J., Casadevall M., Parmentier E. (2012). Sexual dimorphism of sonic apparatus and extreme intersexual variation of sounds in Ophidion rochei (Ophidiidae): first evidence of a tight relationship between morphology and sound characteristics in Ophidiidae. Front. Zool. 9, 1–16. doi: 10.1186/1742-9994-9-34
Kéver L., Boyle K. S., Parmentier E. (2015). Effects of seawater temperature on sound characteristics in Ophidion rochei (Ophidiidae). J. Fish Biol. 87, 502–509. doi: 10.1111/jfb.2015.87.issue-2
Kéver L., Lejeune P., Michel L. N., Parmentier E. (2016). Passive acoustic recording of Ophidion rochei calling activity in Calvi Bay (France). Mar. Ecol. 37, 1315–1324. doi: 10.1111/maec.2016.37.issue-6
Krause B., Farina A. (2016). Using ecoacoustic methods to survey the impacts of climate change on biodiversity. Biol. Conserv. 195, 245–254. doi: 10.1016/j.biocon.2016.01.013
La Manna G., Guala I., Pansini A., Stipcich P., Arrostuto N., Ceccherelli. G. (2024b). Soundscape analysis can be an effective tool in assessing seagrass restoration early success. Sci. Rep. 14, 20910. doi: 10.1038/s41598-024-71975-2
La Manna G., Moro Merella M., Vargiu R., Morello G., Sarà G., Ceccherelli G. (2024a). “Applications of acoustic monitoring for assessing marine biodiversity and human impact,” in National Biodiversity Future Center Forum, Palermo, 20-22 May 2024.
La Manna G., Picciulin M., Crobu A., Perretti F., Ronchetti F., Manghi M., et al. (2021). Marine soundscape and fish biophony of a Mediterranean marine protected area. PeerJ, 9e12551. doi: 10.7717/peerj.12551
La Rivière M., Michez N., Delavenne J., Andres S., Fréjefond C., Janson A-L., et al (2021). Fiches descriptives des biocénoses benthiques de Méditerranée. Paris: UMS PatriNat (OFB-CNRS-MNHN), 660.
Lin T.-H., Akamatsu T., Sinniger F., Harii S. (2021). Exploring coral reef biodiversity via underwater soundscapes. Biol. Conserv. 253, 108901. doi: 10.1016/j.biocon.2020.108901
Luczkovich J. J., Mann D. A., Rountree R. A. (2008). Passive acoustic monitoring of spawning fish populations. Bioacoustics 17, 178–181.
Lyon R. P., Eggleston D. B., Bohnenstiehl D. R., Layman C. A., Ricci S. W., Allgeier J. E. (2019). Fish community structure, habitat complexity, and soundscape characteristics of patch reefs in a tropical, back-reef system. Mar. Ecol. Prog. Ser. 609, 33–48. doi: 10.3354/meps12829
MacQueen J. B. (1967). “Some methods for classification and analysis of multivariate observations,” in Proceedings of the fifth Berkeley symposium on mathematical statistics and probability, vol. Vol. 1 . Eds. Le Cam L. M., Neyman J. (University of California Press, California), 281–297.
Maechler M., Rousseeuw P., Struyf A., Hubert M., Hornik K. (2023). cluster: Cluster Analysis Basics and Extensions. R package version 2.1.6.
Martin C. S., Giannoulaki M., De Leo F., Scardi M., Salomidi M., et al. (2014). Coralligenous and maërl habitats: Predictive modelling to identify their spatial distributions across the Mediterranean Sea. Sci. Rep. 4, 5073. doi: 10.1038/srep05073
Mateos-Molina D., Palma M., Ruiz-Valentin I., Panagos P., Garcia-Charton J. A., Ponti M. (2015). Assessing consequences of land cover changes on sediment deliveries to coastal waters at regional level over the last two decades in the northwestern Mediterranean Sea. Ocean Coast. Manage. 116, 435–442. doi: 10.1016/j.ocecoaman.2015.09.003
McCauley R. D., Cato D. H. (2000). Patterns of fish calling in a nearshore environment in the Great Barrier Reef. Philos. Trans. R. Soc. B: Biol. Sci. 355, 1289–1293. doi: 10.1098/rstb.2000.0686
McWilliam J. N., McCauley R. D., Erbe C., Parsons M. J. G. (2017). Patterns of biophonic periodicity on coral reefs in the Great Barrier Reef. Sci. Rep. 7, 17549. doi: 10.1038/s41598-017-15838-z
Mooney T. A., et al. (2020). Listening forward: Approaching marine biodiversity assessments using acoustic methods. R. Soc Open Sci. 7, 2020. doi: 10.1098/rsos.201287
Mullet T. C., Farina A., Morton J. M., Wilhelm S. R. (2024). Seasonal sonic patterns reveal phenological phases (sonophases) associated with climate change in subarctic Alaska. Front. Ecol. Evol. 12, 1345558. doi: 10.3389/fevo.2024.1345558
Oksanen F. J. (2017).Vegan: community ecology package. R package Version 2.4-3. Available online at: https://CRAN.R-project.org/package=vegan.
Pandolfi J. M., Connolly S. R., Marshall D. J., Cohen A. L. (2011). Projecting coral reef futures under global warming and ocean acidification. Far-field Optical Nanoscopy Sci. 333, 418–422. doi: 10.1126/science.1204794
Panicker D., Stafford K. M. (2023). Temporal variability of a soundscape near a mid-oceanic atoll in the northern Indian ocean. Prog. Oceanogr. 214, 103033. doi: 10.1016/j.pocean.2023.103033
Parmentier E., Bouillac G., Dragicevic B., Dulcic J., Fine M. L., Dragičević B., et al. (2010). Call properties and morphology of the sound-producing organ in Ophidion rochei (Ophidiidae). J. Exp. Biol. 213, 3230–3236. doi: 10.1242/jeb.044701
Parmentier E., Di Iorio L., Picciulin M., Malavasi S., Lagardère J. P., Bertucci F. (2018). Consistency of spatiotemporal sound features supports the use of passive acoustics for longterm monitoring. Anim. Conserv. 21, 211–220. doi: 10.1111/acv.2018.21.issue-3
Parsons M. J., Salgado-Kent C. P., Marley S. A., Gavrilov A. N., McCauley R. D. (2016). Characterizing diversity and variation in fish choruses in Darwin Harbour. ICES J. Mar. Sci. 73, 2058–2074. doi: 10.1093/icesjms/fsw037
Piazzi L., Atzori F., Cadoni N., Cinti M. F., Frau F., Ceccherelli G. (2018). Benthic mucilage blooms threaten coralligenous reefs. Mar. Environ. Res. 140, 145–151. doi: 10.1016/j.marenvres.2018.06.011
Piazzi L., Gennaro P., Balata D. (2012). Threats to macroalgal coralligenous assemblages in the Mediterranean Sea. Mar. pollut. Bull. 64, 2623–2629. doi: 10.1016/j.marpolbul.2012.07.027
Picciulin M., Bolgan M., Codarin A., Fiorin R., Zucchetta M., Malavasi S. (2013). Passive acoustic monitoring of Sciaena umbra on rocky habitats in the Venetian littoral zone. Fishery Res. 145, 76–81. doi: 10.1016/j.fishres.2013.02.008
Picciulin M., Bolgan M., Facca C., Fiorin R., Riccato F., Malavasi S. (2021). Sound discrimination of two sympatric, threatened fish species allows for their in situ mapping. Aquat. Conserv.: Mar. Freshw. Ecosyst. 31, 2103–2118. doi: 10.1002/aqc.3581
Picciulin M., Calcagno G., Sebastianutto L., Bonacito C., Codarin A., Costantini M., et al. (2012). Diagnostics of nocturnal calls of Sciaena umbra (L., fam., Sciaenidae) in a nearshore Mediterranean marine reserve. Bioacoustics 22, 109–120. doi: 10.1080/09524622.2012.727277
Picciulin M., Fiorin R., Facca C., Malavasi S. (2020). Sound features and acoustic rhythms as a proxy for locating the spawning ground of Sciaena umbra in the wild. Aquat. Conserv.: Mar. Freshw. Ecosyst. 30, 1299–1312. doi: 10.1002/aqc.3340
Picciulin M., Kéver L., Parmentier E., Bolgan M. (2018). Listening to the unseen: passive acoustic monitoring reveals the presence of a cryptic fish species. Aquat. Conserv.: Mar. Freshw. Ecosyst. 29, 202–210. doi: 10.1002/aqc.2973
Picciulin M., Rako-Gospic N., Petrizzo A., Rudulovic M., Falkner R. (2022). A fish and dolphin biophony in the boat noise-dominated soundscape of the Cres-Lošinj Archipelago (Croatia). J. Mar. Sci. Eng. 10, 300. doi: 10.3390/jmse10020300
Pinna F., Piazzi L., Cinti M. F., Pansini A., Stipcich P., Ceccherelli G. (2021). Vertical variation of coralligenous cliff assemblages in marine biogeographic areas. Estuar. Coast. Shelf Sci. 261, 107554. doi: 10.1016/j.ecss.2021.107554
Ponti M., Parlini R. A., Ventra V., Greco D., Abbiati M., Cereno C. (2014). Ecological shifts in Mediterranean coralligenous assemblages related to gorgonian forest loss. PloS One 9, e102782. doi: 10.1371/journal.pone.0102782
Popper A. N., Hawkins A. D., Sand O., Sisneros J. A. (2019). Examining the hearing abilities of fishes. J. Acoust. Soc. America 146, 948. doi: 10.1121/1.5120185
R Core Team. (2019). R: a language and environment for statistical computing. Vienna, Austria: R Foundation for Statistical Computing. Available at: http://www.R-project.org/.
Rice A., Soldevilla M. S., Quinnlan J. A. (2017). Nocturnal patterns in fish chorusing off the coasts of Georgia and eastern Florida. Bull. Mar. Sci. 93, 455–474. doi: 10.5343/bms.2016.1043
Risch D., Castellote M., Clark C. W., Davis G. E., Dugan P. J., Hodge L. E., et al. (2014). Seasonal migrations of North Atlantic minke whales: Novel insights from large-scale passive acoustic monitoring networks. Mov. Ecol. 2, 1–17. doi: 10.1186/s40462-014-0024-3
Staaterman E., Paris C. B., DeFerrari H. A., Mann D. A., Rice A. N., D’Alessandro E. K. (2014). Celestial patterns in marine soundscapes. Mar. Ecol. Prog. Ser. 508, 17–32. doi: 10.3354/meps10911
Sueur J., Aubin T., Simonis C. (2008). Seewave: a free modular tool for sound analysis and synthesis. Bioacoustics 18, 213–226.
Sueur J., Farina A. (2015). Ecoacoustics: The ecological investigation and interpretation of environmental sound. Biosemiotics 8, 493–502. doi: 10.1007/s12304-015-9248-x
Sueur J., Krause B., Farina A. (2019). Climate change is breaking Earth’beat. Trends Ecol. Evol. 34, 971–973. doi: 10.1016/j.tree.2019.07.014
Sugai L. S. M., Silva T. S. F., Ribeiro J. W., Llusia D. (2019). Terrestrial passive acoustic monitoring: Review and perspectives. BioScience 69, 15–25. doi: 10.1093/biosci/biy147
Teixidó N., Casas E., Cebrián E., Linares C., Garrabou J. (2013). Impacts on coralligenous outcrop biodiversity of a dramatic coastal storm. PloS One 8, e53742. doi: 10.1371/journal.pone.0053742
Tibshirani R., Walther G., Hastie T. (2001). Estimating the number of clusters in a data set via the gap statistic. J. R. Stat. Soc. Series B 63, 411–423. doi: 10.1111/1467-9868.00293
Wood C. M., Kahl S., Chaon P., Peery M. Z., Klinck H. (2021). Survey coverage, recording duration and community composition affect observed species richness in passive acoustic surveys. Methods Ecol. Evol. 12, 885–896. doi: 10.1111/2041-210X.13571
Keywords: biodiversity, cryptic species, fish acoustic community, fish sounds, monitoring strategies, passive acoustic monitoring
Citation: La Manna G, Moro Merella M, Vargiu R, Morello G, Sarà G and Ceccherelli G (2024) Spatio-temporal patterns of fish acoustic communities in Western Mediterranean coralligenous reefs: optimizing monitoring through recording duration. Front. Mar. Sci. 11:1483661. doi: 10.3389/fmars.2024.1483661
Received: 20 August 2024; Accepted: 31 October 2024;
Published: 20 November 2024.
Edited by:
David Alberto Salas Salas De León, National Autonomous University of Mexico, MexicoReviewed by:
Alejandro Ramos Amezquita, UNAM, MexicoMarta Bolgan, Ocean Science Consulting Ltd., United Kingdom
Copyright © 2024 La Manna, Moro Merella, Vargiu, Morello, Sarà and Ceccherelli. This is an open-access article distributed under the terms of the Creative Commons Attribution License (CC BY). The use, distribution or reproduction in other forums is permitted, provided the original author(s) and the copyright owner(s) are credited and that the original publication in this journal is cited, in accordance with accepted academic practice. No use, distribution or reproduction is permitted which does not comply with these terms.
*Correspondence: Gabriella La Manna, Z2xhbWFubmFAdW5pc3MuaXQ=