- 1Key Laboratory of Microecological Resources and Utilization in Breeding Industry, Ministry of Agriculture and Rural Affairs, Haid Central Research Institute, Guangdong Haid Group Co., Ltd., Guangzhou, Guangdong, China
- 2National Aquafeed Safety Assessment Centre, Institute of Feed Research, Chinese Academy of Agricultural Sciences, Beijing, China
We investigated the effects of dietary supplementation with sodium propionate (SP) and tributyrin (TB) on hepatic lipid deposition and antioxidant capacity of spotted seabass (Lateolabrax maculatus) via an 8-week feeding experiment and a hypoxia stress experiment. The fish were fed five experimental diets: a control diet (CON), a diet supplemented with 2 g/kg SP (SP-0.2%), 4 g/kg SP (SP-0.4%), 2 g/kg TB (TB-0.2%), or 4 g/kg TB (TB-0.4%). No significant difference in growth performance was presented among the groups (P > 0.05). The SP-0.4% and TB-0.2% groups presented significantly lower hepatosomatic and viscerasomatic indexes compared with the CON group. Then, the SP-0.4% and TB-0.2% groups presented stronger resistance to hypoxic stress than the other groups and were analyzed further. The hepatic histology and triglyceride levels revealed that SP-0.4% and TB-0.2% reduced hepatic lipid deposition. Similarly, the downregulation of malondialdehyde and the upregulation of total antioxidant capacity, superoxide dismutase, and catalase activities and the related gene expression levels revealed that SP-0.4% and TB-0.2% improved the antioxidant capacity. Additionally, the RNA sequencing demonstrated that SP-0.4% and TB-0.2% regulated gene expression to a similar extent. Among the 117 differentially expressed genes, 67 genes were enriched in the same pattern, and involved the FoxO signaling, PI3K-Akt signaling, and insulin-related pathways. In conclusion, supplementing SP-0.4% and TB-0.2% as feed additives effectively improved hepatic lipid metabolism, antioxidant capacity, and hypoxic stress resistance of spotted seabass.
1 Introduction
With the rapid development of the global aquaculture industry, the occurrence of fish diseases is increasing, which has become an important factor affecting the sustainable and healthy development of aquaculture (Abdelsalam et al., 2023). Antibiotic misuse has led to the emergence of drug-resistant pathogens and drug residues, which seriously affects sustainable aquaculture development (Okocha et al., 2018; Shao et al., 2021). Therefore, efficient, green, and safe alternative antibiotic additives are increasingly attracting research attention.
Nutritional modulation of the immune system is a potentially powerful tool for improving fish health, and includes amino acids, fatty acids, vitamins, and minerals (Oliva-Teles, 2012; Pohlenz and Gatlin, 2014). Given their wide range of physiological functions and high safety, short-chain fatty acids (SCFAs) have important application potential for improving fish health, and have been identified as a potential alternative to traditional antibiotic treatments (Hoseinifar et al., 2017; Ng and Koh, 2017; Palma et al., 2023). SCFAs generally refer to organic acids with a carbon atomic number < 6, which are produced mainly by intestinal flora fermentation of fiber in food, among which the main functional products are acetic acid, propionic acid, and butyric acid (Tran et al., 2020; Rauf et al., 2022). Sodium propionate (SP) and tributyrin (TB) are stable and efficient forms of propionic acid and butyric acid, respectively (Miyoshi et al., 2011; Silva et al., 2016). Solid-form SCFAs are a practical addition to animal feed formulations due to their better handling and storage properties (Silva et al., 2016), and SP is a high-stability, high-safety chelated form of propionic acid that affects lipid metabolism and regulates the immune system. In a recent study, dietary supplementation with SP improved growth performance, hepatic lipid deposition, and health status in rainbow trout (Oncorhynchus mykiss) (Yousefi et al., 2024). TB is a butyric acid derivative formed by the esterification of three butyric acid molecules and one glycerol molecule (Gerunova et al., 2024), which is a naturally stable compound compared with butyrate and can effectively pass through the stomach and be broken down in the intestine, releasing butyric acid to function (Miyoshi et al., 2011; Hou et al., 2014). In addition, TB has fewer palatability issues than butyrate (Palma et al., 2023). In aquatic animals, TB also play a regulatory role in fish growth, metabolism and health. The growth-promoting effects of TB have been extensively reported, such as in snakehead fish (Channa argus) (Hou et al., 2019), blunt snout bream (Megalobrama amblycephala) (Liang et al., 2021), and common carp (Cyprinus carpio) (Xie et al., 2021), and the improvement of digestive enzyme activities may be an important reason. In addition, TB reduced triglycerides content and cholesterol levels in large yellow croaker (Larimichthys crocea) (Xu et al., 2021) and hybrid grouper (Epinephelus fuscoguttatus♀× Epinephelus lanceolatus♂) (Yin et al., 2021), in which the hepatic lipid metabolism was involved. Moreover, TB could also modulate the antioxidant capacities of fish (Hou et al., 2019; Liang et al., 2021). Therefore, in addition to promoting growth, SP and TB modulated lipid metabolism and immune responses, presenting a stable and safe option for inclusion in animal feed formulations (Silva et al., 2016; Palma et al., 2023). However, few studies have focused on both SP and TB.
Spotted seabass (Lateolabrax maculatus) is a popular farmed fish in China because of its delicious meat, rapid growth, and high economic value, with a production of 246,918 tons in 2023 (Cai et al., 2020; Xing et al., 2023). High stocking density, excess feed, and exogenous pathogens endanger the health of spotted seabass (Dong et al., 2022). Abnormal hepatic lipid deposition and hypoxic stress are two major problems in fish farming, weakening immune function and disease resistance and even leading to death, which seriously damages economic interests (Hou et al., 2023). Therefore, the objectives of the present study were to investigate the effects of SP and TB on the hepatic lipid deposition and health status of spotted seabass, which will provide references for aquatic feed and improve our understanding of the functional mechanism of SCFAs in fish.
2 Materials and methods
2.1 Diet formulation
Five experimental diets were formulated: a control diet (CON), a diet supplemented with 2 g/kg SP (SP-0.2%), 4 g/kg SP (SP-0.4%), 2 g/kg TB (TB-0.2%), or 4 g/kg TB (TB-0.4%) (Table 1), the dosage of the additives used was referred to the previous studies (Safari et al., 2016; Safari et al., 2017; Cheng et al., 2021; Xu et al., 2021). The basis for the formulation of the control diet was referred to those of commercial feeds. White fish meal, poultry byproduct meal, soybean meal, corn gluten meal, and cottonseed protein concentrate were used as the source of protein. Fish oil and soybean oil were used as the source of lipid. Wheat flour was used as the main source of carbohydrate. The crude protein and crude lipid contents of the experimental diets were ~48% and ~16%, respectively. The SP (99.0%, #S100122) and TB (98%, #G106350) were obtained from Shanghai Aladdin Biochemical Technology Co., Ltd. (China), and the other ingredients were obtained from Guangdong Haid Group Co., Ltd. (China). The ingredients were crushed to a fine powder and mixed thoroughly to create pellets. The experimental diets were prepared and stored following previous study procedures (Li et al., 2019). According to a previous study (Li et al., 2023), the proximate composition of experimental diets was analyzed by the standard procedures of AOAC.
2.2 Experimental procedure and sampling
Spotted seabass of similar size (mean weight: ~58 g) were randomly assigned to the five groups. Each group contained four replicates, and each replicate contained 30 fish (in an indoor aquarium with a diameter of 2 meters). The fish were reared for 8 weeks. The fish were fed twice a day at 06:00 and 18:00. During the feeding experiment, the water temperature was maintained at 28 ± 1°, the dissolved oxygen was maintained at 7–7.5 mg/L, and the ammonia nitrogen was maintained at < 0.2 mg/L. After the feeding experiment, the fish were anesthetized using MS222 (1:10,000; Sigma−Aldrich, USA). All fish in each aquarium were weighed, the physical indices were measured, and the fish livers were dissected for analysis.
The feeding experiment was followed by a hypoxic stress experiment. For each group, three fish from four aquariums were gathered in one aquarium. Finally, 12 fish were included in each group. The experimental fish were acclimated to the new environment for one day, and the dissolved oxygen concentration was maintained consistently (the dissolved oxygen was maintained at 7–7.5 mg/L). After a day of acclimatization, the oxygen supply was halted in all aquariums simultaneously. Subsequently, the number of dead fish was counted every 10 min to evaluate the hypoxic stress.
The experimental procedures were performed in strict accordance with the Management Rule of Laboratory Animals (Chinese Order No. 676 of the State Council, revised 1 March 2017).
2.3 Oil red O staining and triglyceride measurement
Oil red O staining was carried out as described previously (Li et al., 2021). Briefly, the fish liver (5 mm × 5 mm pieces) was stored in 4% paraformaldehyde before staining. The samples were sectioned into 6-μm sections using a cryostat microtome, fixed in cold 10% buffered formalin, and stained with oil red O. The TG content of the fish liver was measured using a commercial kit (#A110, Nanjing Jiancheng Bioengineering Institute, China) and the glycerol-3 phosphate oxidase phenol-4-chlorophenol aminophenazone (GPO-PAP) method.
2.4 Antioxidant capacity
Malondialdehyde (MDA, #BC0025), total antioxidant capacity (T-AOC, #BC1310), superoxide dismutase (SOD, #BC0170), and catalase (CAT, #BC1190) were measured using specific commercial kits (Solarbio, China). The reagent preparation, liver tissue homogenization, and operation were performed in strict accordance with the manufacturer’s instructions.
2.5 Real-time quantitative PCR
RT-qPCR was carried out according to a previous study (Cui et al., 2020). Briefly, total RNA was extracted from liver tissue using TRIzol according to the manufacturer’s instructions (Takara, Japan). The RNA was reverse-transcribed into complementary DNA (cDNA) using a PrimeScript™ Reverse RT Reagent Kit (Takara). PCR amplification was performed containing 1 μl cDNA, 1 μl each primer, 12.5 μl PrimeSTAR® Max DNA Polymerase (Takara), and 9.5 μl RNase-free water. The PCR cycling conditions were 10 s at 98°, 15 s at 58°, and 20 s at 72° for 35 cycles. A single PCR product was confirmed by a melting curve analysis. The RT-qPCR primers referenced that of a previous study (Tan et al., 2017) (Table 2). The housekeeping gene was β-actin. The gene expression levels were calculated using the comparative threshold (CT) cycle (2-ΔΔCT) method (Livak and Schmittgen, 2001).
2.6 RNA sequencing
The RNA extraction, cDNA library construction, and sequencing were conducted by Majorbio (China). The gene expression levels were calculated using transcripts per million reads (TPM) in RSEM (http://deweylab.biostat.wisc.edu/rsem/), and the threshold for significance was a P-adjusted value of 0.05. The Kyoto Encyclopedia of Genes and Genomes (KEGG) database (http://www.genome.jp/kegg) was used for pathway enrichment, and the significance threshold in the KEGG pathway enrichment was a P-adjusted value of 0.05. The heatmap analysis was conducted using fastcluster, which used log210 gene expression, and the subcluster was calculated according to the relative gene distance. The raw data were submitted to the National Center for Biotechnology Information (NCBI) Sequence Read Archive (SRA) (PRJNA1150789).
2.7 Calculations and statistical analysis
Survival rate (SR, %) = (final fish number/initial fish number) × 100;
Weight gain (WG, %) = (final body weight − initial body weight) × 100/initial body weight;
Feed conversion ratio (FCR) = feed consumption/body weight gain;
Condition factor (CF, g/cm3) = (final body weight/final body length3) × 100;
Hepatosomatic index (HSI, %) = (liver weight/body weight) × 100;
Viscerosomatic index (VSI, %) = (viscera weight/body weight) × 100.
All statistical analyses were conducted using SPSS 25.0 (IBM, USA). All data are reported as the mean ± standard error of the mean (SEM). All data were analyzed using one-way analysis of variance (ANOVA), followed by Tukey’s multiple range test or independent sample t-test. Differences were considered statistically significant when P < 0.05.
3 Results
3.1 SP and TB supplementation on survival and growth
The survival rates of the five groups ranged from 97-99%, and there were no significant differences among the groups (P > 0.05). Compared with those of the CON group, the growth performance, including final body weight, WG rate, and FCR, was not significantly different among the groups, although the SP-0.2% group presented a slightly higher WG rate and slightly lower FCR (P > 0.05) (Table 3).
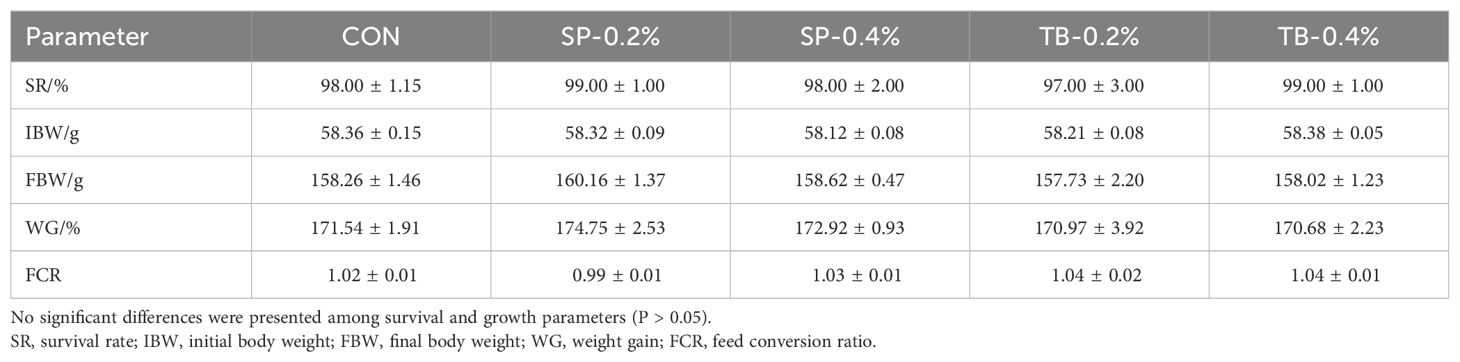
Table 3. Effects of dietary sodium propionate (SP) and tributyrin (TB) on spotted seabass survival and growth.
3.2 SP and TB supplementation on physical indices
The CF was not significantly different among the groups. Notably, the HSI and VSI were different among the groups, and the SP-0.4% and TB-0.2% groups had a significantly lower HSI and VSI (P < 0.05) (Table 4), revealing a potentially lower hepatic lipid deposition.

Table 4. Effects of dietary sodium propionate (SP) and tributyrin (TB) on spotted seabass physical indices.
3.3 SP and TB supplementation on hypoxic stress
Hypoxic stress causes severe losses in farmed spotted seabass, thus the 8-week feeding experiment was followed by a hypoxic stress experiment. The CON fish began to die after 6 h after the oxygen supply was halted (the dissolved oxygen in each group reduced to approximately 1 mg/L), and all CON fish died after 7 h after oxygen was halted, demonstrating the weakest hypoxia resistance in all groups (Figure 1). Notably, all the SP and TB fish began to die later than that of the CON fish. The SP-0.4% and TB-0.2% fish began to die last, and the total death time in the SP-0.4% and TB-0.2% groups was also the longest, as it was ~2 h longer than that of the CON group, indicating stronger hypoxia stress resistance.
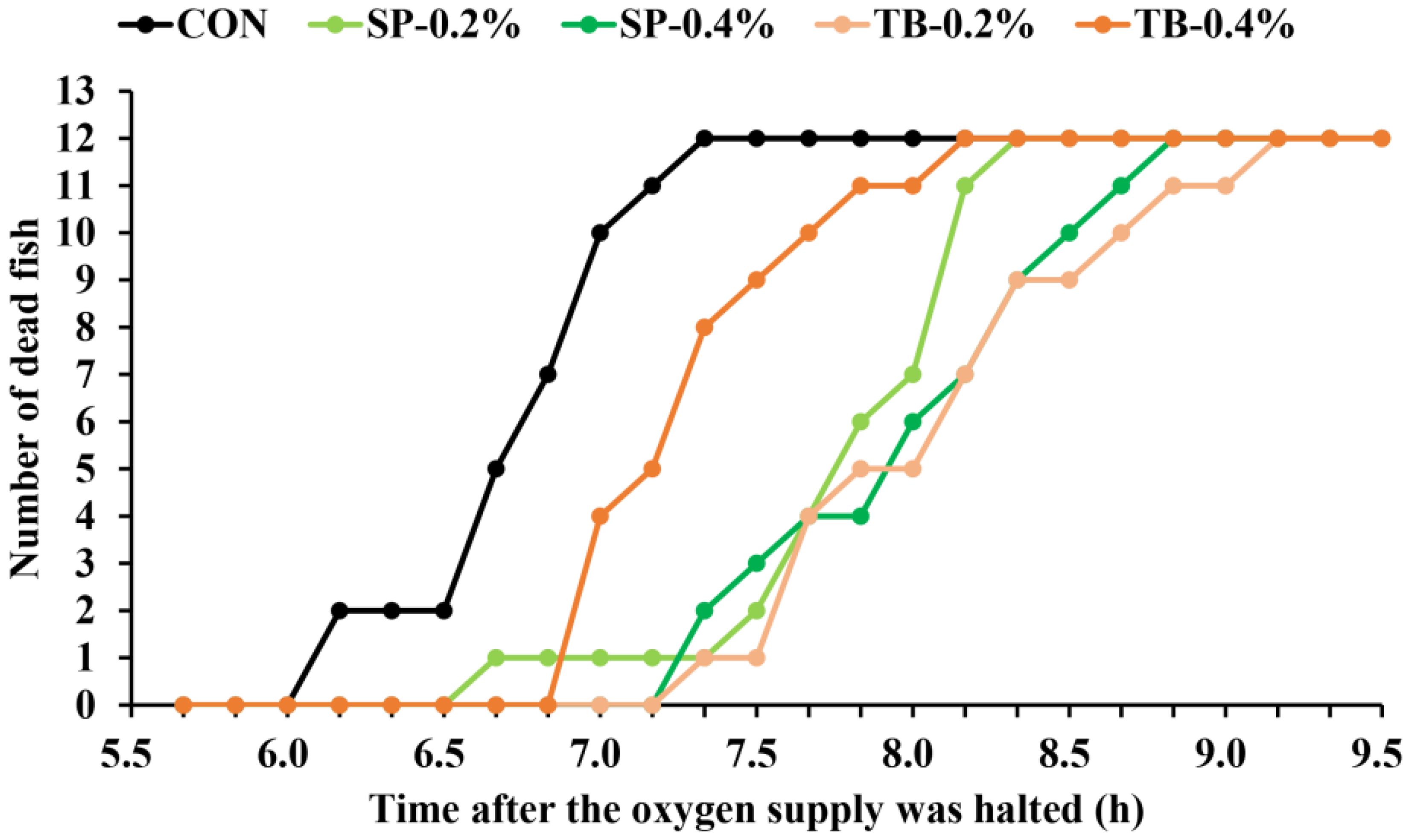
Figure 1. Number of dead fish after the oxygen supply was halted. After the feeding experiment and sampling, the oxygen supply was halted simultaneously, and the number of dead fish in each group was counted every 10 min.
3.4 SP and TB supplementation on oil red O staining and TG levels
Considering that the SP-0.4% and TB-0.2% groups had lower HSI and VSI than the CON group (Table 4), oil red O staining was conducted to determine the fish hepatic TG levels (Figure 2). The results revealed that the SP-0.4% and TB-0.2% groups had smaller lipid droplets and significantly downregulated liver TG levels, indicating that SP-0.4% and TB-0.2% dietary supplementation reduced hepatic lipid deposition.
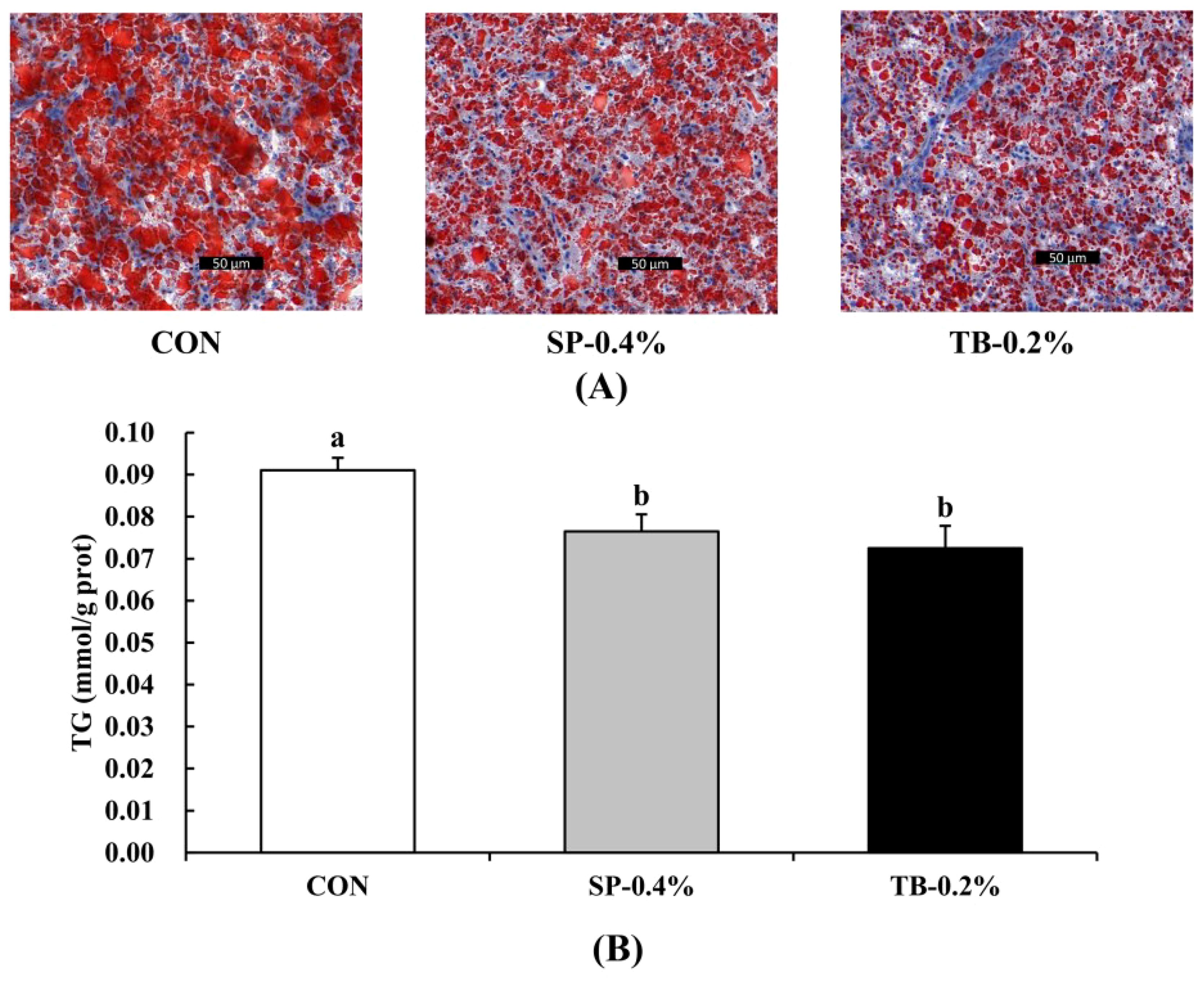
Figure 2. Effects of SP and TB dietary supplementation on hepatic lipid deposition. (A) Liver histochemical characteristics (oil red O staining). Scale bars = 50 μm. (B) Liver TG levels. Mean values (mean ± SEM, n = 4) with different letters are significantly different (P < 0.05).
3.5 SP and TB supplementation on antioxidant capacity
The results of antioxidant capacity revealed that the SP-0.4% and TB-0.2% groups had significantly lower MDA contents (P < 0.05) and significantly upregulated T-AOC, SOD, and CAT, and the expression levels of the antioxidant capacity-related genes: nrf2 (nuclear erythroid 2-related factor 2), cat, and sod (P < 0.05) (Figure 3). The results suggested that the SP-0.4% and TB-0.2% groups had improved antioxidant capacity.
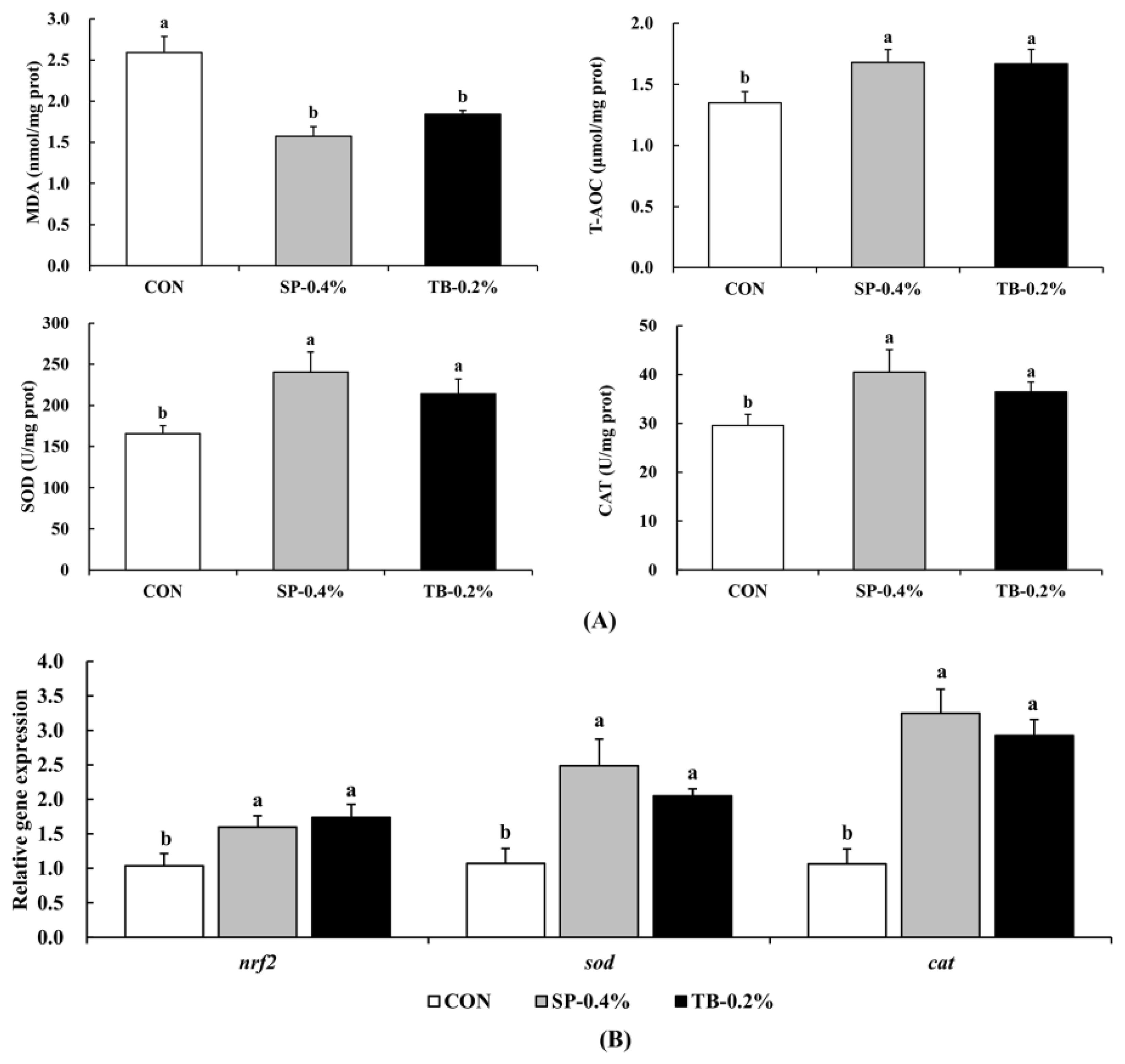
Figure 3. Effects of SP and TB dietary supplementation on antioxidant capacity. (A) MDA, T-AOC, SOD, and CAT levels. (B) Relative gene expression levels of nrf2, sod, and cat. Mean values (mean ± SEM, n = 4) with different letters are significantly different (P < 0.05).
3.6 SP and TB supplementation on RNA-seq
The SP-0.4% and TB-0.2% regulation of hepatic gene expression were analyzed using RNA-seq (Figure 4). Compared with the CON group, SP upregulated 57 genes and downregulated 28 genes, while TB upregulated 43 genes and downregulated 5 genes. Additionally, TB upregulated only 7 genes and downregulated 9 genes compared with SP, revealing that SP and TB had similar effects on gene expression levels (Figure 4A). The Venn diagram and heatmap analyses supported these results (Figures 4B, E). The subcluster analysis revealed that 67 genes from 117 differentially expressed genes were enriched in the same cluster (Figure 4F). KEGG enrichment analysis revealed that the FoxO signaling, PI3K-Akt signaling, and insulin-related pathways were key in SP and TB functions (Figures 4C, D, G).
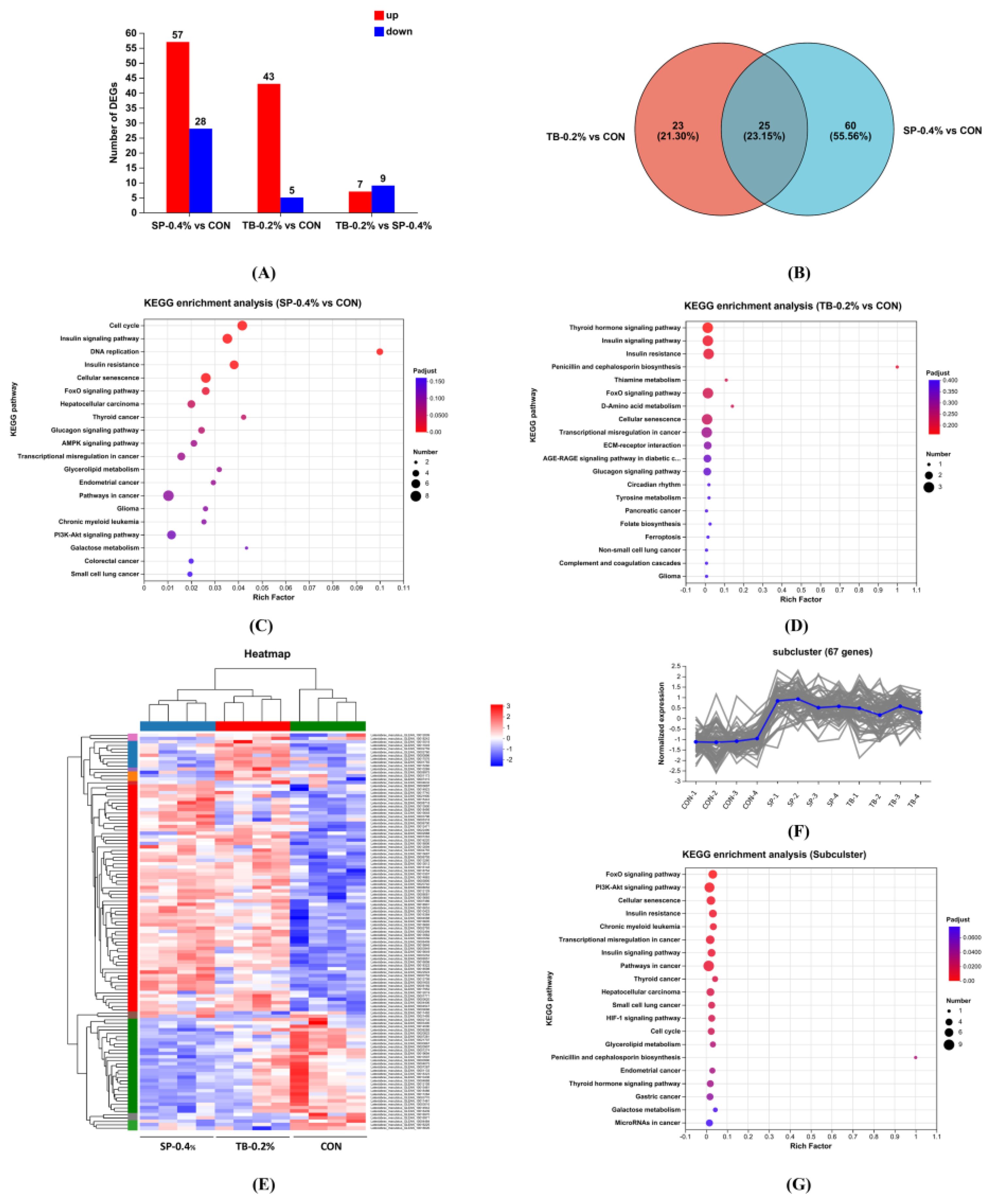
Figure 4. RNA-seq of SP and TB dietary supplementation. (A) The number of differentially expressed genes. (B) Venn diagram of the differentially expressed genes. (C) KEGG enrichment analysis of SP-regulated genes. (D) KEGG enrichment analysis of TB-regulated genes. (E) Heatmap analysis of differentially expressed genes. (F) Subcluster of 67 genes. (G) KEGG enrichment analysis of 67 genes.
4 Discussion
In the present study, the survival rate and growth performance were not significantly different among the groups. Previous studies reported that SCFAs had no significant growth-promoting effects on Atlantic salmon (Salmo salar) (Bjerkeng et al., 1999), rainbow trout (Oncorhynchus mykiss) (Gao et al., 2011), gilthead sea bream (Sparus aurata) (Benedito-Palos et al., 2016), and red hybrid tilapia (Oreochromis sp.) (Ebrahimi et al., 2017). However, SCFAs promoted the growth performance of sea bream (S. aurata) (Robles et al., 2013), grass carp (Ctenopharyngodon idellus) (Liu et al., 2017), Nile tilapia (Oreochromis niloticus) (Hu et al., 2018), turbot (Scophthalmus maximus) (Liu et al., 2019), golden pompano (Trachinotus ovatus) (Zhou et al., 2019), Barramundi (Lates calcarifer) (Aalamifar et al., 2020), and yellow drum (Nibea albiflora) (Wu et al., 2020). There are hundreds of fish species farmed around the world, and they have different feeding habits and different living environments. Therefore, the different results might primarily be due to the fish species and the experimental conditions. Then, the differences in basal feed formula and supplementation levels can also lead to different results.
The liver is key to maintaining normal fish growth and health, and excessive hepatic lipid deposition can severely affect liver function. In mammals, SCFAs play a role in energy homeostasis and metabolism (den Besten et al., 2013; Sahuri-Arisoylu et al., 2016), and SCFAs alleviate lipid deposition in the liver (Morrison and Preston, 2016). In the present study, both SP-0.4% and TB-0.2% downregulated the fish HSI, VSI, and TG, reducing hepatic lipid deposition. The downregulation of HSI was consistent with that reported in previous fish studies. In juvenile Pengze crucian carp (Carassius auratus Pengze), the HSI and lipid were significantly decreased in supplementing SB groups compared with that of the control group, and the antioxidant capacity, intestinal histomorphology, and immune response were all improved (Fang et al., 2021). In juvenile largemouth bass (Micropterus salmoides), dietary supplementation with 2.0 g/kg SB had significantly lower HSI, and the antioxidant activities, inflammatory response, and resistance to hypoxic stress were all improved (Hou et al., 2023). Therefore, the improvement of physical indices by SCFAs is often accompanied by the promotion of health. The factors affecting lipid deposition include absorption, lipogenesis, and lipid oxidation. Under certain conditions of absorption, lipogenesis and lipid oxidation determine the level of lipid deposition. In juvenile large yellow croaker (Larimichthys crocea), replacement of fish oil with soybean oil in diets significantly increased the lipid deposition in the liver, and SCFAs alleviated the abnormal lipid deposition by decreasing the expression of lipogenesis-related genes and increasing the expression of lipid oxidation-related genes (Xu et al., 2021). Therefore, SCFAs could be useful for reducing hepatic lipid deposition in fish, which could regulate lipogenesis and lipid oxidation at the same time.
Unlike terrestrial animals, fish basically obtain their oxygen requirements from water, and hypoxic stress has received more attention. Hypoxic stress is an important factor affecting the survival rate of spotted bass in aquaculture. In the present study, both SP-0.4% and TB-0.2% improved the antioxidant capacity and hypoxic stress resistance of spotted seabass. In aquaculture, water eutrophication and increased culture density can decrease dissolved oxygen concentrations, increasing the risk of hypoxic stress (Hou et al., 2023; Jia et al., 2023), especially for spotted seabass, which has a relatively high oxygen demand. In fish, hypoxia stress leads to excessive accumulation of reactive oxygen species, leading to protein denaturation, lipid peroxidation, and even cell damage (Martínez-Álvarez et al., 2005; Peng et al., 2022), which can cause many diseases and even death (Zhao et al., 2020). The antioxidant system, which mainly consists of the antioxidant enzyme, can prevent the production of reactive oxygen species and avoid cell damage (Hughes, 1999). In previous studies, SCFAs improved the antioxidant enzymes activities, including SOD, CAT, and T-AOC, and reduced the MDA in zebrafish (Danio rerio) (Safari et al., 2016), carp (Cyprinus carpio L.) (Safari et al., 2017), Nile tilapia (O. niloticus) (Dawood et al., 2020), black sea bream (Acanthopagrus schlegelii) (Volatiana et al., 2020), yellow catfish (Pelteobagrus fulvidraco) (Zhao et al., 2021), and grass carp (C. idella) (Cheng et al., 2021), thereby improving the fish health. Furthermore, the improvement of antioxidant capacity improved the stress resistance in fish. SCFAs improved hypoxia stress resistance in largemouth bass (M. salmoides) (Hou et al., 2023) and improved ammonia stress resistance in yellow catfish (P. fulvidraco) (Zhao et al., 2021). Therefore, SCFAs could be used to improve antioxidant capacity and hypoxic stress resistance in aquaculture, thereby improving the survival rate of fish in aquaculture.
RNA-seq was conducted to analyze the global regulation of gene expression and explore the potential functional pathways of SP and TB. The results revealed that SP-0.4% and TB-0.2% had similar effects on the gene expression levels, and the FoxO signaling, PI3K-Akt signaling, and insulin-related pathways might be key pathways involved in their functions. The three signaling pathways all played key roles in lipid metabolism and health (Sell et al., 2012; Martini et al., 2014; Farhan et al., 2017), which improved the understanding of the functional mechanism of SCFAs in fish. There are a few studies on the mechanism of SCFA function in aquatic animals. In grass carp (C. idella), sodium butyrate improved the intestinal immune function associated with the NF-κB and p38-MAPK signaling pathways (Tian et al., 2017) and enhanced the physical barrier function of the Nrf2, JNK, and MLCK signaling pathways (Wu et al., 2018). Furthermore, SCFAs improved largemouth bass (M. salmoides) immunity through the TLR22-MyD88-NF-κB signaling pathway (Hou et al., 2023). In addition, mammalian studies provide more references. For example, SP activated the small intestinal gluconeogenesis pathway through the GPR41 gut-brain axis, improving metabolism (De Vadder et al., 2014). Moreover, SP improved the abnormal lipid deposition induced by a high-fat diet through the PPARγ-UCP2-AMPK pathway (den Besten et al., 2015). Additionally, SCFAs inhibited glycogen synthase kinase 3β and increased Nrf2 activity, increasing the antioxidant capacity (Xing et al., 2016). Therefore, the in-depth functional mechanism of SP, TB, and other SCFAs in fish has many research gaps and should be studied in further studies.
5 Conclusion
Dietary supplementation with 0.4% sodium propionate and 0.2% tributyrin were feasible strategies for improving the hepatic lipid deposition, antioxidant capacity, and hypoxic stress resistance of spotted seabass, which provides references for aquatic feed. The sodium propionate and tributyrin-regulated genes presented similar expression patterns and involved the FoxO signaling, PI3K-Akt signaling, and insulin-related pathways, which could improve our understanding of the functional mechanism of SCFAs in fish. The in-depth functional mechanisms of certain SCFAs should be studied. Subsequently, considering the similar functions and gene regulation of sodium propionate and tributyrin, the synergistic effects of sodium propionate, tributyrin, and other SCFAs should also be examined.
Data availability statement
The raw data were submitted to the National Center for Biotechnology Information (NCBI) Sequence Read Archive (SRA) (PRJNA1150789).
Ethics statement
The experimental procedures were performed in strict accordance with the Management Rule of Laboratory Animals (Chinese Order No. 676 of the State Council, revised 1 March 2017). The study was conducted in accordance with the local legislation and institutional requirements.
Author contributions
KC: Writing – original draft, Methodology, Data curation, Conceptualization. HZ: Writing – review & editing, Methodology, Data curation, Conceptualization. BY: Writing – review & editing, Methodology, Data curation, Conceptualization. JW: Writing – review & editing, Methodology, Data curation, Conceptualization. XQ: Writing – review & editing, Validation, Methodology, Conceptualization. MX: Writing – review & editing, Validation, Methodology, Conceptualization.
Funding
The author(s) declare financial support was received for the research, authorship, and/or publication of this article. The study was supported by the National Key Research and Development Program of China (2023YFD2402000) and the Panyu Innovation and Entrepreneurship Leading Team Project (2021-R01-4).
Acknowledgments
The authors are grateful to XM Chen, BY Guo, SQ Xiao, QZ Bi, XM Zhou, Y Zhang, and GY You for their kind help in the feeding experiments and sampling. The authors are also grateful to W Fang, QC Chen, YT Liu, N Xu, and XN Wang for their kind help in the study.
Conflict of interest
Authors KC, HZ, BY, JW, and XQ were employed by the company Guangdong Haid Group Co., Ltd.
The remaining author declares that the research was conducted in the absence of any commercial or financial relationships that could be construed as a potential conflict of interest.
Publisher’s note
All claims expressed in this article are solely those of the authors and do not necessarily represent those of their affiliated organizations, or those of the publisher, the editors and the reviewers. Any product that may be evaluated in this article, or claim that may be made by its manufacturer, is not guaranteed or endorsed by the publisher.
References
Aalamifar H., Soltanian S., Vazirzadeh A., Akhlaghi M., Morshedi V., Gholamhosseini A., et al. (2020). Dietary butyric acid improved growth, digestive enzyme activities and humoral immune parameters in Barramundi (Lates calcarifer). Aquaculture Nutr. 26, 156–164. doi: 10.1111/anu.12977
Abdelsalam M., Elgendy M. Y., Elfadadny M. R., Ali S. S., Sherif A. H., Abolghait S. K. (2023). A review of molecular diagnoses of bacterial fish diseases. Aquaculture Int. 31, 417–434. doi: 10.1007/s10499-022-00983-8
Benedito-Palos L., Ballester-Lozano G. F., Simó P., Karalazos V., Ortiz A., Calduch-Giner J., et al. (2016). Lasting effects of butyrate and low FM/FO diets on growth performance, blood haematology/biochemistry and molecular growth-related markers in gilthead sea bream (Sparus aurata). Aquaculture 454, 8–18. doi: 10.1016/j.aquaculture.2015.12.008
Bjerkeng B., Storebakken T., Wathne E. (1999). Cholesterol and short-chain fatty acids in diets for Atlantic salmon Salmo salar (L.): Effects on growth, organ indices, macronutrient digestibility, and fatty acid composition. Aquaculture Nutr. 5, 181–191. doi: 10.1046/j.1365-2095.1999.00103.x
Cai L. S., Wang L., Song K., Lu K. L., Zhang C. X., Rahimnejad S. (2020). Evaluation of protein requirement of spotted seabass (Lateolabrax maculatus) under two temperatures, and the liver transcriptome response to thermal stress. Aquaculture 516, 734615. doi: 10.1016/j.aquaculture.2019.734615
Cheng Z., Yang H., Xu Z., Li X., Leng X. (2021). Dietary supplementation of tributyrin improved the growth, feed utilization and intestinal histology of grass carp (Ctenopharyngodon idella). Aquaculture Nutr. 27, 2007–2018. doi: 10.1111/anu.13336
Cui K., Li X., Chen Q., Li Q., Gao S., Tan P., et al. (2020). Effect of replacement of dietary fish oil with four vegetable oils on prostaglandin E2 synthetic pathway and expression of inflammatory genes in marine fish Larimichthys crocea. Fish Shellfish Immunol. 107, 529–536. doi: 10.1016/j.fsi.2020.09.038
Dawood M. A., Eweedah N. M., Elbialy Z. I., Abdelhamid A. I. (2020). Dietary sodium butyrate ameliorated the blood stress biomarkers, heat shock proteins, and immune response of Nile tilapia (Oreochromis niloticus) exposed to heat stress. J. Thermal Biol. 88, 102500. doi: 10.1016/j.jtherbio.2019.102500
den Besten G., Bleeker A., Gerding A., van Eunen K., Havinga R., van Dijk T. H., et al. (2015). Short-chain fatty acids protect against high-fat diet–induced obesity via a PPARγ-dependent switch from lipogenesis to fat oxidation. Diabetes 64, 2398–2408. doi: 10.2337/db14-1213
den Besten G., Lange K., Havinga R., van Dijk T. H., Gerding A., van Eunen K., et al. (2013). Gut-derived short-chain fatty acids are vividly assimilated into host carbohydrates and lipids. Am. J. Physiology-Gastrointestinal Liver Physiol. 305, G900–G910. doi: 10.1152/ajpgi.00265.2013
De Vadder F., Kovatcheva-Datchary P., Goncalves D., Vinera J., Zitoun C., Duchampt A., et al. (2014). Microbiota-generated metabolites promote metabolic benefits via gut-brain neural circuits. Cell 156, 84–96. doi: 10.1016/j.cell.2013.12.016
Dong Y., Li L., Xia T., Wang L., Xiao L., Ding N., et al. (2022). Oxidative stress can be attenuated by 4-PBA caused by high-fat or ammonia nitrogen in cultured spotted seabass: the mechanism is related to endoplasmic reticulum stress. Antioxidants 11, 1276. doi: 10.3390/antiox11071276
Ebrahimi M., Daeman N. H., Chong C. M., Karami A., Kumar V., Hoseinifar S. H., et al. (2017). Comparing the effects of different dietary organic acids on the growth, intestinal short-chain fatty acids, and liver histopathology of red hybrid tilapia (Oreochromis sp.) and potential use of these as preservatives. Fish Physiol. Biochem. 43, 1195–1207. doi: 10.1007/s10695-017-0365-0
Fang L., Wang Q., Guo X., Pan X., Li X. (2021). Effects of dietary sodium butyrate on growth performance, antioxidant capacity, intestinal histomorphology and immune response in juvenile Pengze crucian carp (Carassius auratus Pengze). Aquaculture Rep. 21, 100828. doi: 10.1016/j.aqrep.2021.100828
Farhan M., Wang H., Gaur U., Little P. J., Xu J., Zheng W. (2017). FOXO signaling pathways as therapeutic targets in cancer. Int. J. Biol. Sci. 13, 815. doi: 10.7150/ijbs.20052
Gao Y., Storebakken T., Shearer K. D., Penn M., Øverland M. (2011). Supplementation of fishmeal and plant protein-based diets for rainbow trout with a mixture of sodium formate and butyrate. Aquaculture 311, 233–240. doi: 10.1016/j.aquaculture.2010.11.048
Gerunova L. K., Gerunov T. V., P’yanova L. G., Lavrenov A. V., Sedanova A. V., Delyagina M. S., et al. (2024). Butyric acid and prospects for creation of new medicines based on its derivatives: a literature review. J. Veterinary Sci. 25. doi: 10.4142/jvs.23230
Hoseinifar S. H., Sun Y. Z., Caipang C. M. (2017). Short-chain fatty acids as feed supplements for sustainable aquaculture: An updated view. Aquaculture Res. 48, 1380–1391. doi: 10.1111/are.13239
Hou Y., Hou Y., Yao L., Chen S., Fan J., Qian L. (2019). Effects of chromium yeast, tributyrin and bile acid on growth performance, digestion and metabolism of Channa argus. Aquaculture Res. 50, 836–846. doi: 10.1111/are.2019.50.issue-3
Hou D., Li M., Li P., Chen B., Huang W., Guo H., et al. (2023). Effects of sodium butyrate on growth performance, antioxidant status, inflammatory response and resistance to hypoxic stress in juvenile largemouth bass (Micropterus salmoides). Front. Immunol. 14, 1265963. doi: 10.3389/fimmu.2023.1265963
Hou Y., Wang L., Yi D., Ding B., Chen X., Wang Q., et al. (2014). Dietary supplementation with tributyrin alleviates intestinal injury in piglets challenged with intrarectal administration of acetic acid. Br. J. Nutr. 111, 1748–1758. doi: 10.1017/S0007114514000038
Hu E. D., Chen D. Z., Wu J. L., Lu F. B., Chen L., Zheng M. H., et al. (2018). High fiber dietary and sodium butyrate attenuate experimental autoimmune hepatitis through regulation of immune regulatory cells and intestinal barrier. Cell. Immunol. 328, 24–32. doi: 10.1016/j.cellimm.2018.03.003
Hughes D. A. (1999). Effects of dietary antioxidants on the immune function of middle-aged adults. Proc. Nutr. Soc. 58, 79–84. doi: 10.1079/PNS19990012
Jia Y., Wang F., Gao Y., Qin H., Guan C. (2023). Hypoxia stress induces hepatic antioxidant activity and apoptosis, but stimulates immune response and immune-related gene expression in black rockfish Sebastes schlegelii. Aquat. Toxicol. 258, 106502. doi: 10.1016/j.aquatox.2023.106502
Li X., Cui K., Fang W., Chen Q., Xu D., Mai K., et al. (2019). High level of dietary olive oil decreased growth, increased liver lipid deposition and induced inflammation by activating the p38 MAPK and JNK pathways in large yellow croaker (Larimichthys crocea). Fish Shellfish Immunol. 94, 157–165. doi: 10.1016/j.fsi.2019.08.062
Li X., Sun J., Wang L., Song K., Lu K., Zhang L., et al. (2023). Effects of dietary vitamin E levels on growth, antioxidant capacity and immune response of spotted seabass (Lateolabrax maculatus) reared at different water temperatures. Aquaculture 565, 739141. doi: 10.1016/j.aquaculture.2022.739141
Li J., Xu W., Lai W., Kong A., Zhang Z., Pang Y., et al. (2021). Effect of dietary methionine on growth performance, lipid metabolism and antioxidant capacity of large yellow croaker (Larimichthys crocea) fed with high lipid diets. Aquaculture 536, 736388. doi: 10.1016/j.aquaculture.2021.736388
Liang H., Ji K., Ge X., Xi B., Ren M., Zhang L., et al. (2021). Tributyrin plays an important role in regulating the growth and health status of juvenile blunt snout bream (Megalobrama amblycephala), as evidenced by pathological examination. Front. Immunol. 12, 652294. doi: 10.3389/fimmu.2021.652294
Liu Y., Chen Z., Dai J., Yang P., Xu W., Ai Q., et al. (2019). Sodium butyrate supplementation in high-soybean meal diets for turbot (Scophthalmus maximus L.): effects on inflammatory status, mucosal barriers and microbiota in the intestine. Fish Shellfish Immunol. 88, 65–75. doi: 10.1016/j.fsi.2019.02.064
Liu M., Guo W., Wu F., Qu Q., Tan Q., Gong W. (2017). Dietary supplementation of sodium butyrate may benefit growth performance and intestinal function in juvenile grass carp (Ctenopharyngodon idellus). Aquaculture Res. 48, 4102–4111. doi: 10.1111/are.2017.48.issue-8
Livak K. J., Schmittgen T. D. (2001). Analysis of relative gene expression data using real-time quantitative PCR and the 2– ΔΔCT method. Methods 25, 402–408. doi: 10.1006/meth.2001.1262
Martínez-Álvarez R. M., Morales A. E., Sanz A. (2005). Antioxidant defenses in fish: biotic and abiotic factors. Rev. Fish Biol. Fisheries 15, 75–88. doi: 10.1007/s11160-005-7846-4
Martini M., De Santis M. C., Braccini L., Gulluni F., Hirsch E. (2014). PI3K/AKT signaling pathway and cancer: an updated review. Ann. Med. 46, 372–383. doi: 10.3109/07853890.2014.912836
Miyoshi M., Sakaki H., Usami M., Iizuka N., Shuno K., Aoyama M., et al. (2011). Oral administration of tributyrin increases concentration of butyrate in the portal vein and prevents lipopolysaccharide-induced liver injury in rats. Clin. Nutr. 30, 252–258. doi: 10.1016/j.clnu.2010.09.012
Morrison D. J., Preston T. (2016). Formation of short chain fatty acids by the gut microbiota and their impact on human metabolism. Gut Microbes 7, 189–200. doi: 10.1080/19490976.2015.1134082
Ng W. K., Koh C. B. (2017). The utilization and mode of action of organic acids in the feeds of cultured aquatic animals. Rev. Aquaculture 9, 342–368. doi: 10.1111/raq.2017.9.issue-4
Okocha R. C., Olatoye I. O., Adedeji O. B. (2018). Food safety impacts of antimicrobial use and their residues in aquaculture. Public Health Rev. 39, 1–22. doi: 10.1186/s40985-018-0099-2
Oliva-Teles A. (2012). Nutrition and health of aquaculture fish. J. Fish Dis. 35, 83–108. doi: 10.1111/j.1365-2761.2011.01333.x
Palma M., Magnoni L. J., Morais S., Viegas I. (2023). Tributyrin supplementation in fish and crustacean nutrition: a review. Rev. Aquaculture 15, 785–800. doi: 10.1111/raq.12759
Peng K., Lv X., Zhao H., Chen B., Chen X., Huang W. (2022). Antioxidant and intestinal recovery function of condensed tannins in Lateolabrax maculatus responded to in vivo and in vitro oxidative stress. Aquaculture 547, 737399. doi: 10.1016/j.aquaculture.2021.737399
Pohlenz C., Gatlin D. M. III (2014). Interrelationships between fish nutrition and health. Aquaculture 431, 111–117. doi: 10.1016/j.aquaculture.2014.02.008
Rauf A., Khalil A. A., Rahman U. U., Khalid A., Naz S., Shariati M. A., et al. (2022). Recent advances in the therapeutic application of short-chain fatty acids (SCFAs): An updated review. Crit. Rev. Food Sci. Nutr. 62, 6034–6054. doi: 10.1080/10408398.2021.1895064
Robles R., Lozano A. B., Sevilla A., Márquez L., Nuez-Ortín W., Moyano F. J. (2013). Effect of partially protected butyrate used as feed additive on growth and intestinal metabolism in sea bream (Sparus aurata). Fish Physiol. Biochem. 39, 1567–1580. doi: 10.1007/s10695-013-9809-3
Safari R., Hoseinifar S. H., Kavandi M. (2016). Modulation of antioxidant defense and immune response in zebra fish (Danio rerio) using dietary sodium propionate. Fish Physiol. Biochem. 42, 1733–1739. doi: 10.1007/s10695-016-0253-z
Safari R., Hoseinifar S. H., Nejadmoghadam S., Khalili M. (2017). Non-specific immune parameters, immune, antioxidant and growth-related genes expression of common carp (Cyprinus carpio L. ) fed sodium propionate. Aquaculture Res. 48, 4470–4478. doi: 10.1111/are.2017.48.issue-8
Sahuri-Arisoylu M., Brody L. P., Parkinson J. R., Parkes H., Navaratnam N., Miller A. D., et al. (2016). Reprogramming of hepatic fat accumulation and 'browning' of adipose tissue by the short-chain fatty acid acetate. Int. J. Obes. 40, 955–963. doi: 10.1038/ijo.2016.23
Sell H., Habich C., Eckel J. (2012). Adaptive immunity in obesity and insulin resistance. Nat. Rev. Endocrinol. 8, 709–716. doi: 10.1038/nrendo.2012.114
Shao Y., Wang Y., Yuan Y., Xie Y. (2021). A systematic review on antibiotics misuse in livestock and aquaculture and regulation implications in China. Sci. Total Environ. 798, 149205. doi: 10.1016/j.scitotenv.2021.149205
Silva B. C., Nolasco-Soria H., Magallón-Barajas F., Civera-Cerecedo R., Casillas-Hernández R., Seiffert W. (2016). Improved digestion and initial performance of whiteleg shrimp using organic salt supplements. Aquaculture Nutr. 22, 997–1005. doi: 10.1111/anu.2016.22.issue-5
Tan P., Dong X., Xu H., Mai K., Ai Q. (2017). Dietary vegetable oil suppressed non-specific immunity and liver antioxidant capacity but induced inflammatory response in Japanese sea bass (Lateolabrax japonicus). Fish Shellfish Immunol. 63, 139–146. doi: 10.1016/j.fsi.2017.02.006
Tian L., Zhou X. Q., Jiang W. D., Liu Y., Wu P., Jiang J., et al. (2017). Sodium butyrate improved intestinal immune function associated with NF-κB and p38MAPK signalling pathways in young grass carp (Ctenopharyngodon idella). Fish Shellfish Immunol. 66, 548–563. doi: 10.1016/j.fsi.2017.05.049
Tran N. T., Li Z., Wang S., Zheng H., Aweya J. J., Wen X., et al. (2020). Progress and perspectives of short-chain fatty acids in aquaculture. Rev. Aquaculture 12, 283–298. doi: 10.1111/raq.12317
Volatiana J. A., Wang L., Gray N., Tong S., Zhang G., Shao Q. (2020). Tributyrin-supplemented high-soya bean meal diets of juvenile black sea bream, Acanthopagrus schlegelii: Study on growth performance and intestinal morphology and structure. Aquaculture Res. 51, 135–146. doi: 10.1111/are.14355
Wu P., Tian L., Zhou X. Q., Jiang W. D., Liu Y., Jiang J., et al. (2018). Sodium butyrate enhanced physical barrier function referring to Nrf2, JNK and MLCK signaling pathways in the intestine of young grass carp (Ctenopharyngodon idella). Fish Shellfish Immunol. 73, 121–132. doi: 10.1016/j.fsi.2017.12.009
Wu X., Wang L., Xie Q., Tan P. (2020). Effects of dietary sodium butyrate on growth, diet conversion, body chemical compositions and distal intestinal health in yellow drum (Nibea albiflora, Richardson). Aquaculture Res. 51, 69–79. doi: 10.1111/are.14348
Xie D., Dai Q., Xu C., Li Y. (2021). Dietary tributyrin modifies intestinal function by altering morphology, gene expression and microbiota profile in common carp (Cyprinus carpio) fed all-plant diets. Aquaculture Nutr. 27, 439–453. doi: 10.1111/anu.13197
Xing X., Jiang Z., Tang X., Wang P., Li Y., Sun Y., et al. (2016). Sodium butyrate protects against oxidative stress in HepG2 cells through modulating Nrf2 pathway and mitochondrial function. J. Physiol. Biochem. 73, 405–414. doi: 10.1007/s13105-017-0568-y
Xing S., Liang X., Wang H., Xie X., Wierenga P. A., Schrama J. W., et al. (2023). The impacts of physical properties of extruded feed on the digestion kinetics, gastrointestinal emptying and stomach water fluxes of spotted seabass (Lateolabrax maculatus). Aquaculture 570, 739442. doi: 10.1016/j.aquaculture.2023.739442
Xu N., Ding T., Liu Y., Zheng W., Liu Q., Yin Z., et al. (2021). Effects of dietary tributyrin on growth performance, body composition, serum biochemical indexes and lipid metabolism-related genes expression of juvenile large yellow croaker (Larimichthys crocea) fed with high level soybean oil diets. Aquaculture Nutr. 27, 395–406. doi: 10.1111/anu.13192
Yin B., Liu H., Tan B., Dong X., Chi S., Yang Q., et al. (2021). Supplementing tributyrin to cottonseed protein concentrate-based diets can improve growth performance, lipid metabolism and distal intestinal immunity in hybrid grouper (Epinephelus fuscoguttatus♀× Epinephelus lanceolatus♂). Aquaculture Nutr. 27, 2378–2391. doi: 10.1111/anu.13370
Yousefi M., Hoseini S. M., Kulikov E. V., Kharlitskaya E. V., Petukhov N. V., Khomenets N. G. (2024). Dietary propionate administration improves growth performance, hepatic lipid deposition, and intestinal activity of digestive enzymes, inflammation, bacterial population, and antioxidant capacity in rainbow trout, Oncorhynchus mykiss. Aquaculture 578, 740099. doi: 10.1016/j.aquaculture.2023.740099
Zhao H., Peng K., Wang G., Mo W., Huang Y., Cao J. (2021). Metabolic changes, antioxidant status, immune response and resistance to ammonia stress in juvenile yellow catfish (Pelteobagrus fulvidraco) fed diet supplemented with sodium butyrate. Aquaculture 536, 736441. doi: 10.1016/j.aquaculture.2021.736441
Zhao L., Yuan B. D., Zhao J. L., Jiang N., Zhang A. Z., Wang G. Q., et al. (2020). Amelioration of hexavalent chromium-induced bioaccumulation, oxidative stress, tight junction proteins and immune-related signaling factors by Allium mongolicum Regel flavonoids in Ctenopharyngodon idella. Fish Shellfish Immunol. 106, 993–1003. doi: 10.1016/j.fsi.2020.09.005
Keywords: sodium propionate, tributyrin, hepatic lipid deposition, antioxidant capacity, hypoxic stress
Citation: Cui K, Zhang H, Yun B, Wang J, Qian X and Xue M (2024) Dietary supplementation with sodium propionate and tributyrin alleviated hepatic lipid deposition and improved the antioxidant capacity and hypoxic stress resistance of spotted seabass (Lateolabrax maculatus). Front. Mar. Sci. 11:1481672. doi: 10.3389/fmars.2024.1481672
Received: 16 August 2024; Accepted: 09 September 2024;
Published: 07 October 2024.
Edited by:
Lei Wang, Anhui Normal University, ChinaReviewed by:
Jiamin Li, Jiangxi Agricultural University, ChinaRantao Zuo, Dalian Ocean University, China
Qiang Ma, Chinese Academy of Fishery Sciences (CAFS), China
Kenneth Prudence Abasubong, University of South Bohemia, Czechia
Copyright © 2024 Cui, Zhang, Yun, Wang, Qian and Xue. This is an open-access article distributed under the terms of the Creative Commons Attribution License (CC BY). The use, distribution or reproduction in other forums is permitted, provided the original author(s) and the copyright owner(s) are credited and that the original publication in this journal is cited, in accordance with accepted academic practice. No use, distribution or reproduction is permitted which does not comply with these terms.
*Correspondence: Xueqiao Qian, qxq@haid.com.cn; Min Xue, xuemin@caas.cn