- 1School of Marine Sciences, Sun Yat-Sen University and Southern Marine Science and Engineering Guangdong Laboratory (Zhuhai), Zhuhai, China
- 2Department of Water Ecology and Environment, China Institute of Water Resources and Hydropower Research, Beijing, China
- 3Jiangxi Key Laboratory of Watershed Soil and Water Conservation, Jiangxi Academy of Water Science and Engineering, Nanchang, China
- 4State Key Laboratory of Environmental Criteria and Risk Assessment, Chinese Research Academy of Environmental Sciences, Beijing, China
Coastal biological invasions pose a wide-reaching threat to various ecosystems, affecting both vegetation and herbivores in native communities. Although herbivores often exert strong top-down control on vegetation, the impact of invasive species on consumers that strongly regulate native species in invaded ecosystems remains unclear. Therefore, through field surveys and feeding preference experiments, this study examined the effects of the invasive Spartina alterniflora Loisel. (hereafter, Spartina) on the native moth Laelia coenosa Hübner (hereafter, Laelia), a notorious pest that has been documented to cause significant damage to native Phragmites australis (Cav.) Trin. ex Steud. (hereafter, Phragmites) marshes in coastal wetlands of China. Field surveys showed that Laelia larvae were more abundant and had higher grazing rates in Phragmites than in Spartina marshes in summer, but these patterns reversed in autumn. Feeding experiments consistently showed that the dietary preference of Laelia larvae switched from Phragmites in summer to Spartina in autumn, likely because Spartina has a longer growing season and relatively higher nutritional quality than Phragmites in autumn. Thus, by providing shelters (i.e., dietary sources and habitats) during unfavorable seasons, Spartina invasions may facilitate this insect pest Laelia and potentially amplify its damage to native wetland vegetation. Our work reveals a novel, indirect mechanism of coastal invasion impacts and highlights the importance of incorporating seasonal variation in plant-herbivore interactions.
1 Introduction
Coastal biological invasions pose a significant threat to biodiversity and ecosystem function (Vila et al., 2011; Tekiela and Barney, 2015), as well as the economy and human health (Mack et al., 2000; Liao et al., 2008; Pejchar and Mooney, 2009; Ehrenfeld, 2010; Pysek et al., 2012). Recently, a growing body of literature has demonstrated that consumers may drive the success of invading species in colonizing new areas (Agrawal and Kotanen, 2003; Strauss et al., 2012; Li et al., 2014; Zhai et al., 2024). Invasive plants may facilitate changes in consumer pressure by offering new food sources or protective cover as refuges for native consumers (Dutra et al., 2011; Stewart et al., 2021; Peller and Altermatt, 2024). One of the predominant, and most extensively tested hypotheses linking herbivores and invasion processes is the Enemy Release Hypothesis (ERH). This hypothesis indicates that invasive species release from natural enemies (i.e. herbivores, pathogens) and gain a competitive advantage in their introduced ranges compared to their co-occurring native counterparts (Keane and Crawley, 2002). While a rapidly increasing number of studies have been conducted to test this hypothesis, the conclusions drawn from different studies appear to be mixed. Several empirical studies support this hypothesis (Keane and Crawley, 2002; Agrawal et al., 2005; Parker and Gilbert, 2007), however, numerous exceptions exist (Agrawal and Kotanen, 2003; Colautti et al., 2004; Parker and Hay, 2005; Chun et al., 2010). There is still no general pattern for explaining the interactions between invasive species and native herbivores and the ERH is a context-dependent hypothesis.
Therefore, understanding the impact of invasive plants on native consumers is important, as the indirect effects mediated by consumers can alter the dynamics of coexistence within native communities. Whether the invasive species are released from herbivores may depend on an array of physical and biological factors: environmental conditions (e.g. light, latitudinal gradients, the presence and absence of neighboring plants, etc.) (Bezemer et al., 2014; Biswas et al., 2015), plant conditions (e.g. the degree of invasiveness, residence time, range sizes spread, the density of the host plant, plant diversity, etc.) (Cappuccino and Carpenter, 2005; Pearse and Hipp, 2014; Biswas et al., 2015; Schultheis et al., 2015), herbivores characteristics (specialists and generalists’ native herbivores) (Harvey et al., 2010a; Fortuna et al., 2012), and herbivores’ natural enemies such as parasitoids and predators (Ode, 2006; Harvey et al., 2010b). However, comparisons of herbivores on invasive vegetation and natives overlooks the growing season variability of invasive plants and natives, especially in autumn. Autumn is a hugely important season in plant leaf senescence, insect migration and diapause in temperate and arctic ecosystems (Fridley, 2012; Gallinat et al., 2015). For instance, many invasive plants extend their growing season in comparison with natives in autumn; insects can add generations, delayed migration and diapause in warmer autumn (Bale et al., 2002; Fridley, 2012; Gallinat et al., 2015). In addition, some studies also suggested vegetation quality and dietary selection of insects have seasonal changes (Awmack and Leather, 2002; Bale et al., 2002). However, season variability has received less attention in invasion ecology.
Spartina alterniflora, one of the most aggressive invasive species in coastal wetlands around the world, has been well-studied for its impact on native ecosystems and the mechanisms behind these effects (Zhao et al., 2015; Ma et al., 2019; Sun et al., 2020). Spartina species not only occupies a space where the native salt marshes vegetation is absent, representing an “empty niche”, but also competes with native species (e.g. Phragmites australis, Suaeda salsa (L.) Pall. and Scirpus mariqueter Tang & F. T. Wang) for space in coastal wetlands (Tang et al., 2016; Ma et al., 2020; Zheng et al., 2022). Therefore, Spartina invasions significantly affect the physical structures of the native ecosystems and biotic communities. The invasion of Spartina in coastal marshes has been reported to cause distribution and dietary changes of herbivores, however, the results from past studies are mixed. Some studies suggested that herbivores prefer native vegetation to invasive Spartina in coastal wetlands: insect community (e.g. Calliptamus barbarus Costa, Protohermes costalis Walker and Rhopalosiphum rufiabdominalis S.) (Gao et al., 2006; Peng et al., 2006; Wu et al., 2009), crabs species (e.g. Helicana wuana Rathbun) (Zhang et al., 2023); but others advocated that herbivores prefer invasive Spartina to native species: crabs species (e.g. Sesarma dehaani H.Milne Edwards, Helice tientsinensis Rathbun) (Wang et al., 2008; Qin et al., 2010), nekton species (e.g. Chelon haematocheilus Temminck & Schlegel, Synechogobius ommaturus Richardson) (Quan et al., 2007); and some studies found that it is not distinctly different: snail species (e.g. Assiminea latericea H. Adams & A. Adams and Cerithidea largillierti R. A. Philippi) (Wang et al., 2014). Therefore, there is an ongoing debate as to the relative importance of invasive Spartina and native species to herbivores in coastal wetlands.
Here, we examined how the abundance and diet of the native insect Laelia coenosa are affected by the invasive Spartina from summer to autumn in coastal marshes through laboratory and field experiments. The salt marsh is located in the Yancheng National Nature Reserve of China and has been heavily invaded by Spartina. First, we monitored the density of Laelia coenosa larvae on Phragmites and Spartina from summer to autumn in high, middle and low marshes. Next, we compared the degree of leaf damaged by Laelia coenosa larvae for Phragmites and Spartina to examine whether the native insect Laelia coenosa prefers native Phragmites to invasive Spartina to support the Enemy Release Hypothesis. Finally, we explored whether the Enemy Release Hypothesis is constant in summer and autumn. Specifically, we assessed the larval feeding preference of Laelia coenosa on Spartina and native Phragmites in the laboratory in the different growing seasons.
2 Materials and methods
2.1 Study sites
The study was conducted at the Yancheng National Nature Reserve in Yancheng city of Jiangsu Province of China (32°20′–34°37′N, 119°29′–121°16′E) (Figure 1A), which has experienced the widest and most extensive areal coverage of Spartina in China (Zuo et al., 2012). The Yancheng National Nature Reserve lies in the transition belt between subtropical and warm-temperate zones with distinctive seasons and a rainy summer. The average annual temperature and precipitation are 11.4–13.8°C and 1000-1080 mm, with 54% of precipitation occurring in summer (June-August) (Liu et al., 2007; Zhou et al., 2009). The average seasonal precipitation of Yancheng city amounts to 545.56 mm in summer (June-August) and 203.03 mm in autumn (September-November) from 1960 to 2020, respectively (Qi et al., 2023). The average seasonal temperature of Yancheng city amounts to 25.8°C in summer (June-August) and 16.70°C in autumn (September-November) from 1981 to 2018, respectively (Sheng, 2019). The salt marsh is dominated by native Phragmites australis, Suaeda salsa and by exotic Spartina alterniflora. The distribution of vegetation succession from land to seaward is Phragmites australis, Suaeda salsa, Spartina alterniflora, and tidal flat (Zhang et al., 2013). The three species have some overlap in their distribution zones.
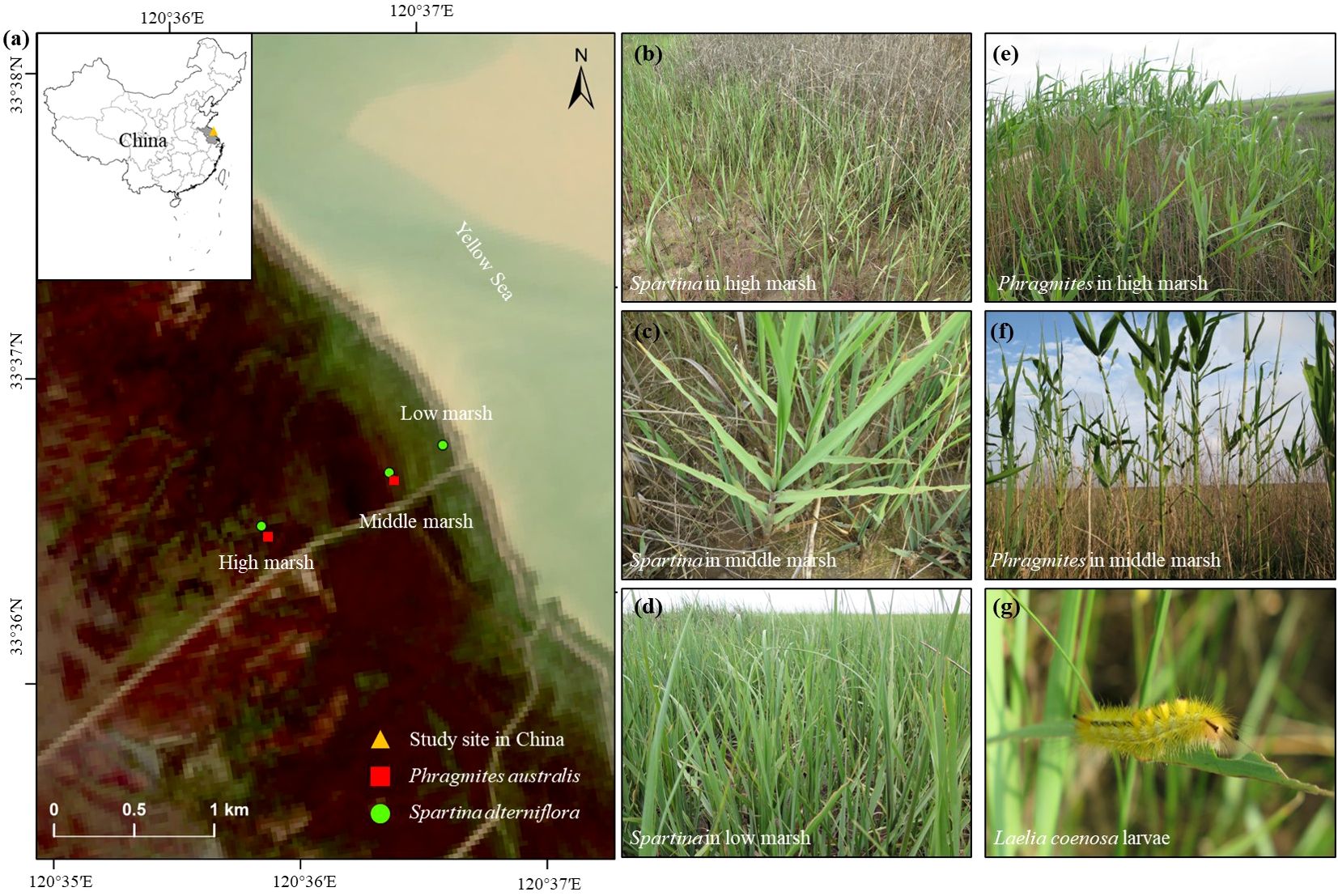
Figure 1. Location of the Yancheng National Nature Reserve in Jiangsu Province of China and sampling sites in the Yancheng National Nature Reserve with a true color remote sensing image on August 28, 2016 (A). Photographs of sample sites in summer: (B) the high marsh dominated by Spartina monoculture; (C) the middle marsh dominated by Spartina monoculture; (D) the low marsh dominated by Spartina monoculture; (E) the high marsh dominated by Phragmites monoculture and (F) the middle marsh dominated by Phragmites monoculture. Photograph of Laelia coenosa larvae in summer (G). Photographs were taken by Zezheng Liu.
2.2 Study species
Laelia coenosa, a generalist herbivorous insect, is a moth of the Lepidoptera order, Lymantriidae family found in North Africa, southern and central Europe, through Russia and eastern Asia up to Japan (Zeng et al., 1988). It is common and widespread in China, and widely distributed in inland (e.g. Hunan, Hubei, Anhui, and Jiangxi Province) and coastal (e.g. Hebei, Shandong, Jiangsu, and Shanghai Province) zones (Zeng et al., 1988; Xia et al., 1993; Ma et al., 2015). It generally produces two or three generations per year (Supplementary Table S1) and each generation has a four-stage life cycle: egg, larva, pupa and adult (Figure 2). In our study sites, the larvae of Laelia coenosa occurred during two periods: from mid-April to mid-June for the first generation, and from mid-June to late September for the second generation (Supplementary Table S1) (Xia et al., 1993). Hibernation takes place as an egg or larvae between the middle of October and early March of next year (Zeng et al., 1988). Laelia coenosa has long larvae life stages (Figure 1G), which primarily feed on a wide range of host plants including Poaceae and some Cyperaceae, especially Phragmites and Miscanthus, and 30%∼40% production of reeds lost mainly by late-instar larvae (Zeng et al., 1988).
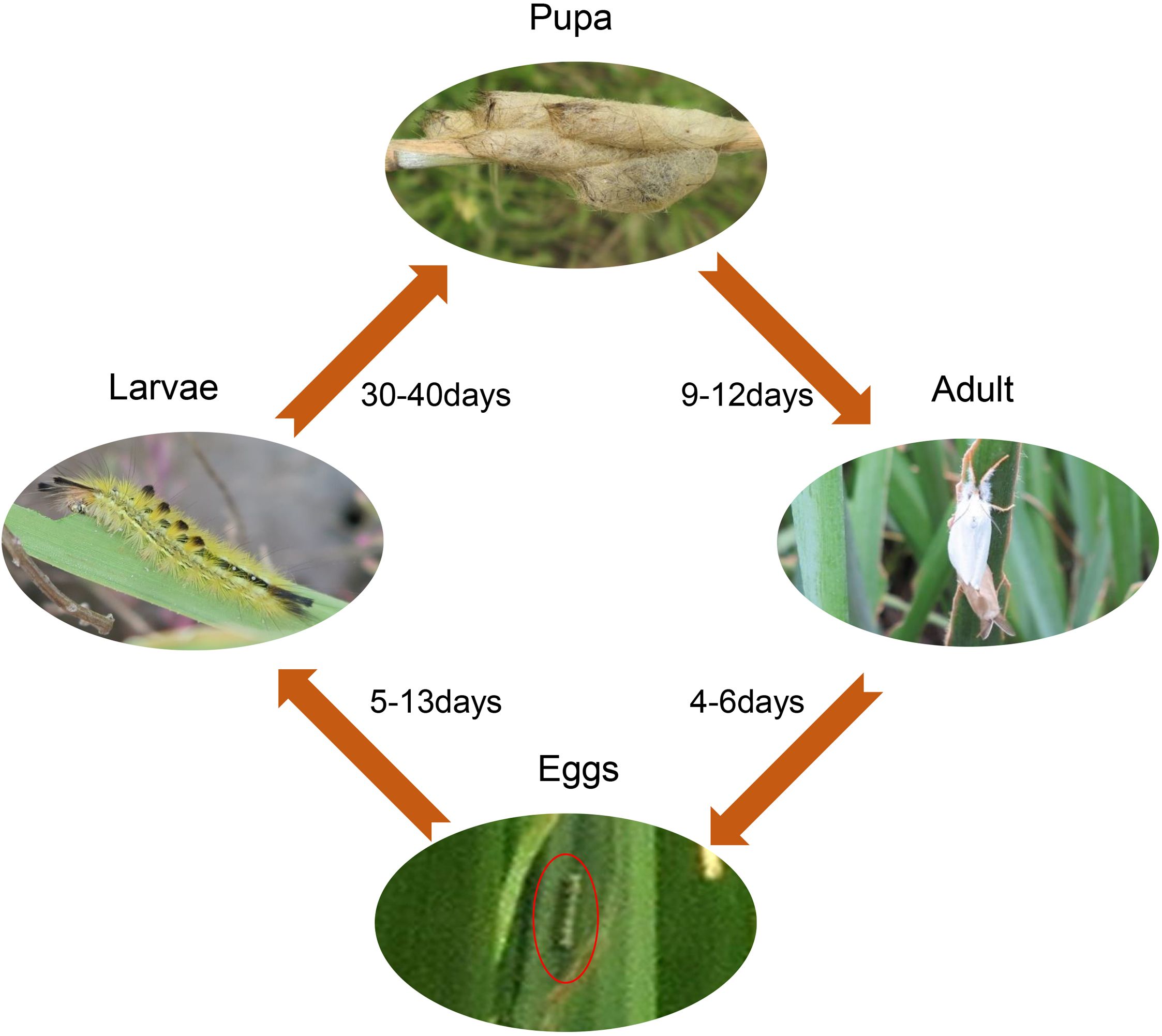
Figure 2. Basic life cycle model of Laelia coenosa. Date from Zeng et al., 1988. Photographs were taken by Zezheng Liu.
2.3 Sampling and analysis
To examine the spatial-temporal distribution of native insect Laelia coenosa, five distinct zones (habitats) were delimited based on elevation and vegetation type in June (summer) and September (autumn): (1) the high marsh dominated by Phragmites monoculture; (2) the high marsh dominated by Spartina monoculture; (3) the middle marsh dominated by Phragmites monoculture; (4) the middle marsh dominated by Spartina monoculture; (5) the low marsh dominated by Spartina monoculture (Figures 1B–F). In each sampling habitat at each sampling season, 0.5×0.5 m quadrat was randomly placed for sampling. The sample sizes are 16 except those in Phragmites marsh whose sample sizes are 8. From the quadrat, numbers of native insect Laelia coenosa were counted, and leaves of five plants randomly selected were collected. In summer, all leaves of selected plants were collected, whereas only the up leaves were collected in autumn because the other leaves obviously were not consumed by the second-generation larvae in autumn, but consumed by the first generation in summer. Samples were stored on ice during transportation to the laboratory.
To assess the degree of leaf damage, the area of leaf damaged and percentage of leaf area damaged were calculated. The area of leaf damaged was calculated for each leaf as “complete area of damaged leaves” minus “remaining leaf area”. The complete area of damaged leaves was estimated separately for Phragmites and Spartina by using regression equations between leaf area and length × width from 115 and 59 undamaged leaves of Phragmites and Spartina from the Yancheng National Nature Reserve salt marshes (Figure 3). When leaves width and length are not available because leaves are severely damaged, we used “average complete area of damaged leaves” minus “remaining leaf area” as the area of leaf damaged. The average complete area of damaged leaves is the mean value of undamaged leaf area in the same habitat as damaged area calculated. The percentage of leaf area damaged was calculated as “area of leaf damaged” divided by “complete area of damaged leaves” or “average complete area of damaged leaves”. The length, width, and area of leaves were measured with a Yaxin-1241 leaf area meter. In autumn, we also calculated the percentage of leaf number damaged between Spartina-invaded and non-invaded Phragmites habitats by dividing the number of damaged leaves by the total number of leaves.
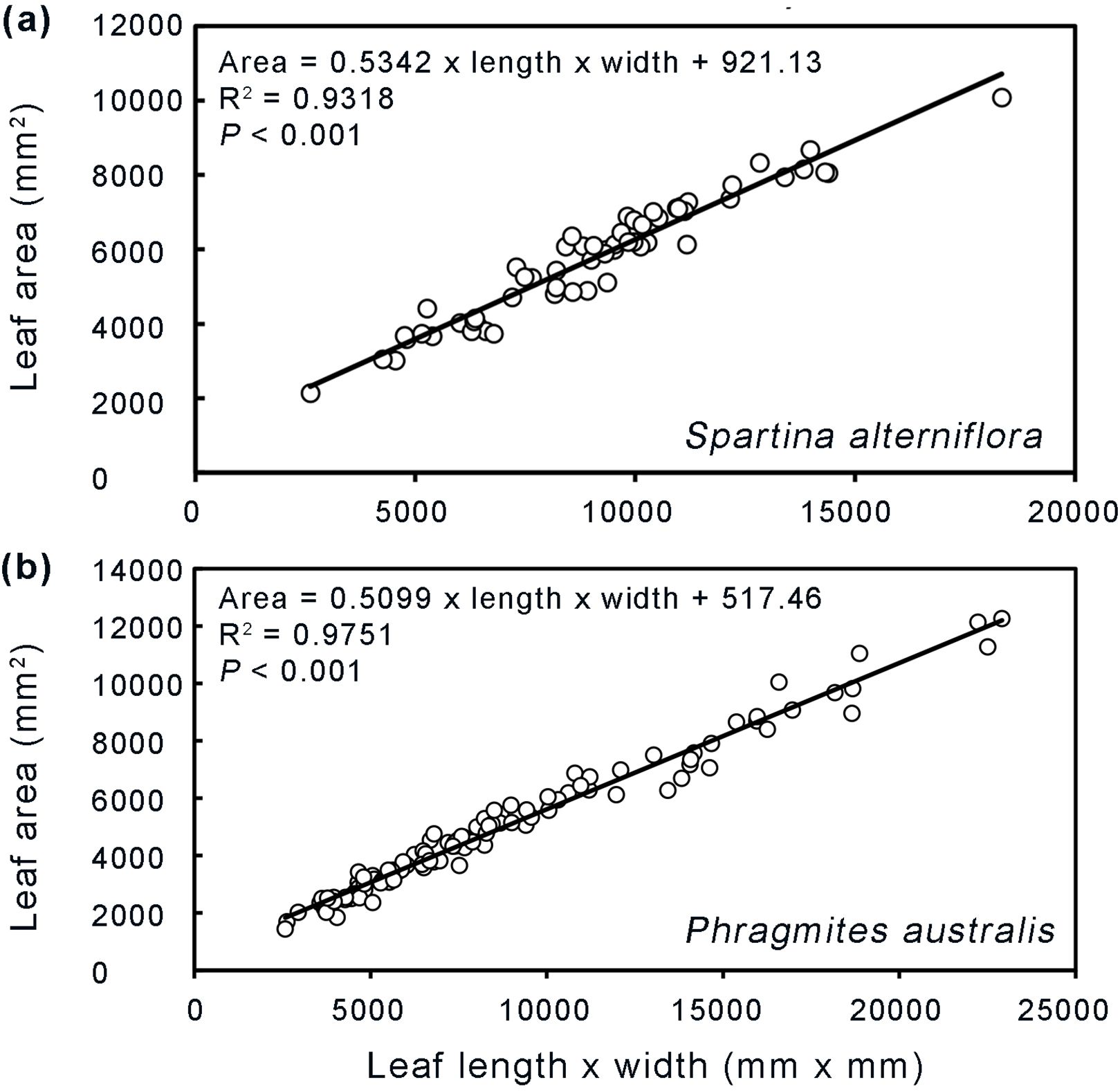
Figure 3. Relationship between leaf area and length×width for Spartina alterniflora (A) and Phragmites australis (B).
2.4 Dietary preferences experiment
The two-choice experiment was designed to investigate the feeding preference of Laelia coenosa between native Phragmites and exotic Spartina. One larva of Laelia coenosa and two leaves of each plant species were placed in a loosely capped 1500 mL wide-mouthed plastic bottle, covering the top with a gauze net in case larvae escaped. To keep the experiment systems moist, pure water (10-20mL) was added to the jar at a depth of approximately 0.5 cm. All the plastic jars were subjected to 24 hours’ nature photoperiod in June and September at field temperature. All larvae of Laelia coenosa were collected from the same area of the middle marsh zone, and the length and color were approximately consistent. The mean length of Laelia coenosa was 37.18 ± 4.96 mm and 30.1 ± 5.86 mm in June and September respectively (Supplementary Figure S1). While collecting the larvae in June and September, we also collected the top leaves of t Spartina alterniflora and Phragmites australis and trimmed them to a length of 20 cm. The feeding experiment was used with twelve replicates, however, only eight replicates data are available, due to the death of Laelia coenosa. The leaf area of damaged was calculated as the leaf area before the experiment minus the remaining leaf area after experiment. The percentage of damaged was calculated as the leaf area of damaged divided by leaf area before the experiment. The leaf area was measured before and after feeding trials with a Yaxin-1241 leaf area meter.
2.5 Statistical analysis
The density of Laelia coenosa larvae and degree of leaf area damaged for different habitat treatments at the same season were subjected to one-way ANOVAs. Laelia coenosa feeding preferences were examined using paired t-tests (two-sided). Before statistical analyses, all data were checked for normality and were log-transformed to improve the normality distribution. The results are represented as means and SE, and the level of statistical significance was set at P < 0.05. All statistical analyses were performed with the statistical package SPSS NLN, 15.0 (SPSS Inc., Chicago, USA).
3 Results
3.1 Spatial-temporal distribution pattern of Laelia larvae
In total, 582 larvae of Laelia coenosa were surveyed in sampling sites in this study. Distribution pattern of larvae at all five sampling plots in two sampling seasons is shown in Figure 4. Laelia coenosa showed significantly different along the sampling plots among the two growing seasons. Generally, the density of larvae in summer was higher than in autumn in five sampling sites (P < 0.01). We surveyed 523 larvae of Laelia coenosa in summer, while the number of larvae in autumn was 59 in all sampling sites. In summer (the first generation of Laelia coenosa), Laelia coenosa exhibited the highest level in Phragmites middle marsh, reaching a density of 23.38 ± 6.22 ind./0.25 m2. The lowest density also appeared in the middle marsh, however, in Spartina habitat with a density of 3.31 ± 0.45 ind./0.25 m2. Compared to the middle marsh, the density of the native insect Laelia coenosa did not significantly differ among the two habitats in the high marsh, with the density of 6.00 ± 0.65 and 6.25 ± 0.87 ind./0.25 m2 in Phragmites and Spartina habitats, respectively (Figure 4). The density of larvae in Spartina low marsh is second to that in Phragmites middle marsh, with a density of 8.44 ± 1.34 ind./0.25 m2 (Figure 4). However, Laelia coenosa preferred Spartina marsh, and was absent from Phragmites habitat in autumn (the second generation of Laelia coenosa) (Figure 4). Laelia coenosa exhibited the highest level in Spartina low marsh, reaching a density of 2.50 ± 0.30 ind./0.25 m2. Therefore, season and vegetation species significantly affected the abundance of the native insect Laelia coenosa.
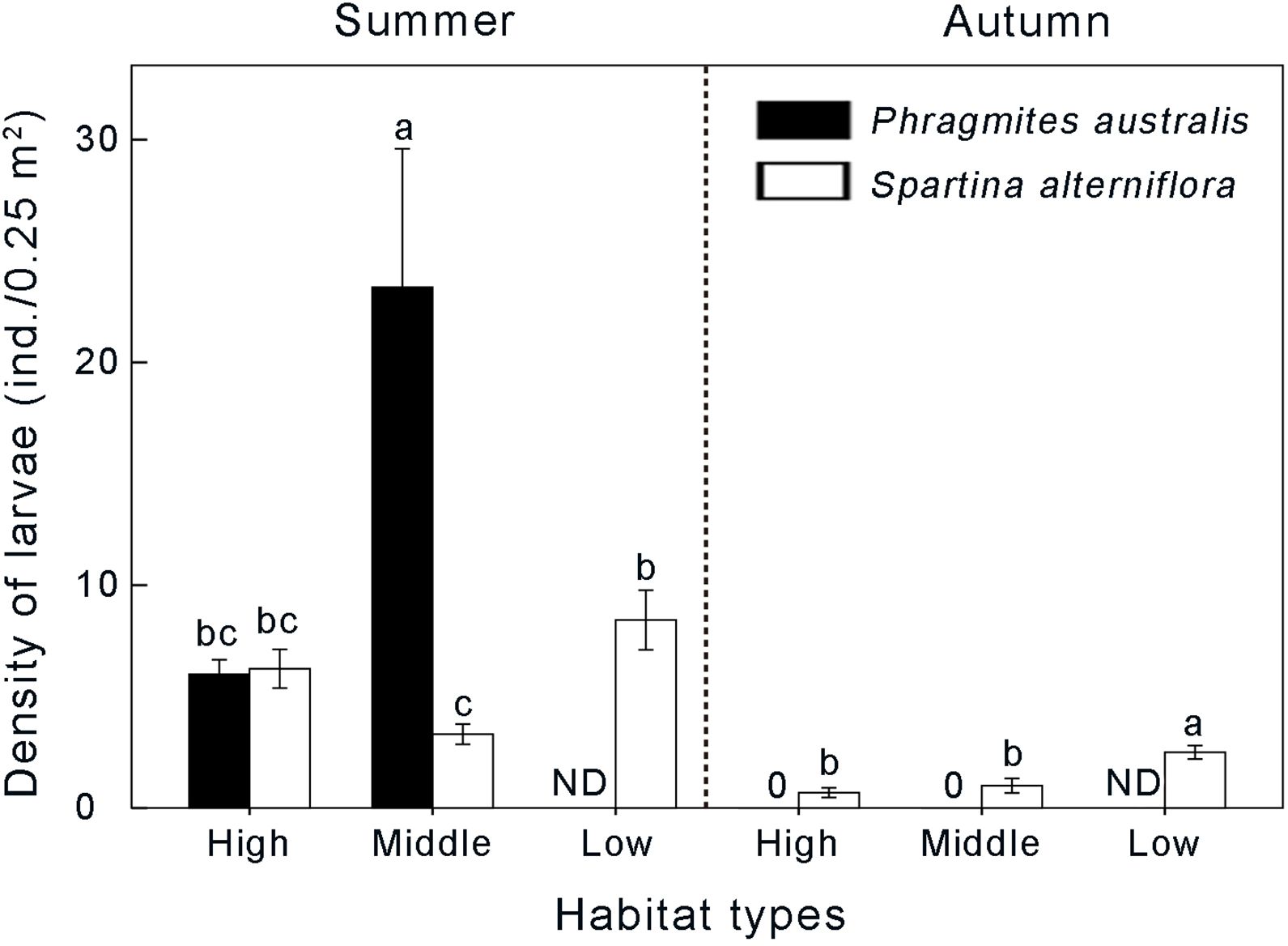
Figure 4. Differences in density of native insect Laelia coenosa between Spartina-invaded and non-invaded Phragmites habitats in summer and autumn. Data are shown as means ± SE. ND indicates no data. Different letters indicate significant differences (P < 0.05).
3.2 Degree of leaf damage for Phragmites and Spartina
Similar to the spatial-temporal distribution pattern of larvae, the degree of leaf damage also exhibited significant differences along the sampling habitats between the two growing seasons (Figure 5). In summer, leaves damaged by the first generation of Laelia coenosa showed the highest average extent of damage in the middle marsh area of the Phragmites community (72.41 ± 2.11%) (Figure 5B), with a damaged area of 5810.28 ± 173.91 mm2 for each leaf (Figure 5A). In the middle marsh, the area of leaf damaged and percentage of leaf area damaged in Phragmites community were significantly higher than in Spartina community (P < 0.05) (Figure 5). In the high marsh, however, there was no significant difference in the area of leaf damaged and percentage of leaf area damaged between Phragmites community and Spartina community (P > 0.05) (Figure 5). The area of leaf damaged in Spartina community did not significantly differ in the high and low marshes, with 1778.66 ± 86.96 mm2 and 1138.34 ± 134.39 mm2 for each leaf, respectively (P > 0.05) (Figure 5). In autumn, the degree of leaf damage by the second generation of Laelia coenosa in the middle marsh area of the Spartina community reached the highest level (1478.26 ± 189.72 mm2), which was significantly higher in the high and low marshes (P < 0.05) (Figure 5). However, native Phragmites didn’t provide food for the native insect Laelia coenosa in autumn.
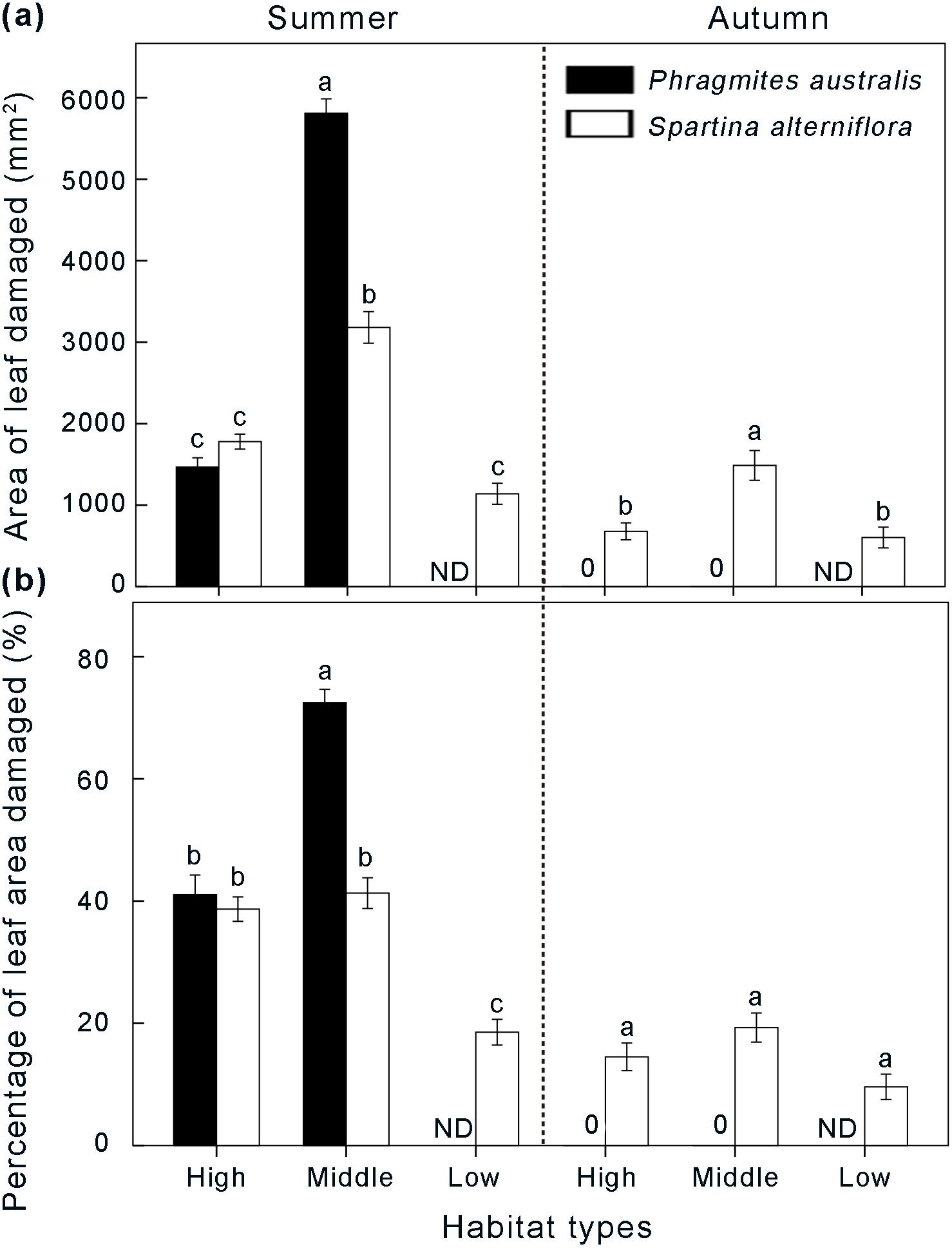
Figure 5. Differences in area of leaf damaged (A) and percentage of leaf area damaged (B) between Spartina-invaded and non-invaded Phragmites habitats in summer and autumn. Data are shown as means ± SE. ND indicates no data. Different letters indicate significant differences (P < 0.05).
In autumn, more than 60% of the leaves were affected by leaves of Laelia coenosa (Figure 6). In general, the proportion of damaged Spartina leaves was significantly higher than that of the Phragmites community (P < 0.001). The proportions of damaged Spartina leaves were more than 90% at different elevation levels, with 90.63 ± 1.90%, 97.98 ± 0.95% and 92.16 ± 1.89% in high, middle and low marshes, respectively. The proportions of damaged Phragmites leaves were significantly higher in the middle elevation marsh (86.58 ± 2.28%) than in the high elevation marsh (65.97 ± 2.39%) (P < 0.001) (Figure 6).
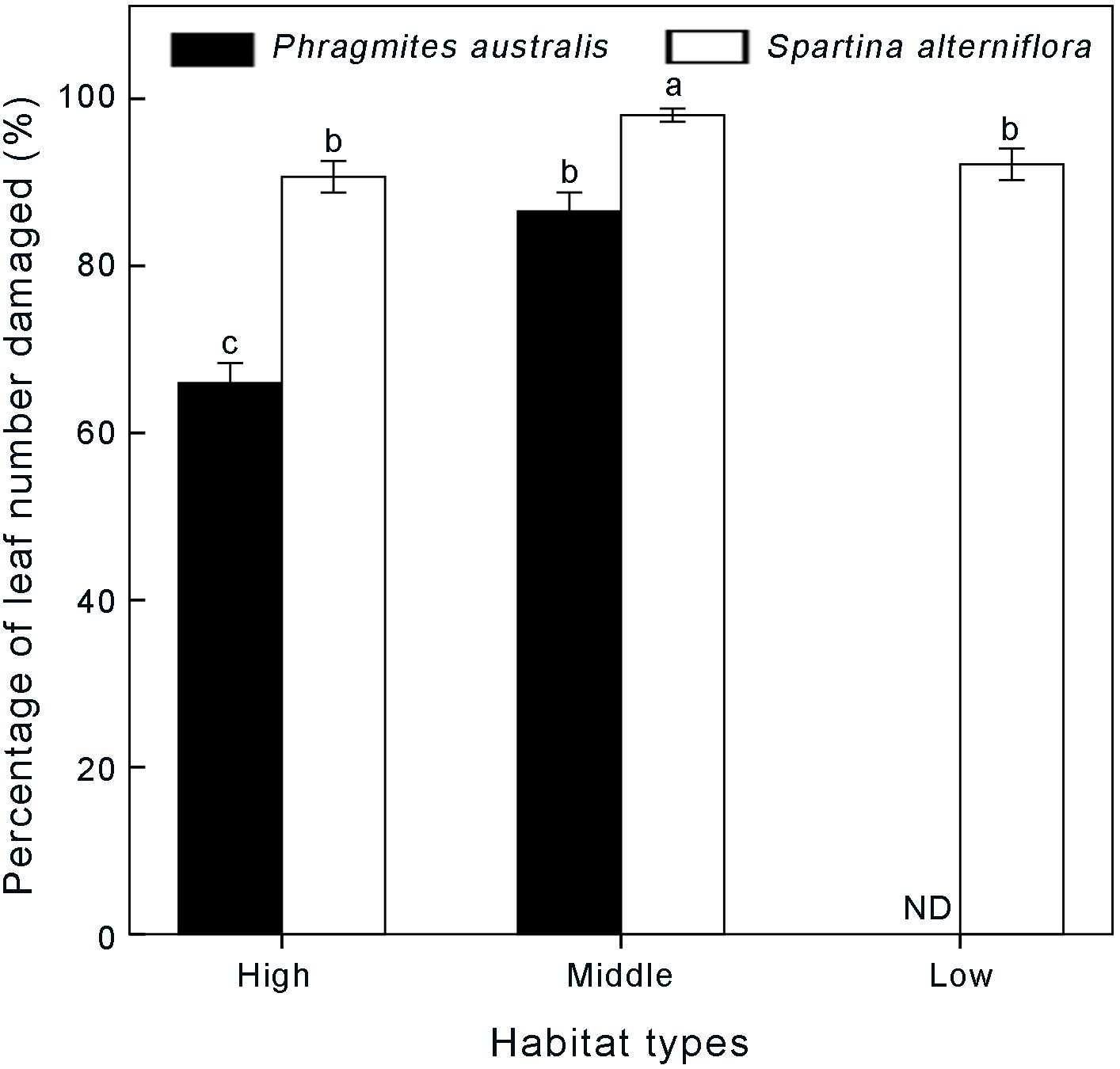
Figure 6. Differences in percentage of leaf number damaged between Spartina-invaded and non-invaded Phragmites habitats in autumn. Data are shown as means ± SE. ND indicates no data. Different letters indicate significant differences (P < 0.05).
3.3 Feeding preference for Phragmites and Spartina
In the lab feeding trial, the amount of Phragmites consumed by Laelia coenosa was significantly higher than Spartina in summer, whereas Laelia coenosa preferred Spartina in autumn (Figure 7). In summer, the leaf area of Phragmites consumed by larvae was 2433.88 ± 410.10 mm2, more than six times higher than that of Spartina (378.9 ± 106.98 mm2, P < 0.001) (Figure 7A). The percentage of Phragmites consumed by Laelia coenosa was also higher than that of Spartina (39.48 ± 6.72% vs. 10.57 ± 3.01%, P < 0.001) (Figure 7B). In autumn, however, the leaf area of Phragmites consumed by larvae was only a quarter as high as that of Spartina (187.22 ± 133.73 mm2 vs. 851.41 ± 218.43 mm2, P < 0.001) (Figure 7A). Therefore, we could predict a shift in feeding preference by Laelia coenosa depending on the growing seasons.
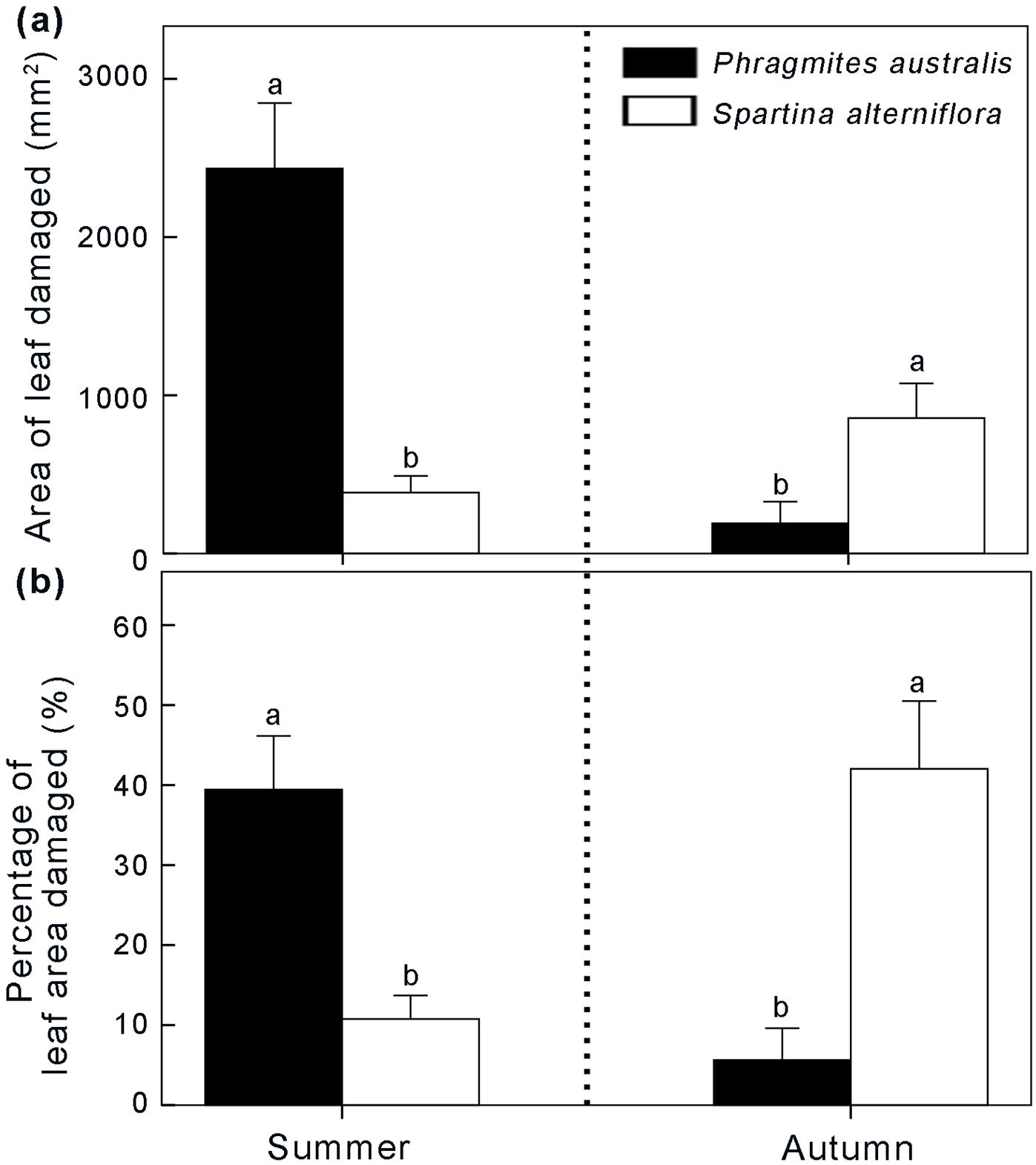
Figure 7. Feeding preference of Laelia coenosa for Phragmites and Spartina in summer and autumn. Data are shown as means ± SE. Different letters indicate significant differences (P < 0.05).
4 Discussion
This study revealed the effects of an invasive Spartina on insect species and related the changes in distributions and dietary breadth across two seasons in coastal wetlands. In our survey, the percentage of damaged leaves across the five sampling plots exceeded 60%, and in some cases, approached nearly 100% (Figure 6). This may significantly underestimate the true extent of leaf damage by herbivores, as it fails to include leaves that were entirely eaten or abscised prematurely due to heavy damage, particularly in Phragmites marshes. Our findings also indicated that Laelia coenosa larvae preferred Phragmites habitats over the invasive Spartina communities in summer, while the preference was reversed in autumn (Figure 4). The characteristics of leaf damaged and the spatial-temporal distribution pattern of the native insect Laelia coenosa exhibited similar patterns of change (Figure 5). Feeding experiments consistently showed that the dietary preference of Laelia larvae switched from Phragmites australis in summer to Spartina alterniflora in autumn (Figure 7). From these findings, we consistently concluded that Laelia coenosa has recently expanded its habitat range, now inhabiting not only native Phragmites communities but also invasive Spartina communities in coastal wetlands.
Invasive plants can affect the spatial and temporal dynamics of native insect populations by disrupting a wide range of trophic interactions (Harvey et al., 2010a; Bezemer et al., 2014). Previous studies have consistently concluded that food source is a primary factor affecting the distribution and survival of herbivorous insects. For example, the invasive Phragmites australis offers a more favorable feeding habitat for the dominant grazers (e.g. Physella gyrina Say and Fossaria (Bakerlymnaea) bulimoides group), increasing their density along the southern shoreline of Lake Erie in the USA (Holomuzki and Klarer, 2010). The introduction of Spartina in salt marshes has also led to dietary shifts in crab species such as Helice tientsinensis and Chiromantes dehaani H. Milne Edwards (Qin et al., 2010). The non-native bryozoan Membranipora membranacea can serve as a new food source for the native nudibranch Onchidoris muricata in the summer and fall in the Gulf of Maine of the USA (Pratt and Grason, 2007). Additionally, invasive predators can disrupt food webs by forcing native predators to feed on suboptimal food sources, and reorganize the low-trophic-level communities, thereby causing significant disruption to native food web structures (Wainright et al., 2021). Thus, invasive species have the potential to alter the resource utilization patterns of native species, leading to both direct and indirect modifications in food web dynamics.
A wide variety of plant traits, such as carbon and nitrogen content, defensive metabolites, morphological characteristics, and phenological traits, are known to significantly influence the preferences for host plants and the associated distribution of herbivorous insects (Awmack and Leather, 2002; Salgado and Saastamoinen, 2019; Bovay et al., 2024). In general, larvae of the Lepidoptera order prefer leaves with high nitrogen and water content and low toughness (Peng et al., 2006; Ma et al., 2015). Native herbivores tend to prefer native plants, because exotic species may not provide a suitable diet (Tallamy and Shropshire, 2009). Previous studies have suggested native insect species (Laelia coenosa) prefer native Spartina in the USA to invasive Spartina in China through greenhouse experiments, due to differences in leaf nitrogen content and toughness rather than volatile compounds (Ma et al., 2015). Some studies suggested that the leaf total nitrogen of Spartina was significantly lower than that of Phragmites in summer in coastal wetlands (Jiang et al., 2009; Guo et al., 2023). In addition, some previous studies indicated that leaves of Spartina are more thickness and toughness than those of Phragmites in coastal wetlands (Hendricks et al., 2011; Guo et al., 2023). Spartina species, known for their salt-secreting capability, have leaves containing salt particles that can reduce the palatability of their diet for herbivorous insects (Smart and Barko, 1978; Smit et al., 2024). Therefore, insects Laelia coenosa tend to prefer feeding on native Phragmites over exotic species Spartina during the summer when food is abundant.
Furthermore, Spartina species have a longer growing season compared to the native Phragmites in China’s salt marshes (Jiang et al., 2009; Wang et al., 2006). The growing season lengths for Spartina and Phragmites were 270 days and 220 days in the Yangtze Estuary of China, respectively (Liao et al., 2007). In autumn, Spartina exhibits higher leaf total nitrogen values and plant tissue water content than Phragmites (Wang et al., 2008; Jiang et al., 2009), which tends to make it a more attractive food source for insects. In September, total plant C and N stock of Spartina stands in the Yangtze Estuary of China were 3.83 kg m−2 and 57.21 g m−2, respectively, while the values for Phragmites stands were significantly lower, at 0.89 kg m−2 and 15.86 g m−2, respectively (Liao et al., 2007). Consequently, the invasive Spartina creates a more palatable diet for insects, enhancing their density and effectively buffering against food deprivation stress in autumn in coastal wetlands. Similarly, many woody invasive plants in North America retained their leaves later in the autumn than native plants, resulting in extended leaf phenology. The extended leaf phenology of invasive species enhances their competitive advantage over native species by providing increased access to understory light (O’Connell and Savage, 2020). Therefore, there is a seasonal shift in insect habitat selection from Phragmites in summer to Spartina in autumn in coastal wetlands.
The invasive Spartina, characterized by its extended growing season, offers a dietary source and habitat for herbivorous insects in autumn. This additional resource may support an extra generation of insects in the fall and be advantageous for their overwintering, resulting in a shorter overwintering period and a higher survival rate of insects in spring. Consequently, this could lead to an increase in both the frequency and intensity of insect outbreaks in coastal wetlands. In turn, these outbreaks may facilitate further invasion of Spartina by causing significant damage to native species, as native insects could contribute to the decline of native species that otherwise might have competed with Spartina. Thus, the differences in damaging ability between the invasive and native plants by the native herbivorous may hinder the conservation and restoration of the invaded ecosystem. Looking ahead, additional research is necessary to validate these assumptions and to achieve a more comprehensive understanding of the impact of Spartina invasion on various insect behaviors, including oviposition preferences and the selection of overwintering sites.
5 Conclusions
In conclusion, our results suggest that the native insect Laelia coenosa preferentially consumes native Phragmites over invasive Spartina in summer, which may contribute to the decline of native Phragmites. Thus, the interaction between invasive Spartina and native insects appears to support the Enemy Release Hypothesis in summer. However, this pattern is not consistent in autumn. The invasive Spartina offers a dietary source and habitat for herbivorous insects Laelia coenosa in autumn with its extended growing season. These differences are anticipated to alter the trophic interactions within the invaded ecosystem, potentially aiding in the further spread of the exotic Spartina and hindering the reestablishment of native Phragmites populations. This finding helps explain the mixed results often observed in hypothesis tests and enhances our understanding of the mechanisms behind coastal invasions. Such insights will aid in understanding and predicting the success of invasive species in natural ecosystems.
Data availability statement
The raw data supporting the conclusions of this article will be made available by the authors, without undue reservation.
Author contributions
TX: Conceptualization, Data curation, Methodology, Software, Visualization, Writing – original draft, Writing – review & editing. XM: Writing – original draft, Writing – review & editing. YL: Supervision, Writing – original draft, Writing – review & editing. HX: Writing – original draft, Writing – review & editing. SZ: Writing – original draft, Writing – review & editing. ZL: Conceptualization, Formal analysis, Funding acquisition, Investigation, Supervision, Writing – original draft, Writing – review & editing.
Funding
The author(s) declare financial support was received for the research, authorship, and/or publication of this article. This work is financially supported by the National Natural Science Foundation of China (42306187, 42176202), the Guangdong Provincial Department of Science and Technology, China (2024B1515020066, 2019ZT08G090), the Innovation Group Project of Southern Marine Science and Engineering Guangdong Laboratory, China (Zhuhai; Grant no. 311021004).
Conflict of interest
The authors declare that the research was conducted in the absence of any commercial or financial relationships that could be construed as a potential conflict of interest.
Publisher’s note
All claims expressed in this article are solely those of the authors and do not necessarily represent those of their affiliated organizations, or those of the publisher, the editors and the reviewers. Any product that may be evaluated in this article, or claim that may be made by its manufacturer, is not guaranteed or endorsed by the publisher.
Supplementary material
The Supplementary Material for this article can be found online at: https://www.frontiersin.org/articles/10.3389/fmars.2024.1478599/full#supplementary-material
References
Agrawal A. A., Kotanen P. M. (2003). Herbivores and the success of exotic plants: a phylogenetically controlled experiment. Ecol. Lett. 6, 712–715. doi: 10.1046/j.1461-0248.2003.00498.x
Agrawal A. A., Kotanen P. M., Mitchell C. E., Power A. G., Godsoe W., Klironomos J. (2005). Enemy release? An experiment with congeneric plant pairs and diverse above- and belowground enemies. Ecology 86, 2979–2989. doi: 10.1890/05-0219
Awmack C. S., Leather S. R. (2002). Host plant quality and fecundity in herbivorous insects. Annu. Rev. Entomology 47, 817–844. doi: 10.1146/annurev.ento.47.091201.145300
Bale J. S., Masters G. J., Hodkinson I. D., Awmack C., Bezemer T. M., Brown V. K., et al. (2002). Herbivory in global climate change research: direct effects of rising temperature on insect herbivores. Glob. Change Biol. 8, 1–16. doi: 10.1046/j.1365-2486.2002.00451.x
Bezemer T. M., Harvey J. A., Cronin J. T. (2014). Response of native insect communities to invasive plants. Ann. Rev. Entomol. 59, 119. doi: 10.1146/annurev-ento-011613-162104
Biswas S. R., Kotanen P. M., Kambo D., Wagner H. H. (2015). Context-dependent patterns, determinants and demographic consequences of herbivory in an invasive species. Biol. Invasions 17, 165–178. doi: 10.1007/s10530-014-0715-0
Bovay B., Descombes P., Chittaro Y., Glauser G., Nomoto H., Rasmann S. (2024). Adapting to change: Exploring the consequences of climate-induced host plant shifts in two specialist Lepidoptera species. Ecol. Evol. 14, e11596. doi: 10.1002/ece3.11596
Cappuccino N., Carpenter D. (2005). Invasive exotic plants suffer less herbivory than non-invasive exotic plants. Biol. Lett. 1, 435–438. doi: 10.1098/rsbl.2005.0341
Chun Y. J., van Kleunen M., Dawson W. (2010). The role of enemy release, tolerance and resistance in plant invasions: linking damage to performance. Ecol. Lett. 13, 937–946. doi: 10.1111/j.1461-0248.2010.01498.x
Colautti R. I., Ricciardi A., Grigorovich I. A., MacIsaac H. J. (2004). Is invasion success explained by the enemy release hypothesis? Ecol. Lett. 7, 721–733. doi: 10.1111/j.1461-0248.2004.00616.x
Dutra H. P., Barnett K., Reinhardt J. R., Marquis R. J., Orrock J. L. (2011). Invasive plant species alters consumer behavior by providing refuge from predation. Oecologia 166, 649–657. doi: 10.1007/s00442-010-1895-7
Ehrenfeld J. G. (2010). Ecosystem consequences of biological invasions. Annu. Rev. ecology evolution systematics 41, 59–80. doi: 10.1146/annurev-ecolsys-102209-144650
Fortuna T. M., Vet L. E. M., Harvey J. A. (2012). Effects of an invasive plant on the performance of two parasitoids with different host exploitation strategies. Biol. Control 62, 213–220. doi: 10.1016/j.biocontrol.2012.05.003
Fridley J. D. (2012). Extended leaf phenology and the autumn niche in deciduous forest invasions. Nature 485, 105–359. doi: 10.1038/nature11056
Gallinat A. S., Primack R. B., Wagner D. L. (2015). Autumn, the neglected season in climate change research. Trends Ecol. Evol. 30, 169–176. doi: 10.1016/j.tree.2015.01.004
Gao H., Peng X., Li B., Wu Q., Dong H. (2006). Effects of the invasive plant Spartina alterniflora on insect diversity in Jiuduansha wetlands in the Yangtze River Estuary. Chin. Biodiversity 14, 400–409. doi: 10.1360/biodiv.060025
Guo Y., Zhang Y., Wu J., Richards C. L., Bossdorf O., Li B., et al. (2023). Geographic variation of litter chemistry and palatability in an invasive plant versus its native competitor. J. Biogeography 50, 1139–1150. doi: 10.1111/jbi.14604
Harvey J. A., Biere A., Fortuna T., Vet L. E. M., Engelkes T., Morrien E., et al. (2010a). Ecological fits, mis-fits and lotteries involving insect herbivores on the invasive plant, Bunias orientalis. Biol. Invasions 12, 3045–3059. doi: 10.1007/s10530-010-9696-9
Harvey J. A., Bukovinszky T., van der Putten W. H. (2010b). Interactions between invasive plants and insect herbivores: A plea for a multitrophic perspective. Biol. Conserv. 143, 2251–2259. doi: 10.1016/j.biocon.2010.03.004
Hendricks L. G., Mossop H. E., Kicklighter C. E. (2011). Palatability and Chemical Defense of Phragmites australis to the Marsh Periwinkle Snail Littoraria irrorata. J. Chem. Ecol. 37, 838–845. doi: 10.1007/s10886-011-9990-8
Holomuzki J. R., Klarer D. M. (2010). Invasive reed effects on benthic community structure in Lake Erie coastal marshes. Wetlands Ecol. Manage. 18, 219–231. doi: 10.1007/s11273-009-9161-7
Jiang L., Luo Y., Chen J., Li B. (2009). Ecophysiological characteristics of invasive Spartina alterniflora and native species in salt marshes of Yangtze River estuary, China. Estuar. Coast. Shelf Sci. 81, 74–82. doi: 10.1016/j.ecss.2008.09.018
Keane R. M., Crawley M. J. (2002). Exotic plant invasions and the enemy release hypothesis. Trends Ecol. Evol. 17, 164–170. doi: 10.1016/S0169-5347(02)02499-0
Li H., Zhang X., Zheng R., Li X., Elmer W. H., Wolfe L. M., et al. (2014). Indirect effects of non-native Spartina alterniflora and its fungal pathogen (Fusarium palustre) on native saltmarsh plants in China. J. Ecol. 102, 1112–1119. doi: 10.1111/1365-2745.12285
Liao C., Luo Y., Jiang L., Zhou X., Wu X., Fang C., et al. (2007). Invasion of Spartina alterniflora enhanced ecosystem carbon and nitrogen stocks in the Yangtze Estuary, China. Ecosystems 10, 1351–1361. doi: 10.1007/s10021-007-9103-2
Liao C. Z., Peng R. H., Luo Y. Q., Zhou X. H., Wu X. W., Fang C. M., et al. (2008). Altered ecosystem carbon and nitrogen cycles by plant invasion: a meta-analysis. New Phytol. 177, 706–714. doi: 10.1111/j.1469-8137.2007.02290.x
Liu J., Zhou H., Qin P., Zhou J. (2007). Effects of spartina alterniflora salt marshes on organic carbon acquisition in intertidal zones of Jiangsu province, China. Ecolo. Eng. 30, 240–249. doi: 10.1016/j.ecoleng.2007.01.010
Ma D., Ju R., Li B. (2015). Preference of Laelia coenosa for native and introduced populations of invasive Spartina alterniflora. Biodiversity Sci. 23, 101–108. doi: 10.17520/biods.2014156
Ma X., Qiu D., Wang F., Jiang X., Sui H., Liu Z., et al. (2020). Tolerance between non-resource stress and an invader determines competition intensity and importance in an invaded estuary. Sci. Total Environ. 724, 138225. doi: 10.1016/j.scitotenv.2020.138225
Ma X., Yan J., Wang F., Qiu D., Jiang X., Liu Z., et al. (2019). Trait and density responses of Spartina alterniflora to inundation in the Yellow River Delta, China. Mar. pollut. Bull. 146, 857–864. doi: 10.1016/j.marpolbul.2019.07.022
Mack R. N., Simberloff D., Lonsdale W. M., Evans H., Clout M., Bazzaz F. A. (2000). Biotic invasions: causes, epidemiology, global consequences, and control. Ecol. Appl. 10, 689–710. doi: 10.1890/1051-0761(2000)010[0689:BICEGC]2.0.CO;2
O’Connell E., Savage J. (2020). Extended leaf phenology has limited benefits for invasive species growing at northern latitudes. Biol. Invasions 22, 2957–2974. doi: 10.1007/s10530-020-02301-w
Ode P. J. (2006). Plant chemistry and natural enemy fitness: Effects on herbivore and natural enemy interactions. Annu. Rev. Entomology 51, 163–185. doi: 10.1146/annurev.ento.51.110104.151110
Parker I. M., Gilbert G. S. (2007). When there is no escape: The effects of natural enemies on native, invasive, and noninvasive plants. Ecology 88, 1210–1224. doi: 10.1890/06-1377
Parker J. D., Hay M. E. (2005). Biotic resistance to plant invasions? Native herbivores prefer non-native plants. Ecol. Lett. 8, 959–967. doi: 10.1111/j.1461-0248.2005.00799.x
Pearse I. S., Hipp A. L. (2014). Native plant diversity increases herbivory to non-natives. Proc. R. Soc. B: Biol. Sci. 281(1794):20141841. doi: 10.1098/rspb.2014.1841
Pejchar L., Mooney H. A. (2009). Invasive species, ecosystem services and human well-being. Trends Ecol. Evol. 24, 497–504. doi: 10.1016/j.tree.2009.03.016
Peller T., Altermatt F. (2024). Invasive species drive cross-ecosystem effects worldwide. Nat. Ecol. Evol. 8, 1087–1097. doi: 10.1038/s41559-024-02380-1
Peng X. W., Gao H., Dong H. Q., Qian-Hong W. U. (2006). Study on diurnal insect communities in different habitats at the jiuduansha wetland national nature reserve. J. Fudan Univ. 45, 783–790. doi: 10.15943/j.cnki.fdxb-jns.2006.06.017
Pratt M. C., Grason E. W. (2007). Invasive species as a new food source: does a nudibranch predator prefer eating an invasive bryozoan? Biol. Invasions 9, 645–655. doi: 10.1007/s10530-006-9065-x
Pysek P., Jarosik V., Hulme P. E., Pergl J., Hejda M., Schaffner U., et al. (2012). A global assessment of invasive plant impacts on resident species, communities and ecosystems: the interaction of impact measures, invading species’ traits and environment. Global Change Biol. 18, 1725–1737. doi: 10.1111/j.1365-2486.2011.02636.x
Qi T., Li Y., Zhu C., Hao W., Hu C. (2023). Variation law of precipitation in Yancheng from 1960 to 2020. Environ. Prot. Sci. 49, 115–120. doi: 10.16803/j.cnki.issn.1004-6216.2022070028
Qin H., Chu T., Xu W., Lei G., Chen Z., Quan W., et al. (2010). Effects of invasive cordgrass on crab distributions and diets in a Chinese salt marsh. Mar. Ecol. Prog. Ser. 415, 177–187. doi: 10.3354/meps08771
Quan W., Fu C., Jin B., Luo Y., Li B., Chen J., et al. (2007). Tidal marshes as energy sources for commercially important nektonic organisms: stable isotope analysis. Mar. Ecol. Prog. Ser. 352, 89–99. doi: 10.3354/meps07160
Salgado A. L., Saastamoinen M. (2019). Developmental stage-dependent response and preference for host plant quality in an insect herbivore. Anim. Behav. 150, 27–38. doi: 10.1016/j.anbehav.2019.01.018
Schultheis E. H., Berardi A. E., Lau J. A. (2015). No release for the wicked: enemy release is dynamic and not associated with invasiveness. Ecology 96, 2446–2457. doi: 10.1890/14-2158.1
Sheng Y. (2019). Characteristics of Climate Change in Yancheng City and lts lmpact on Agricultural Production. J. Agric. Catastrophology 9, 53–54. doi: 10.19383/j.cnki.nyzhyj.2019.06.019
Smart R. M., Barko J. W. (1978). Influence of sediment salinity and nutrients on the physiological ecology of selected salt marsh plants. Estuar. Coast. Mar. Sci. 7, 487–495. doi: 10.1016/0302-3524(78)90125-1
Smit L. A., Adams J. B., Hawkes S. A., Peer N., Rishworth G. M. (2024). Proportional top-down effects of grapsoid crabs on growth of Spartina maritima cordgrass in southern African salt marshes. Mar. Ecol. Prog. Ser. 739, 49–64. doi: 10.3354/meps14612
Stewart P. S., Hill R. A., Stephens P. A., Whittingham M. J., Dawson W. (2021). Impacts of invasive plants on animal behavior. Ecol. Lett. 24, 891–907. doi: 10.1111/ele.13687
Strauss A., White A., Boots M. (2012). Invading with biological weapons: the importance of disease-mediated invasions. Funct. Ecol. 26, 1249–1261. doi: 10.1111/1365-2435.12011
Sun L., Shao D., Xie T., Gao W., Ma X., Ning Z., et al. (2020). How does spartina alterniflora invade in salt marsh in relation to Tidal Channel networks? Patterns processes. Remote Sens. 12, 2983. doi: 10.3390/rs12182983
Tallamy D. W., Shropshire K. J. (2009). Ranking lepidopteran use of native versus introduced plants. Conserv. Biol. 23, 941–947. doi: 10.1111/j.1523-1739.2009.01202.x
Tang L., Gao Y., Li B., Wang Q., Wang C. H., Zhao B. (2016). Spartina alterniflora with high tolerance to salt stress changes vegetation pattern by outcompeting native species. Ecosphere 5, 1–18. doi: 10.1890/ES14-00166.1
Tekiela D. R., Barney J. N. (2015). System-level changes following invasion caused by disruption of functional relationships among plant and soil properties. Ecosphere 6, 1–16. doi: 10.1890/ES15-00412.1
Vila M., Espinar J. L., Hejda M., Hulme P. E., Jarosik V., Maron J. L., et al. (2011). Ecological impacts of invasive alien plants: a meta-analysis of their effects on species, communities and ecosystems. Ecol. Lett. 14, 702–708. doi: 10.1111/ele.2011.14.issue-7
Wainright C. A., Muhlfeld C. C., Elser J. J., Bourret S. L., Devlin S. P. (2021). Species invasion progressively disrupts the trophic structure of native food webs. Proc. Natl. Acad. Sci. 118, e2102179118. doi: 10.1073/pnas.2102179118
Wang S., Chu T., Huang D., Li B., Wu J. (2014). Incorporation of Exotic Spartina alterniflora into Diet of Deposit-Feeding Snails in the Yangtze River Estuary Salt Marsh: Stable Isotope and Fatty Acid Analyses. Ecosystems 17, 567–577. doi: 10.1007/s10021-013-9743-3
Wang Q., Wang C. H., Zhao B., Ma Z. J., Luo Y. Q., Chen J. K., et al. (2006). Effects of growing conditions on the growth of and interactions between salt marsh plants: implications for invasibility of habitats. Biol. Invasions 8, 1547–1560. doi: 10.1007/s10530-005-5846-x
Wang J., Zhang X., Nie M., Fu C., Chen J., Li B. (2008). Exotic Spartina alterniflora provides compatible habitats for native estuarine crab Sesarma dehaani in the Yangtze River estuary. Ecol. Eng. 34, 57–64. doi: 10.1016/j.ecoleng.2008.05.015
Wu Y., Wang C., Zhang X., Zhao B., Jiang L., Chen J., et al. (2009). Effects of saltmarsh invasion by Spartina alterniflora on arthropod community structure and diets. Biol. Invasions 11, 635–649. doi: 10.1007/s10530-008-9279-1
Xia B., Zhao Y., Shen B., Zhang J., Gu B., Yan T. (1993). The ecological control of pests and diseases of reed in Sheyang beach. J. Plant Resour. Environ. 2, 31–36.
Zeng X. S., Xu G. J., Zhang G. A., Zhang Z. G. (1988). Biological study and precautions of Laelia coenosa. Hubei Agricultural Sciences 08, 27–30.
Zhai J., Hou B., Hu F., Yu G., Li Z., Palmer-Young E. C., et al. (2024). Active defense strategies for invasive plants may alter the distribution pattern of pests in the invaded area. Front. Plant Sci. 15, 1428752. doi: 10.3389/fpls.2024.1428752
Zhang Y., Li Y., Jia Y., Qiu C., Wang Y., Huang W., et al. (2023). Impact factors on distribution and habitat of crab in coastal wetland in tiaozini, Jiangsu, China. Oceanologia Et Limnologia Sinica. 54, 1383–1394.
Zhang H., Liu H., Hou M. (2013). Spatiotemporal characteristics of Spartina alterniflora marsh change in the coastal wetlands of Yancheng caused by natural processes and human activities. Acta Ecologica Sin. 33, 4767–4775. doi: 10.5846/stxb201205050649
Zhao H., Yang W., Xia L., Qiao Y., Xiao Y., Cheng X., et al. (2015). Nitrogen-enriched eutrophication promotes the invasion of spartina alterniflora in coastal China. CLEAN - Soil Air Water 43, 244–250. doi: 10.1002/clen.201300844
Zheng S., Shao D., Gao W., Nardin W., Ning Z., Liu Z., et al. (2022). Drainage efficiency and geometric nuances of tidal channel network mediate Spartina alterniflora landward invasion in marsh-channel system. Front. Mar. Sci. 9, 888597. doi: 10.3389/fmars.2022.888597
Zhou C., An S., Deng Z., Yin D., Zhi Y., Sun Z., et al. (2009). Sulfur storage changed by exotic Spartina alterniflora in coastal saltmarshes of China. Ecol. Eng. 35, 536–543. doi: 10.1016/j.ecoleng.2008.01.004
Keywords: salt marshes, Coastal invasions, insect, plant-herbivore interactions, Phragmites australis, Spartina alterniflora
Citation: Xu T, Ma X, Li Y, Xue H, Zhao S and Liu Z (2024) Coastal exotic plant serves as a habitat for a notorious wetland pest in unfavorable seasons: A case study of exotic Spartina alterniflora in China. Front. Mar. Sci. 11:1478599. doi: 10.3389/fmars.2024.1478599
Received: 10 August 2024; Accepted: 03 September 2024;
Published: 23 September 2024.
Edited by:
Qin Zhu, Southern Marine Science and Engineering Guangdong Laboratory (Guangzhou), ChinaReviewed by:
Jiakai Liu, Beijing Forestry University, ChinaZhiyuan Zhao, Royal Netherlands Institute for Sea Research (NIOZ), Netherlands
Limin Sun, University of Maryland, United States
Zhe Huang, Tianjin Universityn, China
Copyright © 2024 Xu, Ma, Li, Xue, Zhao and Liu. This is an open-access article distributed under the terms of the Creative Commons Attribution License (CC BY). The use, distribution or reproduction in other forums is permitted, provided the original author(s) and the copyright owner(s) are credited and that the original publication in this journal is cited, in accordance with accepted academic practice. No use, distribution or reproduction is permitted which does not comply with these terms.
*Correspondence: Zezheng Liu, zezhengliu@hotmail.com; Yunjing Li, 306270856@qq.com