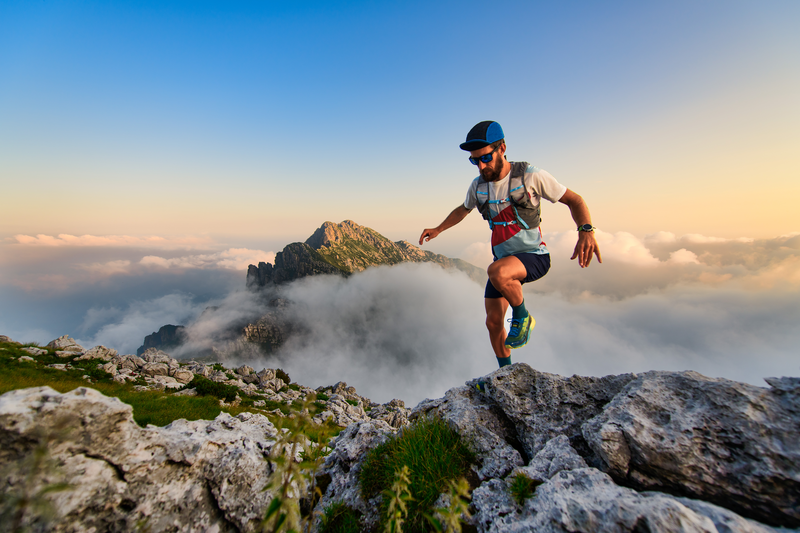
95% of researchers rate our articles as excellent or good
Learn more about the work of our research integrity team to safeguard the quality of each article we publish.
Find out more
BRIEF RESEARCH REPORT article
Front. Mar. Sci. , 20 January 2025
Sec. Marine Fisheries, Aquaculture and Living Resources
Volume 11 - 2024 | https://doi.org/10.3389/fmars.2024.1471204
Seaweed farming is increasingly recognized as a sustainable marine resource management opportunity, but it also poses socioeconomic and environmental risks that require careful evaluation. This quick scoping review (QSR) examines the current state of knowledge on upscaling seaweed farming through co-location with offshore wind energy production. A total of 240 published records from 2001 to 2022 were analyzed, including studies on general seaweed farming and its integration with offshore wind energy, both of which have shown a significant increase in annual publication rates over time. Geographically, the majority of studies on general seaweed farming were conducted in Asia, while most research on wind-focused integration was carried out in Europe. Differences in cultivated species were evident, with red seaweeds dominating the general literature and brown seaweeds dominating wind-focused studies. Ecosystem service analysis revealed that provisioning services were disproportionately emphasized, while cultural services were underrepresented in wind-focused studies as compared to the general literature. Environmental constraints were the most frequently cited challenges across both datasets, but their nature differed: general literature highlighted issues such as pests, diseases, and epiphytes that reduce farm yield, while wind-focused studies emphasized risks of farms to local species, habitats, and ecosystems. While environmental knowledge gaps were the most frequently cited overall, legal knowledge gaps were predominant in wind-focused studies. These findings underscore the need for more geographically and taxonomically diverse studies on seaweed-wind multi-use, along with further investigation into cultural services in offshore contexts, strategies for mitigating environmental risks, and the development of frameworks for shared governance to advance sustainable ocean development.
Seaweed farming, also known as seaweed aquaculture or mariculture, is emerging as a promising source of sustainable biomass with diverse applications (Buschmann et al., 2017; Chopin and Tacon, 2021; Spillias et al., 2023). Compared to terrestrial crops, seaweeds require minimal inputs, such as freshwater, arable land, and fertilizers (Roleda and Hurd, 2019). Conducted in many coastal marine environments worldwide (Food and Agriculture Organization, 2020), seaweed farming primarily takes place in shallow waters but is being trialed at deeper offshore sites (Bak et al., 2020). Cultivated seaweeds include red (phylum Rhodophyta), brown (phylum Ochrophyta including kelps), and green (phylum Chlorophyta) seaweeds, each with distinct properties and commercial value (Food and Agriculture Organization, 2018). The global seaweed farming industry has grown in recent years, driven by increasing demand for seaweed-derived products across sectors such as food, agriculture, cosmetics, and pharmaceuticals (Hafting et al., 2015; Food and Agriculture Organization, 2020; Chopin and Tacon, 2021).
Seaweed farming offers various socioeconomic benefits. For instance, employment across the seaweed value chain (i.e., nursery, harvest, and post-harvest) has diversified livelihoods in coastal communities and empowered women, particularly in regions where the industry is woman-dominated (Sievanen et al., 2005; Larson et al., 2021). However, socioeconomic risks also exist, including adverse health effects on farmers (Fröcklin et al., 2012) and low income due to market volatility and crop failures caused by diseases, pests, and natural disasters (Aslan et al., 2018; Mariño et al., 2019). Additionally, while seaweeds are highly nutritious and rich in dietary fiber and protein (Cherry et al., 2019; Cai et al., 2021), those cultivated in contaminated waters can accumulate heavy metals such as cadmium and inorganic arsenic, posing risks to human health without proper monitoring and regulation (Food and Agriculture Organization and World Health Organization, 2022). Such seaweeds may be more suitable for applications like biofuel production (Fernand et al., 2017).
Seaweed farming also provides environmental benefits (Duarte et al., 2022). Through photosynthesis, seaweeds absorb dissolved carbon dioxide (CO₂) from surrounding waters and release oxygen into the water column, locally mitigating ocean acidification and deoxygenation (Mongin et al., 2016; Jiang et al., 2020; Xiao et al., 2021). At sufficient spatial scales and under suitable oceanographic conditions, seaweed farming could contribute to atmospheric CO2 mitigation; although this pathway remains under active investigation (Bach et al., 2021; Hurd et al., 2022). Furthermore, seaweed farming improves coastal water quality by removing excess nutrients like nitrogen and phosphorus, reducing risks of eutrophication and harmful algal blooms (Yang et al., 2015). It can also enhance biodiversity by providing habitat and food for marine organisms (Kerrison et al., 2015; Walls et al., 2016, Walls et al., 2017; Wood et al., 2017; Visch et al., 2020; Hancke et al., 2021). However, these benefits can vary across ecosystems, as evidenced by studies reporting negative biodiversity impacts in tropical seagrass meadows (reviewed by United Nations Environment Programme, 2023). Environmental problems such as the introduction of invasive species, habitat alteration, and climate change also pose challenges to the sustainable expansion of the seaweed farming industry (Buschmann et al., 2014; United Nations Environment Programme, 2023).
Efforts to strategically expand seaweed farming aim to maximize its socioeconomic and environmental benefits while minimizing associated risks, necessitating improvements in cultivation practices and regulatory frameworks (Costello et al., 2020; Cottier-Cook et al., 2021; Duarte et al., 2022). One innovative approach is co-locating seaweed farms with other marine activities, such as offshore wind energy production (Buck et al., 2008; 2018). Co-location could offer opportunities to upscale sustainable biomass production, optimize marine space use, and share costs for resources like infrastructure, vessels, and personnel (Buck et al., 2018; O'Shea et al., 2024). However, co-location may exacerbate existing environmental and socioeconomic concerns related to offshore development, such as species declines and conflict among stakeholders (O'Shea et al., 2024). Currently, co-location of seaweed farming with offshore wind remains limited to pilot-scale projects, and the factors hindering large-scale implementation, as well as the specific risks and benefits, are poorly understood (Van den Burg et al., 2020; Falconer et al., 2023).
Here, we conduct a quick scoping review (QSR) to evaluate the state of knowledge surrounding the co-location of seaweed farming with offshore wind energy. By synthesizing data from peer-reviewed scientific articles, we provide an overview of the available evidence on the ecosystem services, constraints, and knowledge gaps associated with this multi-use approach. Our findings provide insights for policymakers, researchers, and stakeholders to advance sustainable co-location practices with offshore wind.
To examine the current state of knowledge on co-location of seaweed farming with offshore wind energy, we conducted a quick scoping review (QSR, see Supplementary Methods). Eligible records were identified and reviewed for data across several categories, including: (1) Year of Publication, (2) Location, (3) Scale of Study, (4) Study Protocol Type, (5) Species of Interest, (6) Aquaculture Type, (7) Farm Size, (8) Ecosystem Services, (9) Constraints, (10) Knowledge Gaps, and (11) Multi-use. Some categories were further divided into subcategories, which were in turn divided into specific subtypes, detailed in Supplementary Tables S3–S6. For all eligible records, full texts were reviewed, and data corresponding to each category, subcategory, or subtype were recorded.
Ecosystem Services were subcategorized into Provisioning, Regulating/Habitat, and Cultural Services. Some studies provided evidence for more than one subcategory of Ecosystem Services. Constraints were analyzed using the PESTEL framework (Political, Environmental, Social, Technical, Economic, Legal) (Yüksel, 2012; Bermejo et al., 2022), with the addition of a Study Design subcategory. This subcategory captured studies that identified modeling frameworks with limited data or surveys with potentially biased populations. Keywords such as “constraint,” “challenge,” “bottleneck,” “problem,” “obstacle,” “barrier,” and “restriction” were used to identify relevant information. Knowledge Gaps were similarly subcategorized using the PESTEL framework, with Study Design again included as an additional subcategory.
A total of 240 eligible records were identified relating to seaweed farming in general (hereafter referred to as “All Records”). Of these, a subset focused specifically on the co-location of seaweed farming with offshore wind energy, either as the study’s primary objective or as a dedicated section within the study (hereafter referred to as “Wind Records”). The analysis compared these two datasets (All Records and Wind Records) to examine whether research emphases shift when seaweed farming is co-located with offshore wind.
To evaluate temporal trends in the number of Wind Records and All Records published annually from 2001 to 2022, we conducted Mann-Kendall Tests. Data from 2023 were excluded, as records were only available through March 2023 (see Supplementary Methods).
To assess the state of knowledge, we calculated the frequency of records citing information on Ecosystem Services, Knowledge Gaps, and Constraints within the Wind Records and All Records datasets. Citation frequencies were calculated for each subcategory and subtype within these categories (Supplementary Tables S4–S6). Pie charts were constructed to provide a visual summary of the distribution of citation frequencies for the subcategories and their predominant subtypes.
Chi-square tests were conducted to test for differences in research focus between the Wind Records and All Records datasets. Specifically, we tested for differences between the datasets in citation frequencies across three Ecosystem Services subcategories (Provisioning, Regulating/Habitat, and Cultural Services), and their subtypes. For Constraints, we tested for differences between the datasets across seven subcategories (Political, Environmental, Social, Technical, Economic, Legal, and Study Design Constraints), with additional analysis of the subtypes within the predominant Environmental Constraints subcategory. Finally, for Knowledge Gaps, we tested for differences between the datasets across seven subcategories (Political, Environmental, Social, Technical, Economic, Legal, and Study Design Gaps). As the predominant Knowledge Gap subcategory differed between the datasets, subtype comparisons were not conducted.
Of the 240 eligible records (All Records), 134 (55.8%) mentioned the use of seaweed farming in conjunction with other ocean use activities, and 13 records (5.4%) focused specifically on co-location with offshore wind energy (Wind Records). Among the 134 records referencing multi-use, the majority (77.6%) addressed Integrated Multi-Trophic Aquaculture (IMTA), while the others focused on seaweed farming co-located with offshore wind energy (21.6%) or wave energy (0.7%).
Both datasets showed an increase in the number of publications over time (2001–2022) (Figure 1A). Mann-Kendall tests indicated a significant positive trend for both Wind Records (τ21 = 0.933, p = <0.0001) and All Records (τ21 = 0.998, p = <0.0001) (Figure 1A).
Figure 1. Number of offshore wind-focused records (Wind Records, n = 13) and all eligible seaweed farming records (All Records, n = 240) identified by (A) year of publication and (B) geographic region. General or N/A refers to studies wherein a location was not provided.
The largest proportion of Wind Records (76.9%) originated in Europe, with others in Asia, Africa, and global contexts (Figure 1B). These studies primarily focused on a local scale (36.4%; within cities, villages, individual farming locations, or a small portion of one country), followed by national (27.3%), regional (27.3%), and global (9.1%) scales.
The largest proportion of All Records (37.9%) originated in Asia, followed by Europe and global contexts, and with fewer studies in Africa, Latin America, North America, and Oceania (Figure 1B). Study scales were again primarily local (50.8%), with the remainder spanning national (22.9%), global (15.8%), regional (6.7%), and continental (3.8%) scales.
Wind Records identified six species, and primarily the brown seaweed Saccharina latissima (46.2% of records; junior synonym Laminaria saccharina). Other species included Laminaria digitata (15.9%), Palmaria palmata (15.9%), Kappaphycus alvarezii (7.7%), Euchema denticulatum (7.7%), and Ulva lactuca (7.7%). Regional differences were evident; for instance, records originating in Europe cited Saccharina latissima (54.6%) most frequently, while records originating in Africa cited Euchema denticulatum and Kappaphycus alvarezii most frequently (50.0% each).
For All Records, 75 species or taxonomic groups were cited, spanning red (44.4% of records), brown (24.8% of records), and green (7.5% of records) seaweeds (Supplementary Table S7). Common genera included Kappaphycus, Eucheuma, Saccharina, and Ulva (Supplementary Table S7). Regional trends showed prominence of Kappaphycus spp. in Asia and Saccharina latissima in Europe and North America (Supplementary Table S7).
Provisioning Services were the most frequently cited subcategory of Ecosystem Services in both Wind Records and All Records (Figure 2A). However, a statistically significant difference was observed in the citation frequencies of Provisioning, Regulating/Habitat, and Cultural Services between the datasets (χ² = 12.7, df = 2, p = 0.027) (Figure 2A). Post hoc comparisons revealed that this difference was driven by a significantly higher frequency of Provisioning Services (χ² = 6.1, df = 1, p = 0.013) and lower frequency of Cultural Services (χ² = 5.6, df = 1, p = 0.018) cited in Wind Records as compared to All Records (Figure 2A).
Figure 2. Citation frequencies for Ecosystem Services in offshore wind-focused records (Wind Records, left column, n = 13) and all eligible seaweed farming records (All Records, right column, n = 240) (A) pooled within all subcategories and (B) pooled within subtypes of the Provisioning (top row), Regulating/Habitat (middle row), and Cultural (bottom row) Services subcategories.
There were no statistically significant differences in the citation frequencies of subtypes within each Ecosystem Services subcategory between the datasets (Provisioning Services subtypes: χ2 = 1.7, df = 6, p = 0.947; Regulating/Habitat Services subtypes: χ2 = 6.5, df = 12, p = 0.892; Cultural Services subtypes: χ2 = 1.5, df = 10, p = 0.999) (Figure 2B). Primary subtypes included: seaweed as food and food additives for human consumption and fodder and fertilizer in agriculture (Provisioning Services); bioremediation, nutrient sequestration, habitat/food provisioning for marine life, and climate change mitigation (Habitat/Regulating Services); and improved quality of life and source of income and employment for coastal communities (Cultural Services) (Figure 2B).
Environmental Constraints were the most cited Constraints subcategory in both Wind Records and All Records (Figure 3). However, a statistically significant difference was observed in the citation frequencies of the Constraints subcategories between datasets (χ2 = 19.8, df = 6, p = 0.003) (Figure 3). Post hoc comparisons revealed that this difference was driven by a significantly higher frequency of Environmental Constraints (χ² = 11.6, df = 1, p < 0.001) cited in Wind Records as compared to All Records (Figure 3).
Figure 3. Citation frequencies for Constraints in offshore wind-focused records (Wind Records, left column, n = 13) and all eligible seaweed farming records (All Records, right column, n = 240) pooled within all subcategories (top row) and pooled within subtypes of the predominant Environmental Constraints subcategory (bottom row).
There was also a statistically significant difference observed in the citation frequencies of the subtypes within the Environmental Constraints subcategory between datasets (χ2 = 23.1, df = 12, p = 0.027) (Figure 3). Post hoc comparisons revealed that this difference was driven by a significantly higher frequency of Biological Shifts (χ² = 6.9, df = 1, p = 0.00845) cited in Wind Records as compared to All Records (Figure 3). These biological shifts focused on issues such as competition with local species and risks to marine mammals and birds.
Technical and Social Constraints were also prominent in Wind Records (Figure 3). These related to the need for improved technology and infrastructure for offshore cultivation and stakeholder perception and conflicts, respectively.
Legal Gaps was the primary subcategory of Knowledge Gaps in Wind Records, focusing on marine spatial planning and governance challenges (Figure 4). In contrast, Environmental Gaps were the primary subcategory in All Records, focusing on the wider ecosystem effects of seaweed farming (Figure 4). Accordingly, there was a statistically significant difference observed in the citation frequencies of the Knowledge Gaps subcategories between datasets (χ2 = 15.5, df = 5, p = 0.008) (Figure 4). Post hoc comparisons confirmed that this difference was driven by a significantly higher frequency of Legal Gaps (χ² = 10.2, df = 1, p = 0.001) and lower frequency of Environmental Gaps (χ² = 4.2, df = 1, p = 0.042) cited in Wind Records as compared to All Records (Figure 4).
Figure 4. Citation frequencies for Knowledge Gaps in offshore wind-focused records (Wind Records, left column, n = 13) and all eligible seaweed farming records (All Records, right column, n = 240) pooled within all subcategories (top row) and pooled within subtypes of the predominant Legal and Environmental Gaps subcategories for Wind Records and All Records, respectively (bottom row).
Economic Gaps were also prominent in Wind Records (Figure 4), and related primarily to the market value and viability of seaweed products.
This study provides an overview of available evidence on co-locating seaweed farming with offshore wind energy, highlighting key temporal and spatial trends as well as patterns in the emphasis of general seaweed farming literature versus offshore wind-focused studies on various ecosystem services, constraints, and knowledge gaps. The findings demonstrate a small but growing number of studies on the topic of offshore integration, and indicate opportunities and challenges associated with this emerging multi-use system. For instance, a geographical skew in the literature, with most wind-focused studies concentrated in Europe and limited data from Asia—where the majority of farmed seaweed biomass originates—indicates a need for broader regional representation in future research on co-location. Additionally, the identification of 75 seaweed species or taxonomic groups in general seaweed farming studies, compared to only six species mentioned in studies on offshore integration, highlights the need for greater taxonomic representation in future research. These findings amplify a call for interdisciplinary investigations into the socioeconomic and environmental dimensions of seaweed farming in diverse contexts (United Nations Environment Programme, 2023).
Our ecosystem services analysis indicated a predominant emphasis in seaweed farming research on provisioning services, or the material and tangible benefits that humans obtain directly from ecosystems, here focusing on seaweed in food and agricultural applications. Both the general and wind-focused literature emphasized these provisioning services, but they were even more prominent in the wind literature. This focus highlights a strong and consistent interest in the value of seaweed-derived products, and aligns with extensive literature demonstrating the nutritional benefits of seaweeds (Cherry et al., 2019), their role in enhancing agricultural productivity through seaweed extracts (Nabti et al., 2017; Ali et al., 2021), and their use as feed additives for livestock, including cattle and horses (MacArtain et al., 2007; Pereira et al., 2020).
While regulating or habitat services, such as bioremediation and habitat and food provisioning for marine species, were frequently discussed, cultural services received considerably less attention, particularly in offshore contexts. In the wind-focused records, only two types of cultural services were cited: employment or income generation and improved quality of life. This is in comparison to 11 types of cultural services cited in the general seaweed farming literature, which emphasized small-scale, community-based seaweed farming in shallow, coastal areas, often in poor, rural regions of developing countries (Rimmer et al., 2021; Mariño et al., 2019). This limited focus in the wind literature may reflect insufficient research on the cultural benefits of offshore multi-use. Importantly, scaling seaweed farming to offshore facilities would likely industrialize production, potentially altering its cultural services (Rimmer et al., 2021; Larson et al., 2021). Future studies should examine how scaling up production may impact socioeconomic outcomes, focusing on the demographics of seaweed farming communities, and particularly vulnerable groups.
The early stage of offshore seaweed farming was reflected in our analysis of the wind-focused studies, where legal gaps emerged as the predominant knowledge gap. These gaps underscored the need for clear frameworks for shared legal governance (Van den Burg et al., 2020). Indeed, differences in the emphasis on constraints and knowledge gaps between general seaweed farming literature and wind-focused studies likely reflect the differing stages of their implementation. In wind-focused studies, primary constraints centered on risks to species, habitats, and ecosystems, including potential negative interactions with native species, such as nutrient competition with phytoplankton and cascading effects up the food chain (Campbell et al., 2019; Shi et al., 2011). In contrast, general seaweed farming studies predominantly cited the impact of nuisance species—pests, diseases, and epiphytes—which reduce biomass, degrade product quality, and result in economic losses for farmers (Bannister et al., 2019; Hu et al., 2010; Ward et al., 2020). Notably, wider ecosystem effects were more often cited as a knowledge gap in general seaweed farming rather than an immediate constraint. These distinctions suggest that ecosystem degradation is perceived as a more immediate threat in the emerging offshore seaweed farming industry, while risks of production failure, often due to nuisance species, are more pressing in established shallow-water farming systems. Regardless of the context, strategic farming practices and robust biosecurity frameworks are widely recognized as critical for ensuring the sustainability of commercial seaweed farming (Cottier-Cook et al., 2016, Cottier-Cook et al., 2021).
In conclusion, seaweed farm-offshore wind multi-use holds the potential to provide ecosystem services, yet concerns remain regarding potential environmental impacts and a lack of a regulatory framework. Research relating to the environmental impacts of seaweed farming, and specifically mitigating competition for resources, is a clear area of future research need for offshore wind multi-use. Additionally, research is needed on the legal governance of multi-use sites, and next steps could include examinations into regional stakeholder views and values regarding the opportunities and challenges of seaweed farm-offshore wind co-location. Finally, comparative studies that assess the feasibility and sustainability of different seaweed farming models, including integrated multi-trophic aquaculture (IMTA) and offshore wind co-location, can inform evidence-based decision-making and policy development. Addressing these gaps will require collaboration among stakeholders, including policymakers, industry, and local communities, to move towards sustainable co-location practices.
The original contributions presented in the study are included in the article/Supplementary Material. Further inquiries can be directed to the corresponding author.
BC: Writing – original draft, Writing – review & editing, Data curation, Formal Analysis, Investigation, Methodology, Funding acquisition. CB: Writing – review & editing, Resources, Validation. PL: Writing – review & editing, Funding acquisition, Resources, Validation. CJF: Writing – review & editing, Conceptualization, Formal Analysis, Funding acquisition, Investigation, Methodology, Supervision, Visualization, Writing – original draft, Resources.
The author(s) declare that financial support was received for the research, authorship, and/or publication of this article. This work was supported by Connecticut Sea Grant (#R/ES-28 awarded to CJF), the New Jersey Economic Development Authority and New Jersey Wind Institute (graduate fellowship awarded to BC) and Montclair State University (sabbatical funds awarded to CJF).
This manuscript was developed in partial fulfillment of the requirements for the degree of Master of Science at Montclair State University, and the authors would like to thank the Montclair State University Department of Biology for their contribution.
The authors declare that the research was conducted in the absence of any commercial or financial relationships that could be construed as a potential conflict of interest.
All claims expressed in this article are solely those of the authors and do not necessarily represent those of their affiliated organizations, or those of the publisher, the editors and the reviewers. Any product that may be evaluated in this article, or claim that may be made by its manufacturer, is not guaranteed or endorsed by the publisher.
The Supplementary Material for this article can be found online at: https://www.frontiersin.org/articles/10.3389/fmars.2024.1471204/full#supplementary-material
Ali O., Ramsubhag A., Jayaraman J. (2021). Biostimulant properties of seaweed extracts in plants: Implications towards sustainable crop production. Plants 10, 531. doi: 10.3390/plants10030531
Aslan L. O. M., Supendy R., Taridala S. A. A., Hafid H., Sifatu W. O., Sailan Z., et al. (2018). “Income of seaweed farming households: a case study from Lemo of Indonesia,” in IOP Conference Series: Earth and Environmental Science, vol. 175. (Bristol, United Kingdom: IOP Publishing), 012221.
Bach L. T., Tamsitt V., Gower J., Hurd C. L., Raven J. A., Boyd P. W. (2021). Testing the climate intervention potential of ocean afforestation using the Great Atlantic Sargassum Belt. Nat. Commun. 12, 2556. doi: 10.1038/s41467-021-22837-2
Bak U. G., Gregersen Ó., Infante J. (2020). Technical challenges for offshore cultivation of kelp species: lessons learned and future directions. Botanica marina 63, 341–353. doi: 10.1515/bot-2019-0005
Bannister J., Sievers M., Bush F., Bloecher N. (2019). Biofouling in marine aquaculture: a review of recent research and developments. Biofouling 35, 631–648. doi: 10.1080/08927014.2019.1640214
Bermejo R., Buschmann A., Capuzzo E., Cottier-Cook E., Fricke A., Hernández I., et al. (2022). State of knowledge regarding the potential of macroalgae cultivation in providing climate-related and other ecosystem services (Leipzig, Germany: Eklipse).
Buck B. H., Krause G., Michler-Cieluch T., Brenner M., Buchholz C. M., Busch J. A., et al. (2008). Meeting the quest for spatial efficiency: progress and prospects of extensive aquaculture within offshore wind farms. Helgoland Mar. Res. 62, 269–281. doi: 10.1007/s10152-008-0115-x
Buck B. H., Troell M. F., Krause G., Angel D. L., Grote B., Chopin T. (2018). State of the art and challenges for offshore integrated multi-trophic aquaculture (IMTA). Front. Mar. Sci. 5, 165. doi: 10.3389/fmars.2018.00165
Buschmann A. H., Camus C., Infante J., Neori A., Israel Á., Hernández-González M. C., et al. (2017). Seaweed production: overview of the global state of exploitation, farming and emerging research activity. Eur. J. Phycology 52, 391–406. doi: 10.1080/09670262.2017.1365175
Buschmann A. H., Prescott S., Potin P., Faugeron S., Vasquez J. A., Camus C., et al. (2014). “The status of kelp exploitation and marine agronomy, with emphasis on Macrocystis pyrifera, in Chile,” in Advances in botanical research, vol. 71. (Amsterdam: Academic Press), 161–188.
Cai J., Lovatelli A., Aguilar-Manjarrez J., Cornish L., Dabbadie L., Desrochers A., et al. (2021). Seaweeds and microalgae: an overview for unlocking their potential in global aquaculture development (Rome, Italy: FAO Fisheries and Aquaculture Circular), 1229.
Campbell I., Macleod A., Sahlmann C., Neves L., Funderud J., Øverland M., et al. (2019). The environmental risks associated with the development of seaweed farming in Europe-prioritizing key knowledge gaps. Front. Mar. Sci. 6, 107. doi: 10.3389/fmars.2019.00107
Cherry P., Yadav S., Strain C. R., Allsopp P. J., McSorley E. M., Ross R. P., et al. (2019). Prebiotics from seaweeds: An ocean of opportunity? Mar. Drugs 17, 327. doi: 10.3390/md17060327
Chopin T., Tacon A. G. (2021). Importance of seaweeds and extractive species in global aquaculture production. Rev. Fisheries Sci. Aquaculture 29, 139–148. doi: 10.1080/23308249.2020.1810626
Costello C., Cao L., Gelcich S., Cisneros-Mata M.Á., Free C. M., Froehlich H. E., et al. (2020). The future of food from the sea. Nature 588, 95–100. doi: 10.1038/s41586-020-2616-y
Cottier-Cook E. J., Nagabhatla N., Asri A., Beveridge M., Bianchi P., Bolton J., et al. (2021). Ensuring the sustainable future of the rapidly expanding global seaweed aquaculture industry–a vision (Richmond Hill, Ontario, Canada: United Nations University (INWEH) and Scottish Association for Marine Science Policy Brief).
Cottier-Cook E. J., Nagabhatla N., Badis Y., Campbell M., Chopin T., Dai W., et al. (2016). Safeguarding the future of the global seaweed aquaculture industry (Richmond Hill, Ontario, Canada: United Nations University (INWEH) and Scottish Association for Marine Science Policy Brief).
Duarte C. M., Bruhn A., Krause-Jensen D. (2022). A seaweed aquaculture imperative to meet global sustainability targets. Nat. Sustainability 5, 185–193. doi: 10.1038/s41893-021-00773-9
Falconer L., Cutajar K., Krupandan A., Capuzzo E., Corner R. A., Ellis T., et al. (2023). Planning and licensing for marine aquaculture. Rev. Aquaculture 15, 1374–1404. doi: 10.1111/raq.12783
Fernand F., Israel A., Skjermo J., Wichard T., Timmermans K. R., Golberg A. (2017). Offshore macroalgae biomass for bioenergy production: Environmental aspects, technological achievements and challenges. Renewable Sustain. Energy Rev. 75, 35–45. doi: 10.1016/j.rser.2016.10.046
Food and Agriculture Organization (2018). The global status of seaweed production, trade and utilization Vol. 124 (Rome: Globefish Research Programme), 120.
Food and Agriculture Organization (2020). The State of World Fisheries and Aquaculture 2020 (Rome: Sustainability in action). doi: 10.4060/ca9229en
Food and Agriculture Organization and World Health Organization (2022). Report of the expert meeting on food safety for seaweed – Current status and future perspectives (Rome: The Food and Agriculture Organization of the United Nations (FAO)), 28–29. doi: 10.4060/cc0846en
Fröcklin S., de la Torre-Castro M., Lindström L., Jiddawi N. S., Msuya F. E. (2012). Seaweed mariculture as a development project in Zanzibar, East Africa: A price too high to pay? Aquaculture 356, 30–39. doi: 10.1016/j.aquaculture.2012.05.039
Hafting J. T., Craigie J. S., Stengel D. B., Loureiro R. R., Buschmann A. H., Yarish C., et al. (2015). Prospects and challenges for industrial production of seaweed bioactives. J. Phycology 51, 821–837. doi: 10.1111/jpy.2015.51.issue-5
Hancke K., Broch O. J., Olsen Y., Bekkby T., Hansen P. K., Fieler R., et al. (2021). “Miljøpåvirkninger av taredyrking og forslag til utvikling av overvåkingsprogram,” in Norwegian with English summary. Oslo, Norway.
Hu C., Li D., Chen C., Ge J., Muller-Karger F. E., Liu J., et al. (2010). On the recurrent Ulva prolifera blooms in the Yellow Sea and East China Sea. J. Geophysical Research: Oceans 115. doi: 10.1029/2009JC005561
Hurd C. L., Law C. S., Bach L. T., Britton D., Hovenden M., Paine E. R., et al. (2022). Forensic carbon accounting: Assessing the role of seaweeds for carbon sequestration. J. Phycology 58, 347–363. doi: 10.1111/jpy.13249
Jiang Z., Liu J., Li S., Chen Y., Du P., Zhu Y., et al. (2020). Kelp cultivation effectively improves water quality and regulates phytoplankton community in a turbid, highly eutrophic bay. Sci. Total Environ. 707, 135561. doi: 10.1016/j.scitotenv.2019.135561
Kerrison P. D., Stanley M. S., Edwards M. D., Black K. D., Hughes A. D. (2015). The cultivation of European kelp for bioenergy: site and species selection. Biomass Bioenergy 80, 229–242. doi: 10.1016/j.biombioe.2015.04.035
Larson S., Stoeckl N., Fachry M. E., Mustafa M. D., Lapong I., Purnomo A. H., et al. (2021). Women's well-being and household benefits from seaweed farming in Indonesia. Aquaculture 530, 735711. doi: 10.1016/j.aquaculture.2020.735711
MacArtain P., Gill C. I., Brooks M., Campbell R., Rowland I. R. (2007). Nutritional value of edible seaweeds. Nutr. Rev. 65, 535–543. doi: 10.1111/j.1753-4887.2007.tb00278.x
Mariño M., Breckwoldt A., Teichberg M., Kase A., Reuter H. (2019). Livelihood aspects of seaweed farming in Rote Island, Indonesia. Mar. Policy 107, 103600. doi: 10.1016/j.marpol.2019.103600
Mongin M., Baird M. E., Hadley S., Lenton A. (2016). Optimising reef-scale CO2 removal by seaweed to buffer ocean acidification. Environ. Res. Lett. 11, 034023. doi: 10.1088/1748-9326/11/3/034023
Nabti E., Jha B., Hartmann A. (2017). Impact of seaweeds on agricultural crop production as biofertilizer. Int. J. Environ. Sci. Technol. 14, 1119–1134. doi: 10.1007/s13762-016-1202-1
O'Shea R., Capuzzo E., Hemming V., Grebe G., Stafford R., van den Burg S. W., et al. (2024). Managing offshore multi-use settings: Use of conceptual mapping to reduce uncertainty of co-locating seaweed aquaculture and wind farms. J. Environ. Manage. 358, 120696. doi: 10.1016/j.jenvman.2024.120696
Pereira L., Bahcevandziev K., Joshi N. H. (Eds.) (2020). Seaweeds as plant fertilizer, agricultural biostimulants and animal fodder (Boca Raton, FL, USA: CRC Press), 224–2pp.
Rimmer M. A., Larson S., Lapong I., Purnomo A. H., Pong-Masak P. R., Swanepoel L., et al. (2021). Seaweed aquaculture in Indonesia contributes to social and economic aspects of livelihoods and community wellbeing. Sustainability 13, 10946. doi: 10.3390/su131910946
Roleda M. Y., Hurd C. L. (2019). Seaweed nutrient physiology: application of concepts to aquaculture and bioremediation. Phycologia 58, 552–562. doi: 10.1080/00318884.2019.1622920
Shi J., Wei H., Zhao L., Yuan Y., Fang J., Zhang J. (2011). A physical–biological coupled aquaculture model for a suspended aquaculture area of China. Aquaculture 318, 412–424. doi: 10.1016/j.aquaculture.2011.05.048
Sievanen L., Crawford B., Pollnac R., Lowe C. (2005). Weeding through assumptions of livelihood approaches in ICM: Seaweed farming in the Philippines and Indonesia. Ocean Coast. Manage. 48, 297–313. doi: 10.1016/j.ocecoaman.2005.04.015
Spillias S., Kelly R., Cottrell R. S., O’Brien K. R., Im R. Y., Kim J. Y., et al. (2023). The empirical evidence for the social-ecological impacts of seaweed farming. PLoS Sustainability Transformation 2, e0000042. doi: 10.1371/journal.pstr.0000042
United Nations Environment Programme (2023). Seaweed Farming: Assessment on the Potential of Sustainable Upscaling for Climate, Communities and the Planet (Nairobi: United Nations Environment Programme).
Van den Burg S. W., Röckmann C., Banach J. L., Van Hoof L. (2020). Governing risks of multi-use: seaweed aquaculture at offshore wind farms. Front. Mar. Sci. 7, 60. doi: 10.3389/fmars.2020.00060
Visch W., Kononets M., Hall P. O., Nylund G. M., Pavia H. (2020). Environmental impact of kelp (Saccharina latissima) aquaculture. Mar. pollut. Bull. 155, 110962. doi: 10.1016/j.marpolbul.2020.110962
Walls A. M., Kennedy R., Edwards M. D., Johnson M. P. (2017). Impact of kelp cultivation on the Ecological Status of benthic habitats and Zostera marina seagrass biomass. Mar. pollut. Bull. 123, 19–27. doi: 10.1016/j.marpolbul.2017.07.048
Walls A. M., Kennedy R., Fitzgerald R. D., Blight A. J., Johnson M. P., Edwards M. D. (2016). Potential novel habitat created by holdfasts from cultivated Laminaria digitata: assessing the macroinvertebrate assemblages. Aquaculture Environ. Interact. 8, 157–169. doi: 10.3354/aei00170
Ward G. M., Faisan J. P. Jr., Cottier-Cook E. J., Gachon C., Hurtado A. Q., Lim P. E., et al. (2020). A review of reported seaweed diseases and pests in aquaculture in Asia. J. World Aquaculture Soc. 51, 815–828. doi: 10.1111/jwas.12649
Wood D., Capuzzo E., Kirby D., Mooney-McAuley K., Kerrison P. (2017). UK macroalgae aquaculture: What are the key environmental and licensing considerations? Mar. Policy 83, 29–39. doi: 10.1016/j.marpol.2017.05.021
Xiao X., Agustí S., Yu Y., Huang Y., Chen W., Hu J., et al. (2021). Seaweed farms provide refugia from ocean acidification. Sci. Total Environ. 776, 145192. doi: 10.1016/j.scitotenv.2021.145192
Yang Y., Liu Q., Chai Z., Tang Y. (2015). Inhibition of marine coastal bloom-forming phytoplankton by commercially cultivated Gracilaria lemaneiformis (Rhodophyta). J. Appl. Phycology 27, 2341–2352. doi: 10.1007/s10811-014-0486-0
Keywords: seaweed, macroalgae, cultivation, offshore wind, multi-use
Citation: Coffey B, Borgerson C, Lal P and Feehan CJ (2025) Co-location of seaweed farming with offshore wind energy: a quick scoping review. Front. Mar. Sci. 11:1471204. doi: 10.3389/fmars.2024.1471204
Received: 26 July 2024; Accepted: 20 December 2024;
Published: 20 January 2025.
Edited by:
Søren Laurentius Nielsen, Ocean Institute, DenmarkReviewed by:
Pascal Jean Lopez, Centre National de la Recherche Scientifique (CNRS), FranceCopyright © 2025 Coffey, Borgerson, Lal and Feehan. This is an open-access article distributed under the terms of the Creative Commons Attribution License (CC BY). The use, distribution or reproduction in other forums is permitted, provided the original author(s) and the copyright owner(s) are credited and that the original publication in this journal is cited, in accordance with accepted academic practice. No use, distribution or reproduction is permitted which does not comply with these terms.
*Correspondence: Brianna Coffey, Yi5jb2ZmZXkwOTRAZ21haWwuY29t
Disclaimer: All claims expressed in this article are solely those of the authors and do not necessarily represent those of their affiliated organizations, or those of the publisher, the editors and the reviewers. Any product that may be evaluated in this article or claim that may be made by its manufacturer is not guaranteed or endorsed by the publisher.
Research integrity at Frontiers
Learn more about the work of our research integrity team to safeguard the quality of each article we publish.