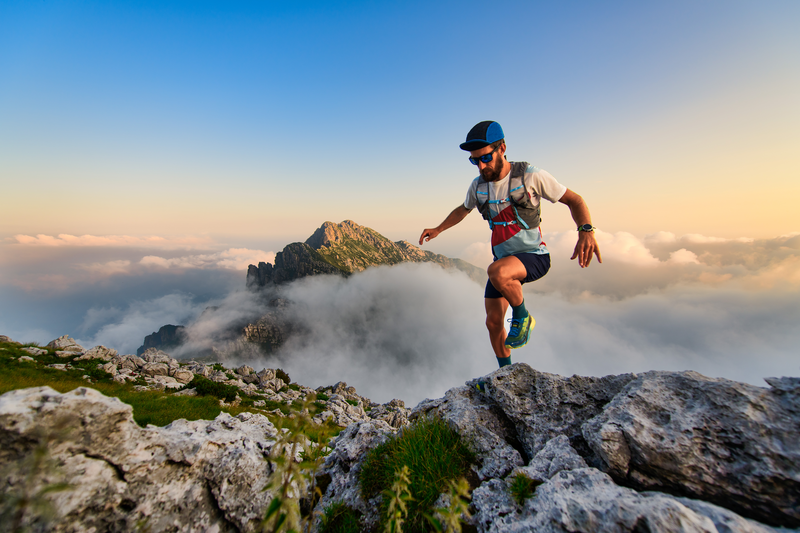
94% of researchers rate our articles as excellent or good
Learn more about the work of our research integrity team to safeguard the quality of each article we publish.
Find out more
ORIGINAL RESEARCH article
Front. Mar. Sci. , 22 January 2025
Sec. Marine Ecosystem Ecology
Volume 11 - 2024 | https://doi.org/10.3389/fmars.2024.1462326
This article is part of the Research Topic Turning with the Tide and Time in the Salish Sea: Change in Estuary and Nearshore Habitats and Species Dependent on Them View all 9 articles
Overexploitation and degradation of water quality nearly depleted Olympia oyster stocks in Puget Sound, Washington, USA by the early 1900s. With an intended goal of creating self-sustaining Olympia oyster populations in a target region in Puget Sound, the Swinomish Indian Tribal Community began reestablishing Olympia oysters at two different sites, Kiket and Lone Tree, from 2015-2017. One of our primary objectives was to quantify the biological successes or failures of our reestablished populations. Our results provide a guide for the evolution of project-specific, evidence-based restoration plans that could allow for further use of adaptive management and conservation aquaculture. Following the creation of experimental plots and restoration beds across two sites using 735 m² of shell habitat, including 245 m² of seeded cultch, we measured temporal change in oyster length and density as proxies for growth, recruitment, and survival. Significant growth was observed each year in each lagoon. Despite the known presence of brooding oysters and competent larvae in the region, we found no evidence of recruitment at either site through six years of monitoring. Survival decreased significantly each year and at each site. Thus, while we quantify evidence of growth and reproduction, we are not meeting the success metrics of recruitment or survival therefore hindering the chances of long-term success. We hypothesize that our restoration efforts are hampered by the relatively small population size within our restored areas, insufficient amounts of appropriate surrounding habitat, and lower water residence time. Our study suggests managers need to consistently monitor restoration projects due to site-specific differences and to determine if local failure is a possibility. Low survival and recruitment do not necessitate termination of projects. However, these measurements do suggest that projects like ours need to consider expanding use of conservation aquaculture as a tool or employing adaptive management by developing and implementing novel strategies to increase naturally-occurring adult populations and available habitat.
Although Indigenous oyster fisheries existed sustainably for millennia (Reeder-Myers et al., 2022), oyster reefs, once dominant throughout coastal estuaries, have declined an estimated 85-88% on a global scale since European colonization (Beck et al., 2011; Zu Ermgassen et al., 2012). A variety of reasons, not limited to overexploitation, loss of habitat, disease, and environmental degradation have contributed to the population decline (e.g., Kirby, 2004; White et al., 2009a; Carranza et al., 2009). The loss of oyster reefs is a cause for concern due to the variety of ecosystem services oysters can provide including water filtration, benthic-pelagic coupling, and sediment stabilization (Coen et al., 2007; Grabowski and Peterson, 2007; Smith et al., 2022). Moreover, oyster reefs structure habitat, serving other marine species in such ways as providing foraging habitat to predators or refuge for prey (as reviewed in Coen et al., 2007). In order to revitalize these ecosystem services, global restoration efforts have recently increased.
Ecological restoration projects can often suffer from a lack of organized, predetermined, criteria for measuring success (Suding, 2011). Yet, in the case of oyster restoration, numerous publications exist to guide practitioners in the development of restoration programs, goals, and subsequent monitoring efforts (Baggett et al., 2015; e.g., Brumbaugh et al., 2006; Wasson et al., 2015; Fitzsimons et al., 2020). Many restoration projects interpret success differently depending on the original goals of the project, with definitions ranging widely from recovery of an oyster fishery (Mann and Powell, 2007), reestablishment of ecosystem services (Smith et al., 2022; Grabowski and Peterson, 2007), or the creation of self-sustaining restored oyster beds (Blake and Bradbury, 2012), among other examples (e.g., Coen and Luckenbach, 2000; Lipcius et al., 2015; Walles et al., 2016). Depending on each project’s goals and their selected measurements of success, results from individual oyster restoration projects can vary in terms of their success or failure based on a number of factors including site selection as well as local abiotic and biotic conditions. For example, Powers et al. (2009) demonstrated that restoration projects located in no-harvest areas generally succeed based on metrics of reef height, oyster density, and recent recruitment. Whereas, Hemraj et al. (2022) found that while initial increases in species abundance and diversity are recorded in oyster restoration projects globally, these increases often failed to fully reestablish ecosystems to the pre-disturbed state. Furthermore, while numerous publications on oyster reef restoration metrics of success or failure exist, the vast majority of this work is based on species that develop large biogenic reef habitat such as Crassostrea virginica and may not be as applicable toward other species that build lower-profile beds with different reproductive strategies (i.e., brooding versus broadcast spawning) such as Olympia oysters, Ostrea lurida, the only oyster native to the west coast of North America.
Olympia oyster populations were once so abundant that the species supported important Indigenous subsistence fisheries and post-colonial commercial fisheries throughout much of its range from Baja California, Mexico to Alaska (Reeder-Myers et al., 2022; Baker, 1995; Steele, 1957; Polson and Zacherl, 2009). However, since European arrival, the population rapidly depleted resulting in range-wide restoration efforts which have increased over the last two decades with an overarching goal to restore populations to prior conditions (as reviewed in Ridlon et al., 2021a). In order to reach this goal, many Olympia oyster restoration programs aim to reestablish self-sustaining populations (‘source populations’) and measure success following published guidelines that include tracking metrics such as recruitment, growth, and density (e.g., Baggett et al., 2015).
Olympia oysters were historically found in all seven of the sub-basins of Washington state’s Salish Sea (Hatch and Wyllie-Echeverria, 2016; Blake and Bradbury, 2012), yet the population has collapsed and ~5% of the original stock remains (Blake and Bradbury, 2012; Baker, 1995; Zu Ermgassen et al., 2012). Recognizing a need to take action to rebuild the native stocks of Olympia oysters in Washington’s region of the Salish Sea, the Washington Department of Fish and Wildlife implemented an updated stock rebuilding plan in 2012 (Blake and Bradbury, 2012). In contrast to Suding’s (2011) review noting that comprehensive measurements of restoration success are often lacking, Blake and Bradbury (2012) suggest clear and attainable benchmarks to track restoration progress and success in Washington.
Beginning with a pilot study in 2012 and the establishment of a larger restoration effort in 2016, the Swinomish Indian Tribal Community implemented the only known Olympia oyster restoration work in the Whidbey sub-basin (herein referred to as Whidbey Basin). Whidbey Basin historically supported several known populations of Olympia oysters, was identified as one of the 19 priority locations for Olympia oyster stock rebuilding by Washington Department of Fish and Wildlife, and was recently listed as one of the top ten priority sites along the northeastern Pacific Coast where conservation aquaculture could boost recovery (Blake and Bradbury, 2012; Ridlon et al., 2021b). From the beginning of the project, we planned collection of data following restoration guidelines and aimed to measure all of the suggested benchmarks defined in Blake and Bradbury (2012), such as reproduction, recruitment, survival, increased oyster coverage, and increased fish use (Greiner et al., 2015). In addition to these benchmarks, we also opted to quantify size structure of the oysters to better understand if conditions at our sites were favorable for growth and survival (Greiner et al., 2015; Baggett et al., 2014; Wasson et al., 2015).
While we have already addressed numerous scientific questions involving the biology and ecology of Olympia oysters from our outplanted populations (Barber et al., 2016; Grossman et al., 2020; Munsch et al., 2021), the objective of this analysis was to quantify the relative successes and failures of our oyster re-establishment efforts in the northern Whidbey Basin. By examining temporal change in our outplanted oysters across multiple treatments and sites, we looked for evidence of (1) growth and presence of multiple size classes, (2) natural recruitment, and (3) high survival rates across our restoration sites to measure the success of our project. We then utilized these results to determine if enhancement efforts should continue in these locations or if we needed to consider adaptive management or increase the use of conservation aquaculture to ensure success. Importantly, we designed these methods to be readily reproduceable for use by other restoration practitioners interested in determining success of their own local oyster restoration projects.
The Swinomish Indian Tribal Community (SITC) Reservation is located on Fidalgo Island, Washington, USA in northern Puget Sound and contains tidelands that extend along the western shore of a peninsula, where our two Olympia oyster enhancement sites are located near Lone Tree Point and Kiket Island, in Skagit Bay and Similk Bay, respectively (Figure 1). Both of these sites are located within Whidbey Basin, one of the seven Puget Sound sub-basins that are largely defined by oceanographic features (e.g., Khangaonkar et al., 2011). The initial restoration sites were both located in pocket estuaries, small subestuaries (i.e., “barrier lagoons”) perched behind spits or barrier beaches with minor ephemeral freshwater inputs (Beamer et al., 2003; Shipman, 2008). These two sites, Lone Tree Lagoon (LT) and Kiket Lagoon (KI), were selected as project sites in conjunction with Washington Department of Fish and Wildlife and the Skagit Marine Resources Committee because they met several criteria including but not limited to: nearby historical presence, presumed extended water residency of surrounding embayments, lower predator populations, constant inundation regardless of tidal cycle, and mitigated effects from storms and extreme temperature events (Figure 1) (Kornbluth et al., 2022; Blake and Bradbury, 2012; Wasson et al., 2015; Sponaugle et al., 2002; Pritchard et al., 2015). Additionally, we hypothesized that the pocket estuary populations could eventually serve as source populations for future restoration areas on adjacent tidelands.
Figure 1. Location of Swinomish Olympia oyster restoration sites. SM, Similk Bay; SK, Skagit Bay; KI, Kiket Lagoon; LT, Lone Tree Lagoon.
When restoration efforts began, temperature and salinity monitoring devices were placed in the two lagoons from 15 April 2015 - 31 August 2015. During that time, LT recorded a mean temperature (± standard error) of 14.7 ± 2.5°C and a mean salinity of 22.9 ± 1.6. Kiket Lagoon recorded a temperature of 17.5 ± 3.6°C and a mean salinity of 19.8 ± 2.3 (Barber et al., 2016).
As our restoration efforts broadened and goals evolved, we began to expand habitat [i.e., adding unseeded Pacific oyster (Magallana gigas) shell to a beach] outside both lagoons to intertidal sites (Figure 1). These intertidal sites are subjected to daily tidal flux and are slightly more exposed to wind wave energy from surrounding Skagit and Similk Bays (SITC Fisheries, personal observation). One of two sites in Skagit Bay is located near LT within an area that may entrain late-stage Olympia oyster larvae, leading to higher potential for settlement (Grossman et al., 2020); a similar study has not yet been conducted in Similk Bay.
Our work involved the creation of two different oyster reestablishment habitats, each with separate goals in mind: (1) 4.6 m² experimental plots and (2) restoration beds ranging in size from 31 m² to 171 m². The experimental plots (as described in Greiner et al., 2015) were established specifically to evaluate growth, recruitment, and survival. The restoration beds were developed to increase Olympia oyster population size and habitat availability by establishing more habitat for oyster use and colonization. Both LT and KI hosted experimental plots and one or more larger restoration bed(s) in separate areas of the lagoons. Tidelands adjacent to the lagoons later became sites of additional intertidal restoration beds, ranging in size from 75 m² to 166 m², to increase habitat availability for future colonization (Table 1).
Table 1. Swinomish Olympia oyster restoration site information including: bed names, year developed, site, area, seeded vs. unseeded cultch, and years seed was added to bed.
Following the success of the pilot efforts in 2012 and 2013 (Barber et al., 2015), SITC received ~364,427 individual hatchery-reared North Sound (Fidalgo Bay) broodstock Olympia oysters from Puget Sound Restoration Fund between 2015 and 2017 to be placed in LT and KI (Table 2). Outplanted size classes, distribution between lagoons and outplanting treatments can be found in McArdle et al. (2022). The plots were only seeded in March of 2016 whereas the beds were seeded throughout multiple years (Table 1). For all plots and beds, we qualitatively noted temporal change in bed perimeter, siltation, and shell movement and loss.
Table 2. Annual Olympia oyster (OO), Ostrea lurida, enhancement statistics for experimental plots and restoration beds combined.
Experimental plots were constructed in 2016 at both LT and KI and consisted of three 4.6 m2 plots, each representing a different treatment: (1) bare substrate (no shell added), (2) seeded cultch (M. gigas shell with Olympia oysters), or (3) unseeded cultch (M. gigas shell with no Olympia oysters) (Greiner et al., 2015). The experimental plots were located in the channel near the lagoon proper at LT or in the lagoon but close to the channel outlet at KI. Each experimental plot, save for the bare substrate plots, received 16 bags of shell (bags = 200-250 M. gigas shells) spread across 4.6 m2. Though not addressed in this paper, these plots were also used to measure change in ecosystem services, another measurable benchmark suggested by Blake and Bradbury (2012) (e.g., Munsch et al., 2021).
Initial restoration beds were centrally located in the lagoons and are referred to as the lagoon restoration beds (Figure 1; Table 1). Lagoon restoration beds were first created in 2013 and expanded in 2015-2017; both seeded and unseeded cultch were spread each of those years to expand both population and habitat.
In the spring of 2018, three restoration beds were established in intertidal areas at approximately 0 m in elevation relative to mean lower low water adjacent to the lagoon outlets. Specifically, two beds were established north of the mouth of the LT channel and one bed was constructed north of the KI channel (Figure 1; Table 1). We refer to these beds as the intertidal restoration beds and all three were established with unseeded cultch only as an attempt to expand habitat. These three beds also provided us with an area to inspect for signs of recruitment.
For both the lagoon and intertidal restoration beds, shell (seeded and/or unseeded) was initially spread at a density that provided about 1-2 shell layer(s) of height (~ 8-10 cm) within a target area of the beach. Total area per bed (for all six beds) was not measured until 2017 (Table 1).
Annually each May, we used 1/16 m2 quadrats to collect data on Olympia oyster height (herein referred to as “shell length”) (mm) and density and to record any signs of recruitment. While we collected the same quadrat data on the experimental plots and the restoration beds, the survey design differed by reestablishment type.
From 2016 through 2019, data collection on the experimental plots consisted of five randomly placed quadrats per seeded and unseeded cultch plots, except in 2017 where 10 quadrats were collected. All emergent substrate and oysters (if present) were collected from each quadrat and oysters were counted and measured in the field with calipers to the nearest 10th of a millimeter. Shell length measurements were made from the umbo to the shell fringe. All contents of the sample were returned to the original sample area when data collection was complete. When sampling the seeded cultch experimental plots, the seed year of 2016 was known and consistent, thus allowing us to track growth and survival of a single cohort, plus any potential recruitment. When sampling the unseeded cultch experimental plots, any recorded oysters would likely be recruits to the unseeded cultch.
From 2015-2017 quadrats were deployed haphazardly within the lagoon restoration beds. From 2018 on, in order to more closely align with methods employed in other Olympia oyster restoration projects, we modified our methods to utilize transects laid out along the longest axis of each bed’s perimeter (Wasson et al., 2015). We utilized systematic random sampling to collect quadrat samples along the transects starting on a random number between 0 - 3 m (using 0.25 m increments) and then sampled quadrats every 3 m from that point until the end of the bed.
In addition to the annual monitoring at LT and KI, starting in 2019, we sampled the intertidal restoration beds that were created in 2018 on the Skagit and Similk Bay tidelands. We utilized the same systematic random quadrat sampling along transects but only to assess recruitment, of which we found none.
For all metrics this study extends through 2019 with the exception of oyster density on lagoon restoration beds, which goes through 2021. Detailed descriptions of all methods are found in Hunter et al. (2021) and McArdle et al. (2022).
We used shell length to ascertain growth, shell length frequency as a proxy for recruitment and for tracking cohort growth patterns, and oyster density to assess survival (Baggett et al., 2014; Blake and Bradbury, 2012).
Since Olympia oysters within the experimental plots originated from the same 2016 cohort, length data were used to discern mean oyster growth. We used a two-way ANOVA and follow-up Tukey tests to determine if oyster length varied by year and site (SYSTAT 13, Sokal and Rohlf, 2012). Despite log transforming length data, we did not meet the assumptions of normality or homogeneity. ANOVA are considered robust even when the data deviate from a normal distribution (Norman, 2010), however, due to the inability to meet the assumption of homogeneity, we adjusted the alpha to 0.01 to protect against Type 1 error (Keppel, 1991).
Restoration bed oyster length data was not used in this growth analysis as a result of being continually reseeded from 2015-2017, thus we were unable to track individual cohorts.
We intended to use data from the unseeded cultch experimental plots and the restoration beds to track oyster recruitment, however, no recruitment was recorded during the time period of this research. While this result is noteworthy, we opted to further explore the possibility of recruitment by plotting shell length frequency by site and year to investigate for signs of recruitment via the histograms. Sampling data from both experimental plots and lagoon restoration beds were combined in these histograms from 2014-2019. Intertidal restoration beds were never seeded and experienced no recruitment, therefore, no shell length frequency data from these beds could be utilized in the histograms.
We used density data (# oysters/m2) from the experimental plots as a proxy for tracking survival of the 2016 cohort by site. We tested for the effects of site, year, and their interaction on oyster density using a two-way ANOVA and follow-up Tukey tests (SYSTAT 13, Sokal and Rohlf, 2012). The assumptions of normality and homogeneity were met for this analysis with untransformed data. Survival rates for each year were calculated by quantifying the change in mean Olympia oyster density per m² from the prior year and converting it to a percentage representing the number of surviving oysters from the prior year.
The addition of shell and seed to the lagoon restoration beds in 2015, 2016, and 2017 meant that density metrics could not be used to accurately assess survival back to 2012 for this oyster bed type. For this reason, only data from 2018-2021 could be utilized for the lagoon restoration bed survival metric. Restoration bed density data were analyzed with log +1 transformed oyster counts using a two-way ANOVA to test the effects of site, year, and their interaction on oyster density in the lagoon restoration beds (SYSTAT 13, Sokal and Rohlf, 2012). Follow-up Tukey tests were used when a significant difference was found. As noted above, ANOVA are considered robust when data deviate from a normal distribution (Norman, 2010) and we adjusted our alpha to 0.01 to protect against Type I error (Keppel, 1991). Lagoon restoration bed survival rates were calculated following the methods described above.
Oyster length differed significantly by site where the LT oysters remained smaller than the KI oysters following the initial seeding year of 2016 (Table 3A; Figure 2). Regardless of site, oyster length increased significantly each year (Table 3; Figure 2, all Tukey HSD pairwise comparisons by year = p < 0.01). There was no interaction between site and year (Table 3A).
Table 3. Two-way ANOVA test statistics for the effects of site and year on oyster length (i.e., growth) and density (i.e., survival).
Figure 2. Olympia oyster shell length (mm) in experimental plots from 2016-2019. (A) Lone Tree Lagoon and (B) Kiket Lagoon.
Although mean growth differed each year, the oysters experienced greater growth in their first full year (post-2016 outplanting) than any subsequent years (Figure 2). For example, LT plot oysters grew an average of 16.15 mm in the initial year compared to a mean growth of 6 mm - 9 mm per year in subsequent years (Figure 2A). Similar to LT, the KI oysters exhibited the greatest growth during the initial year, followed by a decrease in the growth rate to 2019 (Figure 2B). Kiket oyster’s initial mean growth of 20.32 mm in 2016 slowed to a mean growth rate between 5 mm - 9 mm in the following years (Figure 2B).
We found no evidence of recruitment at any of the plots or lagoon or intertidal restoration beds. By combining all size data across oyster plots and lagoon restoration beds, we found that the three outplanted cohorts (2013, 2016, 2017) were clearly visible in the LT and KI sites in 2017 (Figures 3, 4). However, we could not clearly distinguish the 2015 cohort (single oyster seed as opposed to seeded cultch) that was added to the lagoon restoration beds (Figures 3, 4). Qualitatively, we see the progression of growth of these three outplanted cohorts (2013, 2016, and 2017) in both lagoons with no clear evidence of new recruitment in the years following seeding (2018-2019, Figures 3, 4).
Figure 3. Percent frequency of Olympia oyster length (mm) at Lone Tree Lagoon (LT) by year from experimental plots and restoration beds combined. Distinct peaks of populations <10 mm in 2016 and 2017 are direct results of the addition of hatchery-raised seed.
Figure 4. Percent frequency of Olympia oyster length (mm) at Kiket Lagoon (KI) by year from experimental plots and restoration beds combined. Distinct peaks of populations <10 mm in 2016 and 2017 are direct results of the addition of hatchery-raised seed.
Oyster density decreased significantly each year and at each site with no significant interaction term (Table 3B; Figure 5, p < 0.05). Oyster density, and by proxy survival, was significantly lower in KI than in LT (Figure 5). For both sites combined, oyster density differed from 2017 to 2019 and from 2018 to 2019 (Tukey HSD, p < 0.01) but did not differ from 2017 to 2018 (Tukey HSD, p > 0.05). Lone Tree oysters exhibited survival rates of 85.7% from 2017 to 2018 and 56.5% from 2018 to 2019. Kiket oysters exhibited a survival rate of 77.5% from 2017 to 2018, followed by a lower survival rate of 40% from 2018 to 2019 (Figure 5).
Figure 5. Mean density of Olympia oysters in experimental plots at Kiket and Lone Tree from 2017-2019. Standard error bars are shown.
Oyster density at the lagoon restoration beds decreased significantly by site and year (Table 3C; Figure 6, p < 0.01). There was no significant interaction between site and year (Table 3C; Figure 6, p > 0.01). Oyster density decreased significantly across both sites from 2018 to 2021 (Tukey HSD, p < 0.01), but there was no significant difference between other years (Tukey HSD, p > 0.01). While the years were similar to each other except 2018 and 2021, the data exhibit a trend in the decrease in density and, thus, survival of oysters. Lone Tree oyster survival rates were 75.6% (2018-2019), 57.0% (2019-2020), and 50.7% (2020-2021). Kiket oyster survival rates were 40.9% (2018-2019), 26.6% (2019-2020), and 120.9% (2020-2021). The 2020-2021 increase in survival rate fell within the range of the standard error (Figure 6).
Figure 6. Mean density of Olympia oysters in lagoon restoration beds at Kiket and Lone Tree from 2018-2021. Standard error bars are shown.
Our research demonstrates that intensive planning, careful site selection, and subsequent population enhancement do not always result in successful restoration. This outcome serves as an important reminder of the need for consistent long-term monitoring to better understand the successes and failures of restoration projects. Specifically, our results show that while our newly reestablished populations of Olympia oysters in LT and KI are able to increase in shell size, natural recruitment and survival are nonexistent or low, respectively. Because this work and some of our previous work has shown that shell growth occurs and reproduction is successful (Barber et al., 2016; Grossman et al., 2020), we hypothesize that our restoration efforts are hindered by the lower water residence time, the relatively small population size of reintroduced oysters, and the generally small amount of appropriate habitat in the form of hard substrate. The recruitment failure and low survival at our sites, while discouraging, are one of the more important aspects of our work, as comprehensive reports of restoration project successes and failures can be rare despite the value in learning from such efforts (Suding, 2011). Our results demonstrate that even highly intentional and well-planned restoration projects can fail if the surrounding environment remains unfavorable to a species, therefore suggesting that consistent monitoring is necessary to determine future steps rather than curtailing restoration efforts.
Unsurprisingly, our reestablished Olympia oysters grew across all sites and all years. While mean oyster length increased significantly each year, the oysters appeared to grow the most during their first year post-outplanting and then continued growing, but at slower rates in subsequent years. Our first year mean oyster growth rates of 16.15 mm (LT plot) and 20.32 mm (KI plot) appear relatively slow when compared to the 41 mm mean first year growth in Elkhorn Slough, California, USA (Wasson et al., 2020). Furthermore, in their first year of growth following outplanting, Olympia oysters in Willapa Bay and Hood Canal (both in Washington, USA) grew to an average size of 30 mm compared to the average size of 24.9 mm and 19.8 mm from KI and LT plots, respectively (Trimble et al., 2009; Valdez et al., 2017). While these comparisons are interesting, they likely are not ideal because these locations presumably have very different environmental conditions compared to northern Whidbey Basin. A different restoration site in the Salish Sea, Fidalgo Bay, is in closer proximity to our study area although it is located in the San Juan sub-basin. Restoration efforts in Fidalgo Bay began in 2002 and continue to this day with incredibly successful results (e.g., Dinnel et al., 2009; Dinnel, 2023). Dinnel et al. (2009) reported that between May 2002 and August 2003, oysters grew an average of 15.6 mm (15 months), reflecting growth rates that are more similar to our reported growth results.
Consistent with what was reported in the pilot study, the KI oysters grew larger than their counterparts at LT (Barber et al., 2015). While the KI oysters appeared to grow faster than LT oysters, there was no interaction term indicating if one year resulted in higher oyster growth by site than another year. Significant oyster growth, regardless of year or site, demonstrates the likely role of environmental factors specific to both sites having an impact on oyster growth. These site differences could be a result of LT Olympia oysters experiencing slightly higher salinity stress due to freshwater input from the nearby Skagit River as well as the ephemeral stream that feeds into the lagoon (Beamer et al., 2006). Overall, our growth results reflect the fact that both sites likely support favorable growing conditions such as available food supply and dissolved oxygen (Wasson et al., 2015). Although our growth rates could appear slow compared to other restoration areas in California or Washington, comparable growth results from nearby Fidalgo Bay indicate that this particular region may simply produce slower growing oysters.
The apparent lack of natural recruitment to any of our experimental plots or lagoon or intertidal restoration beds is disappointing and a potential threat to the long-term success of our restoration project. Recruitment failure is common enough in Olympia oysters that Wasson et al. (2016) was able to demonstrate that factors such as temperature, salinity, and residence time are related to recruitment failure across the range of this species. Furthermore, Ridlon et al. (2021a) determined lack of successful recruitment as one of the top three challenges facing native oyster restoration projects along the West Coast. Importantly, SITC data used in Ridlon et al.’s (2021a) analysis shows an increase in adult oysters on substrate at our sites; while the calculation is true the increase recorded is solely due to continued population enhancement across multiple years and not natural recruitment.
While we know Olympia oysters once historically occupied the Similk Bay area (Blake and Bradbury, 2012; Kornbluth et al., 2022), outplanting native oysters in a region where they once existed does not always guarantee success. Although Wasson et al. (2016) found that Olympia oyster recruitment failure was often linked to lower summer water temperature and higher winter salinity, we do not believe these factors played as large of a role in our recruitment failure due to the evidence of sufficient brooding in our restored population as well as higher summer water temperature and lower winter salinity in Whidbey Basin relative to Puget Sound conditions (Barber et al., 2016; Swinomish Fisheries Department, unpublished data). More plausible reasons for lack of recruitment in our region include, but are not limited to: insufficient adult populations and consequently low larval density (Ridlon et al., 2021b; Wasson et al., 2016), low residence time in nearby waters (Wasson et al., 2016; Peteiro and Shanks, 2015; Kimbro et al., 2019), and limited availability of suitable habitat (Groth and Rumrill, 2009).
Although we know reproduction is occurring at LT and KI (Barber et al., 2016; Grossman et al., 2020), our adult populations are relatively small and may not be capable of producing high enough densities of larvae for productive recruitment [e.g., Wasson et al. (2015) notes that high adult densities can lead to significant recruitment]. As of 2017, we estimate that we have outplanted a total of ~515,700 oysters between our two sites (Table 2). Conversely, scientists estimate that the successful restoration project in nearby Fidalgo Bay had just under 3 million Olympia oysters in 2018 (Dinnel, 2018). Because there is some evidence that the presence of conspecifics may play a role in successful settlement of larvae (Becker et al., 2020), the adult populations in Skagit and Similk Bays may not be large enough to produce settlement cues. Furthermore, restoration areas with populations exceeding 1 million adults have been known to experience regular recruitment (Dinnel, 2018, 2023; PSRF, 2017). In contrast, Wasson et al. (2020) demonstrated an absence of recruitment in California’s Elkhorn Slough with much smaller populations (~1,000 oysters as of 2020). Additionally, recruitment failure is known to be more common when there are few networks between adult Olympia oysters in estuaries (Wasson et al., 2016). Indeed, our two primary restoration sites are likely not well connected oceanographically, making it harder for larvae from Similk Bay to travel to Skagit Bay or vice versa. These various results suggest that we may need to bolster our adult oyster population in order to produce more larvae to improve chances of successful recruitment.
It is also possible that circulation, particularly low residence time, played a role in exporting larvae away from the restoration areas to regions with unsuitable habitat, causing local recruitment failure (Peteiro and Shanks, 2015; Pritchard et al., 2015; Wasson et al., 2016). Grossman et al. (2020) found that larvae originating in the LT lagoon were more likely to be retained offshore or at intertidal locations, rather than in the lagoon from which they originated. Larvae were also more likely to be exported from LT during an ebb tide, which could have resulted in dispersal to areas of lower residence time or moved the larvae into a small alongshore northward gyre with more favorable retention time (Grossman et al., 2020). Prior to 2018, the tidelands located in this more favorable gyre lacked significant acreage of hard substrate (i.e., appropriate habitat for settling larvae). Yet, we failed to record any recruitment even once unseeded cultch was installed at the two small intertidal restoration beds in Skagit Bay. It is plausible these intertidal beds were still too small in area for competent larvae to detect (Table 1).
The lack of extensive suitable habitat in our surrounding embayments is concerning because the presence of appropriate habitat is an important factor in successful recruitment (White et al., 2009b; Hopkins, 1937). Because utilization of available habitat and colonization is one of the metrics that Blake and Bradbury (2012) suggest measuring to determine restoration success, our project is currently not meeting this metric due to the fact that very little hard substrate exists in the area for settling larvae. Research in Fidalgo Bay demonstrated that the majority of new recruits were found in close proximity to the adult population (Dinnel, 2018; Becker et al., 2020). Oyster larvae of other species have also been shown to cue into habitat-associated sounds of oyster reefs when attempting to locate suitable habitat for settlement (Lillis et al., 2014). Finally, Olympia oysters are known to recruit to various types of hard surfaces and not just oyster shell (Groth and Rumrill, 2009), but many of the coarse sand and gravel beaches near our restoration sites lack hard surfaces almost entirely. Thus, our project’s presumed limitations in larval density were likely compounded by a lack of suitable habitat. In more recent years (2018 through current), we have transitioned our priority sites for habitat enhancement away from the lagoons (which lack available substrate and have demonstrated the inability to retain larvae) to areas in the nearshore which are known to be in close proximity to competent larvae in the water column (Grossman et al., 2020). A clear need for future population expansion in our region is to increase suitable hard bottom habitat, such as adding more Pacific oyster shell to beaches, ideally in concert with increasing adult populations.
It is not surprising that oyster density, used as a proxy to determine sustainable survival, declined when we found no evidence of recruitment to replenish the population. Olympia oysters do not have a known maximum lifespan, but it has been suggested they may live over 10 years (Couch and Hassler, 1989; Baker, 1995). The majority of our oysters, however, are on a trajectory for much shorter lifespans. The mortality of newly outplanted oysters seen in our experimental plots in our first year of data collection was consistent with previously reported mortality rates in nearby areas (14.3% at LT plots and 22.5% at KI plots compared to 22.7% in the early years of Fidalgo Bay restoration) (Dinnel et al., 2005). Our data were also in agreement with mortality rates recorded in year one oysters (9.6% - 28.2%) elsewhere in the region (Gibson, 1974) and our oysters fared better than oysters in eelgrass beds in Hood Canal, Washington (~1% survival in the first year; Valdez et al., 2017). However, our mortality rates from 2018 – 2019 were higher than what the literature reports for > 2 year old oysters in both Fidalgo Bay (Dinnel et al., 2005) and Elkhorn Slough (Wasson et al., 2020). We believe environmental conditions including salinity, temperature, and/or sedimentation, could be responsible for the increased mortality rates in adults.
Low salinity does seem like a plausible explanation for our lower survival rates, specifically due to the relatively low salinities found at KI (9.3 – 25.6) and LT (15.3 – 26.9) (Barber et al., 2016). However, while salinity ranges below 25 have been shown to negatively affect oyster size and recruitment, effect on survival should be minimal since Olympia oysters have been shown to tolerate lower salinity ranges (reviewed in Wasson et al., 2015). Prolonged exposure time to even the fringes of these salinity conditions can act as a stressor (Wasson, 2010), but would seem unlikely to be a main driver in our mortality rates. Temperature may also be a contributing factor to lower survival rates, especially in concert with lower salinities and sedimentation. Water temperatures below 10°C and above 38°C are known to have negative effects on Olympia oysters (Hopkins, 1937; Davis, 1955; Wasson et al., 2015). Additionally, Wasson et al. (2015) demonstrated high air temperature resulted in oyster mortality. Our water temperature range of 9.2 - 29.3°C (combined for LT and KI) was within the margin to stress the animal, but not extreme enough to be a leading culprit for reduced survival. Moreover, the lagoon sites of the KI and LT populations are always inundated regardless of tide height, providing a buffer to protect oysters from extreme summer and winter air temperatures that intertidal populations might be exposed to.
Thus, while temperature and salinity are likely to have an impact on mortality, we suspect it may be minimal at these sites. Instead, we suggest sedimentation is the predominant driver of our higher mortality rates. Sedimentation has been shown to be a significant cause for decreased survival at other restoration sites and is one of the top three challenges facing restoration projects of this species (as reviewed in Ridlon et al., 2021a). Sedimentation is certainly occurring at both of our sites, and is worse at KI where survival was lower. The KI site contains a high percentage of fine sediments and experiences low current flow; we observed KI oysters both sinking into the substrate over time and being buried into an anoxic sediment layer by the deposition of fine sediments over the top of the plots and beds (SITC Fisheries, unpublished data).
We have demonstrated that Olympia oysters sourced from hatchery seed are able to grow, survive, and successfully reproduce in the waters of northern Whidbey Basin (Barber et al., 2015, 2016; Grossman et al., 2020; McArdle et al., 2022). However, the concerning lack of natural recruitment has forced us to reevaluate our long-term restoration plans and consider alternative locations and restoration methods. As shown in Kimbro et al. (2019), Olympia oyster population dynamics are not driven by one singular factor. While we did not model Olympia oyster population dynamics in our region, recognizing the importance of local conditions and habitat on restoration outcomes, we have utilized our intimate knowledge of the region’s oceanographic properties and information from the literature to ascertain factors that may be influencing our small populations of restored oysters (Coen and Luckenbach, 2000). Importantly, recruitment failure need not lead restoration practitioners to cease their restoration activities. Instead, we can utilize regional knowledge, our monitoring data, and information in the published literature to develop better-informed steps for future restoration work.
In our case, it is highly plausible that the water residence time at our selected restoration sites is too low and we should focus future restoration efforts on areas with higher residence time. Specifically, we assume that the terminal end of elongate embayments like the far northeastern end of Similk Bay would have longer residence times than the more centrally-located area of our current restoration sites in Similk and Skagit Bays (Figure 1). Interestingly, the historic documentation of Olympia oysters in this region were from the northwestern portion of Similk Bay, indicating that the northern part of the embayment may be more suitable for successful recruitment (Blake and Bradbury, 2012; Kornbluth et al., 2022). More successful restoration projects in Washington, such as Fidalgo, Liberty, Sequim, and Discovery Bays, all have oyster populations located at the extremities of long embayments or in similarly protected areas. The location of these successful projects further supports our decision to target areas in northern Similk Bay for future restoration.
Even if we refocus efforts on new project areas, there is still a clear need to increase local source populations and expand habitat. It may also be prudent to focus future efforts on one larger restoration site rather than splitting effort between two smaller sites with limited connectivity. Indeed, recent work by Ridlon et al. (2021b) highlights our region in Whidbey Basin as one of the top 10 sites that may benefit more from the rewards of conservation aquaculture over the risks. Although this project began via the use of conservation aquaculture (i.e., hatchery-reared oysters), we had planned to expand our initial populations via natural reproduction and recruitment, a goal not currently realized based on these results. With conservation aquaculture in mind as a potential back-up tool, we first propose attempting to minimize the risks associated with conservation aquaculture (as reviewed in Ridlon et al., 2021b) by adopting unique strategies to (1) increase adult populations and subsequent presumed larval pool, (2) increase optimal settlement habitat in the lower intertidal, and (3) broaden the spatial scale of the restoration area. As we continue toward our goal of restoring self-sustaining populations of Olympia oysters in northern Whidbey Basin, we will continue to monitor the status of our restored beds and employ novel restoration techniques.
The original contributions presented in the study are included in the article. Further inquiries can be directed to the corresponding author.
The manuscript presents research on animals that do not require ethical approval for their study.
JM: Conceptualization, Data curation, Formal analysis, Funding acquisition, Methodology, Resources, Validation, Visualization, Writing – original draft, Writing – review & editing. JB: Conceptualization, Formal analysis, Funding acquisition, Methodology, Project administration, Resources, Supervision, Visualization, Writing – original draft, Writing – review & editing. SG: Conceptualization, Data curation, Funding acquisition, Methodology, Resources, Visualization, Writing – review & editing. LH: Data curation, Methodology, Resources, Writing – review & editing.
The author(s) declare financial support was received for the research, authorship, and/or publication of this article. This work was supported by Conservation, Research and Education Opportunities International, the United States Fish and Wildlife Service Tribal Wildlife Grant (Fl4AP00495), U.S. Environmental Protection Agency (PA-00J322-01) and does not reflect the views of the granting agencies.
We thank the Swinomish Senate and Fish and Game Commission for the continued support of this work. Lorraine Loomis always encouraged and guided our work; this publication is written in her memory and serves as a testament of her tireless work and advocacy for tribes and protecting treaty resources. We would like to thank T. Wilbur, B. Blake, L. Clifton, C. Cook, P. Dinnel, J. Gibson, C. Greiner, and J. Jannetta for their assistance on this project. Additionally, we would like to extend our gratitude to M. Nelson for his assistance with figure design. The Puget Sound Restoration Fund provided SITC with the oysters needed to start and build our enhancement project.
The authors declare that the research was conducted in the absence of any commercial or financial relationships that could be constructed as a potential conflict of interest.
JB declared that she was a guest associate editor of Frontiers in Marine Science at the time of submission.
All claims expressed in this article are solely those of the authors and do not necessarily represent those of their affiliated organizations, or those of the publisher, the editors and the reviewers. Any product that may be evaluated in this article, or claim that may be made by its manufacturer, is not guaranteed or endorsed by the publisher.
Baggett L. P., Powers S. P., Brumbaugh R., Coen L. D., DeAngelis B., Greene J., et al. (2014). Oyster habitat restoration monitoring and assessment handbook (Arlington, VA, USA: The Nature Conservancy), 96pp.
Baggett L. P., Powers S. P., Brumbaugh R. D., Coen L. D., DeAngelis B. M., Greene J. K., et al. (2015). Guidelines for evaluating performance of oyster habitat restoration. Restor. Ecol. 23, 737–745. doi: 10.1111/rec.12262
Baker P. (1995). Review of ecology and fishery of the Olympia oyster, Ostrea lurida, with annotated bibliography. J. Shellfish Res. 14, 501–518.
Barber J. S., Dexter J. E., Grossman S. K., Greiner C. M., McArdle J. T. (2016). Low temperature brooding of Olympia oysters (Ostrea lurida) in northern Puget Sound. J. Shellfish Res. 35, 351–357. doi: 10.2983/035.035.0209
Barber J. S., Greiner C. M., Grossman S. K. (2015). “Olympia oyster, Ostrea lurida, pilot project in northern Puget Sound, Washington: 2014 monitoring report,” in Swinomish Indian Tribal Community Technical Report SWIN-TR-2015-01 (La Conner, WA: Swinomish Indian Tribal Community), 14 pp.
Beamer E., McBride A., Henderson R., Griffith J., Fresh K., Zackey T., et al. (2006). Habitat and fish use of pocket estuaries in the Whidbey Basin and north Skagit County bays 2004 and 2005 (La Conner, Washington: Skagit River System Cooperative).
Beamer E., McBride A., Henderson R., Wolf K. (2003). The importance of non-natal pocket estuaries in Skagit Bay to wild Chinook salmon: An emerging priority for restoration (La Conner, Washington: Skagit River System Cooperative).
Beck M. W., Brumbaugh R. D., Airoldi L., Carranza A., Coen L. D., Crawford C., et al. (2011). Oyster reefs at risk and recommendations for conservation, restoration, and management. Bioscience 61, 107–116. doi: 10.1525/bio.2011.61.2.5
Becker B. J., Behrens M. D., Allen B., Hintz M., Parker H., McCartha M. M., et al. (2020). Spatial and temporal distribution of the early life history stages of the native Olympia oyster Ostrea lurida (Carpenter 1864) in a restoration site in northern Puget Sound, WA. J. Shellfish Res. 39, 43. doi: 10.2983/035.039.0105
Blake B., Bradbury A. (2012). Washington Department of Fish and Wildlife plan for rebuilding Olympia oyster (Ostrea lurida) populations in Puget Sound with a historical and contemporary overview (1000 Point Whitney Road, Brinnon, WA 98230: Washington Department of Fish and Wildlife, Point Whitney Shellfish Laboratory). 26 pp.
Brumbaugh R. D., Beck M. W., Coen L. D., Craig L., Hicks P. (2006). A practitioners’ guide to the design and monitoring of shellfish restoration projects: An ecosystem services approach. Nat. Conservancy.
Carranza A., Defeo O., Beck M. (2009). Diversity, conservation status and threats to native oysters (Ostreidae) around the Atlantic and Caribbean coasts of South America. Aquat. Conserv: Mar. Freshw. Ecosyst. 19, 344–353. doi: 10.1002/aqc.993
Coen L. D., Brumbaugh R. D., Bushek D., Grizzle R., Luckenbach M. W., Posey M. H., et al. (2007). Ecosystem services related to oyster restoration. Mar. Ecol. Prog. Ser. 341, 303–307. doi: 10.3354/meps341303
Coen L. D., Luckenbach M. W. (2000). Developing success criteria and goals for evaluating oyster reef restoration: Ecological function or resource exploitation? Ecol. Eng. 15, 323–343. doi: 10.1016/S0925-8574(00)00084-7
Couch D., Hassler T. J. (1989). Species profiles: Life histories and environmental requirements of coastal fishes and invertebrates (Pacific Northwest)–Olympia oyster. U.S. Fish Wildl. Serv. Biol. Rep. TR EL-82-4 82, 1–8.
Davis H. C. (1955). Mortality of Olympia oysters at low temperatures. Biol. Bull. 109, 404–406. doi: 10.2307/1539172
Dinnel P. A. (2018). Restoration of the Olympia oyster, Ostrea lurida, in Fidalgo Bay and Cypress Island. Skagit Mar. Resour. Committee Rep., 74 pp.
Dinnel P. A. (2023). Restoration of the Olympia oyster, Ostrea lurida, in Fidalgo Bay: Year twenty-one report. Skagit Mar. Resour. Committee Rep., 52 pp.
Dinnel P. A., Peabody B., Peter-Contesse T. (2009). Rebuilding Olympia oysters, Ostrea lurida Carpenter 1864, in Fidalgo Bay, Washington. J. Shellfish Res. 28, 79–85. doi: 10.2983/035.028.0114
Dinnel P., Schwertner M., Barsh R., Robinette J., Dolph I., Giboney J., et al. (2005). Restoration of the native oyster in Fidalgo Bay year three report (Mount Vernon, WA: Northwest Straits Marine Conservation Initiative & Skagit MRC).
Fitzsimons J. A., Branigan S., Gillies C. L., Brumbaugh R. D., Cheng J., DeAngelis B. M., et al. (2020). Restoring shellfish reefs: Global guidelines for practitioners and scientists. Conserv. Sci. Pract. 2, e198. doi: 10.1111/csp2.198
Gibson G. G. (1974). Oyster mortality study summary report 1966-1972 (ed. U.S. Department of Commerce National Marine Fisheries Service Fish Commission of Oregon Management and Research Division).
Grabowski J. H., Peterson C. H. (2007). Restoring oyster reefs to recover ecosystem services. Theoretical Ecology Series, Editor(s) Cuddington K., Byers J. E., Wilson W. G., Hastings .. (Academic Press) 4, 281–298. doi: 10.1016/S1875-306X(07)80017-7
Greiner C. M., Grossman S. K., Barber J. S. (2015). “Swinomish Olympia oyster monitoring plan,” in Swinomish Indian Tribal Community Contribution SWIN-CR-2015-04 (La Conner, WA: Swinomish Indian Tribal Community), 21 pp.
Grossman S. K., Grossman E. E., Barber J. S., Gamblewood S. K., Crosby S. C. (2020). Distribution and transport of Olympia oyster Ostrea lurida larvae in northern Puget Sound, Washington. J. Shellfish Res. 39, 215–233. doi: 10.2983/035.039.0204
Groth S., Rumrill S. (2009). History of Olympia oysters (Ostrea lurida Carpenter 1864) in Oregon estuaries, and a description of recovering populations in Coos Bay. J. Shellfish Res. 28, 51–58. doi: 10.2983/035.028.0111
Hatch M. B. A., Wyllie-Echeverria S. (2016). Historic distribution of Ostrea lurida (Olympia oyster) in the San Juan Archipelago, Washington State. Tribal Coll. Univ. Res. J. 1, 38–45.
Hemraj D. A., Bishop M. J., Hancock B., Minuti J. J., Thurstan R. H., Zu Ermgassen P. S. E., et al. (2022). Oyster reef restoration fails to recoup global historic ecosystem losses despite substantial biodiversity gain. Sci. Adv. 8, eabp8747. doi: 10.1126/sciadv.abp8747
Hopkins A. E. (1937). Experimental observations on spawning, larval development, and setting in the Olympia oyster Ostrea lurida (Washington, D.C: United States Government Printing Office).
Hunter L., McArdle J. T., Grossman S. K., Barber J. S. (2021). “Olympia oyster research protocols,” in Swinomish Indian Tribal Community Contribution SWIN-CR-2021-04 (La Conner, WA: Swinomish Indian Tribal Community), 52.
Keppel G. (1991). Design and analysis: A researcher’s handbook. 3rd ed (Englewood Cliffs, N.J: Prentice Hall).
Khangaonkar T., Yang Z., Kim T., Roberts M. (2011). Tidally averaged circulation in Puget Sound sub-basins: Comparison of historical data, analytical model, and numerical model. Estuar. Coast. Shelf Sci. 93, 305–319. doi: 10.1016/j.ecss.2011.04.016
Kimbro D. L., White J. W., Grosholz E. D. (2019). The dynamics of open populations: Integration of top–down, bottom–up and supply–side influences on intertidal oysters. Oikos 128, 584–595. doi: 10.1111/oik.05892
Kirby M. X. (2004). Fishing down the coast: historical expansion and collapse of oyster fisheries along continental margins. Proc. Natl. Acad. Sci. U.S.A. 101, 13096–13099. doi: 10.1073/pnas.0405150101
Kornbluth A., Perog B. D., Crippen S., Zacherl D., Quintana B., Grosholz E. D., et al. (2022). Mapping oysters on the Pacific coast of North America: A coast-wide collaboration to inform enhanced conservation. PloS One 17, e0263998. doi: 10.1371/journal.pone.0263998
Lillis A., Eggleston D. B., Bohnenstiehl D. R. (2014). Soundscape variation from a larval perspective: The case for habitat-associated sound as a settlement cue for weakly swimming estuarine larvae. Mar. Ecol. Prog. Ser. 509, 57–70. doi: 10.3354/meps10917
Lipcius R. N., Burke R. P., McCulloch D. N., Schreiber S. J., Schulte D. M., Seitz R. D., et al. (2015). Overcoming restoration paradigms: Value of the historical record and metapopulation dynamics in native oyster restoration. Front. Mar. Sci. 2. doi: 10.3389/fmars.2015.00065
Mann R., Powell E. N. (2007). Why oyster restoration goals in the Chesapeake Bay are not and probably cannot be achieved. J. Shellfish Res. 26, 905–917. doi: 10.2983/0730-8000(2007)26[905:WORGIT]2.0.CO;2
McArdle J. T., Grossman S. K., Hunter L., Barber J. S. (2022). “Olympia oyster, Ostrea lurida, restoration in Similk and Skagit Bays, Washington: 2019 monitoring report,” in Swinomish Indian Tribal Community Technical Report SWIN-TR-2022-02, 22 pp.
Munsch S. H., Barber J. S., Cordell J. R., Kiffney P. M., Sanderson B. L., Toft J. D. (2021). Small invertebrates in bivalve-cultivated and unmodified habitats of nearshore ecosystems. Hydrobiologia. 848, 1249–1265. doi: 10.1007/s10750-021-04520-1
Norman G. (2010). Likert scales, levels of measurement and the “laws” of statistics. Adv. Health Sci. Educ. Theory Pract. 15, 625–632. doi: 10.1007/s10459-010-9222-y
Peteiro L. G., Shanks A. L. (2015). Up and down or how to stay in the bay: Retentive strategies of Olympia oyster larvae in a shallow estuary. Mar. Ecol. Prog. Ser. 530, 103–117. doi: 10.3354/meps11283
Polson M. P., Zacherl D. C. (2009). Geographic distribution and intertidal population status for the Olympia oyster, Ostrea lurida Carpenter 1864, from Alaska to Baja. J. Shellfish Res. 28, 69–77. doi: 10.2983/035.028.0113
Powers S. P., Peterson C. H., Grabowski J. H., Lenihan H. S. (2009). Success of constructed oyster reefs in no-harvest sanctuaries: Implications for restoration. Mar. Ecol. Prog. Ser. 389, 159–170. doi: 10.3354/meps08164
Pritchard C., Shanks A., Rimler R., Oates M., Rumrill S. (2015). The Olympia oyster Ostrea lurida: Recent advances in natural history, ecology, and restoration. J. Shellfish Res. 34, 259–271. doi: 10.2983/035.034.0207
PSRF (2017). Final report Port Gamble Olympia oyster restoration (Bainbridge Island, WA: Puget Sound Restoration Fund), 26 pp.
Reeder-Myers L., Braje T. J., Hofman C. A., Elliott Smith E. A., Garland C. J., Grone M., et al. (2022). Indigenous oyster fisheries persisted for millennia and should inform future management. Nat. Commun. 13, 2383. doi: 10.1038/s41467-022-29818-z
Ridlon A. D., Marks A., Zabin C. J., Zacherl D., Allen B., Crooks J., et al. (2021a). Conservation of marine foundation species: Learning from native oyster restoration from California to British Columbia. Estuaries Coast. 44, 1723–1743. doi: 10.1007/s12237-021-00920-7
Ridlon A. D., Wasson K., Waters T., Adams J., Donatuto J., Fleener G., et al. (2021b). Conservation aquaculture as a tool for imperiled marine species: Evaluation of opportunities and risks for Olympia oysters, Ostrea lurida. PloS One 16, e0252810. doi: 10.1371/journal.pone.0252810
Shipman H. (2008). A geomorphic classification of Puget Sound nearshore landforms. U.S. Army Corps Engineers.
Smith R. S., Cheng S. L., Castorani M. C. N. (2022). Meta-analysis of ecosystem services associated with oyster restoration. Conserv. Biol. 37, e13966. doi: 10.1111/cobi.13966
Sokal R. R., Rohlf F. J. (2012). Biometry: The principles and practice of statistics in biological research. 4th ed (New York: W.H. Freeman). Extensively rev.
Sponaugle S., Cowen R. K., Shanks A., Morgan S., Leis J., Pineda J., et al. (2002). Predicting self-recruitment in marine populations: Biophysical correlates and mechanisms. Bull. Mar. Sci. 70, 341–375.
Steele E. N. (1957). The rise and decline of the Olympia oyster (Elma, Washington: Fulco Publications).
Suding K. N. (2011). Toward an era of restoration in ecology: Successes, failures, and opportunities ahead. Annu. Rev. Ecol. Evol. Syst. 42, 465–487. doi: 10.1146/annurev-ecolsys-102710-145115
Trimble A. C., Ruesink J. L., Dumbauld B. R. (2009). Factors preventing the recovery of a historically overexploited shellfish species, Ostrea lurida Carpenter 1864. J. Shellfish Res. 28, 97–106. doi: 10.2983/035.028.0116
Valdez S. R., Peabody B., Allen B., Blake B., Ruesink J. L. (2017). Experimental test of oyster restoration within eelgrass. Aquat. Conserv: Mar. Freshw. Ecosyst. 27, 578–587. doi: 10.1002/aqc.2722
Walles B., Troost K., van den Ende D., Nieuwhof S., Smaal A. C., Ysebaert T. (2016). From artificial structures to self-sustaining oyster reefs. J. Sea Res. 108, 1–9. doi: 10.1016/j.seares.2015.11.007
Wasson K. (2010). Informing Olympia oyster restoration: Evaluation of factors that limit populations in a California estuary. Wetlands 30, 449–459. doi: 10.1007/s13157-010-0056-4
Wasson K., Gossard D. J., Gardner L., Hain P. R., Zabin C. J., Fork S., et al. (2020). A scientific framework for conservation aquaculture: A case study of oyster restoration in central California. Biol. Conserv. 250, 108745. doi: 10.1016/j.biocon.2020.108745
Wasson K., Hughes B. B., Berriman J. S., Chang A. L., Deck A. K., Dinnel P. A., et al. (2016). Coast-wide recruitment dynamics of Olympia oysters reveal limited synchrony and multiple predictors of failure. Ecology 97, 3503–3516. doi: 10.1002/ecy.1602
Wasson K., Zabin C., Bible J., Briley S., Ceballos E., Chang A., et al. (2015). A guide to Olympia oyster restoration and conservation: Environmental conditions and sites that support sustainable populations (San Francisco, California: Elkhorn Slough National Estuarine Research Reserve).
White J. M., Buhle E. R., Ruesink J. L., Trimble A. C. (2009b). Evaluation of Olympia oyster (Ostrea lurida Carpenter 1864) status and restoration techniques in Puget Sound, Washington, United States. J. Shellfish Res. 28, 107–112. doi: 10.2983/035.028.0101
White J., Ruesink J. L., Trimble A. C. (2009a). The nearly forgotten oyster: Ostrea lurida Carpenter 1864 (Olympia oyster) history and management in Washington state. J. Shellfish Res. 28, 43–49. doi: 10.2983/035.028.0109
Keywords: Olympia oyster, Ostrea lurida, growth, habitat, Salish Sea, recruitment, restoration, survival
Citation: McArdle JT, Barber JS, Grossman SK and Hunter LL (2025) Planning for success but facing uncertainty: lessons learned from a native oyster, Ostrea lurida, restoration project in the Salish Sea. Front. Mar. Sci. 11:1462326. doi: 10.3389/fmars.2024.1462326
Received: 09 July 2024; Accepted: 16 December 2024;
Published: 22 January 2025.
Edited by:
Ibon Galparsoro, Technological Center Expert in Marine and Food Innovation (AZTI), SpainReviewed by:
Alfonso Aguilar-Perera, Universidad Autónoma de Yucatán, MexicoCopyright © 2025 McArdle, Barber, Grossman and Hunter. This is an open-access article distributed under the terms of the Creative Commons Attribution License (CC BY). The use, distribution or reproduction in other forums is permitted, provided the original author(s) and the copyright owner(s) are credited and that the original publication in this journal is cited, in accordance with accepted academic practice. No use, distribution or reproduction is permitted which does not comply with these terms.
*Correspondence: James T. McArdle, am1jYXJkbGVAc3dpbm9taXNoLm5zbi51cw==
Disclaimer: All claims expressed in this article are solely those of the authors and do not necessarily represent those of their affiliated organizations, or those of the publisher, the editors and the reviewers. Any product that may be evaluated in this article or claim that may be made by its manufacturer is not guaranteed or endorsed by the publisher.
Research integrity at Frontiers
Learn more about the work of our research integrity team to safeguard the quality of each article we publish.