- 1Chinese Sturgeon Research Institute, China Three Gorges Corporation, Yichang, Hubei, China
- 2Hubei Key Laboratory of Three Gorges Project for Conservation of Fishes, Yichang, Hubei, China
- 3National Pathogen Collection Center for Aquatic Animals, Shanghai Ocean University, Shanghai, China
- 4Marine Science and Engineering, Qingdao Agricultural University, Qingdao, Shandong, China
The study focuses on the critically endangered Dabry’s sturgeon (Acipenser dabryanus), a species on the brink of extinction in the wild. This research investigates the role of the Forkhead box protein L2 (foxl2) in the gonadal development and differentiation of this species. The foxl2 gene, known for its role in various physiological processes, including sexual maturation, is hypothesized to play a significant role in the sex differentiation of Dabry’s sturgeon. This study cloned the full-length cds sequence of the foxl2 gene and analyzed its expression across various tissues, focusing on its response to estradiol treatment. Our findings indicate that foxl2 is predominantly expressed in ovaries and shows a dose-dependent response to estradiol, suggesting its potential role in ovarian differentiation. This research underscores the importance of foxl2 in understanding reproductive biology and offers a foundation for future conservation strategies.
1 Introduction
Dabry’s sturgeon (Acipenser dabryanus), belongs to the Actinopterygii class and the Acipenseridae family (Zhuang et al., 1997). This species predominantly inhabits the upper reaches of the Yangtze River and its tributaries (Zhang et al., 2011). Recognized as a living fossil (Zhang et al., 2012), it was classified as critically endangered by the International Union for Conservation of Nature (IUCN) in 2010 (Wei et al., 1997).
Sexual maturity in Dabry’s sturgeon is typically reached within 4-6 years for males and 6-8 years for females (Zhuang et al., 1997). As for its dietary habits, Dabry’s sturgeon is omnivorous, although food preferences differ between juvenile and adult stages. Overfishing, dam construction, and habitat degradation have resulted in a dramatic decrease in the natural population of Dabry’s sturgeon, with the species nearing extinction in the wild within the Yangtze River (Li et al., 2015).
Efforts to aid the recovery of this endangered species have included the establishment of a national nature reserve, controlled reproduction, and the release of cultured juveniles (Zhang et al., 2011). While the exact location of the natural spawning ground of Dabry’s sturgeon remains unknown, it is believed to be situated in the lower reaches of the Jinsha River, below Maoshui, with feeding grounds located upstream of the Yangtze River (Zhuang et al., 1997).
Fishing of Dabry’s sturgeon was outlawed in 1982. Between 1984 and 1993, 124 Dabry’s sturgeon were inadvertently caught in the Luzhou section of the upper Yangtze River, with an additional 27 caught in the Yibin section between 1994 and 1996. From 2006 to 2010, a total of 39 Dabry’s sturgeon were identified in the upper Yangtze River (Du and Wei, 2016). However, sightings of wild individuals in the upper Yangtze River have been sparse since 2010. Monitoring data suggest that natural breeding of wild Dabry’s sturgeon has not occurred since 2000 (Du and Wei, 2016; Zhang et al., 2020). Consequently, all Dabry’s sturgeon currently residing in the natural waters of the Yangtze River are a result of artificial propagation and release. As such, captive breeding may represent the final opportunity for the survival and sustainability of Dabry’s sturgeon.
Despite progress in our understanding, there is still a lack of standardized methodologies for investigating gonadal development and differentiation. The timing of gonadal sex differentiation is not only late but also varies among species. Hypothetically, genes implicated in sex differentiation may not be active or expressed in the gonads of mature females or males, but rather in immature individuals. The specific mechanism underlying the gonadal differentiation and development in Yangtze Sturgeon remains unreported, posing a significant obstacle to the effective conservation and artificial propagation of Dabry’s sturgeon.
In an effort to facilitate long-term conservation strategies, there is an emerging focus on molecular breeding research for this species. Consequently, a comprehensive investigation into the sex-differentiation mechanisms and reproductive biology of Dabry’s sturgeon is paramount for the sustainable management and preservation of this critically endangered species.
The Forkhead box (Fox) proteins, typified by a forkhead (FH) domain, play integral roles in various physiological processes, including embryogenesis, sexual maturation, and cellular differentiation (Georges et al., 2014; Shen and Wang, 2014). The Forkhead box protein L2 (foxl2), a constituent of the Fox gene family, serves as a pivotal transcriptional regulatory factor. Its significant roles in various physiological processes, particularly in gonadal differentiation and development, have been extensively documented across a broad spectrum of organisms, ranging from invertebrates to vertebrates (Wan et al., 2021).
Foxl2 contributes to the inhibition of follicular apoptosis and the preservation of ovarian reserve function, and has been implicated in sex determination, premature ovarian failure, infertility, neoplasms, and Blepharophimosis syndrome (Liu et al., 2016; Wu et al., 2018). For instance, the ratio of Log10 (DMRT1L/foxl2) derived from two molecular markers for testes and ovaries has been utilized to ascertain the molecular timing of sex differentiation in Yesso scallop (Li et al., 2018).
In vertebrates, foxl2 deletion in mice precipitates abnormal ovarian granulosa cell development, leading to follicular arrest and oocyte atresia (Schmidt et al., 2004). Moreover, homozygous mutations in the foxl2 gene impede the completion of squamous transformation in ovarian granulosa cells, resulting in the absence of secondary follicles and oocyte atresia in mice, thereby underscoring the indispensability of the foxl2 gene in ensuring granulosa cell differentiation and ovarian maintenance (Schmidt et al., 2004). foxl2 has also been implicated in the differentiation of granulosa cells in the ovaries of Oryzias latipes (Nakamoto et al., 2006). In Clarias fuscus, foxl2 expression peaks prior to gonadal differentiation and then significantly decreases post-differentiation (Deng et al., 2015).
In Oreochromis niloticus, foxl2 gene mutations have been associated with a significant upregulation of male differentiation-related gene expression (Sfl, Dmrtl, and Gsdf) and a considerable downregulation of female differentiation-related gene expression (Zhang et al., 2017). The foxl2 mRNA level has been proposed as a suitable early-stage sex identification marker in Amur sturgeon (Yarmohammadi et al., 2017b). In Huso huso, foxl2 is predominantly expressed in female gonads (Yarmohammadi et al., 2017a). Collectively, these findings suggest that the roles foxl2 gene plays in sex differentiation may be evolutionarily conserved.
Despite the critical roles the foxl2 gene plays in orchestrating gonadal development, limited literature exists concerning its function in Dabry’s sturgeon. In this investigation, we simulated the full-length cds sequence of the foxl2 gene in Dabry’s sturgeon and analyzed its expression across various tissues. The objective of this study is to elucidate the molecular mechanisms underpinning gonadal development in Dabry’s sturgeon. This research aims to lay a theoretical foundation for understanding the regulatory mechanisms governing gonadal development in this species.
2 Materials and methods
All fish handling and experimental procedures in this study were approved by the Chinese Sturgeon Research Institute, the China Three Gorges Corporation, and the Hubei Key Laboratory of Three Gorges Project for Conservation of Fishes. All experiments were performed in accordance with relevant guidelines and regulations.
2.1 Experimental fish, sampling and gender identification
All Dabry’s sturgeon specimens were procured from the Chinese Sturgeon Research Institute located in Hubei Province, China. The specimens were the product of controlled artificial spawning and had a consistent genetic background. These specimens were the product of artificial spawning and were hatched at a consistent temperature of 18.0 ± 0.5 °C. Following a 24-hour fasting period, three healthy 1-year-old male and female Dabry’s sturgeon individuals were selected for sampling. Sex determination was carried out using sex-linked markers (F: TAAAGGGAGACGGCAGAT; R: CAGGAAAGGCAAGGATGT) developed by our laboratory (Hu et al., 2021).
Deep anesthesia was induced via a 0.05% solution of ethyl 3-aminobenzoate mesylate-222 (MS-222) from Sigma. Subsequently, various organs and tissues, including the pituitary, brain, hypothalamus, heart, liver, stomach, intestine, muscle, testicles, and ovaries were surgically removed and promptly submerged in liquid nitrogen for subsequent gene cloning and tissue distribution analyses.
2.2 RNA extraction and cDNA preparation
Total RNA was isolated from a variety of tissues utilizing the Total RNA Extraction Kit (Promega, Shanghai, China), strictly adhering to the manufacturer’s guidelines. The integrity and purity of the extracted RNA were evaluated using a Nanodrop 2000 spectrophotometer (Thermo Scientific) and 1% agarose gel electrophoresis.
Following this, complementary DNA (cDNA) was synthesized in a 20 µL reaction mixture containing 1µg of total RNA, 4µL of 5×FastKing-RT SuperMix, and RNase-Free ddH2O (TIANGEN, Beijing, China), in accordance with the manufacturer’s instructions. The synthesized cDNA was subsequently diluted and conserved at -20°C for future use.
2.3 Molecular cloning of foxl2 and bioinformatic analysis
The open reading frame (ORF) of the foxl2 gene was obtained using primers designed in accordance with the expressed sequence tag (EST) sequences of foxl2. These sequences were derived from the transcriptome data previously sequenced in our laboratory. Subsequently, the cDNA fragments of the foxl2 gene from the Dabry’s sturgeon (Adfoxl2) were generated through PCR amplification.
The PCR reaction was conducted in a final volume of 50 μL, incorporating 2 μL of cDNA, 4 μL of a 10 mmol/L dNTP mix, 5 μL of reaction buffer, 1 μL of each primer solution (as per Table 1), 0.4 μL of Taq polymerase (Takara), and 36.6 μL of nuclease-free water. The PCR protocol involved an initial denaturation step at 94°C for 3 minutes, followed by 35 cycles of amplification (94°C for 30 seconds, gene-specific annealing temperature for 30 seconds, and extension at 72°C for 30 seconds). A final extension step was performed at 72°C for 10 minutes.
Following PCR, the products were subjected to electrophoretic separation on a 1.5% agarose gel and subsequently visualized via ethidium bromide staining. The presumptive gene fragments were then cloned into the PMD18-T vector (Takara, Japan) after the purification of the PCR products. Sequencing was carried out with an ABI3730XL sequencer (Applied Biosystems, Foster City, CA).
The DNA sequence was translated and the conserved domains of Adfoxl2 proteins were analyzed using DNAMAN version 7 and the NCBI CDD tools, respectively. The molecular weight (MW) and theoretical isoelectric point (pI) of the inferred Adfoxl2 protein were calculated using the Compute pI/Mw tool on the ExPASy online platform. The SignalP-5.0 Server was utilized to predict signal peptide cleavage sites of largemouth bass lamps. The Simple Modular Architecture Research Tool (SMART) was employed to predict the protein domain features of Adfoxl2, while potential transmembrane regions were anticipated with TMHMM. NetNGlyc-1.0 was used to forecast putative N-linked sites, and NetPhos-3.1 was employed to predict potential phosphorylation sites. Gene ontology predictions were made using the PredictProtein online server. The secondary structure and 3D molecular modeling of Adfoxl2 protein were predicted using PSIPRED and SWISS-MODEL, respectively, under default parameters.
For sequence and phylogenetic analysis of foxl2, online bioinformatics tools were utilized. The sequenced foxl2 and its corresponding amino acid sequence were analyzed using BLAST. Amino acid alignments and phylogenetic analysis were performed using MEGA5.0 software, employing the boot-strapped neighbor joining method. The amino acid sequences of foxl2 from other species were obtained from the NCBI website for multiple sequence alignment with Adfoxl2 (Table 2).
2.4 Quantitative real-time PCR
Quantitative real-time PCR (qRT-PCR) was conducted employing the TB Green® Premix Ex Taq™ II (Tli RNaseH Plus) kit, with β-actin serving as the internal control gene. Primer sequences are provided in Table 1. The cDNA templates were derived from various tissues and ovarian tissues of 1-year-old female and female Dabry’s sturgeon specimens.
The total volume for the qRT-PCR reaction system was set at 20 µL. The reaction protocol consisted of three steps: an initial denaturation at 95°C for 30 seconds, followed by 40 cycles of denaturation at 95°C for 5 seconds, and annealing at 60°C for 34 seconds. The melting curve program was executed as follows: initial denaturation at 95°C for 15 seconds, annealing at 60°C for 1 minute, and a final denaturation at 95°C for 15 seconds.
Quantitative results were analyzed using the 2−ΔΔCt method. SPSS 27.0 and Microsoft Office Excel 2016 were utilized for statistical analysis of the quantitative data. One-way ANOVA and LSD tests were performed to discern significant differences among the data sets. A p-value less than 0.05 denoted a significant difference, while a p-value less than 0.01 indicated an extremely significant difference. Graphs were constructed using GraphPad Prism 9 (GraphPad Software, San Diego, CA, USA).
2.5 Expression analysis of foxl2 in estradiol-treated
The Dabry’s sturgeon specimens used in this study were obtained from the Chinese Sturgeon Research Institute in Hubei Province, China. All specimens in this study were products of controlled artificial spawning and possessed a consistent genetic background. Water quality parameters were monitored daily throughout the experiment. The average conditions were as follows: pH 6.6 ± 0.7 (measured by a YSI PH100 pH meter), dissolved oxygen 6.2 ± 0.6 mg/L (measured by a YSI model 55 oximeter), ammonia concentration less than 0.1 mg/L, and nitrite concentration 0.11 mg/L.
Three cohorts of 5-month-old Dabry’s sturgeon were housed in 16 m3 tanks and fed a diet supplemented with either 0, 50, or 100 mg of 17β-estradiol (E2) per kg of food. The fish were provided with E2-enriched feed for a period of 90 days, from 5 to 8 months post-hatching (mph). This period was chosen as it precedes the critical phase of sexual differentiation for this species. Post the 90-day treatment, the juvenile fish were fed twice daily ad libitum with commercial powdered food devoid of any hormonal supplementation. Fish were sampled at 8 mph.
Three healthy 8-month-old female and male Dabry’s sturgeon were sampled from each group after a 24-hour fasting period. The sex of the Dabry’s sturgeon was determined by identifying sex-linked markers (F: TAAAGGGAGACGGCAGAT; R: CAGGAAAGGCAAGGATGT), a method developed in our lab. Deep anesthesia was induced using a 0.05% solution of MS-222. The testes and ovaries were surgically removed and immediately submerged in liquid nitrogen. Total RNA was then extracted from these samples, and complementary DNA (cDNA) was synthesized as described earlier. Primers for foxl2 (Table 1) were used to assess the impact of E2 on the expression of this gene in relation to sexual differentiation. The qRT-PCR reaction was carried out as per the previously described.
3 Results
3.1 Adfoxl2 gene sequence characteristics
A homolog of the foxl2 gene, termed as Adfoxl2, was identified from transcriptomic data of Dabry’s sturgeon and its accuracy was confirmed using PCR technology (GenBank No. PP942814). The Adfoxl2 gene encompassed an open reading frame (ORF) of 918 base pairs, encoding a protein comprised of 305 amino acid residues. The predicted theoretical molecular weight of this protein was 76.5 kDa, with a theoretical isoelectric point of 5.04. The protein did not possess any transmembrane or signal peptide regions but contained a 90-amino-acid forkhead (FH) domain located from the 45th to the 135th residue (Figure 1).
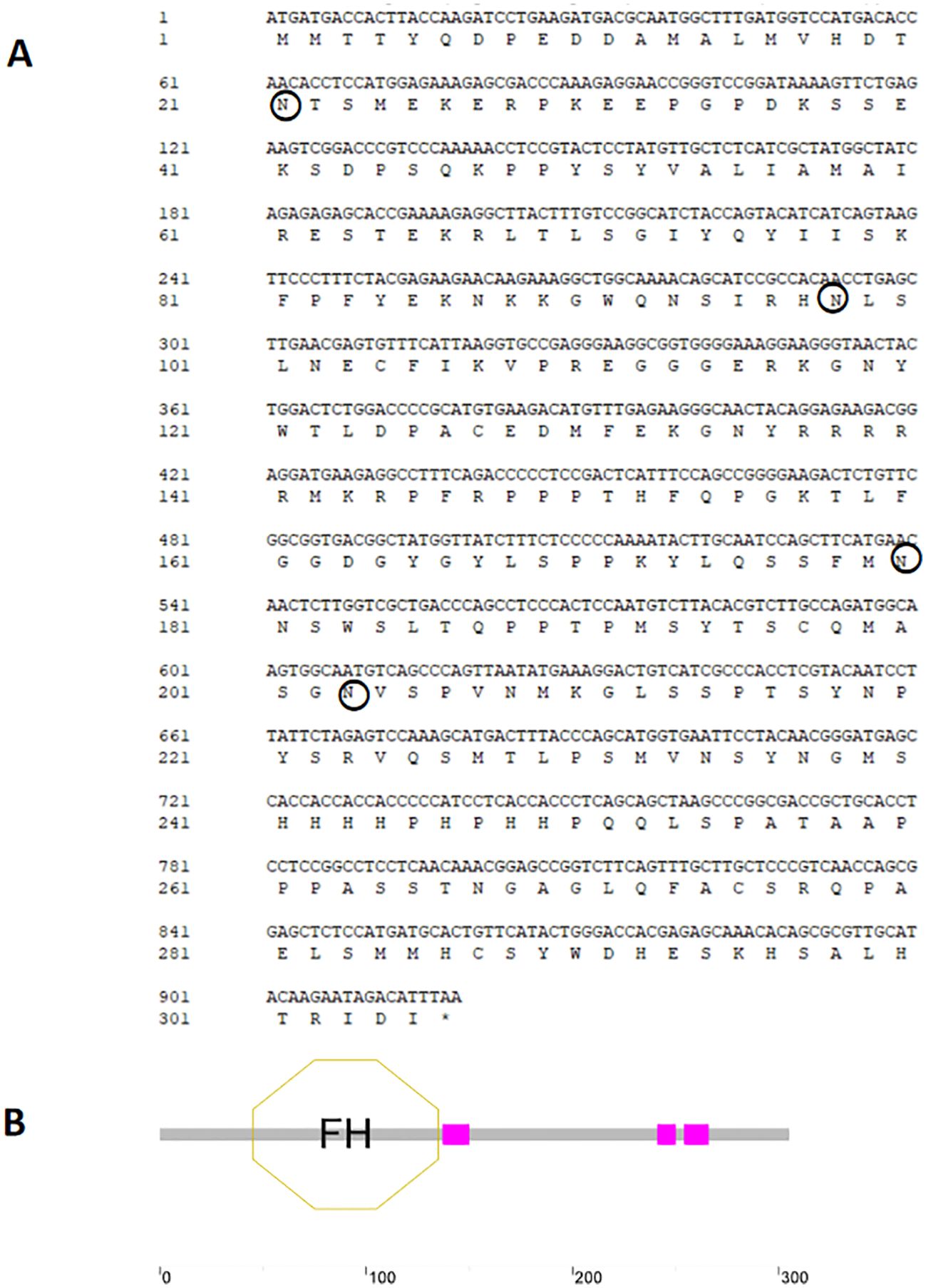
Figure 1. Structure and sequence analysis of Adfoxl2. (A) CDS sequence and deduced protein sequence of Adfoxl2. (B) Schematic illustration of foxl2 protein structure. The nucleotide and amino acid positions are indicated on the left. The stop codon is represented by a black asterisk (*). Potential N-glycosylation sites are marked with circle.
The Adfoxl2 protein exhibited an FH domain, the specifics of which are illustrated in Figure 1. Furthermore, the number of signaling peptides, transmembrane regions, and potential N-glycosylation sites in Adfoxl2 were 0, 0, and 4, respectively.
Secondary structure analysis revealed that the Adfoxl2 protein comprises 8 alpha helices and 2 beta strands, as determined through online protein structure prediction tools (Figure 2A). A three-dimensional (3D) molecular model of Adfoxl2 was constructed using the SWISS-MODEL server. The sequence identity between Adfoxl2 and the template protein (Forkhead box protein L2, AlphaFold DB model of Q1JPV2_DANRE id: Q1JPV2.1.A) was found to be 85.62%. This high degree of similarity suggests that the 3D model of the Adfoxl2 protein is reliable and confirms its classification within the foxl2 protein family (Figure 2B).
The predicted Adfoxl2 protein sequence was aligned with its homologs in different species (Figure 3). As shown in Figure 3 and Table 2, the deduced amino acid sequence of Adfoxl2 was very similar to that of the Acipenser ruthenus (99.7%), and Acipenser schrenckii (96.1%) in analyzed Acipenser. Phylogenetic analysis (Figure 4) showed that foxl2 proteins identified from Acipenser were clustered together. These phylogenetic relationships are consistent with the classification and evolutionary status of these species.
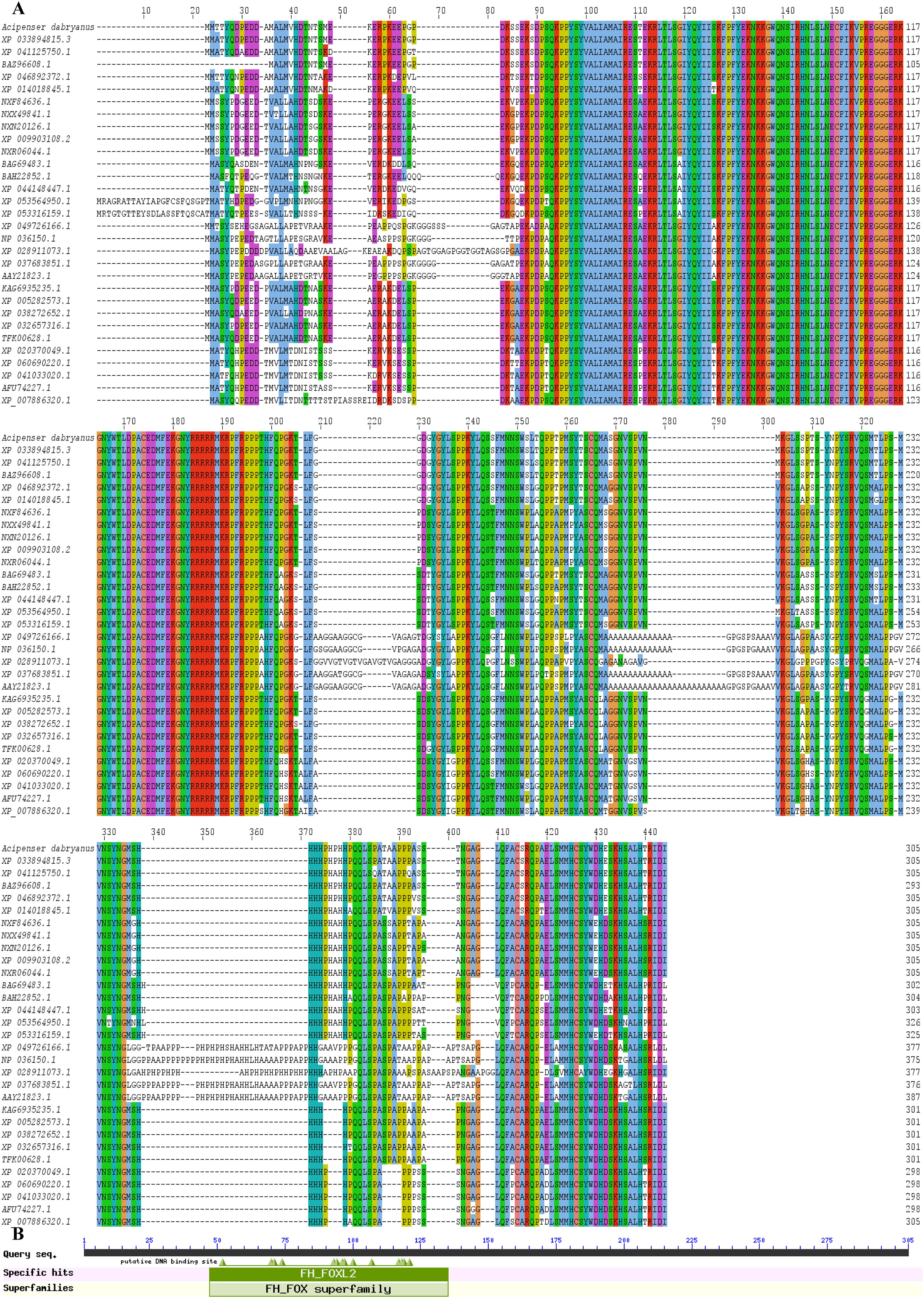
Figure 3. (A) Multiple sequence alignments of Adfoxl2 amino acid sequences with that in other species. The putative conserved domain is indicated. (B) CDD analysis showing the conserved domain of foxl2 proteins.
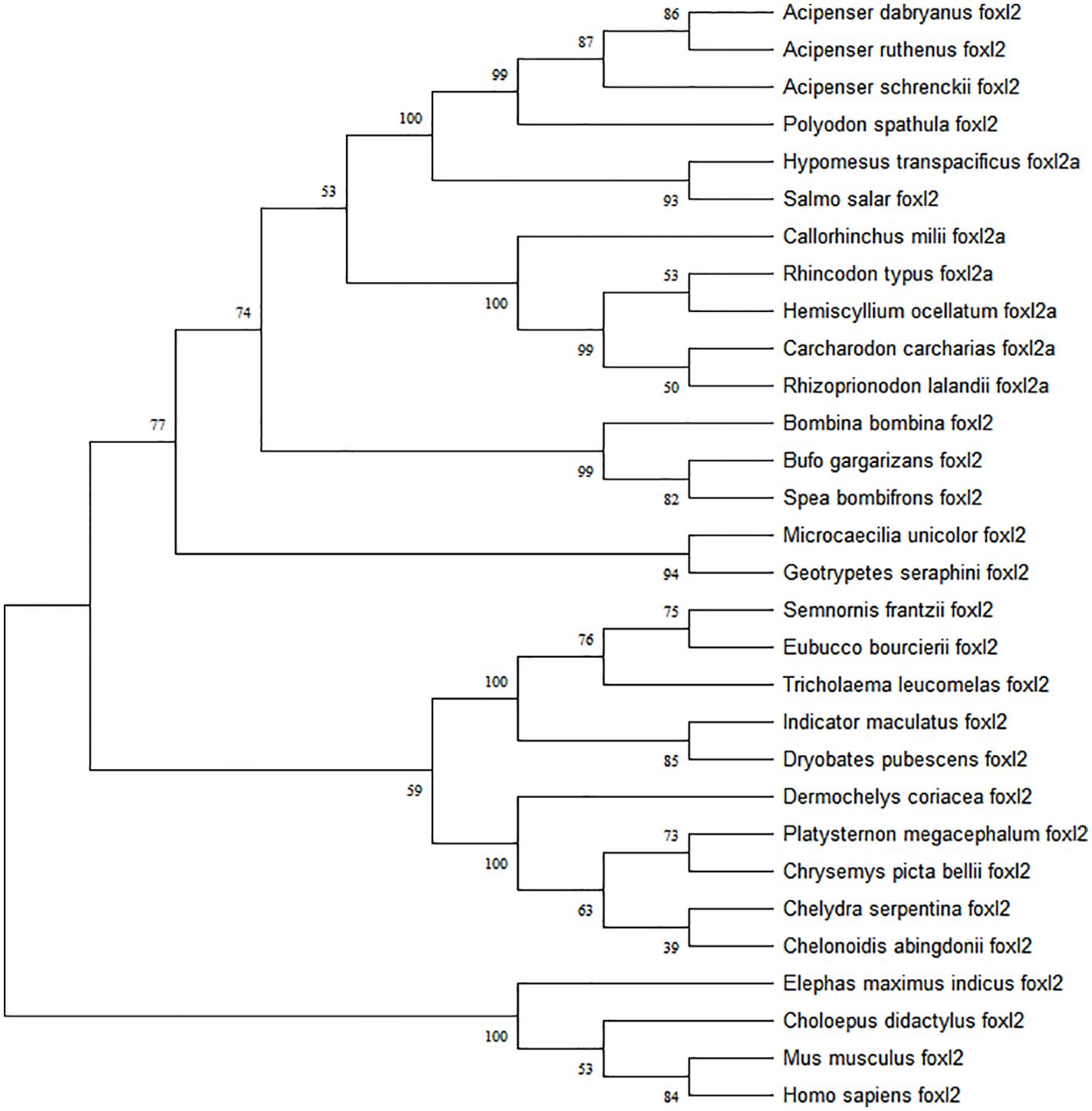
Figure 4. Phylogenetic tree of foxl2 constructed by the neighbor-joining method. Numbers at the tree nodes are bootstrap values with 500 replicates.
To elucidate the functions of Adfoxl2, its expression profile across various tissues was examined. As depicted in Figure 5A, the Adfoxl2 gene exhibited predominant expression in the ovary, with the expression level in the gonad significantly surpassing that in other tissues. Moreover, the expression of Adfoxl2 was generally higher in females compared to males across most tissues. Figure 5B presents the comparative expression levels of the Adfoxl2 gene in the ovaries and testes of the Dabry’s sturgeon at 8 and 12 months of age. Notably, the gene exhibits significantly higher expression in the ovaries compared to the testes for both age groups, as evidenced by the quantitative RT-PCR data. Moreover, there is a discernible increase in Adfoxl2 expression from the 8-month to the 12-month stage in both tissues. However, the rate of increase is substantially more pronounced in the ovaries, suggesting a potentially more critical role of Adfoxl2 in ovarian development or function within the observed period.
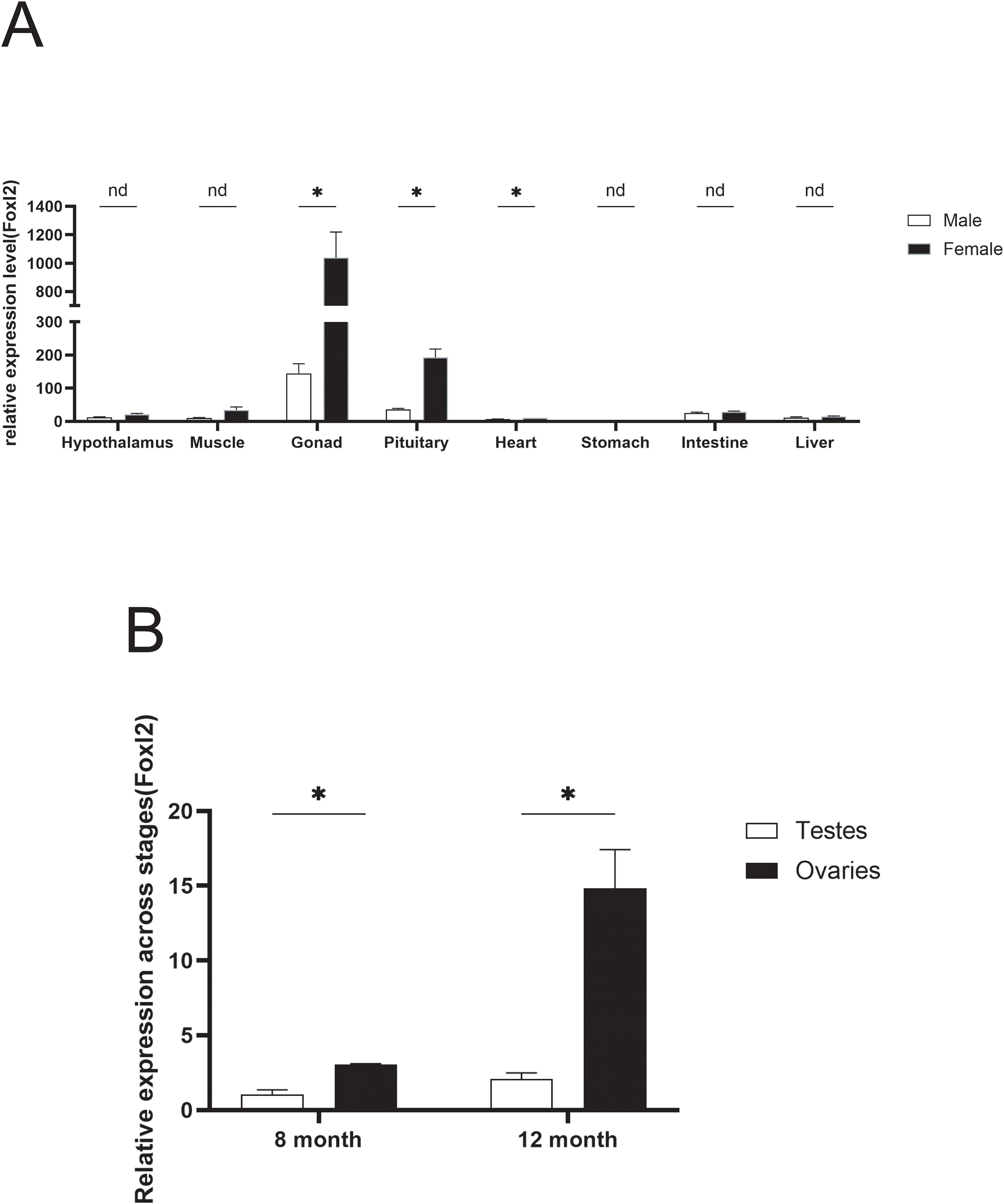
Figure 5. (A) Expression pattern of Adfoxl2 in different tissues of Dabry’s sturgeon determined by qPCR analysis. (B) The expression pattern of Adfoxl2 in testes and ovaries at 8 and 12 month stages. Data with black asterisk was significantly different (p < 0.05).
The expression levels of the foxl2 gene were assessed in testes and ovaries under three different estradiol concentration experimental groups (0, 50, 100 mg/kg) (Figure 6A). In the testes, the expression levels of the foxl2 gene initially increased and then decreased, with significant differences observed between adjacent experimental groups. Specifically, the expression levels were higher in the 50 mg/kg group compared to the 0 mg/kg group and lower in the 100 mg/kg group compared to the 50 mg/kg group. In the ovaries, the foxl2 gene expression levels increased with increasing estradiol concentration. Significant differences were noted between the 100 mg/kg group and the other two groups (0 mg/kg and 50 mg/kg). When comparing the foxl2 gene expression levels between testes and ovaries within each experimental group, significant differences were found in the 0 mg/kg and 100 mg/kg groups (Figure 6B). These results suggest that estradiol concentration affects the expression of the foxl2 gene differently in testes and ovaries, highlighting its potential role in gonadal differentiation and function.
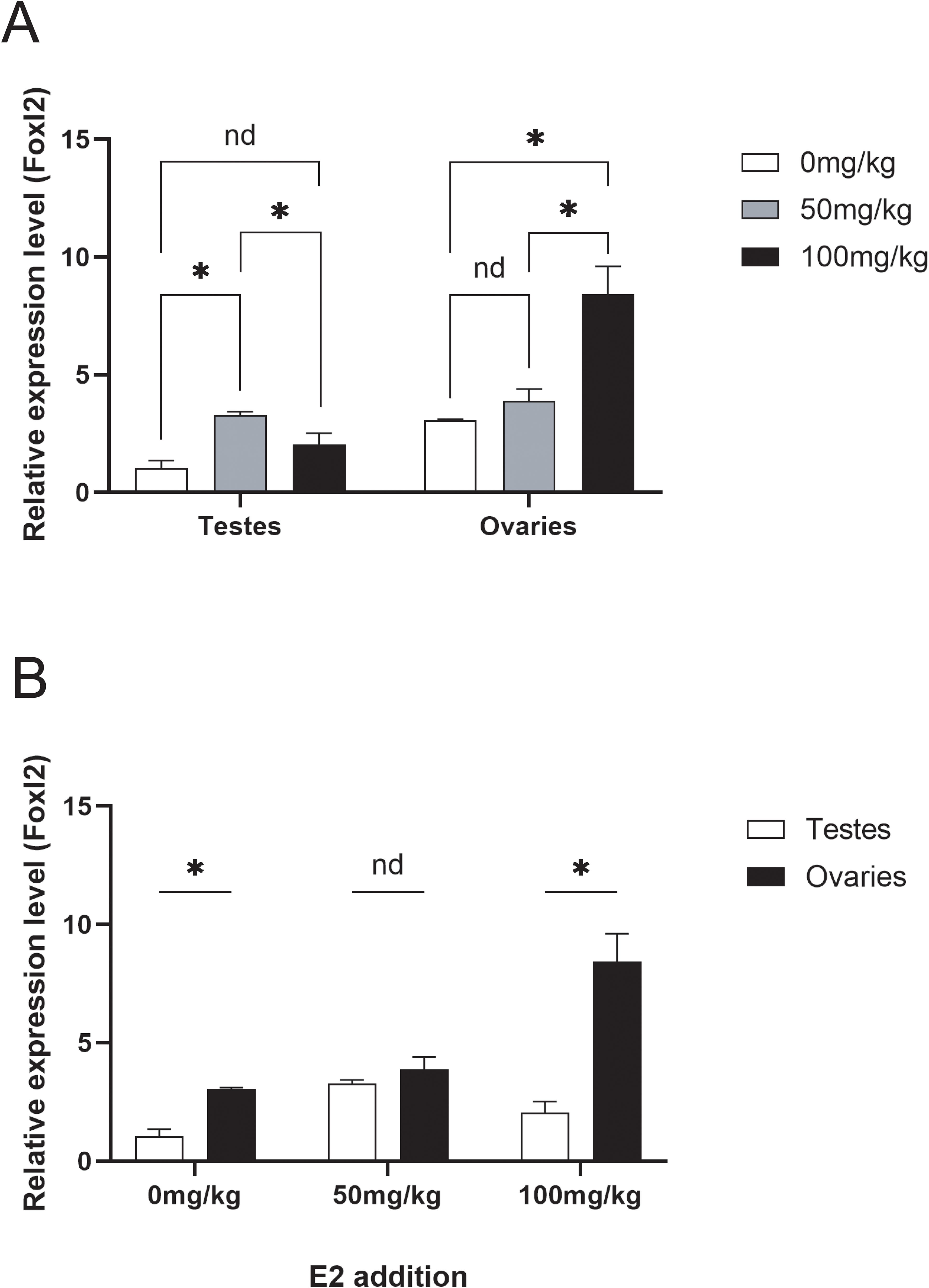
Figure 6. (A) Changes in the expression levels of the foxl2 gene in testes and ovaries under three estradiol concentration experimental groups (0, 50, 100 mg/kg). (B) Comparison of foxl2 gene expression levels in testes and ovaries under the three estradiol concentration experimental groups (0, 50, 100 mg/kg). Data with black asterisk was significantly different (p < 0.05).
4 Discussion
While the mechanisms of sex determination and differentiation have been extensively studied in mammals and various teleost species, yielding the identification of several sex-determining genes, the process remains complex in Dabry’s sturgeon (Amberg et al., 2013; Amberg et al., 2010; Chen et al., 2016; Hale et al., 2010; Wang et al., 2019; Wang et al., 2017; Yarmohammadi et al., 2017a; Yarmohammadi et al., 2017b). In this study, we report the cloning and characterization of a foxl2 gene in Dabry’s sturgeon, including an analysis of its nucleotide and amino acid sequences. We further explored the expression pattern of this gene across different tissues in one-year-old Dabry’s sturgeon, comparing both females and males. In addition, the impact of E2 administration on foxl2 expression in the gonad was assessed.
The foxl2 gene in Dabry’s sturgeon exhibited a close relationship with the foxl2 gene in Acipenser. Similar to other foxl2 proteins, the Adfoxl2 gene cDNA encodes a protein of 305 amino acids, including a highly conserved forkhead (FH) domain, suggesting evolutionary conservation of Adfoxl2. Quantitative RT-PCR results revealed that Adfoxl2 was specifically expressed in the ovaries and testes, with significantly higher expression in the ovary compared to the testis (p < 0.01). As gene expression patterns are typically linked to function, the high expression of Adfoxl2 in the testis and ovary underscores its vital role in gonad development.
Previous studies on Acipenser species, such as Acipenser gueldenstaedtii and Acipenser schrenckii, have consistently reported higher levels of foxl2 expression in the gonad (Okada et al., 2017; Degani et al., 2019). foxl2 expression was observed in undifferentiated gonads in Siberian sturgeon at around 90-120 days post-hatch (dph), with significant variations in expression levels among the individuals studied (Vizziano-Cantonnet et al., 2016). Around the same period (270 dph) in Russian sturgeon, foxl2 demonstrated clear sexual dimorphism with significantly higher expression in presumptive females (Hagihara et al., 2014). A similar dichotomous pattern of expression was observed in Amur sturgeon at around 270 dph (Okada et al., 2017).
Interestingly, around 300 dph in Siberian sturgeon, foxl2 expression levels were higher in males than females, though no explanation was provided for this observation (Vizziano-Cantonnet et al., 2016). By the time of cytological differentiation of the ovaries (420–480 dph), foxl2 transcript abundance was clearly higher in females than males in several species, further emphasizing its importance in maintaining ovarian function in sturgeons. In contrast, in 4-year-old stellate sturgeon, no statistically significant differences in foxl2 expression were found between the sexes, although the expression in females was slightly higher (Burcea et al., 2018). In the beluga, foxl2 expression varied at different stages of oocyte development, likely due to seasonal changes in expression (Yarmohammadi et al., 2017a). The result in this study showed discrepancy between the expected role of foxl2 as a female-biased gene and the observed high expression in the testes of Dabry’s sturgeon. Upon further investigation, we found that this could be attributed to several factors that warrant further exploration. The high expression of Adfoxl2 in the testicle may be associated with its role in testicular function and differentiation, similar to its function in ovarian development. Further studies are needed to explore this aspect. It is possible that the high expression of Adfoxl2 in the testes is related to a yet unidentified function in male reproductive physiology, which might be specific to this species or a broader phenomenon in teleost fish. Collectively, these findings underscore the critical role of foxl2 in gonadal differentiation and development.
Estrogens are synthesized from androgens via the enzymatic action of cytochrome P450 aromatase, encoded by the cyp19a1a and cyp19a1b genes in most teleost fish species (Li et al., 2019). The expression of cyp19a1a and endogenous estrogen production are confined to female gonads during the crucial period of molecular sexual differentiation (Li et al., 2019). In this context, pre-exposure to 17β-estradiol before the critical window of gonadal differentiation has been demonstrated to trigger ovarian differentiation in genetic males across various fish families, including Salmonidae, Cichlidae, Poecilidae, Anguillidae, Sparidae, Cyprinidae, Belontiidae, and Characidae (Chang et al., 1994; Pandian and Sheela, 1995; Piferrer, 2001; de Bem et al., 2012; Díaz and Piferrer, 2017). On the contrary, the administration of aromatase inhibitors prior to sexual differentiation has been reported to induce masculinization in genotypic female fish, as observed in species such as rainbow trout (Oncorhynchus mykiss), protogynous orange-spotted grouper (Epinephelus coioides), tilapia (Oreochromis niloticus), chinook salmon (Oncorhynchus tshawytscha), and European sea bass (Dicentrarchus labrax) (Guiguen et al., 1999; Piferrer and Donaldson, 1992; Navarro-Martín et al., 2009; Tsai et al., 2011).
In this vein, E2 treatments or aromatase inhibitor applications have been extensively employed for sex control in aquaculture, especially for establishing monosex cultures in species demonstrating pronounced sexual dimorphism in weight, size, or growth rate (Piferrer, 2001). Investigations involving South American pejerrey (Odontesthes bonariensis) have demonstrated that E2 treatment during early gonadal development diminishes the expression of testicular differentiation-related genes like amh and dmrt1 (Fernandino et al., 2008a, b), while concurrently enhancing the transcript abundance of cyp19a1a, associated with ovarian differentiation (Fernandino et al., 2008a). Additionally, our gene expression analysis indicated that the relative mRNA levels of foxl2 were significantly upregulated in both male and female Dabry’s sturgeon individuals following E2 exposure. These results showed a concentration-dependent effect of E2 on the expression of Adfoxl2 in the ovary. However, Adfoxl2 expression in the testicle was higher in the group treated with 50 mg of 17β-estradiol/kg food compared to the groups treated with 100 mg/kg and the control group (0 mg/kg). This finding suggests a complex dose-response relationship between E2 exposure and Adfoxl2 gene regulation. We hypothesize that the heightened expression of Adfoxl2 at the 50 mg/kg E2 dosage may be attributed to a sensitive threshold effect, where this particular concentration optimally activates the signaling pathways leading to Adfoxl2 transcription. Beyond this threshold, as evidenced by the 100 mg/kg E2 group, there may be a downregulation or desensitization of these pathways, resulting in reduced Adfoxl2 expression. This phenomenon could be analogous to a bell-shaped dose-response curve, where an optimal stimulus elicits a maximal response, and deviations from this optimal level lead to diminished effects. Furthermore, it is plausible that the 50 mg/kg E2 dosage aligns more closely with endogenous hormonal levels or a biologically relevant threshold for Adfoxl2 upregulation in Dabry’s sturgeon testes. The higher dosage of 100 mg/kg might overload the system’s capacity to respond, leading to a plateau or even inhibitory effect on Adfoxl2 expression due to potential negative feedback mechanisms or saturation of the receptors involved in E2 signaling. Conversely, in the ovaries, Adfoxl2 expression increased progressively with the concentration of E2, suggesting a dose-dependent response.
We propose several hypotheses to elucidate these phenomena. For the testes, the heightened Adfoxl2 expression at the intermediate E2 dosage could be indicative of a sensitive threshold for gene regulation, where this concentration optimally activates specific signaling pathways, leading to increased Adfoxl2 transcription. The reduced expression at the higher dosage might be due to a downregulation or desensitization effect, potentially involving receptor saturation or negative feedback mechanisms that counteract the hormone’s influence at higher concentrations.
In contrast, the ovaries’ response to E2 suggests a more linear, dose-dependent relationship, where the increment in E2 concentration directly correlates with increased Adfoxl2 expression. This could imply a different regulatory mechanism in the ovaries, possibly involving a more direct and proportional response to E2 levels, which may play a role in the ovarian differentiation process as previously suggested.
Collectively, these findings suggest that E2 may influence the gonadal differentiation and development of Dabry’s sturgeon by modulating the expression of foxl2. Given the role of foxl2 in teleost fish, our results suggest that foxl2 is a female-biased gene in Dabry’s sturgeon, potentially playing a role in the ovarian differentiation of this species. As scientific researchers, we recognize the imperative for a deeper investigation into the role of the foxl2 gene in the gonadal differentiation of the Dabry’s sturgeon, a species listed as critically endangered. The elucidation of the gene’s function through gain-of-function and loss-of-function studies would significantly enhance our understanding of its regulatory mechanisms in both ovarian and testicular tissues. However, the execution of such experiments is fraught with challenges due to the species’ protected status and its protracted life cycle. The scarcity of available specimens for research, compounded by the ethical considerations and conservation efforts surrounding this first-class protected species, has restricted our ability to procure additional samples necessary for these experiments. In light of these constraints, we advocate for innovative approaches that could potentially circumvent the need for physical samples. For instance, the application of cutting-edge genomic techniques, such as CRISPR/Cas9-based in vitro assays, could offer a viable alternative for studying the gene’s function without further impacting the already vulnerable population. Furthermore, we call for collaborative efforts among researchers, conservationists, and regulatory bodies to establish guidelines that balance scientific inquiry with the imperative to protect this endangered species. It is through such interdisciplinary cooperation that we can hope to advance our knowledge of the foxl2 gene’s role in Dabry’s sturgeon reproduction while ensuring the species’ survival for future generations.
In summary, this investigation offers an examination of foxl2 expression profiles in Dabry’s sturgeon during the stages of molecular and morphological sex differentiation. The primary expression of foxl2 was observed in the ovary of 1-year-old Dabry’s sturgeon, with significantly less expression detected in various somatic tissues. Moreover, our analysis of gene expression indicated that relative mRNA levels of foxl2 were significantly up-regulated in both male and female Dabry’s sturgeon individuals following E2 exposure. This model opens new avenues for exploring the mechanisms of gonadal sex differentiation and the effects of environmental estrogenic exposure in Acipenser fish. Future research endeavors will aim to deepen our understanding of sex differentiation in sturgeon by studying other sex-related genes.
Data availability statement
The datasets presented in this study can be found in online repositories. The names of the repository/repositories and accession number(s) can be found in the article/supplementary material.
Ethics statement
All fish handling and experimental procedures in this study were approved by the Chinese Sturgeon Research Institute, the China Three Gorges Corporation, and the Hubei Key Laboratory of Three Gorges Project for Conservation of Fishes. All experiments were performed in accordance with relevant guidelines and regulations. The studies were conducted in accordance with the local legislation and institutional requirements. Written informed consent was obtained from the owners for the participation of their animals in this study.
Author contributions
YH: Writing – review & editing, Writing – original draft, Visualization, Validation, Supervision, Software, Resources, Project administration, Methodology, Investigation, Funding acquisition, Formal analysis, Data curation, Conceptualization. RT: Writing – review & editing, Writing – original draft. TS: Writing – review & editing, Writing – original draft. ZC: Writing – review & editing, Writing – original draft. HW: Writing – review & editing, Writing – original draft. YC: Writing – review & editing, Writing – original draft. XZ: Writing – review & editing, Writing – original draft. YL: Writing – review & editing, Writing – original draft. BW: Writing – review & editing, Writing – original draft. BG: Writing – review & editing, Writing – original draft. RW: Writing – review & editing, Writing – original draft. HQ: Writing – review & editing, Writing – original draft. DZ: Writing – review & editing, Writing – original draft. HD: Writing – review & editing, Writing – original draft. ZL: Writing – review & editing, Writing – original draft. DX: Writing – review & editing, Writing – original draft. WJ: Writing – review & editing, Writing – original draft. QL: Writing – review & editing, Writing – original draft. YY: Writing – review & editing, Writing – original draft, Visualization, Validation, Supervision, Software, Resources, Project administration, Methodology, Investigation, Funding acquisition, Formal analysis, Data curation, Conceptualization.
Funding
The author(s) declare financial support was received for the research, authorship, and/or publication of this article. This work was supported by the Hubei Provincial Natural Science Foundation of China (no, 2022CFB738, 2023AFB989, and 2022CFB803) and Hubei Key Laboratory of Three Gorges Project for Conservation of Fishes (no. 2022001- ZHX), China Three Gorges Corporation (no. WWKY-2021-0351 and WWKY-2020-0079), the National Natural Science Foundation of China (32202967), and National Undergraduate Training Program for Innovation and Entrepreneurship of China. The funder was not involved in the study design, analysis, interpretation of data, the writing of this article or the decision to submit it for publication.
Conflict of interest
Authors YH, TS, XZ, YL, BW, BG, RW, HQ, DZ, HD, ZL, WJ, and YY were employed by China Three Gorges Corporation.
The remaining authors declare that the research was conducted in the absence of any commercial or financial relationships that could be construed as a potential conflict of interest.
Publisher’s note
All claims expressed in this article are solely those of the authors and do not necessarily represent those of their affiliated organizations, or those of the publisher, the editors and the reviewers. Any product that may be evaluated in this article, or claim that may be made by its manufacturer, is not guaranteed or endorsed by the publisher.
References
Amberg J. J., Goforth R., Stefanavage T., Sepulveda M. S. (2010). Sexually dimorphic gene expression in the gonad and liver of shovelnose sturgeon (Scaphirhynchus platorynchus). Fish Physiol. Biochem. 36, 923–932. doi: 10.1007/s10695-009-9369-8
Amberg J. J., Goforth R. R., Sepúlveda M. S. (2013). Antagonists to the Wnt cascade exhibit sex-specific expression in gonads of sexually mature shovelnose sturgeon. Sexual Dev. 7, 308–315. doi: 10.1159/000354280
Burcea A., Popa G.-O., Florescu (Gune) I. E., Maereanu M., Dudu A., Georgescu S. E., et al. (2018). Expression characterization of six genes possibly involved in gonad development for stellate sturgeon individuals (Acipenser stellatus, Pallas 1771). Int. J. Genomics 2018, 1–10. doi: 10.1155/2018/7835637
Chang C. F., Lee M. F., Chen G. R. (1994). Estradiol-17β associated with the sex reversal in protandrous black porgy, Acanthopagrus schlegeli. J. Exp. Zool. 268, 53–58. doi: 10.1002/jez.1402680107
Chen Y., Xia Y., Shao C., Han L., Chen X., Yu M., et al. (2016). Discovery and identification of candidate sex-related genes based on transcriptome sequencing of Russian sturgeon (Acipenser Gueldenstaedtii) gonads. Physiol. Genomics 48, 464–476. doi: 10.1152/physiolgenomics.00113.2015
de Bem J. C., Fontanetti C. S., Senhorini J. A., Parise-Maltempi P. P. (2012). Effectiveness of estradiol valerate on sex reversion in Astyanax altiparanae (Characiformes, Characidae). Braz. Arch. Biol. Technol. 55, 283–290. doi: 10.1590/S1516-89132012000200015
Degani G., Hurvitz A., Eliraz Y., Meerson A. (2019). Sex-related gonadal gene expression differences in the Russian sturgeon (Acipenser gueldenstaedtii) grown in stable aquaculture conditions. Anim. Reprod. Sci. 200, 75–85. doi: 10.1016/j.anireprosci.2018.11.013
Deng S. P., Zhu C. H., Sun J., Wang W. D., Wu T. L., Chen H. P., et al. (2015). foxl2 of the Hong Kong catfish (Clarias fuscus): cDNA cloning, tissue distribution and changes in gene expression towards methyltestosterone, estradiol and letrozole exposure of the fries during gonadal differentiation. Genes Genom. 37, 669–677. doi: 10.1007/s13258-015-0296-z
Díaz N., Piferrer F. (2017). Estrogen exposure overrides the masculinizing effect of elevated temperature by a downregulation of the key genes implicated in sexual differentiation in a fish with mixed genetic and environmental sex determination. BMC Genomics. 18, 973. doi: 10.1186/s12864-017-4345-7
Du H., Wei Q. W. (2016). Rebuilding homes for Acipenser dabryanus. China Nat. 6, 12–15. Available online at: http://g.wanf.ytwgk.top:81/D/Periodical_dzr201606004.aspx.
Fernandino J. I., Hattori R. S., Kimura H., Strüssmann C. A., Somoza G. M. (2008b). Expression profile and estrogenic regulation of anti-Müllerian hormone during gonadal development in pejerrey Odontesthes bonariensis, a teleost fish with strong temperature-dependent sex determination. Dev. Dynam. 237, 3192–3199. doi: 10.1002/dvdy.21731
Fernandino J. I., Hattori R. S., Shinoda T., Kimura H., Strobl-Mazzulla P. H., Strüssmann C. A., et al. (2008a). Dimorphic Expression of dmrt1 and cyp19a1a (Ovarian Aromatase) during Early Gonadal Development in Pejerrey, Odontesthes bonariensis. Sexual Dev. 2, 316–324. doi: 10.1159/000195681
Georges A., Auguste A., Bessière L., Vanet A., Todeschini A.-L., Veitia R. A. (2014). foxl2: a central transcription factor of the ovary. J. Mol. Endocrinol. 52, R17–R33. doi: 10.1530/JME-13-0159
Guiguen Y., Baroiller J. F., Ricordel M. J., Iseki K., McMeel O. M., Martin S. A. M., et al. (1999). Involvement of estrogens in the process of sex differentiation in two fish species: the rainbow trout (Oncorhynchus mykiss) and a tilapia (Oreochromis niloticus). Mol. Reprod. Develop.: Incorp. Gamete Res. 54, 154–162. doi: 10.1002/(sici)1098-2795(199910)54:2<154::aid-mrd7>3.0.co;2-5
Hagihara S., Yamashita R., Yamamoto S., Ishihara M., Abe T., Ijiri S., et al. (2014). Identification of genes involved in gonadal sex differentiation and the dimorphic expression pattern in undifferentiated gonads of Russian sturgeon Acipenser gueldenstaedtii Brandt & Ratzebur. J. Appl. Ichthyol. 30, 1557–1564. doi: 10.1111/jai.12588
Hale M. C., Jackson J. R., DeWoody J. A. (2010). Discovery and evaluation of candidate sex-determining genes and xenobiotics in the gonads of lake sturgeon (Acipenser fulvescens). Genetica 138, 745–756. doi: 10.1007/s10709-010-9455-y
Hu Y., Wang B., Du H., Liu X., Xiao K., Zeng Q., et al. (2021). A rapid method for identifying the genetic sex of the Chinese sturgeon using a genomic sequence fragment ZHXF-1 and its application. CN113136437B.
Li J. X., Liu D. Q., Ma Q. Z., Zhang X. Y., Dai W., Chen Y. B., et al. (2015). Discriminating Dabry’s sturgeon (Acipenser dabryanus) and Chinese sturgeon (Acipenser sinensis) basedonDNA barcodeand six nuclear markers. Hydrobiologia 757, 185–196. doi: 10.1007/s10750-015-2251-z
Li M., Sun L., Wang D. (2019). Roles of estrogens in fish sexual plasticity and sex differentiation. Gen. Comp. Endocrinol. 277, 9–16. doi: 10.1016/j.ygcen.2018.11.015
Li R., Zhang L., Li W., Zhang Y., Li Y., Zhang M., et al. (2018). foxl2 and DMRT1L are Yin and Yang genes for determining timing of sex differentiation in the bivalve mollusk Patinopecten yessoensis. Front. Physiol. 9, 1166. doi: 10.3389/fphys.2018.01166
Liu J., Han L., Li R. F., Xu P., Lu Y., Yang M., et al. (2016). Eukaryotic expression and purification of foxl2 gene in leghorn. J. Northw. A F Univ. Nat. Sci. Ed. 44, 10–15. doi: 10.13207/j.cnki.jnwafu.2016.07.002
Nakamoto M., Matsuda M., Wang D. S., Nagahama Y., Shibata N. (2006). Molecular cloning and analysis of gonadal expression of foxl2 in the medaka, Oryzias latipes. Biochem. Biophys. Res. Commun. 344, 353–361. doi: 10.1016/j.bbrc.2006.03.137
Navarro-Martín L., Blázquez M., Piferrer F. (2009). Masculinization of the European sea bass (Dicentrarchus labrax) by treatment with an androgen or aromatase inhibitor involves different gene expression and has distinct lasting effects on maturation. Gen. Comp. Endocrinol. 160, 3–11. doi: 10.1016/j.ygcen.2008.10.012
Okada H., Hagihara S., Yamashita K., Ijiri S., Adachi S. (2017). Expression pattern of foxl2 and Dmrt1 in gonad of Amur sturgeon Acipenser schrenckii in relation to sex differentiation. Aquaculture 479, 712–720. doi: 10.1016/j.aquaculture.2017.07.020
Pandian T. J., Sheela S. G. (1995). Hormonal induction of sex reversal in fish. Aquaculture 138, 1–22. doi: 10.1016/0044-8486(95)01075-0
Piferrer F. (2001). Endocrine sex control strategies for the feminization of teleost fish. Aquaculture 197, 229–281. doi: 10.1016/S0044-8486(01)00589-0
Piferrer F., Donaldson E. M. (1992). The comparative effectiveness of the natural and a synthetic estrogen for the direct feminization of chinook salmon (Oncorhynchus tashawytscha). Aquaculture 106, 183–193.
Schmidt D., Ovitt C. E., Anlag K., Fehsenfeld S., Gredsted L., Treier A. C., et al. (2004). The murine winged-helix transcription factor foxl2 is required for granulosa cell differentiation and ovary maintenance. Development 131, 933–942. doi: 10.1242/dev.00969
Shen Z. G., Wang H. P. (2014). Molecular players involved in temperature-dependent sex determination and sex differentiation in Teleost fish. Genet. Select. Evol. 46, 26. doi: 10.1186/1297-9686-46-26
Tsai Y. J., Lee M. F., Chen C. Y., Chang C. F. (2011). Development of gonadal tissue and aromatase function in the protogynous orange-spotted grouper Epinephelus coioides. Zool. Stud. 50, 693–704. Available online at: https://zoolstud.sinica.edu.tw/Journals/50.6/693.html.
Vizziano-Cantonnet D., Di Landro S., Lasalle A., Martınez A., Mazzoni T. S., Quagio-Grassiotto I. (2016). Identification of the molecular sex-differentiation period in the Siberian sturgeon. Mol. Reprod. Dev. 83, 19–36. doi: 10.1002/mrd.22589
Wan H. F., Zhong J. Y., Zhang Z. P., Xie Y. C., Wang Y. L. (2021). Characterization of the foxl2 gene involved in the vtg expression in mud crab (Scylla paramamosain). Gene 798, 145807. doi: 10.1016/j.gene.2021.145807
Wang W., Zhu H., Dong Y., Dong T., Tian Z., Hu H. (2019). Identification and dimorphic expression of sex-related genes during gonadal differentiation in sterlet Acipenser ruthenus, a primitive fish species. Aquaculture 500, 178–187. doi: 10.1016/j.aquaculture.2018.10.001
Wang W., Zhu H., Dong Y., Tian Z., Dong T., Hu H., et al. (2017). Dimorphic expression of sex-related genes in different gonadal development stages of sterlet, Acipenser ruthenus, a primitive fish species. Fish Physiol. Biochem. 43, 1557–1569. doi: 10.1007/s10695-017-0392-x
Wei Q., Ke F.e., Zhang J., Zhuang P., Luo J., Zhou R., et al. (1997). Biology, fisheries, and conservation of sturgeons and paddlefish in China, Environ. Biol. Fish 48, 241–255. doi: 10.1023/A:1007395612241
Wu J., Wang D., Miao C. L., Han Y. Y. (2018). Current research and the regulation mechanism of foxl2. Chin. J. Cell Biol. 40, 602–607. Available online at: http://en.cnki.com.cn/Article_en/CJFDTotal-XBZZ201804017.htm.
Yarmohammadi M., Pourkazemi M., Kazemi R. (2017a). Differential expression of foxl2 and cyp19a1a mRNA during gonad developmental stages in great sturgeon Huso huso. J. Fish Biol. 90, 1104–1111. doi: 10.1111/jfb.13224
Yarmohammadi M., Pourkazemi M., Kazemi R. (2017b). Expression pattern of foxl2 and dmrt1 in gonad of Amur sturgeon Acipenser schrenckii in relation to sex differentiation. Aquaculture 479, 712–720. doi: 10.1016/j.aquaculture.2017.07.020
Zhang H., Jarić I., Roberts D. L., He Y. F., Du H., Wu J. M., et al. (2020). Extinction of one of the world’s largest freshwater fishes: Lessons for conserving the endangered Yangtze fauna. Sci. Total Environ. 710, 136242. doi: 10.1016/j.scitotenv.2019.136242
Zhang S. H., Luo H., Du H., Wang D. Q., Wei Q. W. (2012). Isolation and characterization of twenty-six microsatellite loci for the tetraploid fish Dabry’s sturgeon (Acipenser dabryanus). Conserv. Genet. Resour. 5, 409–412. doi: 10.1007/s12686-012-9815-2
Zhang S. H., Wei Q. W., Du H., Li L. X. (2011). Present status and risk for extinction of the Dabry’s sturgeon (Acipenser dabryanus) in the Yangtze River watershed: a concern for intensified rehabilitation needs, J. Appl. Ichthyol. 27, 181–185. doi: 10.1111/j.1439-0426.2011.01674.x
Zhang X., Li M., Ma H., Liu X., Shi H., Li M., et al. (2017). Mutation of foxl2 or cyp19a1a results in female to male sex reversal in XX Nile tilapia. Endocrinology 158, 2634–2647. doi: 10.1210/en.2017-00127
Keywords: Dabry’s sturgeon, foxl2, gonadal development, endangered species, 17β-estradiol
Citation: Hu Y, Tan R, Shu T, Chu Z, Wang H, Chen Y, Zhu X, Li Y, Wang B, Guo B, Wang R, Qu H, Zhang D, Du H, Li Z, Xu D, Jiang W, Li Q and Yang Y (2024) Cloning, identification, and functional analysis of foxl2 gene and its expression after 17β‐estradiol (E2) treatment in Dabry’s sturgeon, Acipenser dabryanus. Front. Mar. Sci. 11:1461794. doi: 10.3389/fmars.2024.1461794
Received: 09 July 2024; Accepted: 05 August 2024;
Published: 03 September 2024.
Edited by:
Ylenia Carotenuto, Stazione Zoologica Anton Dohrn, ItalyReviewed by:
Zhigang Shen, Huazhong Agricultural University, ChinaJie Gong, Nantong University, China
Copyright © 2024 Hu, Tan, Shu, Chu, Wang, Chen, Zhu, Li, Wang, Guo, Wang, Qu, Zhang, Du, Li, Xu, Jiang, Li and Yang. This is an open-access article distributed under the terms of the Creative Commons Attribution License (CC BY). The use, distribution or reproduction in other forums is permitted, provided the original author(s) and the copyright owner(s) are credited and that the original publication in this journal is cited, in accordance with accepted academic practice. No use, distribution or reproduction is permitted which does not comply with these terms.
*Correspondence: Yuanjin Yang, eWFuZ195dWFuamluQGN0Zy5jb20uY24=; Qingfei Li, bGlxaW5nZmVpQHFhdS5lZHUuY24=; Wei Jiang, amlhbmdfd2VpNkBjdGcuY29tLmNu; Dan Xu, ZHh1QHNob3UuZWR1LmNu
†These authors have contributed equally to this work and share first authorship