- 1National Coral Reef Institute, Nova Southeastern University, Dania Beach, FL, United States
- 2Center for Marine Science, University of North Carolina Wilmington, Wilmington, NC, United States
Metrics of coral physiology can be used to identify changes in coral health due to environmental stressors or management actions. One of the most unprecedented stressors to Caribbean corals is the spread of stony coral tissue loss disease (SCTLD), which also resulted in the novel management action of in-water amoxicillin treatments on active disease lesions. Though highly effective at halting lesions and preventing coral mortality, possible unintended consequences of topical application of amoxicillin to coral tissue were unknown. We used in-water instrumentation to measure and compare photosynthesis (P), respiration (R), P/R ratios, and calcification of corals that were visually healthy, actively diseased, and diseased but treated with amoxicillin paste. Measurements occurred across three time points and two species – Orbicella faveolata and Montastraea cavernosa. Across all metrics, treatment type did not cause significant differences, indicating that neither SCTLD lesions nor amoxicillin treatments impacted the physiology of adjacent tissues. There were significant variations among time points, which may have resulted from changes to coral health across the reef, variations due to environmental variables, or other unknown factors. We suggest that physiological metrics could be an interesting way to fate track coral health across short- and long-term timeframes. We also conclude that amoxicillin treatments as a tool to halt SCTLD are not detrimental to respiration, photosynthesis, or calcification rates of adult corals.
Introduction
Coral disease is one of the most impactful stressors on Caribbean reefs, leading to widespread mortality and ecosystem changes. Stony coral tissue loss disease (SCTLD) is one of the most virulent and lethal, and has resulted in significant decreases in coral cover, catastrophic losses of highly susceptible species, and ecosystem impacts such as decreased calcification rates, biodiversity, and functionality (Walton et al., 2018; Brandt et al., 2021; Heres et al., 2021; Neely et al., 2021a; Alvarez-Filip et al., 2022). One of the primary mitigation actions for SCTLD has been the use of an in-water amoxicillin paste to halt active disease lesions. This paste was initially tested and applied to corals in Florida (Neely et al., 2020, 2021; Shilling et al., 2021; Walker et al., 2021), and then utilized in other parts of the Caribbean (Forrester et al., 2022) as SCTLD spread through the region.
The amoxicillin paste is effective at halting approximately 90% of disease lesions on O. faveolata in the Florida Keys (Neely et al., 2020, 2021), and long-term monitoring (with additional treatments applied if needed) has resulted in high survivorship over multiple years (Neely, 2023). However, the use of amoxicillin to prevent mortality of wild corals is novel, and secondary or unintended impacts remain a management concern. Recent studies have demonstrated that antibiotics may affect gene regulation of corals (Connelly et al., 2022; Studivan et al., 2023) and/or alter coral-associated bacterial communities (Connelly et al., 2022; Krueger et al., 2024). Studies on aquatic photosynthetic organisms have identified mixed results of amoxicillin, with photosynthetic cyanobacteria exhibiting reduced photosynthesis (Pan et al., 2008; González-Pleiter et al., 2013), but macroalgae exhibiting no effects (González-Pleiter et al., 2013; Gomes et al., 2020). We assessed whether basic metabolic metrics of the coral holobiont were impacted by a) immediate proximity to active SCTLD lesions and b) treatment of lesions with amoxicillin.
Coral physiology can be assessed across multiple metrics. Respiration rate measures the consumption of oxygen by the coral holobiont. Because corals harbor photosynthetic symbionts in the form of Symbiodiniaceae, photosynthetic rates also represent a metric of coral health. Greater photosynthesis by the symbionts results in greater autotrophic food availability for the coral. By dividing the photosynthetic rate by the respiration rate, the metric of P/R identifies whether the coral, when operating in an autotrophic state, is producing more energy than it consumes. Finally, coral growth is measurable by calcification rates. These metrics are all known to vary under different environmental stressors, including temperature, pH, and turbidity (Coles and Jokiel, 1977; Telesnicki and Goldberg, 1995; Reynaud et al., 2003; Al-Horani, 2005; Edmunds, 2005; Junjie et al., 2014). As such, measurements of these physiological parameters can indicate whether external forces or management actions are impacting coral health.
These metrics have been measured in a variety of ways through time, most of which involve either sacrificing the coral or conducting measurements in closed systems. However, the development of an in situ metabolic measuring system termed CISME provides a non-invasive method to assess these metrics on large in situ corals in real time, under natural conditions, and across repeated time points on the same tissue areas (Szmant et al., 2019; Dellisanti et al., 2020a, b).
Methods
Coral selection
Corals from two species (Montastraea cavernosa and Orbicella faveolata) and three health status categories (healthy, diseased, and treated) were selected for sampling. These two species are the two most common reef-building species remaining at Florida Keys offshore reefs and, in that habitat, are the corals most commonly treated for SCTLD. All sampled colonies were located at Looe Key, a spur-and-groove reef located on the bank-barrier reef system 8 km from shore in the Florida Keys National Marine Sanctuary (FKNMS; Figure 1). At the time of sampling, SCTLD had been present at the site for approximately four years. The bases of the selected colonies were all between 6 – 9 meters in depth. All selected corals were tagged, measured, photographed, and mapped (Supplementary Figure 1).
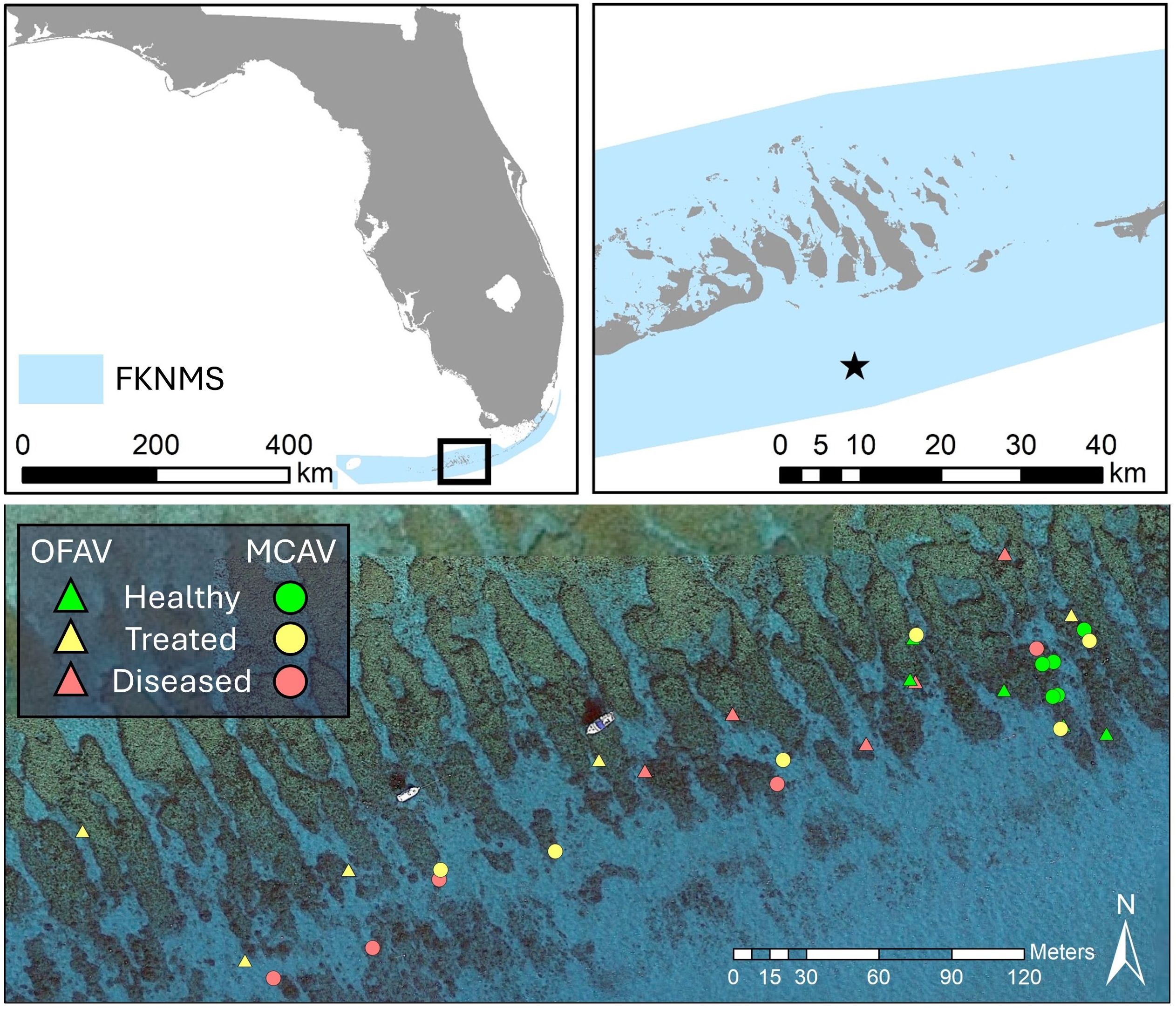
Figure 1. Location of Looe Key (star) within the Florida Keys National Marine Sanctuary (FKNMS), and location of sampled colonies of Orbicella faveolata (OFAV, triangles) and Montastraea cavernosa (MCAV, circles) within the site. Aerial imagery courtesy of Google Earth.
The experimental design (Figure 2) included a sample of five apparently healthy corals from each species (“healthy”), five diseased corals from each species that remained untreated (“diseased”), and five diseased corals from each species that were treated using an amoxicillin paste (“treated”). The treatment is a proprietary Ocean Alchemists topical paste (Base2b) which is mixed in a 1:8 by weight ratio (amoxicillin:Base2b) and applied via catheter syringe directly to disease margins (Figure 3B). The paste is formulated for a three-day time release of amoxicillin directly into the coral tissues, with approximately 55% of the antibiotic released from the ointment in the first 24 hours (Favero and Curtis, 2020).
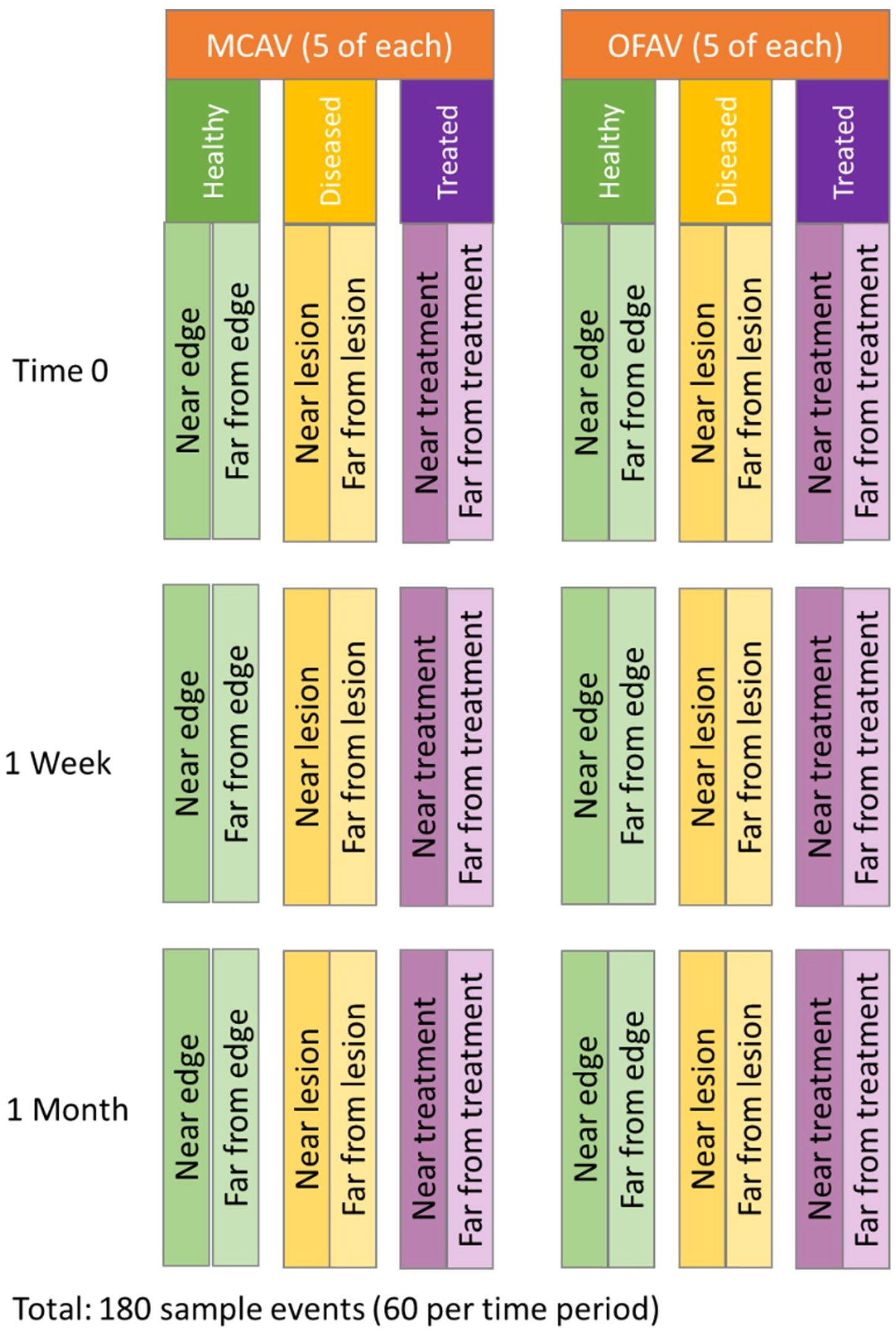
Figure 2. Schematic of sample design. For each species, Montastraea cavernosa (MCAV) and Orbicella faveolata (OFAV), five corals from each of the three treatment groups were selected. On each, two sample runs were conducted on different areas of the colony. Each location was sampled at three time points.
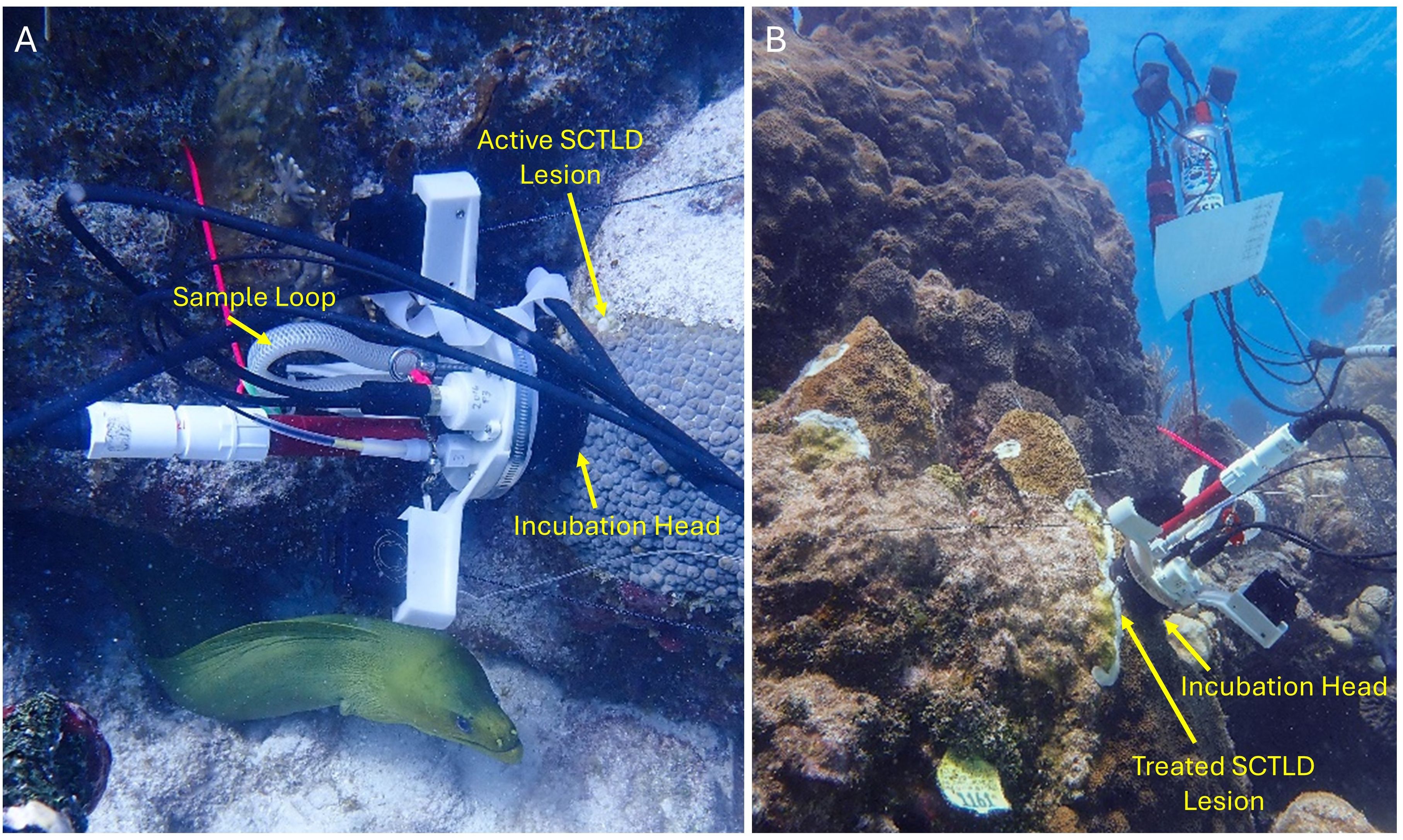
Figure 3. Imagery of the CISME unit sampling a Montastraea cavernosa coral tissue directly adjacent to an SCTLD lesion (A) and an Orbicella faveolata coral immediately following amoxicillin paste treatment on disease lesions (B). In both images, the incubation head is placed directly on the live coral tissue to measure respiration and photosynthesis in real time. An attached sample loop is removed after the sample run for later titration to determine calcification rates.
Corals selected for the diseased and treated groups had 1) an active disease lesion at least 10 cm in length, 2) enough live tissue beyond the lesion that even after one month of lesion progression, there would still be live tissue to sample, and 3) an area of tissue at least 30cm away from any active lesions to use as a “non-diseased” sample site. For O. faveolata in particular, we also preferentially selected smooth colonies over ridged ones to improve the instrumentation seal. Diseased colonies were geographically spread over approximately 0.5 km of reef.
Healthy corals of each species were defined as those having no active signs of disease or recent mortality, near 100% coral cover, and large enough to have edge- and center-colony tissue areas for sampling. These corals were more common than diseased ones and for logistical reasons were geographically more clumped. These corals received follow-up monitoring approximately every other month following this project, and none developed subsequent disease lesions for at least one year; we conclude that they were in fact healthy throughout the sampling.
The goal of 15 corals of each species was supplemented with one additional coral per species. For M. cavernosa, this resulted in a sample size of 16, while in O. faveolata two of the 16 selected corals could not be tested in at least one of the time periods due to skeletal bumps near the disease lesions which prevented a seal on the instrumentation, resulting in a sample size of 14 for that species.
Sampling protocol
Measurements of coral holobiont metabolism for Montastraea cavernosa and Orbicella faveolata colonies were taken from April 27 – June 10, 2022. The timeframe was selected to minimize other variables (Szmant et al., 2019) that could impact the metrics of interest, such as temperature variability from winter cold fronts and rapid changes in Symbiodiniaceae counts from summer bleaching events. We took measurements at the initial timepoint, one week later, and one month later. The time points were chosen to test the immediate, short-term, and medium-term potential impacts of amoxicillin treatments on physiological metrics.
On healthy corals, we conducted one sampling run within 1 cm of the corals’ natural tissue margin and another inward (30+ cm) from any tissue margins. On diseased corals, we conducted one sampling run on tissue directly adjacent to the active SCTLD lesion, with the outside of the incubation head aligning with the live tissue margin, and one on visually healthy tissue 30+ cm from the lesion. On the SCTLD-treated colonies, we applied the amoxicillin paste to any active SCTLD lesions, and then conducted the sampling run within 1 hour of application on tissue directly adjacent to the treatment (the outside of the incubation head aligned with the treatment paste). To standardize dosage, lesions were at least 10 cm in length, and the incubation head was placed so that at least 2 cm of treated lesion extended past each side of the measurement area. An additional sampling run was conducted on visually healthy tissue 30+ cm from the treatment location.
At two subsequent intervals (1 week and 1 month), the same colonies were revisited and resampled. On healthy and treated colonies, subsequent samples were at the same locations on the colony as the initial samples as indicated by two nails placed around the incubation head after the initial measurement. Of the 10 treated corals, the SCTLD lesions halted after treatment on 8 of them. On the two for which lesions did not halt, further runs were not included in analyses. On non-treated diseased colonies, all lesions continued to progress, and so, as possible, sampling occurred adjacent to the disease margin at the time of each sample. All corals continued to be part of ongoing SCTLD monitoring, and healthy corals did not develop any lesions for at least one year after the final sampling event.
Two CISME instruments were used to conduct sample runs. Briefly, CISME instrumentation consists of an electronics housing unit attached to an incubation/pump head (Figure 3). The head has a silicone foam seal that gently seals around a 5.6cm diameter section of coral, creating a closed, recirculating chamber where oxygen concentrations are measured in the seawater in contact with the coral surface. Elastic cords attached to the surrounding substrate secure the instrument. A sample tube is also affixed to the head’s flow path. Incubation samples are returned to the lab for calcification rate determinations via alkalinity anomaly versus ambient seawater after titration for total alkalinity. Instrument control and real-time readouts are available through an underwater affixed tablet. More information on the instrumentation is available in Murphy et al. (2012), and full specifications are available at https://qubitsystems.com/products/aquatic-biology/aquatic-respiration-and-photosynthesis/cisme-community-in-situ-metabolism/.
Calibration of the machines was done in-water at the start of each day of sampling. Open blanks (with sensor open to the water) and closed blanks (with sensor placed in cup) were run, with incubation samples taken and used as controls during post-sampling calcification titration tests. During calibration, real-time readouts were used to confirm pump flow and to set O2 saturation at 100% in the open blank. O2 saturation was occasionally recalibrated throughout the sampling day if values strayed from 100% between sample runs.
Barring technical problems, approximately ten corals could be assessed within a day using both instruments. Sample runs were conducted at least 3 hours after sunrise and no later than 3 hours before sunset. We sampled non-diseased colonies first, then moved onto diseased colonies, with one CISME dedicated to non-diseased areas and the other dedicated to diseased areas. Once an incubation head touched a diseased area of a colony, it was not used on a non-diseased area. Between sample runs, pumps were run continually and were flushed by wafting surrounding saltwater into the head. After each field day, the CISME units were soaked, with the pump running, in fresh water for at least 30 minutes. The incubation seal was then placed in ozonated water for at least twenty additional minutes for further decontamination.
During each sampling run, the incubation head was placed on the selected area of tissue. Elastic cords affixed to the incubation head were also attached to surrounding rocks, coral lips, or other secure points to stabilize the instrument. Flow rates within the incubation head were set at 1125 mL/min. The integrity of the seal was evaluated by looking for light leaks, and the light was turned off for one minute before the run was started to allow the coral to dark acclimate. The incubation head measured respiration during an initial 4 minutes of dark incubation, followed by 16 minutes of light incubation at 450 µEins/m2/s. Preliminary photosynthesis-irradiance (P/I) curves measured for three colonies of each of the two species approximately two months before the experiment identified this value as greater than the peak Δ O2 values (Supplementary Figure 2), ensuring that net photosynthesis would not be light limited during the experiment.
Respiration rates (R) were calculated from the Δ O2 during dark respiration. Net photosynthesis was calculated from the Δ O2 during light incubation (from 2-4 minutes after the light turned on) (Figure 4). Gross photosynthesis was then calculated by adding the respiration rate to the net photosynthesis rate. Photosynthesis to respiration ratios (P/R) were calculated by dividing gross photosynthesis by respiration rates. The sample tubes were removed after the light incubation period and fixed at the end of the day in mercuric chloride. These samples were processed via titration for calculation of calcification rates at UNC Wilmington. Briefly, sea water alkalinity was measured using an open-cell, potentiometric titration. The seawater sample was acidified to a pH of approximately 3.0 in a two-stage hydrochloric acid titration. The final titration was done in 0.05 cm3 increments, recording dispensed volume, electromotive force, and temperature and calculated using a non-linear least-squares approach. The full operating procedures are outlined in SOP3b of the Guide to Best Practices for Ocean CO2 Measurements (Dickson et al., 2007).
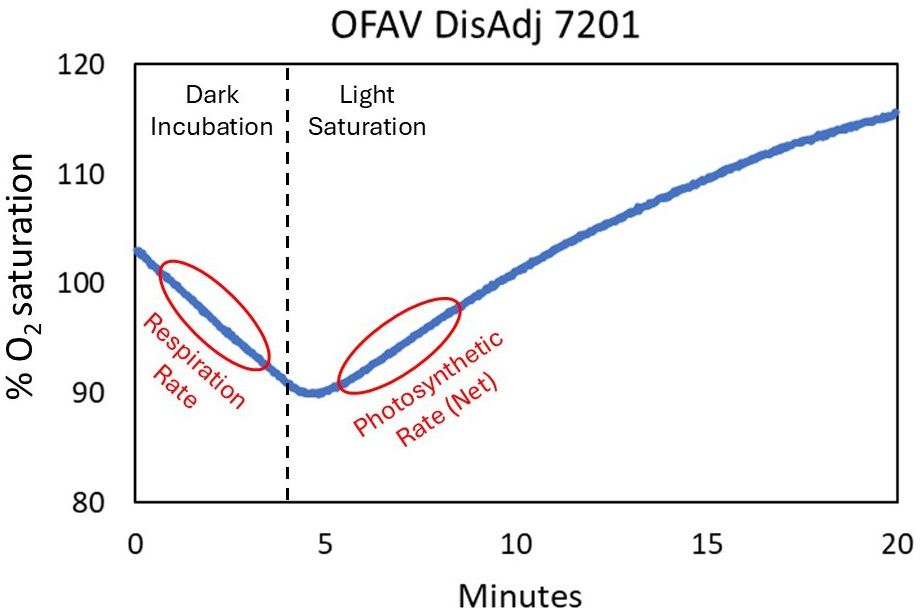
Figure 4. Example readout of a sampling run (this example: placement adjacent to active disease on Orbicella faveolata #7201). The CISME measures oxygen saturation within the incubation head chamber throughout the 20-minute run. The first four minutes are in the dark, with no photosynthesis occurring, and the negative slope in O2 saturation is calculated to respiration rate. Once the light is turned on, the Symbiodiniaceae begin photosynthesizing, and the stable slope increase in O2 saturation is calculated to net photosynthesis.
Statistical analyses
We compared photosynthesis, respiration, P/R, and calcification metrics across the treatment groups (healthy corals edge, healthy corals non-edge, diseased corals adjacent to the lesion, diseased corals far from the lesion, treated corals near the treatment line, treated corals far from the treatment line), and across the three time periods (within 1 hour of treatment: T0, ~1 week after treatment: T1week, and ~1 month after treatment: T1month) using a 2-way ANOVA with Holm-Sidak pairwise comparisons.
Results
Respiration, photosynthesis, P/R, and calcification rates were calculated for each sample run. Similar to results by Lesser et al. (2000), respiration and photosynthetic rates were both notably higher for O. faveolata colonies (Respiration median: 1020 nmol O2/cm2/h. Photosynthesis median: 1629 nmol O2/cm2/h) than for M. cavernosa colonies (Respiration median: 475 nmol O2/cm2/h. Photosynthesis median: 1026 nmol O2/cm2/h), and thus the two species are presented independently.
Among O. faveolata colonies, there was no treatment effect on respiration (2-way ANOVA: p = 0.2. See Supplementary Table 1 for full test results) or photosynthesis (p = 0.2). However, time was a significant factor (p < 0.001 for both respiration and photosynthesis). Respiration rates were significantly different among all three time points, with rates declining across each time point. There was no significant interaction between the two variables. Across all treatments and time periods, P/R ratios were greater than 1, and there was no significant treatment effect, time effect, or interaction. Calcification rates had no significant treatment, time, or interaction effects (Figure 5).
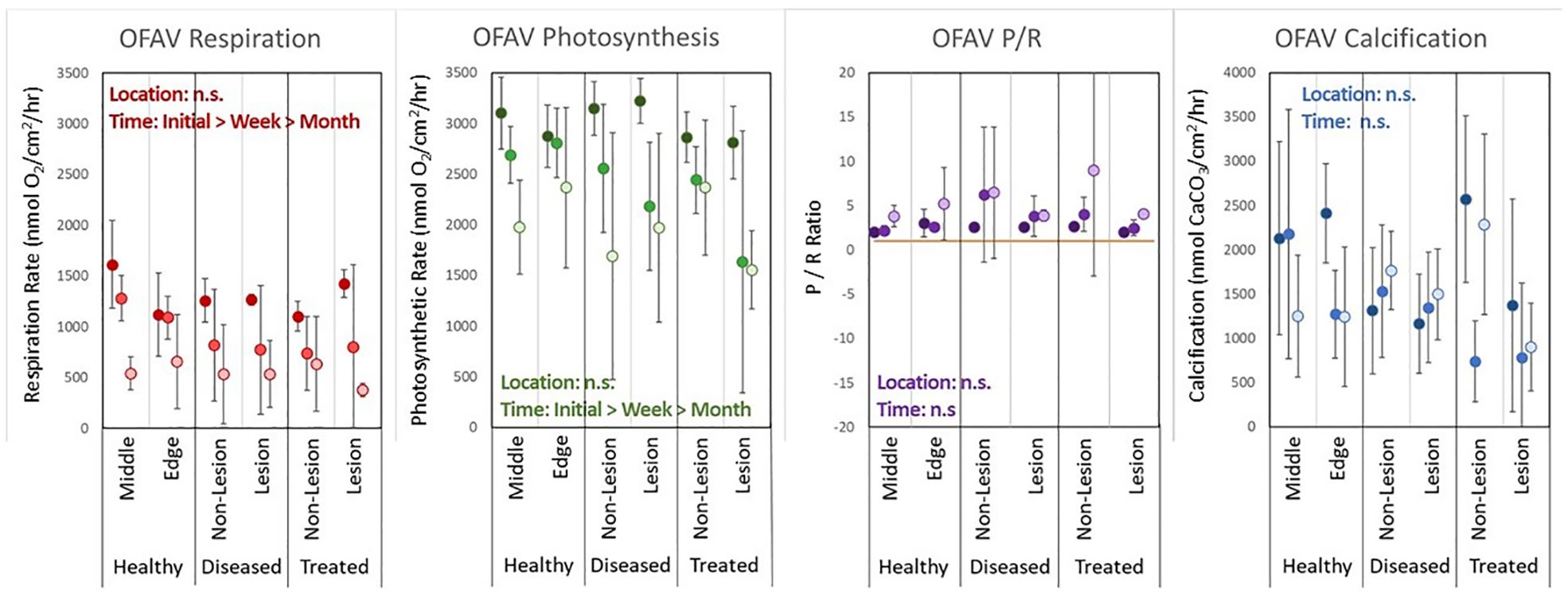
Figure 5. Averages (error bars = standard deviation) of respiration rates, photosynthesis rates, P/R ratios, and calcification rates for each treatment group and each time period (darkest: T0, mid-grade: T1week, lightest: T1month) for Orbicella faveolata (OFAV) corals.
Among M. cavernosa colonies, treatment had no significant impact on respiration rates (p = 0.1). Time did have a significant effect (p < 0.001), with T1month rates significantly lower than T0 rates (p < 0.001) and T1week rates (p = 0.04) (Figure 6). Photosynthetic rates did vary significantly when all treatment groups were compared (p = 0.01), but no pair-wise comparisons were significant. Photosynthetic rates were significantly lower at T1month than at T0 (p < 0.001). P/R ratios did not differ based on treatment (p = 0.1) nor time (p = 0.3). Calcification rates for M. cavernosa did not vary by treatment group (p = 0.96) but did vary by time (p < 0.001), with rates at T1week significantly lower than those at T0 (p < 0.001) and T1month (p = 0.007).
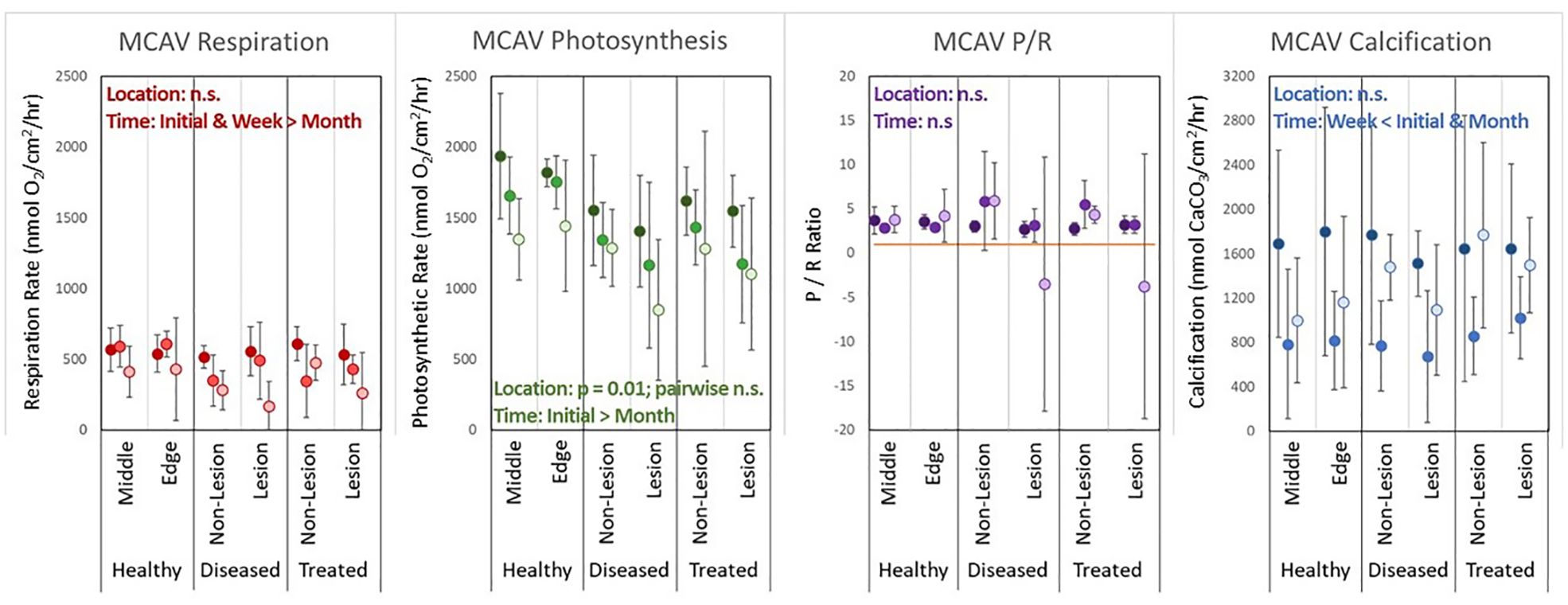
Figure 6. Averages (error bars = standard deviation) of respiration rates, photosynthesis rates, P/R ratios, and calcification rates for each treatment group and each time period (darkest: T0, mid-grade: T1week, lightest: T1month) for Montastraea cavernosa (MCAV) corals.
Discussion
The CISME instrumentation allowed for various metrics of coral physiology to be measured simultaneously, in the field, and across repeated time points without causing damage to the coral. Across both species and all time points, there was no effect of treatment on respiration, photosynthesis, P/R ratios, or calcification rates. The location of the sampling run on the coral (in the middle of tissue or near the edge) and the health status of the coral (healthy, actively diseased, or amoxicillin treated), did not cause any variation in the physiological parameters measured. This suggests that amoxicillin treatments do not result in detrimental nor positive effects to adjacent coral metabolism.
For both species, the respiration and photosynthetic rates across all treatments declined slightly but significantly with time (T0, T1week, T1month). We can only hypothesize as to the cause of this, but offer three possible explanations. The first is mechanical, as the seals on the CISME instrumentation may have become more worn with time and thus did not provide a complete seal, resulting in lowered rates during later sampling dates. However, this would likely have also affected calcification measurements, but these did not vary across time. It is also possible that environmental conditions changed over the three sampling periods, resulting in changing metabolic rates of the colonies. The two most likely changes during this time period would have been light (which increased 38 minutes between sunrise and sunset between the first sampling timepoint and the last), and temperature [which increased 2.7°C during the same interval (NOAA Coral Reef Watch, 2023). We would not expect either of these changes to have made a notable difference, as we standardized light exposure by sampling at least three hours after sunrise and before sunset. Additionally, the temperature range between the first and last sample point (25.9 – 28.6°C) was found by Colombo-Pallotta et al. (2010) to not create notable variation in dark respiration rates for O. faveolata. It is possible that other environmental parameters, such as water quality, may have changed between sample points and affected results, though no notable weather conditions or unusual events occurred during the sampling month that might create deviations. Though very high turbidity is known to affect photosynthesis and respiration (Telesnicki and Goldberg, 1995), lower values as seen on this reef (with similar observations of clarity across all sample dates) are not known to do so. A third alternative may be that coral health declined for some other reason during this time interval. This change did not manifest in any visible way such as tissue loss or changes in coral color, but we cannot discount the possibility of non-visible changes in health status. There is much still to be learned about coral health and, particularly, changes in health through time, and we encourage future use of these metrics on fate tracked corals to assess both natural temporal patterns as well as changes that may be caused by unusual environmental or management events.
Measured physiological parameters of our sampled corals show some variation from other studies of the same species. The respiration rates in this study were generally lower than those found by Colombo-Pallotta et al. (2010) for O. faveolata and by Lasker (1981) for M. cavernosa. Photosynthetic rates were within the range of, but on the high side of measurements found by Lesser et al. (2000) for O. faveolata and by both Lesser et al. (2000) and Lasker (1981) for M. cavernosa. Again, the reasons for these variations are unknown, but could relate to numerous short- or long-term environmental variables among studies, some of which could be resolved in future controlled laboratory experiments. The use of the CISME instrumentation provides an opportunity to do these long-term studies as it allows in-water measurements on colonies of all sizes without any damage to the sample area.
While we find no changes in photosynthesis, respiration, or calcification rates across the three treatment groups in this study, we note that there may be limitations to extrapolating these results. These include:
1. SCTLD lesions can present on O. faveolata and M. cavernosa as either stark lines between apparently healthy tissue and dead skeleton or as lesions with a bleached margin of varying thickness (Florida Coral Disease Response Research & Epidemiology Team, 2018; Aeby et al., 2019). The tissues sampled in this experiment were from stark lesions with no bleaching margins. We would expect that for corals exhibiting a substantial level of paling/bleaching associated with disease lesions, photosynthetic rates would be lower.
2. Results could vary under different environmental conditions. For example, Liu et al. (2015) found that amoxicillin did not affect growth of a photosynthetic cyanobacteria at low phosphorous levels, but did stimulate it at high levels. It is possible that the impact of amoxicillin treatments on physiological metrics of coral hosts or symbionts could vary at other temporal or geographical points.
3. The sampling time periods selected for treated corals (< 1 hour, 1 week, and 1 month) may not adequately capture all possible impacts. These time periods were chosen to reflect any immediate impacts of the treatment itself, the time after the full 3-day dosing had occurred, and time well after treatment when no impacts would be expected to be seen. Though we found that none of these timepoints differed from controls, it is possible that intermediate time points may have yielded different results. However, Studivan et al. (2023) found that gene pathways 13 days after treatments indicated potential recovery of corals, and so we suggest that by this point, physiological metrics would not be expected to vary from controls.
While the temporal variability of our sampled corals offers opportunities for future work on coral health, the lack of variation among the treatment groups suggests that within an individual coral, physiological parameters are relatively consistent across the colony. Further, it suggests that neither SCTLD nor amoxicillin treatments are impacting respiration, photosynthesis, or calcification in adjacent tissues. As this relates to intervention as a management action, the use of this novel in-water action to prevent mortality does not appear to have negative impacts on the surrounding tissues.
Data availability statement
The raw data supporting the conclusions of this article will be made available by the authors, without undue reservation.
Author contributions
KN: Conceptualization, Data curation, Formal analysis, Funding acquisition, Investigation, Methodology, Project administration, Supervision, Writing – original draft, Writing – review & editing. RW: Conceptualization, Data curation, Methodology, Project administration, Resources, Validation, Writing – review & editing. MD: Writing – review & editing.
Funding
The author(s) declare that financial support was received for the research, authorship, and/or publication of this article. This work was supported by the Florida Department of Environmental Protection’s Office of Resilience and Coastal Protection purchase order BA1BE4.
Acknowledgments
We are grateful for substantial fieldwork and topside assistance by Kayla Merrill, Nicole Charnock, and Sydney Gallagher. We also thank Alina Szmant who provided conceptual and practical guidance on experimental design and use of the CISME instrumentation. All work was conducted under Florida Keys National Marine Sanctuary permit FKNMS-2021-247.
Conflict of interest
The authors declare that the research was conducted in the absence of any commercial or financial relationships that could be construed as a potential conflict of interest.
Publisher’s note
All claims expressed in this article are solely those of the authors and do not necessarily represent those of their affiliated organizations, or those of the publisher, the editors and the reviewers. Any product that may be evaluated in this article, or claim that may be made by its manufacturer, is not guaranteed or endorsed by the publisher.
Supplementary material
The Supplementary Material for this article can be found online at: https://www.frontiersin.org/articles/10.3389/fmars.2024.1460163/full#supplementary-material
References
Aeby G., Ushijima B., Campbell J. E., Jones S., Williams G., Meyer J. L., et al. (2019). Pathogenesis of a tissue loss disease affecting multiple species of corals along the Florida Reef Tract. Front. Mar. Sci. 6. doi: 10.3389/fmars.2019.00678
Al-Horani F. A. (2005). Effects of changing seawater temperature on photosynthesis and calcification in the scleractinian coral Galaxea fascicularis, measured with O2, Ca2+ and pH microsensors. Scientia Marina 69, 347–354. doi: 10.3989/scimar.2005.69n3347
Alvarez-Filip L., González-Barrios F. J., Pérez-Cervantes E., Molina-Hernández A., Estrada-Saldívar N. (2022). Stony coral tissue loss disease decimated Caribbean coral populations and reshaped reef functionality. Commun. Biol. 5, 1–10. doi: 10.1038/s42003-022-03398-6
Brandt M. E., Ennis R. S., Meiling S. S., Townsend J., Cobleigh K., Glahn A., et al. (2021). The emergence and initial impact of stony coral tissue loss disease (SCTLD) in the United States Virgin Islands. Front. Mar. Sci. 8, 1105. doi: 10.3389/fmars.2021.715329
Coles S., Jokiel P. (1977). Effects of temperature on photosynthesis and respiration in hermatypic corals. Mar. Biol. 43, 209–216. doi: 10.1007/BF00402313
Colombo-Pallotta M., Rodríguez-Román A., Iglesias-Prieto R. (2010). Calcification in bleached and unbleached Montastraea faveolata: evaluating the role of oxygen and glycerol. Coral Reefs 29, 899–907. doi: 10.1007/s00338-010-0638-x
Connelly M. T., Mcrae C. J., Liu P.-J., Martin C. E., Traylor-Knowles N. (2022). Antibiotics alter Pocillopora coral-symbiodiniaceae-bacteria interactions and cause microbial dysbiosis during heat stress. Front. Mar. Sci. 8. doi: 10.3389/fmars.2021.814124
Dellisanti W., Tsang R. H., Ang J. P., Wu J., Wells M. L., Chan L. L. (2020a). A diver-portable respirometry system for in-situ short-term measurements of coral metabolic health and rates of calcification. Front. Mar. Sci. 7, 932. doi: 10.3389/fmars.2020.571451
Dellisanti W., Tsang R. H., Ang P. Jr., Wu J., Wells M. L., Chan L. L. (2020b). Metabolic performance and thermal and salinity tolerance of the coral Platygyra carnosa in Hong Kong waters. Mar. pollut. Bull. 153, 111005. doi: 10.1016/j.marpolbul.2020.111005
Dickson A. G., Sabine C. L., Christian J. R. (2007). Guide to Best Practices for Ocean CO2 Measurements Vol. 3 (Sidney, BC Canada: PICES Special Publication).
Edmunds P. J. (2005). Effect of elevated temperature on aerobic respiration of coral recruits. Mar. Biol. 146, 655–663. doi: 10.1007/s00227-004-1485-5
Favero M., Curtis K. (2020). Creation and Release Profiles of Novel Biodegradable Ointments for Lesion Based and Whole Colony Treatment Methods to be Utilized in the Response to Stony Coral Tissue Loss Disease (SCTLD) (Miami, FL: Florida DEP).
Florida Coral Disease Response Research & Epidemiology Team (2018). Case definition: Stony coral tissue loss disease. Available online at: https://floridadep.gov/sites/default/files/Copy%20of%20StonyCoralTissueLossDisease_CaseDefinition%20final%2010022018.pdf. (Accessed October 2, 2018).
Forrester G. E., Arton L., Horton A., Nickles K., Forrester L. M. (2022). Antibiotic treatment ameliorates the impact of stony coral tissue loss disease (SCTLD) on coral communities. Front. Mar. Sci. 9. doi: 10.3389/fmars.2022.859740
Gomes M. P., Brito J. C. M., Rocha D. C., Navarro-Silva M. A., Juneau P. (2020). Individual and combined effects of amoxicillin, enrofloxacin, and oxytetracycline on Lemna minor physiology. Ecotoxicology Environ. Saf. 203, 111025. doi: 10.1016/j.ecoenv.2020.111025
González-Pleiter M., Gonzalo S., Rodea-Palomares I., Leganés F., Rosal R., Boltes K., et al. (2013). Toxicity of five antibiotics and their mixtures towards photosynthetic aquatic organisms: implications for environmental risk assessment. Water Res. 47, 2050–2064. doi: 10.1016/j.watres.2013.01.020
Heres M. M., Farmer B. H., Elmer F., Hertler H. (2021). Ecological consequences of stony coral tissue loss disease in the Turks and Caicos Islands. Coral Reefs 40, 609–624. doi: 10.1007/s00338-021-02071-4
Junjie R. K., Browne N. K., Erftemeijer P. L., Todd P. A. (2014). Impacts of sediments on coral energetics: partitioning the effects of turbidity and settling particles. PLoS One 9, e107195. doi: 10.1371/journal.pone.0107195
Krueger Q., Phippen B., Reitzel A. (2024). Antibiotics alter development and gene expression in the model cnidarian Nematostella vectensis. PeerJ 12, e17349. doi: 10.7717/peerj.17349
Lasker H. R. (1981). Phenotypic variation in the coral Montastrea cavernosa and its effects on colony energetics. Biol. Bull. 160, 292–302. doi: 10.2307/1540889
Lesser M. P. L., Mazel C., Phinney D., Yentsch C. S. (2000). Light absorption and utilization by colonies of the congeneric hermatypic corals Montastraea faveolata and Montastraea cavernosa. Limnology Oceanography 45, 76–86. doi: 10.4319/lo.2000.45.1.0076
Liu Y., Chen X., Zhang J., Gao B. (2015). Hormesis effects of amoxicillin on growth and cellular biosynthesis of Microcystis aeruginosa at different nitrogen levels. Microbial Ecol. 69, 608–617. doi: 10.1007/s00248-014-0528-9
Murphy B. A., Mazel C. H., Whitehead R., Szmant A. M. (2012). “CISME: A self-contained diver portable metabolism and energetics system,” in 2012 Oceans. (Oceans, Hampton Roads, VA, USA: IEEE). 1–7. doi: 10.1109/OCEANS.2012.6405075
Neely K. (2023). Florida Keys Coral Disease Intervention Strike Teams: Final Report (Miami, FL: Florida DEP).
Neely K. L., Lewis C. L., Lunz K. S., Kabay L. (2021a). Rapid population decline of the pillar coral Dendrogyra cylindrus along the Florida reef tract. Front. Mar. Sci. 8. doi: 10.3389/fmars.2021.656515
Neely K. L., Macaulay K. A., Hower E. K., Dobler M. A. (2020). Effectiveness of topical antibiotics in treating corals affected by Stony Coral Tissue Loss Disease. PeerJ 8, e9289. doi: 10.7717/peerj.9289
Neely K. L., Shea C. P., Macaulay K. A., Hower E. K., Dobler M. A. (2021b). Short- and long-term effectiveness of coral disease treatments. Front. Mar. Sci. 8. doi: 10.3389/fmars.2021.675349
NOAA Coral Reef Watch (2023). Florida 5 km single-pixel virtual station time series graphs. Available online at: https://coralreefwatch.noaa.gov/product/vs_single_pixel_exp/florida_keys.phpCheecaRocks (Accessed 2024-07-01).
Pan X., Deng C., Zhang D., Wang J., Mu G., Chen Y. (2008). Toxic effects of amoxicillin on the photosystem II of Synechocystis sp. characterized by a variety of in vivo chlorophyll fluorescence tests. Aquat. Toxicol. 89, 207–213. doi: 10.1016/j.aquatox.2008.06.018
Reynaud S., Leclercq N., Romaine-Lioud S., Ferrier-Pagés C., Jaubert J., Gattuso J. P. (2003). Interacting effects of CO2 partial pressure and temperature on photosynthesis and calcification in a scleractinian coral. Global Change Biol. 9, 1660–1668. doi: 10.1046/j.1365-2486.2003.00678.x
Shilling E. N., Combs I. R., Voss J. D. (2021). Assessing the effectiveness of two intervention methods for stony coral tissue loss disease on Montastraea cavernosa. Sci. Rep. 11, 1–11. doi: 10.1038/s41598-021-86926-4
Studivan M. S., Eckert R. J., Shilling E., Soderberg N., Enochs I. C., Voss J. D. (2023). Stony coral tissue loss disease intervention with amoxicillin leads to a reversal of disease-modulated gene expression pathways. Mol. Ecol. 32, 5394–5413. doi: 10.1111/mec.v32.19
Szmant A. M., Whitehead R. F., Farmer J. (2019). CISME (Coral In Situ MEtabolism) A novel diver-portable underwater respirometer for the in situ measurement of the metabolic rates of benthic organisms (Dominican Republic: Association of Marine Labs of the Caribbean).
Telesnicki G. J., Goldberg W. M. (1995). Effects of turbidiy on the photosynthesis and respiration of two south Florida reef coral species. Bull. Mar. Sci. 57, 527–539.
Walker B. K., Noren H., Buckley S., Pitts K. (2021). Optimizing stony coral tissue loss disease (SCTLD) intervention treatments on Montastraea cavernosa in an endemic zone. Front. Mar. Sci. 8, 746. doi: 10.3389/fmars.2021.666224
Keywords: stony coral tissue loss disease, Orbicella faveolata, Montastraea cavernosa, photosynthesis, respiration, P/R, calcification, amoxicillin
Citation: Neely KL, Whitehead RF and Dobler MA (2024) The effects of disease lesions and amoxicillin treatment on the physiology of SCTLD-affected corals. Front. Mar. Sci. 11:1460163. doi: 10.3389/fmars.2024.1460163
Received: 05 July 2024; Accepted: 21 October 2024;
Published: 08 November 2024.
Edited by:
Esti Kramarsky-Winter, Ben-Gurion University of the Negev, IsraelReviewed by:
Lisa May, Consolidated Safety Services (United States), United StatesAine Hawthorn, United States Geological Survey, United States
Copyright © 2024 Neely, Whitehead and Dobler. This is an open-access article distributed under the terms of the Creative Commons Attribution License (CC BY). The use, distribution or reproduction in other forums is permitted, provided the original author(s) and the copyright owner(s) are credited and that the original publication in this journal is cited, in accordance with accepted academic practice. No use, distribution or reproduction is permitted which does not comply with these terms.
*Correspondence: Karen L. Neely, a25lZWx5MEBub3ZhLmVkdQ==