- 1School of Ocean and Earth Science, University of Southampton, National Oceanography Centre Southampton, European Way, Southampton, United Kingdom
- 2Hopkins Marine Station, Stanford University, Pacific Grove, CA, United States
- 3Ocean Sciences & Institute for Marine Sciences, University of California, Santa Cruz, Santa Cruz, CA, United States
- 4Wildscope, Cádiz, Spain
- 5Alfred Wegener Institute Helmholtz Centre for Polar and Marine Research, Bremerhaven, Germany
- 6Marine Ecology Research Centre, Southern Cross University, Lismore, NSW, Australia
- 7Happywhale, Santa Cruz, CA, United States
- 8Projeto Baleias/Brazilian Antarctic Program, Laboratório de Ecologia e Conservação da Megafauna Marinha, Instituto de Oceanografia, Universidade Federal do Rio Grande - FURG, Rio Grande, Brazil
- 9Institute of Marine Ecosystem and Fishery Science, Center for Earth System Research and Sustainability, University of Hamburg, Hamburg, Germany
- 10Cooperative Institute for Climate, Ocean and Ecosystem Studies (CICOES), University of Washington, Seattle, WA, United States
- 11Marine Mammal Laboratory, Alaska Fisheries Science Center, National Marine Fisheries Service, National Oceanic and Atmospheric Administration, Seattle, WA, United States
- 12Marine Ecology and Telemetry Research, Seabeck, WA, United States
We consider how the ongoing recovery of baleen whale populations requires improved understanding when managing the largest commercial fishery in the Southern Ocean, the fishery for Antarctic krill. Baleen whales already represent one of the major consumers of krill, and as such compete with the fishery. However, they are not explicitly included either in the monitoring programme or the krill fisheries management approach of the Commission for the Conservation of Antarctic Marine Living Resources (CCAMLR). Individual baleen whales are at direct mortality risk from ship strike, entanglement, or by-catch. In addition, sub-lethal impacts on individuals, albeit with population-level consequences, via decreased body condition and reproductive rates, may arise from local depletion of prey and prey-field disturbance that increases whale energetic costs. Mitigating these risks requires that baleen whales, including those species not yet recovered, are fully integrated into management procedures. A vital issue will be to facilitate the ongoing recovery of blue, fin and sei whales, populations of which are still heavily depleted relative to their pre-whaling abundance. Part of the answer to this will be how CCAMLR resolves outstanding issues related to its newly emerging revised krill fishery management framework. Management of the krill fishery is data-poor, with much of the scientific support reliant upon data that are now decades old. The decision rules for determining sustainable yields need careful re-evaluation, given they were negotiated at a time when baleen whales were rarely observed, and krill fishery catches were low in relation to the estimated available krill biomass. The Antarctic marine ecosystem is spatially and temporally dynamic, and is changing, yet CCAMLR still assumes a static system. An explicit commitment to collect up-to-date monitoring data about krill and baleen whales is vital to help inform the revised management framework. Precaution argues that catch levels should not increase until adequate data are available for effective management. Knowledge about the status of baleen whales will inevitably require close collaboration with the International Whaling Commission.
1 Introduction
Human impacts in the Southern Ocean have lagged behind those of more-northerly latitudes (Halpern et al., 2008; 2015), including for living resource exploitation. Nevertheless, high-value marine species (e.g., seals and whales) were targeted from the early 17th century, before interests shifted to species of lesser commercial value (see Pauly et al., 1998; Jackson et al., 2001; Croxall and Trathan, 2004). Today, the consequences of that early exploitation continue to have ramifications, not just for those species harvested historically, but also for other ecosystem components, including modern day fisheries. Some species were targeted outside our primary geographic focus, the southwest Atlantic (Figure 1), but connectivity through seasonal migration would nevertheless have influenced our core area of interest.
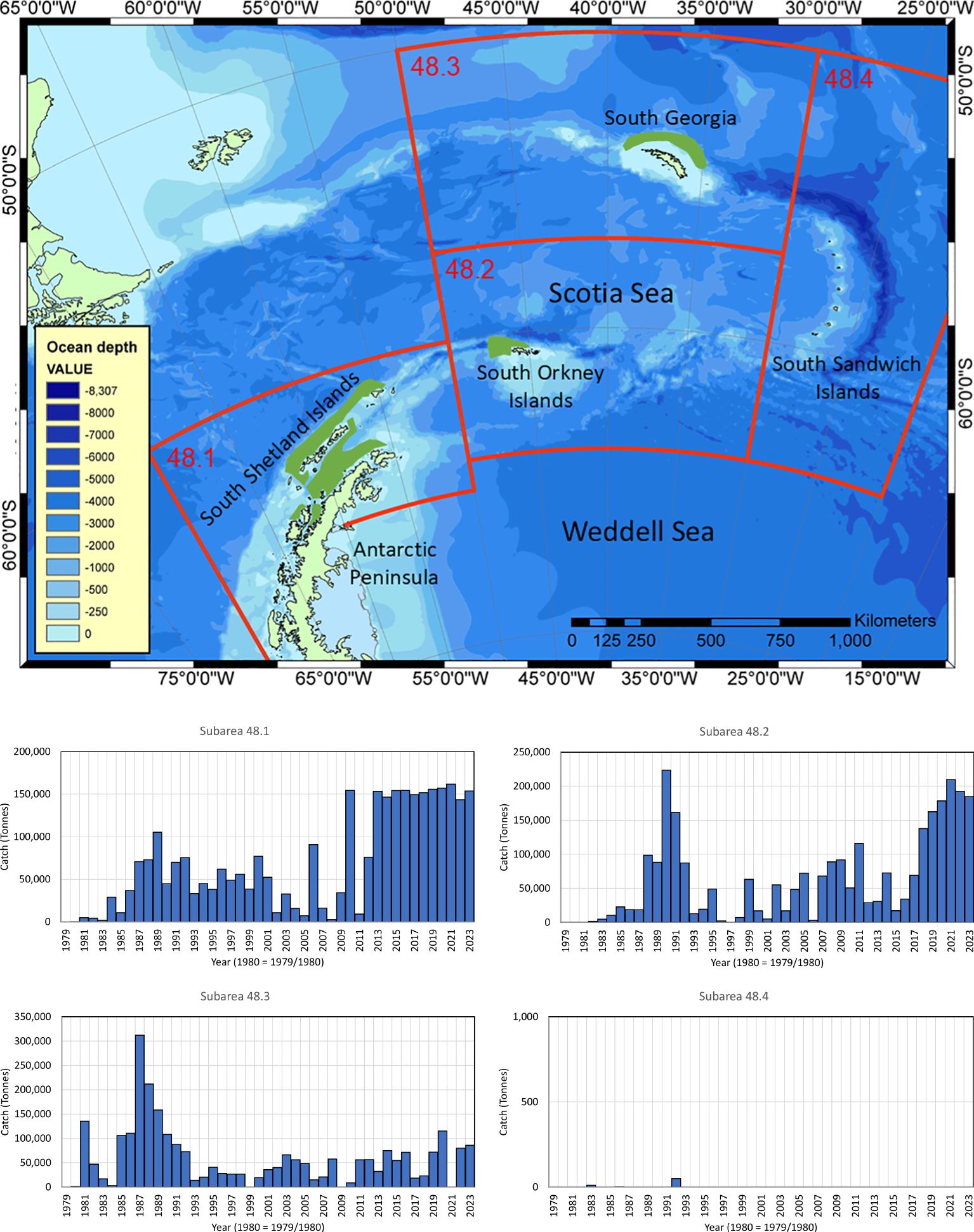
Figure 1. (Map) The southwest Atlantic (Antarctic Peninsula, Scotia Sea and northern Weddell Sea) with FAO Subareas 48.1 (Antarctic Peninsula and South Shetland Islands), 48.2 (South Orkney Islands), 48.3 (South Georgia) and 48.4 (South Sandwich Islands). The fishery for krill operates primarily in coastal waters to the north of the Peninsula, and to the north of the island archipelagos; green polygons are redrawn from Warwick-Evans et al. (2018) and Trathan et al. (2021; 2022b). (Histograms) Catch histories are derived from CCAMLR (2023a; 2023c); note y-axes differ in the histograms.
The changing status of the Southern Ocean and its ecological communities are currently the centre of an important scientific, economic and political debate that is primarily set in the southwest Atlantic, principally along the Antarctic Peninsula and within the Scotia Sea (FAO Subareas 48.1, 48.2, 48.3 and 48.4). In this paper, we consider the fishery for Antarctic krill (Euphausia superba; hereafter, krill) and the implications of ecosystem recovery following historical over-exploitation, particularly the recovery of krill-eating baleen whales. Our focus is on those locations where the highest krill catches have been taken over the past 40 years and where different baleen whale species are increasingly abundant (e.g., Hedley et al., 2001; Baines et al., 2021; 2022; Herr et al., 2022; Biuw et al., 2024).
This review follows from a Workshop (attended by 33 people, in person and on-line) held at the Oxford University Museum of Natural History in October 2023. The Workshop considered the need to improve scientific understanding about interactions between baleen whales and the commercial krill fishery. The Workshop provided the authors with the impetus to develop this paper, augmenting material presented at the Workshop with discussions after the Workshop, and scientific insights from all authors.
We consider how effective management of the krill fishery may be improved so that it meets the challenges presented by a variable and changing ecosystem. In section 1, we provide a brief overview of historical exploitation in the southwest Atlantic, including development of the modern-day legal management instruments. In section 2, we detail the current management approach for the krill fishery, and explore the revised management framework under active development. In section 3, we consider interactions between the krill fishery and recovering populations of baleen whales. In section 4, we discuss the energetic demands of baleen whales, whilst in section 5, we review baleen whale population status. In section 6, we identify knowledge gaps in krill fishery management together with plausible alternative futures for the Southern Ocean. In section 7, we consider future management steps, and in section 8, offer a number of conclusions.
The krill fishery is a legal fishery, but it is data-poor and has been slow to develop. However, economic interests are now leading to increased catches (see CCAMLR, 2023a), with approximately 500,000 t (metric tonnes) taken in the 2023/24 fishing season. To prevent any repeat of ineffective regulation (as with historical sealing, whaling, and fishing), it is essential to ensure that krill fishery management is fit for purpose, including with effective precautionary ecosystem safeguards, given that impacts of krill fishing remain poorly understood.
1.1 Historical exploitation in the southwest Atlantic
Until the early 1600s, the southwest Atlantic remained relatively unaffected by human activities. However, as humans ventured southward seeking new economic opportunities, marine living resource exploitation gradually gained pace. Slow moving southern right whales (Eubalaena australis) were one of the first species targeted, primarily on their wintering grounds off the coast of modern-day Brazil (Hart and Edmundson, 2017; Vieira, 2023). Initially, in the early 17th century, shore-based whaling stations were used, but by the 18th and 19th centuries North American and British pelagic operations dominated (Townsend, 1935; Tønnessen and Johnsen, 1982; Smith et al., 2012). As these industries developed, North American and British sealers also began to exploit the abundant Antarctic fur seal (Arctocephalus gazella) rookeries at South Georgia and other island archipelagos (Payne, 1977; Grant, 2001). As some of these stocks also declined, and as whaling methods evolved, exploitation again shifted, so that by the beginning of the 20th century, harvesting of other baleen whale species and sperm whales (Physeter macrocephalus) (i.e., the “great whales”) was well established. Later, with the demise of the great whales, other species were sought, so that by the latter half of the 20th century, fishing for finfish and krill had begun. Much of the recent history is well-documented (Laws, 1953; Bonner, 1980; 1984; Everson, 1977; Kock, 1992; Trathan and Reid, 2009; Hofman, 2017; 2019; Hart, 2020; 2021). Antarctic whaling finally came to an end in 2018/19 when Japan ended ‘scientific’ whaling in the Southern Ocean (Hofman, 2019).
1.2 Modern regulation of marine living resource exploitation
In 1980, the multilateral Convention on the Conservation of Antarctic Marine Living Resources (hereafter, CAMLR Convention) was agreed, so that exploitation of marine biodiversity was finally brought under strict international control. Importantly, the establishment of the CAMLR Convention was rooted in the whaling problem (Hofman, 2019). The CAMLR Convention is implemented by a Commission (the Commission for the Conservation of Antarctic Marine Living Resources; hereafter, CCAMLR) comprising 26 Member States plus the European Union. The Commission is advised by a Scientific Committee. In 1982, living resource exploitation was further controlled when the International Whaling Commission (IWC) decided that there should be a pause in commercial whaling on all whale species and populations from the 1985/86 season onwards (IWC, 1983). This pause is often referred to as the commercial whaling moratorium, and remains in place today. The IWC comprises 88 Member States, and is also advised by a Scientific Committee. Between them, CCAMLR and the IWC have responsibility for managing the living resources across the Southern Ocean. Although CCAMLR does not regulate whaling, that being the remit of the IWC, it must remain mindful of the role of whales in the ecosystem. See Hofman (2017) for a discussion about the origins of both IWC and CCAMLR.
The serial over-exploitation of living resources led to enormous ecological change in the southwest Atlantic, much of which is not well understood. Many of the species targeted (e.g., Antarctic fur seals, various baleen whale species, demersal fish species) relied upon krill as a key dietary component. Krill is now the target of the largest commercial fishery (by weight) in the Southern Ocean, with all catches currently taken from the southwest Atlantic (CCAMLR, 2023a). This juxtaposition of historical and modern-day exploitation now presents important management challenges for CCAMLR, albeit challenges foreseen by the authors of the CAMLR Convention (Box 1).
Box 1. Article II from the CAMLR Convention (CCAMLR, 1980), states:
1 The objective of this Convention is the conservation of Antarctic marine living resources.
2 For the purposes of this Convention, the term ‘conservation’ includes rational use.
3 Any harvesting and associated activities in the area to which this Convention applies shall be conducted in accordance with the provisions of this Convention and with the following principles of conservation:
a) prevention of decrease in the size of any harvested population to levels below those which ensure its stable recruitment. For this purpose its size should not be allowed to fall below a level close to that which ensures the greatest net annual increment;
b) maintenance of the ecological relationships between harvested, dependent and related populations of Antarctic marine living resources and the restoration of depleted populations to the levels defined in sub-paragraph (a) above; and
c) prevention of changes or minimisation of the risk of changes in the marine ecosystem which are not potentially reversible over two or three decades, taking into account the state of available knowledge of the direct and indirect impact of harvesting, the effect of the introduction of alien species, the effects of associated activities on the marine ecosystem and of the effects of environmental changes, with the aim of making possible the sustained conservation of Antarctic marine living resources.
Article II is key for interpreting how CCAMLR must manage modern harvesting and the need to restore historically depleted populations. Further, Article II also offers advice about climate change, an issue that increases management uncertainty.
Today, only three marine species are commercially exploited in the southwest Atlantic (Agnew, 2004); krill, mackerel icefish (Champsocephalus gunnari) and Patagonian toothfish (Dissostichus eleginoides). In this paper, we focus upon the krill fishery.
2 Management of the krill fishery
Vessels from the former Soviet Union and Japan initiated exploratory fishing for krill in the Antarctic in the 1960s (Hofman, 2017). However, the CCAMLR database only holds data on krill catches from 1973. Since then, catches of krill have been reported by 19 nations. Over the past decade, six nations have fished for krill (CCAMLR, 2023a).
2.1 CCAMLR’s krill yield model
CCAMLR sets a precautionary catch limit for the krill fishery using a set of decision rules (Box 2) that estimate the proportion of the krill stock that could be fished whilst still achieving the objective of the CAMLR Convention (CCAMLR, 2023a). This precautionary catch limit is set on the basis of a sustainable yield (gamma; see Box 2) that can be taken as a constant catch. CCAMLR determines the yield using a stochastic stock assessment model intended to assess the status of the krill stock under various levels of uncertainty (Constable and de la Mare, 1996). The latest model is coded in R and is publicly available at https://github.com/ccamlr/Grym_Base_Case/tree/Simulations. The precautionary catch limit is derived using the yield estimator from the model and an estimate of stock biomass derived from a large-scale acoustic survey undertaken in 2000 (Fielding et al., 2011). A large-scale survey was used to avoid complexities associated with movement of krill within the ecosystem.
Box 2. The following rules (Butterworth et al., 1992; Constable et al., 2000) are applied to determine the precautionary harvest rate for krill:
1. Choose the yield, gamma1, such that the probability of the spawning biomass dropping below 20% of its median pre-exploitation level over a 20-year harvesting period is 10%.
2. Choose the yield, gamma2, such that the median escapement at the end of a 20-year period is 75% of the median pre-exploitation level.
3. Select the lower of gamma1 and gamma2 as the sustainable yield.
The precautionary catch limit (PCL) is derived from the yield estimator selected in step 3 (gamma; λ) multiplied by an estimate of biomass from a stock survey, intended to represent the unexploited biomass (B0), viz: PCL = λβ0.
According to Constable et al. (2000): the first rule is intended to protect the krill stock itself (the ‘recruitment’ criterion of Article II 3(a)); the second rule is intended to protect natural predators (the ‘predator’ criterion of Article II 3(b)); whilst the length of time over which the risk is evaluated (20 years) relates to Article II 3(c) (Box 1). The third rule selects the most precautionary option between rules one and two.
Since the decision rules were first negotiated, scientific information about both the krill stock and about krill-dependent predators has been increasing, given more than four decades of ongoing scientific research (e.g., Meyer et al., 2020; Bestley et al., 2020). In particular, with respect to the first rule, it has now been well-established that krill abundance varies intra- (e.g., Reid et al., 2010), and inter-annually (e.g., Reiss et al., 2008; Skaret et al., 2023; Fielding et al., 2014; Trathan et al., 2022a). Indeed, natural temporal variability in biomass may mean that stock levels do naturally fall below 20% of the pre-exploitation biomass with a probability greater than 10% even in the absence of fishing (SC-CAMLR-XXIX, 2010 [Annex 6, paragraph 2.78]). In years with naturally low krill abundance, it would be precautionary to restrict, or even close, the krill fishery (e.g., South Georgia in 1999 and 2009; Trathan et al., 2021). However, without appropriately timed stock surveys, CCAMLR has not been able to do this. Consequently, with the added impact of climate change on recruitment variability, the current decision rule relating to the maintenance of stable recruitment should be re-visited (CCAMLR, 2023a).
With respect to the second rule, CCAMLR allows an escapement of 75% of the available krill biomass for natural predators. This level has been chosen as the mid-point between two differing management approaches (see Constable et al., 2000). Firstly, taking no account of predator needs (50% escapement; that is, treating krill as a single-species fishery, discounting the ecosystem approach; but see Pauly and Froese, 2021), or secondly, providing complete protection for predators (100% escapement; that is, no krill fishery). However, the ecological complexities of rule two have not been evaluated in detail, including whether different predators might influence the level of krill consumed by other species (i.e., indirect predator-predator interactions), or even alter the overall demand for krill. Resolving such complexities depends upon insights into various predator-prey (and predator-predator) relationships. It also requires understanding about trophic dependencies, which CCAMLR must grasp to fully implement an ecosystem approach to fisheries management.
Currently, CCAMLR has not explicitly defined how it will facilitate the recovery of previously depleted species and stocks (including populations of predators), or how much krill should be available so that such populations can recover. Importantly, the 75% escapement under rule two is the only means of facilitating the recovery of depleted stocks, including krill predators. Consequently, understanding how total natural consumption may change in the future as more populations of baleen whales recover, suggests that CCAMLR should now consider how to implement a precautionary strategy that allows for baleen whale recovery.
To achieve this, CCAMLR should now determine if the second decision rule is adequate, and that 75% escapement is adequate to facilitate the recovery of baleen whale populations at rates which ensure their stable recruitment. However, CCAMLR will still need to understand how predator-prey relationships for one predator taxa might influence those for other predator taxa. Indeed, such information will be vital for identifying whether the fishery does lead to any changes in species abundance, or whether any such observed changes might be a result of predator-predator interactions, or a combination of interactions.
The decision rules were agreed following considerable negotiation within CCAMLR, yet they have never been tested, as krill catches have always remained small in relation to the estimated available biomass. Now, with krill consumption by baleen whales increasing, at the same time that commercial krill catches are also increasing, and with pressure to increase catches even further, the decision rules should be re-evaluated to ensure that they are fit for purpose. This requires that any intrinsic assumptions are grounded in science. However, there is little documentation available about the decision rules and about: ecological assumptions inherent in the rules; possible ecological consequences should assumptions be invalid; or research being undertaken to validate relevant assumptions (Hofman, 2017; 2019). Given the central importance of these rules, CCAMLR should undertake a detailed evaluation as a matter of urgency.
Determining a revised set of decision rules is challenging and hampered by mismatches in the scales of relevant data (e.g., seasonal krill distribution and abundance and associated predator survival and reproductive performance), as well as a lack of causal understanding about confounding climatic and other ecosystem factors (see Sydeman et al., 2017). Nevertheless, some studies have shown that when prey biomass decreases below 15 to 18% of its maximum recorded value, predation by seabirds becomes an important pressure (Saraux et al., 2021). This suggests that a threshold of 18% should be considered a limit to secure stocks of forage fish, and a point below which extra cautious management may be required. Sydeman et al. (2017) offer a set of best practices for investigations of fisheries-seabird competition. However, most studies to date have primarily considered the needs of seabirds, and to our knowledge none have considered the spatial and temporal resource demands of baleen whales. Given the resurgence of baleen whales, a review is now overdue.
2.2 CCAMLR’s current krill fishery management approach and catch allocations
In the southwest Atlantic, CCAMLR’s current management approach for the krill fishery has relied upon two Conservation Measures (CM), CM 51-01 (CCAMLR, 2010) and CM 51-07 (CCAMLR, 2023b). Respectively, these identified the catch limit and the spatial distribution of that limit. CM 51-01 has no expiration date, and continues until CCAMLR agrees otherwise (CCAMLR, 2010). CM 51-07 expired at the end of the 2023/24 fishing season (CCAMLR, 2023b).
CCAMLR has agreed an annual precautionary catch limit of 5.61 Mt (million t) across the southwest Atlantic (Box 3), based upon the decision rules (Box 2) and an acoustic survey undertaken in 2000 which reported a standing stock of 60.3 Mt (Fielding et al., 2011). The survey was repeated in 2019 using slightly different acoustic techniques and different vessels, but the overall abundance of krill remained very similar, albeit with a differing spatial distribution (Krafft et al., 2021). Nevertheless, CCAMLR has never permitted catches up to this precautionary catch limit due to concerns about potential ecosystem impacts from fishing if catches aggregate at small spatial and temporal scales. Consequently, in the southwest Atlantic, CCAMLR has instigated an interim catch limit of 620,000 t (the so-called ‘trigger level’) to reduce the risk of ecosystem impacts from catch aggregation.
Box 3. Acoustic biomass of krill in the southwest Atlantic and current catch limits:
60.300 Mt—The acoustic biomass of krill in the southwest Atlantic (Fielding et al., 2011).
5.610 Mt—The precautionary catch limit for krill across FAO Subareas 48.1, 48.2, 48.3 and 48.4 based on the acoustic biomass and the CCAMLR decision rules (Box 2) is identified by CM 51-01 (CCAMLR, 2010). This is a theoretical sustainable catch limit, but has never been implemented.
0.620 Mt—The interim catch limit (the ‘trigger’ level) is identified by CM 51-01 (CCAMLR, 2010). The trigger level was calculated as the sum of the historical maximum catch in Subareas 48.1, 48.2, 48.3 and 48.4 prior to 1991 (SC-CAMLR-X, 1991; paragraph 3.106). Its relationship to the stock biomass or the precautionary catch limit is not defined. The trigger level is the effective catch limit until such time as CCAMLR has defined an allocation of the precautionary catch limit amongst smaller management units. CM 51-01 has no expiration date, and continues until CCAMLR agrees otherwise.
0.155 Mt—The historical maximum allocation of the ‘trigger’ level in FAO Subarea 48.1 identified by CM 51-07 (CCAMLR, 2023b). CM 51-07 expired at the end of the 2023/24 fishing season.
0.279 Mt—The historical maximum allocation of the ‘trigger’ level in FAO Subarea 48.2 identified by CM 51-07 (CCAMLR, 2023b). CM 51-07 expired at the end of the 2023/24 fishing season.
0.279 Mt—The historical maximum allocation of the ‘trigger’ level in FAO Subarea 48.3 identified by CM 51-07 (CCAMLR, 2023b). CM 51-07 expired at the end of the 2023/24 fishing season.
0.930 Mt—The historical maximum allocation of the ‘trigger’ level in FAO Subarea 48.4 identified by CM 51-07 (CCAMLR, 2023b). CM 51-07 expired at the end of the 2023/24 fishing season.
Currently, it is not operationally feasible to undertake large-scale acoustic surveys every year; however, annual small-scale surveys provide confidence about the continuing status of the krill stock (e.g. Reiss et al., 2008; Skaret et al., 2023; Fielding et al., 2014; Trathan et al., 2022a). Variability is known to exist in krill distribution and abundance, plausibly driven by physical forcing factors (Trathan and Murphy, 2003; Murphy et al., 2004; Trathan et al., 2022a). Approximately 87% of the circumpolar krill stock occurs offshore over waters >2000 m deep (Atkinson et al., 2008), although the fishery primarily operates over shelf and shelf slope areas (Figure 1) where krill density is much higher. Most catches are taken around the Antarctic Peninsula (Subarea 48.1), to the west of the South Orkney Islands (Subarea 48.2) and in waters adjacent to South Georgia (Subarea 48.3). Catches at the South Sandwich Islands (Subarea 48.4) have been negligible since CCAMLR was agreed in 1980 (Figure 1). Pressure to increase catches in Subarea 48.1 continues to drive the development of CCAMLR’s krill fishery management procedures. However, the expiration of CM 51-07 (CCAMLR, 2023b) might now lead to unintended consequences if increased catches repeatedly aggregate in space and time, particularly before a revised management framework is ready.
2.3 CCAMLR’s revised krill fisheries management framework
The spatial subdivision of the trigger level has served to limit catches whilst fishery demand has remained low. However, with demand increasing, a revised scientifically-based krill fishery management framework is being developed to facilitate catches up to the precautionary catch limit (Constable et al., 2023). Thus, instead of using the trigger level which was not founded on science (SC-CAMLR-X, 1991; paragraph 3.109), CCAMLR is moving towards use of the decision rules to set catch limits (Box 2), recognising that krill fishery management remains data-poor.
The revised framework is intended to specifically account for the needs of different krill-predators across both space and time. It has been under active development since 2019, including with a pilot analytical study focused on the Antarctic Peninsula (Trathan et al., 2018a; 2022b; Warwick-Evans et al., 2022a; 2022b; 2022c). The revised framework relies upon the development of three core elements (CCAMLR, 2019, paragraph 5.17 to 5.19):
i. A krill stock assessment to estimate precautionary harvest rates;
ii. Regular updates of krill biomass estimates, potentially at multiple scales; and,
iii. A risk assessment framework to inform the spatial allocation of krill catch.
This revised framework extends the existing precautionary approach through the addition of a risk assessment. With perfect knowledge, catches could be distributed to avoid areas of high ecosystem risk, equivalent to using an accurate dynamic ecosystem model for determining a spatially and temporally resolved fishing strategy (Constable et al., 2023). However, without perfect knowledge, the aim is to guide fishery catch distribution towards areas of lower ecosystem risk. Consequently, this revised framework is an improvement over the current approach in that it requires more detailed information about the needs of predators and their important feeding areas. Fully parameterised spatial and temporal consumption estimates of krill by whales and other krill predators are now key for CCAMLR moving forward.
In addition to the pilot study, CCAMLR has also recognised the need for enhanced ecosystem monitoring, as well as the need for spatial management at the Antarctic Peninsula (see Trathan, 2023a). Consequently, appropriate monitoring together with spatial management tools are also under active consideration so as to ensure that biodiversity is adequately maintained, especially given regional climate change.
Once fully tested, the analytical pilot study could be implemented and eventually rolled out for the whole of the southwest Atlantic, providing a science-based, precautionary krill fishery management framework. Field testing and implementation will be contingent upon reaching consensus within CCAMLR. If CCAMLR cannot reach agreement, or if the analytical pilot project fails for any reason, the existing highly precautionary management approach should remain in place.
3 Interactions between krill fisheries and baleen whales
The spatial and temporal distribution of krill is a critical matter for krill, krill predators and the krill fishery. Whilst most of the krill population exists over deep water, albeit at lower density (Atkinson et al., 2008), many predators (e.g., Weinstein et al., 2017; Friedlaender et al., 2021; Reisinger et al., 2022; Trathan et al., 2022b), and the fishery primarily focus on shelf and shelf slope areas (e.g., Trathan et al., 2021). Krill densities are known to be higher in these areas (Trathan et al., 2003; 2022a; Warwick-Evans et al., 2022a). Moreover, seasonal movements of krill associated with reproduction are also thought to occur over shelf and shelf slope areas (Siegel, 1988; Trathan et al., 1993; Espinasse et al., 2012), making these areas important for krill itself. Therefore, understanding the ecological processes that lead to krill aggregating over shelf areas (e.g., Trathan and Murphy, 2003; Murphy et al., 2004; Trathan et al., 2022a) will be critical for understanding ecological interactions between krill, krill predators and the krill fishery. Land-based predators are restricted in their foraging ambit during the breeding season. However, baleen whales may be able to exploit aggregations of krill further offshore over deeper water. Nevertheless, the most intense interactions are highly likely to occur where baleen whales, other krill predators, and the krill fishery aggregate over shelf areas. The close proximity of predators and fishing vessels is likely to lead to a variety of interactions, with both direct and indirect threats to predators (see also Supplementary Material, SM 1). Direct threats to whales include ship strike, by-catch, or entanglement, as well as pollution, including sound pollution which impacts whale acoustic communication. Important indirect threats include competition for local prey resources, or prey-field disturbance through altered krill swarm structure. Here we focus upon these indirect threats as these can lead to sub-lethal impacts on individuals that can have significant population-level consequences, including increased energetic costs, decreased body condition and reduced reproductive rates.
Baleen whale and fishery interactions are readily studied at the Antarctic Peninsula, where krill fishing vessels concentrate their effort in the Bransfield Strait (Santa Cruz et al., 2018; Reisinger et al., 2022). In this area, most krill catches (>99%) have been taken between March and June in recent years (CCAMLR, 2023a). When targeting a local area, fishing vessels tend to focus their efforts for 4 or 5 days, before moving elsewhere when catch rates drop (Trathan and Hill, 2016; SC-CAMLR-XXXV, 2016 Annex 6, paragraphs 2.215 to 2.221). Subsequently, Santa Cruz et al. (2018) showed that fishing hotspots last from 3 to 17 days, depending upon the number of vessels present and the spatial scale of a hotspot. Presumably fishing vessels preferentially operate in areas where krill are abundant, which they then target, until catch rates decline, either because the krill swarm is depleted, or the swarm structure is disturbed (see Hamner et al., 1983). Further work is needed to determine which is most likely.
The relatively small spatial scales at which fishing vessels operate (~10 to 20 km) are critical for krill predators, and it is at these scales that interactions are likely to be most intense. For krill predators, including baleen whales, the duration of feeding within a given area will depend upon local krill density (abundance and distribution of swarms), the rate of consumption, and how krill move in ocean currents (so-called ‘krill flux’) (Trathan et al., 2022b). An important concern therefore, must be to determine how predators, including whales, respond to changes in prey abundance and distribution at small scales, both in the presence and absence of fishing vessels. Land-based predators may be directly affected by resource competition with fisheries, whilst baleen whales may be able to relocate, although the energetic cost of missed foraging opportunities may be considerable (Czapanskiy et al., 2021). This highlights the need to better understand how predators locate prey at densities that are critical for effective foraging, including associated energetic costs.
Interactions at these small spatial scales highlight an important mismatch between CCAMLR’s current spatial management approach (Subarea scale; 100’s to 1000’s km) and the spatial scales of ecosystem operation (1’s to 10’s to 100’s km) (Murphy et al., 1988). Further, CCAMLR’s current management approach takes no account of the temporal or seasonal scales of ecosystem operation. However, interactions at relevant scales can be addressed through the revised management framework which can (theoretically) encapsulate any management scale. Currently, with available data, management should be feasible with spatial scales of 100’s to 250’s km, and two seasons, winter and summer. The revised framework should also better account for the habitat use of different taxa (e.g., fish, penguins, flying seabirds, pinnipeds and baleen whales). Nevertheless, though a major step forward, the revised management framework assumes the ecosystem is static, whereas dynamic management may be more suitable for pelagic systems (Hazen et al., 2018; Maxwell et al., 2015).
Characterising predator-prey-fishery interactions at ecologically meaningful scales requires further study, especially given the different feeding behaviours found amongst the broad guild of krill consumers. Energetically, baleen whales probably require aggregations of krill that may be quite similar to those targeted by krill trawlers. For example, baleen whale telemetry data have revealed that humpback whales (Megaptera novaeangliae) exhibit characteristic spatial scales of feeding of ~36.2 km (based on first passage time and the spatial scale at which an animal concentrates its effort; Fauchald and Tveraa, 2003), whilst minke whales (Balaenoptera bonaerensis) exhibit characteristic scales of ~19.7 km (Hutchinson, 2023). In contrast, the characteristic scale of krill fishing vessel operations, based on vessel automatic identification system data, is ~18.13 km (Hutchinson, 2023). Thus, it is plausible, though untested, that these spatial scales reflect aspects of the distribution of krill. If correct, this means that the feeding scales of baleen whales and the operational scales of fishing vessels will be similar, requiring that CCAMLR exercises cautious management in areas attractive to both whales and fishing vessels. Spatial scales for broader search behaviour are likely to be much larger. Depending upon the distribution and frequency of krill patches with a particular density, fisheries have the potential to deplete the local prey-field (Trathan and Hill, 2016; Santa Cruz et al., 2018), including those of baleen whales, leading to greater energetic costs. Such relationships will potentially have a seasonal component, given observed seasonal variation in krill swarm structure (Lascara et al., 1999).
The overlap in the spatial and temporal scales exhibited by whales and fishing vessels suggests that subdivision of any catch limit should not allow large catches to aggregate in areas preferred by whales (or other predators). The geographic scale of management units and associated catch is therefore an important determinant in avoiding ecosystem impacts from fishing.
3.1 Spatial overlap between krill fisheries and baleen whales
Despite signs of recovery of different baleen whale populations, distribution data remain relatively sparse, and for some whale species almost non-existent (Bamford et al., 2023), when compared with historical whale distribution data (derived from historical catches, Figures 2–6). Knowledge of krill distribution and consumption by whales at scales relevant to the fishery is now vital for estimates of overlap and competition (see Warwick-Evans et al., 2022b). Overlap is only likely to increase into the future.
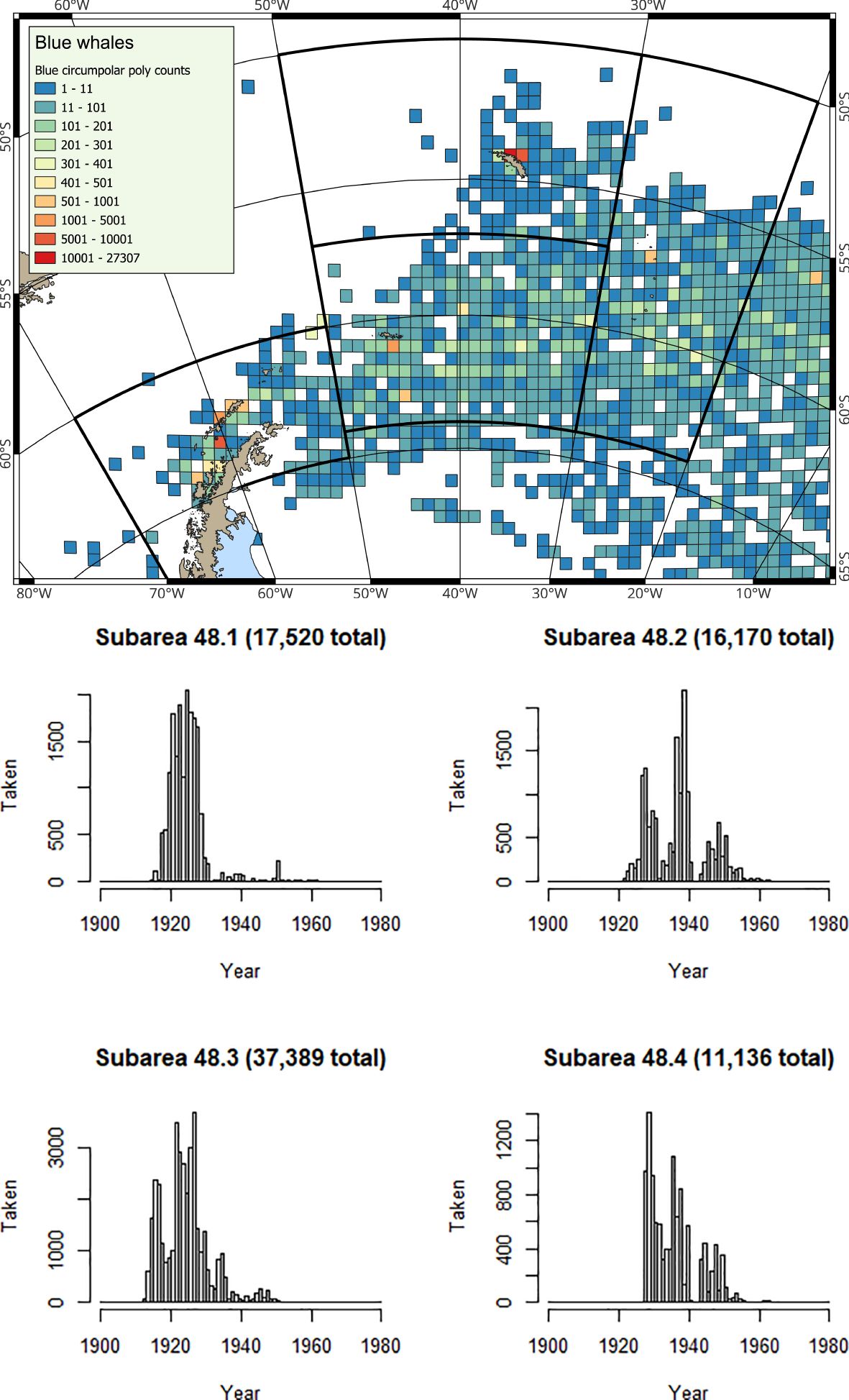
Figure 2. (Map) Spatial and (Histograms) Temporal distribution of historical catches for Antarctic blue whales from Allison (2016); note y-axes differ in the histograms.
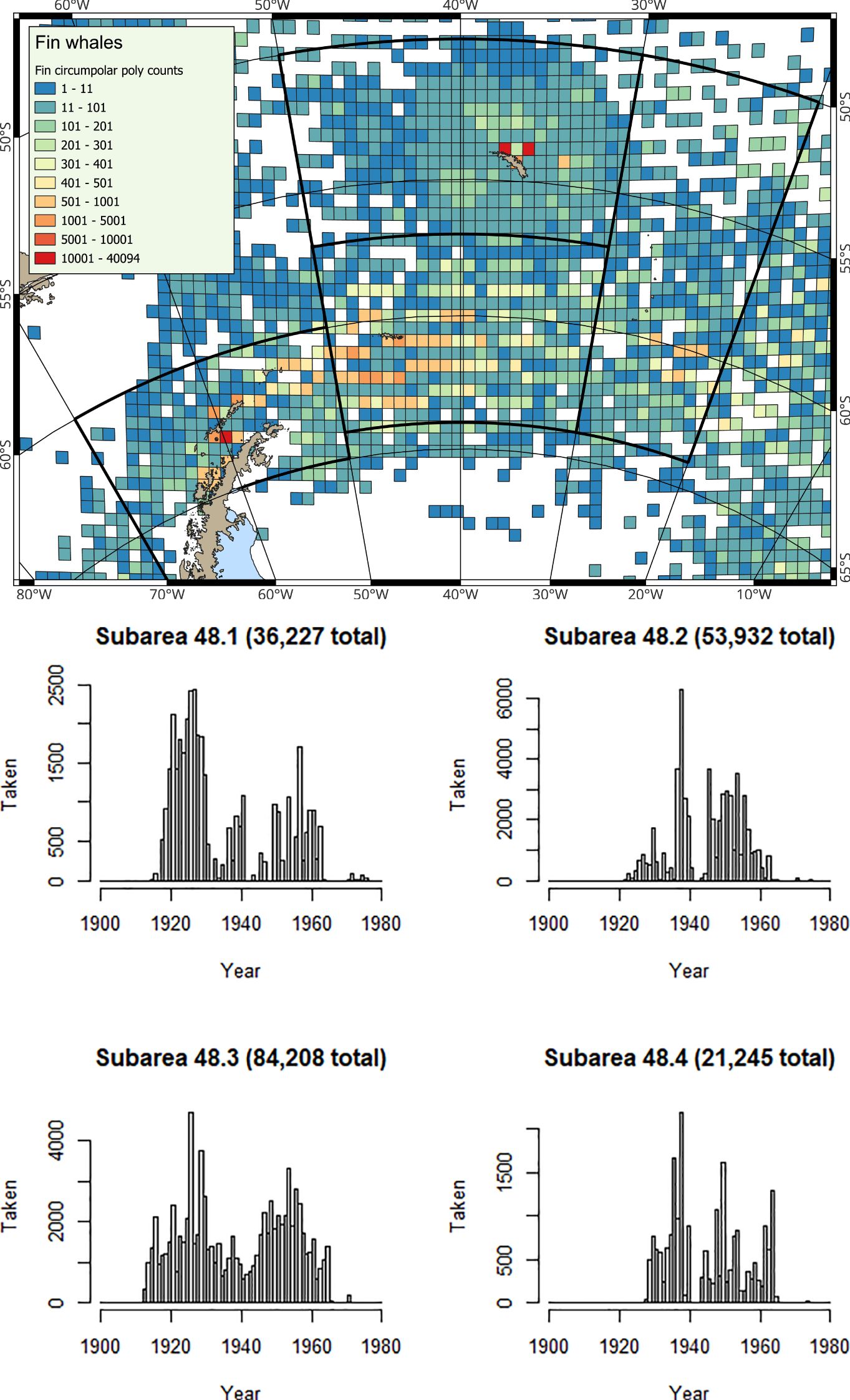
Figure 3. (Map) Spatial and (Histograms) Temporal distribution of historical catches for fin whales from Allison (2016); note y-axes differ in the histograms.
At the Antarctic Peninsula (Subarea 48.1), where krill catches have been restricted (Box 3) in most years since 2010, the most frequently observed baleen whale species is the humpback whale (Secchi et al., 2001; Nowacek et al., 2011; Friedlaender et al., 2021). The smaller, but much more abundant sea ice-associated Antarctic minke whale is also frequently observed (Friedlaender et al., 2014; 2021). Fin whales (Balaenoptera physalus) are also now regularly sighted in inshore waters (Warwick-Evans et al., 2022b), and occur in higher densities over deeper waters along the shelf edge (Herr et al., 2016; 2022). Fin whales have not yet returned en masse to ancestral feeding grounds in the Bransfield Strait, where overlap with krill fisheries would be extensive (Herr et al., 2022). However, they are showing strong signs of recovery, particularly in the eastern Bransfield Strait and around Elephant Island, but still with fewer numbers around the South Shetland Islands (Herr et al., 2022; Biuw et al., 2024).
At the South Orkney Islands (Subarea 48.2), the most frequently observed baleen whale species are fin whales (e.g., Viquerat et al., 2022; Ryan et al., 2023; Biuw et al., 2024), although humpback whales and southern right whales are occasionally sighted (Kennedy et al., 2024; Biuw et al., 2024). Considerable numbers of fin whales are now regularly seen in areas used by the krill fishery to the west of the archipelago, at the same time of year that the krill fishery operates (e.g., Viquerat and Herr, 2017; Viquerat et al., 2022; Ryan et al., 2023; Biuw et al., 2024).
At South Georgia (Subarea 48.3) and at the South Sandwich Islands (Subarea 48.4), the predominant baleen whale species observed is the humpback whale (Baines et al., 2021; 2022), although southern right whales are also regularly sighted (Leaper et al., 2006; Zerbini et al., 2018; Jackson et al., 2020; Calderan et al., 2023; Kennedy et al., 2024). Fin whales are now increasingly common (Biuw et al., 2024), whilst blue whales (Balaenoptera musculus) are only occasionally sighted (Kennedy et al., 2020), consistent with the situation that this species is still very much depleted. Whaling catch history data (e.g. Calderan et al., 2020) show that early blue whale catches were taken from the area now used by the krill fishery at South Georgia (Trathan et al., 2021), suggesting that as blue whales recover, it is highly likely that spatial overlap with blue whales will occur.
The rate of increase in baleen whale biomass in the southwest Atlantic is considerable; at the time of the CCAMLR 2000 krill stock assessment survey, the ratio between whale biomass and krill biomass was 1:131, by the time of the 2019 krill survey this had changed to 1:24 (Baines et al., 2022). In the future, as baleen whale populations continue to recover, it is highly likely that this ratio will further decrease. It is therefore apparent that the overlap between krill fisheries and baleen whales is already extensive, and is likely to expand as still-depleted whale species such as fin, sei (Balaenoptera borealis) and blue whales recover further.
Protecting food supplies for baleen whales is now vital. Warwick-Evans et al. (2022b) have reported that, amongst a guild of 17 krill predators feeding at the northwest Antarctic Peninsula, humpback and fin whales together account for ~73% of krill consumption. Indeed, consumption by all members of the guild is only approximately four times the trigger level (620,000 t; Box 3). Therefore, aggregation of catches up to the trigger level at the Peninsula could have unintended consequences. Importantly, whales (and other predators) forage in the region for several months, whereas the fishery reaches its catch within weeks, so information from dedicated overlap analyses (e.g., Reisinger et al., 2022) will now be important. In the future, relative consumption by different krill consumers will change, both spatially and temporally, in response to the ongoing recovery of baleen whales. Consequently, understanding fishery overlap at different times of year should now be a central focus for krill fishery management.
3.2 Ensuring adequate prey remain for predators
CCAMLR’s management principles mandate that sufficient krill biomass must be available for natural predators (see Box 1 and Box 2; Constable and de la Mare, 1996; Constable et al., 2000; also Constable, 2001). However, ensuring that adequate krill remains available, including at small spatial and temporal scales, requires agreement on the appropriate spatial and temporal scales of management and corresponding catch allocations.
The recent focus for krill fisheries management within CCAMLR has been to distribute the catch in space and time so that krill-dependent predators, such as penguins and seals, are not affected by catch aggregation within small areas (e.g., Trathan et al., 2022b). This is an important issue within CCAMLR, particularly for central place foragers, that is, predators that must return to land regularly to feed their offspring. Whilst provisioning young, penguins and seals can only travel relatively short distances, which means that catches in the vicinity of breeding sites must be carefully regulated. More recently, with the ongoing recovery of baleen whale populations, feeding areas important to whales are also becoming a concern. Most such areas are thought to be close to land, mainly over the shelf or at the shelf edge (e.g., Weinstein et al., 2017; Reisinger et al., 2022; Trathan et al., 2022b) in areas where the fisheries predominantly operate. However, additional work is needed to determine whether the identification of such areas may be a result of observational bias, given that offshore areas are hardly ever fished and are less frequently surveyed.
Developments in krill fishing technology have been important over the past two decades. The ‘traditional’ fishing method utilises trawling methods whereby the net is hauled on board as part of fishing operations. The ‘continuous’ fishing system was introduced into the krill fishery in 2004 (CCAMLR, 2023a), and utilises a system whereby the cod-end of the net is continually emptied via a pump connected to the vessel. Currently, both fishing methods are used, although most catches are now taken with the continuous fishing method. Traditional krill trawlers can harvest 100 to 400 t d-1, whilst modern continuous trawlers can harvest 800 to 1,000 t d-1 (Nicol et al., 2012). The use of continuous trawlers can lead to more concentrated catches, particularly in coastal areas where predator concentrations are at their greatest (Trathan et al., 2021; 2022b).
4 Estimates of baleen whale krill consumption rates
To comply with Article II (Box 1) and to parameterise the revised krill fishery management framework, CCAMLR requires up-to-date information about the changing status (including seasonal abundance and distribution) of both krill and krill-eating predator populations. This information is needed both regionally and locally for predator foraging areas and preferred fishing grounds. CCAMLR also needs information about seasonal rates of krill consumption by different predators (e.g., Johannessen et al., 2024). Warwick-Evans et al. (2022b) estimated both the absolute and relative importance of krill consumption by baleen whales, while also highlighting several aspects of uncertainty associated with their population status, growth rate, energetic requirements and krill consumption. Given that baleen whales are now the dominant air-breathing consumers of krill, better information is needed about their consumption rates, duration of feeding, and any dietary relationships with survival and reproductive output.
4.1 Different estimates of consumption
Historically, estimates of prey consumption for baleen whales were determined by consideration of energetic demands, often based on allometric models (e.g., Leaper and Lavigne, 2007). Given the uncertainty associated with these estimates, empirically based estimates of consumption are needed, especially as baleen whale abundance increases (Warwick-Evans et al., 2022b). The current pilot analyses for the CCAMLR revised management framework use data from Reilly et al. (2004), assuming 497 kg d-1 for humpback whales and 693 kg d-1 for fin whales, based on a 120-day summer feeding period. However, Savoca et al. (2021) provide revised estimates of prey consumption for Antarctic humpback and minke whales, using empirical tag-derived data on lunge-feeding rate, volume of engulfment and acoustic estimates of prey density. The results reported by Savoca et al. (2021) (i.e., 685 kg d-1 for minke whales, 3,151 kg d-1 for humpback whales), indicate that daily estimates of krill consumption may be around three to six times greater per species than previously thought. However, prey consumption varies, based on time of season and demographic class (Bierlich et al., 2022; Nichols et al., 2022); thus, a more nuanced view is needed. A critical gap remains in directly measuring feeding and consumption rates for Southern Ocean fin and blue whales.
Further, research is also needed to better understand whale-krill interactions. For example, information on prey-patch preferences (e.g., Friedlaender et al., 2016; Cade et al., 2022; 2023), as well as prey patch dynamics (including how prey patches form, how long they last, and how they dissipate). Lunge feeding may also be cheaper energetically than previously thought (Videsen et al., 2023). Suggesting that baleen whales may be flexible in the quality of the prey patches they exploit and therefore more resilient to environmental variability and perturbation. Thus, better understanding about baleen whale prey interactions, improved understanding about energy expenditure and about how baleen whales utilise the entire water column, in contrast to the vertical distribution of krill, and the distribution of fishing depths, is now urgent, if these interactions are to be properly understood.
Populations of fin and blue whales have not yet fully recovered, or reoccupied all of their historical feeding grounds (Calderan et al., 2020; Herr et al., 2022; Biuw et al., 2024; Savoca et al., 2024). Consequently, continued recovery (Supplementary Material SM 2)is highly likely to place increasing pressure on krill stocks. Further work on krill consumption is therefore needed. Recent estimates of historical consumption are of such a scale that the current estimate of krill biomass (Krafft et al., 2021) could not support the pre-exploitation populations of baleen whales (Savoca et al., 2021; 2024). Even conservative estimates of consumption suggest that the historical krill stock in the southwest Atlantic must have been larger than currently reported (Savoca et al., 2024).
4.2 Duration of the feeding season
Estimates of both individual- and population-level annual prey consumption require assessment of basic information about the number of days spent feeding, and the seasonal phenology of feeding, details of which are only poorly understood. The current pilot analyses for the revised krill fishery management framework use historical estimates of 120 days for the duration of the feeding season, approximately December to April (Lockyer, 1972b). However, contemporary data suggest that some individuals may remain on the feeding grounds for at least five to six months (e.g., humpback whales, Bedriñana-Romano et al., 2022). Improved population-level information is therefore required, including the actual number of days feeding, as opposed to the number of days present on the feeding grounds. Estimates for the number of feeding days vary, ranging from 90 to 120 days (Lockyer, 1972b; 1981; Leaper and Lavigne, 2007).
Understanding differences in feeding rates throughout the feeding season are also important (Johannessen et al., 2024), as hyperphagia may only be present immediately after whales return to the feeding grounds in spring (see Baines et al., 2022; Nichols et al., 2022). However, a continuous increase in body condition throughout the feeding season (Bierlich et al., 2022) implies that whales continue to grow despite decreased feeding rates, something potentially explained by changes in krill patch density. Generally, krill exist within different aggregation states, but with the numbers and sizes of swarms in an area changing across space and time. Smaller, more widely dispersed swarms occur in summer, whilst larger, more infrequent swarms occur in winter, with the patterns and seasonal changes unlikely to be solely the consequence of oceanographic circulation (Lascara et al., 1999). Thus, feeding rates may also be a consequence of prey aggregation state and swarm distribution. In addition to better seasonal information about feeding (Johannessen et al., 2022), improved understanding about diel feeding patterns (e.g., Friedlaender et al., 2013), particularly in relation to krill vertical diel migration is also required. Importantly, the fishery has recently shifted towards the autumn (Trathan et al., 2022b), coincident with the time when larger, more-dense krill swarms are present (Lascara et al., 1999).
Most individual baleen whales leave their feeding grounds in autumn and winter. However, based on modelled tracking data (e.g., Bamford et al., 2022; Bedriñana-Romano et al., 2022), passive acoustic data (e.g. Širović et al., 2004; Širović and Hildebrand, 2011; Thomisch et al., 2016; Filun et al., 2020) and visual observation data (e.g., Kennedy et al., 2020; Owen et al., 2024), whales are present on their feeding grounds throughout the annual cycle (e.g., Smith et al., 2024), albeit at lower numbers in the winter. Individuals that winter on the feeding grounds are most likely not reproductively active (i.e., immature or resting adult females). At least for humpback whales, given the male positive sex bias reported from breeding grounds (Clapham, 1996), those that remain on the feeding grounds may be predominantly females taking a breeding hiatus. Several species (e.g., blue, fin, southern right) have a calving interval of 2 to 3 years (Taylor et al., 2007), although this varies with biological and ecological conditions. Sub-adult whales not yet sufficiently physically mature enough to breed may be present in winter. These individuals may represent important demographic components of the population that require special consideration. Determining feeding rates in winter, compared with the summer, together with identifying the proportion of the population on the feeding grounds in winter, will be important for assessments of fisheries overlap and estimates of seasonal krill consumption.
4.3 Krill density and baleen whale reproductive output
Baleen whales are capital breeders (Stephens et al., 2014), thus, even marginal reductions in krill availability can impact on their near-future reproductive rates. Therefore, to fulfil its obligation under Article II (Box 1), CCAMLR needs to better understand baleen whale recovery, including modelling efforts to improve estimates of baleen whale pre-exploitation biomass. Various estimates are available (Christensen, 2006; Tulloch et al., 2018; Zerbini et al., 2019), but not at the scale necessary for comparison with CCAMLR krill fisheries. Part of this analysis requires information about whale reproductive output relative to krill availability.
Krill density (food availability) affects whale (and other predators) nutritional condition (e.g., Fortune et al., 2013; Bengtson-Nash et al., 2018) and therefore reproductive success (e.g., Cooke et al., 2003; Leaper et al., 2006; Seyboth et al., 2016; 2021). For males, food availability probably affects age of first sexual maturation, sperm production and quality, and their copulation success (Reeves et al., 2001). For females, nutritional condition probably affects age of first sexual maturation, ovulation and fertility, quality and quantity of milk production and hence calf survival, and potentially calving interval either through time between pregnancies or late-term abortions (Leaper et al., 2006; Pallin et al., 2023).
Nutritional status and social interactions may combine to modify reproductive success. Examples include changes in the age of attainment of sexual maturity in fin whales, as determined by ear plug transition layers (Lockyer, 1972a). Pregnancy rates may also change, tending to increase, as population size declines (Lockyer, 1984). More recently, Seyboth et al. (2016; 2021) demonstrated that southern right whales and humpback whales exhibit a close relationship between krill availability and calving rate. Further, inter-annual variability in humpback whale pregnancy rates (determined from skin-blubber biopsy samples) in the western Antarctic Peninsula, show positive correlations with krill availability and fluctuations in sea ice cover in the previous year (Pallin et al., 2023). This suggests that humpback whales may now be at a threshold for population growth due to prey limitations (Pallin et al., 2023). Consequently, increased fishery catches within the feeding grounds of humpback whales in Subarea 48.1 might now have unintended consequences for their population status.
5 Population status of different baleen whale species
Baleen whale population size is a key factor in determining total krill consumption (Savoca et al., 2021; 2024). Most historical whale populations (when catches were taken across the whole southwest Atlantic; Figures 2–6) were much larger than observed today. The pilot analyses for the revised krill fisheries management framework in Subarea 48.1 only include krill consumption estimates for humpback whales and fin whales (Warwick-Evans et al., 2022b). Revisions in the future should include all of the baleen whale species listed in Table 1 (see also Supplementary Material, SM 2). Revisions should include updated estimates of both abundance and krill consumption. Plausibly, other predators should also be re-evaluated, should their population status also change.
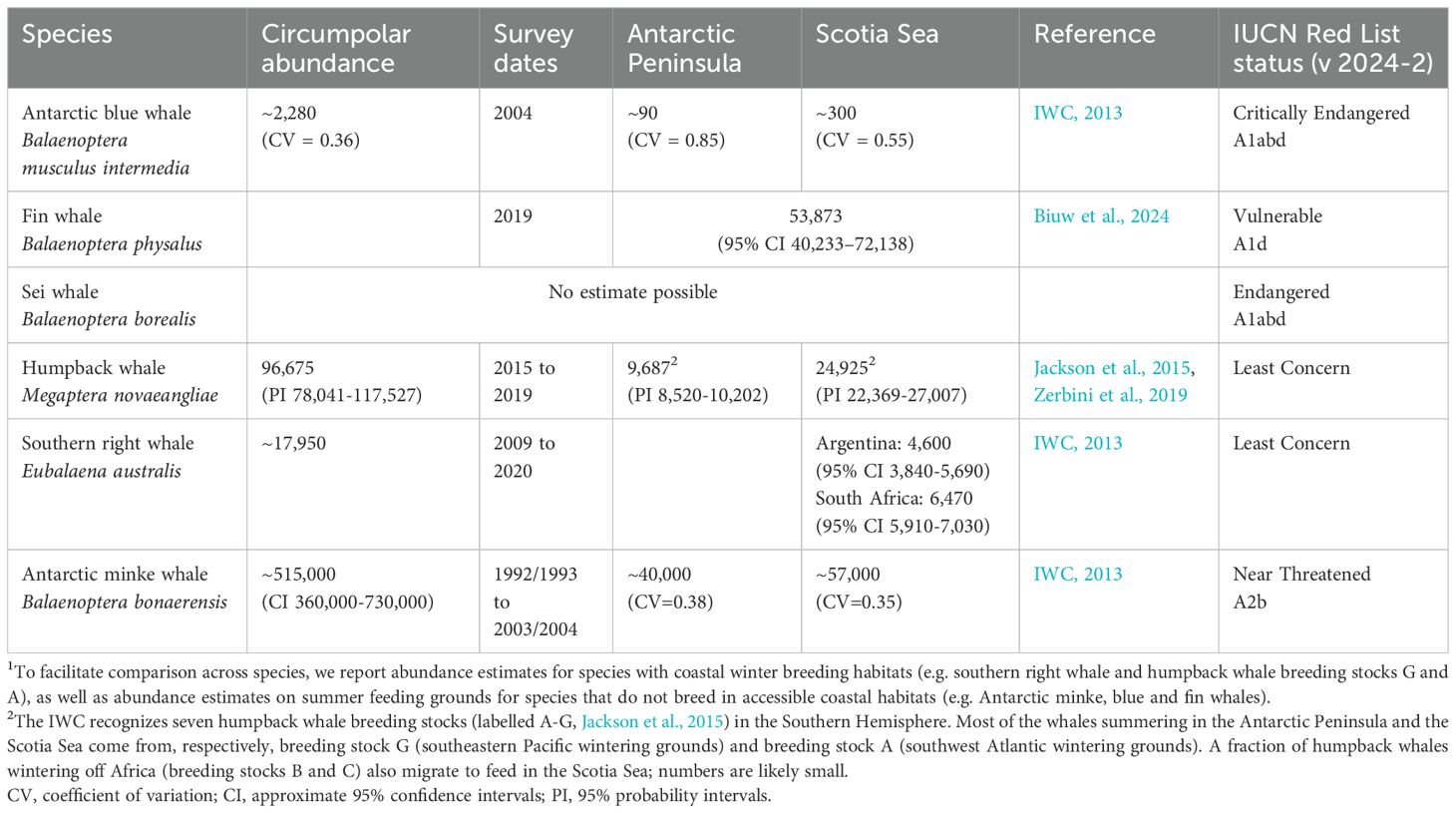
Table 1. Estimated abundance1 for various krill-eating baleen whales that have feeding grounds at the Antarctic Peninsula and Scotia Sea.
Many ecological interactions relevant to CCAMLR’s ecosystem approach to fisheries management are not well understood. In particular, those between baleen whales, their preferred habitats and their prey, or between whales and other krill-dependent predator species, or even between different whale species. Some interactions are little studied, for example, how whale habitat use and prey preferences vary intra- and inter-annually, or how these might vary as other species of whale recover. Plausibly, the distribution and abundance of baleen whales observed today will change as populations continue to recover. As populations increase, density dependent effects (e.g., Williams et al., 2013; Crespo et al., 2019) and inter-specific competitive effects (García-Vernet et al., 2021) may emerge. The distributions of some species may shift to more marginal habitats or alternative prey species, whilst others that are strong competitors will dominate. Mechanisms behind density dependence effects on population growth rate in baleen whales need to be explored (Kanaji et al., 2024). In theory, baleen whale population growth is maximal when there is sufficient food, but food availability is variable, and depletion below some threshold may impact calving, with the potential to lead to population declines (Kanaji et al., 2024).
6 Knowledge gaps in krill fishery management
6.1 Baleen whale population ecology
CCAMLR’s ecological understanding about baleen whale populations has been slow to be revised. Possibly because CCAMLR was agreed in 1980, at a time when baleen whale populations were severely depleted. Consequently, though whales were included in CCAMLR’s ecological considerations, they were only included generically as ‘krill-dependent populations’. More explicitly, CCAMLR’s existing ecosystem monitoring program (CEMP; Box 4) does not consider baleen whales (CCAMLR, 2014). Minke whales were originally included, but dropped in 1991 because no suggestions had been forthcoming about how monitoring should take place (Agnew, 1997). Now, CEMP has developed an extensive database documenting land-based predators, mainly penguins, but has no details about pelagic predators. Long-term data concerning baleen whales exist outside CCAMLR, and are certain to be highly informative. For example, a biopsy database for humpback whales with 15 years of consecutive data exists, a resource vital for comparing pregnancy rates with sea ice metrics and various krill indices (e.g., Pallin et al., 2023). Other information gaps include:
a. Estimation of baleen whale abundance is necessary at scales relevant to krill fishery management. This requires information on the spatial density and distribution of whale populations over relevant temporal scales. Key is the statistical power needed to detect baleen whale population trends; this not only requires a long time series but also depends upon the precision of population estimates. Dedicated surveys and mark-recapture efforts covering the area of interest are therefore needed. Related to this, is the precision needed for distinguishing local changes in abundance from broader scale changes in distribution. Thus, it may be more important to determine changes in the patterns of whale distribution than it is to determine changes in overall population size. For example, despite indications of continual population growth on the breeding grounds of humpback whales (breeding stock A) (Zerbini et al., 2019), changes in abundance on the feeding grounds at South Georgia have been relatively recent (Baines et al., 2021; 2022). Understanding such changes, and any associated lags, requires continued monitoring, probably over large areas.
b. Seasonal movements for some species of baleen whales are poorly understood, including nearly no movement data for key species such as fin and blue whales.
c. Baleen whale krill consumption rates are variable depending upon the time within the feeding season, although there is a lack of data, let alone seasonal data (e.g., Baines et al., 2022) for many species. Linked to consumption is the need to improve understanding about baleen whale prey searching behaviour, the types of swarms preferred, and the duration of feeding on a given swarm.
d. As populations recover, there are likely to be interactions between different species of baleen whales and between whales and other krill predators. Understanding how different whale species may affect other species is especially important, given the environment’s carrying capacity and how it may be changing. For instance, the ongoing recovery of fin whales may have an impact on humpback whale distribution and abundance, at least at a scale relevant to krill management. As populations recover, other krill-dependent species may also be affected. Changes in the abundance and distribution of other ecosystem components, such as krill-eating penguins, may be related to marine mammal recovery (e.g., Laws, 1977; Ballance et al., 2006; Trathan et al., 2012).
6.2 Movement of krill in oceanographic currents to feeding grounds and fishing grounds
The relationship between krill standing stock, predator consumption, and commercial harvesting highlights the importance of CCAMLR’s ecosystem approach to management. Important aspects include the seasonal, dynamic nature of the Antarctic marine ecosystem (e.g., Lascara et al., 1999) and spatial aspects of krill population dynamics (Perry et al., 2019). Reliable metrics of predator-prey-fishery overlap are vital if CCAMLR is to understand ecological risks associated with harvesting. Such metrics require knowledge about the residence time of krill, which in turn requires information about local and regional oceanography, krill movement in ocean currents and krill behaviour (Meyer et al., 2020; Trathan et al., 2022a; Young et al., 2024). To date, such information is not readily available, and is unlikely to be available at scales relevant to either fishery operation or predator habitat use (e.g., spatial scales of 10s to 100s of km, and temporal scales of days to months). Such complexity previously led CCAMLR to focus upon a large-scale static realisation of the ecosystem, instead of a small-scale dynamic assessment.
A static assessment might be precautionary as it does not account for continual replenishment of krill in ocean currents for areas where krill are depleted by natural consumption or harvesting. However, a static assessment may not be precautionary if catches regularly aggregate at high levels within small areas important to predators. Large-scale assessments of biomass (e.g., Fielding et al., 2011; Krafft et al., 2021) assume that the consequences of krill movement are minimal at the scale of the survey (e.g., 100s to 1000s km). However, at smaller scales of ecosystem operation (10s to 100s of km), the movement and replenishment of krill is likely to be a major issue (Trathan et al., 2022b). Disturbance or depletion of krill aggregations by fishing vessels over a few days (Trathan and Hill, 2016; Santa Cruz et al., 2018) may have important implications.
6.3 Alternative futures – the enhanced productivity hypothesis, or the krill surplus hypothesis
With the recovery of baleen whales, ecosystems may change, possibly dramatically. However, it is uncertain how they will change. Two possible scenarios are plausible.
Firstly, Smetacek (2008) and Nicol et al. (2010) hypothesised that pre-exploitation populations of baleen whales and krill stored larger amounts of available iron than is the case today. These species would also have recycled substantial amounts throughout the surface waters, thereby enhancing overall ocean productivity. This hypothesis reiterates iron as the limiting micronutrient in the Southern Ocean (Martin et al., 1990), and defecation by baleen whales as a major mechanism for recycling and making iron available. Consequently, as baleen whales recover, Southern Ocean productivity might increase overall. Recently, Gilbert et al. (2023) showed that released nutrient cocktails vary geographically, driven by the composition of cetacean communities. Thus, the ecosystem function and species diversity of cetacean communities expands beyond their role as predators to include their role as active nutrient vectors.
Secondly, Sladen (1964) and Laws (1977), hypothesised that the removal of the great whales should have resulted in an enormous annual release of prey and that substantial changes in the marine ecosystem would have then resulted in major ecosystem responses. Consequently, the return of the great whales suggests that the marine ecosystem across the southwest Atlantic might now change fundamentally, with some species declining as baleen whales recover (Murphy, 1995).
Determining whether either hypothesis is correct is challenging. Nonetheless, managers should appreciate and understand that a major ecosystem change is likely, and that management of harvesting should remain precautionary (Trathan, 2023b). CCAMLR might therefore consider alternative scenarios and whether particular management approaches are robust to different future states. It is probable that the current biomass of krill is insufficient to support baleen whale populations at historical levels (Savoca et al., 2021; 2024), supporting reports of a historical decline in krill biomass (Atkinson et al., 2019). The recovery of baleen whales and their nutrient recycling services (Roman and McCarthy, 2010; Lavery et al., 2014; Doughty et al., 2016) could augment productivity and restore ecosystem function lost during 20th century whaling (Estes et al., 2016).
6.4 The impact of climate change on krill and the krill-based ecosystem
The southwest Atlantic has been the region with the most pronounced warming in the Southern Ocean (e.g., Vaughan et al., 2003; Whitehouse et al., 2008; Stammerjohn et al., 2008; Turner et al., 2022). The interplay of altered physical properties, including fast ice (e.g., Fraser et al., 2021), sea ice (e.g., Turner et al., 2022), ocean temperatures (e.g., Whitehouse et al., 2008), wind speed, and ocean freshening (Hobbs et al., 2016), have the potential to alter ocean ecosystems. Changes may occur in ecological niches, species abundance and distribution, with plausibly new trophic interactions. For example, climate change may cause alteration to krill distribution and abundance (e.g., Flores et al., 2012; Atkinson et al., 2019; Veytia et al., 2020) and impact krill larval development (Kawaguchi et al., 2013). This could have important consequences for both krill predators and the fishery. In the context of a warming and acidifying ocean, particular concerns arise regarding baleen whales, given that they are capital breeders that rely upon the accumulation of resources during a short period of intensive feeding. Baleen whales are likely to be susceptible to regional warming (Tulloch et al., 2019; Bestley et al., 2020).
Atmospheric drivers, such as El Niño, have already been shown to influence population processes of some species (e.g., Leaper et al., 2006; Schall et al., 2021). Whilst the 2014-2016 marine heatwave across the northern Pacific probably led to a 20% decline in humpback populations in that region (Cheeseman et al., 2024).
Further, the ecosystem responses to climate change and historical exploitation may act in concert to alter ecosystem dynamics. Disentangling these confounding drivers may be difficult and complex (Trathan and Reid, 2009).
7 The immediate next important steps in krill fishery management
CCAMLR has resolved to utilise the best available science (CCAMLR, 2009 [Resolution 31/XXVIII]) in formulating its management decisions. However, given the enormous geographic extent of the Southern Ocean, many areas remain data-poor (e.g., Trathan, 2023a). Consequently, CCAMLR maintains a precautionary approach.
With the recovery of baleen whale populations, CCAMLR should evaluate threats to baleen whales. Currently, these main threats include, inter alia, death (e.g., from whale ship strike, by-catch, or entanglement), physiological impacts (e.g., pollution, whether chemical, marine debris including plastics, or noise), and energetic impacts (e.g., competitive effects for prey, with possible associated disturbance to the prey-field aggregation state). Mitigating each of these requires different management actions. Those threats that should be most straightforward to manage are those that result from physical interactions, or pollution (Table 2), see also Supplementary Material, SM 1. Threats that may lead to energetic impacts are potentially more challenging to quantify and to mitigate (Table 2). Nevertheless, these are vital to address, given the ecological foundation underpinning CCAMLR’s revised management framework (CCAMLR, 2019, paragraph 5.17 to 5.19).
7.1 Consideration of the krill fishery sustainable yield
Article II (Box 1) has been interpreted by some as a licence to fish. However, Article II actually specifies the conditions under which fishing is allowed, and these are highly restrictive. One major constraint is the maintenance of ecological relationships, including those of previously depleted populations. Land-based predators are highly constrained when breeding and therefore subject to variation in local krill availability. In contrast, predators with an ideal-free distribution (Kennedy and Gray, 1993) have the potential to adjust foraging location. However, if baleen whales also have the potential to outcompete various other krill-dependent species (see Murphy, 1995; Ballance et al., 2006; Trathan et al., 2012), then baleen whales become critical to any assessment of ecosystem status. The current increase in humpback whales (Jackson et al., 2015), and decrease in chinstrap penguins (Strycker et al., 2020), highlights the need for broader ecological understanding (see Ballance et al., 2006).
As CCAMLR moves towards a science-based ecosystem approach for krill fishery management, the decision rules need to be fully grounded in evidence. Of particular importance will be to challenge the decision rules at different spatial and temporal scales. Decision rules that are appropriate at large scales may be inadequate at smaller scales, or vice versa. The existing decision rules are currently specified without any ecological or geographic context, but must operate across species and across different spatial and temporal scales.
Any revision of decision rules might include more direct consideration of baleen whales, given they have the potential to outcompete other species such as penguins (see Murphy, 1995). For example, it may be important to reference their population status, reproductive success, or other more immediate proxies, such as pregnancy rate (Pallin et al., 2023). Data on such indicators remain sparse (Cury et al., 2011; Saraux et al., 2021), hence development of new decision rules will require concerted effort. A precautionary approach would preserve any ‘krill surplus’ for natural predators, restricting fishery expansion whilst baleen whale populations recover (Table 3 cf Table 4). Such an approach might eventually allow ‘enhanced productivity’ to be exploited, if ever available.
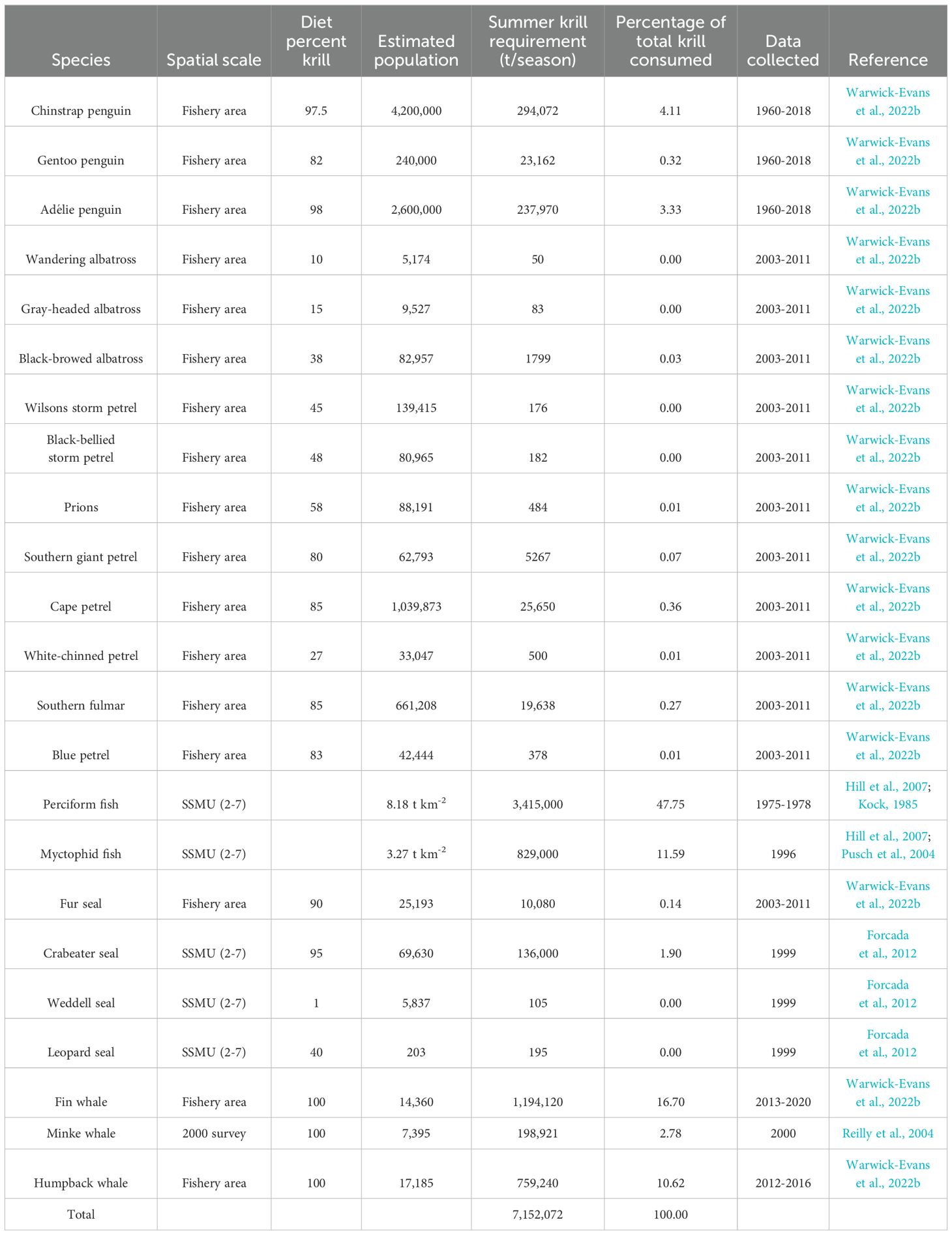
Table 3. Estimated consumption of krill by various predators within Subarea 48.1; see Figure 7 for spatial scale of analyses.
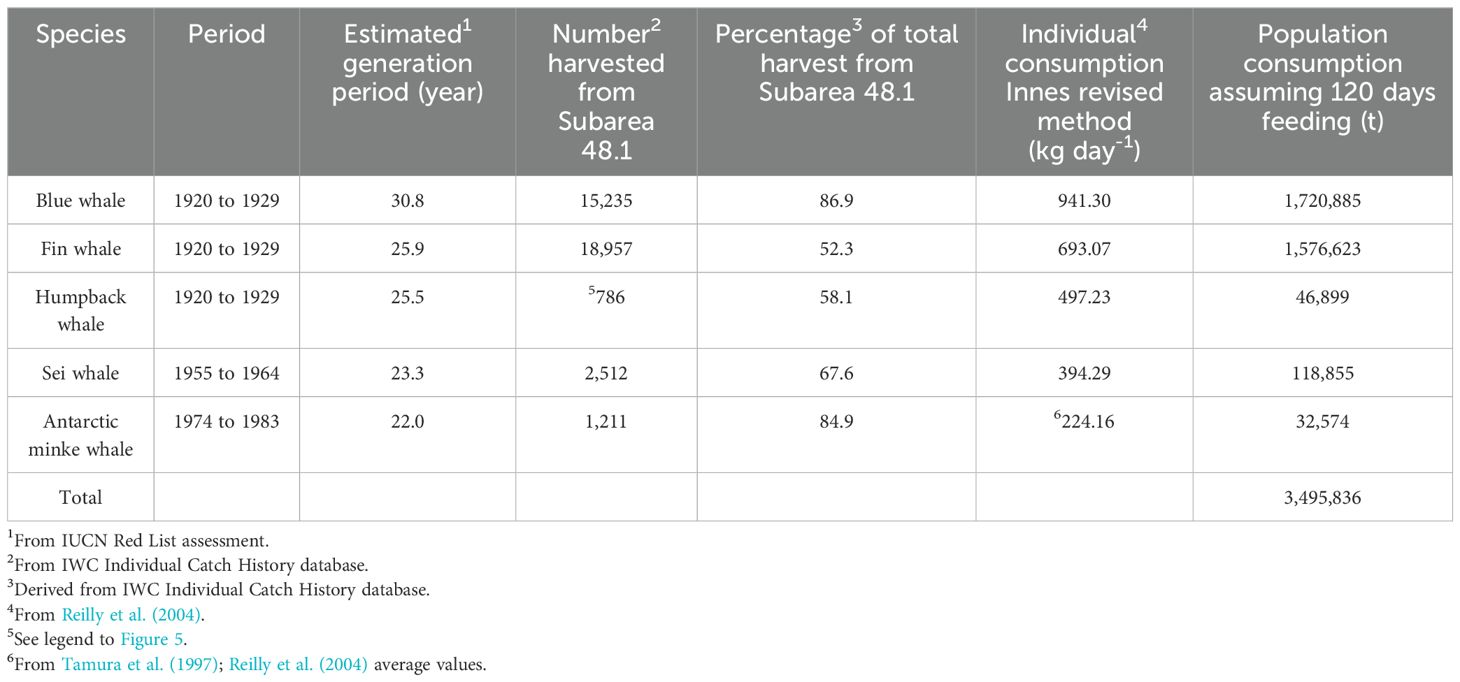
Table 4. Conservative estimates of historical krill consumption by five species of baleen whale within Subarea 48.1.
Currently, the decision rules assume a static albeit variable ecosystem, whereas in reality the Antarctic ecosystem is highly dynamic, both spatially and temporally, including with ongoing biological and physical changes. Managing fisheries in dynamic, sensitive ecosystems requires new approaches; as catches increase in relation to the available (local) biomass of krill, decision rules will become increasingly important. Hence, monitoring the effective exploitation rate in each management unit, especially in years of low krill biomass, will be fundamental for CCAMLR’s ecosystem approach to management (see Punt et al., 2023).
Key sources of uncertainty in ecosystem assessments include how prey abundance and availability impact predator demography (e.g., Koehn et al., 2021) and the extent to which the population dynamics of prey are driven by environmental factors (e.g., Licandeo et al., 2023). Data are available to address only some of these, necessitating exploration of sensitivity to alternative model formulations and parameter values when providing advice on management strategies to decision makers (Punt et al., 2016).
7.2 Revision of the krill fishery risk assessment layers
The revised krill fishery management framework includes a risk assessment which considers the spatial and temporal demands for krill by natural predators in order to distribute catches (Constable et al., 2023). The pilot analytical study for Subarea 48.1 includes the most up-to-date data for a broad guild of krill consumers, including three species of penguin, eleven species of flying seabird, Antarctic fur seals and two species of baleen whale (Warwick-Evans et al., 2022b). Krill consumption by fish (Hill et al., 2007) and pack-ice seals (Forcada et al., 2012), both important consumers, are also included, but data are only available at different scales of spatial resolution (Warwick-Evans et al., 2022c). Spatially explicit consumption estimates for these latter taxa are based on a set of Small-scale Management Units (Hewitt et al., 2004; Figure 7) which differ from the spatial framework under consideration by CCAMLR (Warwick-Evans et al., 2022c; Figure 7). Nevertheless, given the importance of these taxa as krill consumers (Table 3), their inclusion is essential. Indeed, it is now evident, despite the differences in spatial extent, that pelagic predators (fish, pack-ice seals and baleen whales) are the major consumers of krill, whereas CCAMLR has so far focused largely on land-based predators (penguins, fur seals and a limited number of flying seabirds).
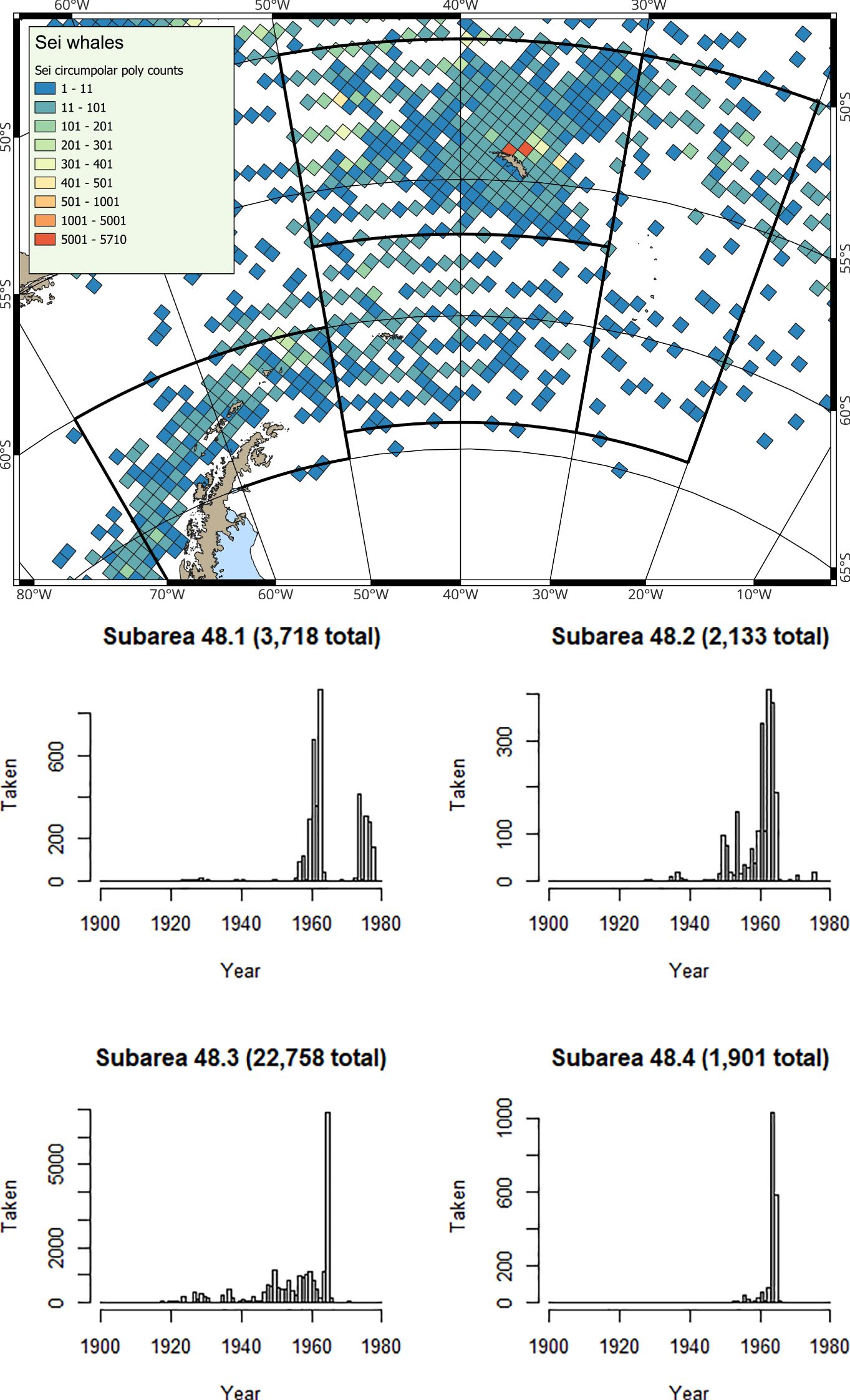
Figure 4. (Map) Spatial and (Histograms) Temporal distribution of historical catches for sei whales from Allison (2016); note y-axes differ in the histograms.
Importantly, many of the data used in the pilot analyses were derived from field survey data that were spatially limited, or are now many years old, in some cases many decades old (Table 3). For example, the spatial estimates of krill consumption by fish (Hill et al., 2007 and references therein) were based on limited data from the west Antarctic Peninsula. Biomass density and krill consumption estimates for myctophids were based on a limited study on the shelf slope near King George Island (Pusch et al., 2004), whilst estimates for perciform fish were based on data reported by Kock (1985).
Given the rate of ecosystem change, particularly levels of warming in the Antarctic Peninsula region and across the Scotia Sea (Vaughan et al., 2003; Meredith and King, 2005; Whitehouse et al., 2008), and the regional recovery of some baleen whale populations (e.g., Jackson et al., 2015; Zerbini et al., 2019; Biuw et al., 2024), it would be precautionary to update data for the risk assessment. This requires the initiation of new field data collection programmes to better establish the spatial and temporal distribution of krill consumption levels. If the krill risk assessment is not correctly parameterised, including with representative winter data for krill abundance and distribution (see criticisms by Trathan, 2023a), putative management units may inadvertently facilitate catch aggregation such that local exploitation rates increase to levels beyond expectation. In particular, the winter period remains data-poor; all existing survey data for krill, land-based predators, and pelagic predators are biased towards the summer. As such, the krill fishery risk assessment might result in poorly designed management units with inappropriate catch allocations.
7.3 Development of a revised CCAMLR ecosystem monitoring program
The krill fishery risk assessment requires ongoing data collection to revise and update estimates of krill distribution and abundance, and estimates of krill consumption by predators (Table 3). Such data collection should be integrated into CEMP. This would extend the utility of CEMP and integrate outputs directly into management, something that CCAMLR intended at the outset when formulating CEMP (Box 4), but has yet to fully achieve. As major krill consumers, fish (Everson et al., 1997), pack-ice seals (Hückstädt et al., 2020) (Table 3) and baleen whales (Table 1) should now be explicitly integrated into CEMP.
Box 4. CEMP was established with the following aims (Agnew, 1997):
i Detect and record significant changes in critical components of the marine ecosystem within the Convention Area, to serve as a basis for the conservation of Antarctic marine living resources.
ii Distinguish between changes due to harvesting of commercial species and changes due to environmental variability, both physical and biological.
To better inform CEMP (Box 4), CCAMLR needs to initiate a field programme to determine whether populations of some taxa will decline as others recover (see Sladen, 1964; Laws, 1977; Murphy, 1995), based on the ‘krill surplus’ hypothesis (Laws, 1977). Under this hypothesis, a reassortment of predator populations might occur, given the carrying capacity of the ecosystem. As CEMP currently relies upon penguin monitoring (CCAMLR, 2014), and because baleen whales have the potential to outcompete penguins (Murphy, 1995), it is now urgent to include baleen whale monitoring in CEMP. A revised CEMP could also monitor ecosystem productivity. Under the ‘enhanced productivity’ hypothesis (see Smetacek, 2008; Nicol et al., 2010), ocean production may increase leading to changes in ecosystem carrying capacity. Understanding such drivers will help inform any interpretation of CEMP data, particularly in the context of altered primary production (Montes-Hugo et al., 2009) and high levels of carbon sequestration (Cavan et al., 2024). A critical analysis will be to determine whether baleen whale populations continue to recover as krill catches rise towards the precautionary catch limit.
Exploitation of blue and fin whales in the early 20th century (e.g., in the 1920s; see Table 4; Figures 2, 3) probably had implications for other krill consumers (e.g., fish, seals and whales). Altered availability of krill may have contributed to changes in baleen whale age of maturity, generation time (Lockyer, 1972a; 1972b; 1984; Laws, 1977) and other vital rates. Plausibly, the abundance of baleen whale species targeted later in the 20th century (Table 4; Figures 4, 6) might also have increased, compared with their previous status in the unexploited ecosystem.
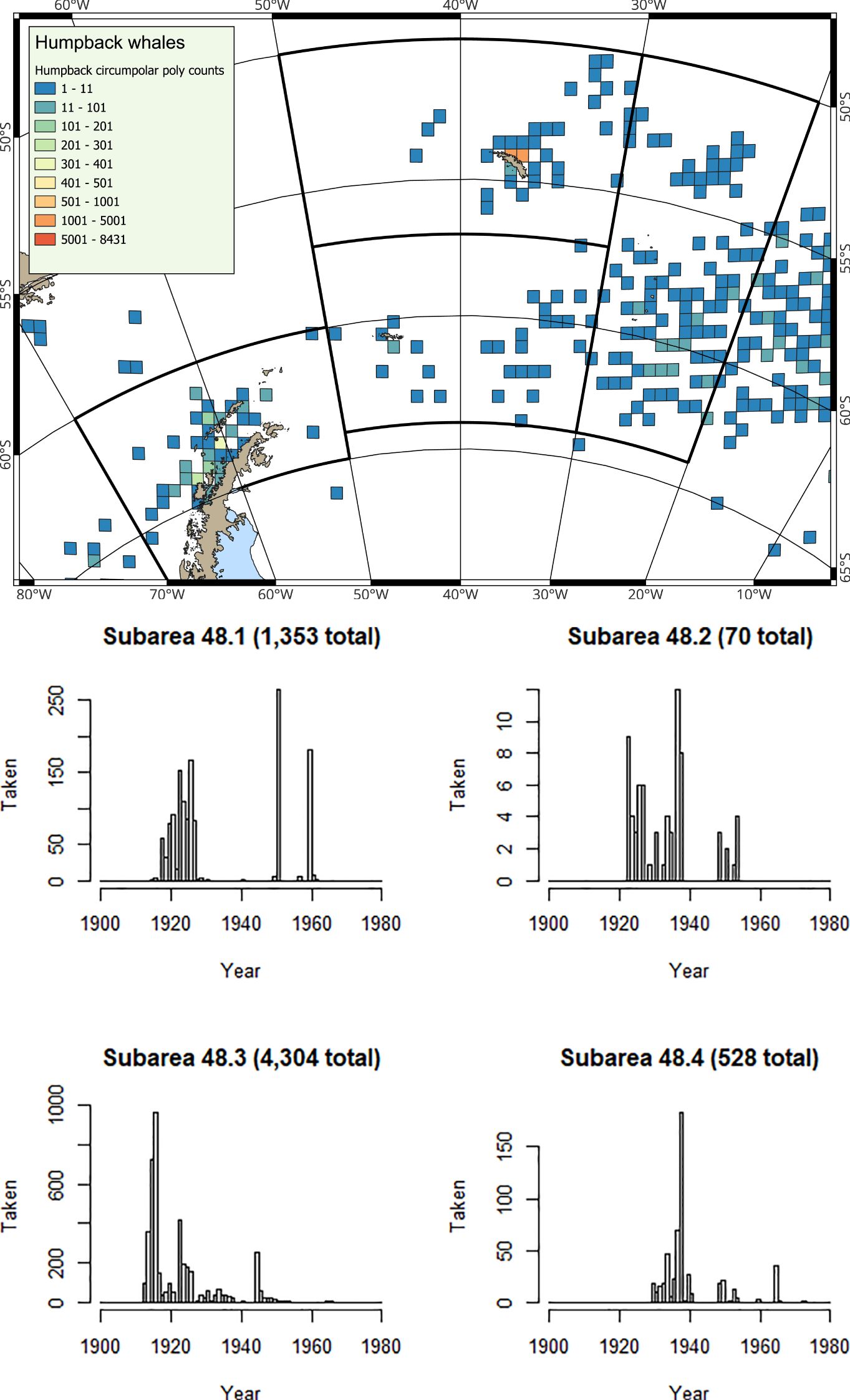
Figure 5. (Map) Spatial and (Histograms) Temporal distribution of historical catches for humpback whales from Allison (2016); note y-axes differ in the histograms. Additionally, a large number of humpback whales were caught between the early 1900s and the 1920s (e.g. about 11,000 near the Antarctic Peninsula and about 25,000 near South Georgia) that are not reflected here; these catches are only available in a summary form (and not in the IWC Individual Catch Database) as no precise information is available on the catch location or for any biological parameters of the whales taken.
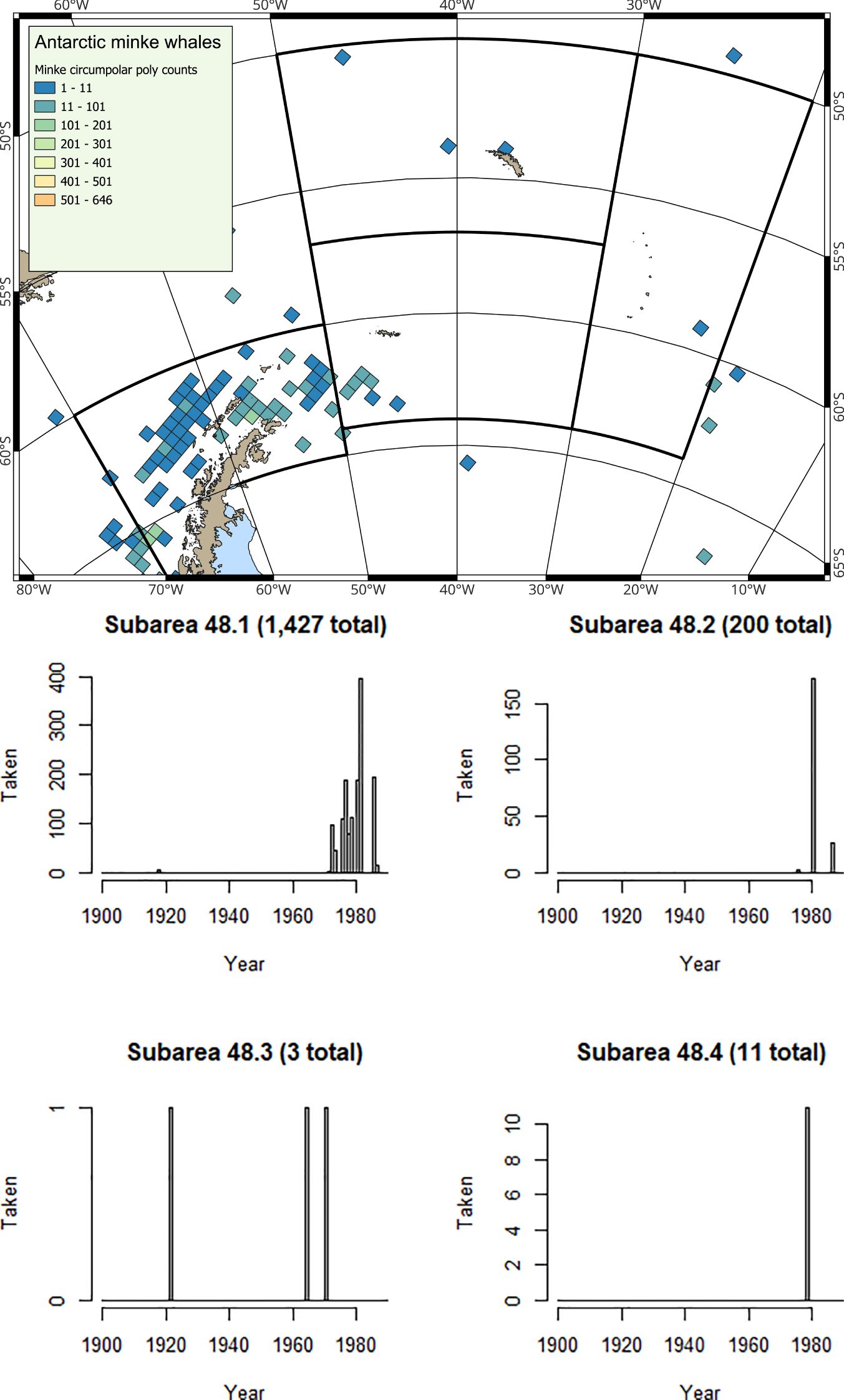
Figure 6. (Map) Spatial and (Histograms) Temporal distribution of historical catches for Antarctic minke whales from Allison (2016); note y-axes differ in the histograms.
Understanding the consequential serial impacts of whaling on the krill-based ecosystem is now difficult, but certainly some of those impacts may continue to operate. Whilst these consequences play out, krill fishery management should be cautious (Box 1) and will require adequate monitoring (Box 4). Management decisions should include a safety factor to allow for the facts that knowledge is limited and institutions are imperfect (Hofman, 2019). Critically, details of functional overlap with fisheries through studies on the (local) distribution and density of krill patches, and predator scales of feeding will now be important.
Monitoring baleen whale populations (with associated confidence intervals), requires dedicated sightings surveys. However, such surveys are expensive and require substantial funding. Nevertheless, large-scale scientific surveys that target both krill and whales (e.g., Fielding et al., 2011; Krafft et al., 2021) and which include areas of krill habitat, baleen whale feeding habitat, and preferred fishing grounds are now needed on a regular basis. Surveys should be frequent (e.g., every 5 to 10 years; see also Hofman, 2017), given the current rate of (physical and biological) ecosystem change in the southwest Atlantic. Eventually, CEMP should include standardised, circumpolar baleen whale metrics.
In the absence of dedicated surveys, other methods may be helpful (e.g., Hofman, 2017), albeit with associated higher levels of uncertainty. Where feasible, methods should include concurrent monitoring of whales and krill using any platforms of opportunity, including research ships, tourist vessels, and krill fishing boats. Opportunities to obtain data, including near-real-time data, on seasonal distribution and habitat use through passive acoustic and telemetry methods should be considered. Finally, ‘proxies’ that can be measured in real time to provide information about krill stocks and krill predators (e.g., progesterone to determine pregnancy rates and leptin to identify metabolic condition; Pallin et al., 2023) should be explored.
Surveys from tourist vessels now facilitate collection of information about temporal differences in the timing of when species arrive on the feeding grounds, and when shifts in distribution occur within the feeding season, aspects not generally captured by traditional research surveys (Henderson et al., 2023). However, opportunistic platforms may not cover all relevant areas and may create regional bias. Moreover, formal protocols would need to be agreed to ensure that observations meet acceptable scientific standards, sufficient for providing management advice.
Passive acoustics is now proving invaluable (Van Parijs et al., 2009), and a combination of visual and passive acoustic surveys can help determine whether a visual survey covered the main areas of whale distribution (Leaper et al., 2023). Photo-identification, where adequate images are available, can support mark-recapture studies and hence estimates of abundance (e.g., https://www.happywhale.com). Machine learning artificial intelligence photo-identification methods work well with humpback whales (Cheeseman et al., 2022), and are likely to work with Antarctic blue whales (Patton et al., 2023), but may be less suitable for large populations of fin whales.
Telemetry is an effective way to track large-scale movements and fine-scale habitat use of whales (Zerbini et al., 2006; 2018; Dalla Rosa et al., 2008; Bamford et al., 2022; Bedriñana-Romano et al., 2022), as well as their foraging ecology (Friedlaender et al., 2014; Savoca et al., 2021). Telemetry methods are particularly useful to monitor whale populations in remote areas such as the Southern Ocean, where visual observation is difficult or impractical.
Satellite surveys potentially represent an exciting development for high-resolution image-based cetacean observation at sea, particularly in inaccessible regions; however, adjustment for surface availability and weather conditions still require further effort (Bamford et al., 2020).
8 Conclusion
The marine ecosystem in the Southern Ocean is changing, highlighting the importance of ensuring that marine capture fisheries remain precautionary. Climate change, coupled with the recovery of baleen whales, means that CCAMLR is now facing several important challenges that require re-evaluation of previously accepted dogmas. Fundamental questions relate to: a) how to estimate precautionary yields (section 7.1), especially in the context of environmental change (section 5, section 6); b) how to distribute catch in space and time (section 2.3); c) how to determine ecosystem impacts from harvesting based on a revised CEMP (section 7.3); and d) how to improve modelling efforts since many of the available data for krill management are decades old, have high levels of uncertainty, or are seasonally biased (section 7.2). Key will be to better predict variation in the spatial and temporal distribution and abundance of krill (section 7.3), and how predators utilise cues to seek out available foraging opportunities (section 3), competing with the krill fishery. Future management must include explicit reference to the major krill consumers, both now and into the future.
CCAMLR first met in 1982. Since then, it has sought to implement a management system that satisfies all Members. In 2019, CCAMLR endorsed a revised management framework, aspects of which require further scrutiny. Once fully implemented and tested, the pilot analytical project in Subarea 48.1 could be rolled out for other locations where krill fishing takes place. Given the historical abundance of baleen whales within the southwest Atlantic (see Figures 2–5), certain areas will require considerable analysis and field testing. Important areas within Subarea 48.1 include Elephant Island, the South Shetland Islands, the Bransfield Strait, and the Gerlache Strait. Similarly, within Subarea 48.2 the area to the west of Coronation Island is important. Whilst within Subarea 48.3 the area to the north of South Georgia is important. If CCAMLR is unable to reach consensus, or if the pilot project fails for any reason, the existing highly precautionary management approach must remain in place. Vitally, CCAMLR must grasp the levels of management uncertainty.
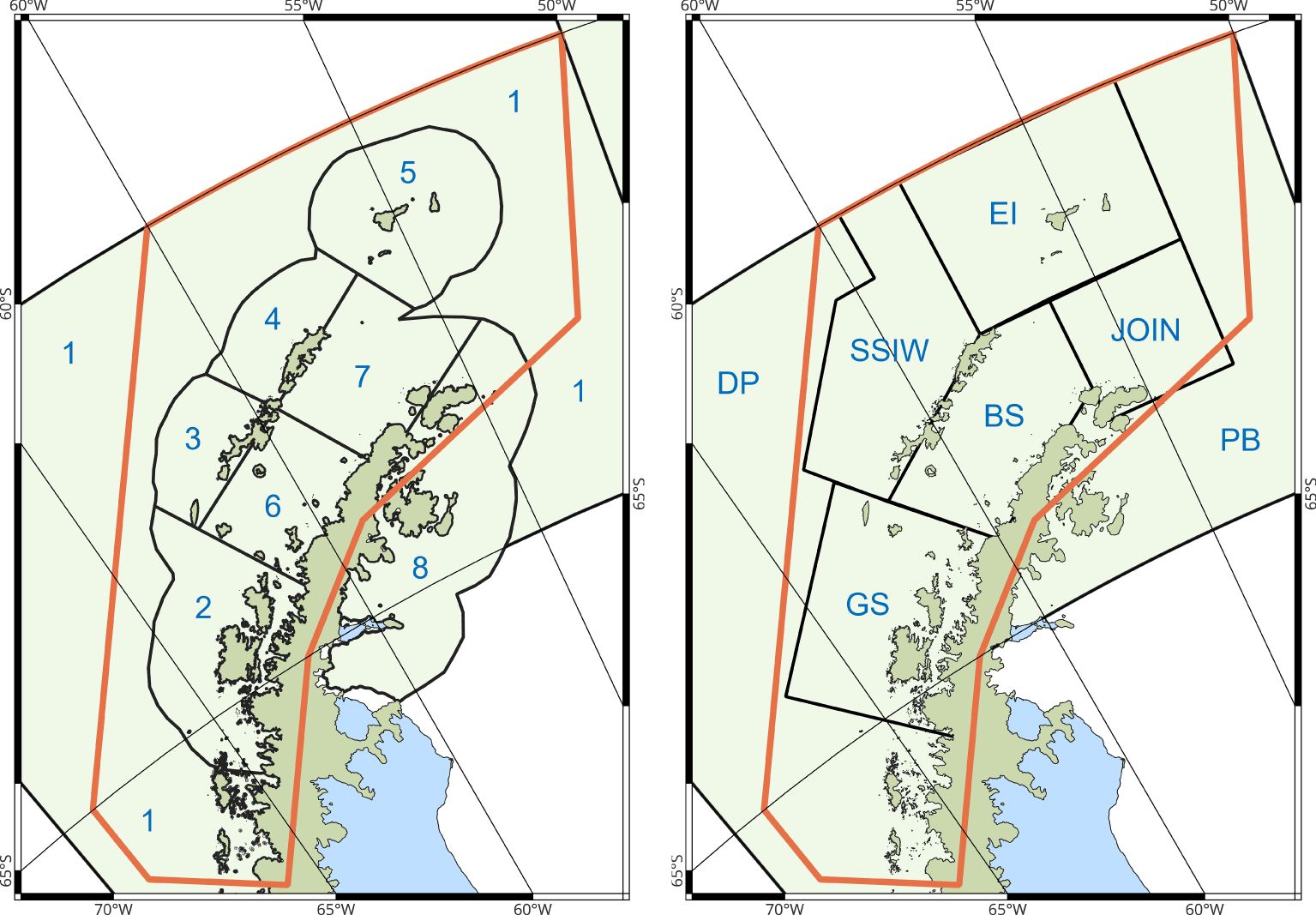
Figure 7. (Left) Small-scale management units (SSMUs; Hewitt et al., 2004) in Subareas 48.1. SSMUs are: (1) Antarctic Peninsula Pelagic Area (APPA); (2) Antarctic Peninsula West (APW); (3) Drake Passage West (APDPW); (4) Drake Passage East (APDPE); (5) Elephant Island (APEI); (6) Bransfield Strait West (APBSW); (7) Bransfield Strait East (APBSE); (8) Antarctic Peninsula East (APE). Note that SSMU APE extends partly into Subarea 48.5. (Right) Provisional krill management units within Subarea 48.1 under consideration by CCAMLR: (EI) Elephant Island; (JOIN) Joinville; (BS) Bransfield Strait; (SSIW) South Shetland Islands West; (GS) Gerlache Strait; (DP) Drake Passage; (PB) Powell Basin. The orange polygon outlines the wider area used by the krill fishery in Subarea 48.1 (Trathan et al., 2018b), and used as the modelling domain by Warwick-Evans et al. (2022b; 2022c).
The revised management framework relies upon the best-available science (see CCAMLR, 2009 [Resolution 31/XXVIII]). However, there are a number of important caveats, raised here and by Trathan (2023a). Time will tell whether best-available science is also adequate for managing an expanding krill fishery. The expiration of CM 51-07 (CCAMLR, 2023b) now increases the urgency for developing the revised framework.
Providing data for parameterising the revised management framework will be beyond the scope of any single National Antarctic Program (Trathan, 2023a). International collaboration will be key. CCAMLR requires an ecosystem approach to fisheries management. The recovery of baleen whale populations will now challenge the implementation of this approach. Improved understanding about baleen whale population status, and their role in the ecosystem will require close collaboration with the IWC Scientific Committee.
Author contributions
PNT: Conceptualization, Formal analysis, Funding acquisition, Investigation, Methodology, Project administration, Supervision, Visualization, Writing – original draft, Writing – review & editing. MSS: Conceptualization, Investigation, Methodology, Validation, Writing – review & editing. AF: Conceptualization, Investigation, Methodology, Validation, Writing – review & editing. MB: Investigation, Methodology, Validation, Writing – review & editing. EB: Investigation, Methodology, Validation, Writing – review & editing. TC: Investigation, Methodology, Validation, Writing – review & editing. LDR: Investigation, Methodology, Validation, Writing – review & editing. HH: Investigation, Methodology, Validation, Writing – review & editing. ERS: Investigation, Methodology, Validation, Writing – review & editing. ANZ: Investigation, Methodology, Validation, Writing – review & editing. RRR: Conceptualization, Investigation, Methodology, Validation, Writing – review & editing.
Funding
The author(s) declare financial support was received for the research, authorship, and/or publication of this article. The Workshop held at the Oxford University Museum of Natural History was funded by the Great Blue Ocean coalition (comprising The Pew Charitable Trusts, the Blue Marine Foundation, the Zoological Society of London, Greenpeace UK, the Marine Conservation Society and the Royal Society for the Protection of Birds), with support from The Blue Nature Alliance and Bloomberg Philanthropies Ocean Initiative and Oceans 5, a sponsored project of Rockefeller Philanthropy Advisors. PNT was supported by The Blue Nature Alliance and Bloomberg Philanthropies Ocean Initiative and Oceans 5, a sponsored project of Rockefeller Philanthropy Advisors. RRR was supported by the UK government through Darwin Plus grant DPLUS185. MSS was supported by MAC3 Impact Philanthropies.
Acknowledgments
We thank two reviewers and the editor for helpful comments. The scientific results and conclusions, as well as any views or opinions expressed herein, are those of the authors and do not necessarily reflect those of their institutes, or government departments, including NOAA or the US Department of Commerce.
Conflict of interest
Author MB is a self-employed consultant trading as Wildscope.
The remaining authors declare that the research was conducted in the absence of any commercial or financial relationships that could be construed as a potential conflict of interest.
Publisher’s note
All claims expressed in this article are solely those of the authors and do not necessarily represent those of their affiliated organizations, or those of the publisher, the editors and the reviewers. Any product that may be evaluated in this article, or claim that may be made by its manufacturer, is not guaranteed or endorsed by the publisher.
Supplementary material
The Supplementary Material for this article can be found online at: https://www.frontiersin.org/articles/10.3389/fmars.2024.1458042/full#supplementary-material
References
Agnew D. J. (1997). The CCAMLR ecosystem monitoring programme. Antarctic Sci. 9, 235–242. doi: 10.1017/S095410209700031X
Agnew D. J. (2004). Fishing South (St. Albans: The History and Management of South Georgia Fisheries. The Penna Press).
Allison C. (2016). IWC summary catch database Version 6.1; Date: 18 July 2016 (Histon, Cambridge, UK: IWC Secretariat).
Atkinson A., Hill S. L., Pakhomov E. A., Siegel V., Reiss C. S., Loeb V. J., et al. (2019). Krill (Euphausia superba) distribution contracts southward during rapid regional warming. Nat. Climate Change 9, 142–147. doi: 10.1038/s41558-018-0370-z
Atkinson A., Siegel V., Pakhomov E. A., Rothery P., Loeb V., Ross R. M., et al. (2008). Oceanic circumpolar habitats of Antarctic krill. Mar. Ecol. Prog. Ser. 362, 1–23. doi: 10.3354/meps07498
Baines M., Jackson J. A., Fielding S., Warwick-Evans V., Reichelt M., Trathan P. N. (2022). Ecological interactions between Antarctic krill (Euphausia superba) and baleen whales in the South Sandwich Islands region – exploring predator-prey biomass ratios. Deep Sea Res. I 189, 103867. doi: 10.1016/j.dsr.2022.103867
Baines M., Kelly N., Reichelt M., Lacey C., Pinder S., Fielding S., et al. (2021). Population abundance of recovering humpback whales Megaptera novaeangliae and other baleen whales in the Scotia Arc, South Atlantic. Mar. Ecol. Prog. Ser. 676, 77–94. doi: 10.3354/meps13849
Ballance L. T., Pitman R. L., Hewitt R. P., Siniff D. B., Trivelpiece W. Z., Clapham P. J., et al. (2006). “The removal of large whales from the Southern Ocean: evidence for long-term ecosystem effects?,” in Whales whaling, and ocean ecosystems. Eds. Estes J. A., DeMaster D. P., Doak D. F., Williams T. M., Brownell R. L. (Oakland, California, USA: University of California Press).
Bamford C., Kelly N., Herr H., Seyboth E., Jackson J. A. (2023). The recovery of Antarctica’s giants – baleen whales. Available online at: https://environments.aq/publications/the-recovery-of-Antarcticas-giants-baleen-whales/ (accessed November 21, 2024).
Bamford C. C. G., Jackson J. A., Kennedy A. K., Trathan P. N., Staniland I. J., Andriolo A., et al. (2022). Humpback whale (Megaptera novaeangliae) distribution and movements in the vicinity of South Georgia and the South Sandwich Islands Marine Protected Area. Deep Sea Res. II 198, 10507. doi: 10.1016/j.dsr2.2022.105074
Bamford C. C. G., Kelly N., Rosa L. D., Cade D. E., Fretwell P. T., Trathan P. N., et al. (2020). A comparison of baleen whale density estimates derived from overlapping satellite imagery and a shipborne survey. Sci. Rep. 10, 12985. doi: 10.1038/s41598-020-69887-y
Bedriñana-Romano L., Zerbini A. N., Andriolo A., Danilewicz D., Sucunza F. (2022). Individual and joint estimation of humpback whale migratory patterns and their environmental drivers in the Southwest Atlantic Ocean. Sci. Rep. 12, 7487. doi: 10.1038/s41598-022-11536-7
Bengtson-Nash S. M., Castrillon J., Eisenmann P., Fry B., Shuker J. D., Cropp R. A., et al. (2018). Signals from the south; humpback whales carry messages of Antarctic sea-ice ecosystem variability. Global Change Biol. 24, 1500–1510. doi: 10.1111/gcb.14035
Bestley S., Ropert-Coudert Y., Nash S. B., Brooks C. M., Cotte C., Dewar M., et al. (2020). Marine ecosystem assessment for the southern ocean: birds and marine mammals in a changing climate. Front. Ecol. Evol. 8. doi: 10.3389/fevo.2020.566936
Bierlich K. C., Hewitt J., Schick R. S., Pallin L., Dale J., Friedlaender A. S., et al. (2022). Seasonal gain in body condition of foraging humpback whales along the Western Antarctic Peninsula. Front. Mar. Sci. 9. doi: 10.3389/fmars.2022.1036860
Biuw M., Lindstrøm U., Jackson J. A., Baines M., Kelly N., McCallum G., et al. (2024). Estimated summer abundance and krill consumption of fin whales throughout the Scotia Sea during the 2018/2019 summer season. Sci. Rep. 14, 7493. doi: 10.1038/s41598-024-57378-3
Bonner W. N. (1984). “Conservation in the Antarctic,” in Antarctic ecology volume II. Ed. Laws R. M. (Academic Press, London, UK).
Butterworth D. S., Punt A. E., Basson M. (1992). A simple approach for calculating the potential yield of krill from biomass survey results. SC-CAMLR Selected Sci. Papers 8, 207–217. Available online at: https://www.ccamlr.org/en/system/files/science_journal_papers/12-Butterworth-et-al.pdf.
Cade D. E., Kahane-Rapport S. R., Gough W. T., Bierlich K. C., Linsky J. M. J., Calambokidis J., et al. (2023). Minke whale feeding rate limitations suggest constraints on the minimum body size for engulfment filtration feeding. Nat. Ecol. Evol. 7, 535–546. doi: 10.1038/s41559-023-01993-2
Cade D. E., Kahane-Rapport S. R., Wallis B., Goldbogen J. A., Friedlaender A. S. (2022). Evidence for size-selective predation by Antarctic humpback whales. Front. Mar. Sci. 9. doi: 10.3389/fmars.2022.747788
Calderan S., Black A., Branch T., Collins M. A., Kelly N., Leaper R., et al. (2020). South Georgia blue whales five decades after the end of whaling. Endangered Species Res. 43, 359–373. doi: 10.3354/esr01077
Calderan S. V., Dornan T., Fielding S., Irvine R., Jackson J. A., Leaper R., et al. (2023). Observations of southern right whales (Eubalaena australis) surface feeding on krill in austral winter at South Georgia. Mar. Mammal Sci. 39, 1306–1312. doi: 10.1111/mms.13025
Cavan E. L., Mackay N., Hill S. L., Atkinson A., Belcher A., Visser A. (2024). Antarctic krill sequester similar amounts of carbon to key coastal blue carbon habitats. Nat. Commun. 15, 7842. doi: 10.1038/s41467-024-52135-6
CCAMLR (1980). Convention on the conservation of antarctic marine living resources. (Hobart, Australia: Commission for the Conservation of Antarctic Marine Living Resources). Available online at: https://www.ccamlr.org/en/organisation/camlr-convention-text (accessed November 21, 2024).
CCAMLR (2008). Resolution 28/XXVII Ballast water exchange in the Convention Area. (Hobart, Australia: Commission for the Conservation of Antarctic Marine Living Resources). Available online at: https://cm.ccamlr.org/en/resolution-28/xxvii-2008 (accessed November 21, 2024).
CCAMLR (2009). Resolution 31/XXVIII Best available science (Hobart, Australia: Commission for the Conservation of Antarctic Marine Living Resources). Available online at: https://cm.ccamlr.org/en/resolution-31/xxviii-2009 (accessed November 21, 2024).
CCAMLR (2010). Conservation Measure 51-01 Precautionary catch limitations on Euphausia superba in Statistical Subareas 48.1, 48.2, 48.3 and 48.4 (Hobart, Australia: Commission for the Conservation of Antarctic Marine Living Resources). Available online at: https://cm.ccamlr.org/en/measure-51-01-2010 (accessed November 21, 2024).
CCAMLR (2014). CCAMLR ecosystem monitoring program standard methods (Hobart, Australia: Commission for the Conservation of Antarctic Marine Living Resources). Available online at: https://www.ccamlr.org/en/document/publications/ccamlr-ecosystem-monitoring-program-standard-methods (accessed November 21, 2024).
CCAMLR (2019). Report of the thirty-eighth meeting of the Commission (Hobart, Australia: Commission for the Conservation of Antarctic Marine Living Resources). Available online at: https://meetings.ccamlr.org/system/files/e-cc-38_0.pdf (accessed November 21, 2024).
CCAMLR (2021). “Summary of incidental mortality associated with fishing activities collected in scientific observer and vessel data during the 2020 and 2021 seasons,” in Working Group paper WG-FSA-2021/04 Rev. 1 submitted to CCAMLR WG-FSA-21. (Hobart, Australia: Commission for the Conservation of Antarctic Marine Living Resources). Available online at: https://meetings.ccamlr.org/en/wg-fsa-2021/04-rev-1 (accessed November 21, 2024).
CCAMLR (2022). Conservation Measure 26-01 General environmental protection to be taken by fishing vessels. (Hobart, Australia: Commission for the Conservation of Antarctic Marine Living Resources). Available online at: https://cm.ccamlr.org/en/measure-26-01-2022 (accessed November 21, 2024).
CCAMLR (2023a). CCAMLR krill fishery report (Hobart, Australia: Commission for the Conservation of Antarctic Marine Living Resources). Available online at: https://fishdocs.ccamlr.org/FishSum_48_KRI_2023.pdf https://fishdocs.ccamlr.org/FishRep_48_KRI_2023.pdf https://fishdocs.ccamlr.org/SpDescr_KRI_2023.pdf https://fishdocs.ccamlr.org/SAreport_48_KRI_2023.pdf (accessed November 21, 2024).
CCAMLR (2023b). Conservation Measure 51-07 Interim distribution of the trigger level in the fishery for Euphausia superba in Statistical Subareas 48.1, 48.2, 48.3 and 48.4 (Hobart, Australia: Commission for the Conservation of Antarctic Marine Living Resources). Available online at: https://cm.ccamlr.org/en/measure-51-07-2023 (accessed November 21, 2024).
CCAMLR (2023c). Statistical bulletin volume 35. (Hobart, Australia: Commission for the Conservation of Antarctic Marine Living Resources). Available online at: https://www.ccamlr.org/en/document/data/ccamlr-statistical-bulletin-vol-35 (accessed November 21, 2024).
CCAMLR (2023d). Conservation Measure 25-03 Minimisation of the incidental mortality of seabirds and marine mammals in the course of trawl fishing in the Convention Area. (Hobart, Australia: Commission for the Conservation of Antarctic Marine Living Resources). Available online at: https://cm.ccamlr.org/en/measure-25-03-2023 (accessed November 21, 2024).
Cheeseman T., Barlow J., Acebes J. M., Audley K., Bejder L., Birdsall C., et al. (2024). Bellwethers of change: population modelling of North Pacific humpback whales from 2002 through 2021 reveals shift from recovery to climate response. R. Soc. Open Sci. 11, 231462. doi: 10.1098/rsos.231462
Cheeseman T., Southerland K., Park J., Olio M., Flynn K., Calambokidis J., et al. (2022). Advanced image recognition: a fully automated, high-accuracy photo-identification matching system for humpback whales. Mamm. Biol. 2021, 915–929. doi: 10.1007/S42991-021-00180-9
Christensen L. B. (2006). “Marine mammal populations: Reconstructing historical abundances at the global scale. Fisheries Centre Research Reports 14(9),” in Fisheries Centre (Vancouver: University of British Columbia). Available online at: https://open.library.ubc.ca/soa/cIRcle/collections/facultyresearchandpublications/52383/items/1.0074757.
Clapham P. J. (1996). The social and reproductive biology of humpback whales: an ecological perspective. Mammal Rev. 26, 27–49. doi: 10.1111/j.1365-2907.1996.tb00145.x
Constable A. J. (2001). The ecosystem approach to managing fisheries: achieving conservation objectives for predators of fished species. CCAMLR Sci. 8, 37–64. Available online at: https://www.ccamlr.org/en/system/files/science_journal_papers/03constable.pdf.
Constable A. J., de la Mare W. K. (1996). A generalised model for evaluating yield and the long-term status of fish stocks under conditions of uncertainty. CCAMLR Sci. 3, 31–54. Available online at: https://www.ccamlr.org/en/system/files/science_journal_papers/03constable-delamare.pdf.
Constable A. J., de la Mare W. K., Agnew D. J., Everson I., Miller D. (2000). Managing fisheries to conserve the Antarctic marine ecosystem: practical implementation of the Convention on the Conservation of Antarctic Marine Living Resources (CCAMLR). ICES J. Mar. Sci. 57, 778–791. doi: 10.1006/jmsc.2000.0725
Constable A., Kawaguchi S., Sumner M., Trathan P., Warwick-Evans V. (2023). A dynamic framework for assessing and managing risks to ecosystems from fisheries: demonstration for conserving the krill-based food web in Antarctica. Front. Ecol. Evol. 11. doi: 10.3389/fevo.2023.1043800
Cooke J., Rowntree V., Payne R. (2003). Analysis of inter-annual variation in reproductive success of South Atlantic right whales (Eubalaena australis) from photo-identifications of calving females observed off Península Valdés, Argentina, during 1971–2000, in Paper SC/55/O23 submitted to the Scientific Committee of the International Whaling Commission (Histon, Cambridge, UK: IWC Secretariat).
Crespo E. A., Pedraza S. N., Svendsen G. M., Degrati M., Coscarella M. A. (2019). The southwestern Atlantic southern right whale, Eubalaena australis, population is growing but at a decelerated rate. Mar. Mammal Sci. 35, 93–107. doi: 10.1111/mms.12526
Croxall J. P., Trathan P. N. (2004). “The Southern Ocean: a model system for conserving resources?,” in Defying Ocean's end: an agenda for action. Eds. Glover L. K., Earle S. A. (Island Press, Washington D.C).
Cury P. M., Boyd I. L., Bonhommeau S., Anker-Nilssen T., Crawford R. J. M., Furness R. W., et al. (2011). Global seabird response to forage fish depletion: One-third for the birds. Science 334, 1703–1706. doi: 10.1126/science.1212928
Czapanskiy M. F., Savoca M. S., Gough W. T., Segre P. S., Wisniewska D. M., Cade D. E., et al. (2021). Modelling short-term energetic costs of sonar disturbance to cetaceans using high-resolution foraging data. J. Appl. Ecol. 58, 1643–1657. doi: 10.1111/1365-2664.13903
Dalla Rosa L., Secchi E. R., Maia Y. G., Zerbini A. N., Heide-Jørgensen M. P. (2008). Movements of satellite-monitored humpback whales on their feeding ground along the Antarctic Peninsula. Polar Biol. 31, 771–781. doi: 10.1007/s00300-008-0415-2
da Silva J. R. M. C., Bergami E., Gomes V., Corsi I. (2023). Occurrence and distribution of legacy and emerging pollutants including plastic debris in Antarctica: Sources, distribution and impact on marine biodiversity. Mar. pollut. Bull. 186, 114353. doi: 10.1016/j.marpolbul.2022.114353
Doughty C. E., Roman J., Faurby S., Wolf A., Haque A., Bakker E. S., et al. (2016). Global nutrient transport in a world of giants. Proc. Natl. Acad. Sci. U.S.A. 113, 868–873. doi: 10.1073/pnas.15025491
Elemans C. P. H., Jiang W., Jensen M. H., Pichler H., Mussman B. R., Nattestad J., et al. (2024). Evolutionary novelties underlie sound production in baleen whales. Nature 627, 123–129. doi: 10.1038/s41586-024-07080-1
Erbe C., Marley S. A., Schoeman R., Smith J. N., Trigg L. E., Embling C. B. (2019). The effects of ship noise on marine mammals — A review. Front. Mar. Sci. 6, 606. doi: 10.3389/fmars.2019.00606
Espinasse B., Zhou M., Zhu Y., Hazen E. L., Friedlaender A. S., Nowacek D. P., et al. (2012). Austral fall-winter transition of mesozooplankton assemblages and krill aggregations in an embayment west of the Antarctic Peninsula. Mar. Ecol. Prog. Ser. 452, 63–80. doi: 10.3354/meps09626
Estes J. A., Heithaus M., McCauley D. J., Rasher D. B., Worm B. (2016). Megafaunal impacts on structure and function of ocean ecosystems. Annu. Rev. Environments Resour. 41, 83–116. doi: 10.1146/annurev-environ-110615-085622
Everson I. (1977). “The living resources of the Southern Ocean,” in Southern ocean fisheries survey programme GLO/SO/77/1 (FAO, Rome, Italy).
Everson I., Kock K.-H., Parkes G. (1997). Interannual variation in condition of the mackerel icefish. J. Fish Biol. 51, 146–154. doi: 10.1111/j.1095-8649.1997.tb02520.x
Fauchald P., Tveraa T. (2003). Using First-Passage Time in the analysis of area-restricted search and habitat selection. Ecology 84, 282–288. doi: 10.1890/0012-9658(2003)084[0282:UFPTIT]2.0.CO;2
Fielding S., Watkins J., Cossio A., Reiss C., Watters G., Calise L., et al. (2011). “The ASAM 2010 assessment of krill biomass for Area 48 from the Scotia Sea CCAMLR 2000 Synoptic Survey,” in Working Group paper WG-EMM-11/20 submitted to CCAMLR WG-EMM-11. (Hobart, Australia: Commission for the Conservation of Antarctic Marine Living Resources). Available online at: https://meetings.ccamlr.org/en/wg-emm-11/20.
Fielding S., Watkins J. L., Trathan P. N., Enderlein P., Waluda C. M., Stowasser G., et al. (2014). Interannual variability in Antarctic krill (Euphausia superba) density at South Georgia, Southern Ocean: 1997-2013. ICES J. Mar. Sci. 71, 2578–2588. doi: 10.1093/icesjms/fsu104
Filun D., Thomisch K., Boebel O., Brey T., Širović A., Spiesecke S., et al. (2020). Frozen verses: Antarctic minke whales (Balaenoptera bonaerensis) call predominantly during austral winter. R. Soc. Open Sci. 7, 192112. doi: 10.1098/rsos.192112
Findlay C. R., Rojano-Doñate L., Tougaard J., Johnson M. P., Madsen M. P. (2023). Small reductions in cargo vessel speed substantially reduce noise impacts to marine mammals. Sci. Adv. 9, eadf2987. doi: 10.1126/sciadv.adf2987
Flores H., Atkinson A., Kawaguchi S., Krafft B. A., Milinevsky G., Nicol S., et al. (2012). Impact of climate change on Antarctic krill. Mar. Ecol. Prog. Ser. 458, 1–19. doi: 10.3354/meps09831
Forcada J., Trathan P. N., Boveng P. L., Boyd I. L., Burns J. M., Costa D. P., et al. (2012). Responses of Antarctic pack-ice seals to environmental change and increasing krill fishing. Biol. Conserv. 149, 40–50. doi: 10.1016/j.biocon.2012.02.002
Fortune S. M. E., Trites A. W., Mayo C. A., Rosen D. A. S., Hamilton P. K. (2013). Energetic requirements of North Atlantic right whales and the implications for species recovery. Mar. Ecol. Prog. Ser. 478, 253–272. doi: 10.3354/meps10000
Fraser A. D., Massom R. A., Handcock M. S., Reid P., Ohshima K. I., Raphael M. N., et al. (2021). Eighteen-year record of circum-Antarctic landfast-sea-ice distribution allows detailed baseline characterisation and reveals trends and variability. Cryosphere 15, 5061–5077. doi: 10.5194/tc-15-5061-2021
Friedlaender A. S., Goldbogen J. A., Nowacek D. P., Read A. J., Johnston D., Gales N. (2014). Feeding rates and under-ice foraging strategies of the smallest lunge filter feeder, the Antarctic minke whale (Balaenoptera bonaerensis). J. Exp. Biol. 217, 2851–2854. doi: 10.1242/jeb.106682
Friedlaender A. S., Johnston D. W., Tyson R. B., Kaltenberg A., Goldbogen J. A., Stimpert A. K., et al. (2016). Multiple-stage decisions in a marine central-place forager. R. Soc. Open Sci. 3, 160043. doi: 10.1098/rsos.160043
Friedlaender A., Joyce T., Johnston D., Read A., Nowacek D., Goldbogen J., et al. (2021). Sympatry and resource partitioning between the largest krill consumers around the Antarctic Peninsula. Mar. Ecol. Prog. Ser. 669, 1–16. doi: 10.3354/meps13771
Friedlaender A. S., Tyson R. B., Stimpert A. K., Read A. J., Nowacek D. P. (2013). Extreme diel variation in the feeding behavior of humpback whales along the western Antarctic Peninsula during autumn. Mar. Ecol. Prog. Ser. 494, 281–289. doi: 10.3354/meps10541
Garcia-Garin O., Sahyoun W., Net S., Vighi M., Aguilar A., Ouddane B., et al. (2022). Intrapopulation and temporal differences of phthalate concentrations in North Atlantic fin whales (Balaenoptera physalus). Chemosphere 300, 134453. doi: 10.1016/j.chemosphere.2022.134453
García-Vernet R., Borrell A., Víkingsson G., Halldórsson S. D., Aguilar A. (2021). Ecological niche partitioning between baleen whales inhabiting Icelandic waters. Prog. Oceanography 199, 102690. doi: 10.1016/j.pocean.2021.102690
Gilbert L., Jeanniard-du-Dot T., Authier M., Chouvelon T., Spitz J. (2023). Composition of cetacean communities worldwide shapes their contribution to ocean nutrient cycling. Nat. Commun. 14, 5823. doi: 10.1038/s41467-023-41532-y
Goldbogen J. A., Southall B. L., DeRuiter S. L., Calambokidis J., Friedlaender A. S., Hazen E. L., et al. (2013). Blue whales respond to simulated mid-frequency military sonar. Proc. R. Soc. Ser. B 280, 20130657. doi: 10.1098/rspb.2013.0657
Grant S. M. (2001). “Population increase of the Antarctic fur seal (Arctocephalus gazella) in the Scotia Sea: implications for management,” in M.Phil. Thesis in polar studies. Cambridge, UK: Scott Polar Research Institute, University of Cambridge.
Halpern B. S., Frazier M., Potapenko J., Casey K. S., Koenig K., Longo C., et al. (2015). Spatial and temporal changes in cumulative human impacts on the world’s ocean. Nat. Commun. 6, 7615. doi: 10.1038/ncomms8615
Halpern B. S., Walbridge S., Selkoe K. A., Kappel C. V., Micheli F., D’Agrosa C., et al. (2008). A global map of human impact on marine ecosystems. Science 319, 948. doi: 10.1126/science.1149345
Hamer D. J., Ward T. M., McGarvey R. (2008). Measurement, management and mitigation of operational interactions between the South Australian Sardine Fishery and short-beaked common dolphins (Delphinus delphis). Biological Conservation 141 (11), 2865–2878. doi: 10.1016/j.biocon.2008.08.024
Hamner W. M., Hamner P. P., Strand S. W., Gilmer R. W. (1983). Behavior of Antarctic krill (Euphausia superba): Chemoreception, Feeding, Schooling, and Molting. Science 220, 433–435. doi: 10.1126/science.220.4595.433
Hart I., Edmundson W. (2017). A history of whaling in Brazil: from royal fish to Japanese delicacy (Newton St Margarets, Herefordshire: Pequena).
Hazen E. L., Scales K. L., Maxwell S. M., Briscoe D. K., Welch H., Bograd S. J., et al. (2018). A dynamic ocean management tool to reduce bycatch and support sustainable fisheries. Sci. Adv. 4, eaar3001. doi: 10.1126/sciadv.aar3001
Hedley S., Reilly S., Borberg J., Holland R., Hewitt R., Watkins J., et al. (2001). “Modelling whale distribution: a preliminary analysis of data collected on the CCAMLR-IWC Krill Synoptic Survey,” in Paper SC/53/E9 submitted to the Scientific Committee of the International Whaling Commission (Histon, Cambridge, UK: IWC Secretariat).
Henderson A. F., Hindell M. A., Wotherspoon S., Biuw M., Lea M.-A., Kelly N., et al. (2023). Assessing the viability of estimating baleen whale abundance from tourist vessels. Front. Mar. Sci. 10. doi: 10.3389/fmars.2023.1048869
Herr H., Viquerat S., Devas F., Lees A., Wells L., Gregory B., et al. (2022). Return of large fin whale feeding aggregations to historical whaling grounds in the Southern Ocean. Sci. Rep. 12, 9458. doi: 10.1038/s41598-022-13798-7
Herr H., Viquerat S., Siegel V., Kock K.-H., Dorschel B., Huneke W. G. C., et al. (2016). Horizontal niche partitioning of humpback and fin whales around the West Antarctic Peninsula: evidence from a concurrent whale and krill survey. Polar Biol. 39, 799–818. doi: 10.1007/s00300-016-1927-9
Hewitt R. P., Watters G., Trathan P. N., Croxall J. P., Goebel M. E., Ramm D., et al. (2004). Options for allocating the precautionary catch limit of krill among small-scale management units in the Scotia Sea. CCAMLR Sci. 11, 81–97. Available online at: https://www.ccamlr.org/en/publications/science_journal/ccamlr-science-volume-11/ccamlr-science-volume-1181-97.
Hill S., Reid K., Thorpe S. E., Hinke J. T., Watters G. M. (2007). A compilation of parameters for ecosystem dynamics models of the Scotia Sea–Antarctic Peninsula region. CCAMLR Sci. 14, 1–25. Available online at: https://www.ccamlr.org/en/publications/science_journal/ccamlr-science-volume-14/ccamlr-science-volume-141-25.
Hobbs W. R., Massom R., Stammerjohn S., Reid P., Williams G., Meier W. (2016). A review of recent changes in Southern Ocean sea-ice, their drivers and forcings. Global Planetary Change 143, 228–250. doi: 10.1016/j.gloplacha.2016.06.008
Hofman R. J. (2017). Sealing, whaling and krill fishing in the Southern Ocean: past and possible future effects on catch regulations. Polar Rec. 53, 88–99. doi: 10.1017/S0032247416000644
Hofman R. J. (2019). Stopping overexploitation of living resources on the high seas. Mar. Policy 103, 91–100. doi: 10.1016/j.marpol.2019.02.037
Hückstädt L. A., Piñones A., Palacios D. M., McDonald B. I., Dinniman M. S., Hofmann E. E., et al. (2020). Projected shifts in the foraging habitat of crabeater seals along the Antarctic Peninsula. Nat. Climate Change 10, 472–477. doi: 10.1038/s41558-020-0745-9
Hutchinson A. (2023). Using First Passage Time and Time-Spent modelling to compare krill search behaviour of baleen whales and krill fishing vessels in the West Antarctic Peninsula. Dissertation submitted to the University of Southampton. University of Southampton, Southampton, UK.
IMO (2009). Guidance document for minimizing the risk of ship strikes with cetaceans. Available online at: https://wwwcdn.imo.org/localresources/en/MediaCentre/HotTopics/Documents/674.pdf. (accessed November 21, 2024).
IWC (1983). Thirty-third report of the International Whaling Commission (Cambridge, UK: International Whaling Commission).
Jackson J. A., Kennedy A., Moore M., Andriolo A., Bamford C. C. G., Calderan S., et al. (2020). Have whales returned to a historical hotspot of industrial whaling? The pattern of southern right whale Eubalaena australis recovery at South Georgia. Endangered Species Res. 43, 323–339. doi: 10.3354/esr01072
Jackson J. B. C., Kirby M. X., Berger W. H., Bjorndal K. A., Botsford L. W., Bourque B. J., et al. (2001). Historical overfishing and the recent collapse of coastal ecosystems. Science 293, 629. doi: 10.1126/science.1059199
Jackson J. A., Ross-Gillespie A., Butterworth D., Findlay K., Holloway S., Robbins J., et al. (2015). Southern hemisphere humpback whale comprehensive assessment. A synthesis and summary: 2005–2015. Paper SC/66a/SH/3 submitted to the Scientific Committee of the International Whaling Commission (Histon, Cambridge, UK: IWC Secretariat).
Johannessen J. E. D., Biuw M., Lindstrøm U., Ollus V. M. S., López L. M. M., Gkikopoulou K. C., et al. (2022). Intra-season variations in distribution and abundance of humpback whales in the West Antarctic Peninsula using cruise vessels as opportunistic platforms. Ecol. Evol. 12, e8571. doi: 10.1002/ece3.8571
Johannessen E. D., Krafft B., Donovan C. R., Wiff R., Caneco B., Lowther A. (2024). Sensitivity of the stock assessment for the Antarctic krill fishery to time-varying natural and fishing mortality. Fisheries Manage. Ecol. 00, e12721. doi: 10.1111/fme.12721
Kanaji Y., Williams R., Zerbini A. N., Branch T. A. (2024). Density dependence only affects increase rates in baleen whale populations at high abundance levels. J. Appl. Ecol. 61, 2258–2269. doi: 10.1111/1365-2664.14744
Kawaguchi S., Ishida A., King R., Raymond B., Waller N., Constable A., et al. (2013). Risk maps for Antarctic krill under projected Southern Ocean acidification. Nat. Climate Change 3, 843–847. doi: 10.1038/nclimate1937
Keen E. M., Scales K. L., Rone B. K., Hazen E. L., Falcone E. A., Schorr G. S. (2019). Night and day: diel differences in ship strike risk for fin whales (Balaenoptera physalus) in the California current system. Front. Mar. Sci. 6. doi: 10.3389/fmars.2019.00730
Kennedy A. S., Carroll E. L., Baker C. S., Bassoi M., Buss D. L., Collins M. A., et al. (2020). Whales return to the epicentre of whaling? Preliminary results from the 2020 cetacean survey at South Georgia (Islas Georgias del Sur). Paper SC/68B/CMP/22 submitted to the Scientific Committee of the International Whaling Commission. (Histon, Cambridge, UK: IWC Secretariat).
Kennedy A. S., Carroll E. L., Zerbini A. N., Baker C. S., Bassoi M., Beretta N. A., et al. (2024). Photo-identification and satellite telemetry connect southern right whales from South Georgia Island (Islas Georgias del Sur) with multiple feeding and calving grounds in the southwest Atlantic. Mar. Mammal Sci. 40, e13089. doi: 10.1111/mms.13089
Kennedy M., Gray R. D. (1993). Can ecological theory predict the distribution of foraging animals? A critical analysis of experiments on the Ideal Free Distribution. Oikos 68, 158–166. Available online at: https://www.jstor.org/stable/3545322.
Kock K.-H. (1985). “Krill consumption by Antarctic notothenioid fish,” in Antarctic nutrient cycles and food webs. Eds. Siegfried W. R., Condy P. R., Laws R. M. (Springer-Verlag, Berlin Heidelberg).
Koehn L. E., Siple M. C., Essington T. E. (2021). A structured seabird population model reveals how alternative forage fish control rules benefit seabirds and fisheries. Ecol. Appl. 31, e02401. doi: 10.1002/eap.2401
Krafft B. A., Macaulay G. J., Skaret G., Knutsen T., Bergstad O. A., Lowther A., et al. (2021). Standing stock of Antarctic krill (Euphausia superba Dana 1850) (Euphausiacea) in the Southwest Atlantic sector of the Southern Ocean 2018-19. J. Crustacean Biol. 41, ruab046. doi: 10.1093/jcbiol/ruab046
Lascara C. M., Hofmann E. E., Ross R. M., Quetin L. B. (1999). Seasonal variability in the distribution of Antarctic krill, Euphausia superba, west of the Antarctic Peninsula. Deep Sea Res. I 46, 951–984. doi: 10.1016/S0967-0637(98)00099-5
Lavery T. J., Roudnew B., Seymour J., Mitchell J. G., Smetacek V., Nicol S. (2014). Whales sustain fisheries: blue whales stimulate primary production in the Southern Ocean. Mar. Mammal Sci. 30, 888–904. doi: 10.1111/mms.12108
Laws R. M. (1953). The elephant seal industry at South Georgia. Polar Rec. 6, 746–754. doi: 10.1017/S0032247400048154
Laws R. M. (1977). Seals and whales of the southern ocean. Philos. Trans. R. Soc. Ser. B 279, 81–96. doi: 10.1098/rstb.1977.0073
Leaper R., Collins M. A., Calderan S., Irvine R., Liszka C., Olson P., et al. (2023). Cruise report from winter surveys (May – September 2022) around South Georgia (Islas Georgias del Sur). Paper SC/69A/ASI/08 submitted to the Scientific Committee of the International Whaling Commission. (Histon, Cambridge, UK: IWC Secretariat).
Leaper R., Cooke J., Trathan P. N., Reid K., Rowntree V., Payne R. (2006). Global climate drives southern right whale (Eubalaena australis) population dynamics. Biol. Lett. 2, 289–292. doi: 10.1098/rsbl.2005.0431
Leaper R., Lavigne D. M. (2007). How much do large whales eat? J. Cetacean Res. Manage. 9, 179–188. Available online at: https://journal.iwc.int/index.php/jcrm/article/view/666.
Licandeo R., de la Puente S., Christensen V., Hilborn R., Walters C. (2023). A delay-differential model for representing small pelagic fish stock dynamics and its application for assessing alternative management strategies under environmental uncertainty. Fish Fisheries 24, 544–566. doi: 10.1111/faf.12743
Lockyer C. (1972a). The age at sexual maturity of the southern fin whale (Balaenoptera physalus) using annual layer counts in the ear plug. ICES J. Mar. Sci. 34, 276–294. doi: 10.1093/icesjms/34.2.276
Lockyer C. (1972b). A review of the weights of cetaceans with estimates of the growth and energy budgets of the large whales (Royal Holloway College, UK: University of London). Available online at: https://repository.royalholloway.ac.uk/items/f48ce3f6-19b3-4c21-9c62-2f31d68a1cad/1/.
Lockyer C. (1981). Growth and energy budgets of large baleen whales from the southern hemisphere. FAO Fisheries Ser. 5, 379–487.
Lockyer C. (1984). Review of baleen whale (Mysticeti) reproduction and implications for management. Rep. Int. Whaling Commission 6, 27–50.
Martin J., Gordon R., Fitzwater S. (1990). Iron in Antarctic waters. Nature 345, 156–158. doi: 10.1038/345156a0
Maxwell S. M., Hazen E. L., Lewison R. L., Dunn D. C., Bailey H., Bograd S. J., et al. (2015). Dynamic ocean management: Defining and conceptualizing real-time management of the ocean. Mar. Policy 58, 42–50. doi: 10.1016/j.marpol.2015.03.014
Meredith M. P., King J. C. (2005). Rapid climate change in the ocean west of the Antarctic Peninsula during the second half of the 20th century. Geophysical Res. Lett. 32, L19604. doi: 10.1029/2005GL024042
Meyer B., Atkinson A., Bernard K. S., Brierley A. S., Driscoll R., Hill S. L., et al. (2020). Successful ecosystem-based management of Antarctic krill should address uncertainties in krill recruitment, behaviour and ecological adaptation. Commun. Earth Environ. 1, 28. doi: 10.1038/s43247-020-00026-1259
Montes-Hugo M., Doney S. C., Ducklow H. W., Fraser W., Martinson D., Stammerjohn S. E., et al. (2009). Recent changes in phytoplankton communities associated with rapid regional climate change along the Western Antarctic Peninsula. Science 323, 147–1473. doi: 10.1126/science.1164533
Murphy E. J. (1995). Spatial structure of the Southern Ocean ecosystem: predator-prey linkages in Southern Ocean food webs. J. Anim. Ecol. 64, 333–347. doi: 10.2307/5895
Murphy E., Morris D. J., Watkins J. L., Priddle J. (1988). “Scales of interaction between Antarctic krill and the environment,” in Antarctic Ocean and resources variability. Ed. Sahrhage D. (Springer, Berlin, Germany).
Murphy E. J., Watkins J. L., Meredith M. P., Ward P., Trathan P. N., Thorpe S. E. (2004). Southern Antarctic Circumpolar Current Front to the northeast of South Georgia: Horizontal advection of krill and its role in the ecosystem. J. Geophysical Res. 109, C01029. doi: 10.1029/2002JC001522
Murphy E. L., Gerber L. R., Rochman C. M., Polidoro B. (2024). A macroplastic vulnerability index for marine mammals, seabirds, and sea turtles in Hawai’i. Sci. Total Environ. 908, 168247. doi: 10.1016/j.scitotenv.2023.168247
Nichols R. C., Cade D. E., Kahane-Rapport S., Goldbogen J., Stimpert A., Nowacek D., et al. (2022). Intra-seasonal variation in feeding rates and diel foraging behaviour in a seasonally fasting mammal, the humpback whale. R. Soc. Open Sci. 9, 211674. doi: 10.1098/rsos.211674
Nicol S., Bowie A., Jarman S., Lannuzel D., Meiners K. M., van der Merwe P. (2010). Southern Ocean iron fertilization by baleen whales and Antarctic krill. Fish Fisheries 11, 203–209. doi: 10.1111/j.1467-2979.2010.00356.x
Nicol S., Foster J., Kawaguchi S. (2012). The fishery for Antarctic krill – recent developments. Fish Fisheries 13, 30–40. doi: 10.1111/j.1467-2979.2011.00406.x
Nowacek D. P., Friedlaender A. S., Halpin P. N., Hazen E. L., Johnston D. W., Read A. J., et al. (2011). Super-aggregations of krill and humpback whales in Wilhelmina Bay, Antarctic Peninsula. PloS One 6, e19173. doi: 10.1371/journal.pone.0019173
Owen K. A., Goggins M., Black A., Ashburner J., Wilson A., Hollyman P. R., et al. (2024). At-sea distribution of marine predators around South Georgia during austral winter (and implications for fisheries management). Polar Biol. 47, 663–679. doi: 10.1007/s00300-024-03257-6
Pallin L. J., Kellar N. M., Steel D., Botero-Acosta N., Baker C. S., Conroy J. A., et al. (2023). A surplus no more? Variation in krill availability impacts reproductive rates of Antarctic baleen whales. Global Change Biol. 29, 2108–2121. doi: 10.1111/gcb.16559
Patton P. T., Cheeseman T., Abe K., Yamaguchi T., Reade W., Southerland K., et al. (2023). A deep learning approach to photo–identification demonstrates high performance on two dozen cetacean species. Methods Ecol. Evol. 14, 2611–2625. doi: 10.1111/2041-210X.14167
Pauly D., Christensen V., Dalsgaard J., Froese R., Torres F. Jr. (1998). Fishing down marine food webs. Science 279, 860–863. doi: 10.1126/science.279.5352.860
Pauly D., Froese R. (2021). MSY needs no epitaph—but it was abused. ICES J. Mar. Sci. 78, 2204–2210. doi: 10.1093/icesjms/fsaa224
Payne M. R. (1977). Growth of a fur seal population. Philos. Trans. R. Soc. London Ser. B 27, 67–79. doi: 10.1098/rstb.1977.0072
Perry F., Atkinson A., Sailley S. F., Tarling G. A., Hill S. L., Lucas C. H., et al. (2019). Habitat partitioning in Antarctic krill: spawning hotspots and nursery areas. PloS One 14, e0219325. doi: 10.1371/journal.pone.0219325
Punt A. E., Furness R. W., Parma A. M., Plagányi-Lloyd E., Sanchirico J. N., Trathan P. N. (2023). Report of the international review panel regarding fishing closures adjacent to South Africa’s African penguin breeding colonies and declines in the penguin population (Cape Town, South Africa: Department of Forestry, Fisheries and the Environment (DFFE)). Available online at: https://www.dffe.gov.za/sites/default/files/Reports/penguinbreeding_coloniesreport.pdf.
Punt A. E., MacCall A. D., Essington T. E., Francis T. B., Hurtado-Ferro F., Johnson K. F., et al. (2016). Exploring the implications of the harvest control rule for Pacific sardine, accounting for predator dynamics: A MICE model. Ecol. Model. 337, 79–95. doi: 10.1016/j.ecolmodel.2016.06.004
Pusch C., Hulley P. A., Kock K.-H. (2004). Community structure and feeding ecology of mesopelagic fishes in the slope waters of King George Island (South Shetland Islands, Antarctica). Deep-Sea Res. I 51, 1685–1708. doi: 10.1016/j.dsr.2004.06.008
Reeves R. R., Rolland R., Clapham P. J. (2001). “Causes of reproductive failure in North Atlantic right whales: new avenues and research. Report of a Workshop held 26–28 April 2000 in Falmouth, Massachusetts,” in Northeast Fisheries Science Center reference document 01–16 (166 Water St., Woods Hole, MA: National Marine Fisheries Service), 46.
Reid K., Watkins J. L., Murphy E. J., Trathan P. N., Fielding S., Enderlein P. (2010). Krill population dynamics at South Georgia: implications for ecosystem-based fisheries management. Mar. Ecol. Prog. Ser. 399, 243–252. doi: 10.3354/meps08356
Reidenberg J. S. (2024). An innovative way for whales to sing. Nature 627, 40–42. doi: 10.1038/d41586-024-00307-1
Reilly S., Hedley S., Borberg J., Hewitt R., Thiele D., Watkins J., et al. (2004). Biomass and energy transfer to baleen whales in the South Atlantic Sector of the Southern Ocean. Deep Sea Res. Part II 51, 1397–1409. doi: 10.1016/j.dsr2.2004.06.008
Reisinger R. R., Trathan P. N., Johnson C. M., Joyce T. W., Durban J. W., Pitman R. L., et al. (2022). Spatiotemporal overlap of baleen whales and krill fisheries in the western Antarctic Peninsula region. Front. In Mar. Sci. 9. doi: 10.3389/fmars.2022.914726
Reiss C. S., Cossio A. M., Loeb V., Demer D. A. (2008). Variations in the biomass of Antarctic krill (Euphausia superba) around the South Shetland Islands 1996-2006. ICES J. Mar. Sci. 65, 497–508. doi: 10.1093/icesjms/fsn033
Rockwood R. C., Adams J., Silber G., Jahncke J. (2020). Estimating effectiveness of speed reduction measures for decreasing whale-strike mortality in a high-risk region. Endangered Species Res. 43, 145–166. doi: 10.3354/esr01056
Roman J., McCarthy J. J. (2010). The whale pump: marine mammals enhance primary productivity in a coastal basin. PloS One 5, e13255. doi: 10.1371/journal.pone.0013255
Ryan C., Santangelo M., Stephenson B., Branch T. A., Wilson E. A., Savoca M. S. (2023). Commercial krill fishing within a foraging supergroup of fin whales in the Southern Ocean. Sci. Nat. 104, e4002. doi: 10.1002/ecy.4002
Santa Cruz F., Ernst B., Arata J. A., Parada C. (2018). Spatial and temporal dynamics of the Antarctic krill fishery in fishing hotspots in the Bransfield Strait and South Shetland Islands. Fisheries Res. 208, 157–166. doi: 10.1016/j.fishres.2018.07.020
Saraux C., Sydeman W. J., Piatt J. F., Anker-Nilssen T., Hentati-Sundberg J., Bertrand S., et al. (2021). Seabird-induced natural mortality of forage fish varies with fish abundance: Evidence from five ecosystems. Fish Fisheries 22, 262–279. doi: 10.1111/faf.12517
Savoca M. S., Czapanskiy M. F., Kahane-Rapport S. R., Gough W. T., Fahlbusch J. A., Bierlich K. C., et al. (2021). Baleen whale prey consumption based on high-resolution foraging measurements. Nature 599, 85–90. doi: 10.1038/s41586-021-03991-5
Savoca M. S., Kumar M., Sylvester Z., Czapanskiy M. F., Meyer B., Goldbogen J. A., et al. (2024). Whale recovery and the emerging human-wildlife conflict over Antarctic krill. Nat. Commun. 15, 7708. doi: 10.1038/s41467-024-51954-x
SC-CAMLR-X (1991). Report of the tenth meeting of the Scientific Committee (Hobart, Australia: CCAMLR). Available online at: https://meetings.ccamlr.org/system/files/e-sc-xxix.pdf.
SC-CAMLR-XXIX (2010). Report of the twenty-ninth meeting of the Scientific Committee (Hobart, Australia: Commission for the Conservation of Antarctic Marine Living Resources). Available online at: https://meetings.ccamlr.org/system/files/e-sc-xxix.pdf.
SC-CAMLR-XXXV (2016). Report of the thirty-fifth meeting of the Scientific Committee (Hobart, Australia: Commission for the Conservation of Antarctic Marine Living Resources). Available online at: https://www.ccamlr.org/en/system/files/e-sc-xxxv.pdf.
Schall E., Thomisch K., Boebel O., Gerlach G., Woods S. M., El-Gabbas A., et al. (2021). Multi-year presence of humpback whales in the Atlantic sector of the Southern Ocean but not during El Niño. Commun. Biol. 4, 790. doi: 10.1038/s42003-021-02332-6
Schoeman R. P., Patterson-Abrolat C., Plön S. (2020). A global review of vessel collisions with marine animals. Front. Mar. Sci. 7. doi: 10.3389/fmars.2020.00292
Secchi E. R., Dalia Rosa L., Kinas P. G., Santos M. C. O., Zerbini A. N., Bassoi M., et al. (2001). Encounter rates of whales around the Antarctic Peninsula with special reference to humpback whales, Megaptera novaeangliae, in the Gerlache Strait: 1997/98 to1999/2000. Memoirs Queensland Museum 47, 571–578.
Seyboth E., Félix F., Lea M.-A., Dalla Rosa L., Watters G. M., Reid K., et al. (2021). Influence of krill (Euphausia superba) availability on humpback whale (Megaptera novaeangliae) reproductive rate. Mar. Mammal Sci. 37, 1498–1506. doi: 10.1111/mms.12805
Seyboth E., Groch K. R., Dalla Rosa L., Reid K., Flores P. A., Secchi E. R. (2016). Southern right whale (Eubalaena australis) reproductive success is influenced by krill (Euphausia superba) density and climate. Sci. Rep. 6, 28205. doi: 10.1038/srep28205
Siegel V. (1988). “A concept of seasonal variation of krill (Euphausia superba) distribution and abundance west of the Antarctic Peninsula,” in Antarctic Ocean and resources variability. Ed. Sahrhage D. (Springer-Verlag, Berlin).
Širović A., Hildebrand J. A. (2011). Using passive acoustics to model blue whale habitat off the Western Antarctic Peninsula. Deep-Sea Res. II 58, 1719–1728. doi: 10.1016/j.dsr2.2010.08.019
Širović A., Hildebranda J. A., Wiggins S. M., McDonald M. A., Moore S. E., Thiele D. (2004). Seasonality of blue and fin whale calls and the influence of sea ice in the Western Antarctic Peninsula. Deep-Sea Res. II 51, 2327–2344. doi: 10.1111/j.1748-7692.2008.00239.x
Skaret G., Macaulay G. J., Pedersen R., Wang X., Klevjer T. A., Krag L. A., et al. (2023). Distribution and biomass estimation of Antarctic krill (Euphausia superba) of the South Orkney Islands during 2011-2020. ICES J. Mar. Sci. 80, 1472–1486. doi: 10.1093/icesjms/fsad076
Sladen W. J. L. (1964). “The distribution of the Adélie and chinstrap penguins,” in Biologie antarctique. Eds. Carrick R., Holdgate M. W., Prevost J. (Hermann, Paris).
Smetacek V. (2008). “Are declining Antarctic krill stocks a result of global warming or of the decimation of the whales?,” in Impacts of global warming on Polar ecosystems. Ed. Duarte C. M. (Palacia de Marqués de Salamanca, Madrid, Spain: Fundaćion BBVA).
Smith M. E. K., Ososky J. J., Hunt K. E., Cioffi W. R., Read A. J., Friedlaender A. S., et al. (2024). Historical baleen plates indicate that once abundant Antarctic blue and fin whales demonstrated distinct migratory and foraging strategies. Ecol. Evol. 14, e11376. doi: 10.1002/ece3.11376
Smith T. D., Reeves R. R., Josephson E. A., Lund J. N. (2012). Spatial and seasonal distribution of American whaling and whales in the age of sail. PloS One 7, e34905. doi: 10.1371/journal.pone.0034905
Stammerjohn S., Massom R., Rind D., Martinson D. (2008). Regions of rapid sea ice change: an inter-hemispheric seasonal comparison. Geophysical Res. Lett. 39, L06501. doi: 10.1029/2012GL050874
Stephens P. A., Houston A. I., Harding K. C., Boyd I. L., McNamara J. M. (2014). Capital and income breeding: the role of food supply. Ecology 95, 882–896. doi: 10.1890/13-1434.1
Strycker N., Wethington M., Borowicz A., Forrest S., Witharana C., Hart T., et al. (2020). A global population assessment of the chinstrap penguin (Pygoscelis antarctica). Sci. Rep. 10, 19474. doi: 10.1038/s41598-020-76479-3
Sydeman W. J., Thompson S. A., Anker-Nilssen T., Arimitsu M., Bennison A., Bertrand S., et al. (2017). Best practices for assessing forage fish fisheries-seabird resource competition. Fisheries Res. 194, 209–221. doi: 10.1016/j.fishres.2017.05.018
Tamura T., Ichii T., Fujise Y. (1997). Consumption of krill by minke whales in Areas IV and V of the Antarctic. Paper SC/M97/17 submitted to the Scientific Committee of the International Whaling Commission. (Histon, Cambridge, UK: IWC Secretariat).
Taylor B. L., Chivers S. J., Larese J., Perrin W. F. (2007). Generation length and percent mature estimates for IUCN assessments of cetaceans. (La Jolla Shores Drive, La Jolla, CA: National Marine Fisheries Service, Southwest Fisheries Science Centre).
Thomisch K., Boebel O., Clark C., Hagen W., Spiesecke S., Zitterbart D., et al. (2016). Spatio-temporal patterns in acoustic presence and distribution of Antarctic blue whales Balaenoptera musculus intermedia in the Weddell Sea. Endangered Species Res. 30, 239–253. doi: 10.3354/esr00739
Tønnessen J. N., Johnsen A. O. (1982). The history of modern whaling (Oakland, California, USA: University of California Press).
Torres L. G., Brander S. M., Parker J. I., Bloom E. M., Norman R., Van Brocklin J. E., et al. (2023). Zoop to poop: assessment of microparticle loads in gray whale zooplankton prey and fecal matter reveal high daily consumption rates. Front. Mar. Sci. 10. doi: 10.3389/fmars.2023.1201078
Tort Castro B., Prieto González R., O’Callaghan S. A., Dominguez Rein-Loring P., Degollada Bastos E. (2022). Ship Strike Risk for Fin Whales (Balaenoptera physalus) off the Garraf coast, Northwest Mediterranean Sea. Front. Mar. Sci. 9. doi: 10.3389/fmars.2022.867287
Townsend C. H. (1935). The distribution of certain whales as shown by logbook records of American whale ships. Zoologica: Sci. Contributions New York Zoological Soc. 19, 3–50. doi: 10.5962/p.203715
Trathan P. N. (2023a). What is needed to implement a sustainable expansion of the Antarctic krill fishery in the Southern Ocean? Mar. Policy 155, 105770. doi: 10.1016/j.marpol.2023.105770
Trathan P. N. (2023b). The future of the South Georgia and South Sandwich Islands marine protected area in a changing environment: The choice between industrial fisheries, or ecosystem protection? Mar. Policy 155, 105773. doi: 10.1016/j.marpol.2023.105773
Trathan P. N., Brierley A. S., Brandon M. A., Bone D. G., Goss C., Grant S. A., et al. (2003). Oceanographic variability and changes in Antarctic krill (Euphausia superba) abundance at South Georgia. Fish. Oceanogr. 12, 569–583. doi: 10.1046/j.1365-2419.2003.00268.x
Trathan P. N., Fielding S., Hollyman P. R., Murphy E. J., Warwick-Evans V., Collins M. A. (2021). Enhancing the ecosystem approach for the fishery for Antarctic krill within the complex, variable and changing ecosystem at South Georgia. ICES J. Mar. Sci. 78, 2065–2081. doi: 10.1093/icesjms/fsab092
Trathan P. N., Fielding S., Warwick-Evans V., Freer J., Perry F. (2022a). Seabird and seal responses to the physical environment and to spatio-temporal variation in the distribution and abundance of Antarctic krill at South Georgia, with implications for local fisheries management. ICES J. Mar. Sci. 79, fsac168. doi: 10.1093/icesjms/fsac168
Trathan P. N., Hill S. L. (2016). Spatial aggregation of harvesting in Subarea 48.1, in particular during the summer and close to the coast. Working Group paper WG-EMM-16/17 submitted to CCAMLR WG-EMM-16. (Hobart, Australia: Commission for the Conservation of Antarctic Marine Living Resources). Available online at: https://meetings.ccamlr.org/en/wg-emm-16/17 (accessed November 21, 2024).
Trathan P. N., Murphy E. J. (2003). Sea surface temperature anomalies near South Georgia: Relationships with the Pacific El Niño regions. J. Geophysical Res. 108, 8075. doi: 10.1029/2000JC000299
Trathan P. N., Priddle J., Watkins J. L., Miller D. G. M., Murray A. W. A. (1993). Spatial variability of Antarctic krill in relation to mesoscale hydrography. Mar. Ecol. Prog. Ser. 98, 61–71. doi: 10.3354/MEPS098061
Trathan P. N., Ratcliffe N., Masden E. A. (2012). Ecological drivers of change at South Georgia: the krill surplus, or climate variability. Ecography 35, 983–993. doi: 10.1111/j.1600-0587.2012.07330.x
Trathan P. N., Reid K. (2009). Exploitation of the marine ecosystem in the sub-Antarctic: historical impacts and current consequences. Papers Proceeding R. Soc. Tasmania 143, 9–14. doi: 10.26749/rstpp.143.1.9
Trathan P. N., Warwick-Evans V., Hinke J. T., Young E. F., Murphy E. J., Carneiro A. P. B., et al. (2018b). Managing fishery development in sensitive ecosystems: identifying penguin habitat use to direct management in Antarctica. Ecosphere 9, e02392. doi: 10.1002/ecs2.2392
Trathan P. N., Warwick-Evans V., Young E. F., Friedlaender A., Kim J.-H., Kokubun N. (2022b). The ecosystem approach to management of the Antarctic krill fishery-the 'devils are in the detail' at small spatial and temporal scales. J. Mar. Syst. 225, 103598. doi: 10.1016/j.jmarsys.2021.103598
Trathan P., Warwick-Evans V., Young E., Thorpe S., Murphy E., Kelly N., et al. (2018a). Developing the risk assessment framework for the Antarctic krill fishery in Area 48. Working Group paper WS-SM-18/04 submitted to CCAMLR WG-EMM-18 and to CCAMLR WS-SM-18. Available online at: https://meetings.ccamlr.org/en/ws-sm-18/04 (accessed November 21, 2024).
Tulloch V. J. D., Plagányi É.E., Brown C., Richardson A. J., Matear R. (2019). Future recovery of baleen whales is imperiled by climate change. Global Change Biol. 25, 1263–1281. doi: 10.1111/gcb.14573
Tulloch V. J. D., Plagányi É.E., Matear R., Brown C. J., Richardson A. J. (2018). Ecosystem modelling to quantify the impact of historical whaling on Southern Hemisphere baleen whales. Fish Fisheries 19, 117–137. doi: 10.1111/faf.12241
Turner J., Holmes C., Caton Harrison T., Phillips T., Jena B., Reeves-Francois T., et al. (2022). Record low Antarctic sea ice cover in February 2022. Geophysical Res. Lett. 49, e2022GL098904. doi: 10.1029/2022GL098904
Van Parijs S., Clark C. W., Sousa-Lima R. S., Parks S. E., Rankin S., Risch D., et al. (2009). Management and research applications of real-time and archival passive acoustic sensors over varying temporal and spatial scales. Mar. Ecol. Prog. Ser. 395, 21–36. doi: 10.3354/meps08123
Vaughan D. G., Marshall G. J., Connolley W. M., Parkinson C., Mulvaney R., Hodgson D. A., et al. (2003). Recent rapid regional climate warming on the Antarctic Peninsula. Climatic Change 60, 243–274. doi: 10.1023/A:1026021217991
Veytia D., Corney S., Meiners K. M., Kawaguchi S., Murphy E. J., Bestley S. (2020). Circumpolar projections of Antarctic krill growth potential. Nat. Climate Change 10, 568–575. doi: 10.1038/s41558-020-0758-4
Videsen S. K. A., Simon M., Christiansen F., Friedlaender A., Goldbogen J., Malte H., et al. (2023). Cheap gulp foraging of a giga-predator enables efficient exploitation of sparse prey. Sci. Adv. 9, eade3889. doi: 10.1126/sciadv.ade3889
Vieira N. (2023). Whales Lost and Found: Rescuing a history of biodiversity loss in early modern Brazil. Exchanges Exchanges 10, 106–130. doi: 10.31273/eirj.v10i2.976
Viquerat S., Herr H. (2017). Mid-summer abundance estimates of fin whales Balaenoptera physalus around the South Orkney Islands and Elephant Island. Endanger. Species Res. 32, 515–524. doi: 10.3354/esr00832
Viquerat S., Waluda C. M., Kennedy A. S., Jackson J. A., Hevia M., Carroll E. L., et al. (2022). Identifying seasonal distribution patterns of fin whales across the Scotia Sea and the Antarctic Peninsula region using a novel approach combining habitat suitability models and ensemble learning methods. Front. Mar. Sci. 9. doi: 10.3389/fmars.2022.1040512
Warwick-Evans V., Constable A., Dalla Rosa L., Secchi E. R., Seyboth E., Trathan P. N. (2022c). Using a Risk Assessment Framework to spatially and temporally spread the fishery catch limit for Antarctic krill in the west Antarctic Peninsula: a template for krill fisheries elsewhere. Front. Mar. Sci. 9. doi: 10.3389/fmars.2022.1015851
Warwick-Evans V., Fielding S., Reiss C. S., Watters G. M., Trathan P. N. (2022a). Estimating the average distribution of Antarctic krill at the northern Antarctic Peninsula during austral summer and winter. Polar Biol. 45, 857–871. doi: 10.1007/s00300-022-03039-y
Warwick-Evans V., Kelly N., Dalla Rosa L., Friedlaender A., Hinke J. T., Kim J. H., et al. (2022b). Using seabird and whale distribution models to estimate spatial consumption of Antarctic krill to inform fishery management. Ecosphere 13, e4083. doi: 10.1002/ecs2.4083
Warwick-Evans V., Ratcliffe N., Lowther A. D., Manco F., Ireland L., Clewlow H. L., et al. (2018). Using habitat models for chinstrap penguins Pygoscelis Antarctica to advise krill fisheries management during the penguin breeding season. Diversity Distributions 24, 1756–1771. doi: 10.1111/ddi.12817
Watters G. M., Hinke J. T., Reiss C. S. (2020). Long-term observations from Antarctica demonstrate that mismatched scales of fisheries management and predator-prey interaction lead to erroneous conclusions about precaution. Sci. Rep. 10, 2314. doi: 10.1038/s41598-020-59223-9
Weinstein B. G., Double M., Gales N., Johnston D. W., Friedlaender A. L. (2017). Identifying overlap between humpback whale foraging grounds and the Antarctic krill fishery. Biol. Conserv. 210, 184–191. doi: 10.1016/j.biocon.2017.04.014
Werner T. B., Northridge S., Press K. M., Young N. (2015). Mitigating bycatch and depredation of marine mammals in longline fisheries. ICES J. Mar. Sci. 72, 1576–1586. doi: 10.1093/icesjms/fsv092
Whitehouse M. J., Meredith M. P., Rothery P., Atkinson A., Ward P., Korb R. E. (2008). Rapid warming of the ocean around South Georgia, Southern Ocean, during the 20th century: Forcings, characteristics and implications for lower trophic levels. Deep-Sea Res. I 55, 1218–1228. doi: 10.1016/j.dsr.2008.06.002
Williams R., Vikingsson G. A., Gislason A., Lockyer C., New L., Thomas L., et al. (2013). Evidence for density-dependent changes in body condition and pregnancy rate of North Atlantic fin whales over four decades of varying environmental conditions. ICES J. Mar. Sci. 70, 1273–1280. doi: 10.1093/icesjms/fst059
Young E. F., Thorpe S. E., Renner A. H. H., Murphy E. J. (2024). Environmental and behavioural drivers of Antarctic krill distribution at the South Orkney Islands: A regional perspective. J. Mar. Syst. 241, 103920. doi: 10.1016/j.jmarsys.2023.103920
Zerbini A., Adams G., Best J., Clapham P. J., Jackson J. A., Punt A. E. (2019). Assessing the recovery of an Antarctic predator from historical exploitation. R. Soc. Open Sci. 6, 190368. doi: 10.1098/rsos.190368
Zerbini A. N., Ajo A. F., Andriolo A., Clapham P. J., Crespo E., Gonzalez R., et al. (2018). Satellite tracking of Southern right whales (Eubalaena australis) from Golfo San Matías, Rio Negro Province, Argentina. Paper SC/67B/CMP/17 submitted to the Scientific Committee of the International Whaling Commission, La Jolla Shores Drive, La Jolla, CA.
Zerbini A. N., Andriolo A., Heide-Jørgensen M. P., Pizzorno J. L., Maia Y. G., VanBlaricom G. R., et al. (2006). Satellite-monitored movements of humpback whales Megaptera novaeangliae in the Southwest Atlantic Ocean. Mar. Ecol. Prog. Ser. 313, 295–304. doi: 10.3354/meps313295
Zitterbart D. P., Kindermann L., Burkhardt E., Boebel O. (2013). Automatic round-the-clock detection of whales for mitigation from underwater noise impacts. PloS One 8, e71217. doi: 10.1371/journal.pone.0071217
Keywords: CCAMLR, Antarctic krill fishery, baleen whale recovery, mitigation, resource competition
Citation: Trathan PN, Savoca MS, Friedlaender A, Baines M, Burkhardt E, Cheeseman T, Dalla Rosa L, Herr H, Secchi ER, Zerbini AN and Reisinger RR (2024) Integrating the needs of recovering populations of baleen whales into the revised management framework for the commercial fishery for Antarctic krill. Front. Mar. Sci. 11:1458042. doi: 10.3389/fmars.2024.1458042
Received: 02 July 2024; Accepted: 25 November 2024;
Published: 20 December 2024.
Edited by:
Maria Lourdes D. Palomares, University of British Columbia, CanadaReviewed by:
David Ainley, H.T. Harvey & Associates, United StatesEva Papaioannou, Helmholtz Association of German Research Centres (HZ), Germany
Copyright © 2024 Trathan, Savoca, Friedlaender, Baines, Burkhardt, Cheeseman, Dalla Rosa, Herr, Secchi, Zerbini and Reisinger. This is an open-access article distributed under the terms of the Creative Commons Attribution License (CC BY). The use, distribution or reproduction in other forums is permitted, provided the original author(s) and the copyright owner(s) are credited and that the original publication in this journal is cited, in accordance with accepted academic practice. No use, distribution or reproduction is permitted which does not comply with these terms.
*Correspondence: Philip N. Trathan, cC50cmF0aGFuQHNvdG9uLmFjLnVr
†ORCID: Philip N. Trathan, orcid.org/0000-0001-6673-9930