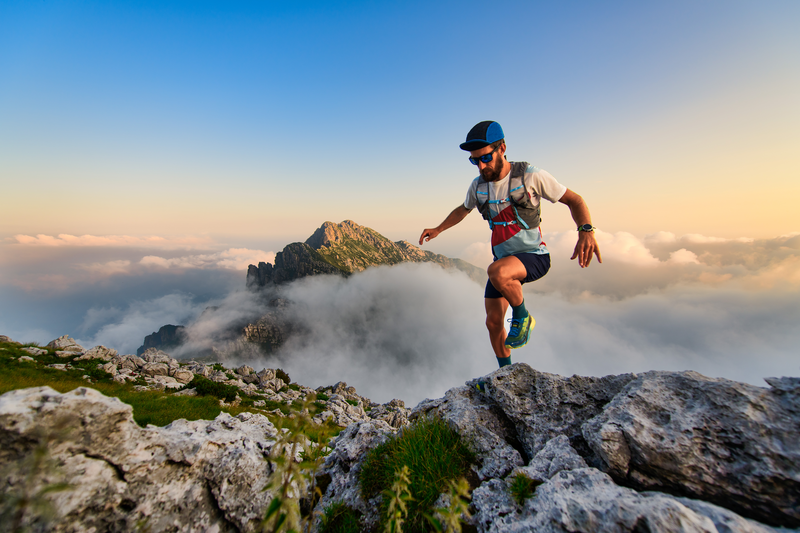
95% of researchers rate our articles as excellent or good
Learn more about the work of our research integrity team to safeguard the quality of each article we publish.
Find out more
REVIEW article
Front. Mar. Sci. , 26 September 2024
Sec. Marine Fisheries, Aquaculture and Living Resources
Volume 11 - 2024 | https://doi.org/10.3389/fmars.2024.1455905
This article is part of the Research Topic Alternative Feed Ingredients and their Functional Properties in Aquaculture View all 10 articles
Today’s increasing demand for aquaculture production is accompanied by various challenges such as diseases, broodstock improvement, domestication, development of suitable pellets and feeding methods, hatchery technology, and water quality management. Thus, probiotic usage has been reported as the ideal alternative to antibiotics, other chemotherapeutics, and additional supplements to other alternative ingredients. The main beneficial roles of probiotics include the enhancement of disease and stress resistance, immunity, promotion of growth and reproduction, improvement of digestion, provide several nutrients, and enhancement of water microbial composition. To guarantee safety, the probiotics provided must be non-invasive and non-pathogenic. The use of probiotics in aquaculture, either directly or in combination with alternative materials such as plant protein diets, vitamins, microalgae, fermented products, and so on, has been shown to improve the health and growth of aquatic animals and offer significant benefits to the sustainability of the industry. There is advocacy for a systematic approach to conducting innovative research to unearth new putative strains, which is substantial in ensuring sustainable probiotic usage and, thus, can help in the continuous development of the aquaculture industry especially in China. Some examples of the probiotics found in China are mainly photosynthetic bacteria (PSB) which are autotroph bacteria capable of photosynthesis, antagonistic bacteria (Pseudoalteromonas sp., Flavobacterium sp., Alteromonas sp., Phaeobacter sp., Bacillus sp., etc.), bacteria that contribute nutrients and enzymes during digestion (lactic acid bacteria, yeasts, etc.), bacteria that improve water quality (nitrifying bacteria, denitrifying bacteria, etc.), Bdellovibrio, and other probiotics. This review also focuses on the potential use of probiotics in aquaculture, especially in China, and probiotics’ prospective future role.
Over the past few years, global fish aquaculture production has increased rapidly, reaching 82 million tons worth USD 250 billion in 2018 from less than 1 million tons in the early 1950s. Aquaculture’s contribution to global fish production increased from 25.7% in the year 2000 to 46% in 2018 (Figure 1, FAO, 2023). Global food fish consumption has also increased at twice the rate of world population growth per year and is higher than other animal protein types (FAO, 2020). It can be concluded from the data obtained that global fish consumption is increasing faster than the average growth of 5.3% per year. Therefore, to meet the rapidly growing demand, aquaculture production practices must be intensified and developed to a higher level in terms of technology and practice (Tuan et al., 2013).
Figure 1. World aquaculture production from 1991–2020 (FAO, 2023).
The word “probiotic” is derived from the Latin word “pro” which means “for” and the Greek word “biotic” which also means “life.” Lilly and Stillwell used the word in 1965 to describe chemicals that stimulate microbial growth. The FAO/WHO (2001) defines probiotics as beneficial living microorganisms that, when taken effectively, enhance host health. They achieve this by modifying the microbial populations associated with the host. Probiotics have been demonstrated to improve nutrition, feed utilization, and enhance immunity, ultimately increasing disease resistance. Lazado and Caipang (2014) proved the practical advantages of probiotics in aquaculture in 1986.
According to statistics released in October 2023, China accounted for about 35% of the global aquatic product production volume in 2021, making it the world’s largest producer of aquatic products. The consumer market for aquatic products surpassed the total retail market revenue of USD 80 billion. The production volume of aquatic products have increased rapidly over the years, especially in aquaculture which while recording an estimated production volume of 4.5 million tons in 1980 increased its production to 69 million tons as of 2022. Meanwhile, the production of capture fisheries has been relatively stable at 13 to 15 million tons in same year. Therefore, considering China as the largest consumer, the fisheries development plan published in January 2022 seeks to further increase aquaculture production in quality and quantity while reducing the volume of wild fish catch by the year 2025 and probiotics application is to play critical role.
The increasing demand for aquaculture production today is accompanied by many challenges, such as diseases and epizootics, improvement of broodstock and domestication, development of compliant pellets and feeding mechanisms, hatchery and rearing technologies, water quality management by increasing intensification and commercialization of aquaculture production. Epizootic Ulcerative Syndrome (EUS) is a seasonal epizootic disorder that affects various species cultivated in freshwater and brackish water fish, with a complicated etiology of infection. The fungus of the genus Aphanomyces is the cause of EUS, and according to current epidemiological data, the disease can be transmitted by water and, in some cases, the movement of fish without sufficient quarantine and health certification. Of all the problems facing farmers, disease outbreaks are a major challenge factor hindering the economic and social development of the aquaculture sector in many countries (Qi et al., 2009; Tuan et al., 2013). Over the past decades and even today, antibiotics have often been used to control and prevent disease, improve growth, and aid feed efficiency performance.
The use of antibiotics or antimicrobials in 2020 was noted to reach 99,502 tons (95% CI: 68,535-198,052) and has been projected to increase in 2030 by 8.0% to 107,472 tons (95% CI: 75,927-202,661) based on the current trends. Most of the use of antimicrobials is concentrated in Asia (67%), while less than 1% is in Africa. These findings indicate an increase in global antimicrobial use by 2030 compared to previous projections based on 2017 data, likely due to revisions to antimicrobial use in Asia/Oceania (~6,000 tons) and the Americas (~4,000 tons) (Mulchandani et al., 2023). This enormous usage of antibiotics imposes selection pressure on resistance among bacteria, which have adapted to this situation, especially with horizontal and unclear resistance gene flow. The resistance mechanisms may be established by chromosome acquisition, and chromosomal modification cannot be transmitted to the other bacteria. Some bacterial pathogens can develop resistance mediated by plasmids (Bondad-Reantaso et al., 2005; FAO, 2020).
Probiotics and antibiotics have quite different mechanisms of action, and most antibiotics can only treat diseases but do not necessarily overcome the basic problems that occur. The FAO/OIE/WHO (The Food and Agriculture Organization of the United Nations/Office International des Epizooties/World Health Organization) expert meeting on antimicrobial resistance in its application in aquaculture in 2017 concluded that two main hazards could be caused by antimicrobials, namely antimicrobial residues and antimicrobial resistance. While probiotics have numerous modes of action, they play an essential role in maintaining the health of aquatic organisms (Balcazar et al., 2006; Banerjee and Ray, 2017; Schar et al., 2020).
Probiotics can be used to enhance growth, improve feed utilization, strengthen immune function, and improve water quality in aquaculture (Tabassum et al., 2021). Figure 2 illustrates the graphical representation of this review. Briefly, the figure describes the application of probiotics in the scope of aquaculture. Generally, there are three methods used in the application of probiotics to aquatic animals, and these include the addition via supplemented pellets, addition directly to the water column in which the fish live, and addition directly via injection to the host. According to Lauzon et al. (2014), the best way to give probiotics to cod farming is by adding them directly to the water column, which is the only method that can be applied to all stages of fish. One is bound to encounter several limitations when probiotics are added and fed via supplemented pellet/food, which live in the early larval stage of fish since the fish’s digestive tract at that stage is not matured enough to aid in proper digestion. As for the method of addition directly via injection to the host, it is feared that it will increase stress, affecting fish development; thus, the most recommended method is when added directly to the water column (Sveinsdottir et al., 2009).
The primary benefits for aquatic animals after probiotic administration can be separated into two groups such as aquatic microbes (nitrification, denitrification, water quality, and pathogen) and gut microbes (disease and stress resistance, improvement of digestion, promotor of growth, promotor of reproduction, immune function, and source of nutrients) as shown in Figure 2. The active components of probiotics, encompassing antagonistic compounds (which provide anti-viral, anti-biofilm, anti-virulence, anti-quorum sensing, anti-bacterial, anti-fungal, anti-inflammatory and siderophore effects), are presented clearly, providing information regarding the mechanisms underlying the positive effects of probiotics in maintaining the balance of aquatic ecosystems and increasing disease resistance. So, the figure illustrates the great potential of probiotics in stimulating sustainable growth and improving fish health in aquaculture, highlighting the importance of sustainable research and development in this area to support the sustainability of the aquaculture industry in future.
According to Jahid et al. (2015), pathogens induce several infections that can benefit from quorum sensing (QS) processes through regulation based on cell density. Quorum quenching (QQ) is an effective and promising bio-control tool because it disrupts QS, preventing and controlling infections. Acyl homoserine lactones (AHLs), which are autoinducers (AI), are reported to act as the key QS signal molecules in gram (negative) bacteria, thus helping in coordinating gene expression to activate several processes, including the formation of biofilm and the production of virulence factors in some pathogens. In prior studies conducted by Eickhoff and Bassler (2018), AHL lactonase AiiK has been identified with diverse features and the construction of a pELX1 constitutive expression vector to forward heterologous protein expression in Lactobacillus casei MCJΔ1. Based on research conducted by Dong et al. (2020), recombinant strains of pELCW-AiiK/L. casei MCJΔ1 (LcAiiK) together with wild Aeromonas hydrophila have been co-cultured to test LcAiiK’s ability to perform quorum quenching on A. hydrophila. An expression vector related to the cell wall, pELCW, has been designed for L. casei MCJΔ1. The results of localization testing showed that the expressed AiiK was connected with the LcAiiK surface via the pELCW-aiiK vector. At OD600 = 0.5, LcAiiK was noted to reduce the concentration of 24.13 μM C6-HSL within 2 hours, 40.99 μM C6-HSL within 12 hours, and 46.63 μM C6-HSL within 24 hours. Over 50% of LcAiiK cells successfully retained the pELCW-aiiK plasmid after undergoing 15 generations of cultures without erythromycin. In addition, LcAiiK was an inhibitor of swimming motility, extracellular proteolytic activity, hemolytic activity, and biofilm formation of A. hydrophila AH-1 and AH-4. AHL lactonase AiiK was expressed first and constitutive in the surface layer of L. casei MCJΔ1. LcAiiK demonstrated significant AHL lactonase activity as well as a great ability to perform QQ of A. hydrophila AH-1 and AH-4, by reducing their QS process without killing them. Based on the results of this study, AHL lactonase AiiK showed very promising potential to control pathogenic bacteria A. hydrophila by interfering with the QS mechanism. LcAiik was also known to successfully reduce the production of compounds involved during QS, such as C6-HSL, as well as inhibitors of the biological activity of A. hydrophila. In addition, AiiK was able to also constitutively express on the surface layer of L. casei MCJΔ1. The results suggested that LcAiiK showed potential as an alternative in developing anti-pathogenic drugs or bio-control agents that can be used to overcome pathogenic bacterial infections in the aquaculture industry. However, there is a need for further studies to confirm the effectiveness and safety of using LcAiiK in the context of practical applications in the field.
As summarized in Figure 3, QS plays an active role against bacteria because when the Autoinducer is in a low-density state, it will not show any effect. On the contrary, in high-density conditions, it will interact with QS receptors, which will then help with biofilm formation, virulence factors, and antibiotic resistance. QQ plays a role in the host (aquatic organisms), where it will block the signal repression and all the steps of QS activity to help no bacteria resistance to occur, thus inhibiting the growth of pathogenic bacteria, and promoting the growth of the good bacteria (competitors). This review summarizes the development and potential of probiotics in aquaculture.
Figure 3. The mechanism and application of quorum sensing (QS) and quorum quenching (QQ) in bacteria.
In aquaculture, probiotics must have antimicrobial activity and be safe for the host, aquatic environment, and humans. Microorganisms to be used as probiotics must meet the several standard criteria set in order to be considered. There are several characteristics to consider in the selection of probiotics, namely host origin, strain safety, antimicrobial substance production, ability to modulate host immune response, and efficient competition with pathogens at an intestinal mucosal adhesion site (Balcazar et al., 2006; Perez-Sanchez et al., 2014). Merrifield et al. (2010) advocated increasing the list of probiotic requirements such that if additional probiotic species exhibit more established features, the suitable species would be considered more effective. It is very difficult to find a probiotic candidate with all these criteria, so several types of probiotics will be used simultaneously with prebiotics, symbiotics, and other alternate additives (Patterson and Watts, 2003). The simultaneous application of several types of probiotic candidates and even prebiotics is expected to produce greater and more targeted benefits than the application of probiotics individually. Figure 4 (modified from the works of Balcazar et al. (2006)) illustrates the selection of probiotics as a biocontrol agent as well as its development for commercial use in aquaculture. Table 1 also provides concise information on some of the probiotics used in aquaculture.
Based on intensive research previously conducted (Ibrahem, 2015), it is clear probiotics play an important role in animals, including fish. Banerjee and Ray (2017) complement this by showing that through several studies, the beneficial effects of probiotics on the host are highly visible, considering the positive impacts of probiotics on bacterial, fungal, and viral diseases in fish. Probiotic bacteria selections for aquaculture include the use of in vitro and in vivo methods. The in vitro screening methods involve assessing isolated probiotic strains obtained from healthy fish species and running some tests such as auto-aggregation, cell surface hydrophobicity, biochemical tests (e.g., DNase activity, hemolytic activity, sorbitol, mannitol, Simon’s citrate, Vorges-Proskauer (VP), biofilm production/formation), bacterial growth in various media (e.g., Luria-Bertani (LB), Tryptic Soy Broth (TSB)), biosafety screening using a challenge model, tolerance of isolates to bile salts and high temperatures, and pathogen inhibition. Blood hemolysis, which is an indicator of the activity of pathogens such as Aeromonas spp. and Streptococcus spp. in the digestive tract of fish, can be caused by virulence genes that produce hemolysin and aerolysin (Abdel-Latif et al., 2020). Such hemolytic activity can be evaluated using different blood types, including human, horse, sheep, etc... (Nayak and Mukherjee, 2011). On the other hand, in vivo characterization involves the assessment of the isolates, such as their ability to enhance growth performance, feed utilization, increase immune and antioxidant enzyme activities, their effects on the whole body or muscle proximate chemical composition, effects on intestinal morphology, effects on hematological parameters, gene expression, and others (Figure 4). So it can be concluded that the results of fish health screening during outbreaks using both methods (in-vitro and in-vivo) can provide an understanding of various probiotic properties that might be significant for maintaining the health and performance of the aquatic animal, especially when employed commercially.
Probiotic candidates should be derived from healthy individuals and preferably directly from the target species in endogenous probiotic selection protocols (Carnevali et al., 2004). Studies have shown that feeding rainbow trout supplemented with Lactobacillus sp. can result in higher survival rates of 97.8% to 100%, compared to 65.6% for those not treated (Balcazar et al., 2007; Vendrell et al., 2008; Perez-Sanchez et al., 2011). The probiotic candidates to be used should be harmless to the host and have beneficial effects. In order to guarantee safety, the probiotics provided must be non-invasive and non-pathogenic. Furthermore, they must exhibit metabolic activity to provide an impact, maintain viability during manufacturing processes (a critical prerequisite for commercial manufacture), and demonstrate resilience during storage.
Probiotic selection commonly involves in vitro antagonism tests where candidate probiotics or their extracellular products are exposed to pathogens in either liquid (Sotomayor and Balcazar, 2003; Vine et al., 2004a) or solid (Chythanya et al., 2002) mediums. A previous study identified and characterized several probiotic species based on in vitro evaluation techniques. For example, Bacillus species such as B. tequilensis GPSAK2, B. velezensis GPSAK4, and B. subtilis GPSAK9 with NCBI (National Center for Biotechnology Information) GenBank accession numbers MW548630, MW548635, and MW548634, respectively, have been identified and characterized (Amoah et al., 2021a). Despite the prevalence of in vitro activity assessments in agar well-diffusion assays and broth cultures, Gram et al. (1999) cautioned against using them to predict in vivo effects. For instance, the in vitro antagonism of Pseudomonas fluorescens (strain AH2) against Aeromonas salmonicida does not necessarily translate to protection against furunculosis in Atlantic salmon but proves effective as a probiotic in rainbow trout, providing defense against vibriosis (Gram et al., 2001). It is vital to consider the origin (preferably strains isolated from the host), safety (non-pathogenicity), and the strain’s ability to survive the host’s gastrointestinal tract (GIT) transit (e.g., resistance to bile salts, low pH, and proteases). The ability of microorganisms to efficiently adhere to intestinal epithelial cells, reducing or preventing pathogen colonization, is often a key criterion in selecting potential probiotics (Vine et al., 2004a). In addition to adherence, potential probiotics must demonstrate beneficial effects such as enhanced nutrition and increased immune response within the host. Finally, a viable probiotic should not only meet these criteria but also be capable of surviving under standard storage conditions and be technologically suitable for industrial processes (e.g., lyophilization).
The evaluation of candidate probiotics necessitates in vivo testing, where candidate species are introduced to the host in a controlled setting, and subsequent monitoring involving the assessment of parameters such as growth, colonization (especially in the gut), survival, and physicochemical aspects are conducted (Vine et al., 2004b) (Figure 4). When seeking to control the host’s microbiota biologically, the appropriate tool for assessing the potential impact of probiotics is standard in vivo assessment using high-throughput sequencing technologies. For potential probiotics to be considered effective, they must demonstrate beneficial effects within the host organism, such as enhanced nutrition and an increased immune response.
Regarding pathogen inhibition or disease resistance, probiotics produce several compounds such as bacteriocin, siderophores, lysozyme, proteases, and hydrogen peroxide, which then aid against pathogen growth. Although Bacillus subtilis in vegetative and spore form has been shown to inhibit pathogens under in vivo conditions in various fish species, it is uncertain whether this activity is solely due to the principle of competitive exclusion or is the result of multiple mechanisms acting simultaneously, including competition for adhesion sites, essential nutrients, antimicrobial inhibition, and enhancement of host immune responses. In addition, probiotics in synbiotic form can also promote the rapid reproduction of probiotics in the host body and provide beneficial effects (Nayak, 2021).
Banos et al.’s (Banos et al., 2019) research involving Enterococcus faecalis UGRA10 has been shown to significantly inhibit Lactococcus garvieae in vivo and reduce mortality in Rainbow trout. The continuous treatment of UGRA10 promoted colonization in the GIT while protecting against pathogen invasion. In addition, enterocin AS-48 displayed anti-lactococcal action in vivo, lowering the pathogen’s fatal effects when administered via immersion or intraperitoneal injection. These findings further emphasize the potential of sustainable enterocin AS-48 bath treatments as a viable therapy for controlling lactococcosis in Rainbow trout, using a simple approach to generate semi-purified AS-48 from inexpensive, food-based substrates. Although the specific role of AS-48 in the preventative action of UGRA10 remains unclear, its potential synthesis within the gut of animals, given this injection, indicates a major contribution to the prevention of lactococcosis.
Probiotics have several mechanisms of action, though the manner in which they employ their effects is still not completely clarified. These mechanisms range from producing bacteriocin and short-chain fatty acid, lowering gut pH, and competing nutrients to stimulate mucosal barrier function and immunomodulation. There are several hypotheses on the mode of action of probiotics in host organisms. Some of these procedures are observed in vitro, but the efficiency of probiotics performed in vitro may change significantly upon administration to the host in its natural environment. Factors affecting probiotic organisms include unique feeding (Balcazar et al., 2006), manipulation of the intestinal tract (Vine et al., 2006), and more complex microbial interactions and/or the essential chemical ecosystem. These factors can serve as benchmarks in the success and failure of probiotics’ natural physiology.
Some of the mechanisms of action of probiotics that are commonly used in aquaculture, suggesting probiotics’ beneficial effects include (i) competitive exclusion of pathogenic bacteria; (ii) enhancement of host nutrition and enzymatic contributions to digestion; and (iii) stimulation of host immune responses (Irianto and Austin, 2002; Gomez and Balcazar, 2008; Merrifield et al., 2010) (Figure 5). As explained in Figure 4, probiotics can have many positive impacts on the aquaculture environment, including increased growth, improved health and quality of aquatic organisms, and increased overall production efficiency which can be achieved by the following: (I) Probiotics need competitive exclusion against pathogens by occupying intestinal surface areas or mucus glands in aquatic organisms, thus becoming a barrier for pathogens to live and multiply; (II) Probiotics must compete directly with pathogenic bacteria to produce nutritional resources in their environment, for example water-soluble or water-insoluble nutrients that are present so that pathogens will have difficulty in growing; (III) Contribute to modifying microbial populations in their environment (water), thereby increasing the number of beneficial microbes while suppressing harmful microbes; (IV) Act as a breaker of complex organic compounds (feed waste and organism carcasses) so as to improve water quality from pollution; (V) Be a stimuli of the immune response of aquatic organisms, thereby increasing resistance to disease and stress to the environment by activating immune cells or modulating the immune response that stimulates the production of anti-inflammatory cytokines.
The mode of action in probiotics is multifactorial or complex and is very difficult to explain with certainty. So some of the recommended modes of action of probiotics for aquatic organisms are the production of inhibitory compounds, competing for available chemicals or energy, competing for attachment sites, inhibition of virulence gene expression or disturbance in quorum perception, improvement of water quality, improved immune response, macro and/or micronutrient sources, and enzymatic contribution to digestion. Tinh et al. (2008), in a more detailed analogy, explained that the mechanism of application of probiotics in aquatic organisms, include having better resistance to disease, growth performance, better use of feed, carcass composition, gastric morphology, reduction of abnormalities, intestinal colonization, and modulation of microbes, although not always fully aware of the exact mode which works at a particular time.
The primary sources of probiotics in aquaculture often involve strains, such as Lactic Acid Bacteria (LAB) and Bacillus spp., isolated from natural aquatic environments or specific host organisms.
Numerous studies have investigated the potential of probiotics in aquaculture. Research by Sotomayor and Balcazar (2003) and Vine et al. (2004a) emphasizes the importance of in vitro antagonism tests in selecting probiotic strains that can effectively inhibit pathogenic organisms. Chythanya et al. (2002) have explored the efficacy of probiotics, particularly in controlling diseases in aquaculture settings. Gram et al. (2001) demonstrated the strain-specific nature of probiotic effects, emphasizing the need for careful selection based on host and pathogen specificity.
Most probiotic strains are ineffective in providing the effects aquatic organisms’ need, as they come from non-fish sources. There have not been many studies on the potential of probiotics isolated from the host. A previously conducted study investigated the possibility of bacterial species isolated from the digestive tract of hybrid groupers (Epinephelus fuscoguttatus♀ × Epinephelus lanceolatus♂) and the isolation procedure included the ability of the isolates to survive in high bile salt concentrations, low pH, high temperatures, as well as their adhesion ability (auto-aggregation and cell surface hydrophobicity), antimicrobial activity and safety, compatibility among the three isolates to be used as multispecies, hemolytic activity, and antibiotic susceptibility tests (Amoah et al., 2021b). After identification and undergoing various types of procedures, the results obtained showed that the Bacillus strains GPSAK2, GPSAK4, and GPSAK9 strains can be one of the potential probiotic alternative candidates that can be used in efforts to improve the growth and health status of aquatic animals, especially in grouper fish species since their in vivo experiments showed better effects on fish (Amoah et al., 2023). Similarly, a recent work by the same author isolated and characterized four new Bacillus probiotic strains, namely, B. velezensis strain PGSAK01 (accession number OQ726606), B. stercoris strain PGSAK05 (accession number OQ726607), B. velezensis strain PGSAK17 (accession number OQ726601), and B. subtilis strain PGSAK19 (accession number OQ726605), based on their morphological characteristics, biochemical properties, and 16S rRNA sequencing homology analysis (Amoah et al., 2024).
Another study by Coulibaly et al. (2023) also dealt with the isolation of probiotic strains (lactic acid bacteria, LAB) from the intestines of nile tilapia (Oreochromis niloticus). In the study, there were 12 LAB strains, including the genera Pediococcus (P. acidilactici and P. pentosaceus) and Lactobacillus (L. plantarum), with the predominance of P. acidilactici identified via morphological and 16S rDNA gene sequence with the results revealing that all LAB isolates showed high antagonistic activity against bacterial pathogens such as Escherichia coli, Klebsiella pneumoniae, Pseudomonas aeruginosa, Proteus mirabilis, and Staphylococcus aureus. In addition, when tested with hexane, xylene, and chloroform, the isolates showed various degrees of cell surface hydrophobicity and were noted to have the ability to produce both lipase and β-galactosidase enzymes. The effectiveness of cryoprotective agents is isolate-dependent, with a high affinity for D-sorbitol and sucrose. So, based on all the characteristics shown, the LAB was noted as revealing promising probiotic properties that could be used in the aquaculture industry.
Furthermore, studies by Vine et al. (2004b) said that probiotics enhance the immune response of aquatic organisms in unstable water environments, contributing to a more robust defense against pathogens. The selection of probiotics in aquaculture also considers factors such as the ability of strains to adhere to mucosal surfaces and survive under environmental conditions, as highlighted in research by Vine et al. (2004a) and Chythanya et al. (2002). The sources of probiotics in aquaculture are diverse, often involving beneficial bacteria derived from natural aquatic environments. The research referenced contributes to understanding of how these probiotics can be effectively utilized to enhance the health and performance of aquatic organisms.
Several reports have highlighted the beneficial roles of probiotics, including promoting disease and stress resistance, promoting growth, improving digestion, promoting reproduction, enhancing immunity, providing several nutrients, and enhancing the water microbial composition (Table 2). This section dealt deeply with the beneficial effects of probiotics.
The interaction between fish and their environment is much more complex than that of terrestrial animals. According to Irianto and Austin (2003), microbial interaction with its host does not only occur in the digestive tract but also the gills, skin, or environment. Probiotics that are administered toward the suitable target will play an important role in improving host growth (Table 3). This happens because probiotic bacteria act as microbial balance controllers in the digestive tract, enhancing the absorption of feed nutrients and improving the nutritional value of feed (Hasyimi et al., 2020).
Table 3. Type of different probiotics that have the potential to tackle the disease of aquatic organisms.
According to Agustina et al. (2018), the in vitro bacterial inhibition test stage analysis refers to the diameter of antibiotic inhibition. Staphylococcus edaphicus is one of the potential probiotics that can increase the immunity component in Kelabau fish (Osteochilus melanopleurus) after a challenge test with bacteria A. hydrophila AH-1 and Pseudomonas sp. PS-1. The best results showed that the addition of intestinal bacteria isolates (Staphylococcus edaphicus) can increase the survival rate by 86.67% in fish compared to control 56.67%, hemoglobin content of 6.75 ± 0.25 g/dL as against the control group with 4.58 ± 0.52 g/dL, hematocrit content 16.67 ± 1.53% as against the control group with 6.83 ± 0.76%, total erythrocytes of 1.35 ± 0.07×106 cells/mm3 as against the control group with 0.97 ± 0.07×106 cells/mm3, and total leukocytes of 9.90 ± 0.20×104 cells/mm3 as against the control group with 12.01 ± 0.54×104 cells/mm3. This proves that giving Staphylococcus edaphicus to fish can inhibit the proliferation of pathogenic bacteria to support the survival rate of fish. After finding probiotic candidates in Kelabau fish, probiotics can be optimized as a safer way to overcome red spot disease in fish. Interestingly, previously conducted research by Istiqomah et al. (2019) showed that Staphylococcus sp. strain JC20 could also be a potential aquaculture probiotic. After isolating and characterizing the bacterial strain Staphylococcus sp., it was proven to have strong cellulolytic activity and had the potential to be used as a candidate probiotics for fish. In this study, the isolate was obtained with high similarity (>99.7%) to Staphylococcus spp., and while showing no pathogenic properties in red tilapia, also showed susceptibility to all the four antibiotics tested (oxytetracycline, kanamycin, ampicillin, and rifampicin). Bacteria with antibiotic-resistant gene traits also need to be avoided because they are at risk of gene transfer to other microorganisms in the fish farming environment, which can have a detrimental impact.
Recently, gut microflora has attracted substantial interest due to its role in structure formation. It is reported to form a defense barrier to protect the host from pathogen invasion by enhancing the immune system (Ramirez and Dixon, 2003; Claus et al., 2017). In this regard, probiotics have been confirmed to mainly generate antibacterial agents by altering the gut microflora to inhibit the growth activity of other microorganisms (Spinler et al., 2008; Miao et al., 2018). Amoah et al. (2021b) found a significantly lower relative abundance of supposedly pathogenic genera such as Enterovibrio and Shewanella and a higher relative abundance of professedly beneficial genera such as Ruegeria and Lactobacillus after the supplementation of B. coagulans ATCC 7050, B. licheniformis ATCC 11946, and Paenibacillus polymyxa ATCC 842 in fish diet.
Previously conducted research by Afrilasari et al. (2016) using gnotobiotic fish (with antibiotic treatment) and normal fish (without antibiotic treatment) after a 30 day culture experiment revealed an enhancement in the protease and amylase enzyme activity, as well as specific growth rates in the treatment group supplemented with basal feed and 1% probiotics compared to other treatments. B. megaterium PTB 1.4 was noted to aid in increasing the activity of digestive and growth enzymes in catfish. Probiotic supplementation enhanced the digestive enzymes to help fish use and digest feed nutrients properly, unlike the results obtained in the gnotobiotics (gnotoplus) (Bairagi et al., 2002). The high digestive enzyme activity witnessed in the treated group was presumed to result from the probiotic bacteria’s ability to stimulate the synthesis of endogenous digestive enzymes produced by fish. Probiotics can increase digestive enzymes by stimulating the synthesis of endogenous enzymes in the digestive tract (Mohapatra et al., 2012).
Information obtained by Gao et al. (2014) suggests the microbes in the gut of A. japonicus are complex. In this study, 188,623 optimized readings were taken from ten intestinal content samples and four surface sediment samples using the 454-pyrosequencing technique. The number of readings of each sample varied widely, ranging from 9,606 to 16,030, with an average of about 13,473 ± 2,181 readings. The method used is 16S-based molecular microbiology, and the results obtained show significant success. Potential probiotics were found in the gut of A. japonicus, including sequences associated with Bacillus, LAB (Lactobacillus, Lactococcus, and Streptococcus), and Pseudomonas. In addition, there are characteristic differences among bacterial communities in the contents of the anterior intestine, posterior intestine, and surrounding sediments. So, selective feeding of A. japonicus is thought to be the main factor affecting the composition of bacteria in the anterior stomach contents and surrounding sediments.
Based on some of these research results, it can be proven that probiotic bacteria play an important role in increasing digestive activity and health of aquatic animals. One of them is by optimizing the work of intestinal microflora and the activity of digestive enzymes. Therefore, applying sustainable probiotics is claimed to be a very effective strategy for increasing the productivity and welfare of the aquaculture industry.
Feeding in aquaculture is extremely costly, so many cultivators are looking for ways to reduce the cost by increasing the growth rate of aquaculture fish. There are many types of commercial probiotics, such as EM4 (Effective), containing a mixture of fermented microorganisms, lactic acid bacteria (Lactobacillus casei), and yeast (Saccharomyces cerevisiae) (Ardita et al., 2015). As has been reported by Anis and Hariani (2019), EM4 administration showed a significantly high effect on the specific growth rate (SGR), feed conversion ratio (FCR), and survival rate (SR) of catfish with the best treatment showing SGR values of 5.91 ± 0.04%, FCR values of 0.88 ± 0.05, and SR value of 73.50 ± 1.91%. In addition, Noor and Pakaya (2018) have also reported that administering EM4 with a dose of 3 mL shows more optimal results on the growth rate of carp fish (Cyprinus carpio).
The addition of probiotic bacteria (white fungus powder/Agaricus bisporus) (WBMP) to the feed of rainbow trout (Oncorhynchus mykiss) for 8 weeks showed an enhancement in the total protein levels and lysozyme activity in skin mucus of trout given 1% or 2% WBMP. In contrast, no significant difference was observed for those fed 0.5% WBMP when compared to the control group. The electrophoresis results of polyacrylamide dodecyl sulfate sodium gel showed changes in the protein profile of skin mucus after WBMP feed. Molecular studies showed a significant increase in short-threaded RNA tumor necrosis factor (TNF)-α in the intestines of WBMP-fed trout, regardless of the degree of inclusion. In addition, fish receiving 1% or 2% WBMP treatment significantly increased interleukin (IL)-1β expression compared to the control group. Similarly, intestinal IL-8 expression was improved with 1% and 2% WBMP treatments, whereas no significant difference was found between the control group and the 0.5% WBMP treatment group regarding the IL-8 gene expression (Amiri et al., 2018).
Aquaculture production is greatly influenced by the reproduction process, which is regulated by many factors such as fish species, nutrients, and water environment. Research in recent years has focused on probiotics’ role in reproduction, with special emphasis on aquatic organisms (Ibrahem, 2015). Research conducted by Ghosh et al. (2004) tested incorporating Bacillus subtilis isolated from the gut of Cirrhinus mrigala, into the feed of four ornamental fish species for one year in a feeding experiment. The results obtained showed an increase in gonadosomatic index (GSI), fecundity, viability, and fry production in the female of the the fish species tested. The use of B vitamins synthesized by probiotics, especially vitamins B1 and B12, contributed to reducing the number of dead or deformed alevins. Thus, probiotics used as food additives appear to promote overall health benefits including reproduction for the host.
Lombardo et al. (2013) investigated the effects of feeding Lactobacillus rhamnosus IMC 501 on the growth and survival of offspring obtained from probiotic-fed Fundulus heteroclitus. The examined larvae showed improvements in gonadal growth, fecundity, embryo survival, and hatching rate, but no significant changes were detected in other aspects. In the hatching process, the lymphoid system is still developing, unlike what occurs in adult fish, and no organization or functional capabilities are apparent. Thus, whether or not fish embryos are capable of initiating a complete immune response, it is unknown at this time, but previous evidence has shown that 1 dpf (days post fertilization) carp (Cyprinus carpio) embryos respond to microinjection of bacterial lipopolysaccharide by increasing levels of interleukin transcripts (Rombout et al., 2005), and transcription of genes involved in the innate immune response induced in zebrafish embryos (Watzke et al., 2007). In addition, maternally derived immune components in eggs, embryos, and hatchlings have been found in rainbow trout (O. mykiss) (Lovoll et al., 2006) and killifish (Hunt and Rice, 2008). Based on these data, and because killifish embryos are not directly exposed to probiotics, there is potential that L. rhamnosus IMC 501® influences the activation of the embryonic immune complement system through the inheritance of appropriate metabolic information and/or immunological cues from the mother, aiding embryonic development and survival. As no functional investigations have been conducted, this idea should be considered purely speculative, and there is still a great need for further research on this subject.
Thus, a scientific explanation is needed for the mechanism of action of probiotics on the reproductive axis as well as the nutritional/immunological mediation of interactions and broodstock profiles on fertilization, larval development, and growth. Carnevali et al. (2013) restudied the reproductive effects of L. rhamnosus on zebrafish as a food additive. Administration of L. rhamnosus over a while can accelerate larval growth by acting on growth-promoting factors such as insulin-like growth factors I and II (IGF I and IGF II), receptors α and β of peroxisome proliferators (ppar-α, -β), vitamin D receptor-α (vdr-α), and retinoic acid receptor-γ (rar-γ).
Rawls et al. (2004) showed that the microbiota changes may affect the transcription of 212 genes, 8 of which are involved in stimulating food metabolism. Avella et al. (2012) found that the presence of L. rhamnosus in the zebrafish digestive system influenced the expression of numerous genes involved in larval development. Myostatin (mstn) levels were considerably lower after probiotic transmission. Changes in IGFs and mstn levels were associated with increased zebrafish growth. IGF I and II up-regulation (Avella et al., 2012) in zebrafish treated with L. rhamnosus was observed to coincide with expected backbone development. These modifications led to increased expression of vdrα and rarγ genes. The ligands of these two receptors (vitamin D and retinoic acid) play critical roles in morphogenesis and chondrogenesis (Mendelsohn et al., 1994a, b, c). Vitamin D promotes calcium intake and retention (Saggese et al., 2002), but IGFs are the primary determinants of backbone calcification and bone mass accretion, influencing muscle and skeletal cell proliferation and division. Lactobacillus spp. naturally release vitamins and fatty acids that bind to nuclear receptors such as vdr, rar (Chawla et al., 2001; Narva et al., 2004; Teusink and Smid, 2006), and ppar-α and –β, which are involved in skeletal development (Burdick et al., 2006). The calcification examination revealed increased calcification of centra in probiotic-treated groups, indicating more rapid backbone calcification (Avella et al., 2012). Avella et al. (2012) reported that host development is also affected by the continuous administration of exogenous probiotics. In zebrafish, after administration of L. rhamnosus for 2 months, the period tested started from eggs, larvae, to sexual maturation. Since 6 dpf, fish showed increased levels of gene expression on IGF I and II, peroxisome proliferator-activated receptors-α and -β, VDR-α and RAR-γ. Higher expression of GnRH3 was found at different intervals of L. rhamnosus. The resulting larvae showed earlier maturation and development of bone and gonadal calcifications.
GnRH is indispensable during the reproductive process; its function is mainly to regulate vertebrate puberty and gametogenesis. In two zebrafish species, GnRH3 was shown to have gonadotropin releasing activity that is thought to assimilate the non-redundant function of GnRH1 (Steven et al., 2003; Okubo and Nagahama, 2008; Zohar et al., 2010). To understand whether the presence of probiotic bacteria (L. rhamnosus) will be influential in the process of gonadal development and sex differentiation through the GnRH pathway, a parallel GnRH3-GFP transgenic line, as well as larvae being determinants of ongoing backbone calcification was used in this study (Abraham et al., 2008). Zebrafish are a juvenile protogynous hermaphroditic species, first developing ovary-like gonads regardless of their genetic sex; bisexual differentiation occurs when protogynous ovaries from several specimens in a population undergo differentiation, first turning into an intermediate phase called ‘altered ovary’ and then becoming testicles (Maack and Segner, 2003). In determining whether L. rhamnosus could act on the onset of gonadal differentiation, a calculation of the time of appearance of the first testicle has been carried out. Progress of gonadal maturation by histological analysis and sex ratio was registered at the end of the experiment.
One of the main beneficial effects of probiotics is their immune and antioxidant enhancement properties (Nayak, 2010). Immune and antioxidant indexes such as lysozyme (LYZ), alkaline and acid phosphatase (AKP and ACP), complement 3 and 4 (C3 and C4), immunoglobulin M (IgM), aspartate and alanine aminotransferase (AST/ALT), superoxidase dismutase (SOD), catalase (CAT), glutathione peroxidase, glutathione reductase, total antioxidant activity and malondialdehyde (MDA), and others play significant roles in the immunity enhancement of fish which later transcends into having a healthy host. LYZ is noted as the first line of defense as they mainly aid in the attacks, hydrolysis, and the breakage of peptidoglycan glycosidic bonds; AKP and ACP being some kind of nonspecific phosphohydrolase can help in catalyzing the hydrolysis of phosphate monesters; High C3 and C4 levels in fish blood/serum plays ardent role in the prevention of external microorganism; IgM while being liable to phagocyte destruction in host organism helps in toxin and virus neutralization; T-AOC serves as overall indicator of host antioxidant status; AST, ALT, and LDH function as reliable damaged tissue indicators as a result of toxicants; MDA shows the degree of lipid-peroxidation portraying all the toxic processes caused as a result of free radicals (Amoah et al., 2021b; Yu et al., 2022; Amoah et al., 2023, 2024; Li et al., 2024; Niu et al., 2024). Measuring the immune and antioxidant enzyme indexes is imperative in probiotic studies as it provides an overview of the health status of the fish. Several studies conducted have shown significant enhancement in LYZ, ACP, AKP, GSH-Px, GR, T-AOC, SOD, CAT, C3, C4, T-AOC, IgM, while mostly decreasing the activities/levels of AST, ALT, LDH, and MDA after the supplementation of probiotics in fish.
Amoah et al. (2023) reported in their study that one of the widely reported probiotic bacteria, such as Bacillus spp. probiotics isolated from host organisms’ gut can improve the immune response, growth performance and hematological parameters, gut histology, and gut microbial composition. After the 6-week trial, it was noted that there was an increase in the group that had been given the relevant probiotics, which was higher than the control group, namely increasing final weight, WGR (weight gain rate), SGR, CF (condition factor), HSI (hepatosomatic index), VSI (viscerosomatic index), and proximate chemical composition (crude protein and fish ash content) in hybrid grouper. The group given the Bacillus sp. supplementation showed an upregulation of the expression of inflammatory genes (including IL1β, IL6, IL8, TNFα, and MyD88), anti-inflammatory genes (IL10 and TGFβ), and tight junction protein genes (occluding and ZO1). IL1β is a key component in the immune response of fish that serves as an arbitrator in response to microbial invasion and tissue damage, just as it does in mammals (Niu et al., 2024). The addition of probiotics to feed isolated from Bacillus strains derived from the intestines of hybrid grouper fish showed significant improvements in IL1β gene expression. The study showed that, among the probiotics supplemented in diets, the highest IL1β gene expression was observed in the B. subtilis GPSAK9 treated group, whereas for the B. velezensis GPSAK4 and B. tequilensis GPSAK2 treated groups, no significant difference was witnessed between them even though there was an upregulation of IL1β gene compared to the control (Amoah et al., 2023).
Another way to trigger this is by stimulating other cytokines capable of activating macrophages. TNFα is an effective mediator in inflammatory and immune responses that control the development and differentiation of many cell types (Zou et al., 2003). TGFβ and IL10 serve as very important anti-inflammatory cytokines that limit the inflammatory response. TGFβ is a powerful immune cytokine whose main role is to trigger active immune tolerance in marginal tissues and mucosa. These cytokines reflect on various immune cells, including macrophages, lymphocytes, and dendritic cells (Singh et al., 2019; Zhang et al., 2021). Occluding and ZO1 are tightly interconnected membrane proteins that regulate epithelial intercellular space, thus preventing the diffusion of gut bacteria and other antigens between epithelial cells (Zhao et al., 2014). IL8 is a chemoattractant cytokine, and its production is initiated by various types of tissue and blood cells, which encourage neutrophils to stimulate chemotaxis, produce free lysozyme enzymes, regulate angiogenesis, and inflammatory processes (Das et al., 2011). It can be concluded that this study aimed to reveal an increase in positive regulation of IL1β, IL6, IL8, TNFα, MyD88, IL10, TGFβ, occludin, and ZO1 in the probiotic group compared to the non-probiotic group, which showed significantly higher expression.
The addition of lactic acid Lactobacillus rhamnosus (strain ATCC 53103) at a level of 105 CFU g-1 feed will stimulate the respiratory burst (RB) in rainbow trout (Oncorhynchus mykiss) (Nikoskelainen et al., 2003). After observation, it was seen that the probiotic bacteria aided in the protection of host from pathogens by blocking the attachment site on the skin, namely adhesion receptors. L. rhamnosus which colonizes epidermal mucus comes from the GIT, probably because feeding was only done manually and once a day. Thus, the entire feed will most likely be eaten up by the fish within a few minutes, allowing the probiotic bacteria to develop properly. This study showed that administering probiotic bacteria into fish feed could stimulate RB activity in the LAB4 group after two weeks of feeding L. rhamnosus. This report is the first to show the effect of probiotics on RB in fish. However, no correlation was found between the amount of L. rhamnosus in gut content and RB activity in each group. Previous research (Nikoskelainen et al., 2001) has shown that including L. rhamnosus into fish feed can reduce the mortality of fish tested with virulent strains of A. salmonicida. Probiotic bacteria given in optimal doses (from 104 CFU/g to 108 CFU/g feed) to fish can stimulate RB activity, which can be a protective mechanism. So, research conducted by Nikoskelainen et al. (2001) demonstrates that adding probiotics can affect fish-specific and innate immunity, thus providing new perspectives and innovations in screening new probiotic strains and matching the doses needed by fish for optimal performance is very significant.
In aquaculture, probiotics are highly expected to have direct involvement in growth efforts both directly and through the absorption of nutrients or vitamins (Ringo and Gatesoupe, 1998), with enhanced immunity and weight gain (Lin et al., 2012). The development of complex microbiota and the return to normal absorption of nutrients shows that the gut microbiota has a large share in the host’s absorption and use of nutrients. Probiotics can convert compounds that were initially difficult for the host to digest into a more digestible form (Wuertz et al., 2021). Various microbial enzymes, namely lipase, phytase, amylase, cellulase, trypsin, and other proteases can also play a role (Santos et al., 2020; Wang et al., 2020; Tarkhani et al., 2020a; Niu et al., 2021; Zhang et al., 2021). Microbes directly play a role in stimulating the activity and secretion of enzymes in the host (Hmani et al., 2017; Tarkhani et al., 2020b; Kong et al., 2021). In modern commercial feed, usually enriched with large amounts of raw vegetable materials, certain probiotics serve to improve the digestion of feed components such as non-starch hydrocarbons, cellulose, or chitin, which are usually difficult for fish hosts to digest. In addition, probiotics such as Lactobacillus can serve as a source of vitamins (Ray et al., 2012; Merrifield and Ringo, 2014; Nguyen et al., 2018). However, it is still an interesting discussion whether the host absorbs this vitamin or vice versa (Eck and Friel, 2014). Bacteria can also be a source of PUFAs (Polyunsaturated Fatty Acids), but their concentrations can vary significantly between bacterial species (Wanka et al., 2018). Vibrio species are especially rich in EPA (Eicosapentaenoic Acid) and DHA (Docosahexaenoic Acid) (Estupinan et al., 2020). The high content of DHA is found in deep-sea fish and is an evolutionary adaptation to high pressure and low temperatures. Although filtration techniques have improved, screening bacteria that produce PUFAs is uncommon (Nichols and Davies, 2002; Nichols and McMeekin, 2002). These examples suggest that probiotics can increase the nutritional value of feed by improving digestion or providing microbial metabolites such as coenzymes, vitamins, or essential fatty acids. Wuertz et al. (2021) added that the effects of probiotics in the GIT related to nutrition and growth can count on several aspects, including the secretion of digestive enzymes, absorption of nutrients such as coenzymes, vitamins, and unsaturated fatty acids, as well as indirect effects that enhance nutrient absorption, stimulation of enzyme secretion, and neuroendocrine stimulation of appetite and growth in aquatic animals. Besides that, Ringo et al. (1995) reported members of the genera Agrobacterium, Pseudomonas, Brevibacterium, Microbacterium, and Staphylococcus contributed to the nutrient process of Arctic char (Salvelinus alpinus). It has been suggested in previous studies that the larvae of the European sea bass (Dicentrarchus labrax) when given probiotic live yeast (Debaryomyces hansenii) showed increased activity and concentration of trypsin mRNA and lipase, respectively.
The probiotic yeast was noted to stimulate enzyme activity in the European sea bass larvae following some mechanism of actions such as (i) increased intestinal maturity triggered by the presence of live yeast in the larval diet, as indicated by increased activity and concentration of mRNA trypsin and lipase; (ii) the presence of probiotic yeast in the larval diet can accelerate the process of pancreatic maturity, it can be seen that there is an increase in the activity and concentration of mRNA trypsin and lipase. These enzymes play a role in the digestion of proteins and fats; (iii) the activity of intestinal enzymes such as AKP, aminopeptidase N, and maltase was higher in the group given 1.1% yeast compared to the other group. This indicates a faster development of intestinal digestion, which can improve nutrient absorption; (iv) The effect of yeast on greater performance is dose-dependent as the best results were shown at a concentration of 1.1% yeast cell biomass in the diet. This means there was an optimal level of probiotic supplementation to obtain a maximum benefit; and (v) The effect of yeast dose on greater performance is associated with the amount of polyamine secreted by live yeast in the intestinal lumen of the larvae. Polyamine is an organic compound that has an important role in cell growth and differentiation, and its presence may also contribute to increased intestinal maturity and observed enzyme activity. Overall, probiotic yeast stimulated enzyme activity by improving intestinal maturity, pancreatic function, and digestion, with effects influenced by dosage and the amount of polyamines produced (Tovar-Ramirez et al., 2004).
It is crucial to ensure that probiotics can efficiently improve animal health without negatively affecting the surrounding water quality to make it commercially viable (Sugimura et al., 2011). In addition, to improve feed efficiency, probiotics have also been shown to break down organic matter into carbon dioxide, which helps reduce the buildup of liquid organic carbon and particulates throughout the growing season. This approach can ultimately improve water quality by balancing phytoplankton production with eutrophication in certain situations (Cruz et al., 2012).
To continuously improve water quality in an aquaculture environment, farmers must constantly remove chemicals or toxic materials in the water. The mechanism of action of probiotics has a positive effect on water quality in the early stages after the addition of probiotics to the water which later translates in enhancing the water quality and the balancing of the microbial composition of host organism and their environment. Heterotrophic probiotic bacteria can also catalyze various chemical reactions such as nitrogen fixation, oxidation, nitrification, denitrification, and sulfurization. Thus, the addition of probiotics is proven to decompose various organic materials and food waste, plankton, and organic salts such as phosphate, CO2, and nitrate. Photosynthetic bacteria that saturate the water and inhibit the growth of other pathogenic microorganisms. The micro-algae formed to provide a suitable medium for usable bacteria and cultured animals (Boyd and Gross, 1998).
The success of water bioremediation in aquaculture assisted by probiotics can be achieved through several mechanisms, namely: (i) nitrification levels must be regulated to maintain ammonia concentrations; (ii) the need to increase the denitrification rate so that excess nitrogen can be removed as nitrogen gas; (iii) increase sulfide oxidation to reduce hydrogen sulfide accumulation; (iv) increase mineralization of carbon to carbon dioxide reduced sludge formation; (v) the productivity of primary ponds needs to be increased so that the production of aquatic animals and secondary crops also increases; and (vi) maintain the diversity and stability of pond communities to prevent non-threatening species domination (Balcazar et al., 2006; Devaraja et al., 2013; Divya et al., 2015).
Bacillus species are known to be the most commonly used probiotics, and several studies have shown their close relation to improving water quality (Xie et al., 2013; Divya et al., 2015). This is due to gram-positive bacteria that are more efficient in converting organic matter into CO2 compared to gram-negative bacteria (Kumar et al., 2016) (Table 4). Based on Chavez-Crooker and Obreque-Contreras (2010), through pathogenic microorganisms and the decomposition of organic substances that are not needed in water and sediment, probiotic bacteria can help microbiota populations continue to increase so that they are useful in the aquatic environment.
Table 4. Association of different Bacillus species as a multispecies/multi-strain used for water quality maintenance.
The use of probiotics, especially in aquaculture, in addition to giving probiotics directly to the target, can also be combined with alternative ingredients such as plant protein diets, vitamins, microalgae, fermented products, etc. This method has been proven to improve aquatic animals’ health and overall growth. The addition of probiotics also offers a very profitable approach as research and technology in this field develop; hence, it is expected to contribute to the sustainability of the aquaculture industry. The supplementation of plant protein diets (such as soybean meal (SM), cottonseed meal, cottonseed protein concentrate (CPC), castor meal, peanut meal, soy protein concentrate, wheat meal, corn meal, cassava meal, etc.) to animals has come to the fore due to the costly fishmeal (FM) protein ingredient since the wild capture fisheries are being depleted and unable to meet the high demand, making fishmeal (the primary protein source) a scarce commodity. Replacing fishmeal with plant protein diets wholly or partially has been reported to cause adverse effects due to the antinutritional factors (ANFs) and secondary metabolites for fish. Other factors, such as a less-than-optimal or imbalanced composition of amino acids and minerals, are noted to cause adverse effect on the health and immunity of the organism (Azeredo et al., 2017; Piazzon et al., 2017; Estruch et al., 2018). Almost all of these challenges can be dealt with by adding probiotics, which in turn aid in adjusting the intestinal microbiota and intestinal health, thereby increasing the digestion rate of food feed ingredients (Wuertz et al., 2021). Some probiotics, such as Bacillus, can also serve as a source of vitamins, but it is still debated whether these vitamins can be absorbed optimally by the host (Ray et al., 2012; Eck and Friel, 2014; Merrifield and Ringo, 2014; Nguyen et al., 2018).
Probiotic supplementation with plant proteins has aided in enhancing growth, response to stress, immunity, nutrition intake, intestine structure, and microbial makeup in shrimp, bullfrogs, fish, etc (Wang et al., 2021b; Zheng et al., 2022). One of the most widely used microorganisms as a fermentation agent is Bacillus subtilis. Using bacteria in the fermentation process can eliminate ANFs as a provider of good microbial metabolites, as well as quality nutrients (Jakobsen et al., 2015; Oso et al., 2015; Shiu et al., 2015). Based on research conducted by Wang et al. (2021b), the damaging effects caused by SM-based high-level basal diets can be alleviated with the help of B. subtilis LCBS1. Fermentation of B. subtilis LCBS1 directly improved the nutritional quality of SM in terms of growth performance, feed digestibility, intestinal morphology, and microbial composition of bullfrogs. For example, LAB and B. subtilis natto as probiotics have been noted to provide various health benefits for consumers, encouraging the development of certain functional foods (Molina et al., 2012). LAB, known as the primary intestinal microflora, has been widely used in producing fermented soy milk products and other foods (Kim et al., 2012). Lactobacillus plantarum is reported as being able to reduce the immunoreactivity of soybean flour by decreasing IgE immunoreactivity (Nguyen et al., 2007; Frias et al., 2008). B. subtilis has been reported to enhance the antioxidant, anti-allergic, and fibrinolytic functions of soybeans (Kwon et al., 2000; Juan et al., 2010; Kirubakaran et al., 2010). Several studies show that LAB is often used in the fermentation of soybean derivative products, such as tofu (mostly fermented Chinese food made from soybean), soybean milk, and soybean flour (Han et al., 2004; Georgetti et al., 2009; Marazza et al., 2012).
Using probiotics in aquaculture has greatly influenced fisheries production. Applying probiotics in aquaculture can help improve disease resistance and stress tolerance, digestion, reproduction, immune function, serve as a source of nutrients, and improve water quality. Biogenic amines (BAs) compounds in fish are usually neutralized using oxidase or microorganism activity with decarboxylase activity. Histamine toxicity results in special regulatory limits for fishery products. Wide diversity of microorganisms can decarboxylate histidine to produce biogenic amines called histamine, often found in fishmeal content. The population of these microorganisms can develop and grow in fish exposed to high temperatures, causing an increase in BA levels by residual enzyme activity. In this case, control procedures and prevention strategies such as the use of probiotics are needed to face these challenges to protect consumer health and improve the fisheries industry (Visciano et al., 2020). The use of inappropriate or high doses of histamine can harm aquatic organisms, as has been reported in several studies conducted on grouper fish (Liu et al., 2021a), American eel fish (Zhai et al., 2020), and yellow catfish (Li et al., 2018). The addition of autochthonous probiotics has been shown to overcome the negative effects caused by histamine in fish feed, thereby reducing inflammation, improving gut microbiota, and enhancing the growth and immunity of aquatic animals (Dawood, 2021; Yeganeh et al., 2021). In a research conducted by Liu et al. (2021b), three autochthonous probiotics (B. pumilus SE5, Psychrobacter sp. SE6, and B. clausii DE5) were supplemented separately to diets containing 0.3% of histamine and their effects on growth performance, innate immunity, and gut health on grouper (Epinephelus coioides) were enhanced in a 56-day feeding trial.
Enzyme and probiotic supplementation although not replacements for FM but rather serving as complements to alternative ingredients, can enhance microbial cooperation in tissue analysis and increase the abundance of lactic acid bacteria and Bacillus species. In a recently published work, the effects of enzyme (phytase and xylanase) and probiotic (three strains of B. amyloliquefaciens) supplementation were tested on nutrient digestion kinetics and volatile fatty acid content along the gut as well as gut microbiome diversity in Nile tilapia. Probiotic supplementation increased volatile fatty acid content in the foregut, whereas lactate content decreased with enzyme supplementation along the gut when measured for the first time in fish. Enzyme supplementation increased the digestion of crude protein, calcium, and phosphorus in the fore- and midgut. Enzyme and probiotic supplementation also improved microbial interactions, demonstrated through tissue analysis, and increased the number of lactic acid bacteria and Bacillus species. These results suggest that supplementation with enzymes and probiotics improves nutrient availability, and gut health and contributes to a more stable microbiome environment (Maas et al., 2021).
Algae exhibit antimicrobial effects that can benefit other organisms, often known as the plant microbiome (phytobiome). Among the potentials obtained from the use of microalgae, microalgae can be combined with beneficial microbes and have great potential in increasing algae biomass production and multiplying the composition of compounds that will be beneficial to other organisms and the environment, especially the aquatic environment (Makut et al., 2019; Lee and Ryu, 2021; Perkovic et al., 2022). Based on Perkovic et al. (2022), the combination of microalgae and probiotics will have a great impact on other organisms, as it can produce a variety of compounds that are beneficial to host health, wastewater treatment, biorefinery, biofertilization, and also in the improvement of food and nutrition biotechnology. Microalgae are not only used as extracts that can accelerate the performance of probiotics, but the interaction between the two can add more value to the resulting product. The interaction between the two organisms will increase the activity and production of bioactive compounds so that they can fight pathogens.
Subedi and Shrestha (2020) concluded that despite having a beneficial effect on aquaculture management, probiotics are still very limited in inclusive research and studies. The use of probiotics still has various obstacles, including the inability of aquatic species strains to produce on a large scale and the unpreparedness of the industry to process probiotic products for the needs of aquatic organisms; hence, the use of terrestrial probiotics is more frequent. Moreover, the lack of knowledge and understanding of the methods of administration and the benefits of probiotics occurs among fish farmers. To overcome this limitation, further research is needed on the mechanism of action of probiotics, their impact on microbial communities in the aquatic environment, and their potential ecological risks. In this case, collaboration between aquaculturists, fish nutritionists, and microbiologists is very important. By overcoming these limitations, probiotics can be used more widely and effectively in aquaculture to increase production sustainably.
Some other obstacles, such as the difficulty of isolation and functional verification of probiotics isolated from the organs of host organism (intestines, liver, stomach, oral mucosa, mucus-secreting glands, etc.) are very difficult so this will severely limit the effective application of probiotics to aquatic organisms. In addition, very limited works have been done Bacillus species derived from the host intestine in regulating mucosal immunity and intestinal microbiota, making it difficult to explore superior strains.
Furthermore, the interaction of probiotics with culture media is also very influential; several parameters such as pH level and temperature, can affect the effectiveness of probiotic strains. Most bacteria have an optimal pH range, so if bacteria are placed in a medium that is too acidic or alkaline, their viability and efficacy will be reduced. Alkalinity is a buffering agent to resist dramatic changes in pH levels through the interaction between carbonate-bicarbonate with neutralizing acids or acids and bases (Makori et al., 2017). The survival and function of probiotics are also affected by the fluctuations that occur in alkalinity. Another factor that affects the performance of probiotics is temperature, which can affect the metabolic activity of probiotics. Dissolved oxygen levels are one of the most important factors in the growth environment of probiotics, but its effect depends on the type of probiotic used and the type of fermentation or culture process applied. Anjum et al. (2022) reported that high temperatures can cause a decrease in the viability and stability of probiotics, either during the storage period or in the digestive tract of organisms.
It can be seen that native species that originate from a specific environment or ecosystem are being explored for their potential in aquaculture. It is necessary to perform more effective trials to find out in detail and direct the effectiveness and mechanism of action of native species in their function as disease prevention and increase the growth of aquaculture animals. Understanding the potential use of probiotics against microbial communities and the potential ecological risks that will be posed is not fully known, so further research is still urgently needed. Several other supporting factors also affect the effectiveness of probiotic action, such as the type of strain candidate that must be evaluated according to global standards, the suitability of the dose needed with the capacity of the cultivated organism, and the application method used in its application.
China (mainland) has produced more aquatic animals and cultured algae than any other country since 1991. Its share of world aquaculture production was 56.7% for aquatic animals and 59.5% for cultured algae over the past 10 years (FAO, 2020, 2023). Countries such as China dominate the world’s export of finfish species farmed in cages. Atlantic salmon are representative of marine cage farming of cold-water species, while the finfish produced by marine cage farmers in China are mainly warm-water species and are much with regard to species.
According to data from FAO (2023), China being one of the global producers of aquaculture has made significant contribution to the fishery industry and as such, highlighting the successes chalked with regards to the use of probiotics is of great significance. When referring to the potential generated, China has a very large market, so it has the potential to develop and apply probiotics sustainably. Information on the use of probiotics in China can be accessed easily and provides useful insights to researchers and technicians, who want to adopt probiotics in their aquaculture activities.
Although many studies have been reported in China on probiotics in aquaculture, the approach is generally empirical. The most basic mechanism of action of probiotics in aquatic systems is not often studied, so the resulting adverse effects tend to be overlooked. Further studies are needed to determine the positive and negative effects caused by probiotics to direct the selection and benefit-oriented manipulation of safe and appropriate probiotics. Considering the negative effects of probiotics, the addition of certain compounds is expected to mitigate these negative impacts and help maximize the health benefits obtained from the consumption of probiotics in aquaculture.
V. alginolyticus is one of the most commonly known disease causing bacteria that cause detrimental effects such as high mortality rates of aquatic animals, which go a long way affecting the aquaculture industry’s development. So, the selection of probiotic strains before direct administration needs accuracy to help tackle the disease menace (Selvaraju, 2015). Some commonly used probiotic strains, namely Bifidobacteria, Lactobacilli, and even Streptococcus thermophilus, are known as additional feed supplements (Kim et al., 2007; Denev et al., 2009). The addition of these probiotics to the diets of aquatic animals during breeding enhances the population of microbes in the intestinal contents greater than its environment, making it potentially beneficial for host organisms. Additionally, several factors need to be considered in the selection of probiotics, which include the type of species cultivated, the type of probiotic bacteria to be used and the right dosage, and the conditions of the cultivation environment, such as whether the provision of probiotics can disrupt the balance of the aquatic environment ecosystem (interactions with other organisms including plankton and other bacteria), and aquaculture management. In a case where there is supposedly an inappropriate use of probiotics, it is feared that there will be higher increase of waste production (undigested feed residues and fish excretion) which can heighten the spreading of disease if not isolated properly.
Future research perspectives on probiotics in China are promising to advance our understanding of probiotic interventions’ potential applications and benefits. The evolving landscape of probiotic research in China is marked by a growing interest in exploring diverse strains and their applications across various domains, including human health, animal husbandry, and agriculture. Research endeavors in China, as reflected in studies by authors such as Wang et al. (2018) and Li et al. (2012), are delving into the intricate mechanisms by which probiotics influence gut health, immune responses, and overall well-being in humans. There is a significant emphasis on determining original probiotic strains with special characteristics that correspond with the unique health requirements of the Chinese population. Additionally, research is expanding to explore the potential of probiotics in addressing prevalent health concerns, such as metabolic disorders and gastrointestinal diseases.
In the field of animal husbandry and aquaculture, studies like those conducted by Huang et al. (2023) and Wang et al. (2021a) are paving the way for innovative applications of probiotics to enhance growth promotor, disease resistance and overall productivity in livestock and aquatic species. The exploration of probiotics as alternatives to traditional antimicrobial agents is gaining momentum, aligning with global efforts to promote sustainable and eco-friendly practices in agriculture. Incorporating probiotics in soil and plant systems is an emerging area of interest; as such, investigating probiotics’ role in promoting soil health, nutrient uptake, and crop yield has implications for sustainable agriculture practices and food security in China (Qi et al., 2009). As China continues to invest in research infrastructure and collaborative initiatives, future studies are anticipated to elucidate the intricate interplay between probiotics and various ecosystems. The holistic understanding gained from such research endeavors will contribute to developing tailored probiotic interventions that align with the unique contexts and challenges in China’s diverse landscapes. The multifaceted exploration of probiotics in China underscores its potential to shape the future of healthcare, agriculture, and environmental sustainability.
In conclusion, using probiotics in aquaculture has greatly influenced fisheries production. Applying probiotics in aquaculture can help enhance disease and stress tolerance, improve digestion, promote reproduction, boost immunity, and improve water quality. Among the several probiotics used, the most commonly used ones, namely, Lactobacillus sp., Pseudomonas sp., and Bacillus sp., have the potential to enhance fish production due to the vast research work conducted on them aside from their enormous beneficial effects on fish performance (increase the survival rate of the fish and as an immune stimulation).
SR: Conceptualization, Data curation, Methodology, Visualization, Writing – original draft. KA: Conceptualization, Data curation, Funding acquisition, Methodology, Writing – original draft, Writing – review & editing. YH: Methodology, Visualization, Writing – review & editing. JC: Formal analysis, Supervision, Writing – review & editing. BW: Data curation, Project administration, Writing – review & editing. VMS: Writing – review & editing, Formal analysis. XJ: Writing – review & editing, Formal analysis. MAA: Writing – review & editing, Visualization. MJ: Conceptualization, Funding acquisition, Methodology, Supervision, Writing – review & editing.
The author(s) declare financial support was received for the research, authorship, and/or publication of this article. The research leading to these results was financially supported by the Program for Scientific Research Start-up Funds of Guangdong Ocean University (060302022310), the Zhanjiang City Science and Technology Projects (2022A01015), the Science and Technology Plan of Guangdong Province (2023B0202010016), and the Program for Scientific Research Start-up Funds of Guangdong Ocean University (060302022101).
The authors declare that the research was conducted in the absence of any commercial or financial relationships that could be construed as a potential conflict of interest.
All claims expressed in this article are solely those of the authors and do not necessarily represent those of their affiliated organizations, or those of the publisher, the editors and the reviewers. Any product that may be evaluated in this article, or claim that may be made by its manufacturer, is not guaranteed or endorsed by the publisher.
Abdel-Latif H. M. R., Dawood M. A. O., Menanteau-Ledouble S., El-Matbouli M. (2020). The nature and consequences of co-infections in tilapia: A review. J. Fish Dis. 43, 651–664. doi: 10.1111/jfd.13164
Abraham E., Palevitch O., Ijiri S., Du S. J., Gothilf Y., Zohar Y. (2008). Early development of forebrain gonadotrophin-releasing hormone (GnRH) neurones and the role of gnRH as an autocrine migration factor. J. Neuroendocrinol. 20, 394–405. doi: 10.1111/j.1365-2826.2008.01654.x
Afrilasari W., Widanarni, Meryandini A. (2016). Effect of Probiotic Bacillus megaterium PTB 1.4 on the Population of Intestinal Microflora, Digestive Enzyme Activity and the Growth of Catfish (Clarias sp.). HAYATI J. Biosci. 23, 168–172. doi: 10.1016/j.hjb.2016.12.005
Agustina, Prayitno S. B., Sabdono A., Saptiani G. (2018). Antagonistic activity of kelabau fish (Osteochilus melanopleurus) gut bacteria against Aeromonas hydrophila and Pseudomonas sp. AACL Bioflux 11, 1859–1868. doi: 10.22059/ijvm.2018.235444.1004816
Alishahi M., Dezfuly Z. T., Mohammadian T., Mesbah M. (2018). Effects of two probiotics, lactobacillus plantarum and lactobacillus bulgaricus on growth performance and intestinal lactic acid bacteria of cyprinus carpio. Iran. J. Vet. Med. 12, 207–217. doi: 10.22059/ijvm.2018.235444.1004816
Amiri O., Miandare H. K., Hoseinifar S. H., Shabni A., Safari R. (2018). Skin Mucus Protein Profile, Immune Parameters, Immune-Related Gene Expression, and Growth Performance of Rainbow Trout (Oncorhynchus mykiss) Fed White Button Mushroom (Agaricus bisporus) Powder. Int. J. Med. Mushrooms 20, 337–347. doi: 10.1615/IntJMedMushrooms.2018025825
Amoah K., Cai J., Huang Y., Wang B., Shija V. M., Wang Z., et al. (2024). Identification and characterization of four Bacillus species from the intestine of hybrid grouper (Epinephelus fuscoguttatus♀ × E. lanceolatus♂), their antagonistic role on common pathogenic bacteria, and effects on intestinal health. Fish Shellfish Immunol. 152, 109795. doi: 10.1016/j.fsi.2024.109795
Amoah K., Dong X., Tan B., Zhang S., Chi S., Yang Q., et al. (2021a). Effects of three probiotic strains (Bacillus coagulans, Bacillus licheniformis and Paenibacillus polymyxa) on growth, immune response, gut morphology and microbiota, and resistance against Vibrio harveyi of northern whitings, Sillago sihama Forsskal, (1775). Anim. Feed Sci. Technol. 277, 114958. doi: 10.1016/j.anifeedsci.2021.114958
Amoah K., Dong X., Tan B., Zhang S., Kuebutornye F. K. A., Chi S., et al. (2021b). In vitro Assessment of the Safety and Potential Probiotic Characteristics of Three Bacillus Strains Isolated From the Intestine of Hybrid Grouper (Epinephelus fuscoguttatus♀ × Epinephelus lanceolatus♂). Front. Vet. Sci. 8. doi: 10.3389/fvets.2021.675962
Amoah K., Tan B., Zhang S., Chi S., Yang Q., Liu H., et al. (2023). Host gut-derived Bacillus probiotics supplementation improves growth performance, serum and liver immunity, gut health, and resistive capacity against Vibrio harveyi infection in hybrid grouper (♀Epinephelus fuscoguttatus × ♂Epinephelus lanceolatus). Anim. Nutr. 14, 163–184. doi: 10.1016/j.aninu.2023.05.005
Anis M. Y., Hariani D. (2019). The administration of commercial feed with addition of EM4 (Effective microorganism 4) to increase catfish (Clarias sp.) growth rate. J. Biol. Res. Its Appl. 1, 1–8.
Anjum F. M., Saeed F., Afzaal M., Ikram A., Azam M. (2022). “The effect of thermal processing on probiotics stability,” in Advances in Dairy Microbial Products (Woodhead Publishing). 295–302. doi: 10.1016/B978-0-323-85793-2.00004-7
Ardita N., Budiharjo A., Sari S. L. A. (2015). Growth and feed conversion ratio of tilapia fish (Oreochromis niloticus) with addition of probiotics. Biotechnology 12, 16–21. doi: 10.13057/biotek/c120103
Avella M. A., Place A., Du S.-J., Williams E., Silvi S., Zohar Y., et al. (2012). Lactobacillus rhamnosus Accelerates Zebrafish Backbone Calcification and Gonadal Differentiation through Effects on the GnRH and IGF Systems. PloS One 7, e45572. doi: 10.1371/journal.pone.0045572
Azeredo R., MaChado M., Kreuz E., Wuertz S., Oliva-Teles A., Enes P., et al. (2017). The European seabass (Dicentrarchus labrax) innate immunity and gut health are modulated by dietary plant-protein inclusion and prebiotic supplementation. Fish Shellfish Immunol. 60, 78–87. doi: 10.1016/j.fsi.2016.11.019
Bairagi A., Ghosh K. S., Sen S. K., Ray A. K. (2002). Enzyme-producing bacterial flora isolated from fish digestive tracts. Aquac. Int. 10, 109–121. doi: 10.1023/1021355406412
Balcazar J., Blas I., Ruizzarzuela I., Cunningham D., Vendrell D., Muzquiz J. (2006). The role of probiotics in aquaculture. Vet. Microbiol. 114, 173–186. doi: 10.1016/j.vetmic.2006.01.009
Balcazar J. L., de Blas I., Ruiz-Zarzuela I., Vendrell D., Gironacs O., Muzquiz J. L. (2007). Enhancement of the immune response and protection induced by probiotic lactic acid bacteria against furunculosis in rainbow trout (Oncorhynchus mykiss). FEMS Immunol. Med. Microbiol. 51, 185–193. doi: 10.1111/j.1574-695X.2007.00294.x
Banerjee G., Ray A. K. (2017). The advancement of probiotics research and its application in fish farming industries. Res. Vet. Sci. 115, 66–77. doi: 10.1016/j.rvsc.2017.01.016
Banos A., Ariza J. J., Nunez C., Gil-Martinez L., Garcia-Lopez J. D., Martinez-Bueno M., et al. (2019). Effects of Enterococcus faecalis UGRA10 and the enterocin AS-48 against the fish pathogen Lactococcus garvieae. Studies in vitro and in vivo. Food Microbiol. 77, 69–77. doi: 10.1016/j.fm.2018.08.002
Bondad-Reantaso M. G., Subasinghe R. P., Arthur J. R., Ogawa K., Chinabut S., Adlard R., et al. (2005). Disease and health management in Asian aquaculture. Vet. Parasitol. 132, 249–272. doi: 10.1016/j.vetpar.2005.07.005
Boyd C. E., Gross A. (1998). Use of probiotics for improving soil and water quality in aquaculture ponds. Dep. Fish. Allied Aquac.
Burdick A. D., Kim D. J., Peraza M. A., Gonzalez F. J., Peters J. M. (2006). The role of peroxisome proliferator-activated receptor-β/δ in epithelial cell growth and differentiation. Cell. Signal. 18, 9–20. doi: 10.1016/j.cellsig.2005.07.009
Carnevali O., Avella M. A., Gioacchini G. (2013). Effects of probiotic administration on zebrafish development and reproduction. Gen. Comp. Endocrinol. 188, 297–302. doi: 10.1016/j.ygcen.2013.02.022
Carnevali O., Zamponi M. C., Sulpizio R., Rollo A., Nardi M., Orpianesi C., et al. (2004). Administration of probiotic strain to improve sea bream wellness during development. Aquac. Int. 12, 377–386. doi: 10.1023/B:AQUI.0000042141.85977.bb
Chavez-Crooker P., Obreque-Contreras J. (2010). Bioremediation of aquaculture wastes. Curr. Opin. Biotechnol. 21, 313–317. doi: 10.1016/j.copbio.2010.04.001
Chawla A., Repa J. J., Evans R. M., Mangelsdorf D. J. (2001). Nuclear receptors and lipid physiology: opening the X-files. Sci. (80-.). 294, 1866–1870. doi: 10.1126/science.294.5548.1866
Chythanya R., Karunasagar I., Karunasagar I. (2002). Inhibition of shrimp pathogenic vibrios by a marine Pseudomonas I-2 strain. Aquaculture 208, 1–10. doi: 10.1016/S0044-8486(01)00714-1
Claus S. P., Guillou H., Ellero-Simatos S. (2017). Erratum: The gut microbiota: a major player in the toxicity of environmental pollutants? NPJ Biofilms Microbiomes 3, 17001. doi: 10.1038/npjbiofilms.2017.1
Coulibaly W. H., Kouadio N. R., Camara F., Diguta C., Matei F. (2023). Functional properties of lactic acid bacteria isolated from Tilapia (Oreochromis niloticus) in Ivory Coast. BMC Microbiol. 23, 152. doi: 10.1186/s12866-023-02899-6
Cruz P. M., Ibanez A. L., Hermosillo A. O. M., Saad H. C. R. (2012). Use of probiotics in aquaculture. ISRN Microbiol. 2012, 13. doi: 10.5402/2012/916845
Das A., Sahoo P. K., Mohanty B. R., Jena J. K. (2011). Pathophysiology of experimental Aeromonas hydrophila infection in Puntius sarana: Early changes in blood and aspects of the innate immune-related gene expression in survivors. Vet. Immunol. Immunopathol. 142, 207–218. doi: 10.1016/j.vetimm.2011.05.017
Dash P., Tandel R. S., Bhat R. A. H., Mallik S., Pandey N. N., Singh A. K., et al. (2018). The addition of probiotic bacteria to microbial floc: Water quality, growth, non-specific immune response and disease resistance of Cyprinus carpio in mid-Himalayan altitude. Aquaculture 495, 961–969. doi: 10.1016/j.aquaculture.2018.06.056
Dawood M. A. O. (2021). Nutritional immunity of fish intestines: important insights for sustainable aquaculture. Rev. Aquac. 13, 642–663. doi: 10.1111/raq.12492
Denev S., Staykov Y., Moutafchieva R., Beev G. (2009). International Aquatic Research Microbial ecology of the gastrointestinal tract of fish and the potential application of probiotics and prebiotics in finfish aquaculture. Int. Aquat. Res. Int. Aquat Res. 1, 1–29.
Devaraja T., Banerjee S., Yusoff F., Shariff M., Khatoon H. (2013). A holistic approach for selection of Bacillus spp. as a bioremediator for shrimp postlarvae culture. Turkish J. Biol. 37, 92–200. doi: 10.3906/biy-1203-19
Divya M., Aanand S., Srinivasan A., Ahilan B. (2015). Bioremediation –An eco-friendly tool for effluent treatment: A Review. Int. J. Appl. ied Res. 1, 530–537.
Dong W., Cai Y., Xu Z., Fu B., Chen Q., Cui Y., et al. (2020). Heterologous expression of AHL lactonase AiiK by Lactobacillus casei MCJΔ1 with great quorum quenching ability against Aeromonas hydrophila AH-1 and AH-4. Microb. Cell Fact. 19, 191. doi: 10.1186/s12934-020-01448-4
Eck P., Friel J. (2014). Should Probiotics be considered as Vitamin Supplements? Vitam. Miner. 03, 1–2. doi: 10.4172/2376-1318.1000e124
Eickhoff M. J., Bassler B. L. (2018). SnapShot: bacterial quorum sensing. Cell 174, 1328–1328.e1. doi: 10.1016/j.cell.2018.08.003
Elsabagh M., Mohamed R., Moustafa E. M., Hamza A., Farrag F., Decamp O., et al. (2018). Assessing the impact of Bacillus strains mixture probiotic on water quality, growth performance, blood profile and intestinal morphology of Nile tilapia, Oreochromis niloticus. Aquac. Nutr. 24, 1613–1622. doi: 10.1111/anu.12797
Estruch G., Collado M. C., Monge-Ortiz R., Tomas-Vidal A., Jover-Cerda M., Penaranda D. S., et al. (2018). Long-term feeding with high plant protein-based diets in gilthead seabream (Sparus aurata, L.) leads to changes in the inflammatory and immune-related gene expression at intestinal level. BMC Vet. Res. 14, 302. doi: 10.1186/s12917-018-1626-6
Estupinan M., Hernandez I., Saitua E., Bilbao M. E., Mendibil I., Ferrer J., et al. (2020). Novel vibrio spp. Strains producing omega-3 fatty acids isolated from coastal seawater. Mar. Drugs 18, 99. doi: 10.3390/md18020099
FAO (2020). The State of World Fisheries and Aquaculture 2020. Sustainability in action (Rome). doi: 10.4060/ca9229en
FAO (2023). “Fishery and Aquaculture Statistics. Global aquaculture production 1950–2021 (FishStatJ),” in FAO Fisheries and Aquaculture Division(Rome).
Frias J., Song Y. S., Martínez-Villaluenga C., De Mejia E. G., Vidal-Valverde C. (2008). Immunoreactivity and amino acid content of fermented soybean products. J. Agric. Food Chem. 56, 99–105. doi: 10.1021/jf072177j
Gao F., Li F., Tan J., Yan J., Sun H. (2014). Bacterial Community Composition in the Gut Content and Ambient Sediment of Sea Cucumber Apostichopus japonicus Revealed by 16S rRNA Gene Pyrosequencing. PloS One 9, e100092. doi: 10.1371/journal.pone.0100092
Georgetti S. R., Vicentini F. T. M. C., Yokoyama C. Y., Borin M. F., Spadaro A. C. C., Fonseca M. J. V. (2009). Enhanced in vitro and in vivo antioxidant activity and mobilization of free phenolic compounds of soybean flour fermented with different β-glucosidase-producing fungi. J. Appl. Microbiol. 106, 459–466. doi: 10.1111/j.1365-2672.2008.03978.x
Ghosh K., Sen S. K., Ray A. K. (2004). Growth and survival of rohu, Labeo rohita (Hamilton) spawn fed diets fermented with intestinal bacterium, Bacillus circulans. Acta Ichthyol. Piscat. 34, 155–165. doi: 10.3750/AIP2004.34.2.04
Giri S. S., Sen S. S., Sukumaran V. (2012). Effects of dietary supplementation of potential probiotic Pseudomonas aeruginosa VSG-2 on the innate immunity and disease resistance of tropical freshwater fish, Labeo rohita. Fish Shellfish Immunol. 32, 1135–1140. doi: 10.1016/j.fsi.2012.03.019
Giri S. S., Sukumaran V., Sen S. S., Jena P. K. (2014). Effects of dietary supplementation of potential probiotic Bacillus subtilis VSG1 singularly or in combination with Lactobacillus plantarum VSG3 or/and Pseudomonas aeruginosa VSG2 on the growth, immunity and disease resistance of Labeo rohita. Aquac. Nutr. 20, 163–171. doi: 10.1111/anu.12062
Gomez G. D., Balcazar J. L. (2008). A review on the interactions between gut microbiota and innate immunity of fish: Table 1. FEMS Immunol. Med. Microbiol. 52, 145–154. doi: 10.1111/j.1574-695X.2007.00343.x
Gram L., Lovold T., Nielsen J., Melchiorsen J., Spanggaard B. (2001). In vitro antagonism of the probiont Pseudomonas fluorescens strain AH2 against Aeromonas salmonicida does not confer protection of salmon against furunculosis. Aquaculture 199, 1–11. doi: 10.1016/S0044-8486(01)00565-8
Gram L., Melchiorsen J., Spanggaard B., Huber I., Nielsen T. F. (1999). Inhibition of Vibrio Anguillarum by Pseudomonas fluorescens AH2, a Possible Probiotic Treatment of Fish. Appl. Environ. Microbiol. 65, 969–973. doi: 10.1128/AEM.65.3.969-973.1999
Han B.-Z., Cao C.-F., Rombouts F. M., Nout M. J. R. (2004). Microbial changes during the production of Sufu—-a Chinese fermented soybean food. Food Control 15, 265–270. doi: 10.1016/S0956-7135(03)00066-5
Han B., Long W., He J., Liu Y., Si Y., Tian L. (2015). Effects of dietary Bacillus licheniformis on growth performance, immunological parameters, intestinal morphology and resistance of juvenile Nile tilapia (Oreochromis niloticus) to challenge infections. Fish Shellfish Immunol. 46, 225–231. doi: 10.1016/j.fsi.2015.06.018
Hao K., Wu Z.-Q., Li D.-L., Yu X.-B., Wang G.-X., Ling F. (2017). Effects of Dietary Administration of Shewanella xiamenensis A-1, Aeromonas veronii A-7, and Bacillus subtilis, Single or Combined, on the Grass Carp (Ctenopharyngodon idella) Intestinal Microbiota. Probiot. Antimicrob. Proteins 9, 386–396. doi: 10.1007/s12602-017-9269-7
Hasyimi W., Widanarni W., Yuhana M. (2020). Growth performance and intestinal microbiota diversity in pacific white shrimp litopenaeus vannamei fed with a probiotic bacterium, honey prebiotic, and synbiotic. Curr. Microbiol. 77, 2982–2990. doi: 10.1007/s00284-020-02117-w
Hmani H., Daoud L., Jlidi M., Jalleli K., Ben Ali M., Hadj Brahim A., et al. (2017). A Bacillus subtilis strain as probiotic in poultry: selection based on in vitro functional properties and enzymatic potentialities. J. Ind. Microbiol. Biotechnol. 44, 1157–1166. doi: 10.1007/s10295-017-1944-x
Huang L., Shui X., Wang H., Qiu H., Tao C., Yin H., et al. (2023). Effects of Bacillus halophilus on growth, intestinal flora and metabolism of Larimichthys crocea. Biochem. Biophys. Rep. 35, 1–13. doi: 10.1016/j.bbrep.2023.101546
Huang J., Wu Y. C., Chi S. C. (2014). Dietary supplementation of Pediococcus pentosaceus enhances innate immunity, physiological health and resistance to Vibrio Anguillarum in orange-spotted grouper (Epinephelus coioides). Fish Shellfish Immunol. 39, 196–205. doi: 10.1016/j.fsi.2014.05.003
Hunt L. R., Rice C. D. (2008). Lymphoid tissue ontogeny in the mummichog, fundulus heteroclitus. Anat. Rec. 291, 1236–1245. doi: 10.1002/ar.20740
Ibrahem M. D. (2015). Evolution of probiotics in aquatic world: Potential effects, the current status in Egypt and recent prospectives. J. Adv. Res. 6, 765–791. doi: 10.1016/j.jare.2013.12.004
Irianto A., Austin B. (2002). Probiotics in aquaculture. J. Fish Dis. 25, 633–642. doi: 10.1046/j.1365-2761.2002.00422.x
Irianto A., Austin B. (2003). Use of dead probiotic cells to control furunculosis in rainbow trout, Oncorhynchus mykiss (Walbaum). J. Fish Dis. 26, 59–62. doi: 10.1046/j.1365-2761.2003.00414.x
Istiqomah I., Atitus I. N., Rohman A. F., Isnansetyo A. (2019). Isolation of Cellulolytic Bacterium Staphylococcus sp. JC20 from the Intestine of Octopus (Octopus sp.) for Fish Probiotic Candidate. J. Perikan. Univ. Gadjah Mada 21, 93. doi: 10.22146/jfs.39525
Jahid I. K., Mizan M. F. R., Ha A. J., Ha S.-D. (2015). Effect of salinity and incubation time of planktonic cells on biofilm formation, motility, exoprotease production, and quorum sensing of Aeromonas hydrophila. Food Microbiol. 49, 142–151. doi: 10.1016/j.fm.2015.01.016
Jakobsen G. V., Jensen B. B., Knudsen K. E. B., Canibe N. (2015). Improving the nutritional value of rapeseed cake and wheat-dried distillers grains with solubles by addition of enzymes during liquid fermentation. Anim. Feed Sci. Technol. 208, 198–213. doi: 10.1016/j.anifeedsci.2015.07.015
Juan M.-Y., Wu C.-H., Chou C.-C. (2010). Fermentation with Bacillus spp. as a bioprocess to enhance anthocyanin content, the angiotensin-converting enzyme inhibitory effect, and the reducing activity of black soybeans. Food Microbiol. 27, 918–923. doi: 10.1016/j.fm.2010.05.009
Kim D.-H., Brunt J., Austin B. (2007). Microbial diversity of intestinal contents and mucus in rainbow trout (Oncorhynchus mykiss). J. Appl. Microbiol. 102, 1654–1664. doi: 10.1111/j.1365-2672.2006.03185.x
Kim D.-M., Lee H., Yoo S.-H. (2012). Compositional changes and physical properties of soymilk prepared with pre-soaked-fermented soybean. J. Korean Soc Appl. Biol. Chem. 55, 121–126. doi: 10.1007/s13765-012-0021-4
Kirubakaran C. J. W., Alexander C. P., Michael R. D. (2010). Enhancement of non-specific immune responses and disease resistance on oral administration of Nyctanthes arbortristis seed extract in Oreochromis mossambicus (Peters). Aquac. Res. 41, 1630–1639. doi: 10.1111/j.1365-2109.2010.02516.x
Kong Y., Li M., Chu G., Liu H., Shan X., Wang G., et al. (2021). The positive effects of single or conjoint administration of lactic acid bacteria on Channa argus: Digestive enzyme activity, antioxidant capacity, intestinal microbiota and morphology. Aquaculture 531, 735852. doi: 10.1016/j.aquaculture.2020.735852
Kumar V., Roy S., Meena D. K., Sarkar U. K. (2016). Application of probiotics in shrimp aquaculture: importance, mechanisms of action, and methods of administration. Rev. Fish. Sci. Aquac. 24, 342–368. doi: 10.1080/23308249.2016.1193841
Kwon S., Lee P. C., Lee E. G., Keun Chang Y., Chang N. (2000). Production of lactic acid by Lactobacillus rhamnosus with vitamin-supplemented soybean hydrolysate. Enzyme Microb. Technol. 26, 209–215. doi: 10.1016/S0141-0229(99)00134-9
Lauzon H. L., Perez-Sanchez T., Merrifield D. L., Ringo E., Balcazar J. L. (2014). “Probiotic Applications in Cold Water Fish Species,” in Aquaculture Nutrition (Wiley), 223–252. doi: 10.1002/9781118897263.ch9
Lazado C. C., Caipang C. M. A. (2014). Mucosal immunity and probiotics in fish. Fish Shellfish Immunol. 39, 78–89. doi: 10.1016/j.fsi.2014.04.015
Lee S.-M., Ryu C.-M. (2021). Algae as new kids in the beneficial plant microbiome. Front. Plant Sci. 12. doi: 10.3389/fpls.2021.599742
Li X., Liu S. F., Yan Y. M., Zhang R. J., Wang T. J., Fu B. R. (2020). Effect of Bacillus complex on water quality and bacterial community structure of Carassius aumtus culture water. J. Ecol. Rural Environ. 36, 522–530. doi: 10.19741/j.issn.1673-4831.2019.0573
Li W., Pan X., Cheng W., Cheng Y., Yin Y., Chen J., et al. (2018). Serum biochemistry, histology and transcriptomic profile analysis reflect liver inflammation and damage following dietary histamine supplementation in yellow catfish (Pelteobagrus fulvidraco). Fish Shellfish Immunol. 77, 83–90. doi: 10.1016/j.fsi.2018.03.036
Li M. Y., Shi Y. C., Xu W. X., Zhao L., Zhang A. Z. (2024). Exploring Cr(VI)-induced blood-brain barrier injury and neurotoxicity in zebrafish and snakehead fish, and inhibiting toxic effects of astaxanthin. Environ. pollut. 355, 124280. doi: 10.1016/j.envpol.2024.124280
Li X., Wang T., Fu B., Mu X. (2022). Improvement of aquaculture water quality by mixed Bacillus and its effects on microbial community structure. Environ. Sci. pollut. Res. 29, 69731–69742. doi: 10.1007/s11356-022-20608-0
Li Y., Xiang Q., Zhang Q., Huang Y., Su Z. (2012). Overview on the recent study of antimicrobial peptides: Origins, functions, relative mechanisms and application. Peptides 37, 207–215. doi: 10.1016/j.peptides.2012.07.001
Lin S., Mao S., Guan Y., Luo L., Luo L., Pan Y. (2012). Effects of dietary chitosan oligosaccharides and Bacillus coagulans on the growth, innate immunity and resistance of koi (Cyprinus carpio koi). Aquaculture 342–343, 36–41. doi: 10.1016/j.aquaculture.2012.02.009
Liu C.-H., Chiu C.-H., Wang S.-W., Cheng W. (2012). Dietary administration of the probiotic, Bacillus subtilis E20, enhances the growth, innate immune responses, and disease resistance of the grouper, Epinephelus coioides. Fish Shellfish Immunol. 33, 699–706. doi: 10.1016/j.fsi.2012.06.012
Liu Z.-Y., Yang H.-L., Hu L.-H., Yang W., Ai C.-X., Sun Y.-Z. (2021a). Dose-dependent effects of histamine on growth, immunity and intestinal health in juvenile grouper (Epinephelus coioides). Front. Mar. Sci. 8. doi: 10.3389/fmars.2021.685720
Liu Z.-Y., Yang H.-L., Hu L.-H., Yang W., Ai C.-X., Sun Y.-Z. (2021b). Autochthonous probiotics alleviate the adverse effects of dietary histamine in juvenile grouper (Epinephelus coioides). Front. Microbiol. 12. doi: 10.3389/fmicb.2021.792718
Lombardo F., Gioacchini G., Carnevali O. (2013). Probiotic-based nutritional effects on killifish reproduction. Fish. Aquac. J. 02, 1–11. doi: 10.4172/2150-3508.1000033
Lovoll M., Kilvik T., Boshra H., Bogwald J., Sunyer J. O., Dalmo R. A. (2006). Maternal transfer of complement components C3-1, C3-3, C3-4, C4, C5, C7, Bf, and Df to offspring in rainbow trout (Oncorhynchus mykiss). Immunogenetics 58, 168–179. doi: 10.1007/s00251-006-0096-3
Maack G., Segner H. (2003). Morphological development of the gonads in zebrafish. J. Fish Biol. 62, 895–906. doi: 10.1046/j.1095-8649.2003.00074.x
Maas R. M., Deng Y., Dersjant-Li Y., Petit J., Verdegem M. C. J., Schrama J. W., et al. (2021). Exogenous enzymes and probiotics alter digestion kinetics, volatile fatty acid content and microbial interactions in the gut of Nile tilapia. Sci. Rep. 11, 8221. doi: 10.1038/s41598-021-87408-3
Makori A. J., Abuom P. O., Kapiyo R., Anyona D. N., Dida G. O. (2017). Effects of water physico-chemical parameters on tilapia (Oreochromis niloticus) growth in earthen ponds in Teso North Sub-County, Busia County. Fish. Aquat. Sci. 20, 30. doi: 10.1186/s41240-017-0075-7
Makut B. B., Das D., Goswami G. (2019). Production of microbial biomass feedstock via co-cultivation of microalgae-bacteria consortium coupled with effective wastewater treatment: A sustainable approach. Algal Res. 37, 228–239. doi: 10.1016/j.algal.2018.11.020
Marazza J. A., Nazareno M. A., de Giori G. S., Garro M. S. (2012). Enhancement of the antioxidant capacity of soymilk by fermentation with Lactobacillus rhamnosus. J. Funct. Foods 4, 594–601. doi: 10.1016/j.jff.2012.03.005
Meidong R., Nakao M., Sakai K., Tongpim S. (2021). Lactobacillus paraplantarum L34b-2 derived from fermented food improves the growth, disease resistance and innate immunity in Pangasius bocourti. Aquaculture 531, 735878. doi: 10.1016/j.aquaculture.2020.735878
Mendelsohn C., Larkin S., Mark M., LeMeur M., Clifford J., Zelent A., et al. (1994a). RARβ isoforms: distinct transcriptional control by retinoic acid and specific spatial patterns of promoter activity during mouse embryonic development. Mech. Dev. 45, 227–241. doi: 10.1016/0925-4773(94)90010-8
Mendelsohn C., Lohnes D., Decimo D., Lufkin T., LeMeur M., Chambon P., et al. (1994b). Function of the retinoic acid receptors (RARs) during development: (II) Multiple abnormalities at various stages of organogenesis in RAR double mutants. Development 120, 2749–2771. doi: 10.1242/dev.120.10.2749
Mendelsohn C., Mark M., Dolle P., Dierich A., Gaub M. P., Krust A., et al. (1994c). Retinoic acid receptor beta 2 (RAR beta 2) null mutant mice appear normal. Dev. Biol. 166, 246–258. doi: 10.1006/dbio.1994.1311
Merrifield D. L., Dimitroglou A., Foey A., Davies S. J., Baker R. T. M., Bøgwald J., et al. (2010). The current status and future focus of probiotic and prebiotic applications for salmonids. Aquaculture 302, 1–18. doi: 10.1016/j.aquaculture.2010.02.007
Merrifield D. L., Ringo E. (2014). Aquaculture Nutrition. Eds. Merrifield D., Ringø E. (Wiley). doi: 10.1002/9781118897263
Miao S., Zhao C., Zhu J., Hu J., Dong X., Sun L. (2018). Dietary soybean meal affects intestinal homoeostasis by altering the microbiota, morphology and inflammatory cytokine gene expression in northern snakehead. Sci. Rep. 8, 113. doi: 10.1038/s41598-017-18430-7
Mohammadian T., Monjezi N., Peyghan R., Mohammadian B. (2022). Effects of dietary probiotic supplements on growth, digestive enzymes activity, intestinal histomorphology and innate immunity of common carp (Cyprinus carpio): a field study. Aquaculture 549, 737787. doi: 10.1016/j.aquaculture.2021.737787
Mohammadian T., Nasirpour M., Tabandeh M. R., Mesbah M. (2019). Synbiotic effects of β-glucan, mannan oligosaccharide and Lactobacillus casei on growth performance, intestine enzymes activities, immune-hematological parameters and immune-related gene expression in common carp, Cyprinus carpio: An experimental infectio. Aquaculture 511, 634197. doi: 10.1016/j.aquaculture.2019.06.011
Mohapatra S., Chakraborty T., Prusty A. K., Das P., Paniprasad K., Mohanta K. N. (2012). Use of different microbial probiotics in the diet of rohu, Labeo rohita fingerlings: effects on growth, nutrient digestibility and retention, digestive enzyme activities and intestinal microflora. Aquac. Nutr. 18, 1–11. doi: 10.1111/j.1365-2095.2011.00866.x
Molina V., Medici M., Font de Valdez G., Taranto M. P. (2012). Soybean-based functional food with vitamin B12-producing lactic acid bacteria. J. Funct. Foods 4, 831–836. doi: 10.1016/j.jff.2012.05.011
Mulchandani R., Wang Y., Gilbert M., Van Boeckel T. P. (2023). Global trends in antimicrobial use in food-producing animals: 2020 to 2030. PloS Glob. Public Heal. 3, 1–11. doi: 10.1371/journal.pgph.0001305
Narva M., Halleen J., Vaananen K., Korpela R. (2004). Effects of Lactobacillus helveticus fermented milk on bone cells in vitro. Life Sci. 75, 1727–1734. doi: 10.1016/j.lfs.2004.04.011
Nayak S. K. (2010). Probiotics and immunity: A fish perspective. Fish Shellfish Immunol. 29, 2–14. doi: 10.1016/j.fsi.2010.02.017
Nayak S. K. (2021). Multifaceted applications of probiotic Bacillus species in aquaculture with special reference to Bacillus subtilis. Rev. Aquac. 13, 862–906. doi: 10.1111/raq.12503
Nayak S. K., Mukherjee S. C. (2011). Screening of gastrointestinal bacteria of Indian major carps for a candidate probiotic species for aquaculture practices. Aquac. Res. 42, 1034–1041. doi: 10.1111/j.1365-2109.2010.02686.x
Ng W.-K., Kim Y.-C., Romano N., Koh C.-B., Yang S.-Y. (2014). Effects of Dietary Probiotics on the Growth and Feeding Efficiency of Red Hybrid Tilapia, Oreochromis sp., and Subsequent Resistance to Streptococcus agalactiae. J. Appl. Aquac. 26, 22–31. doi: 10.1080/10454438.2013.874961
Nguyen T. L., Chun W.-K., Kim A., Kim N., Roh H. J., Lee Y., et al. (2018). Dietary Probiotic Effect of Lactococcus lactis WFLU12 on Low-Molecular-Weight Metabolites and Growth of Olive Flounder (Paralichythys olivaceus). Front. Microbiol. 9. doi: 10.3389/fmicb.2018.02059
Nguyen T. T. T., Loiseau G., Icard-Verniere C., Rochette I., Treche S., Guyot J.-P. (2007). Effect of fermentation by amylolytic lactic acid bacteria, in process combinations, on characteristics of rice/soybean slurries: A new method for preparing high energy density complementary foods for young children. Food Chem. 100, 623–631. doi: 10.1016/j.foodchem.2005.09.080
Nichols D. S., Davies N. W. (2002). Improved detection of polyunsaturated fatty acids as phenacyl esters using liquid chromatography-ion trap mass spectrometry. J. Microbiol. Methods 50, 103–113. doi: 10.1016/S0167-7012(02)00030-1
Nichols D. S., McMeekin T. A. (2002). Biomarker techniques to screen for bacteria that produce polyunsaturated fatty acids. J. Microbiol. Methods 48, 161–170. doi: 10.1016/S0167-7012(01)00320-7
Nikoskelainen S., Ouwehand A. C., Bylund G., Salminen S., Lilius E.-M. (2003). Immune enhancement in rainbow trout (Oncorhynchus mykiss) by potential probiotic bacteria (Lactobacillus rhamnosus). Fish Shellfish Immunol. 15, 443–452. doi: 10.1016/S1050-4648(03)00023-8
Nikoskelainen S., Ouwehand A., Salminen S., Bylund G. (2001). Protection of rainbow trout (Oncorhynchus mykiss) from furunculosis by Lactobacillus rhamnosus. Aquaculture 198, 229–236. doi: 10.1016/S0044-8486(01)00593-2
Niu K.-M., Kothari D., Lee W.-D., Zhang Z., Lee B.-J., Kim K.-W., et al. (2021). Probiotic potential of the farmed olive flounder, paralichthys olivaceus, autochthonous gut microbiota. Probiot. Antimicrob. Proteins 13, 1106–1118. doi: 10.1007/s12602-021-09762-y
Niu X.-T., Sun C., Zhao L., Chen X.-M., Wang G.-Q., Li M.-Y. (2024). The major role of glucocorticoid receptor (GR) in astaxanthin alleviates immune stress in Channa argus lymphocyte. Aquaculture 584, 740637. doi: 10.1016/j.aquaculture.2024.740637
Noor S. Y., Pakaya R. (2018). Effect of addition of probiotics EM4 (Effective microorganism 4) in feed on the growth and survival of gurame fish (Ophronemus gouramy). Gorontalo Fish. J. 1, 51. doi: 10.32662/.v1i1.106
Okubo K., Nagahama Y. (2008). Structural and functional evolution of gonadotropin-releasing hormone in vertebrates. Acta Physiol. 193, 3–15. doi: 10.1111/j.1748-1716.2008.01832.x
Oso A. O., Li L., Zhang B., Uo R., Fan J. X., Wang S., et al. (2015). Effect of fungal fermentation with Aspergillus Niger and enzyme supplementation on metabolizable energy values of unpeeled cassava root meal for meat-type cockerels. Anim. Feed Sci. Technol. 210, 281–286. doi: 10.1016/j.anifeedsci.2015.09.015
Patterson R. N., Watts K. C. (2003). Micro-particles in recirculating aquaculture systems: particle size analysis of culture water from a commercial Atlantic salmon site. Aquac. Eng. 28, 99–113. doi: 10.1016/S0144-8609(03)00003-7
Perez-Sanchez T., Balcazar J. L., Merrifield D. L., Carnevali O., Gioacchini G., de Blas I., et al. (2011). Expression of immune-related genes in rainbow trout (Oncorhynchus mykiss) induced by probiotic bacteria during Lactococcus garvieae infection. Fish Shellfish Immunol. 31, 196–201. doi: 10.1016/j.fsi.2011.05.005
Perez-Sanchez T., Ruiz-Zarzuela I., de Blas I., Balcazar J. L. (2014). Probiotics in aquaculture: a current assessment. Rev. Aquac. 6, 133–146. doi: 10.1111/raq.12033
Perkovic L., Djedovic E., Vujovic T., Bakovic M., Paradzik T., Coz-Rakovac R. (2022). Biotechnological enhancement of probiotics through co-cultivation with algae: future or a trend? Mar. Drugs 20, 142. doi: 10.3390/md20020142
Piazzon M. C., Calduch-Giner J. A., Fouz B., Estensoro I., Simo-Mirabet P., Puyalto M., et al. (2017). Under control: how a dietary additive can restore the gut microbiome and proteomic profile, and improve disease resilience in a marine teleostean fish fed vegetable diets. Microbiome 5, 164. doi: 10.1186/s40168-017-0390-3
Qi Z., Zhang X.-H., Boon N., Bossier P. (2009). Probiotics in aquaculture of China — Current state, problems and prospect. Aquaculture 290, 15–21. doi: 10.1016/j.aquaculture.2009.02.012
Rahimnejad S., Guardiola F. A., Leclercq E., Ángeles Esteban M., Castex M., Sotoudeh E., et al. (2018). Effects of dietary supplementation with Pediococcus acidilactici MA18/5M, galactooligosaccharide and their synbiotic on growth, innate immunity and disease resistance of rockfish (Sebastes schlegeli). Aquaculture 482, 36–44. doi: 10.1016/j.aquaculture.2017.09.020
Ramirez R. F., Dixon B. A. (2003). Enzyme production by obligate intestinal anaerobic bacteria isolated from oscars (Astronotus ocellatus), angelfish (Pterophyllum scalare) and southern flounder (Paralichthys lethostigma). Aquaculture 227, 417–426. doi: 10.1016/S0044-8486(03)00520-9
Rawls J. F., Samuel B. S., Gordon J. I. (2004). Gnotobiotic zebrafish reveal evolutionarily conserved responses to the gut microbiota. Proc. Natl. Acad. Sci. U. S. A. 101, 4596–4601. doi: 10.1073/pnas.0400706101
Ray A. K., Ghosh K., Ringo E. (2012). Enzyme-producing bacteria isolated from fish gut: A review. Aquac. Nutr. 18, 465–492. doi: 10.1111/j.1365-2095.2012.00943.x
Ringo E., Gatesoupe F.-J. (1998). Lactic acid bacteria in fish: a review. Aquaculture 160, 177–203. doi: 10.1016/S0044-8486(97)00299-8
Ringo E., Strom E., Tabachek J.-A. (1995). Intestinal microflora of salmonids: a review. Aquac. Res. 26, 773–789. doi: 10.1111/j.1365-2109.1995.tb00870.x
Rombout J., Huttenhuis H., Picchietti S., Scapigliati G. (2005). Phylogeny and ontogeny of fish leucocytes. Fish Shellfish Immunol. 19, 441–455. doi: 10.1016/j.fsi.2005.03.007
Safari R., Adel M., Lazado C. C., Caipang C. M. A., Dadar M. (2016). Host-derived probiotics Enterococcus casseliflavus improves resistance against Streptococcus iniae infection in rainbow trout (Oncorhynchus mykiss) via immunomodulation. Fish Shellfish Immunol. 52, 198–205. doi: 10.1016/j.fsi.2016.03.020
Saggese G., Baroncelli G. I., Bertelloni S. (2002). Puberty and bone development. Best Pract. Res. Clin. Endocrinol. Metab. 16, 53–64. doi: 10.1053/beem.2001.0180
Santos K. O., Costa-Filho J., Spagnol K. L., Nornberg B. F., Lopes F. M., Tesser M. B., et al. (2020). The inclusion of a transgenic probiotic expressing recombinant phytase in a diet with a high content of vegetable matter markedly improves growth performance and the expression of growth-related genes and other selected genes in zebrafish. Aquaculture 519, 734878. doi: 10.1016/j.aquaculture.2019.734878
Schar D., Klein E. Y., Laxminarayan R., Gilbert M., Van Boeckel T. P. (2020). Global trends in antimicrobial use in aquaculture. Sci. Rep. 10, 21878. doi: 10.1038/s41598-020-78849-3
Selim K. M., Reda R. M. (2015). Improvement of immunity and disease resistance in the Nile tilapia, Oreochromis niloticus, by dietary supplementation with Bacillus amyloliquefaciens. Fish Shellfish Immunol. 44, 496–503. doi: 10.1016/j.fsi.2015.03.004
Selvaraju R. (2015). Beneficial and destructive effects of probiotics in aquaculture systems-A review. Int. J. Fish. Aquat. Stud. 2, 153–159.
Shiu Y.-L., Hsieh S.-L., Guei W.-C., Tsai Y.-T., Chiu C.-H., Liu C.-H. (2015). Using Bacillus subtilis E20-fermented soybean meal as replacement for fish meal in the diet of orange-spotted grouper (Epinephelus coioides, Hamilton). Aquac. Res. 46, 1403–1416. doi: 10.1111/are.12294
Singh R., Alape D., de Lima A., Ascanio J., Majid A., Gangadharan S. P. (2019). Regulatory T cells in respiratory health and diseases. Pulm. Med. 2019, 1–13. doi: 10.1155/2019/1907807
Soltani M., Kane A., Taheri-Mirghaed A., Pakzad K., Hosseini-Shekarabi P. (2019). Effect of the probiotic, Lactobacillus plantarum on growth performance and haematological indices of rainbow trout (Oncorhynchus mykiss) immunized with bivalent streptococcosis/lactococcosis vaccine. Iran. J. Fish. Sci. 18, 283–295. doi: 10.22092/ijfs.2018.117757
Sotomayor M. A., Balcazar J. L. (2003). Inhibicion de vibrios patogenos de camaron por mezclas de cepas probioticas. Rev. Aquat. 19, 9–15.
Spinler J. K., Taweechotipatr M., Rognerud C. L., Ou C. N., Tumwasorn S., Versalovic J. (2008). Human-derived probiotic Lactobacillus reuteri demonstrate antimicrobial activities targeting diverse enteric bacterial pathogens. Anaerobe 14, 166–171. doi: 10.1016/j.anaerobe.2008.02.001
Steven C., Lehnen N., Kight K., Ijiri S., Klenke U., Harris W. A., et al. (2003). Molecular characterization of the GnRH system in zebrafish (Danio rerio): cloning of chicken GnRH-II, adult brain expression patterns and pituitary content of salmon GnRH and chicken GnRH-II. Gen. Comp. Endocrinol. 133, 27–37. doi: 10.1016/S0016-6480(03)00144-8
Subedi B., Shrestha A. (2020). A review : Application of probiotics in aquaculture. Int. J. For. Anim. Fish. Res. 4, 52–60. doi: 10.22161/ijfaf.4.5.1
Sugimura Y., Hagi T., Hoshino T. (2011). Correlation between in vitro mucus adhesion and the in vivo colonization ability of lactic acid bacteria: screening of new candidate carp probiotics. Biosci. Biotechnol. Biochem. 75, 511–515. doi: 10.1271/bbb.100732
Sun Y., He M., Cao Z., Xie Z., Liu C., Wang S., et al. (2018). Effects of dietary administration of Lactococcus lactis HNL12 on growth, innate immune response, and disease resistance of humpback grouper (Cromileptes altivelis). Fish Shellfish Immunol. 82, 296–303. doi: 10.1016/j.fsi.2018.08.039
Sun Y.-Z., Yang H.-L., Huang K.-P., Ye J.-D., Zhang C.-X. (2013). Application of autochthonous Bacillus bio encapsulated in copepod to grouper Epinephelus coioides larvae. Aquaculture 392–395, 44–50. doi: 10.1016/j.aquaculture.2013.01.037
Sveinsdottir H., Steinarsson A., Gudmundsdottir A. (2009). Differential protein expression in early Atlantic cod larvae (Gadus morhua) in response to treatment with probiotic bacteria. Comp. Biochem. Physiol. Part D Genomics Proteomics 4, 249–254. doi: 10.1016/j.cbd.2009.06.001
Tabassum T., Sofi Uddin Mahamud A. G. M., Acharjee T. K., Hassan R., Akter Snigdha T., Islam T., et al. (2021). Probiotic supplementations improve growth, water quality, hematology, gut microbiota and intestinal morphology of Nile tilapia. Aquac. Rep. 21, 100972. doi: 10.1016/j.aqrep.2021.100972
Tarkhani R., Imani A., Hoseinifar S. H., Ashayerizadeh O., Sarvi Moghanlou K., Manaffar R., et al. (2020a). Comparative study of host-associated and commercial probiotic effects on serum and mucosal immune parameters, intestinal microbiota, digestive enzymes activity and growth performance of roach (Rutilus rutilus caspicus) fingerlings. Fish Shellfish Immunol. 98, 661–669. doi: 10.1016/j.fsi.2019.10.063
Tarkhani R., Imani A., Hoseinifar S. H., Sarvi Moghanlou K., Manaffar R. (2020b). The effects of host-associated Enterococcus faecium CGMCC1.2136 on serum immune parameters, digestive enzymes activity and growth performance of the Caspian roach (Rutilus rutilus caspicus) fingerlings. Aquaculture 519, 734741. doi: 10.1016/j.aquaculture.2019.734741
Tarnecki A. M., Wafapoor M., Phillips R. N., Rhody N. R. (2019). Benefits of a Bacillus probiotic to larval fish survival and transport stress resistance. Sci. Rep. 9, 1–11. doi: 10.1038/s41598-019-39316-w
Teusink B., Smid E. J. (2006). Modelling strategies for the industrial exploitation of lactic acid bacteria. Nat. Rev. Microbiol. 4, 46–56. doi: 10.1038/nrmicro1319
Tinh N. T. N., Dierckens K., Sorgeloos P., Bossier P. (2008). A review of the functionality of probiotics in the larviculture food chain. Mar. Biotechnol. 10, 1–12. doi: 10.1007/s10126-007-9054-9
Tovar-Ramirez D., Zambonino Infante J., Cahu C., Gatesoupe F., Vazquez-Juarez R. (2004). Influence of dietary live yeast on European sea bass (Dicentrarchus labrax) larval development. Aquaculture 234, 415–427. doi: 10.1016/j.aquaculture.2004.01.028
Tuan T. N., Duc P. M., Hatai K. (2013). Overview of the use of probiotics in aquaculture. Int. J. Res. Fish. Aquac. 3, 89–97.
Vendrell D., Luis Balcazar J., de Blas I., Ruiz-Zarzuela I., Girones O., Luis Muzquiz J. (2008). Protection of rainbow trout (Oncorhynchus mykiss) from lactococcosis by probiotic bacteria. Comp. Immunol. Microbiol. Infect. Dis. 31, 337–345. doi: 10.1016/j.cimid.2007.04.002
Vine N. G., Leukes W. D., Kaiser H. (2004a). In vitro growth characteristics of five candidate aquaculture probiotics and two fish pathogens grown in fish intestinal mucus. FEMS Microbiol. Lett. 231, 145–152. doi: 10.1016/S0378-1097(03)00954-6
Vine N. G., Leukes W. D., Kaiser H. (2006). Probiotics in marine larviculture. FEMS Microbiol. Rev. 30, 404–427. doi: 10.1111/j.1574-6976.2006.00017.x
Vine N. G., Leukes W. D., Kaiser H., Daya S., Baxter J., Hecht T. (2004b). Competition for attachment of aquaculture candidate probiotic and pathogenic bacteria on fish intestinal mucus. J. Fish Dis. 27, 319–326. doi: 10.1111/j.1365-2761.2004.00542.x
Vinoj G., Jayakumar R., Chen J.-C., Withyachumnarnkul B., Shanthi S., Vaseeharan B. (2015). N-hexanoyl-L-homoserine lactone-degrading Pseudomonas aeruginosa PsDAHP1 protects zebrafish against Vibrio parahaemolyticus infection. Fish Shellfish Immunol. 42, 204–212. doi: 10.1016/j.fsi.2014.10.033
Visciano P., Schirone M., Paparella A. (2020). An overview of histamine and other biogenic amines in fish and fish products. Foods 9, 1795. doi: 10.3390/foods9121795
Wang Y., Al Farraj D. A., Vijayaraghavan P., Hatamleh A. A., Biji G. D., Rady A. M. (2020). Host associated mixed probiotic bacteria induced digestive enzymes in the gut of tiger shrimp Penaeus monodon. Saudi J. Biol. Sci. 27, 2479–2484. doi: 10.1016/j.sjbs.2020.07.010
Wang X.-L., Liu Z.-Y., Li Y.-H., Yang L.-Y., Yin J., He J.-H., et al. (2021a). Effects of dietary supplementation of lactobacillus delbrueckii on gut microbiome and intestinal morphology in weaned piglets. Front. Vet. Sci. 8. doi: 10.3389/fvets.2021.692389
Wang A. R., Ran C., Ringo E., Zhou Z. G. (2018). Progress in fish gastrointestinal microbiota research. Rev. Aquac. 10, 626–640. doi: 10.1111/raq.12191
Wang Z., Yang M., Wang L., Lu K., Song K., Zhang C. (2021b). Bacillus subtilis LCBS1 supplementation and replacement of fish meal with fermented soybean meal in bullfrog (Lithobates catesbeianus) diets: Effects on growth performance, feed digestibility and gut health. Aquaculture 545, 737217. doi: 10.1016/j.aquaculture.2021.737217
Wanka K. M., Damerau T., Costas B., Krueger A., Schulz C., Wuertz S. (2018). Isolation and characterization of native probiotics for fish farming. BMC Microbiol. 18, 119. doi: 10.1186/s12866-018-1260-2
Watzke J., Schirmer K., Scholz S. (2007). Bacterial lipopolysaccharides induce genes involved in the innate immune response in embryos of the zebrafish (Danio rerio). Fish Shellfish Immunol. 23, 901–905. doi: 10.1016/j.fsi.2007.03.004
Wu Z.-Q., Jiang C., Ling F., Wang G.-X. (2015). Effects of dietary supplementation of intestinal autochthonous bacteria on the innate immunity and disease resistance of grass carp (Ctenopharyngodon idellus). Aquaculture 438, 105–114. doi: 10.1016/j.aquaculture.2014.12.041
Wuertz S., Schroeder A., Wanka K. M. (2021). Probiotics in fish nutrition—Long-standing household remedy or native nutraceuticals? Water 13, 1348. doi: 10.3390/w13101348
Xie F., Zhu T., Zhang F., Zhou K., Zhao Y., Li Z. (2013). Using Bacillus amyloliquefaciens for remediation of aquaculture water. Springerplus 2, 119. doi: 10.1186/2193-1801-2-119
Xu Y., Wang Y., Lin J. (2014). Use of Bacillus coagulans as a Dietary Probiotic for the Common Carp, Cyprinus carpio. J. World Aquac. Soc 45, 403–411. doi: 10.1111/jwas.12139
Yeganeh S., Adel M., Nosratimovafagh A., Dawood M. A. O. (2021). The Effect of Lactococcus lactis subsp. lactis PTCC 1403 on the Growth Performance, Digestive Enzymes Activity, Antioxidative Status, Immune Response, and Disease Resistance of Rainbow Trout (Oncorhynchus mykiss). Probiot. Antimicrob. Proteins 13, 1723–1733. doi: 10.1007/s12602-021-09787-3
Yu Z., Zhao L., Zhao J.-L., Xu W., Guo Z., Zhang A.-Z., et al. (2022). Dietary Taraxacum mongolicum polysaccharide ameliorates the growth, immune response, and antioxidant status in association with NF-κB, Nrf2 and TOR in Jian carp (Cyprinus carpio var. Jian). Aquaculture 547, 737522. doi: 10.1016/j.aquaculture.2021.737522
Zhai S., Wang Y., He Y., Chen X. (2020). Oligomeric proanthocyanidins counteracts the negative effects of high level of dietary histamine on American eel (Anguilla rostrata). Front. Mar. Sci. 7. doi: 10.3389/fmars.2020.549145
Zhang W., Tan B., Deng J., Dong X., Yang Q., Chi S., et al. (2021). Mechanisms by which fermented soybean meal and soybean meal induced enteritis in marine fish juvenile pearl gentian grouper. Front. Physiol. 12. doi: 10.3389/fphys.2021.646853
Zhao J., Feng L., Liu Y., Jiang W., Wu P., Jiang J., et al. (2014). Effect of dietary isoleucine on the immunity, antioxidant status, tight junctions and microflora in the intestine of juvenile Jian carp (Cyprinus carpio var. Jian). Fish Shellfish Immunol. 41, 663–673. doi: 10.1016/j.fsi.2014.10.002
Zheng X., Liu B., Wang N., Yang J., Zhou Q., Sun C., et al. (2022). Low fish meal diet supplemented with probiotics ameliorates intestinal barrier and immunological function of Macrobrachium rosenbergii via the targeted modulation of gut microbes and derived secondary metabolites. Front. Immunol. 13. doi: 10.3389/fimmu.2022.1074399
Zohar Y., Muñoz-Cueto J. A., Elizur A., Kah O. (2010). Neuroendocrinology of reproduction in teleost fish. Gen. Comp. Endocrinol. 165, 438–455. doi: 10.1016/j.ygcen.2009.04.017
Keywords: antagonistic bacteria, water quality control, immune response, disease control, plant proteins
Citation: Rahayu S, Amoah K, Huang Y, Cai J, Wang B, Shija VM, Jin X, Anokyewaa MA and Jiang M (2024) Probiotics application in aquaculture: its potential effects, current status in China and future prospects. Front. Mar. Sci. 11:1455905. doi: 10.3389/fmars.2024.1455905
Received: 27 June 2024; Accepted: 23 August 2024;
Published: 26 September 2024.
Edited by:
Marty Riche, Florida Atlantic University, United StatesReviewed by:
Dongming Zhang, Jilin Agriculture University, ChinaCopyright © 2024 Rahayu, Amoah, Huang, Cai, Wang, Shija, Jin, Anokyewaa and Jiang. This is an open-access article distributed under the terms of the Creative Commons Attribution License (CC BY). The use, distribution or reproduction in other forums is permitted, provided the original author(s) and the copyright owner(s) are credited and that the original publication in this journal is cited, in accordance with accepted academic practice. No use, distribution or reproduction is permitted which does not comply with these terms.
*Correspondence: Kwaku Amoah, YW1vYWhrMjAxMEB5YWhvby5jb20=; Mouyan Jiang, amlhbmdtb3V5YW5AZ2RvdS5lZHUuY24=
†ORCID: Kwaku Amoah, orcid.org/0000-0001-8986-4451
Disclaimer: All claims expressed in this article are solely those of the authors and do not necessarily represent those of their affiliated organizations, or those of the publisher, the editors and the reviewers. Any product that may be evaluated in this article or claim that may be made by its manufacturer is not guaranteed or endorsed by the publisher.
Research integrity at Frontiers
Learn more about the work of our research integrity team to safeguard the quality of each article we publish.