- 1Hunan Fisheries Science Institute, Changsha, Hunan, China
- 2Key Laboratory of Health Aquaculture and Product Processing in Dongting Lake Area of Hunan Province, Hunan University of Arts and Science, Changde, Hunan, China
- 3College of Biology and Environmental Sciences, Jishou University, Zhangjiajie, Hunan, China
- 4Fisheries College, Hunan Agricultural University, Changsha, Hunan, China
The present study was conducted to evaluate the hormesis and toxicity of short-term low-dose aflatoxin B1 in grass carp (Ctenopharyngodon idellus). Triplicate isonitrogenous and isocaloric aflatoxin B1 diets—CD (control, 0 ug/kg), D1 (20 ug/kg), and D2 (500 ug/kg)—were prepared and fed to grass carp with an initial mean body weight of (15.2 ± 0.1) g for 56 days. The results showed that the weight gain rate and specific growth rate of grass carp fed diet D2 were significantly higher, and the feed coefficient and crude fat content of the whole body were significantly lower (P < 0.05) compared with those fed diet CD. Serum superoxide dismutase content of grass carp fed D1 diet increased significantly (P < 0.05) with an increasing dose of aflatoxin B1, but when the dose reached 500 ug/kg (D2), serum superoxide dismutase, complement C3, and immunoglobulin M of grass carp decreased significantly (P < 0.05), while malondialdehyde increased significantly (P < 0.05). After short-term feeding of aflatoxin B1-containing diets (D1 and D2), liver body index, visceral body index, serum total cholesterol, triglyceride, and urea nitrogen content of grass carp increased significantly (P < 0.05), total bile acid secretion decreased significantly (P < 0.05), and structural damages such as increase in vacuoles, organizational structure loosening, and nucleus translocation were observed in the liver. Meanwhile, liver function indexes such as serum alanine aminotransferase, aspartate aminotransferase, and alkaline phosphatase increased significantly with the increase of aflatoxin B1 dose (P < 0.05). In addition, the height of intestinal villi, crypt depth, villus–crypt ratio, and tubular cell number, as well as the content of trypsin and lipase activities in the intestine of grass carp in the D2 group, were significantly higher than those in the CD group (P < 0.05). In conclusion, after a short-term intake of low doses of aflatoxin B1 (≤500 ug/kg), the toxicological damage of aflatoxin B1 was pronounced, although it produced a certain degree of hormesis on the growth performance and intestinal structure and function of grass carp. At a dose of 20 ug/kg, the non-specific immune system and liver structure and function of grass carp showed obvious toxic damage.
1 Introduction
The term “hormesis” dates back to the 19th century to describe the low-dose excitatory responses of fungi to redwood extracts (Southam and Ehrlich, 1943; Edward and Linda, 2001). Nowadays, “hormesis” is defined as an adaptive biphasic dose response, i.e., low doses stimulate the body’s defense response, presenting a certain stimulatory enhancement of the body’s physiological functions, which results in short-term self-protection and repair capacity in response to low-intensity stimuli, whereas at high doses, the body’s defense system cannot cope with long-term or high-intensity stimulation, resulting in impaired body performance (Edward et al., 2007; Edward, 2016a, 2016b). The enhanced defensive ability triggered by mild exposure to chemical, biological, or abiotic stressors are known as the organism’s adaptive protection response. The key core is that small amounts of reactive oxygen species (ROS) are produced when exposed to mild stress. ROS acts as a signaling molecule that actively activates cellular defenses against the consequent oxidative damage (Maximiliano et al., 2019), which is similar to the oxidative stress preparation (POS) hypothesis (Márcio et al., 2020).
With the increasing scarcity and rising price of fishmeal resources, the proportion of replacement and types of application of plant protein sources in aquatic feed are increasing day by day. These plant protein source plants are prone to mold contamination due to improper environment or management during their growth, processing, transportation, and storage. Among them, Aspergillus flavus is one of the molds that widely exist in the raw materials of moldy plants and feeds. Due to the high toxicity, carcinogenicity, and mutagenicity of its toxin (aflatoxin B1, AFB1), it poses a serious threat to the health of aquaculture targets and humans and has therefore received much attention (Anna et al., 2009).
In recent years, as consumers are more concerned about the quality and safety of aquatic products, the toxic effects of AFB1 on aquatic animals have also become the focus of research scholars at home and abroad. The toxic damage of AFB1, such as growth toxicity (Deng et al., 2010; Mohamed Salah et al., 2018; Rui et al., 2018; Nunes et al., 2019), immune toxicity (Sahoo and Mukherjee, 2003; Nunes et al., 2019), tissue damage (Wang et al., 2012; Arana et al., 2014; Huang et al., 2014; Nunes et al., 2019), and reproductive toxicity, have been reported in Litopenaeus vannamei (Wang et al., 2012; Zeng et al., 2015; Qi et al., 2017; Yu et al., 2018; Nunes et al., 2019), rainbow trout (Oncorhynchus mykiss) (Arana et al., 2014), Colossoma macropomum (Nunes et al., 2019), Nile tilapia (Oreochromis niloticus) (Deng et al., 2010; Katherine et al., 2013; Mohamed Salah et al., 2018), Pangasius hypophthalmus (Rui et al., 2018), perch (Lateolabrax japonicus) (El-Sayed and Riad, 2009), allogenetic silver carp (Carassius auratus gibel) (Huang et al., 2012, 2014), and other aquatic animals. These studies found that the extent of AFB1 toxic damage is influenced by concentration, exposure time, and other factors, and aquatic animals of different species and growth stages have different tolerance levels to AFB1. Etelvina et al. found that feed contaminated with AFB1 in concentrations higher than 500 μg/kg could cause a reduction in growth and weight gain. When the feed was contaminated with 1,000 and 2,000 μg/kg of AFB1, it caused severe deterioration in the hepatic and renal tissues (Nunes et al., 2019). Rui et al. (2018) showed that AFB1 at a level of 50 μg/kg and above could affect the performance and disease resistance of Tra catfish (Rui et al., 2018). However, Huang et al. indicated that AFB1 exposure up to approximately 2,000 μg/kg diet showed no evident effect on the growth and liver function of gibel carp, and AFB1 in muscles were all below the safety limitation of 5 μg/kg wet weight, but it would do harm to the fecundity of the fish (Huang et al., 2014).
Grass carp (Ctenopharyngodon idellus) is the herbivorous fish with the highest aquaculture production in China and the highest feed demand in the world, with most of the production using plant-based protein sources. Because of the high temperature and high humidity climate conditions in southern China, raw materials and feed are susceptible to mold contamination like Aspergillus flavus. In breeding process, feeds with mild mildew and low toxin content are easily ignored due to limited detection methods and unobvious pathological damage characteristics induced by low-toxin feeds. Therefore, exploring the effect of low-dose toxin (AFB1) on fish is more in line with the needs of healthy industrial development. At present, there are still few studies on the toxic effects of AFB1 on grass carp. In this experiment, the stimulation effect and toxic damage degree of short-term low-dose AFB1 on grass carp from the perspective of short-term low-dose toxic stimulation (excitation) were evaluated. The design of aflatoxin concentration in this test mainly referred to the Chinese national standard (GB 13078-2017) and FDA Compliance Policy Guidance Section 555.400 (CPG Sec 555.400), in which the limit value of aflatoxin was ≤20 μg/kg.
2 Materials and methods
2.1 Ethics statement
Grass carp was widely cultivated in mainland China and not listed as endangered or protected species. The whole experiment was conducted according to the guidelines established by the National Institutes of Health. All experimental fish were anesthetized with eugenol (1:12,000, Shanghai Reagent Corporation) before sampling to minimize suffering.
2.2 Experimental diets
Three isonitrogenous and isolipic experimental diets (30.77% protein and 6.54% fat) were designed to include white fish meal and first-grade soybean meal as protein source as well as soybean oil as fat source: 0, 20, and 500 μg/kg AFB1 was added to the basal diet, named control group (CD), D1, and D2, respectively. Pure aflatoxin (AFB1) was purchased from Sigma Company. According to the method of Sanhoo and Mukherjee (2003), AFB1 was dissolved in chloroform and soybean oil, and the soybean oil was mixed with the raw material mixture. Mixing and extruding machine (single-screw extruder SLP-45) made sinking pellet feed with a diameter of 2 mm, air-dried in a cool place, sealed in plastic bag, and stored at -20°C for subsequent use. The basic feed formula and the actual dosage of AFB1 in each group are shown in Tables 1 and 2.
2.3 Experimental fish and feeding management
The experimental grass carp was purchased from a provincial-level improved seed farm in Xiangyin (Hunan, China), and the experiment was carried out at Hunan Institute of Fisheries Science. Before the experiment began, grass carp were acclimatized with the CD diet for 2 weeks, and after being starved for 24 h, 270 grass carp with the same size, robust physique, and initial body weight of (15.2 ± 0.1) g were selected and randomly divided into three groups. There are three replicates in each group and a total of nine breeding ponds (1.5 m × 1.2 m × 1.2 m). Each breeding pond was stocked with 30 grass carp. Fed three times (8:00, 12:30, and 17:00) a day according to 3%–5% of the body weight, and appropriate adjustments are made according to the weather, water temperature, and fish weight gain. The experiment continued for 8 weeks; during this period, micro-flow water was maintained and oxygenation was continued for 24 h to ensure dissolved oxygen ≥ 5.0 mg/L, pH 7.3–7.8, water temperature 24°C–30°C, and ammonia nitrogen concentration ≤ 0.2 mg/L.
2.4 Sample collection
At the end of the experiment, after 24 h of starvation, the total weight of grass carp in each experimental pool was weighed, and the total number of grass carp was recorded. A total of eight to 10 grass carp were randomly selected from each pool. To anesthetize with MS-222 (Sigma, 89 Ronkonkoma, NY, USA), a test anesthetic with low irritation was prepared at 100 mg/L (Guo et al., 2023a), and the test was sampled after the fish were quiet and stopped swimming. The fish body weight and length were measured, and blood was taken from the tail vein. The blood samples were placed in a 1.5-mL centrifuge tube, left at 4°C overnight, and centrifuged at 3,500 r/min for 10 min. The supernatant was taken and stored at –80°C in a refrigerator for the determination of serum biochemical indicators. Five grass carp were randomly selected, anesthetized, and quickly dissected on an ice tray. The liver weight and visceral mass were weighed to calculate the liver-to-body ratio and the visceral-to-body ratio. The foregut was quickly taken, placed in a 10-mL centrifuge tube, and stored at –80°C for use in the determination of intestinal digestive enzyme activity; the liver and intestine of three other grass carp were also taken (for H&E staining) and fixed with 4% paraformaldehyde for 24 h. Six to eight other grass carp were randomly selected, dried with filter paper, packed in a sealed bag, and stored at –20°C for the determination of fish body components.
2.5 Indicator determination
The determination of conventional components was performed according to the AOAC official method (AOAC, 1997). Moisture content was determined by drying the samples to a constant weight at 105°C, the crude protein content of the samples was determined by using the Kjeldahl method, crude lipid content was determined by the Soxhlet extraction method, and crude ash content was determined by the burning method in a muffle furnace at 550°C. The intestinal samples were homogenized in an ice-water bath and centrifuged at 2,500 r/min for 10 min, and the supernatant was taken for the determination of digestive enzymes. Commercial kits (Nanjing JianCheng Bioengineering Institute, Nanjing, China) were used to measure the contents of serum glucose (GLU), total cholesterol (TC), triacylglycerols (TG), urea nitrogen (BUN), and malondialdehyde (MDA) as well as activities of alkaline phosphatase (ALP), glutamic pyruvic transaminase (GPT), glutamic oxaloacetic transaminase (GOT), catalase (CAT), and superoxide dismutase (SOD) (Guo et al., 2023b). ELISA kit (Nanjing Jiancheng Institute of Biological Engineering, Nanjing, China) was used to measure the contents of complement C3, complement C4, and immunoglobulin M (IgM) as well as the activities of protease (PRS), amylase (AMS), and lipase (LPS). The experimental procedures were carried out according to the manufacturer’s instructions.
2.6 Tissue section preparation and observation
At the end of the aquaculture experiment, after 24 h of fasting, three grass carp were randomly selected from each pond, dissected, sampled for liver and intestines, and fixed with 4% paraformaldehyde for 24 h. The slices were routinely dehydrated with alcohol, transparent with xylene, dipped in wax, embedded, sliced, and sealed with H&E staining (Nanjing Jiancheng Institute of Biological Engineering, Nanjing, China). Three non-continuous sections were selected from each group and sent to Wuhan Servicebio Technology Co., Ltd., for section scanning. After scanning, the sections were analyzed by using the Case Viewer image analysis system. The same field of view was selected for each section to observe the tissue structure of the liver and intestine of the grass carp at ×100 and ×400, respectively, and then photographed.
The ×100 image is observed through a combination of ×10 eyepiece and ×10 objective lens; the ×400 image is observed through a combination of ×4 eyepiece and ×100 objective lens.
2.7 Data calculation and analysis
One-way analysis of variance (ANOVA) was performed using Microsoft Excel 2007 and SPSS 19.0 software, and differences between groups were evaluated with the Duncan multiple-comparison test. Data are expressed as mean ± standard deviation (SD), and P ≤ 0.05 was considered a significant difference.
3 Results
3.1 Effects of short-term low-dose AFB1 on growth performance and body composition of grass carp
After short-term feeding of grass carp with low-dose AFB1 feed, its weight gain rate (WGR) and specific growth rate (SGR) showed a certain upward trend, and the WGR in the D2 group was significantly higher than that in control group (CD) (P < 0.05); the feed coefficient showed a downward trend with the increase of AFB1 content, with the lowest performance in the D2 group (P < 0.05). There was no significant difference in terms of body fatness (CF) (P > 0.05) among the groups (Figure 1).
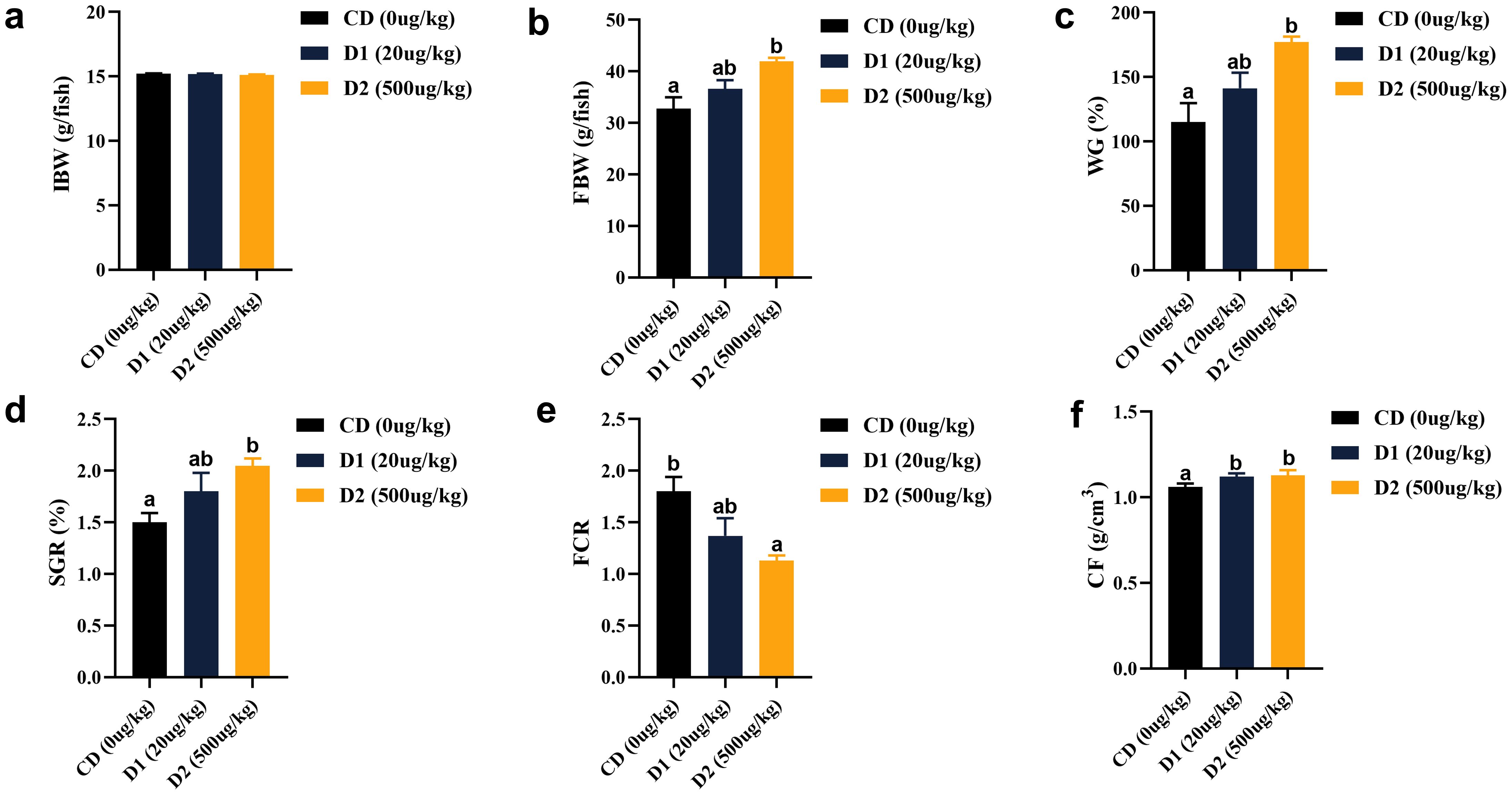
Figure 1. (A–F) Effects of short-term low-dose AFB1 on growth performance and physical indicators of grass carp. The different letters (a, b) indicate significant differences (P < 0.05) among groups.
Short-term low-dose AFB1 had no effect on the contents of crude protein, moisture, and ash in fish (P > 0.05). Crude lipid was significantly lower in the D1 and D2 groups than in the CD group, and there was no significant difference in groups D1 and D2 (P > 0.05) (Figure 2).
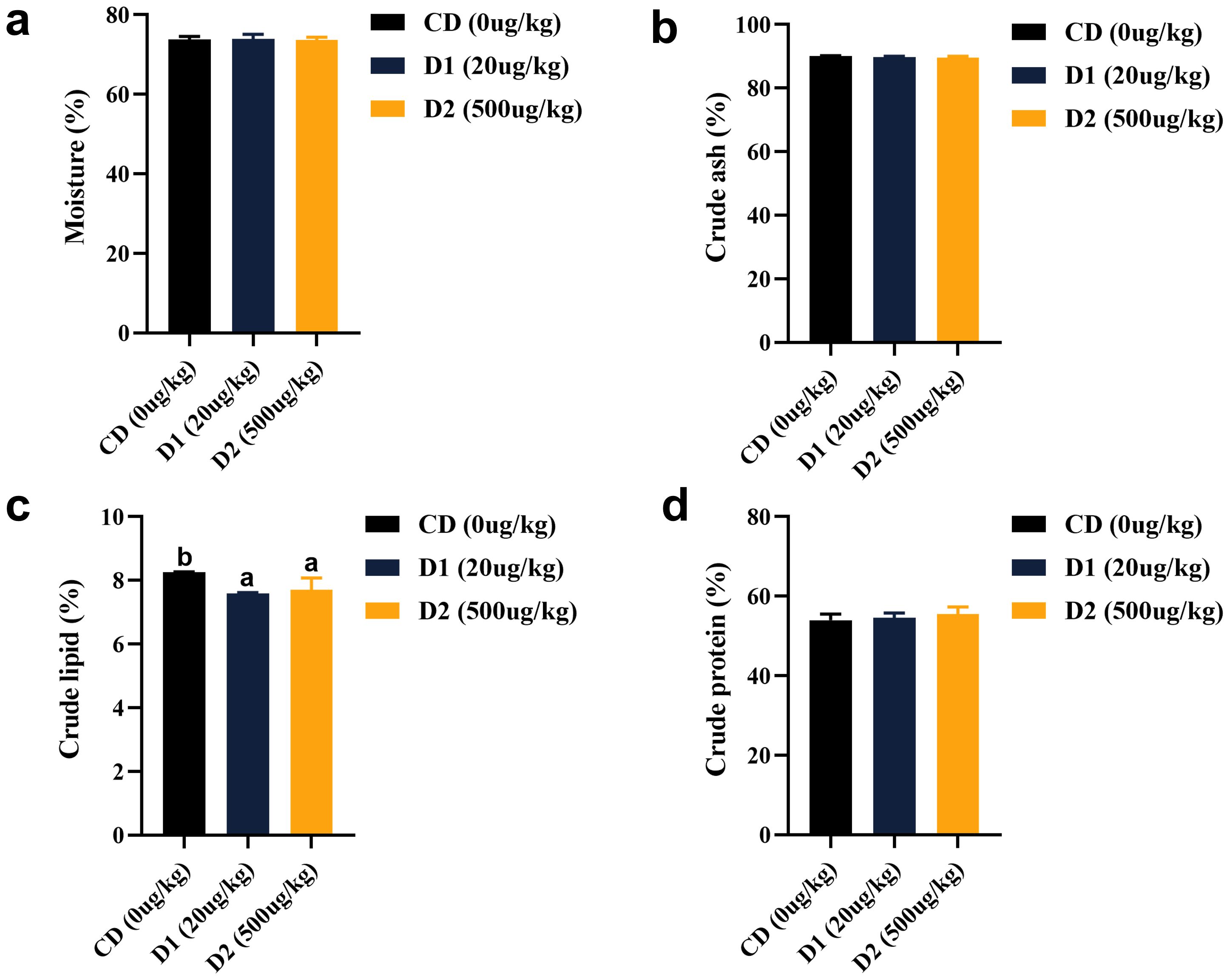
Figure 2. (A–D) Effects of short-term low-dose AFB1 on body composition of grass carp. The different letters (a, b) indicate significant differences (P < 0.05) among groups.
3.2 Effects of short-term low-dose AFB1 on antioxidant and nonspecific immunity of grass carp
It can be seen from Figure 3 that grass carp fed with D1 and D2 diets showed significant stress response and significantly higher blood glucose (GLU) levels than those fed with CD diets after short-term consumption of feed (P < 0.05). There was no significant difference in catalase (CAT) activity among the groups (P > 0.05), but superoxide dismutase (SOD) showed a trend of increasing first and then decreasing with the content of AFB1. The activity was the highest in the D1 group (P < 0.05); the content of malondialdehyde (MDA) had no significant difference between the D1 group and the CD group (P > 0.05), but it was highest in the D2 group (P < 0.05). In terms of non-specific immunity, the activities of C3 and C4 in the D2 group were significantly lower than those in the control group (P < 0.05), the activity of C3 in the D1 group was no different from that in the CD group (P > 0.05) while it was significantly higher than the D2 group (P < 0.05); the C4 activity in the D1 group was no different from the D2 group (P > 0.05) but significantly lower than the CD group (P < 0.05); and the activity of immunoglobulin M (IgM) increased significantly as the content of AFB1 decreased, the lowest in the D2 group (P < 0.05).
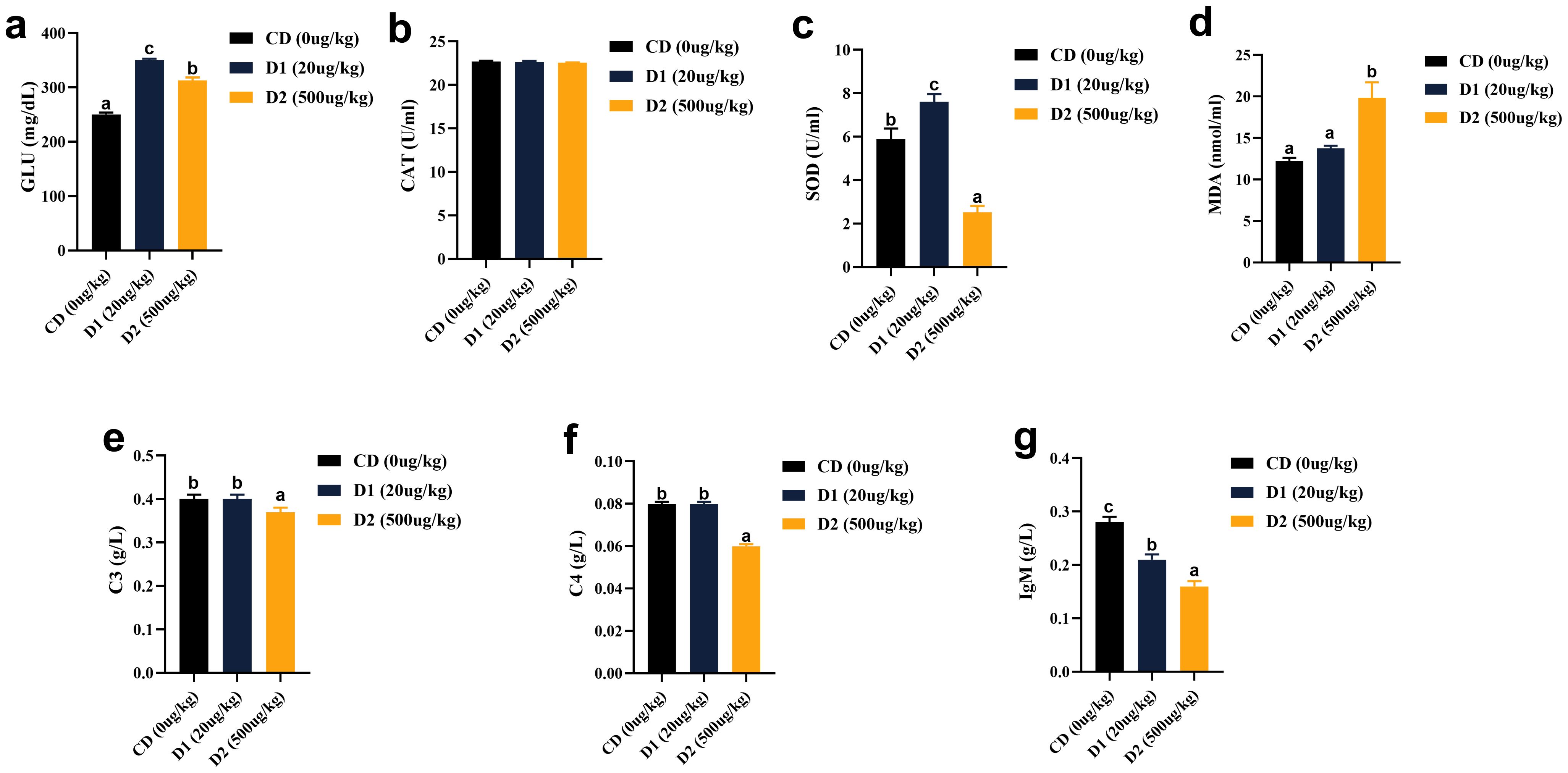
Figure 3. (A–G) Effects of short-term low-dose AFB1 on non-specific immunity indexes of grass carp in serum. The different letters (a, b, c) indicate significant differences (P < 0.05) among groups.
3.3 Effects of short-term low-dose AFB1 on liver structure and function of grass carp
The liver-to-body ratio (HSI) and visceral-to-body ratio (VSI) of the D1 and D2 groups were significantly higher than those of the CD group (P < 0.05), and there was no significant difference between the D1 and D2 groups (P > 0.05) (Figure 4). The liver tissue structure of grass carp fed the D1 and D2 diets for a short period of time showed significant structural damage compared to the CD group (Figure 5), and the extent of damage increased with the increase of AFB1 concentration.
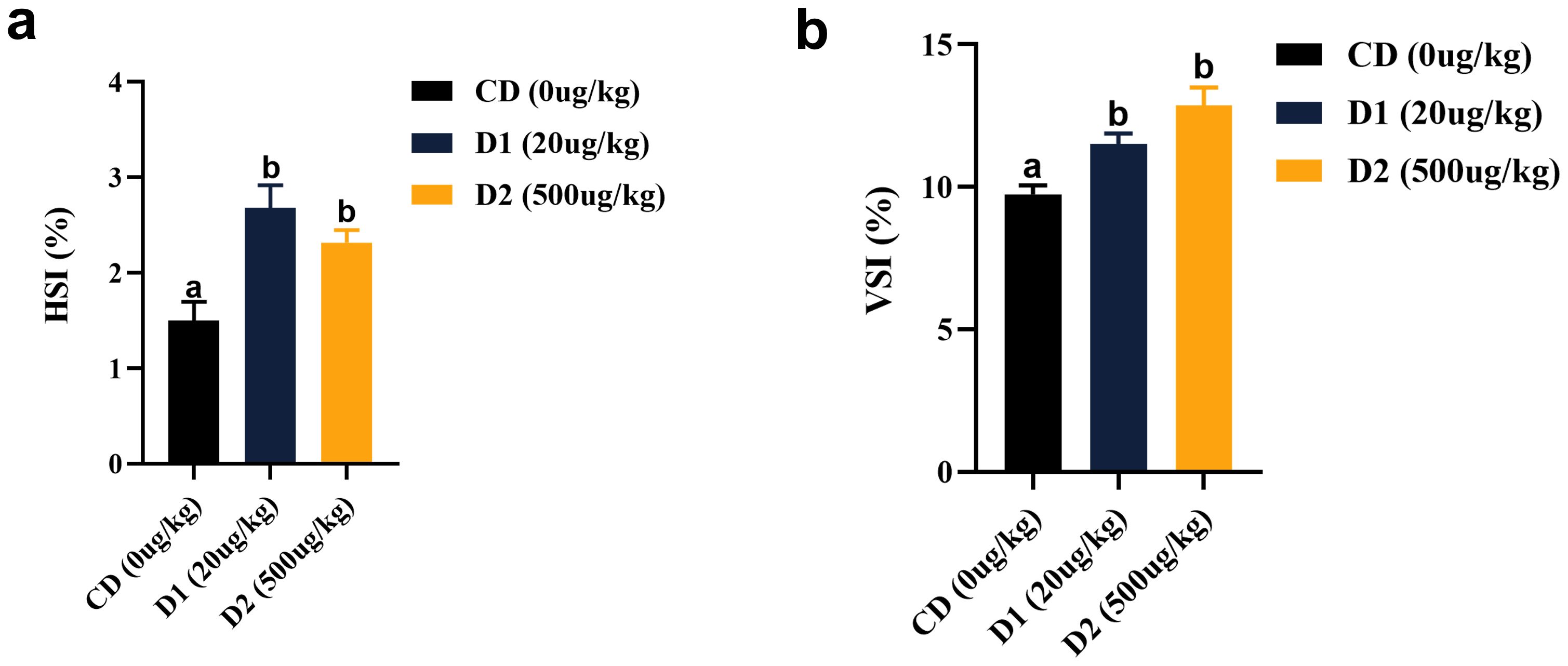
Figure 4. (A, B) Effects of short-term low-dose AFB1 on viscera indexes of grass carp. The different letters (a, b) indicate significant differences (P < 0.05) among groups.
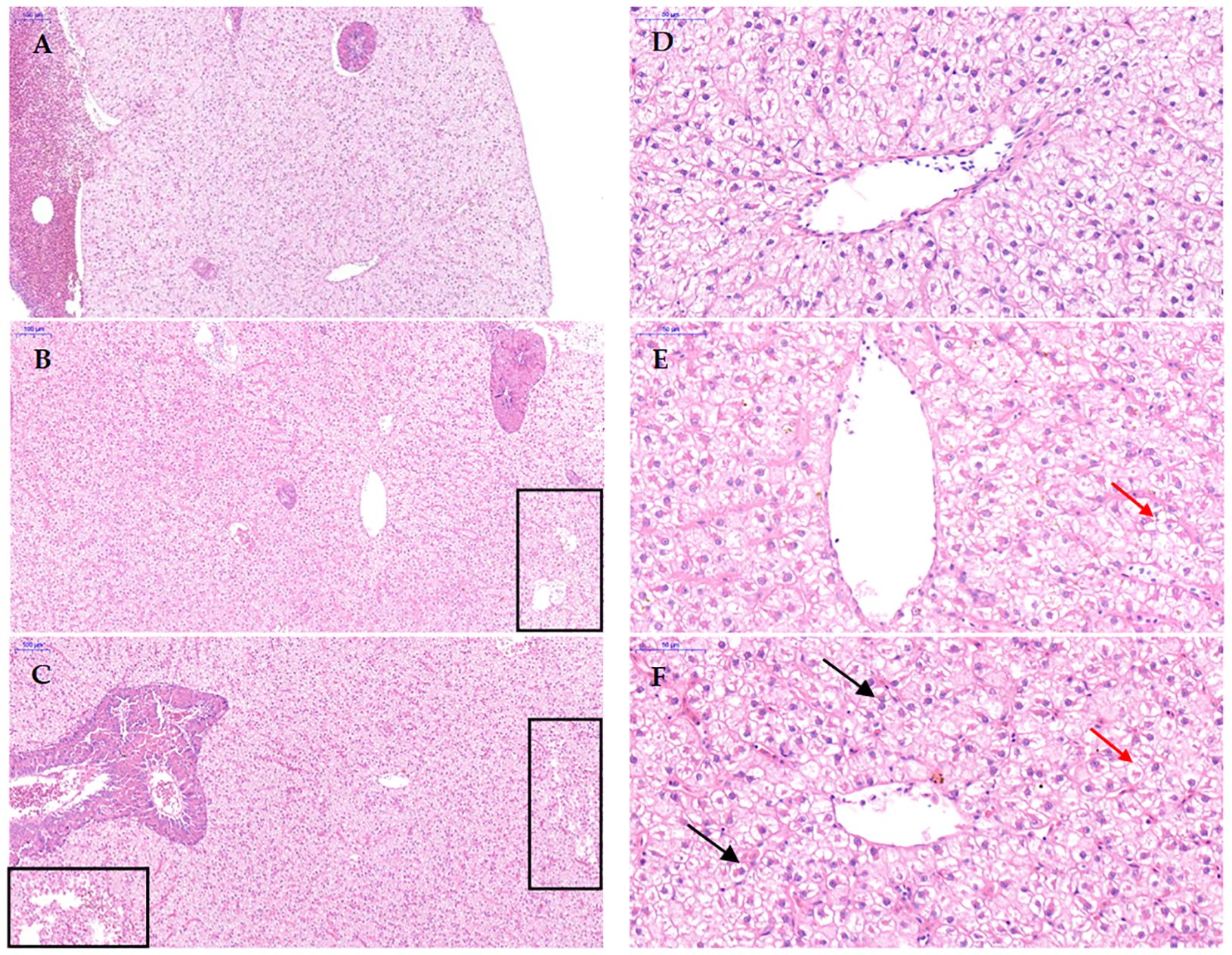
Figure 5. Effect of short-term low-dose AFB1 on the liver tissue structure of grass carp. (A, D) ×100 and ×400 images of the liver tissue of the control group (0 ug/kg), respectively. (B, E) ×100 and ×400 images of the liver tissue of the D1 group (20 ug/kg), respectively. (C, F) ×100 and ×400 images of the liver tissue of the D2 group (500 ug/kg), respectively. The boxes indicate that the liver tissue has loose interstitialization. The black arrows indicate hepatocyte nuclear translocation. The red arrows indicate hepatocyte vacuolization.
In terms of liver function, after short-term ingestion of D1 and D2 group feeds, liver total cholesterol (TC), triglyceride (TG), urea nitrogen (BUN), alanine aminotransferase (GPT), aspartate aminotransferase (GOT), and alkaline phosphatase (ALP) were significantly higher than those in the CD group (P < 0.05), and the contents of ALP, GPT, and GOT were the highest in D2 group (P < 0.05); the contents of TBA in the D1 and D2 groups were significantly lower than CD (P < 0.05) (Figure 6).
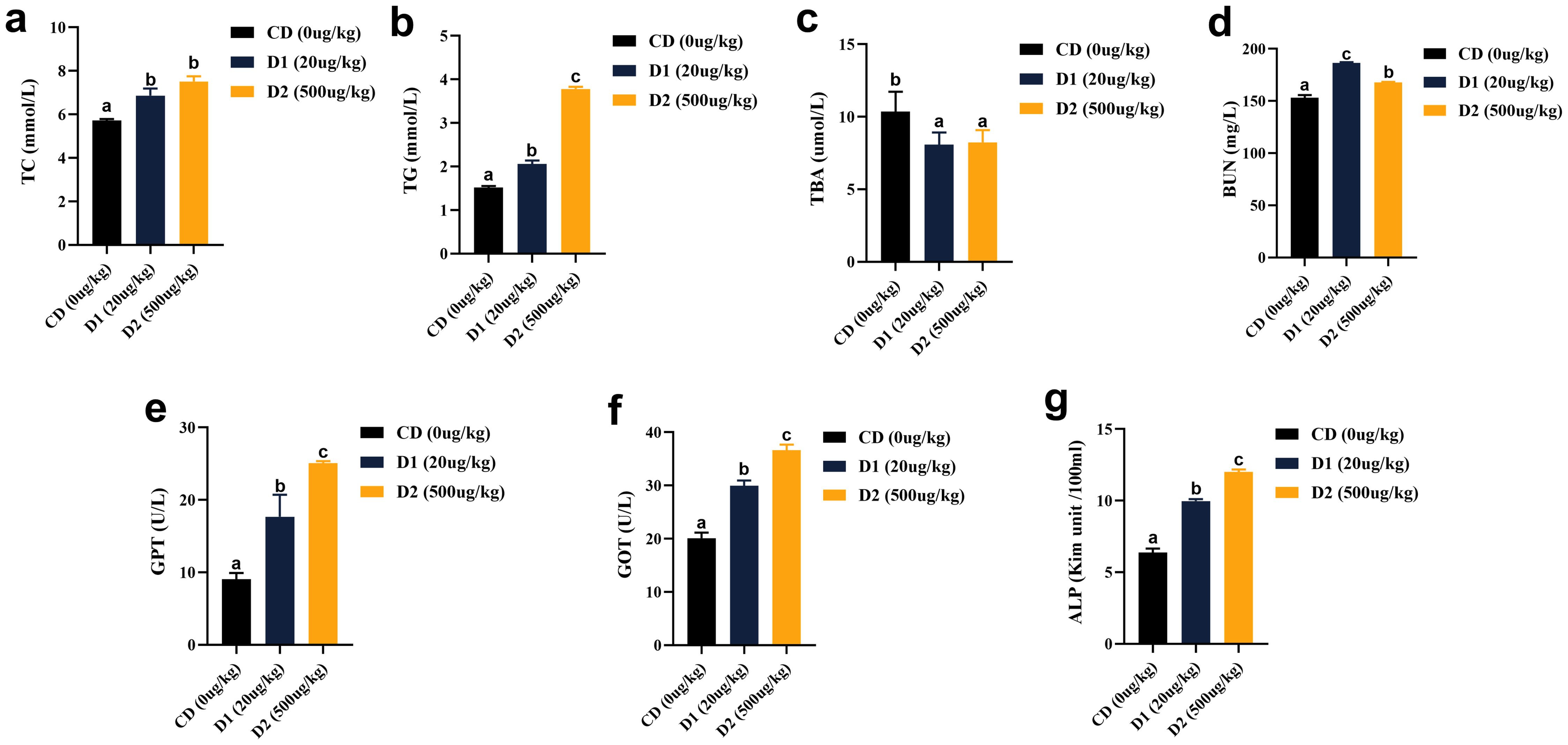
Figure 6. (A–G) Effects of short-term low-dose AFB1 on biochemical indexes of liver function of grass carp. The different letters (a, b, c) indicate significant differences (P < 0.05) among groups.
3.4 Effects of short-term low-dose AFB1 on intestinal structure and function of grass carp
Compared with the CD group, the D1 group had little effect on the intestinal structure of grass carp; however, the D2 group had significantly higher intestinal villus height, crypt fossa depth, villus–crypt fossa ratio, and goblet cell count than the CD group (P < 0.05) (Figures 7, 8).
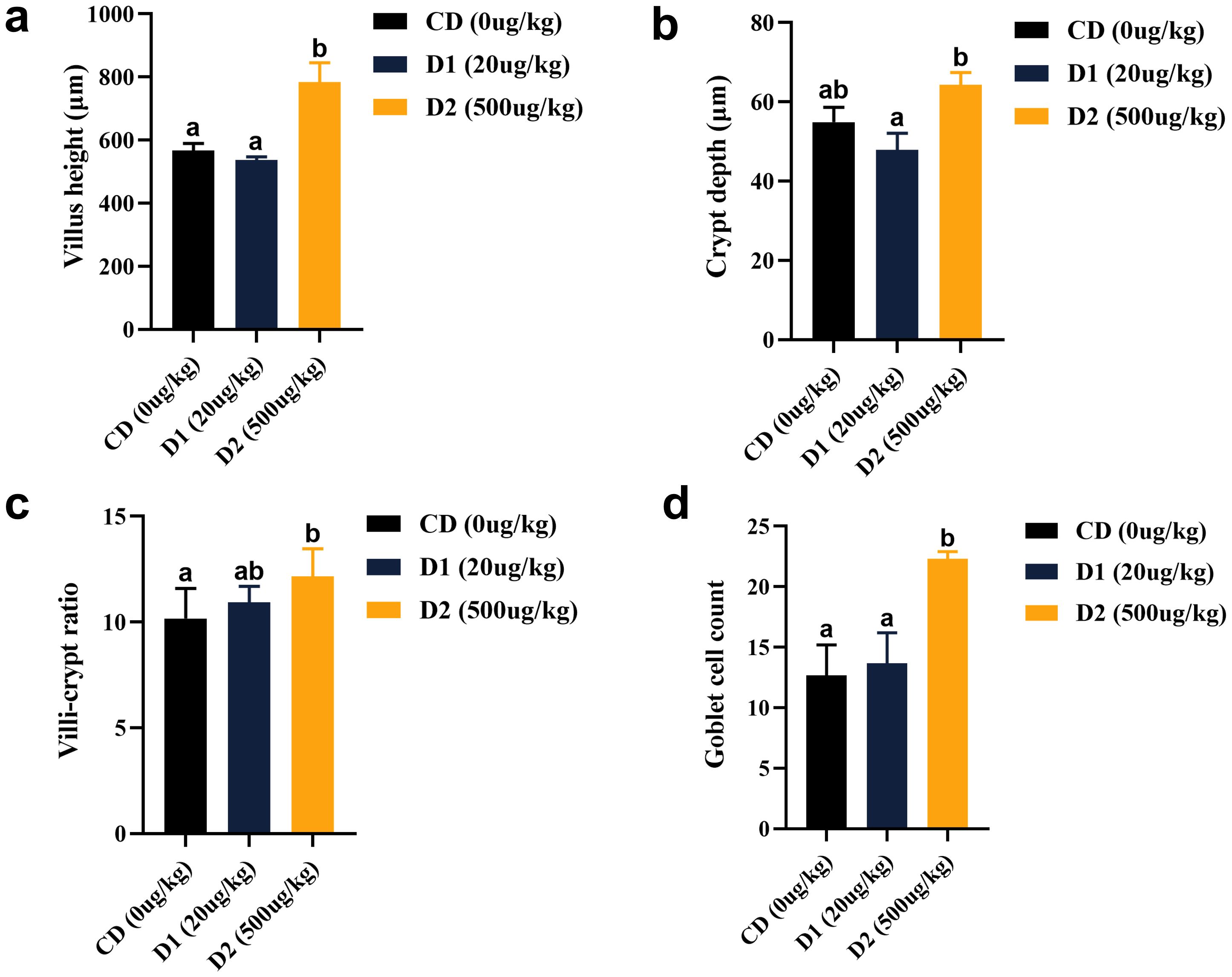
Figure 7. (A–D) Effect of short-term low-dose AFB1 on the intestinal tissue structure of grass carp. The different letters (a, b) indicate significant differences (P < 0.05) among groups.
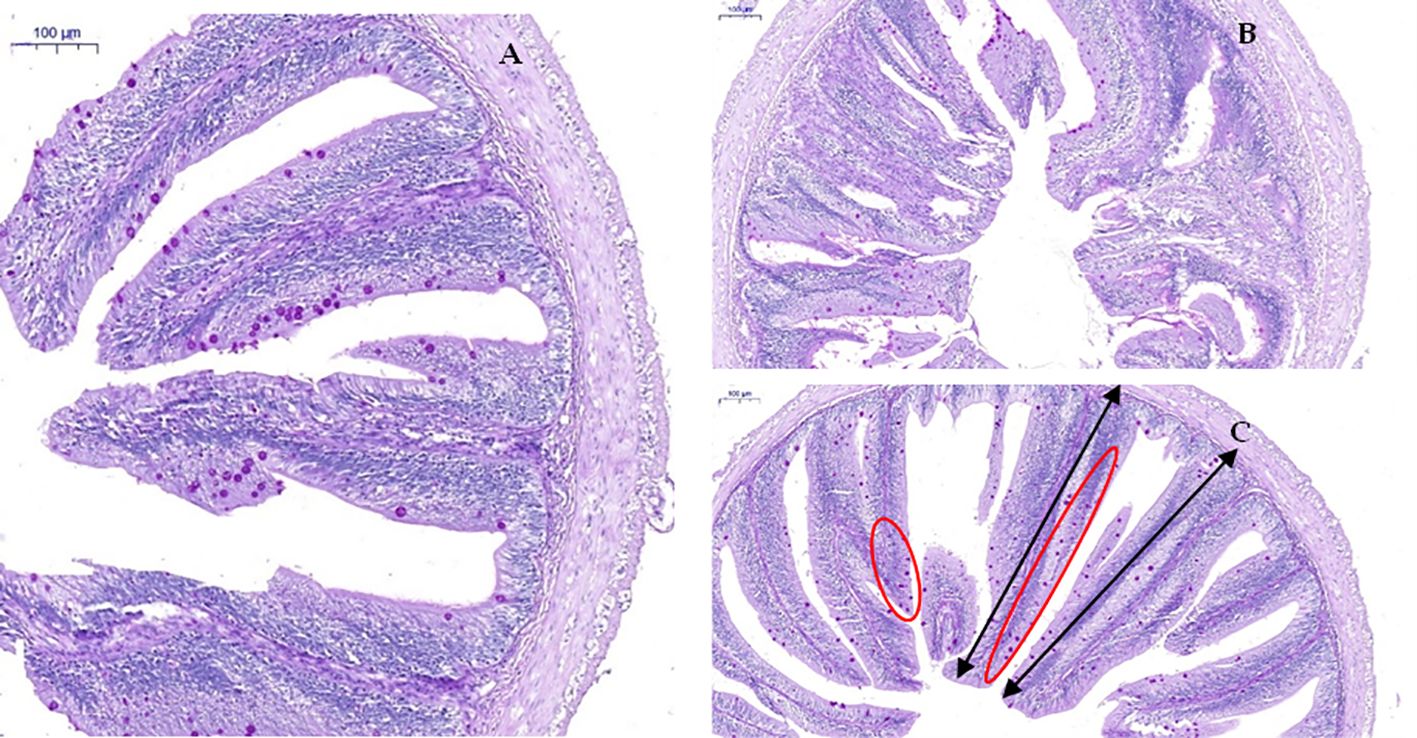
Figure 8. Effect of short-term low-dose AFB1 on the intestinal tissue structure of grass carp. (A) Control group, 0 ug/kg (×100). (B) D1 group, 20 ug/kg (×100). (C) D2 group, 500 ug/kg (×100). The black bi-directional arrows indicate the height (length) of the intestinal villus. The red oval area indicates the distribution of goblet cells on the intestinal villus.
Dietary AFB1 for a short period of time significantly affects intestinal digestive enzyme activity. The intestinal amylase (AMS) activity of grass carp showed a trend of first decreasing and then increasing, with the lowest activity in the D1 group (P < 0.05), but there was no difference between the D2 group and the CD group (P > 0.05). The activities of protease (PRS) in D1 and D2 were significantly higher than those in the CD group (P < 0.05); there was no difference in lipase (LPS) activity between the D1 group and the CD group (P > 0.05), and the highest activity was in the D2 group (P < 0.05) (Figure 9).
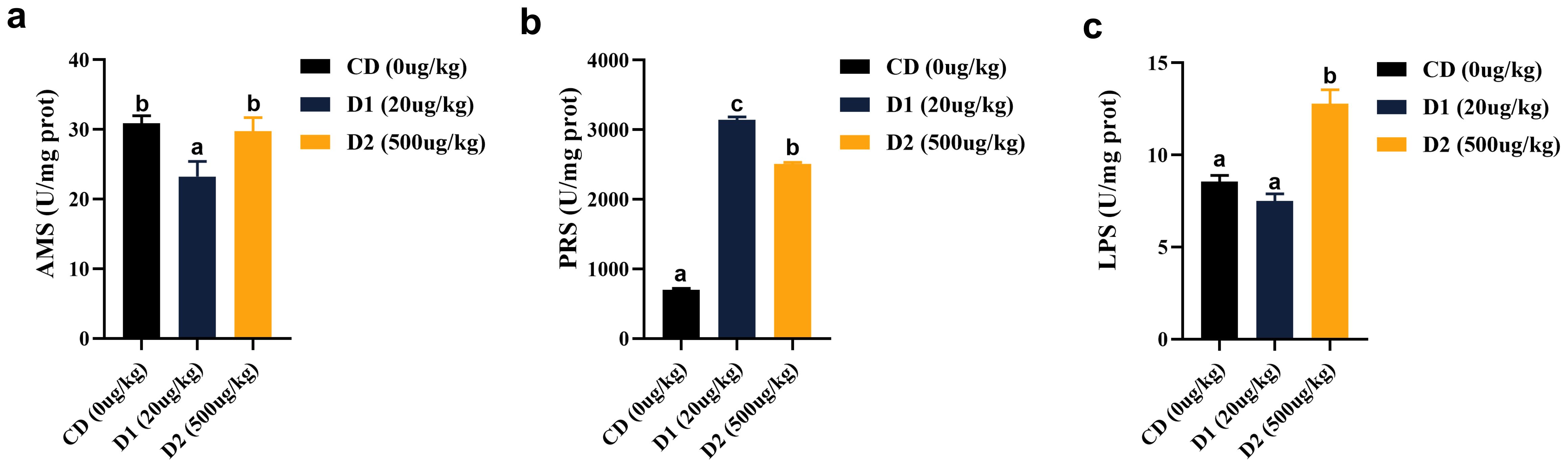
Figure 9. (A–C) Effects of short-term low-dose AFB1 on intestinal digestive enzymes of grass carp. The different letters (a, b, c) indicate significant differences (P < 0.05) among groups.
4 Discussion
The research on hormesis effect has largely forced on comparative physiology (Raymond and Giancarlo, 2020), most of which have been characterized by environmental radiation, heavy metals, pesticides, etc. In actual production, animals are often exposed to these low-dose environmental stressors, which can affect their survival and production. It was found that abalone (H. diversicolor aquatilis) with low-level gamma rays could significantly increase its survival rate and shell length under high temperature stress (Jwa and Hong, 2020). Low concentration (Cd) improved the growth rate of Bufo americanus (Stacy and Edward, 2003) and leopard frog (Jackson et al., 2007) and also increased the size and metamorphosis rate of the Chinese toad (Ya et al., 2019). The AFB1 studied in this experiment is an environmental toxin that exists widely in the natural environment and is one of the environmental stressors that farmed animals are often exposed to. Most of the previous studies focused on the toxic effects of AFB1 on aquatic animals. Different types of aquatic animals have different tolerance levels to AFB1, and its growth toxicity performance is different. When the AFB1 content exceeded 10, 25, and 50 ug/kg, the weight gain rate and specific growth rate in Litopenaeus vannamei (Zeng et al., 2015) and giant catfish (Rui et al., 2018) were significantly reduced. Tilapia (Deng et al., 2010) and Colossoma macropomum (Nunes et al., 2019) showed a significant decrease in food intake and weight gain rate after ingesting AFB1 feeds exceeding 245 and 500 ug/kg. Cyprinids such as Carassius auratus gibelio (Huang et al., 2012) showed high tolerance to AFB1, and their food intake, specific growth rate, and feed efficiency were not significantly affected by feeding concentrations of 500 and 5,000 ug/kg AFB1. In this experiment, the growth performance of grass carp showed a certain stimulatory enhancement after short-term feeding of low-dose AFB1, and the weight gain rate (WG), specific growth rate (SGR), and feed efficiency (FE) increased with the increase of AFB1 content. The D2 group (500 ug/kg) performed the best (P < 0.05). It can be seen that grass carp has a high tolerance to AFB1. Deng et al. (2010) found that for tilapia fed a low dose of AFB1 (85 ug/kg) for 20 weeks continuously, its weight gain was better than that of the control group. This indicates that short-term low-dose AFB1 exposure may have a stimulating effect on the growth performance of some herbivorous or omnivorous fish (hormesis) and can reduce body fat deposition. This result was similar to that reported by Zeng et al. (2015) and Deng et al. (2010). This corroborates with the conclusion that the intestinal digestive and absorptive functions of grass carp were stimulatingly enhanced after ingesting low doses of AFB1 as shown in the following section of this experiment. The enhancement of intestinal digestion and absorption function was conducive to the accelerated absorption and utilization of feed nutrients by the fish, thus showing a more obvious growth advantage.
Another important manifestation of hormesis is to stimulate the adaptive response mechanism, activate endogenous antioxidants, improve cell defense capabilities, and protect the body. The mechanism of action is similar to the hypothesis of oxidative stress (POS) (Marcelo et al., 2015; Maximiliano et al., 2019).
This experiment found that the antioxidant capacity of grass carp showed a certain hormesis effect after a short-term consumption of low-dose AFB1: the serum GLU content of grass carp in D1 and D2 groups was significantly increased, indicating that an obvious stress response occurred in ≥20 ug/kg AFB1. With the increase of the dose of AFB1, the SOD activity decreased significantly, and the MDA content increased significantly, which indicated that the toxic stimulation of 500 ug/kg AFB1 exceeded the carrying capacity of the body’s homeostasis adaptive repair, resulting in an imbalance in the endogenous antioxidant enzyme system and an increase in the content of oxidized products. The results were similar to those of Wang et al. (2012).
The complement system is an important part of the fish immune system and involved in fish-specific and non-specific immune responses (Wang et al., 2008). This experiment found that the non-specific immune system of grass carp was affected after a short-term consumption of low-dose AFB1 (20 ug/kg). Complement C4, as the precursor to activate C3, was first affected (Wang et al., 2008), and its activity was significantly lower than that of the control group, while the activity of C3 was not significantly different from the control group. With the increase of AFB1 dose (D2, 500 ug/kg), the activities of C3 and C4 were significantly decreased. Immunoglobulin M (IgM) is the largest antibody in humoral immunity, and it is also the earliest antibody in the body’s primary immune response. The experimental results showed that the IgM content in the blood of grass carp was significantly reduced after ingesting AFB1, and the higher the dose of AFB1, the lower the IgM content.
The antioxidant defense system in the body includes both endogenous antioxidant system and non-specific immune system. From the above-mentioned results, short-term low-dose AFB1 can produce a certain hormesis effect on the endogenous antioxidant system of grass carp and stimulate the body’s antioxidant capacity, but it shows obvious toxic damage to the body’s non-specific immune system at the same time.
The liver is the predominant site for AFB1 metabolism in the body. AFB1 is absorbed and metabolized by hepatic cytochrome P450 oxidase and converted into aflatoxin M1 (AFM1), aflatoxin P1 (AFP1), aflatoxin Q1 (AFQ1), aflatoxin (AFL), etc. AFL is further oxidized to carcinogen AFB-8,9-epoxide (AFB-8,9-epoxide, AFBO) (Valsta et al.,1988), which results in liver damage.
A large number of studies have shown that ingestion of AFB1 in aquatic animals can cause abnormal liver morphology (Deng et al., 2010; Rui et al., 2018), liver function disorders (El-Sayed and Riad, 2009; Deng et al., 2010; Selim et al., 2014; Zeng et al., 2015; Rui et al., 2018), and tissue structural lesions (Deng et al., 2010; Wang et al., 2012; Arana et al., 2014; Nunes et al., 2019). Zeng et al. (2015) found that addition of AFB1 significantly altered serum GPT, GOT, total antioxidant capacity (T-AOC), and glutathione S-transferase (GST) activities as well as cholesterol (CHO) levels in shrimp. Pangasius hypophthalmus (Rui et al., 2018) fed 50-, 100-, and 250-μg/kg AFB1 diets had higher GPT and GOT activities, indicating liver damage. Arana et al. (2014) found that aflatoxins can cause chronic liver damage and tissue fibrosis in rainbow trout. This experiment found that the activities of serum GPT, GOT, and ALT of grass carp fed with low-dose AFB1 (D1 and D2) diet for a short period of time were significantly higher than those in the control group (CD) and increased with the increase in the dose of AFB1 (P < 0.05). The visceral body ratio (VSI) was significantly higher than the control group (CD). The above-mentioned results indicated that short-term consumption of low-dose AFB1 caused obvious toxic damage to the liver function of grass carp, and the degree of damage was positively correlated with the exposure dose of AFB1, and the conclusion was basically consistent with the research results of El-Sayed and Riad (2009) and Rui et al. (2018).
Another important biological function of the liver is to participate in the synthesis and metabolism of body fat and protein. In this study, results suggested that after a short-term consumption of low-dose AFB1 feed (D1 and D2), the serum total cholesterol (TC), triglyceride (TG), and the content of urea nitrogen (BUN) were significantly higher than those of the control group (CD), which indicated that the disorder of liver function led to the significant inhibition of the synthesis of body fat and protein, and the bile acid (TBA) secreted by the liver was significantly reduced, which then affected the digestion and absorption of lipids in the intestines. This result was consistent with the above-mentioned results of body fat deposition. In addition to causing abnormal liver morphology and function disorder, AFB1 also causes liver tissue lesions. Through histological observation of the livers of the experimental fish in each group, it was found that the number of vacuoles in the liver tissue structure of grass carp fed diets containing AFB1 (D1 and D2) increased, and the liver structural damage, such as cell atrophy and deformation, irregular and obvious displacement of nuclei, blurred and incomplete cell membrane boundaries, and evolution of tissue structure from dense type to loose gap type, and the degree of damage aggravated with the increase of AFB1 dose.
The intestine is another organ site outside the liver where AFB1 and its metabolites directly act. Studies have shown that AFB1 can cause toxic damage to the animals’ intestinal structure, shorten and thin the intestinal villi (Awad et al., 2004), reduce the duodenum mucosal thickness and intestinal villus height (Song, 2012), and affect intestinal function. However, low-dose AFB1 (D1, 20 ug/kg) did not show a significant effect on the intestinal structure of grass carp. In this study, structures such as intestinal villus height, crypt fossa depth, and villus–crypt fossa ratio were significantly better than those of the control group, and the contents of digestive enzymes such as trypsin and lipase in the intestines were also significantly enhanced. This indicates that after a short-term consumption of low-dose AFB1 in grass carp, the body promotes the proliferation of intestinal epithelial cells through endogenous regulation, increases the surface area of villi, and strengthens the secretion of digestive enzymes. The digestion and absorption of exogenous nutrients in the intestine are promoted in order to store material energy for the body to jointly cope with the toxic damage of AFB1, as a large amount of substances and energy are required to resist AFB1 toxicity. This is a high degree of coordination between intestine structure and function, which echoes the performance of the feed coefficient in this experiment.
Intestine, the main place for digestion and absorption of nutrients, is also the body’s first barrier against damage (Lei et al., 2011). Qi et al. (2017) found that AFB1 severely affected the barrier function of the intestinal mucosa of Litopenaeus vannamei. Goblet cells are important cells that synthesize and secrete mucin, form a mucosal barrier, and participate in intestinal immune protection. This study found that 500 ug/kg ABF1 (group D2) significantly increased the number of thrush cells in the intestinal villi of grass carp, suggesting that when the dose of AFB1 reaches 500 ug/kg, the body’s own immune system activates the differentiation and proliferation of thrush cells and increases the secretion of mucin, which strengthens the barrier function of the intestinal mucosa against more toxins entering the body.
5 Conclusions
In summary, short-term low-dose AFB1 (20 ug/kg) has a certain stimulating and enhancing effect on the growth performance and antioxidant capacity of grass carp, while with the increase of AFB1 dose (500 ug/kg), the non-specific immune system is significantly damaged by toxicity. At the same time, the body’s self-regulation ability is further activated, and the digestive and absorption functions of the intestine and the barrier function of the intestinal mucosa are strengthened. However, the liver shows impaired function, inhibited fat and protein synthesis, and damaged tissue structure.
Data availability statement
The original contributions presented in the study are included in the article/supplementary material. Further inquiries can be directed to the corresponding authors.
Ethics statement
The animal study was approved by the Biomedical Research Ethics Committee of Hunan Agricultural University (approval no. 2023-118). The study was conducted in accordance with the local legislation and institutional requirements.
Author contributions
J-lL: Writing – review & editing, Writing – original draft, Conceptualization. J-rG: Writing – review & editing. PW: Writing – review & editing. J-zZ: Writing – review & editing, Investigation, Formal analysis. Z-gH: Writing – review & editing, Investigation, Formal analysis. J-lW: Writing – review & editing, Project administration, Conceptualization. YH: Writing – review & editing, Conceptualization.
Funding
The author(s) declare that financial support was received for the research, authorship, and/or publication of this article. This study was funded by the National Key Research and Development Plan Program of China (2023YFD2401605) and the Hunan Provincial Key Research and Development Program (2020NK2016).
Conflict of interest
The authors declare that the research was conducted in the absence of any commercial or financial relationships that could be construed as a potential conflict of interest.
Publisher’s note
All claims expressed in this article are solely those of the authors and do not necessarily represent those of their affiliated organizations, or those of the publisher, the editors and the reviewers. Any product that may be evaluated in this article, or claim that may be made by its manufacturer, is not guaranteed or endorsed by the publisher.
References
Anna K., Katarzyna W., Paweł K., Małgorzata M. W. (2009). Aflatoxins: characteristics and impact on human health. Postępy Higieny i Medycyny Doświadczalnej 71, 315–327. doi: 10.5604/01.3001.0010.3816
AOAC (1997). Official methods of analysis of AOAC International. 16th ed Vol. 35 (Rockville:Choice Reviews Online), 35–0912. doi: 10.5860/choice.35-0912
Arana S., Alves V. A. F., Sabino M., Tabata Y. A., Nonogaki S., Zaidan-Dagli M. L., et al. (2014). Immunohistochemical evidence for myofibroblast-like cells associated with liver injury induced by Aflatoxin B1 in Rainbow Trout (Oncorhynchus mykiss). J. Comp. Pathol. 150, 258–265. doi: 10.1016/j.jcpa.2013.07.003
Awad W. A., Böhm J., Razzazi-Fazeli E., Hulan H. W., Zentek J. (2004). Effects of deoxynivalenol on general performance and electrophysiological properties of intestinal mucosa of broiler chickens. Poultry Sci. 83, 1964–1972. doi: 10.1093/ps/83.12.1964
Deng S. X., Tian L. X., Liu F. J., Jin S. J., Liang G. Y., Yang H. J., et al. (2010). Toxic effects and residue of aflatoxin B1 in tilapia (Oreochromis niloticus×O. aureus) during long-term dietary exposure. Aquaculture 307, 233–240. doi: 10.1016/j.aquaculture.2010.07.029
Edward J. C. (2016a). Preconditioning is hormesis part I: documentation, dose-response features and mechanistic foundations. Pharmacol. Res. 110, 242–264. doi: 10.1016/j.phrs.2015.12.021
Edward J. C. (2016b). Preconditioning is hormesis part II: how the conditioning dose mediates protection: dose optimization within temporal and mechanistic frameworks. Pharmacol. Res. 110, 265–275. doi: 10.1016/j.phrs.2015.12.020
Edward J. C., Kenneth A. B., John B. A., Michael B. P., Jonathan B., Lu C., et al. (2007). Biological stress response terminology: Integrating the concepts of adaptive response and preconditioning stress within a hormetic dose-response framework. Toxicol. Appl. Pharmacol. 222, 122–128. doi: 10.1016/j.taap.2007.02.015
Edward J. C., Linda A. B. (2001). Hormesis: U-shaped dose responses and their centrality in toxicology. Trends Pharmacol. Sci. 22, 285–291. doi: 10.1016/S0165-6147(00)01719-3
El-Sayed Y. S., Riad H. K. (2009). Toxicity, biochemical effects and residue of aflatoxin B 1 in marine water-reared sea bass (Dicentrarchus labrax L.). Food Chem. Toxicol. 47, 1606–1609. doi: 10.1016/j.fct.2009.04.008
Guo J. R., Lin J. B., Li X. S., Wang L., Song K., Lu K. L., et al. (2023a). Enhanced intestinal microflora composition and phosphorus-transportation efficiency in fast-growing spotted seabass (Lateolabrax maculatus) fed a low-phosphorus diet. Aquaculture 577, 739916. doi: 10.1016/J.AQUACULTURE.2023.739916
Guo J. R., Wang L., Song K., Lu K. L., Li X. S., Zhang C. X. (2023b). Physiological response of spotted seabass (Lateolabrax maculatus) to different dietary available phosphorus levels and water temperature: changes in growth, lipid metabolism, antioxidant status and intestinal microbiota. Antioxidants 12, 2128. doi: 10.3390/ANTIOX12122128
Huang Y., Han D., Xiao X. C., Zhu X. M., Yang Y. X., Jin J. Y., et al. (2014). Effect of dietary aflatoxin B1 on growth, fecundity and tissue accumulation in gibel carp during the stage of gonad development. Aquaculture 428-429, 236–242. doi: 10.1016/j.aquaculture.2014.03.010
Huang Y., Zhu X. M., Han D., Yang X. Y., Jin J. Y., Li H. Y., et al. (2012). Growth and aflatoxin B_1 accumulation of gibel carp adult fed with diets of different levels of aflatoxin B1. Acta Hydrobiologica Sin. 36, 817–825. doi: 10.3724/SP.J.1035.2012.00817
Jackson A. G., Chen T. H., William H. K. (2007). Lethal and sublethal effects of chronic cadmium exposure on northern leopard frog (Rana pipiens) tadpoles. Environ. Toxicol. Chem. 26, 1192–1197. doi: 10.1897/06-479R.1
Jwa M. S., Hong C. Y. (2020). Physiological Responses of Intrinsic Small Abalone Haliotis diversicolor aquatilis under High Temperature Stress by Low Level 60CO Gamma Irradiation-Mediated Hormetic Effect. J. Mar. Sci. Eng. 8, 906–916. doi: 10.3390/jmse8110906
Katherine E. Z., Camilo P., Travis M., Amelia R., Michael H., Alejandro B., et al. (2013). The effect of NovaSil dietary supplementation on the growth and health performance of Nile tilapia (Oreochromis niloticus) fed aflatoxin-B1 contaminated feed. Aquaculture 376-379, 117–123. doi: 10.1016/j.aquaculture.2012.11.020
Lei X. Y., Chen D. W., Mao X. B., Mao Q., Chen H., Zhang L., et al. (2011). Effects of maize contaminated with naturally-occuring mycotoxins on intestinal microecology in Piglets. Chin. J. Anim. Nutr. 23, 1790–1799. doi: 10.3969/j.issn.1006-267x.2011.10.020
Marcelo H. L., Daniel C. M., Georgina A. R. I., Maximiliano G. B., Thiago C. G. M., Élida G. C. (2015). Preparation for oxidative stress under hypoxia and metabolic depression: Revisiting the proposal two decades later. Free Radical Biol. Med. 89, 1122–1143. doi: 10.1016/j.freeradbiomed.2015.07.156
Márcio A. G., Daniel C. M., Giancarlo L. M., Marina M., Marlize F. C., Juan M. C. F., et al. (2020). Commentary: Ultraviolet radiation triggers “preparation for oxidative stress” antioxidant response in animals: Similarities and interplay with other stressors. Comp. Biochem. Physiology Part A 239, 110585. doi: 10.1016/j.cbpa.2019.110585
Maximiliano G. B., Georgina A. R. I., Daniel C. M., Thorsten B., Alfredo C. V., Juan M. C. F., et al. (2019). Twenty years of the ‘Preparation for oxidative Stress’ (POS) theory: ecophysiological advantages and molecular strategies. Comp. Biochem. Physiol. Part A 234, 36–49. doi: 10.1016/j.cbpa.2019.04.004
Mohamed Salah A., Ahmed Mohamed N. A., Adham Abdullah A. S., Abd El-Aziz Mohamed E. H. (2018). Effect of some safe feed additives on growth performance, blood biochemistry, and bioaccumulation of aflatoxin residues of Nile tilapia fed aflatoxin-B1 contaminated diet. Aquaculture 495, 27–34. doi: 10.1016/j.aquaculture.2018.05.030
Nunes E. M.C.G., Pereira. M. M. G., Costa. A. P. R., Araripe. M. N. B. A., Calvet. R. M., Pereyra. C. M., et al. (2019). Effects of aflatoxin B 1 on performance and health of tambaqui fingerlings (Colossoma macropomum). Int. Aquat. Res. 11, 73–83. doi: 10.1007/s40071-019-0220-2
Qi C. C., Wang B. J., Liu M., Jiang K. Y., Zhao W., Ren C. H., et al. (2017). Effects of short-term addition of aflatoxin B1(AFB1) on the intestinal mucosal barrier of Litopenaeus vannamei. J. Fisheries China 41, 1936–1945. doi: 10.11964/jfc.20161010594
Raymond B., Giancarlo L. M. (2020). A dose of experimental hormesis: When mild stress protects and improves animal performance. Comp. Biochem. Physiology Part A 242, 110658. doi: 10.1016/j.cbpa.2020.110658
Rui A. G., Tu C., Nguyen N. T., Gonçalo A. S., Pedro E., Le T. H. (2018). Aflatoxin B1 (AFB1) reduces growth performance, physiological response, and disease resistance in Tra catfish (Pangasius hypophthalmus). Aquaculture Int. 26, 921–936. doi: 10.1007/s10499-018-0259-x
Sahoo P. K., Mukherjee S. C. (2003). Immunomodulation by dietary vitamin C in healthy and aflatoxin B1-induced immunocompromised rohu (Labeo rohita). Comp. Immunology Microbiol. Infect. Dis. 26, 65–76. doi: 10.1016/S0147-9571(01)00038-8
Selim K. M., El-hofy H., Khalil R. H. (2014). The efficacy of three mycotoxin adsorbents to alleviate aflatoxin B1-induced toxicity in Oreochromis niloticus. Aquaculture Int. 22, 523–540. doi: 10.1007/s10499-013-9661-6
Song H. G. (2012). Effects of Aflatoxin B1 on the digestive tract structure and function, blood biochemical parameters and production performance of Meat Duck (Changsha: Hunan Agriculture University).
Southam C. M., Ehrlich J. (1943). Effects of extracts of western red-cedar heartwood on certain wood-decaying fungi in culture. Phytopathology 33, 517–524.
Stacy M. J., Edward E. L. (2003). The effects of chronic cadmium exposure on American toad (Bufo americanus) tadpoles. Environ. Toxicol. Chem. 22, 377–380.
Valsta L. M., Hendricks J. D., Bailey G. S. (1988). The significance of glutathione conjugation for aflatoxin B1 metabolism in rainbow trout and coho salmon. Food Chem. Toxicol. 26, 129–135. doi: 10.1016/0278-6915(88)90109-3
Wang J., Guo R., Su L., Xia H., Cui M. (2012). Toxic effects of aflatoxin B1 on growth performance, biochemical and hepatopancreas microstructure of Litopanaeus vannamei. J. Fisheries China 36, 952–957. doi: 10.3724/SP.J.1231.2012.27844
Wang Z. P., Zhang S. F., Wang G. F. (2008). Advances on the complement components characteristic and function of complement system in fish. Acta Hydrobiologica Sin. 32, 760–769. doi: 10.3724/SP.J.0000.2008.50760
Ya J., Ju Z. Q., Wang H. Y., Zhao H. F. (2019). Exposure to cadmium induced gut histopathological damages and microbiota alterations of Chinese toad (Bufo gargarizans) larvae. Ecotoxicology Environ. Saf. 180, 449–456. doi: 10.1016/j.ecoenv.2019.05.038
Yu Y. Y., Niu J., Yin P., Mei X. T., Liu Y. J., Tian L. X., et al. (2018). Detoxification and immunoprotection of Zn (II)-curcumin in juvenile Pacific white shrimp (Litopenaeus vannamei) feed with aflatoxin B1. Fish Shellfish Immunol. 80, 480–486. doi: 10.1016/j.fsi.2018.05.034
Zeng S. L., Long W. Q., Tian L. X., Xie S. W., Chen Y. J., Yang H. J., et al. (2015). Effects of dietary aflatoxin B1 on growth performance, body composition, haematological parameters and histopathology of juvenile Pacific white shrimp (Litopenaeus vannamei). Aquaculture Nutr. 22, 1152–1159. doi: 10.1111/anu.12331
Keywords: aflatoxin B1, Ctenopharyngodon idellus, growth performance, nonspecific immunity, organizational structure
Citation: Li J-l, Guo J-r, Wang P, Zhang J-z, He Z-g, Wang J-l and Hu Y (2024) Assessment of the hormesis effect and toxic damage of short-term low-dose aflatoxin B1 in grass carp (Ctenopharyngodon idellus). Front. Mar. Sci. 11:1451204. doi: 10.3389/fmars.2024.1451204
Received: 18 June 2024; Accepted: 25 July 2024;
Published: 20 August 2024.
Edited by:
Songlin Li, Shanghai Ocean University, ChinaReviewed by:
Xuexi Wang, Fujian Agriculture and Forestry University, ChinaMin Jin, Ningbo University, China
Lefei Jiao, Ningbo University, China
Copyright © 2024 Li, Guo, Wang, Zhang, He, Wang and Hu. This is an open-access article distributed under the terms of the Creative Commons Attribution License (CC BY). The use, distribution or reproduction in other forums is permitted, provided the original author(s) and the copyright owner(s) are credited and that the original publication in this journal is cited, in accordance with accepted academic practice. No use, distribution or reproduction is permitted which does not comply with these terms.
*Correspondence: Jin-long Wang, d2psNzUwMkAxMjYuY29t; Yi Hu, aHV5aTc0MDMyMkAxNjMuY29t