- 1Research and Development Center for Watershed Environmental Eco-Engineering, Advanced Institute of Natural Sciences, Beijing Normal University, Zhuhai, China
- 2Key Laboratory of Coastal Water Environmental Management and Water Ecological Restoration of Guangdong Higher Education Institutes, Beijing Normal University, Zhuhai, China
- 3Yellow River Estuary Wetland Ecosystem Observation and Research Station, Ministry of Education, Dongying, China
- 4Technical Management Center, Dongjiang Environmental Company Limited, Shenzhen, China
- 5State Key Laboratory of Water Environmental Simulation, School of Environment, Beijing Normal University, Beijing, China
- 6Dawenliu Management Station, Shandong Yellow River Delta National Nature Reserve Administration Committee, Dongying, China
Tidal inundation is a major stress in salt marshes that regulates the patterns of plant distribution and the associated functions provided by vegetation communities. Usually, frequency is used to represent inundation intensity and can be estimated using elevation. However, frequency is only a statistical indicator of tidal inundation conditions during a given period, which ignores many details of tidal inundation characteristics based on a single tidal event. On the scale of a single tidal event, duration and water depth are important characteristics for describing inundation conditions, which vary along the elevation gradient. The frequency of tidal events of a specific duration and water depth also varied. To unravel the impact of varied inundation characteristics on the key life stages of a foundation plant, we designed an experiment with varied inundation treatments of different frequencies, durations, and depths. Our results showed that the frequency, duration, and depth of inundation events significantly influenced seed emergence, seedling survival, and growth. Stress can be strengthened by a higher frequency with a longer duration and larger depth. Among these factors, frequency had a dominant impact, followed by duration and water depth. Specifically, there is a trade-off between frequency, duration, and depth, suggesting that an inundation event with shallower depth and/or shorter duration would reduce the stress from higher frequency. The findings fill a gap in the loss of details of varied inundation characteristics on plant establishment on a fine scale. Further, it will help explicit inundation stress more accurately and clearly and provide important implications for stress relief solutions in coastal ecological restoration.
1 Introduction
Coastal salt marshes are buffer zones between land and sea that are regularly or irregularly inundated by tides, and covered with herbaceous vegetation or shrubs (Adam, 1990; Friess et al., 2012). It is one of the most productive ecosystems in the world (Schubauer and Hopkinson, 1984; Bouchard and Lefeuvre, 2000; Janousek et al., 2020), providing a variety of valuable functions, such as storm protection, flood control, nutrient cycling, and carbon storage (Friess et al., 2012; Waring and Maricle, 2012; Zhao et al., 2019; Janousek et al., 2020). All of these functions depend on the pattern and stability of vegetation, which is susceptible to the dynamic tidal waters and inundations (Redelstein et al., 2018). Although the increase in sea level rise may be counterbalanced by sediment accretion and increased belowground biomass production (Morris et al., 2002; Cherry et al., 2009; SChile et al., 2014), coastal wetlands are likely to lose relative elevation and experience increased rates of tidal inundation, leading to increased anaerobic stress (Sandi et al., 2018). In addition, salt marsh plants are facing variable inundation patterns due to sea level rise (Kirwan and Megonigal, 2013; SChile et al., 2017), which might reduce their adaptability and cause a higher degradation risk (Barnuevo and Asaeda, 2018; Elsey-Quirk et al., 2024) and lower recovery rates (van Belzen et al., 2017).
Inundation is the main and key factor in wetland ecosystems, which drives vegetation patterns (Crain et al., 2004; Todd et al., 2010; Guo and Pennings, 2012), important processes (i.e., plant life history and establishment, Friess et al., 2012; Sloey et al., 2016), and functions (i.e., productivity, carbon storage, Waring and Maricle, 2012; Zhao et al., 2019; Janousek et al., 2020). Inundation affects plant survival, height, biomass and morphology at the individual level in a number of ways (Colmer and Voesenek, 2009; Voesenek and Bailey-Serres, 2015; Loreti et al., 2016; Redelstein et al., 2018; Zhao et al., 2023), and biodiversity, distribution patterns and functions at community level (Lopez and Kursar, 2003; Kirwan and Guntenspergen, 2012; Jones et al., 2018; Janousek et al., 2020). Its role in driving and affecting wetland ecosystem has been realized for a long time (Adam, 1990; Pezeshki, 2001; Lopez and Kursar, 2003; Crain et al., 2004; Redelstein et al., 2018). However, inundation intensity is often treated roughly as a statistical indicator over a period of time, such as inundation frequency. In reality, the tidal inundation process over a period of time consists of multiple inundation events, each with its own characteristics, such as inundation duration and water depth. Furthermore, during a tidal inundation event, the duration and water depth of inundation vary along the elevation gradient. Thus, various inundation patterns are generated along the elevation gradient when different tidal inundation events occur. Additionally, inundation patterns are likely to be highly affected by climate change (Kirwan and Megonigal, 2013) and human activities (Wang et al., 2022). These influence factors including changes in precipitation, sea-level rise, or modification by dikes (Wang et al., 2022). This might pose challenges to the present understanding of tidal inundation and its effect on salt marsh plants. Whether treating tidal inundation roughly as frequency and neglecting the details of individual inundation events is sufficient to explain the establishment patterns and variability of plants remains to be discussed. In nature with variable environment, it is necessary to have a deeper and more comprehensive understanding of inundation and its impact on salt marsh plants.
Salt marsh plants have different tolerance to inundation. Thus, plant patterns tend to adapt to specific inundation patterns in ecosystems, such as the zonal distribution of salt marsh plants in many coastal areas of the world (Crain et al., 2004; Guo and Pennings, 2012; He, 2012). Tidal inundation affects salt marsh plants in many ways, such as by changing soil permeability and chemical characteristics (Adam, 1990; Colmer and Flowers, 2008). During high tide, especially spring tides, salt marsh plants are often partially or completely submerged by the sea water for several hours or even longer. Frequent inundation causes the depletion of oxygen in salt marsh soil (Pezeshki, 2001), which is harmful to root respiration (Kirwan and Guntenspergen, 2012; Redelstein et al., 2018). In addition to creating a soil anoxic environment, frequent flooding also leads to the enrichment of other compounds and ions in the soil that affect plant growth, including CO2, ethylene, S2-, etc (Brinkman and Van Diepen, 1990; Colmer and Flowers, 2008; Suo et al., 2023).
Accordingly, the adaptive mechanisms of vegetation to inundation are viral, which can be detected by morphology and physiology indicators. This has also become a focus of inundation stress on plants (Colmer and Voesenek, 2009; Jackson et al., 2009; Voesenek and Bailey-Serres, 2015; Loreti et al., 2016). However, the inundation stress is usually considered as inundation frequency or period (Li et al., 2020), water depth or level (Fu et al., 2014; Zhao et al., 2020 and 2023; Mozo et al., 2021; Wang et al., 2024) or duration (Lan et al., 2019; Chen et al., 2020) independently. As we know, water or tidal regime can be described by the depth, duration, frequency, rate of filling and drying, timing and predictability of flooded and dried phases in a wetland (Bunn et al., 1997). The combined effect from different aspects of inundation has not been well addressed (Casanova and Brock, 2000; Miller and Zedler, 2003). Inundation frequency, depth and duration describe different aspects of tidal regime across the marsh, and shape the specific plant traits, patterns and functions, but their effects may differ (Casanova and Brock, 2000; Miller and Zedler, 2003; Barnuevo and Asaeda, 2018; Zhao et al., 2021).
Based on a tidal event, the duration and water depth or level are important aspects for describing the characteristics of the flooding and ebbing process on salt marsh surfaces (Wang et al., 2019 and 2022). The depth and duration of inundation depend on the height of the plants relative to the mean sea level (Silvestri et al., 2005; Colmer and Flowers, 2008). The frequency or period is used to describe the total number of inundation events or intervals between two tidal events. For convenience and accessibility, a statistical indicator, frequency, is usually used to represent inundation stress in salt marshes (He, 2012; Wang et al., 2018). In many studies, the frequency is sufficient to depict the inundation stress and can be easily estimated through the relationship with elevation (SChile et al., 2017; Wang et al., 2019). In some cases, elevation is used to represent inundation frequency, as it has a robust negative correlation with inundation frequency (He, 2012). Water depth is also used to represent inundation stress (Fu et al., 2014; Zhao et al., 2020 and 2023; Mozo et al., 2021; Wang et al., 2024). Water depth was used from a static perspective to illustrate the optimum range of water depth for different vegetation types, which is rarely considered with other factors, such as frequency. In conclusion, although inundation is a common stress, its utilization is usually simply based on frequency or depth, without the combination of different aspects of the inundation process. This approximate estimation of inundation stress may cause some inaccuracy or misjudgment of its real effect on plant adaptation process, thereby affecting our understanding of tidal inundation in salt marshes.
To reveal the influence of varied characteristics of tidal inundation events on salt marsh plants, we conducted an experiment to simulate different types of inundation events with varied durations, depths, and frequencies in a greenhouse. Specifically, we focused on the frequency, duration and depth of tidal events on seed emergence and seedling growth stage. We aimed to address the following questions: 1) whether there is an independent and cumulative effect of multiple factors from tidal inundation on the early life stages of a plant and 2) how those factors interact with each other when they act on different life stages. By comprehensively considering the effects of inundation stress from different aspects in the early stage of the plant, we could predict the response of plants to multiple stresses caused by climate change or human activity in the inundation regime and take adaptive management measures to mitigate the adverse effects of multiple stresses.
2 Methods and materials
2.1 Species selection and collection
The salt marsh plant species used in this study was Suaeda salsa (S. salsa), an annual herbaceous salt species. It is the dominant species in the salt marsh of the Yellow River Delta and has the widest distribution range from the low tide zone to the supratidal zone (Wang et al., 2022). The plant has a high tolerance to inundation and salinity stress to some extent (Song, 2009), whereas the appropriate range of adaptation to salinity ranges from 0 to 10 ppt, according to our previous study (Wang et al., 2019). S. salsa seeds are characterized by a low mass and a waxy seed coat (Li et al., 2005), which enables them to be easily dispersed by the tide throughout the salt marshes. After seed maturation in autumn, they fall to the ground and are picked up by the tide, which then disperses them across mudflats and salt marshes (Wang et al., 2022).
Seeds and seedlings of S. salsa were used to synchronously conduct seed emergence and seedling survival experiments in spring. The seeds were collected in the field when S. salsa matured, fell to the ground (in autumn), and stored in cool and dry conditions for the emergence experiment. S. salsa seedlings were collected in April from undisturbed soil for the survival experiment and were cultivated in the greenhouse for a week for acclimatization.
2.2 Experimental design
On a single tidal event scale, a tidal event can generate different inundation patterns along an elevation gradient. That is, a higher water depth and longer duration at low elevations, lower water depths, and shorter durations at high elevations. In addition, the inundation frequency varied along the elevation gradient. To examine the effects of tidal inundation patterns on salt marsh plants along the elevation gradient, we designed inundation treatments of different durations, water depths, and frequencies. We set up two types of inundation duration of 3h and 6h, with four types of water depths of 10 cm, 20 cm, 30 cm, and 40 cm. The frequency of occurrence was set based on a daily scale, according to the frequency of occurrence of field tidal inundation events. They were conducted with four frequencies once a day (1/1), once every five days (1/5), once every ten days (1/10) and once every fifteen days (1/15), simulating variations in inundation frequency along the elevation gradient. Totally 32 inundation treatments were set up according to the field tidal inundation patterns along the elevation gradient. Because we focused on the inundation characteristics, salinity stress was not considered in the experiment. The salinity in the inundation treatment was approximately 2–3 ppt, which was suitable for S. salsa to germinate and grow and would not induce salinity stress.
Specifically, 32 treatments were adopted in both S. salsa seed emergence and seedling survival experiments, and each treatment had three replicates (n=96 in each experimental group). Flowerpots with a diameter of 10 cm were used to conduct the experiments, and in situ salt marsh soil was used to plant seeds or seedings in the flowerpots. All flowerpots were placed in different tanks to control the inundation duration, depth, and frequency. (1) Seed emergence experiment. One hundred S. salsa seeds were sowed evenly in each flowerpot, with a 1 cm soil layer and a 0.5 cm sand layer covered above. As S. salsa seeds are light and easy to float, a 0.5 cm sand layer was covered above the soil to prevent the loss of floating seeds. (2) Seedling survival experiment. Seedlings of the same height (approximately 8 cm) and stem diameter (approximately 1 mm) in the field were selected for transplanting in the flowerpot with the original soil. Each flowerpot was transplanted to six seedlings. (3) Control treatment. As a reference, we also used watering treatments for seed emergence and seedling survival experiments. We set three groups of watering treatments, including once a day, once every five days and once every ten days, with each group of three replications (n=9 in each experiment).
The experiment was carried out in an indoor greenhouse from May to July to ensure that the other conditions, such as light, temperature, and soil moisture, were the same as the wild growing conditions. The experiment lasted for two months. The emerged seeds and seedlings were counted every five days before inundation. In the seed emergence experiment, emerged seedlings were removed from the flowerpot after recording for easy counting. In the seedling experiment, the height of each seedling was measured using a tapeline every five days and recorded.
2.3 Data analysis
The cumulative seed emergence rate, survival rate and plant height of S. salsa were used to reflect stress. They can be obtained using the following equations:
ERi and SRi are the emergence and survival rates on the ith day, respectively, and Hi is the mean height of S. salsa in a flowerpot on the ith day. NEi is the number of emerged seeds on the ith day, NSi is the number of surviving S. salsa on the ith day, 100 and 6 are the total number of seeds and seedings, respectively, hij is the height of individual j on the ith day, and only live individuals are counted.
The cumulative seed emergence rate, survival rate, and height on the 60th day were used to compare the differences. To test the effects of frequency, duration, and water depth on S. salsa seed emergence, survival, and growth, a three-way ANOVA was used to compare the differences among the 32 treatments (n=96). Cohen’s f was used to compare the effect size (ES) of each inundation component (Cohen, 1988).
Where F is the observed value of the sample F statistic, n is the sample size of each group, and higher values of Cohen’s f represent a higher effect. Specifically, Cohen’s f < 0.1 represents small effect, Cohen’s f ≈0.25 represents medium effect, and Cohen’s f > 0.4 represents high effect. Additionally, one-way ANOVA was used to compare the differences between the watering and inundation groups (n=105). Mean values of the three replicates in each treatment on the record day (ith day) were used to depict the emergence, survival, and growth curves during the experiment, and Kruskal-Wallis analysis was used to test the differences among these curves of each frequency-duration-depth group in the inundation treatment and each frequency group in the watering treatment. All statistical analysis were conducted using SPSS 22. To compare the final results of emergence rate, survival rate, and plant height in each treatment more directly and to explore the interaction of frequency, duration and depth, contour lines were used to seek the equivalent groups of frequency, duration and depth.
3 Results
3.1 Effect of varied inundation characteristics on seed emergence
From the perspective of the total emergence rate, the inundation treatment showed significant stress compared to the watering treatment (Figure 1A). The emergence rate in the watering treatment was significantly higher than that of the inundation treatment. According to the three-way ANOVA, frequency, duration and depth of inundation events had a significant impact on seed emergence, and seed emergence rate decreased with increasing inundation frequency, duration and depth (Figures 1B, C). Specifically, the decrease in emergence rate in 10 cm and 20 cm depth treatments was not significant (Figure 1D). According to the effect size of inundation frequency, duration and depth, frequency had the most obvious effect on the emergence rate (ES=1.81, P=0.000), followed by duration (ES=1.10, P=0.000), and depth (ES=0.72, P=0.000).
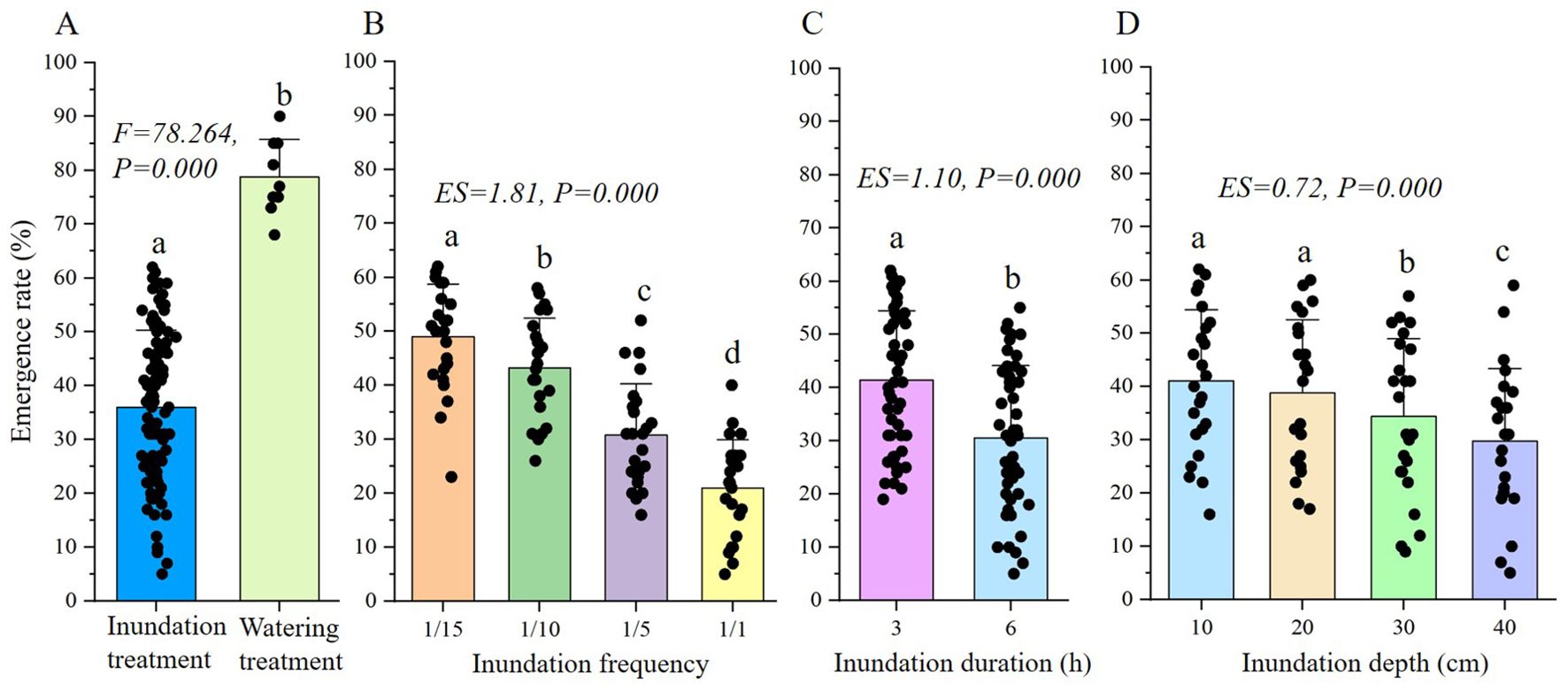
Figure 1. ANOVA analysis of total emergence rate of S. salsa seeds under different treatment. (A) shows the result of one-way ANOVA of inundation and watering treatment, (B-D) show the results of three-way ANOVA of frequency, duration and depth in inundation treatment. The same letter above the bar shows no significant differences.
From the view of the cumulative emergence curve during the two months (Figure 2), most seeds emerged within one month, revealing a fast emergence characteristic. Differences between inundation treatments with different frequencies, durations, depths and watering treatments with different frequencies were significant according to Kruskal-Wallis analysis (χ2 = 343.673, df=34, P=0.000). The emergence rate was significantly higher in the watering treatment than in the inundation treatment, at a frequency of 1/1. Generally, the inundation treatment with a frequency of 1/15, duration of 3h, depth of 10 and 20 cm had the highest emergence rate among inundation treatments.
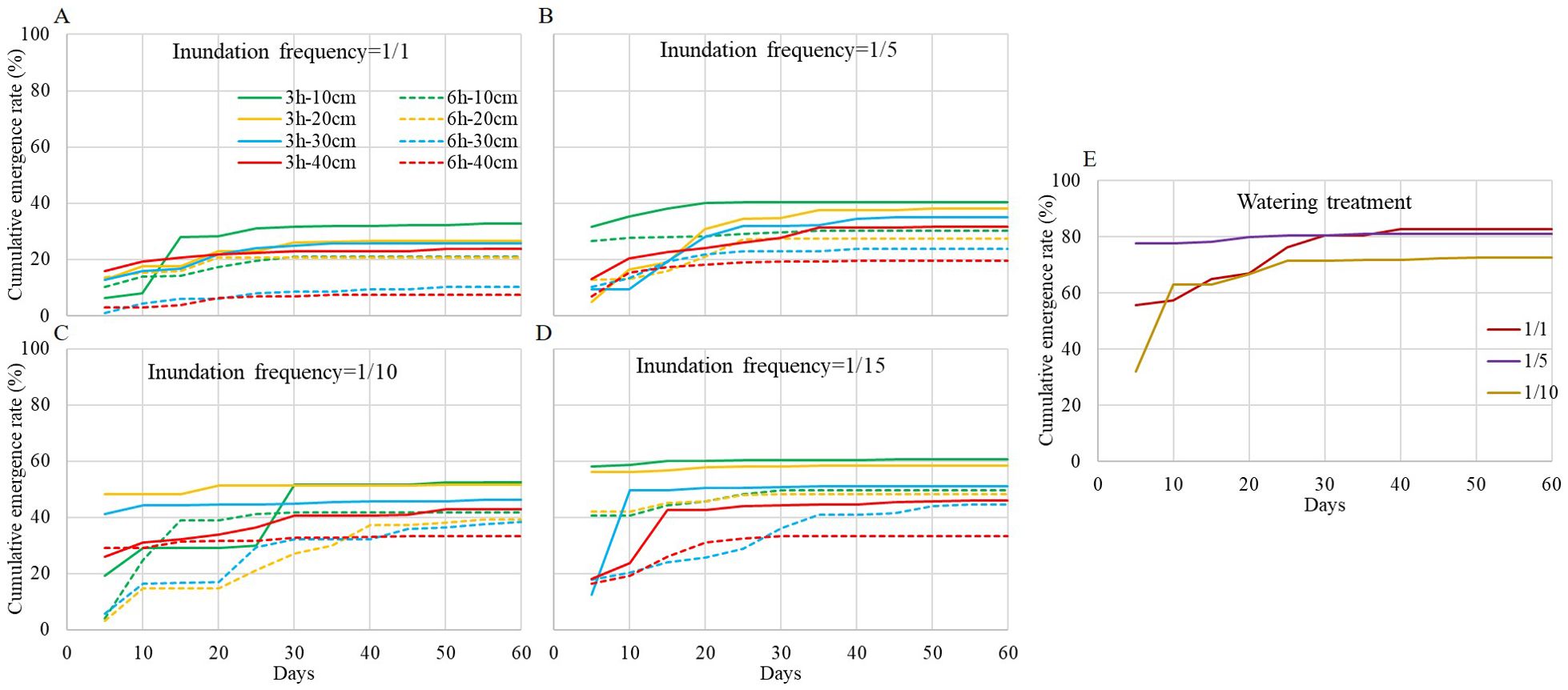
Figure 2. S. salsa emergence curve during the experiment. (A-D) are inundation treatment with frequency at 1/1, 1/5, 1/10 and 1/15, respectively. Each full line with different color shows inundation event of 3h-duration with different depth, and each dash line with different color shows inundation event of 6h-duration with different depth. (E) is watering treatment with watering frequency at 1/1, 1/5 and 1/10, respectively.
3.2 Effect of varied inundation characteristics on seedling survival
All S. salsa seedlings were alive during the experiment in the watering treatment, which was significantly different from the inundation treatment (Figure 3A). In the inundation treatment, frequency, duration and depth of inundation events significantly affected seedling survival (Figures 3B-D). The seedling survival rate decreased with increasing frequency, duration and water depth. Specifically, the decrease in emergence rate in the 10 and 20 cm was not significant (Figure 3D). According to the effect size, frequency had the most obvious effect on survival rate (ES=2.94, P=0.000), followed by duration (ES=1.04, P=0.000) and depth (ES=0.62, P=0.000).
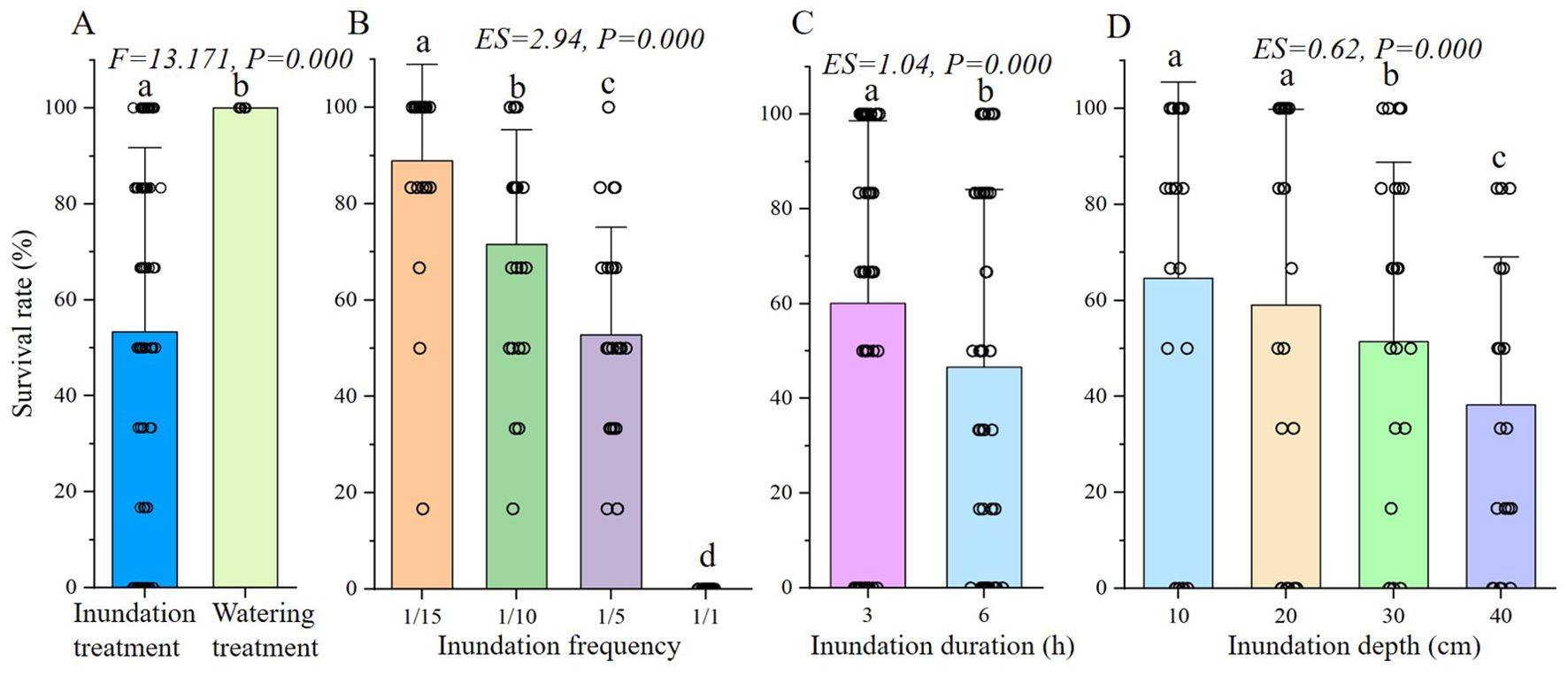
Figure 3. ANOVA analysis of final survival rate of S. salsa seedlings under different treatment. (A) shows the result of one-way ANOVA of inundation and watering treatment, (B-D) show the results of three-way ANOVA of frequency, duration and depth in inundation treatment. The same letter above the bar shows no significant differences.
When the inundation frequency was 1/1, all seedlings died within 40 days (Figure 4A), and inundation event of duration at 6 h, depth at 40 cm accelerated the death of seedlings (within 20 days). In other inundation treatments (Figures 4B-D), the survival rate decreased within 30 days, and then became stable. Differences between inundation treatments with different frequencies, durations, depths and watering treatments with different frequencies were significant according to Kruskal-Wallis analysis (χ2 = 269.935, df=34, P=0.000). The survival rate in the inundation treatment with a frequency of 1/1 was significantly different from that of the other inundation and watering treatments. The inundation treatment with a frequency of 1/15, duration of 3h, depth of 10 and 20 cm had the highest survival rate among inundation treatments.
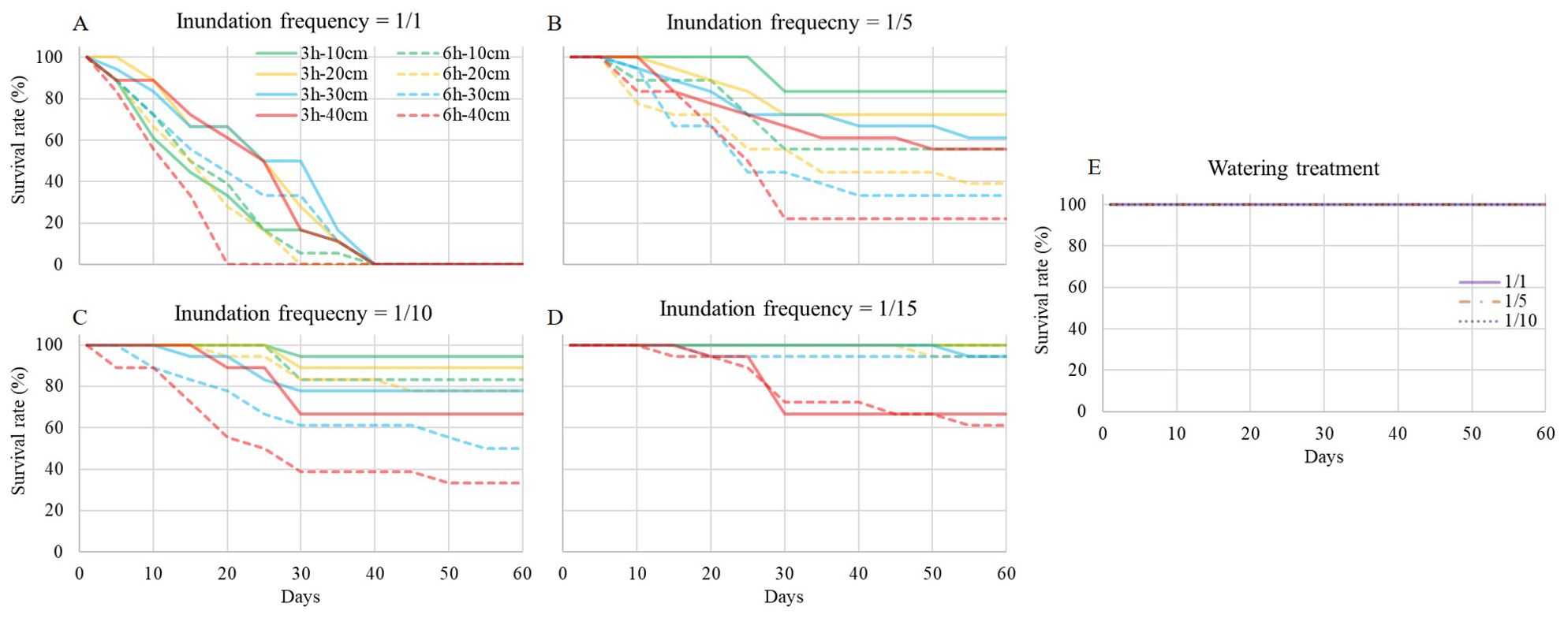
Figure 4. S. salsa survival curve during the experiment. (A-D) are inundation treatment with frequency at 1/1, 1/5, 1/10 and 1/15, respectively. Each full line with different color shows inundation event of 3h-duration with different depth, and each dash line with different color shows inundation event of 6h-duration with different depth. (E) is watering treatment with watering frequency at 1/1, 1/5 and 1/10, respectively.
3.3 Effect of varied inundation characteristics on seedling growth
The height of S. salsa seedlings in the inundation treatment was significantly lower than that in the watering treatment (Figure 5A). The influence of frequency, duration and depth was also significant on seedling growth (Figures 5B-D), but the difference among groups decreased. Except for the fatal treatment with a frequency of 1/1, the height in frequency of 1/5, 1/10 and 1/15 were close, and the differences between the frequency of 1/15 and 1/5 was not significant (Figure 5B). In addition, differences among groups in depth treatment were further reduced (Figure 5D). According to the effect size, frequency had the most obvious effect on seedling growth (ES=4.81, P=0.000), followed by duration (ES=0.89, P=0.000), and depth (ES=0.33, P=0.003).
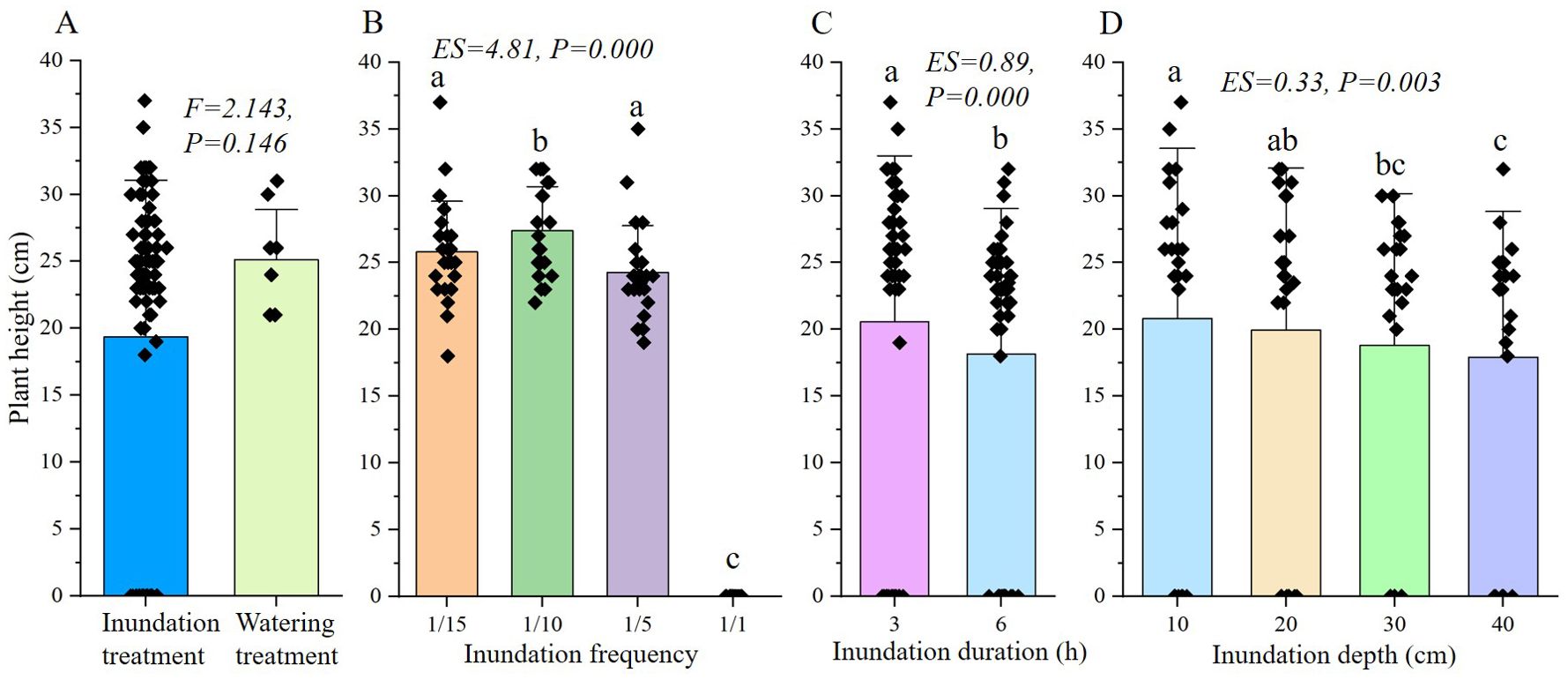
Figure 5. ANOVA analysis of final height of S. salsa seedlings under different treatment. (A) shows the result of one-way ANOVA of inundation and watering treatment, (B-D) show the results of three-way ANOVA of frequency, duration and depth in inundation treatment. The same letter above the bar shows no significant differences.
In the inundation treatment at a frequency of 1/1, the mean seedling height showed a slowly increasing trend at the beginning of the experiment and then decreased abruptly due to the death of some individuals (Figure 6A). Specifically, the mean height in the treatment of duration at 6 h and depth at 40 cm decreased to 0 at first (Figure 6A). In other inundation and watering treatments (Figures 6B-E), seedling height increased stably until the end of the experiment, and the curves were close to each other. Differences between inundation treatments with different frequencies, durations, depths and watering treatments with different frequencies were significant according to Kruskal-Wallis analysis (χ2 = 178.142, df=34, P=0.000). Significant differences were observed in the frequency of inundation treatment at 1/1 with the other treatments.
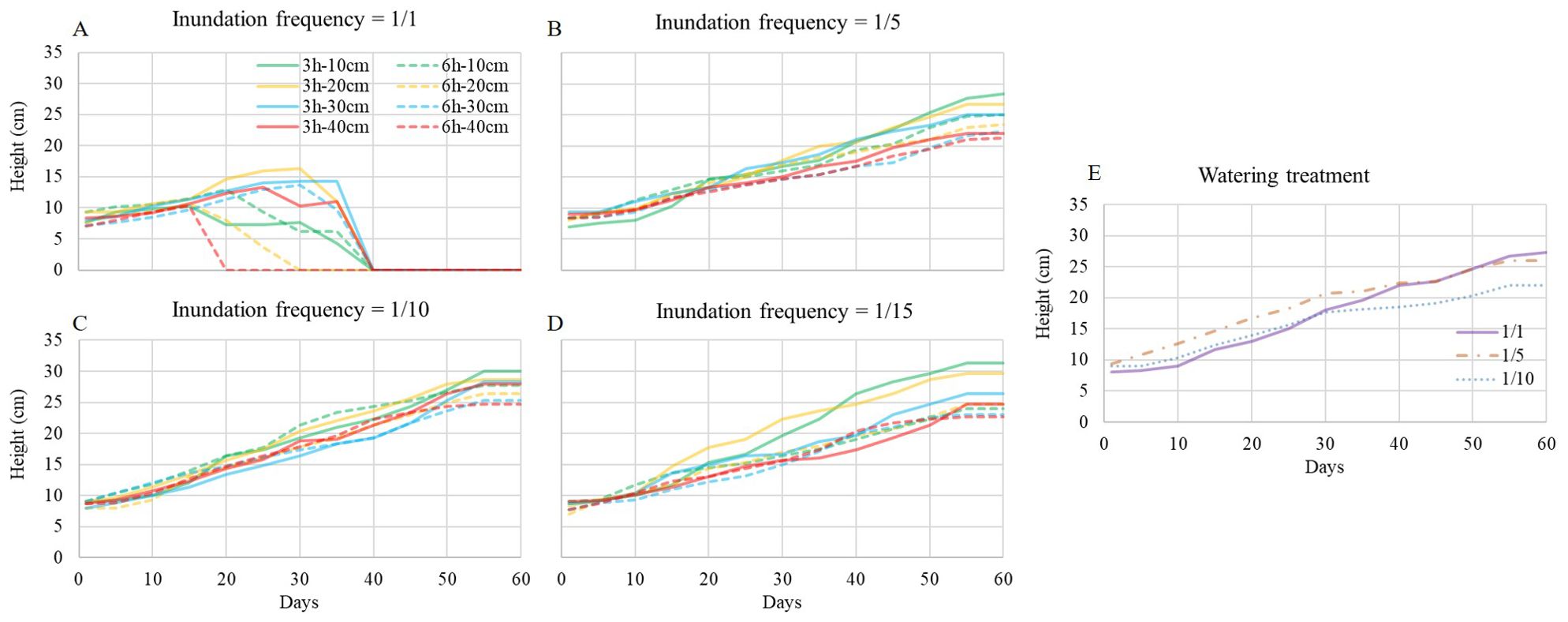
Figure 6. S. salsa growth curve during the experiment. (A-D) are inundation treatment with frequency at 1/1, 1/5, 1/10 and 1/15, respectively. Each full line with different color shows inundation event of 3h-duration with different depth, and each dash line with different color shows inundation event of 6h-duration with different depth. (E) is watering treatment with watering frequency at 1/1, 1/5 and 1/10, respectively.
3.4 Interactions of varied inundation characteristics
By calculating the mean values of emergence rate, survival rate and height in each frequency-duration-depth group, the interactions among inundation frequency, duration and depth can be summarized as shown in Figure 7. The emergence rate, survival rate and height of each frequency-duration group decreased with increasing depth, indicating a regular variation trend along the grouping of frequency, duration and depth. Specifically, the emergence rates of the 1/1-6 h-20 cm, 1/1- 6h-10 cm and 1/5-6 h-40 cm treatments were the same, at 20% (Figure 7A). Similarly, the emergence rates of the 1/1-3 h-10 cm, 1/10-6 h-40 cm and 1/15-6 h-40 cm treatments were approximately 34%, and the emergence rates of the 1/15-3 h-40 cm, 1/15-6 h-30 cm and 1/10-3 h-30 cm were approximately 45% (Figure 7A). These inundation groups shared the same emergence rate revealing a trade-off between frequency, duration and depth. Inundation events with shallower water depths and/or shorter durations would reduce the stress from higher frequencies. Similar trade-offs of frequency, duration and depth were found in seedling survival and growth (Figures 7B, C). For example, the survival rates of the 1/10-6 h-40 cm and 1/5-6 h-30 cm treatments were the same at 26%, the survival rates of the 1/15-6 h-40 cm and 1/5-3 h-30 cm treatments were the same at 60%, and the survival rates of the 1/10-6 h-10 cm and 1/5-3 h-10 cm treatments were the same at 82% (Figure 7B). In terms of plant height, treatments of 1/15-3 h-40 cm, 1/15-6 h-20 cm, 1/10-6 h-40 cm,1/5-3 h-30 cm and 1/5-6 h-10 cm were the same at 25 cm height (Figure 7C).
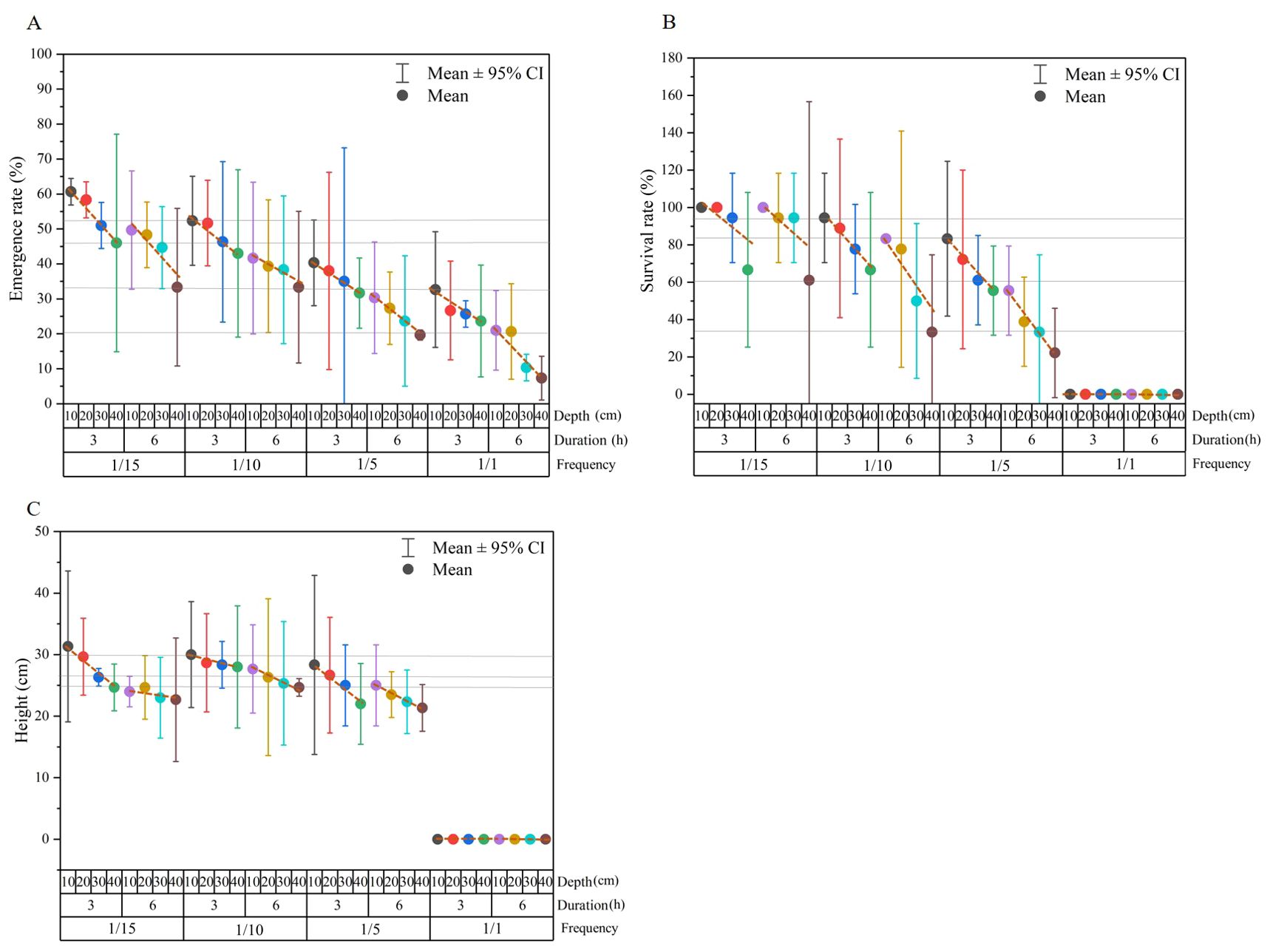
Figure 7. Mean values of emergence rate (A), survival rate (B) and height (C) in each frequency-duration-depth group. Dash lines are used to reveal the trend of emergence rate, survival rate and height along water depth gradient in each frequency-duration group, and grey full lines are used to reveal equal values and the corresponding inundation groups.
4 Discussion
Since inundation is usually represented by a statistical indicator, such as frequency, details of each specific tidal event and their cumulative effects on plants might be ignored. Thus, we conducted this experiment to explore the effects of various inundation characteristics based on the inundation event scale. The results showed that stress could be strengthened by a higher inundation frequency with a longer duration and deeper depth. Among these factors, frequency had a dominant effect, followed by duration and water depth. Specifically, there was a trade-off among frequency, duration and depth, which showed that inundation events with shallower depths and/or shorter durations would reduce stress from higher frequencies. This might be important in multi-stress systems and in enlightening stress relief solutions for ecological restoration.
4.1 Inundation stress on S. salsa seed emergence
As a seed diffusion propagates plant, S. salsa seed are widely spread by tides (He, 2012). This make it a ubiquitous species and has potential for extensive colonization in intertidal zone. The small and light attributes and waxy coat make it can float with tidal waters for a long time, until fall onto the ground (Chang et al., 2008; Wang et al., 2022). Thus, it seems less susceptible to inundation stress and little attention has been paid to its response to inundation. Instead, researches focused on the effect of soil moisture, salinity, sediment thickness and temperature on S. salsa seed germination (Li et al., 2005; Xie et al., 2017). However, when it sucks up the water and settled down, it would probably suffer the stress from inundation. Our experiment used sand to cover them to prevent them from floating, and verified the stress of varied inundation characteristics on S. salsa seed emergence. Specifically, we detected the independent and cumulative effect of inundation frequency, duration and depth on seed emergence, and recognized the trade-off among the multi-stress from different aspects of inundation.
S. salsa seed emergence speed was very fast (Figure 2). As long as the conditions were suitable, the seeds could emerge immediately. All the active seeds could emerge completely within one month (Figure 2). This strategy of rapid germination under suitable conditions is very conducive to the colonization of S. salsa in the intertidal zone, which makes it the most widely distributed species (He, 2012). In the severe and changeable environment of the intertidal zone, S. salsa can seize the window of opportunity (Balke et al., 2014) for rapid germination and realize the first step of self-colonization. S. salsa seeds can germinate when these key factors (moisture, salinity, sediment thickness, etc.) just fall into its threshold (Li et al., 2005; Xie et al., 2017). The important is that, while waiting for the ‘window’, the seeds should remain active. However, during this waiting for a ‘window’ period, tidal inundation makes the biggest risk for S. salsa seeds to remain active. And this risk of inactivation from inundation has not been systematically studied.
For S. salsa seeds, it had a high germination rate on a wet surface without inundation (Xie et al., 2017). The pre-germination experiment of the seeds showed that under laboratory conditions, the germination rate of the seeds on the surface of wet filter paper was more than 80%. Similarly, the watering control treatment also demonstrated S. salsa seeds had suffer different levels of stress from inundation. As demonstrated by other physiological studies, seeds of most plants are very sensitive to inundation (Abdelbagi et al., 2012). In riparian systems, seed viability and nutrient are significantly decreasing with the increasing of flooding duration (Ma et al., 2018). When starved of oxygen, the seeds of Zostera marina completely lose their ability to germinate (Hootsmans et al., 1987). The decreasing of seed germination rate under inundation stress is mainly caused by hypoxia (Abdelbagi et al., 2012; Suo et al., 2023).
Our experiment verified the significant stress of inundation on S. salsa seed emergence, and the effects varied when inundation events had different characteristics. Generally, stress increases with the increasing frequency, duration and depth of inundation events. This means that the tidal inundation regime with a combination of high frequency, long duration and deep depth is extremely stressful for S. salsa seeds, which will decrease the emergence rate in the field and lead to early degradation of the S. salsa community. Specifically, the impacts of different inundation factors varied. Frequency had a dominant effect on seed emergence, followed by duration and water depth. This means that, in most situations, frequency is a robust indicator of inundation intensity. However, when incorporating the duration and depth characteristics into inundation, the impact is modified on a smaller scale. When the frequency is fixed, a shorter duration and/or shallower depth will reduce the stress compared to longer durations and/or deeper depths. Specifically, there is a trade-off between frequency, duration, and depth to some extent, and the effects of higher frequency, longer duration, and deeper depth might be equal to the effects of lower frequency, shorter duration, and shallower depth. Thus, the total stress can be regarded as a combination of frequency, duration and depth, and the increasing stress caused by one factor can be relieved by the decrease in the other one or two factors. However, the contribution of each inundation factor must be studied further. Importantly, this trade-off among different factors of inundation events should be considered when considering the impact of inundation on seed emergence.
4.2 Inundation stress on S. salsa seedling survival and growth
During the inundation, plant root soil ventilation is affected, and the sediments may be in an anaerobic environment, thus affecting plant root respiration and growth (Voesenek and Bailey-Serres, 2015; Redelstein et al., 2018; Wei et al., 2021). According to the two-month experiment, we found that the stress had a cumulative effect, mainly because we did not design the extreme long duration treatment, and did not detect the one-time fatal inundation duration. This situation can occur when salt marshes experience a storm tide (Leonardi et al., 2016; Lagomasino et al., 2021), and the inundation duration may last for several days, which leads to the death of many plants (Crooks et al., 2002; Kirwan and Gedan, 2019). Our experiment shows the cumulative stress of multiple inundation events, and the earliest death of S. salsa occurred on the 20th day, with a frequency of 1/1, depth of 40 cm and duration of 6 h (Figures 4, 6). In general, fatal cumulative stress occurs at a frequency of 1/1. With the decrease in depth and duration, the days of death were delayed, but all the groups died within 40 days. The survival rate increased significantly with decreasing frequency. This indicates that, except for the extreme fatal inundation event (such as storm tide), the cumulative stress from the high-frequency inundation is also deadly, which cannot sustain seedling survival to maturity. The death of juvenile S. salsa is advantageous to S. salsa communities, which decreases the yield of seeds and affects successive establishment (Crooks et al., 2002; Davy et al., 2011). The effects of shorter duration and shallower depth only delayed the death day of S. salsa, but not their destiny. With decreasing frequency, the situation of S. salsa becomes better, at least not fatal.
The dominant effect of inundation is in frequency, followed by duration and depth. Compared with seed emergence, seedling survival revealed a stricter death threshold at high frequency (1/1) and higher tolerance in other inundation situations. The differences in seedling height between the inundation and watering treatments were further reduced. This indicates that, except for the fatal inundation situation, the seedling stage is less susceptible to inundation, which reflects another trait of the species that is widely established. There is also a trade-off between the frequency, duration, and depth of inundation events at the seedling stage. However, the trade-off may lie only within a specific range within the threshold. Compared with seed emergence, seedlings showed a fatal effect at a high inundation frequency of 1/1 and a stabilizing high survival rate when the frequency was reduced to 1/15 (Figure 7B). In both inundation treatments of frequency at 1/1 and frequency at 1/15 with shallower depths (10–30 cm), the trade-off vanished. This was more obvious in seedling height (Figure 7C). For plant height, the treatment frequency of 1/5 and duration of 6 h reduced the growth speed significantly, leading to a shorter plant height. At this frequency, a shorter duration can relieve stress on growth (represented by height). Since plant height is often positively related to biomass, higher plant height means not only greater seed yield (Xie et al., 2017) but also higher primary productivity and more carbon storage (Bouchard and Lefeuvre, 2000; Mcleod et al., 2011), which is crucial to the function provided by salt marshes. In addition, it is a key factor in accelerating sediment deposition and defending storms (Leonardi et al., 2016). In this way, the various tidal regimes with different frequencies, durations, and water depths not only influence the survival of plants, but also the functions associated with height (biomass).
4.3 Implications in explaining vegetation patters and stress-relief solutions
The interaction of inundation factors may contribute to explaining vegetation patterns in the field. Inundation is usually represented by frequency to account for its effects on salt marsh plants. Our results demonstrated the combined effects of different characteristics during inundation events on the early life stages of plants, which provides more details on how different durations, depths, and frequencies influence seed emergence, seedling survival, and growth. This finding also provides important implications for plant establishment in relation to different inundation patterns. For example, low elevation is dominant with low water levels but a high frequency of inundation events (i.e., the nearby tidal creek area), which is consistent with the high-frequency and low-depth treatment in our experiment. This may induce different responses in the plant seeds and seedlings. In these areas, plants may germinate, but fail to survive. In contrast, high-water inundation events submerge at higher elevations and induce deeper inundation depths, but the frequency is relatively low. This may lead to low-frequency but high-depth inundation events at higher elevations, which are also stressful compared to high-frequency but low-depth inundation events. In terms of duration, both long and short durations may occur at low depths with high frequency and high depth with low-frequency inundation events. It may depend not only on the tide itself but also on the drainage conditions.
Moreover, the trade-off among the frequency, duration, and depth of inundation events can provide important insight into stress-relief solutions for ecological restoration. The tidal inundation regime is more complex and changeable when considering multiple tidal events, rather than simply reducing the frequency along the elevation gradient. It can be regulated to reduce inundation stress. For example, in cases of higher frequency (not fatal), inundation stress can be alleviated by reducing the inundation duration and depth. This can be achieved by improving the drainage conditions or lifting elevations to a certain extent. In other cases, reducing the frequency of high-water inundation events may also relieve stress at high water depths or for long durations.
Data availability statement
The original contributions presented in the study are included in the article/supplementary material. Further inquiries can be directed to the corresponding author.
Author contributions
QW: Visualization, Validation, Supervision, Software, Resources, Project administration, Methodology, Data curation, Writing – review & editing, Writing – original draft, Investigation, Funding acquisition, Formal analysis, Conceptualization. ML: Writing – original draft, Methodology, Investigation, Conceptualization. BC: Writing – review & editing, Supervision, Funding acquisition, Conceptualization. CC: Writing – review & editing, Formal analysis, Data curation. TX: Writing – review & editing, Methodology, Investigation, Funding acquisition. XL: Writing – review & editing, Validation, Supervision, Data curation. FL: Writing – review & editing, Supervision, Data curation, Methodology, Formal analysis, Conceptualization, Visualization, Resources, Project administration.
Funding
The author(s) declare financial support was received for the research, authorship, and/or publication of this article. The study was supported financially by the Natural Science Foundation of China (42107057, U2243208, 52271256, 42330705) and Science & Technology Fundamental Resources Investigation Program (Grant No. 2022FY100304).
Acknowledgments
We thank for the help from the Yellow River Delta National Nature Reserve Administration Bureau.
Conflict of interest
Author ML was employed by the company Dongjiang Environmental Company Limited.
The remaining authors declare that the research was conducted in the absence of any commercial or financial relationships that could be construed as a potential conflict of interest.
Publisher’s note
All claims expressed in this article are solely those of the authors and do not necessarily represent those of their affiliated organizations, or those of the publisher, the editors and the reviewers. Any product that may be evaluated in this article, or claim that may be made by its manufacturer, is not guaranteed or endorsed by the publisher.
References
Abdelbagi M. I., Johnson D. E., Ella E. S., Vergara G. V., Baltazar A. M. (2012). Adaptation to flooding during emergence and seedling growth in rice and weeds, and implications for crop establishment. AoB Plants 2012, pls019. doi: 10.1093/aobpla/pls019
Balke T., Herman P. M. J., Bouma T. J. (2014). Critical transitions in disturbance-driven ecosystems: identifying Windows of opportunity for recovery. J. Ecol. 102, 700–708. doi: 10.1111/jec.2014.102.issue-3
Barnuevo A., Asaeda T. (2018). Integrating the ecophysiology and biochemical stress indicators into the paradigm of mangrove ecology and a rehabilitation blueprint. PloS One 13, e0202227. doi: 10.1371/journal.pone.0202227
Bouchard V., Lefeuvre J. C. (2000). Primary production and macro-detritus dynamics in a European salt marsh: carbon and nitrogen budgets. Aquat. Bot. 67, 23–42. doi: 10.1016/S0304-3770(99)00086-8
Brinkman R., Van Diepen C. A. (1990). “Mineral soils,” in Wetlands and Shallow Continental Water Bodies. Vol. 1. Natural and Human Relationships. Ed. Patten B. C. (SPB Academic Publishing, Hague, The Netherlands), 37–59.
Bunn S. E., Boon P. I., Brock M. A., Schofield N. J. (1997). National Wetlands R & D Program Scoping Review. Land and Water Resources Research and Development Corporation Occasional Paper, 01/97. (Canberra: Land and Water Resources Research and Development Corporation).
Casanova M. T., Brock M. A. (2000). How do depth, duration and frequency of flooding influence the establishment of wetland plant communities? Plant Ecol. 147, 237–250. doi: 10.1023/A:1009875226637
Chang E. R., Veeneklaas R. M., Buitenwerf R., Bakker J. P., Bouma T. J. (2008). To move or not to move: determinants of seed retention in a tidal marsh. Funct. Ecol. 22, 720–727. doi: 10.1111/j.1365-2435.2008.01434.x
Chen Y., Stagg C. L., Cai Y., Lv X., Wang. X., Shen R., et al. (2020). Scaling responses of leaf nutrient stoichiometry to the lakeshore flooding duration gradient across different organizational levels. Sci. Total Environ. 740, 139740. doi: 10.1016/j.scitotenv.2020.139740
Cherry J. A., McKee K. L., Grace J. B. (2009). Elevated CO2 enhances biological contributtons to elevatton change in coastal wetlands by offsetttng stressors associated with sea- level rise. J. Ecol. 97, 67–77. doi: 10.1111/j.1365-2745.2008.01449.x
Cohen J. (1988). Statistical Power Analysis for the Behavioral Sciences. 2nd ed (New Jersey: Lawrence Erlbaum Associates, Inc).
Colmer T. D., Flowers T. J. (2008). Flooding tolerance in halophytes. New Phytol. 179, 964–974. doi: 10.1111/j.1469-8137.2008.02483.x
Colmer T. D., Voesenek L. A. C. J. (2009). Flooding tolerance: suites of plant traits in variable environments. Funct. Plant Biol. 36, 665–681. doi: 10.1071/FP09144
Crain C. M., Silliman B. R., Bertness S. L., Bertness M. D. (2004). Physical and biotic drivers of plant distribution across estuarine salinity gradients. Ecology 85, 2539–2549. doi: 10.1890/03-0745
Crooks S., Schutten J., Sheern G. D., Pye K., Davy A. J. (2002). Drainage and elevation as factors in the restoration of salt marsh in Britain. Restor. Ecol. 10, 591–602. doi: 10.1046/j.1526-100X.2002.t01-1-02036.x
Davy A. J., Brown M. J. H., Mossman H. L., Grant A. (2011). Colonization of a newly developing salt marsh: disentangling independent effects of elevation and redox potential on halophytes. J. Ecol. 99, 1350–1357. doi: 10.1111/j.1365-2745.2011.01870.x
Elsey-Quirk T., Lynn A., Jacobs M. D., Diaz R., Cronin J.T., Wang L., et al. (2024). Vegetation dieback in the Mississippi River Delta triggered by acute drought and chronic relative sea-level rise. Nat. Commun. 15, 3518. doi: 10.1038/s41467-024-47828-x
Friess D. A., Krauss K. W., Horstman E. M., Balke T., Bouma T. J., Galli D., et al. (2012). Are all intertidal wetlands naturally created equal? Bottlenecks, thresholds and knowledge gaps to mangrove and saltmarsh ecosystems. Biol. Rev. 87, 346–366. doi: 10.1111/j.1469-185X.2011.00198.x
Fu H., Zhong J., Yuan G., Xie P., Guo L., Zhang X., et al. (2014). Trait-based community assembly of aquatic macrophytes along a water depth gradient in a freshwater lake. Freshw. Biol. 59, 2462–2471. doi: 10.1111/fwb.2014.59.issue-12
Guo H., Pennings S. C. (2012). Mechanisms mediating plant distributions across estuarine landscapes in a low- latitude tidal estuary. Ecology 93, 90–100. doi: 10.1890/11-0487.1
He Q. (2012). Bottom-up, interspecific and top-down determinants of plant communities in salt marshes in the yellow river estuary. PhD thesis. Shanghai: Shanghai Jiao Tong University.
Hootsmans M. J. M., Vermaat J. E., VanVierssen W. (1987). Seed-bank deveopment, germination and early seedling survival of two seagrass species from the Nethelands: Zostera marina L. and Zostera noltii Hornem. Aquat. Bot. 28, 275–285. doi: 10.1016/0304-3770(87)90005-2
Jackson M. B., Ishizawa K., Ito O. (2009). Evolution and mechanisms of plant tolerance to flooding stress. Ann. Bot. 103, 137–142. doi: 10.1093/aob/mcn242
Janousek C. N., Dugger B. D., Drucker B. M., Thorne K.M. (2020). Salinity and inundation effects on productivity of brackish tidal marsh plants in the San Francisco Bay-Delta Estuary. Hydrobiologia 847, 4311–4323. doi: 10.1007/s10750-020-04419-3
Jones S. F., Stagg C. L., Krauss K. W., Hester. M. W. (2018). Flooding alters plant-mediated carbon cycling independently of elevated atmospheric CO2 concentrations. J. Geophysical Res.: Biogeosciences 123, 1976–1987. doi: 10.1029/2017JG004369
Kirwan M. L., Gedan K. B. (2019). Sea-level driven land conversion and the formation of ghost forests. Nat. Climate Change 9, 450–457. doi: 10.1038/s41558-019-0488-7
Kirwan M. L., Guntenspergen G. R. (2012). Feedbacks between inundation, root production, and shoot growth in a rapidly submerging brackish marsh. J. Ecol. 100, 764–770. doi: 10.1111/j.1365-2745.2012.01957.x
Kirwan M., Megonigal J. (2013). Tidal wetland stability in the face of human impacts and sea-level rise. Nature 504, 53–60. doi: 10.1038/nature12856
Lagomasino D., Fatoyinbo T., Castañeda-Moya. E., Cook B. D., Montesano P. M., Neigh C. S. R., et al. (2021). Storm surge and ponding explain mangrove dieback in southwest Florida following Hurricane Irma. Nat. Commun. 12, 4003. doi: 10.1038/s41467-021-24253-y
Lan Z. C., Huang H., Chen Y. S., Liu J. S., Chen J. G., Li L., et al. (2019). Testing mechanisms underlying responses of plant functional traits to flooding duration gradient in a lakeshore meadow. J. Freshw. Ecol. 34, 481–495. doi: 10.1080/02705060.2018.1550022
Leonardi N., Ganju N. K., Fagherazzi S. (2016). A linear relationship between wave power and erosion determines salt-marsh resilience to violent storms and hurricanes. Proc. Natl. Acad. Sci. 113, 64–68. doi: 10.1073/pnas.1510095112
Li H., Li Z., Shen Z. J., Luo M., Liu Y., Wei M., et al. (2020). Physiological and proteomic responses of mangrove plant Avicennia marina seedlings to simulated periodical inundation. Plant Soil. 450, 231–254. doi: 10.1007/s11104-020-04474-8
Li W. Q., Liu X. J., Khan M. A., Yamaguchi S. (2005). The effect of plant growth regulators, nitric oxide, nitrite and light on the germination of dimorphic seeds of Suaeda salsa under saline conditions. J. Plant Res. 118, 207–214. doi: 10.1007/s10265-005-0212-8
Lopez O. R., Kursar T. A. (2003). Does flood tolerance explain tree species distribution in tropical seasonally flooded habitats? Oecologia (Berlin) 136, 193–204. doi: 10.1007/s00442-003-1259-7
Loreti E., van Veen H., Perata. P. (2016). Plant responses to flooding stress. Curr. Opin. Plant Biol. 33, 64–71. doi: 10.1016/j.pbi.2016.06.005
Ma Y. R., Chen S. H., Chen F. Q., Chen G. H., Xie Z. Q., Liu Y. Y. (2018). Effects of flooding on seed viability and nutrient composition in three riparian shrubs and implications for restoration. J. Freshw. Ecol. 33, 449–460. doi: 10.1080/02705060.2018.1520156
Mcleod E., Chmura G. L., Bouillon S., Salm R., Björk M., Duarte C. M., et al. (2011). A blueprint for blue carbon: toward an improved understanding of the role of vegetated coastal habitats in sequestering CO2. Front. Ecol. Environ. 9, 552–560. doi: 10.1890/110004
Miller R. C., Zedler J. B. (2003). Responses of native and invasive wetland plants to hydroperiod and water depth. Plant Ecol. 167, 57–69. doi: 10.1023/A:1023918619073
Morris J. T., Sundareshwar P. V., Nietch C. T., Kjerfve B., Cahoon D. R. (2002). Responses of coastal wetlands to rising sea level. Ecology 83, 2869–2877. doi: 10.1890/0012-9658(2002)083[2869:ROCWTR]2.0.CO;2
Mozo I., Rodríguez M. E., Monteoliva S., Luquez V. M. C. (2021). Floodwater depth causes different physiological responses during post-fooding in willows. Front. Plant Sci. 12, 575090. doi: 10.3389/fpls.2021.575090
Pezeshki S. R. (2001). Wetland plant responses to soil flooding. Environ. Exp. Bot. 46, 299–312. doi: 10.1016/S0098-8472(01)00107-1
Redelstein R., Dinter T., Hertel D., Leuschner C. (2018). Effects of inundation, nutrient availability and plant species diversity on fine root mass and morphology across a saltmarsh flooding gradient. Front. Plant Sci. 9, 98. doi: 10.3389/fpls.2018.00098
Sandi S. G., Rodriguez J. F., Saintilan N., Riccardi G., Saco P. M. (2018). Rising tides, rising gates: The complex ecogeomorphic response of coastal wetlands to sea-level rise and human interventions. Adv. Water Resour. 114, 135–148. doi: 10.1016/j.advwatres.2018.02.006
SChile L. M., Callaway J. C., Morris J. T., Stralberg D., Parker V. T., Kelly M. (2014). Modeling ttdal marsh distributton with sea- level rise: Evaluattng the role of vegetatton, sediment, and upland habitat in marsh resiliency. PloS One 9, e88760. doi: 10.1371/journal.pone.0088760
SChile L. M., Callaway J. C., Suding K. N., Kelly N. M. (2017). Can community structure track sea- level rise? Stress and competitive controls in tidal wetlands. Ecol. Evol. 7, 1276–1285. doi: 10.1002/ece3.2017.7.issue-4
Schubauer J. P., Hopkinson C. S. (1984). Above- and belowground emergent macrophyte production and turnover in a coastal marsh ecosystem, Georgia. Limnol. Oceanogr. 29, 1052–1065. doi: 10.4319/lo.1984.29.5.1052
Silvestri S., Defina A., Marani M. (2005). Tidal regime, salinity and salt marsh plant zonation. Estuar. Coast. Shelf Sci. 62, 119–130. doi: 10.1016/j.ecss.2004.08.010
Sloey T. M., Howard R. J., Hester M. W. (2016). Response of Schoenoplectus acutus and Schoenoplectus californicus at Different Life- History Stages to Hydrologic Regime. Wetlands 36, 37–46. doi: 10.1007/s13157-015-0713-8
Song J. (2009). Root morphology is related to the phenotypic variation in waterlogging tolerance of two populations of Suaeda salsa under salinity. Plant Soil 324, 231–240. doi: 10.1007/s11104-009-9949-5
Suo R., Sandhu K., Wang M., You F., Conner R., Cober E., et al. (2023). Soybean (Glycine max L.) seed germination in response to waterlogging and cold climate: a review on the genetics and molecular mechanisms of resistance to the abiotic stress. Can. J. Plant Sci. 103, 13–28. doi: 10.1139/cjps-2022-0111
Todd M. J., Muneepeerakul R., Pumo D., Azaele S., Miralles-Wilhelm F., Rinaldo A., et al. (2010). Hydrological drivers of wetland vegetation community distribution within Everglades National Park, Florida. Adv. Water Resour. 33, 1279–1289. doi: 10.1016/j.advwatres.2010.04.003
van Belzen J., van de Koppel J., Kirwan M., van der Wal D., Herman P. M. J., Dakos V., et al. (2017). Vegetation recovery in tidal marshes reveals critical slowing down under increased inundation. Nat. Commun. 8, 15811. doi: 10.1038/ncomms15811
Voesenek L. A. C. J., Bailey-Serres J. (2015). Flood adaptive traits and processes: an overview. New Phytol. 206, 57–73. doi: 10.1111/nph.2015.206.issue-1
Wang Q., Cui B. S., Luo M. (2018). Effectiveness of microtopographic structure in species recovery in degraded salt marshes. Mar. pollut. bulletin 133, 173–181. doi: 10.1016/j.marpolbul.2018.05.037
Wang Q., Cui B. S., Luo M., Qiu D. D., Shi W., Xie C. J. (2019). Microtopographic structures facilitate plant recruitment across a salt marsh tidal gradient. Aquat. conservation: Mar. Freshw. ecosystem 29, 1336–1346. doi: 10.1002/aqc.v29.8
Wang Q., Shao D., Cui B., Ren Z., Ning Z., Gao F., et al. (2022). Artificial modifications lead to the formation of persistent bare patches in saltmarshes. Front. Mar. Sci. 9, 1026736. doi: 10.3389/fmars.2022.1026736
Wang L., Zhao M., Wei S., Song W., Chu X., Li P., et al. (2024). Inundation depth controls leaf photosynthetic capacity by regulating leaf area and N content in an estuarine wetland. Plant Soil. 496, 375–390. doi: 10.1007/s11104-023-06368-x
Waring E. F., Maricle B. R. (2012). Photosynthetic variation and carbon isotope discrimination in invasive wetland grasses in response to flooding. Environ. Exp. Bot. 77, 77–86. doi: 10.1016/j.envexpbot.2011.10.013
Wei G. W., Chen Y. H., Sun X. S., Matsubara S., Luo F. L., Yu F. H. (2021). Elevation-dependent selection for plasticity in leaf and root traits of Polygonum hydropiper in response to flooding. Environ. Exp. Bot. 182, 104331. doi: 10.1016/j.envexpbot.2020.104331
Xie T., Cui B., Li S. (2017). Analysing how plants in coastal wetlands respond to varying tidal regimes throughout their life cycles. Mar. pollut. Bull. 123, 113–121. doi: 10.1016/j.marpolbul.2017.09.017
Zhao M., Han G., Wu H., Song W., Chu X., Li J., et al. (2020). Inundation depth affects ecosystem CO2 and CH4 exchange by changing plant productivity in a freshwater wetland in the Yellow River Estuary. Plant Soil 454, 87–102. doi: 10.1007/s11104-020-04612-2
Zhao M., Li P., Song W., Chu X., Eller F., Wang X., et al. (2023). Inundation depth stimulates plant-mediated CH4 emissions by increasing ecosystem carbon uptake and plant height in an estuarine wetland. Funct. Ecol. 37, 536–550. doi: 10.1111/1365-2435.14258
Zhao J., Malone S. L., Oberbauer S. F., Olivas P. C., Schedlbauer J. L., Staudhammer C. L., et al. (2019). Intensified inundation shifts a freshwater wetland from a CO2 sink to a source. Global Change Biol. 25, 3319–3333. doi: 10.1111/gcb.v25.10
Keywords: tidal inundation, stress, seed emergence, seedling survival, salt marsh
Citation: Wang Q, Luo M, Cui B, Chen C, Xie T, Li X and Lu F (2024) Effects of varied inundation characteristics on early life stages of a salt marsh plant. Front. Mar. Sci. 11:1449034. doi: 10.3389/fmars.2024.1449034
Received: 01 July 2024; Accepted: 26 September 2024;
Published: 16 October 2024.
Edited by:
Donata Melaku Canu, National Institute of Oceanography and Applied Geophysics, ItalyCopyright © 2024 Wang, Luo, Cui, Chen, Xie, Li and Lu. This is an open-access article distributed under the terms of the Creative Commons Attribution License (CC BY). The use, distribution or reproduction in other forums is permitted, provided the original author(s) and the copyright owner(s) are credited and that the original publication in this journal is cited, in accordance with accepted academic practice. No use, distribution or reproduction is permitted which does not comply with these terms.
*Correspondence: Feng Lu, Y3JhYmNhbHZpbkAxMjYuY29t