- 1Lithuanian Sea Museum, Klaipeda, Lithuania
- 2Marine Research Institute, Klaipeda University, Klaipeda, Lithuania
- 3Institute for Terrestrial and Aquatic Wildlife Research, University of Veterinary Medicine Hannover Foundation, Büsum, Germany
The ethical considerations and scepticism over the ecological benefits have fuelled debate about the rehabilitation of wildlife. Although there is evidence that many rehabilitated species are able to survive after rehabilitation, there is a paucity of research on the behaviour of rehabilitated pinnipeds, including grey seals (Halichoerus grypus). In this study, 14 rehabilitated grey seal juveniles were equipped with biotelemetry devices in order to conduct remote post-release monitoring and to investigate ontogeny of their movements in the Baltic Sea. The study revealed that their movements were similar to wild, non-rehabilitated grey seal pups when leaving their natal site: at first, they exhibited highly exploratory behaviour with largely transient movements, then switched into a resident movement pattern, while maximising foraging and minimising travelling time. Neither sex nor year of release, which varied in terms of rehabilitation time and body mass, had a significant effect on the ontogeny of these movements. Movements were significantly influenced by the time after release, suggesting that rehabilitated juveniles have gained experience and developed their movements over time in order to survive in the wild.
1 Introduction
In order to reduce anthropogenic impacts on natural habitats, a number of conservation tools have been established, including the rehabilitation of wildlife (Robinson et al., 2021; Kwok et al., 2021). During the rehabilitation, temporary care is provided for weak, sick or orphaned animals until the individuals become healthy and are released back into their natural environment (O’Hara, 2019; Robinson et al., 2021; Sayer et al., 2021). However, ethical questions and scepticism over the ecological benefits have fuelled debate on rehabilitative treatment of wildlife (Moore et al., 2007; Mullineaux, 2014; Jensen et al., 2017). Sceptics argue, that rehabilitation raises concerns due to risk of disease introduction into the wild populations and genetic changes from disruption of natural selection and host-pathogen co-evolution (Jensen et al., 2017). In addition, elevated stress for animals undergoing care can be counterproductive or even traumatic to the animal as an inciting event (Moore et al., 2007; Mullineaux, 2014). On the other hand, the need for rehabilitation often arises from anthropogenic causes, therefore, there is an obligation to rectify impacts made by humanity, and studies indicate that some species are fully capable to adapt to their natural environment after rehabilitation (Sangster et al., 2020; Hanson et al., 2021; Sayer et al., 2021; Robinson et al., 2021; Cope et al., 2022).
Pinnipeds are one of the most frequently rehabilitated marine mammals worldwide (Simeone et al., 2024). While there is evidence that wildlife rehabilitation is a beneficial tool in conservation efforts directly and indirectly through education, there is a lack of research on the behaviour of rehabilitated pinnipeds with post-release monitoring (Sayer et al., 2021; Cope et al., 2022). However, monitoring them once they are released is a challenge. Pinnipeds spend a significant proportion of time at sea, submerged, or hauled-out on land, rocks or remote islands, limiting the possibility for visual observations (Alava, 2017; Carter et al., 2017; Horning et al., 2017, Horning et al., 2019; Sayer et al., 2021). With rapid technological advancement over the past several decades, a suite of bio-telemetry devices are now available that can remotely monitor animals in these challenging environments (Hussey et al., 2015; Heylen and Nachstheim, 2018; Ronkon et al., 2018). Although bio-telemetry is rapidly increasing the ability to study the behaviour and distribution of marine mammals across their range, the equipment is relatively expensive (Sequeira et al., 2019; Sayer et al., 2021), and this is one of the main reasons for the lack of such studies on rehabilitated animals. Post-release monitoring is crucial for rehabilitation centres (Mullineaux, 2014; Bubac et al., 2019; Sayer et al., 2021) as their goal is to release animals that survive and behave naturally in their native habitat (Wimberger et al., 2010; Sangster et al., 2020), however, the purchase and application of bio-telemetry devices can be challenging due to limited funding (Garces, 2022).
Three seal species are native to the Baltic Sea: ringed seals (Pusa hispida), harbour seals (Phoca vitulina) and grey seals (Halichoerus grypus) (HELCOM, 2018; Ahlgren et al., 2022). The distribution of the species during foraging and annual migrations covers the entire sea, although there are no terrestrial haul-out sites in Latvia and Lithuania (HELCOM, 2018). The Baltic grey seal (Halichoerus grypus grypus) is the most abundant and widespread pinniped in the Baltic Sea (HELCOM, 2018; HELCOM, 2023; Suuronen et al., 2023). These marine mammals are included in the Red Lists of the International Union for Conservation of Nature (IUCN) (Bowen, 2016), HELCOM (2013, 2023) and Lithuania (Rašomavičius, 2021). They are also protected under the European Union Habitats Directive and listed in Annexes II and V (Council of Europe, 1979; European Union, 1992).
Grey seals do not breed or moult on the Lithuanian coast (HELCOM, 2018), which is densely populated by humans. Nevertheless, weak and sick Baltic grey seal pups are brought to shore by currents and found on the coast of Lithuania every year. Baltic grey seals prefer to breed on ice, but the trend of decreasing ice cover in the Baltic forces females to give birth to their pups on the land (Jüssi et al., 2008; HELCOM, 2023). Breeding success is considerably greater when they bread on ice (Jüssi et al., 2008), and additionally, early ice break-up may cause pups to enter the water earlier or more often, which affects their thermoregulation (HELCOM, 2023). This consequence of climate change is thought to be one of the main reasons why these pups end up on Lithuanian shores emaciated and sick. As grey seals are a nationally protected species in Lithuania, they have been rehabilitated at the Lithuanian Sea Museum since 1987.
The investigation of ontogeny of foraging behaviour in juvenile grey seals presents a unique opportunity, given that they do not receive any parental assistance in this regard. In the wild, the weaning process occurs abruptly when mothers return to the sea to replenish their energy stores after lactation phase (Mellish et al., 1999; Bowen et al., 2006). Prior to their initial foraging trip, weaned pups undergo a fasting period that lasts between nine and forty days (Bennett et al., 2007; Noren et al., 2008). Consequently, the pups neither receive parental guidance nor support with regard to the identification of food sources, the location of suitable prey, or the techniques required to capture it. While certain behaviours are genetically predetermined, other aspects of foraging behaviour appear to be the result of learning and experience. After entering the marine habitat for the first time, pups mainly exhibit environmental exploration behaviour (Nowak et al., 2023; Carter et al., 2020; Peschko et al., 2020). Over time and with experience, the duration of their foraging trips decreases, their movements become more tortuous and their behaviour is linked to foraging (Carter et al., 2017, Carter et al., 2020; Peschko et al., 2020; Nowak et al., 2023). The timing and scale of these changes exhibit variation between the sexes and regional sub-populations (Bennett et al., 2010; Carter et al., 2017). Nevertheless, typically adult-like movement strategies are adopted within four months (Carter et al., 2020), and characterised by discrete foraging trips, with the seals commuting between preferred foraging grounds and terrestrial haul-out sites (Russell et al., 2015).
In the case of juvenile grey seals admitted to rehabilitation centres, the loss of their mothers usually occurs prematurely, resulting in the first months of their lives being spent in human care. The initial stages of these individuals’ lives diverge from the typical trajectory of wild seals, prompting inquiries into whether the ontogeny of foraging behaviour adheres to a comparable pattern to that of wild, non-rehabilitated individuals and whether this can be shaped by the specific rehabilitation programmes implemented in different rehabilitation centres. However, there is a knowledge gap in the published studies regarding the movements and behaviour of rehabilitated seals. This makes it challenging to draw meaningful comparisons between the results of different studies.
In this study, 14 rehabilitated grey seal juveniles were equipped with Argos satellite tags in order to conduct a remote post-release monitoring and to investigate the development of foraging trip characteristics. Specifically, we looked into foraging trip duration and distance changes over time as a measure of how juvenile seals adapt their movement patterns in the new environment. We also studied the development of behavioural states within each foraging trip as the seals develop foraging skills and tactics. To accomplish this, a state-space model was applied to investigate the movement behaviour of grey seal juveniles in the Baltic Sea. State-space models are an effective instrument for location data quality control and the derivation of behavioural inferences from animal tracking data (Patterson et al., 2008).
To date, there are no published studies investigating the ontogeny of horizontal movements of grey seal juveniles in the Baltic Sea by analysing trip characteristics and behavioural states. This is true for both rehabilitated and wild, non-rehabilitated seals making this study an important baseline for the dispersal and movement ecology of grey seal juveniles in the Baltic Sea.
2 Materials and methods
2.1 Study area
The Baltic Sea is a shallow, brackish semi-enclosed and non-tidal water body (Rutgersson et al., 2014; Szymczycha and Pempkowiak, 2016; Olli et al., 2022). It covers an area of approximately 400,000 km2 with a coastline of 8,000 km, and is located in northern Europe (Rosentau et al., 2017; Rana et al., 2021). The large latitudinal extent, significant freshwater inflows from the mainland and limited saline, oxygen-rich inflows from the Atlantic Ocean result in strong differences in water characteristics between the different parts of the Baltic Sea (Omstedt et al., 2014; Lappe and Umlauf, 2016; Kaskela and Kotilainen, 2017). This has a significant impact on the distribution and assemblage of prey available to seals, which together with water and topographic characteristics (Szymczycha and Pempkowiak, 2016; Rosentau et al., 2017; Lehmann et al., 2021) may influence the ontogeny of foraging behaviour (Nowak et al., 2023). Grey seals forage and travel in the entire Baltic Sea (van Beest et al., 2019), although no haul-out sites occur along the Latvian and Lithuanian coasts (HELCOM, 2018).
2.2 Study animals and tag deployments
The grey seal pups were rehabilitated at the Lithuanian Sea Museum’s Baltic Sea Animal Rehabilitation Centre, which is located in Smiltyne, Lithuania. After rehabilitation, juveniles were released into their natural habitat. The release site was at the Baltic Sea (55.65042 N, 20.82925 E), approx. 20 km from the Lithuanian coast, and juveniles were transported there by boat.
Grey seal pups admitted to the rehabilitation centre suffered from various health conditions including dehydration and malnutrition, parasitic, bacterial and viral infections, injuries, lesions and bite marks from conspecifics. Upon admittance, the majority of pups still had some or all of their lanugo fur, indicating that they had recently undergone weaning or abandonment while still being dependent. It is worthy of note that grey seal pups are born with a lanugo fur, which is not waterproof. Consequently, they are unable to enter the water until they have shed this fur and attained their adult coat (Hall and Russel, 2018). The average weight of juveniles was 13.9 ± 1.95 kg (mean ± standard deviation). When animals were deemed clinically healthy, exhibited normal behaviour and weighed more than 40 kg (average weight at weaning for wild grey seal pups), all seals were released back into their natural habitat. In total, eighteen randomly selected seals (eleven males and seven females) were equipped with a satellite tag and were subsequently released: eight of them on September 23rd, 2022; ten on July 30th, 2023 (Table 1). Additionally, a flipper tag with a unique code, colour and the name of Lithuanian Sea Museum was attached to the seals’ left hind flipper.
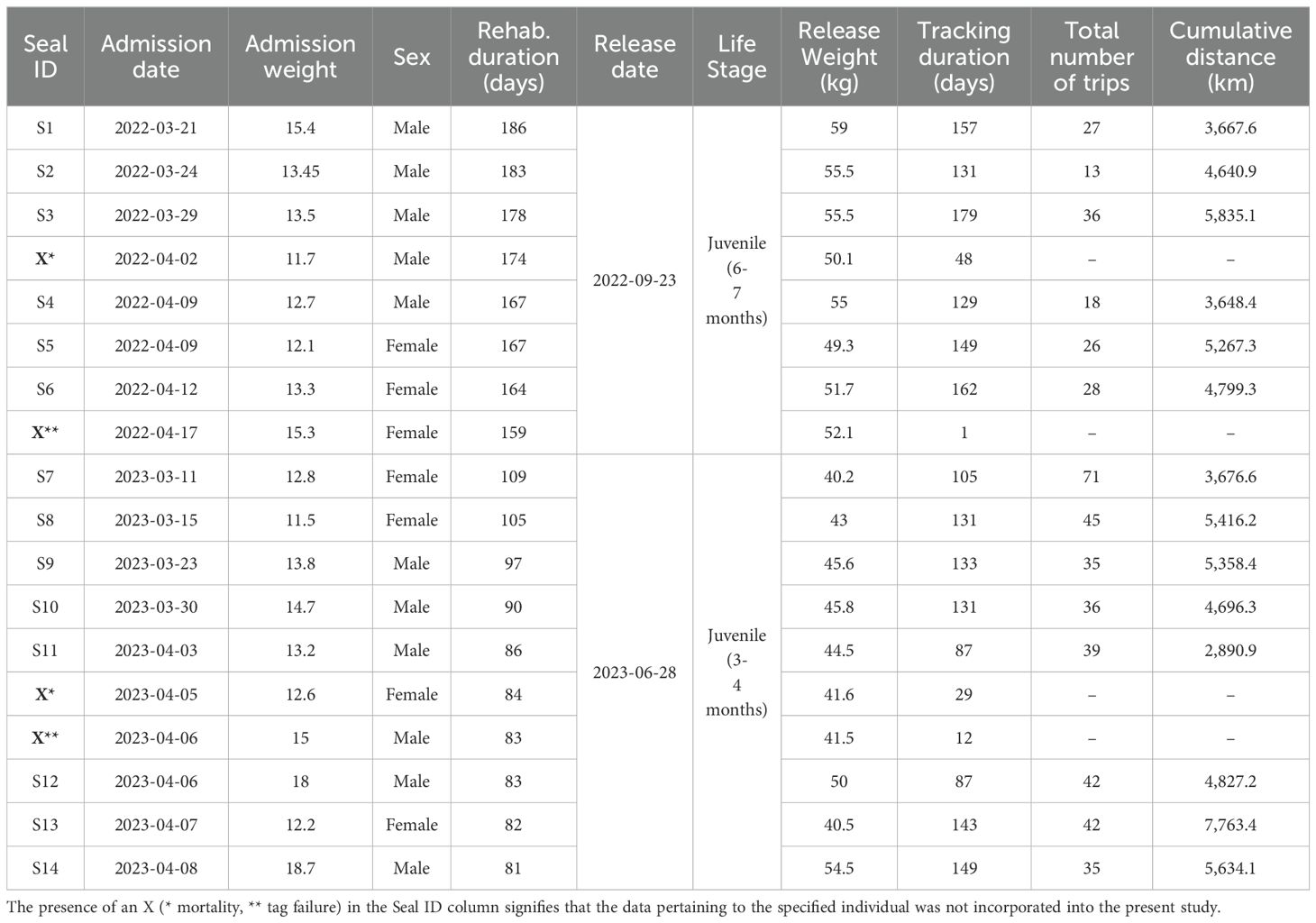
Table 1. A summary of the rehabilitation information and resulting deployments data for all rehabilitated seals.
SPLASH10-297 tags (Wildlife Computers, Redmond, WA) were glued to the seals’ fur on the upper back using epoxy resin (Devcon 5 Minute Epoxy 14270), according to the manufacturer’s instructions. The tag weight was 132 g (size: length - 86 mm, width - 55 mm, height - 29 mm). Devices were attached one day before the release into the wild. These remain on the animal until the following annual moult (Heylen and Nachstheim, 2018; Field et al., 2012). Further details on the tagging system and programming may be found in section 2.3.
Four individuals were excluded from the analysis due to relatively short deployment durations (Table 1). This was caused by the mortality of two individuals, and two tag failures. In order to identify these events, previous movements, the tag’s signal strength and frequency, dry time, temperature and depth histograms were evaluated. If a tag had reported normally and then stopped all transmissions, it was assumed that the seal was alive, but the tag itself was damaged or fell off. Detached tags would sink and not transmit if detached at sea. If a tag started to continuously transmit high-quality location data, indicating that the seal was floating at the surface or lying on the land for too long, mortality of the animal was assumed.
Both of the seals which suffered mortality stopped their transmissions near the coast of Estonia. One of them was reportedly bycaught in fishing gear, the other one’s cause of death remains unknown. After two weeks, the tag stopped all transmissions and re-started them a month later, but the locations remained high quality for several days, with constant depth and temperature, indicating that the animal was floating on the surface. The other two cases were attributed to tag failure, since tags had reported normally and then stopped all the transmissions.
2.3 Location data filtering and analysis
The SPLASH10-297 tags provide location estimates via the Argos satellite network using the Doppler shift. Typical location errors range between 500 m and 10 km (Costa et al., 2010). To account for this, a state-space model (SSM) was fitted to the animal tracks using the “aniMotum” package (Jonsen et al., 2023) in the R software version 4.3.2. In a first step, locations were filtered exceeding a maximum speed of 3 m/s in accordance to previous studies on grey seals (Nowak et al., 2020; Planque et al., 2020; Baylis et al., 2019). The SSM then provided improved, regularised location estimates at 2 h intervals, by taking the associated location uncertainties (Argos location quality class, semi-major axis, semi-minor axis, ellipse orientation) into account. Since the SSM implemented in “aniMotum” does not consider potential barriers to animal movement, the predicted path follows over land, which is implausible for marine species such as grey seals. Therefore, the “pathroutr” package (London, 2020) was used to efficiently re-route locations from land back to water. Further details on this approach may be found in Jonsen et al. (2023).
In addition, the SSMs provide behavioural state estimation along the movement track. The SSM estimates the persistence of consecutive pairs of seal relocations along an entire track, identifying segments of relatively low or high persistence (Jonsen et al., 2018) movements. Move persistence, an index of movement behaviour (γt), is a continuous (0 – low persistence, 1 – high persistence), time-varying latent variable that represents changes in movement pattern based on autocorrelation in travel speed and direction (Jonsen et al., 2023, Jonsen et al., 2018). Thus, it was assumed that track segments of low movement persistence represented a resident behavioural state indicative of area-restricted search and foraging (γt values from 0 to 0.5), while segments of high movement persistence characterised a transient behavioural state indicative of travelling (γt values from 0.5 to 1).
Any movements between haul-out events were defined as a foraging trip, covering distances of ≥1 km, and a duration of ≥6 hours. Argos locations within the vicinity of haul-out sites are often discarded in the analyses due to the difficulty of interpreting behaviour near haul-out sites (Vincent et al., 2016), therefore shorter trips were omitted. The tags used in the study carried wet-dry sensors, thus we were able to identify haul-out behaviour and locations. An hour was assumed as “dry” if tag measured dry for more than 60% of the hour, and “wet” if it was deeper than 2 m for more than 60% of the hour. The trip distance was defined as summed distances between each consecutive pair of interpolated locations between two haul-out events, and calculated using the function “trackDistance” from the R package “trip” (Sumner et al., 2009). The trip duration was assumed as the time period between the first and last location after and before a haul-out event, as indicated by the wet-dry sensor. However, as the definition of the haul-out was established, it is important to emphasise that the precise duration and distance of a trip cannot be calculated with certainty.
2.4 Statistical analysis
All statistical analyses were conducted in R version 4.3.2 using R studio 2023.12.0.369 for macOS. Generalised additive mixed models (GAMMs) were used to investigate the effect of sex (male/female), release year (2022/2023) and trip sequence number since release (i.e. number of trips after release) on the three response variables: trip duration, trip distance and proportion of behavioural states (resident/transient) during each trip. Furthermore, an interaction term between sex and trip sequence number was added to analyse sex-specific ontogenetic trends over time. In order to assess development of trip characteristics since release, the consecutive trip sequence number was used as a metric instead of time. This was based on the relatively large inter-individual variability in trip duration and distance.
All GAMMs were fitted using the function “gamm” and F values were found using the function “summary” from the “mgcv” package (Wood, 2017). Trip duration and distance were modelled with a gamma distribution, and the proportion of a behavioural states with a binomial distribution. Number of knots (k) were selected according to the estimated degrees of freedom (EDF), k-index as well as p-value obtained with the function “gam.check”. In order to account for individual variability, seal identities were included as random effects. Diagnostic plots were inspected to assess overall model fit and to accept the final model under the assumptions of homoskedasticity and normality of residuals. Diagnostic plots included Q-Q, histogram of residuals, residuals vs linear predictor as well as observed vs fitted values. Additionally, autocorrelation of residuals was tested, using residual autocorrelation plotting functions in R. As temporal autocorrelation was identified, an auto-regressive model of the order 1 (AR1) was included to the model, completely removing the autocorrelation (Marton, 2017).
Model selection was based on the Akaike’s Information Criterion (AIC) and the difference in AIC between candidate models from the package “MuMIn” (Burnham and Anderson, 2002), using the “dredge” function. Thus, the most parsimonious model was the one with all diagnostic plots meeting the required assumptions, the lowest AIC value with a delta (Δi) <2 and highest deviance explained. Model selection followed the steps described by Symonds and Moussalli (2010).
2.5 Ethics approval
The collection of orphaned or sick seal pups from the wild, their rehabilitation and release back into the Baltic Sea with tags was carried out in accordance with national legislation of the Republic of Lithuania. State Food and Veterinary Service registered and granted a veterinary registration number (LT 96-21-002) to the Baltic Sea Animal Rehabilitation Centre of the Lithuanian Sea Museum, which allows this institution to carry out rehabilitation of wild animals legally. According to the Lithuanian Wildlife Act (Date of adoption 1997-11-06; summary version from 2021-05-01 to 2024-04-30), keeping of wild animals for the purposes of care and treatment does not require a separate permit from the government and is therefore not presented here. However, seal tagging was conducted under the permissions (SR-215 and SR-216) of the Environmental Protection Agency of the Republic of Lithuania.
3 Results
3.1 Spatial usage in the Baltic Sea
The rehabilitated Baltic grey seal juveniles were tracked during two periods: in 2022-2023 and 2023-2024. Both release events took place at the same location (55.65042 N, 20.82925 E) in the Baltic Sea, approx. 20 km from the coast, when seals were released directly into the water (Figure 1).
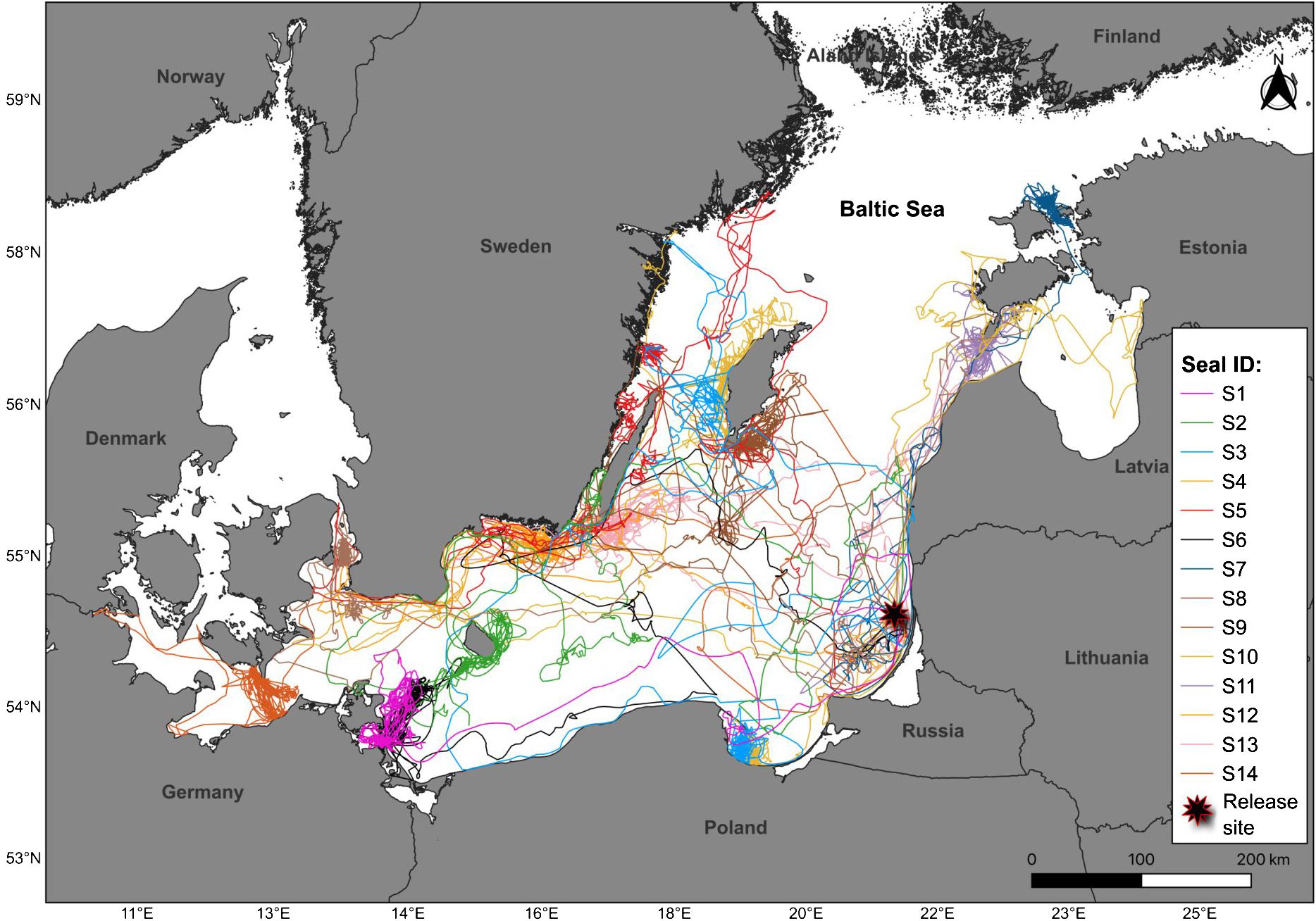
Figure 1. Map showing the horizontal movements of rehabilitated grey seal pups (N=14) in the Baltic Sea. Release site is indicated by a black star. Visualisation was done in Qgis 3.30.0-s Hertogenbosch for macOS. The data set of administrative boundaries at country level belongs to EuroGeographics and UN-FAO (https://ec.europa.eu/eurostat/web/gisco/geodata/reference-data/administrative-units-statistical-units/countries/).
Seal juveniles dispersed widely across the Baltic Sea and showed a high inter-individual variability in directionality, but there were a few main areas targeted, including the north-eastern, southern and south-western parts of the Baltic Sea (Figure 1). Until the tags stopped transmitting, S3 and S4 - were in the bay of Gdansk near Poland, S1 and S6 - in the bay of Greifswald near Germany, S14 – in the bay of Mecklenburg near Germany and Denmark, S8 – the Öresund between the Danish and Swedish coasts, S2 – near the island of Bornhlom, S9 and S10 - near the Swedish island Gotland, S13 – near the island of Öland, S12 and S5 – in the bay of Hanö and south-eastern coast of Sweden, S7 and S11 – near the islands of Estonia, including Saaremaa, Hiiumaa and Vormsi. None of the juveniles appeared near the coasts of Lithuania, Latvia, Finland and Russia until the tags stopped transmitting.
3.2 Trip characteristics
During the study period, juveniles made a total of 493 trips, covering 68,121.7 km. These values varied between individuals: the number of trips ranged from 13 to 71, and cumulative distances from 2,890.9 to 7,763.4 km (Table 1).
In terms of trip characteristics, the median duration among the individuals varied quite widely from 14 h to 132 h, and the distance from 14.9 to 169.7 km. The maximum values for trip duration and distance covered 1,800h (75 days) and 3,460.8 km, respectively. In general, median for trip characteristics were 20 ± 8.74 h and 26.6 ± 11.74 km (Figure 2).
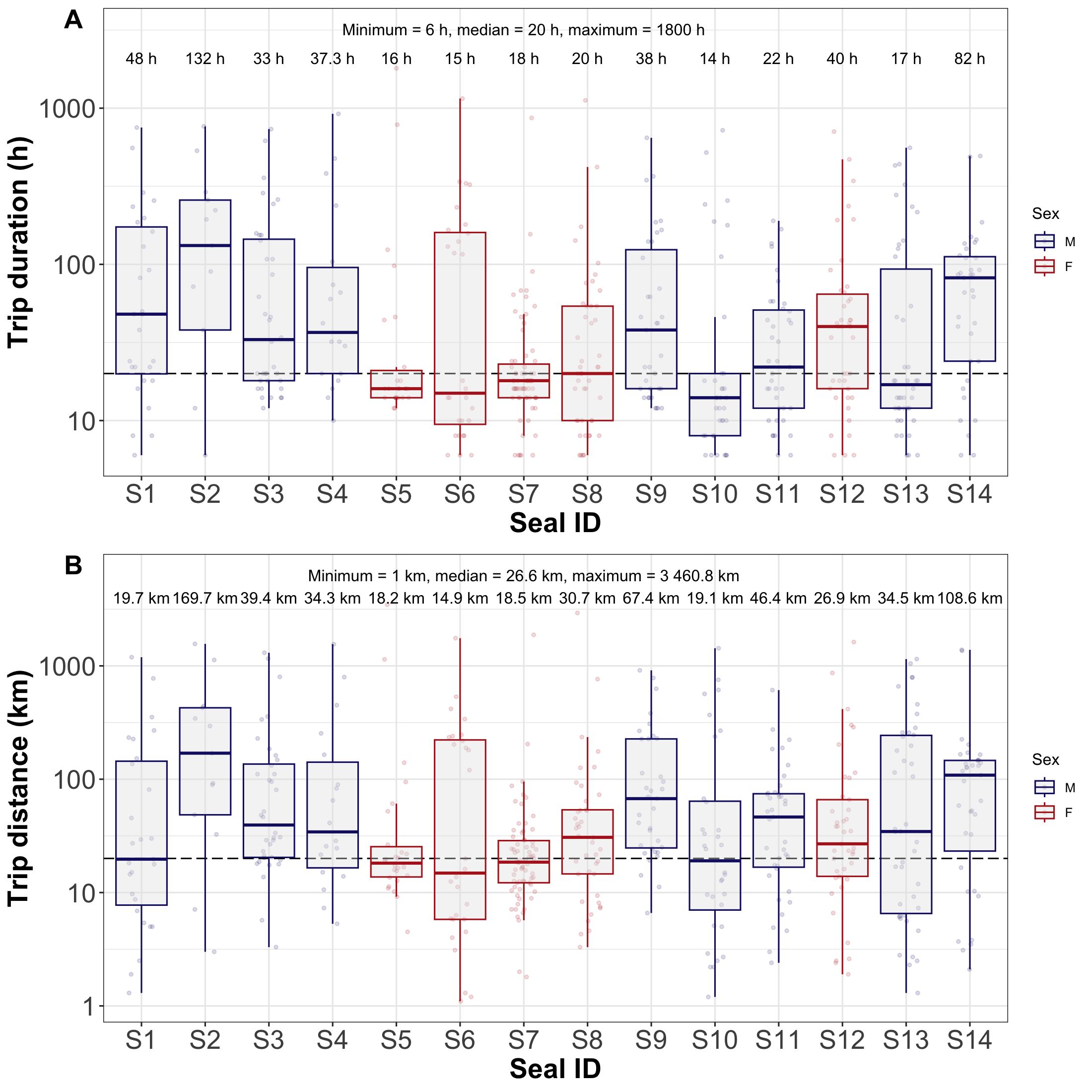
Figure 2. Box plots showing the duration (A) and distance (B) of trips for each individual disaggregated by sex. Median values of each individual are represented at the upper part; overall minimum, median and maximum values are shown in the top-centre of box plots. Black dashed line shows overall median value. The values on the y-axis were log-transformed in order to account for outliers and achieve a better data visualisation.
Statistical analysis revealed that neither the year of release nor sex influenced the ontogeny of trip characteristics. The interaction between sex and trip sequence number was also not significant, and therefore was not retained in the final model. However, the trip sequence number since release had a significant impact on both the trip duration (F=7.24 p<0.0001) and distance (F=7.91 p<0.0001) (Figure 3).
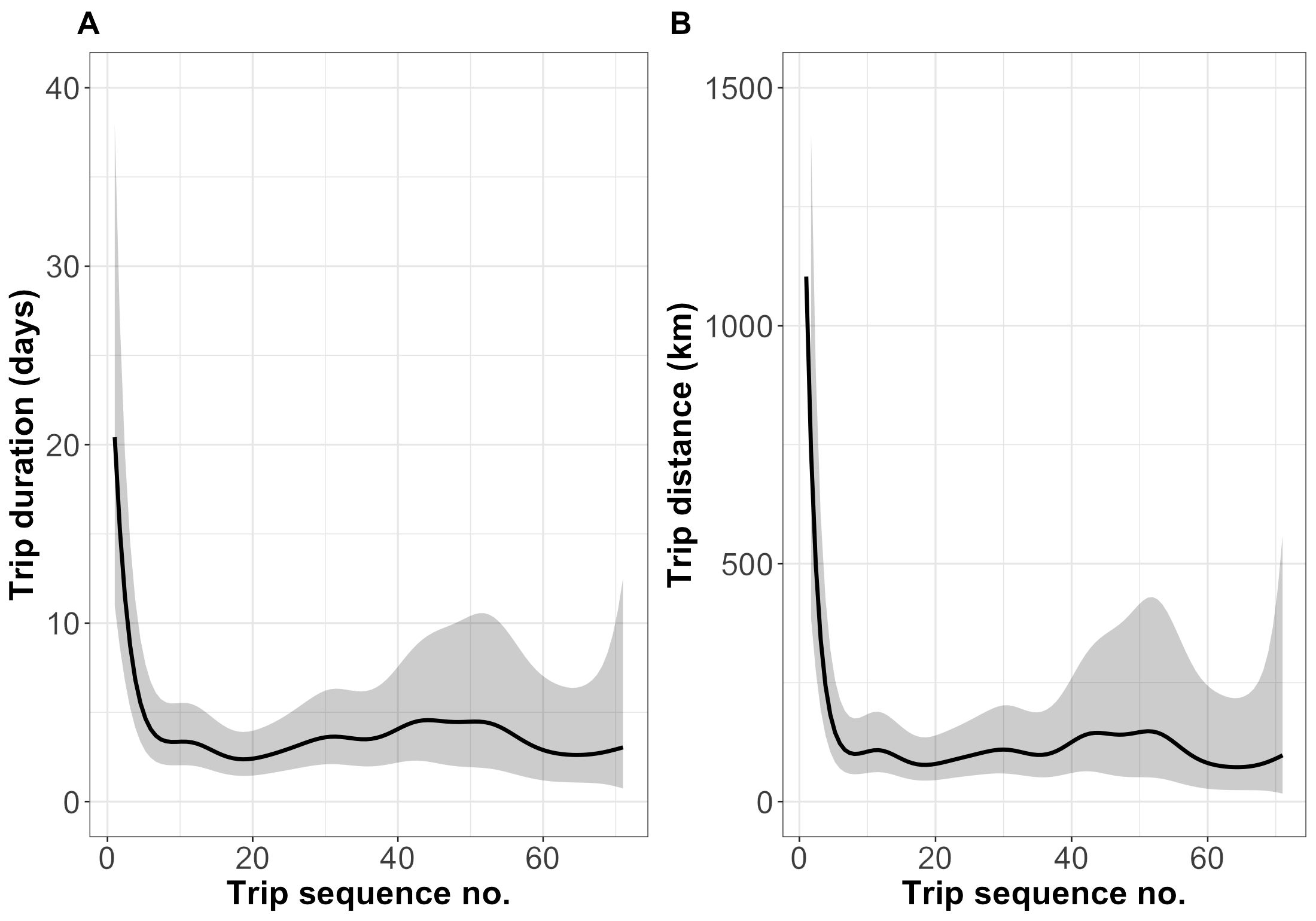
Figure 3. Model-predicted development of trip duration (A) and distance (B) over time, represented as number of trips since release. Solid lines show population mean responses, with associated GAMM-based 95% confidence intervals (shaded areas).
The model predictions show that the duration and distance of trips peaked during the first trips. Thus, the previously reported maximum values of the trip characteristics were found within the 1-2 months after release. With seemingly every trip, and as time passed after the release, trips became shorter in both duration and distance, until stabilised. Following the 20th trip, a stable pattern appears to emerge, with trip duration and distance approximating 2.5 to 4 days and 60 to 120 km, respectively (Figure 3).
3.3 Behavioural states
In this study, we also aimed to determine whether the year of release, sex and trip sequence number had an influence on the allocation of behavioural states across the trips of rehabilitated grey seals. In the map representing behavioural states of rehabilitated seals (Figure 4) can be seen that low move persistence seems to occur mainly in coastal areas and around islands. Thus, grey seal juveniles seem to cross the Baltic Sea in many ways but do not stop for foraging in the deeper, more offshore parts in most cases.
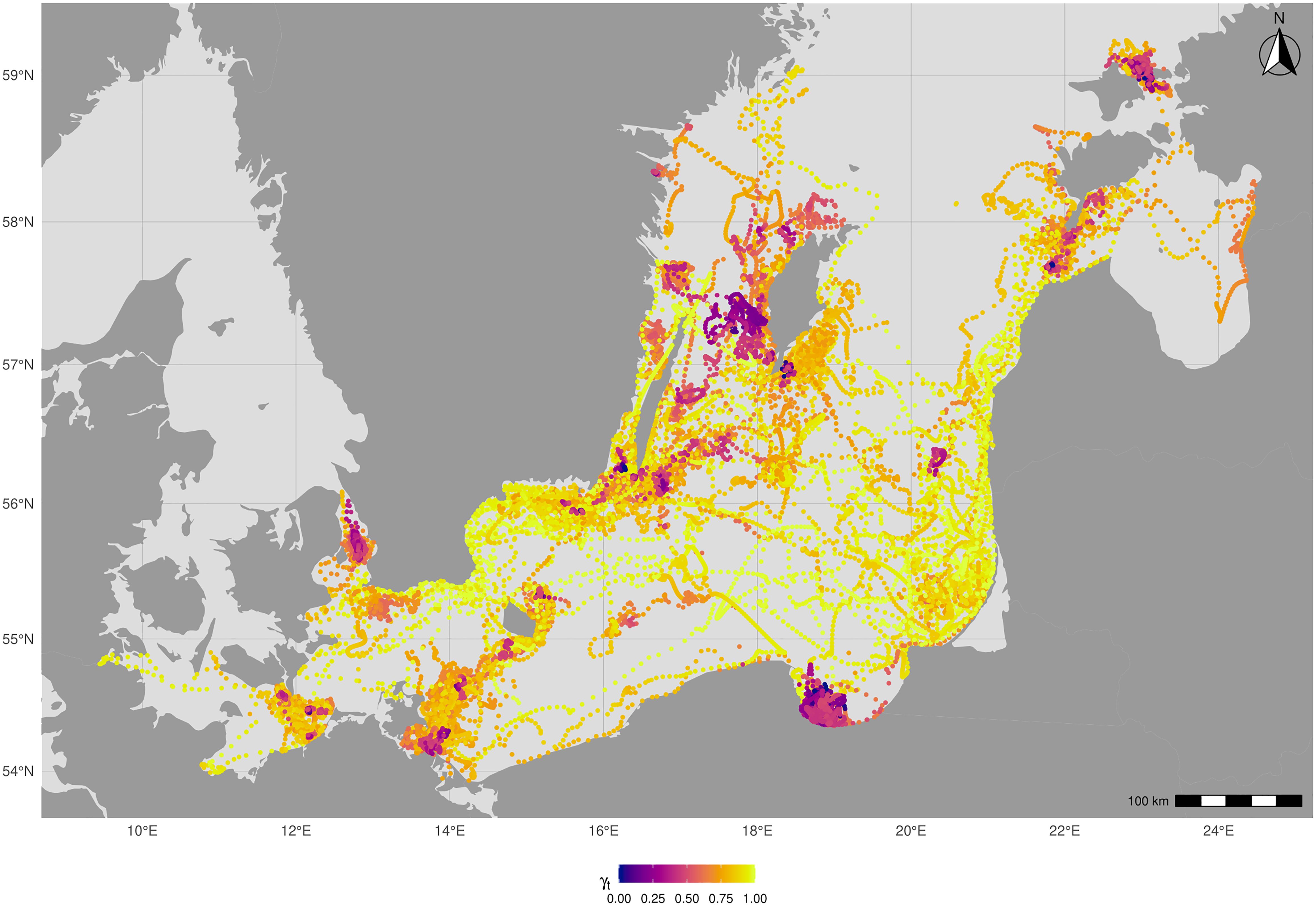
Figure 4. Map showing the behavioral state of rehabilitated grey seal pups in the Baltic Sea. The state was characterised by the γt value: lower values indicate resident areas, higher values indicate transit areas. Visualisation was done in R 4.3.2 using R studio 2023.12.0.369 for macOS (https://cran.r-project.org/bin/macosx/).
Statistical analysis showed that release year and sex had no significant effect on the ontogeny of behavioural states of rehabilitated seals. There was also no effect of the interaction between sex and trip sequence number. However, similarly as in the case of trip characteristics, trip sequence number significantly affected the probability of behavioural states (F=8.61, p<0.0001) (Figure 5). As predicted by the model, the patterns of behavioural states changed rapidly. By the 10th trip, the proportion of transient movements reached a maximum, subsequently declining, while the proportion of resident movements reached a minimum, subsequently increasing. After the 20th trip, a stable pattern of mostly resident movements and few phases of transient movements seemed to be established.
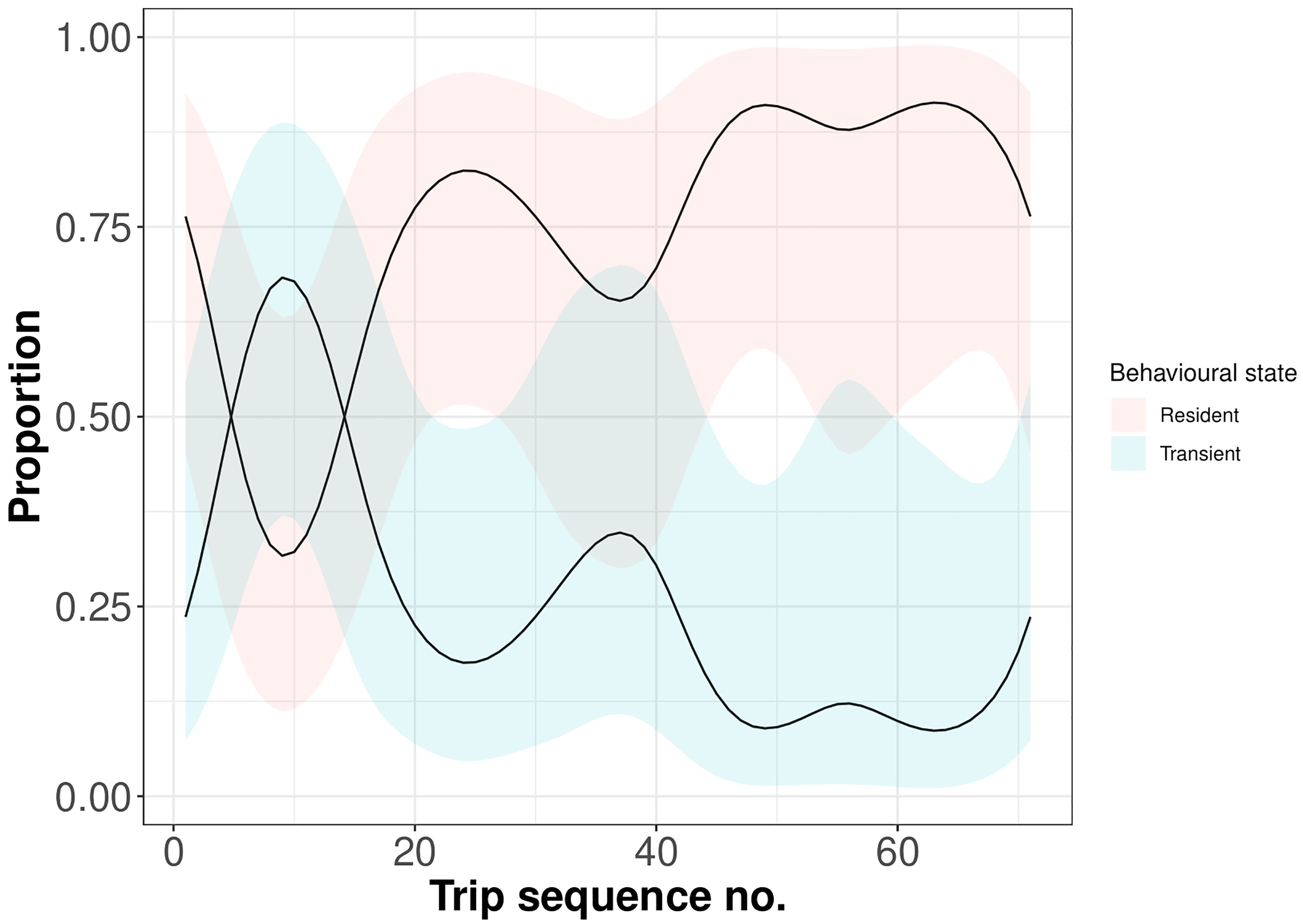
Figure 5. Model-predicted ontogeny of behavioural states over time since release. Solid lines show population mean responses, with associated GAMM-based 95% confidence intervals (shaded areas). Red denotes the movement state inferred as resident; blue denotes the movement state inferred as transient. Proportion values are derived from move persistence γt parameter (scaled from 0 to 1).
4 Discussion
Rehabilitation is not a new concept and has been carried out globally for centuries (O’Hara, 2019). While there is evidence that many rehabilitated species are able to survive after rehabilitation, there is a lack of post-release monitoring of rehabilitated pinnipeds, especially with respect to their behaviour (Mullineaux, 2014; Bubac et al., 2019; Sayer et al., 2021; Cope et al., 2022). In this study, we conducted remote post-release monitoring and aimed to analyse the development of horizontal movement patterns of rehabilitated grey seal juveniles in the Baltic Sea.
4.1 Spatial usage in the Baltic Sea
The study revealed that the rehabilitated seals targeted areas in the north-eastern, southern and south-western parts of the Baltic Sea, including the bays of Gdansk, Mecklenburg, Greifswald and Hanö, the islands of Bornholm, Gotland, Öland, Saaremaa, Hiiumaa and Vormsi, the Öresund and the south-eastern part of the Swedish coast. Until the tags stopped transmitting, none of the juveniles appeared near the coasts of Lithuania, Latvia, Finland and Russia. According to current knowledge, all of the targeted areas have known haul-out sites (HELCOM, 2018; Galatius et al., 2020), and therefore it appears that rehabilitated seal juveniles have joined wild grey seal colonies in the Baltic Sea. As for the Lithuanian and Latvian coasts, there are no known seal haul-out sites along these countries, and the rehabilitated seals have not settled in these areas either (HELCOM, 2018). However, for reasons that remain unknown, none of the rehabilitated seals have appeared near the coasts of Finland or Russia, although grey seal haul-out sites also exist in these areas (Loseva et al., 2023; HELCOM, 2018). It is possible that the presence of seals near the coasts of these countries was not detected due to the small sample size of the study and the relatively short monitoring period, as the tags used in the study have a limited battery lifespan (Sequeira et al., 2019; Sayer et al., 2021). It is therefore possible that they appeared in mentioned areas at a later date when they were no longer being remotely monitored.
For long-term monitoring, internal tags can be used. These offer the advantage of a death signal, which could prove beneficial for post-release monitoring of rehabilitated animals. However, an application requires anaesthesia and surgery to insert the devices, as well as the involvement of skilled personnel and veterinary care. Furthermore, these devices have the disadvantage of not being able to provide the periodic signals which are traditionally used to check that the tags are still deployed and to ensure the quality of the data (Horning et al., 2008; Horning and Mellish, 2009; Horning et al., 2017). The summary data are transmitted only post-mortem, once the positively buoyant device has been released from the decomposing or consumed body (Horning et al., 2017). Therefore, their use should be carefully considered in light of these limitations.
Flipper tagging represents another option for long-term monitoring of rehabilitated seals. Although this technique does not allow for continuous tracking of the animals at sea, some seals can be resighted and identified when they haul-out (Sayer et al., 2021). This cost-effective method has been used for decades to assess survival, home range, migration, population development, and a plethora of other research questions (Hastings et al., 2017; van Neer et al., 2020). However, tag loss is a common occurrence that must be taken into account to prevent biased estimates of vital rates (Testa and Rothery, 1992; Vincent et al., 2002; Hastings et al., 2017).
4.2 Development of trip characteristics and comparison with wild, non-rehabilitated juveniles
In terms of the factors influencing the development of horizontal movements of rehabilitated seals, it was found that the trip characteristics were significantly influenced by the trip sequence number after release, i.e. a measure of time after release. In other studies analysing the behaviour of wild, non-rehabilitated grey seal pups after leaving the colony, time was also identified as a significant factor (Carter et al., 2017, Carter et al., 2020; Peschko et al., 2020; Nowak et al., 2023).
Nevertheless, minor discrepancies were identified in the duration of behavioural development between rehabilitated and wild, non-rehabilitated juveniles. Carter et al. (2020) found that grey seal pups developed their adult-like movement strategies within four months of leaving the natal site. Our results show, that trip characteristics of rehabilitated seals stabilised over the first 1-2 months of their lives at-sea, suggesting that they gained experience more quickly. It is important to note that during rehabilitation, seals spend much of their time in the pools where they are fed, and this may be the reason for the faster adaptation, whereas wild, non-rehabilitated animals may need more time to become accustomed to the marine environment after being weaned. Furthermore, it is essential to consider the impact of seasonal variations. Grey seal pups are born and weaned during February-March in the Baltic Sea (Jüssi et al., 2008) and during November-January in the North Sea (Abt and Engler, 2009). In contrast, those that have been rehabilitated are typically released back into the sea during the warmer season. This suggests that wild, non-rehabilitated seals may be exposed to more extreme environmental conditions, including storms, colder water temperatures, reduced prey availability. Such circumstances may also be a contributing factor to the challenges encountered in the development of the movements of wild, non-rehabilitated pups.
Neither sex nor year of release had a significant effect on trip duration and distance in rehabilitated seals. Nowak et al. (2023) found a similar result in their study and intrinsic factors such as body mass did not influence the development of trip characteristics of wild, non-rehabilitated grey seal pups in the Scotian Shelf. Baylis et al. (2019) studied the spatial usage of grey seal pups along the Iceland coast, and also found that body mass provided little insight into individual variation in reported movements. The effect of sex was only weakly supported in the study of Nowak et al. (2023) and was therefore not retained in the final model. On the other hand, Carter et al. (2017) found that sex did not influence trip duration, but did influence trip distance in the Celtic and Irish Seas. However, no compelling evidence was observed to suggest such a difference in the North Sea. This suggests that the results of the study may also depend on the geographical region and local environmental conditions. The different subspecies of seals being compared may also be relevant: the Baltic Sea is home to subspecies Halichoerus grypus grypus, while grey seals from the North Atlantic Ocean belong to the Atlantic subspecies Halichoerus grypus atlantica (Galatius et al., 2020; Olsen et al., 2016). Nevertheless, this study analysed the behaviour of 5 females and 9 males, which may be an insufficient sample size to reveal subtle statistical differences between the sexes.
We also found that the very first trips immediately after release were the longest in terms of trip duration and distance. Based on model predictions, the first trips lasted about 20 days and covered 1100 km, on average. Long first trips may indicate an exploratory behaviour, which is also common in wild, non-rehabilitated grey seal pups after leaving their natal haul-out site (Nowak et al., 2023; Peschko et al., 2020). Additionally, it is a characteristic of many seabird species, including juvenile grey-headed albatross (Thalassarche chrysostoma), wandering albatross (Diomedea exulans), king penguin (Aptenodytes patagonicus), and other pinnipeds, such as northern fur seals (Callorhinus ursinus) (Frankish et al., 2022; Orgeret et al., 2019; Riotte-Lambert and Weimerskirch, 2013; Lea et al., 2010). The observation of analogous behavioural patterns between species and even taxa suggests that the development of foraging behaviour in the wild can often be explained by the exploration-refinement hypothesis. This hypothesis predicts that foraging behaviour is learned during individual exploratory behaviours in early life, and eventually canalised with age and experience (Votier et al., 2017). However, it is important to note that all of the aforementioned species are abandoned at their natal site and naïve when they venture to the sea for the first time. They do not receive parental guidance in foraging strategies, which may make this particular ontogenic process relevant only to these species.
As time passed since release and rehabilitated seals gained experience in foraging, trips became shorter and more consistent in terms of duration and distance. Similarly, the trips of wild pups shortened with time since leaving the colony, which the authors also attributed to individuals gaining experience and developing their movements (Nowak et al., 2023; Carter et al., 2020; Peschko et al., 2020). The model prediction showed that during the period when trip characteristics had already stabilised, the trip duration of rehabilitated seals was approximately 2.5 to 4 days. Compared to the results of other authors who conducted studies in other regions, the trip duration in the Celtic and Irish Seas was around 2.5 days (Carter et al., 2017) and 9-10 days for pups in the North Sea and Scotian Shelf (Nowak et al., 2023; Peschko et al., 2020). Regarding the distance of the trips, the situation was similar and varied among regions. As predicted by the model, stabilised trip distance for rehabilitated seals varied from around 60 to 120 km, compared to around 50-130 km and 300-350 km in the Celtic and Irish Seas and the North Sea, respectively (Carter et al., 2017). Thus, the results for rehabilitated seals were similar to those for wild, non-rehabilitated seals in the Irish and Celtic Seas, but relatively different from those in the North Sea. However, different geographical regions may lead to differences in movement patterns, for instance due to local physical characteristics (McNamara and Houston, 2008; Illius and O’Connor, 2003), prey abundance and distribution (McNamara and Houston, 2008; Tinker et al., 2008), haul-out distribution and availability, predation risk, etc (McNamara and Houston, 2008). All of these factors may partly explain the differences in trip duration and distance between regions, but the exact mechanism and underlying processes causing these differences remain to be determined.
4.3 Development of behavioural states and comparison with wild, non-rehabilitated juveniles
The study revealed that the number of trips had a significant effect on the ontogeny of behavioural states. However, sex and year of release (when there were variations in body mass and rehabilitation period for juveniles) were found to have no influence. In comparison to other studies, Nowak et al. (2023) identified sex as a significant predictor of movement persistence, with females demonstrating lower movement persistence than males on the Scotian Shelf. Movement persistence is estimated using autocorrelation in both speed and direction as a behavioural index that varies continuously between 0 (low movement persistence, i.e. area-restricted search) and 1 (high movement persistence, i.e. directed travelling) (Jonsen et al., 2018). Accordingly, the data indicates that females on the Scotian Shelf demonstrated a greater tendency towards area-restricted search behaviour than males. Carter et al. (2020) employed a different methodology, but also identified sex-specific outcomes. It was found that there were significant differences in activity budgets between male and female Welsh pups, but not Scottish pups. The research indicated that Welsh females demonstrated higher probability of remaining in a foraging state than males, equating to longer foraging bouts. Regarding the body mass, Nowak et al. (2023) pointed out that body mass was not a useful predictor, as we also found in our study. Previously, it was noted that the first trips made by grey seal juveniles immediately after release were long, suggesting that their behaviour was exploratory rather than targeted foraging. This hypothesis was supported by analysing the development of move persistence since release. Based on the model predictions, the probability of a transient state, or simply travelling behaviour, characterised by high move persistence increased immediately after release until it reached a peak and started to decrease. In opposite to this, the probability of a resident state initially decreased and then started to increase until it reached a dominant phase. A stable pattern seems to establish following the 20th trip with largely resident movements and only low proportion of transient movements. This means that after an exploratory phase, juveniles switched to a resident-dominated behavioural pattern - maximising foraging time and minimising travel time - suggesting that rehabilitated seals have gained experience and adapted their foraging tactics over time in order to survive in the wild.
The findings of other authors also support the results of our study. In recent studies, Nowak et al. (2023) and Carter et al. (2020) demonstrated that, following departure from the colony, non-rehabilitated wild pups exhibited a similar behavioural pattern, with a focus on exploration. The study conducted by Nowak et al. (2023) revealed that, initially, move persistence was high but then decreased over time, indicating a foraging behaviour pattern. Carter et al. (2020) observed that with each passing week travel behaviour became more directed and foraging behaviour more tortuous. In both studies, the juveniles switched from exploratory to foraging behaviour as they gained experience. It is important to note that this manner of behavioural development is not only common to grey seal juveniles, but has also been observed in other species of pinnipeds and seabirds. These include juvenile southern elephant seal (Mirounga leonina), northern fur seal, grey-headed albatross, wandering albatross, Amsterdam albatross (Diomedea amsterdamensis), king penguin, and ect (Delord et al., 2024; Foo et al., 2023; Frankish et al., 2022; Campioni et al., 2019; Orgeret et al., 2019; Riotte-Lambert and Weimerskirch, 2013; Lea et al., 2010). These results for grey seal juveniles, as well as for seabirds and other pinniped species, futher support the idea that the development of foraging behaviour can be defined by the exploration-refinement hypothesis (Votier et al., 2017). This also appears to be a common phenomenon in rehabilitated grey seal pups, as suggested by the results of this study.
While our findings align with those of other studies it is important to acknowledge the potential for bias in this investigation, as seals are also capable of undertaking foraging trips in a direct and straightforward manner across the sea, which are typically classified as travel trips (Vincent et al., 2016). However, we do not anticipate that these activities constituted a significant proportion of the time-activity budget for these animals, given the validity of SSMs and the established link between area-restricted search and foraging behaviour (Dragon et al., 2012).
Furthermore, low movement persistence can be attributed to surface behaviours such as resting at sea, which might be conflated with foraging. Grey seal pups engage in this behaviour with some regularity, representing approximately 27% of their time at sea on average (Carter et al., 2020). It is therefore possible that seals are spending extended amounts of time at the surface due to starvation and exhaustion. However, this is unlikely as seals continue to transmit and do not suffer mortality once this pattern is established. Although resting may account for some proportion of the activity budget, the areas of restricted movement are certainly related to foraging, as resting is more likely to be associated with digestion and recovery from active feeding (Vance et al., 2021).
The findings of our study demonstrated that both trip characteristics and behavioural states stabilised over time, with seals exhibiting a consistent pattern of movements between the shore and the sea, indicating normal behaviour of the seals. However, to fully validate this hypothesis, further analysis of diving behaviour is required in order to establish a link between the horizontal and vertical movements of rehabilitated seals.
5 Conclusion
In order to assess the ontogeny of horizontal movements of rehabilitated grey seal juveniles in the Baltic Sea, Argos satellite tags were deployed on fourteen individuals, which collected a total of 1 873 days of data following their release. The study revealed that the seals moved towards the north-eastern, southern and south-western parts of the Baltic Sea. None of them appeared near the coasts of Lithuania, Latvia, Finland and Russia, as observed until the end of deployment. Their movements were remarkably similar to those of wild, non-rehabilitated grey seal pups after leaving their natal site, and involved several phases: at first, they exhibited highly exploratory behaviour with largely transient movements and long foraging trips, then switched into a resident movement pattern with substantially shorter foraging trips, while maximising foraging and minimising travelling time. Neither sex nor year of release, which varied in terms of rehabilitation time and body mass, had a significant effect on the ontogeny of these movements. Movement patterns were significantly influenced by the time after release, suggesting that juveniles have gained experience and adapted their foraging tactics over time in order to survive in the wild.
This study highlighted a gap in the field of rehabilitated grey seals, particularly in the area of behavioural analysis, making it challenging to draw meaningful comparisons between study results. As the benefits of rehabilitation are still being debated, we encourage researchers to address this knowledge gap in future studies. Ideally, both wild and rehabilitated seals should be studied in a comparative approach.
Data availability statement
The raw data supporting the conclusions of this article will be made available by the authors, without undue reservation.
Ethics statement
The animal study was approved by Environmental Protection Agency of the Republic of Lithuania. The study was conducted in accordance with the local legislation and institutional requirements.
Author contributions
LL-K: Conceptualization, Data curation, Formal analysis, Investigation, Methodology, Visualization, Writing – original draft, Writing – review & editing, Funding acquisition. DN: Conceptualization, Formal analysis, Investigation, Methodology, Supervision, Writing – review & editing. US: Conceptualization, Investigation, Supervision, Writing – review & editing. ŽK: Data curation, Supervision, Writing – review & editing, Funding acquisition.
Funding
The author(s) declare financial support was received for the research, authorship, and/or publication of this article. The purchase of the satellite tags was funded by LIDL. The company had no influence on the results of the study.
Acknowledgments
We would like to thank all staff and volunteers involved in the seal rehabilitation in the Lithuanian Sea Museum’s Baltic Sea Animal Rehabilitation Centre for their relentless effort and dedication. Also, thank you to everyone who took part and helped to release the seals back into the wild. Finally, we would like to thank our sponsors, Lidl Lithuania, whose support enabled us to purchase the transmitters used in this study.
Conflict of interest
The authors declare that the research was conducted in the absence of any commercial or financial relationships that could be construed as a potential conflict of interest.
Publisher’s note
All claims expressed in this article are solely those of the authors and do not necessarily represent those of their affiliated organizations, or those of the publisher, the editors and the reviewers. Any product that may be evaluated in this article, or claim that may be made by its manufacturer, is not guaranteed or endorsed by the publisher.
References
Abt K., Engler J. (2009). Rapid increase of the grey seal (Halichoerus grypus) breeding stock at Helgoland. Helgoland Mar. Res. 63, 177–180. doi: 10.1007/s10152-008-0143-6
Ahlgren H., Bro-Jørgensen M. H., Glyko A., Schmölcke U., Angerbjörn A., Olse M. T., et al. (2022). The Baltic grey seal: A 9000-year history of presence and absence. Holocene 32 (6), 569–577. doi: 10.1177/09596836221080764
Alava J. J. (2017). “Introduction to tropical and subtropical pinnipeds,” in Tropical Pinnipeds: Bio-Ecology, Threats and Conservation (Broken Sound Parkway Nw, suite: CRC Press and Taylor & Francis Group). doi: 10.1201/9781315151588-2
Baylis A. M. M., Þorbjörnsson J. G., Santos E., Granquist S. M. (2019). At- sea spatial usage of recently waned grey seal pups in Iceland. Polar Biol. 42 (11), 2165–2170. doi: 10.1007/s00300-019-02574-5
Bennett K. A., McConnell B. J., Moss S. E. W., Speakman J. R., Pomeroy P. P., Fedak M. A. (2010). Effects of age and body mass on development of diving capabilities of gray seal pups: costs and benefits of the postweaning fast. Physiol. Biochem. Zoology 83, (6). doi: 10.1086/656925
Bennett K. A., Speakman J. R., Moss S. E., Pomeroy P., Fedak M. A. (2007). Effects of mass and body composition on fasting fuel utilisation in grey seal pups (Halichoerus grypus Fabricius): an experimental study using supplementary feeding. J. Exp. Biol. 210, 3043–53. doi: 10.1242/jeb.009381
Bowen D. (2016). Halichoerus grypus (The IUCN Red List of Threatened Species: e.T9660A45226042). doi: 10.2305/IUCN.UK.20161.RLTS.T9660A45226042.en. (accessed August 08, 2024).
Bowen D. W., Iverson S. J., Mcmillan J. I., Boness D. J. (2006). Reproductive performance in grey seals: age-related improvement and senescence in a capital breeder. J. Anim. Ecol. 75, 1340–51. doi: 10.1111/j.1365-2656.2006.01157.x
Bubac C. M., Johnson A. C., Fox J. A., Cullingham C. I. (2019). Conservation translocations and post-release monitoring: Identifying trends in failures, biases, and challenges from around the world. Biol. Conserv. 238. doi: 10.1016/j.biocon.2019.108239
Burnham K. P., Anderson D. R. (2002). Model selection and multimodel inference: a practical information-theoretic approach. 2nd ed (New York: Springer-Verlag).
Campioni L., Dias M. P., Granadeiro J. P., Catry P. (2019). An ontogenetic perspective on migratory strategy of a long-lived pelagic seabird: Timings and destinations change progressively during maturation. J. Anim. Ecol. 89, (1). doi: 10.1111/1365-2656.13044
Carter M. I., Russel D. J. F., Embling C. B., Blight C. J., Thompson D., Hosegood P. J., et al. (2017). Intrinsic and extrinsic factors drive ontogeny of early-life at-sea behaviour in a marine top predator. Sci. Rep. 1. doi: 10.1038/s41598-017-15859-8
Carter M. I. D., McClintock B. T., Embling C. B., Bennett K. A., Thompson D., Russel D. J. F. (2020). From pup to predator: generalized hidden Markov models reveal rapid development of movement strategies in a naïve long-lived vertebrate. OIKOS 129, 630–642. doi: 10.1111/oik.06853
Cope H. R., McArthur C., Dickman C. R., Newsome T. N., Gray R., Herbert C. A. (2022). A systematic review of factors affecting wildlife survival during rehabilitation and release. PloS One 17, e0265514. doi: 10.1371/journal.pone.0265514
Costa D. P., Robinson P. W., Arnould J. P. Y., Harrison A.-L., Simmons S. E., Hassrick J. L., et al. (2010). Accuracy of ARGOS locations of pinnipeds at-sea estimated using fastloc GPS. PloS One 5. doi: 10.1371/journal.pone.0008677
Council of Europe (1979). Bern Convention on the Conservation of the European Wildlife and Natural Habitats, Appendix III, Protected Fauna species. Strasbourg, France: Council of Europe.
Delord K., Weimerskirch H., Barbraud C. (2024). The challenges of independence: ontogeny of at-sea behaviour in a long-lived seabird. Peer Community J. 4. doi: 10.24072/pcjournal.386/
Dragon A. C., Bar-Hen A., Monestiez P., Guinet C. (2012). Comparative analysis of methods for inferring successful foraging areas from Argos and GPS tracking data. Mar. Ecol. Prog. Ser. 452, 253–267. doi: 10.3354/meps09618
European Union (1992). The Council Directive 92/43/EEC on the conservation of natural habitats and of wild fauna and flora. Off. J. Eur. Communities 206, 0007–0050. Available at: https://eurlex.europa.eu/legalcontent/EN/TXT/HTML/?uri=CELEX:31992L0043&from=EN.
Field I. C., Harcourt R. G., Boehme L., Bruyn P. J. N., Charrassin J. B., McMahon C. R., et al. (2012). Refining instrument attachment on phocid seals. Mar. Mammal Sci. 28, (3). doi: 10.1111/j.1748-7692.2011.00519.x
Foo D., McMahon C. R., Hindel M. A., Fedak M., Biuw M., McConell B., et al. (2023). Swimming with the current improves juvenile survival in southern elephant seals. bioRxiv. doi: 10.1101/2023.11.05.565668
Frankish C. K., Manica A., Clay T. A., Wood A. G., Phillips R. A. (2022). Ontogeny of movement patterns and habitat selection in juvenile albatrosses. Oikos 2022 (6). doi: 10.1111/oik.09057
Galatius A., Teilmann J., Dahne M., Ahola M., Westphal L., Kyhn L. A., et al. (2020). Grey seal Halichoerus grypus recolonisation of the southern Baltic Sea, Danish Straits and Kattegat. Wildlife Biol. 2020, 1–10. doi: 10.2981/wlb.00711
Garces A. (2022). The importance of Wildlife Rehabilitation Center in the conservation of wild species. Int. J. Advanced Multidiscip. Res. Stud. 2, 1094–1099.
Hall A. J., Russel D. J. F. (2018). Gray seal Halichoerus grypus. Encyclopedia of marine mammals (London, United Kingdom: Academic Press), 420–422.
Hanson M., Hollingshead N., Schuler K., Siemer W. F., Martin P., Bunting E. M. (2021). Species, causes, and outcomes of wildlife rehabilitation in New York State. PloS One 16, e0257675. doi: 10.1371/journal.pone.0257675
Hastings K. K., Johnson D. S., Gelatt T. S. (2017). Flipper tag loss in Steller sea lions. Mar. Mammal Sci. 34 (1). doi: 10.1111/mms.12448
HELCOM (2013). Species information sheet, Halichoerus grypus (Helsinki, Finland: Red List Marine Mammal Expert Group).
HELCOM (2018). “Distribution of Baltic seals,” in HELCOM core indicator report. Available at: https://helcom.fi/wp-content/uploads/2019/08/Distribution-of-Baltic-seals-HELCOM-core-indicator-2018.pdf.
HELCOM (2023). “State of the baltic sea,” in Third HELCOM holistic assessment 2016-2021. Baltic Sea Environment Proceedings n°194. Helsinki, Finland: Baltic Marine Environment Protection Comission.
Heylen B. C., Nachstheim D. A. (2018). “Bio-telemetry as an essential tool in movement ecology and marine conservation,” in YOUMARES 8 – Oceans Across Boundaries: Learning from each other. Switzerland AG, Cham, Switzerland: Springer Nature. doi: 10.1007/978-3-319-93284-2_7
Horning M., Andrews R., Bishop A. M., Boveng P. L., Costa D. P., Crocker D. E., et al. (2019). Best practice recommendations for the use of external telemetry devices on pinnipeds. Anim. Biotelemetry 7, 20. doi: 10.1186/s40317-019-0182-6
Horning M., Haulena M., Rosenberg J. F., Nordstrom C. (2017). Intraperitoneal implantation of life-long telemetry transmitters in three rehabilitated harbor seal pups. BMC Veterinary Res. 13, (139). doi: 10.1186/s12917-017-1060-1
Horning M., Haulena M., Tuomi P. A., Melish J. E. (2008). Intraperitoneal implantation of life-long telemetry transmitters in otariids. BMC Veterinary Res. 4, (51). doi: 10.1186/1746-6148-4-51
Horning M., Mellish J. E. (2009). Spatially explicit detection of predation on individual pinnipeds from implanted post-mortem satellite data transmitters. Endangered Species Res. 10, (1). doi: 10.3354/esr00220
Hussey N. E., Kessel S. T., Aarestrup K., Cooke S., Cowley P. D., Fisk A. T., et al. (2015). Aquatic animal telemetry: A panoramic window into the underwater world. Science 348, (6240). doi: 10.1126/science.1255642
Illius A. W., O’Connor T. G. (2003). Resource heterogeneity and ungulate population dynamics. OIKOS 89, (2). doi: 10.1034/j.1600-0706.2000.890209.x
Jensen L. F., Ejbye-Ernst R., Michaelsen T. Y., Jensen A., Hansen D. M., Nielsen M. E., et al. (2017). Assessing the genetic effects of rehabilitating harbor seals (Phoca vitulina) in the Wadden Sea using stochastic simulations. Mammal Res. 62, 363–372. doi: 10.1007/s13364-017-0323-3
Jonsen I. D., Grecian W. J., Phillips L., Carroll G., McMahon C., Harcourt R. G., et al. (2023). AniMotum, an R package for animal movement data: Rapid quality control, behavioural estimation and simulation. Methods Ecol. Evol. 14, (3). doi: 10.1111/2041-210X.14060
Jonsen I. D., McMahon C. R., Patterson T. A., Auger-Méthé M., Harcourt R., Hindell M. A., et al. (2018). Movement responses to environment: Fast inference of variation among southern elephant seals with a mixed effects model. Ecology 100, e02566. doi: 10.1101/314690
Jüssi M., Härkönen T., Helle E., Jüssi I. (2008). Decreasing ice coverage will reduce the breeding success of baltic grey seal (Halichoerus grypus) females. A J. Hum. Environ. 37, 80–5. doi: 10.1579/0044-7447(2008)37[80:DICWRT]2.0.CO;2
Kaskela A. M., Kotilainen A. T. (2017). Seabed geodiversity in a glaciated shelf area, the Baltic Sea. Geomorphology 295, (1–2). doi: 10.1016/j.geomorph.2017.07.014
Kwok A. B. C., Haering R., Travers S. T., Stathis P. (2021). Trends in wildlife rehabilitation rescues and animal fate across a six-year period in New South Wales, Australia. PloS One 16, e0257209. doi: 10.1371/journal.pone.0257209
Lappe C., Umlauf L. (2016). Efficient boundary mixing due to near-inertial waves in a nontidal basin: Observations from the Baltic Sea. J. Geophysical Research: Oceans 121, (11). doi: 10.1002/2016JC011985
Lea M. A., Johnson D., Melin S., Ream R., Gelatt T. (2010). Diving ontogeny and lunar responses in a highly migratory mammal, the northern fur seal Callorhinus ursinus. Mar. Ecol. Prog. Ser. 419, 233–247. doi: 10.3354/meps08758
Lehmann A., Myrberg K., Post P., Chubarenko I., Dailidiene I., Hinrichsen H., et al. (2021). Salinity dynamics of the Baltic Sea. Earth System Dynamics 13, 373–392. doi: 10.5194/esd-13-373-2022
London J. M. (2020). pathroutr: An R Package for Re-Routing Paths Around Barriers (Version v0.1.1-beta) (Switzerland: Zenodo). doi: 10.5281/zenodo.4321827
Loseva A., Chirkova O., Shakhnazarova V. (2023). Haul-out patterns of the Baltic grey seal (Halichoerus grypus) in the suburbs of a metropolis. Regional Stud. Mar. Sci. 65, 103104. doi: 10.1016/j.rsma.2023.103104
Marton S. (2017). Generalised additive mixed models for dynamic analysis in linguistics: a practical introduction. White Rose Res. Monograph. The University of York.
McNamara J. M., Houston A. I. (2008). Optimal annual routines: behaviour in the context of physiology and ecology. Philos. Transactions: Biol. Sci. 363, 301–319. doi: 10.1098/rstb.2007.2141
Mellish J.-A. E., Iverson S. J., Bowen W. D. (1999). Variation in milk protuction and lactation performance in grey seals and consequences for pup growth and weaning characteristics. Physiol. Biochem. Zoology 72, 677–90. doi: 10.1086/316708
Moore M., Early G., Touhey K., Gulland F., Wells R. (2007). Rehabilitation and release of marine mammals in the United States: Risks and benefits. Mar. Mammal Sci. 23, 731–750. doi: 10.1111/j.1748-7692.2007.00146.x
Mullineaux E. (2014). Veterinary treatment and rehabilitation of indigenous wildlife. J. Small Anim. Pract. 55, 293–300. doi: 10.1111/jsap.12213
Noren S. R., Boness D. J., Iverson S. J., McMillan J., Bowen W. D. (2008). Body condition at weaning affects the duration of the postweaning fast in gray seal pups (Halichoerus grypus). Physiol. Biochem. Zoology 81, 269–77. doi: 10.1086/528777
Nowak B. V. R., Bowen W. D., Heyer C. E., Lang S. L. C., Lidgard D. C. (2023). Ontogeny of movement patterns in naïve grey seal pups inhabiting a complex continental shelf ecosystem. PloS One 18, e0290707. doi: 10.1371/journal.pone.0290707
Nowak B. V. R., Bowen W. D., Whoriskey K., Lidgard D. C., Mills F. J. E., Iverson S. J. (2020). Foraging behaviour of a continental shelf marine predator, the grey seal (Halichoerus grypus), is associated with in situ, subsurface oceanographic conditions. Movement Ecol. 8. doi: 10.1186/s40462-020-00225-7
O’Hara D. A. (2019). Does rehabilitation work? Investigating sex-and species-specific differences in daily weight gain of harbour and grey seal pups in rehabilitation. university of Bangor, Bangor.
Olli K., Nyman E., Tamminen T. (2022). Half-century trends in alpha and beta diversity of phytoplankton summer communities in the helsinki archipelago, the baltic sea. J. Plankton Res. 45, (1). doi: 10.1093/plankt/fbac029
Olsen M. T., Galatius A., Biard V., Gregersen K., Kinze C. C. (2016). The forgotten type specimen of the grey seal [Halichoerus grypus (Fabricius 1791)] from the island of Amager, Denmark. Zoological J. Linn. Soc. 178, (3). doi: 10.1111/zoj.12426
Omstedt A., Elken J., Lehmann A., Leppäranta M., Meier H. E. M., Myrberg K., et al. (2014). Progress in physical oceanography of the Baltic Sea during the 2003-2014 period. Prog. Oceanoraphy 128, (3). doi: 10.1016/j.pocean.2014.08.010
Orgeret F., Peron C., Enstipp M. R., Delord K., Weimerskirch H., Bost C. A. (2019). Exploration during early life: distribution, habitat and orientation preferences in juvenile king penguins. Movement Ecol. 7, (1). doi: 10.1186/s40462-019-0175-3
Patterson T. A., Thomas L., Wilcox C., Ovaskainen O., Matthiopoulos J. (2008). State-space models of individual animal movement. Trends Ecol. Evol. 23, (2). doi: 10.1016/j.tree.2007.10.009
Peschko V., Muller S., Schwemmer P., Mercker M., Lienau P., Rosenberger T., et al. (2020). Wide dispersal of recently weaned grey seal pupns in the Southerm North Sea. ICES J. Mar. Sci. 77, 1762–1771. doi: 10.1093/icesjms/fsaa045
Planque Y., Huon M., Caurant F., Pinaud D., Vincent C. (2020). Coparing the horizontal and vertical approaches used to identify foraging areas of two diving marine predators. Mar. Biol. 167, (25). doi: 10.1007/s00227-019-3636-8
Rana V., Galczynska M., Milke J. (2021). “Inorganic and organic pollutants in the baltic sea region and feasible circular economy perspectives for waste management,” in Handbook of Soldi Waste Management (Springer, Singapore). doi: 10.1007/978-981-15-7525-9_80-1
Rašomavičius V. (Ed.) (2021). Red Data Book of Lithuania. Animals, plants, fungi. – Vilnius. Available online at: http://www.raudonojiknyga.lt/. Accessed [August 19, 2024].
Riotte-Lambert L., Weimerskirch H. (2013). Do naive juvenile seabirds forage differently from adults? Proceeding R. Soc. B 280, (1768). doi: 10.1098/rspb.2013.1434
Robinson D. P., Hyland K., Beukes G., Vettan A., Mabadikate A., Jabado R. W. (2021). Satellite tracking of rehabilitated sea turtles suggests a high rate of short-term survival following release. PloS One 16, e0246241. doi: 10.1371/journal.pone.024624
Ronkon J., Bestley S., McMahon C. R., Wienecke B. (2018). View from below: Inferring behavior and physiology of Southern ocean marine predators from dive telemetry. Front. Mar. Sci. 5. doi: 10.3389/fmars.2018.00464
Rosentau A., Bennike O., Uscinowicz S., Miotk-Szpiganowicz G. (2017). “The baltic sea basin,” in Submerged Landscapes of the European Continental Shelf: Quaternary Paleoenvironments (New Jersey, U.S.: John Wiley & Sons). doi: 10.1002/9781118927823.ch5
Russell D. J. F., McClintock B. T., Matthiopoulos J., Thompson P. M., Thompson D., Hammond P. S., et al. (2015). Intrinsic and extrinsic drivers of activity budgets in sympatric grey and harbour seals. Oikos 124, 1462–1472. doi: 10.3354/cr01244
Rutgersson A., Jaagus J., Schenk F., Stendel M. (2014). Observed changes and variability of atmospheric parameters in the Baltic Sea region during the last 200 years. Climate Res. 61 (2), 177–190. doi: 10.3354/cr01244
Sangster S., Haulena M., Nordstrom C., Gaydos J. K. (2020). Interannual differences in postrelease movements of rehabilitated harbor seal pups (Phoca vitulina richardii) in the Salish Sea. Mar. Mammal Sci. 37, 64–79. doi: 10.1111/mms.12739
Sayer S., Allen R., Bellman K., Beaulieu M., Cooper T., Dyer N., et al. (2021). Post release monitoring of rehabilitated gray seal pups over large temporal and spatial scales. Mar. Mammal Sci. 38, 539–556. doi: 10.1111/mms.12885
Sequeira A. M. M., Heupel M. R., Lea M. A., Equilu V. M., Duarte C. M., Meekan M. G., et al. (2019). The importtance of sample size in marine megafauna tagging studies. Ecol. Appl. 29, 1344–1360. doi: 10.1002/eap.1947
Simeone C. A., Rousselet E., Atkin C., Trez M. D., Delemotte M., Johnson S. P. (2024). A systematic review of global marine mammal rehabilitation and refloating 2000–2023. Sustainability 16, (11). doi: 10.3390/su16114589
Sumner M. D., Wotherspoon S. J., Hindell M. A. (2009). Bayesian estimation of animal movement from archival and satellite tags. PloS One 4. doi: 10.1371/journal.pone.0007324
Suuronen P., Lunneryd S.-G., Königson S., Coelho N. F., Waldo Å., Eriksson V., et al. (2023). Reassessing the management criteria of growing seal populations: The case of Baltic grey seal and coastal fishery. Mar. Policy 155, (43). doi: 10.1016/j.marpol.2023.105684
Symonds M. R. E., Moussalli A. (2010). A brief guide to model selection, multimodel inference and model averaging in behavioural ecology using Akaike’s information criterion. Behav. Ecol. Sociobiology 65, (1). doi: 10.1007/s00265-010-1037-6
Szymczycha B., Pempkowiak J. (2016). “Characteristics of the baltic sea,” in The Role of Submarine Groundwater Discharge as Material Source to the Baltic Sea (Copyright Springer International Publishing, Switzerland), ISBN: 978-3-319-79865-3. doi: 10.1007/978-3-319-25960-4
Testa J. W., Rothery P. (1992). Effectiveness of various cattle ear tags as markers for weddell seals. Mar. Mammal Sci. 8, (4). doi: 10.1111/j.1748-7692.1992.tb00050.x
Tinker M. T., Bentall G., Estes J. A. (2008). Food limitation leads to behavioral diversification and dietary specialization in sea otters. Pnas 105 (2), 560–565. doi: 10.1073/pnas.0709263105
van Beest F. M., Mews S., Elkenkamp S., Schuhmann P., Tsolak D., Wobbe T., et al. (2019). Classifying grey seal behaviour in relation to environmental variability and commercial fishing activity - a multivariate hidden Markov model. Sci. Rep. 9, (1). doi: 10.1038/s41598-019-42109-w
Vance H. M., Hooker S. K., Mikkelsen L., van Neer A., Teilmann J., Siebert U., et al. (2021). Drivers and constraints on offshore foraging in harbour seals. Sci. Rep. 11, 6514. doi: 10.1038/s41598-021-85376-2
van Neer A., Gross A., Arriba-Garcia A., Rubio-Garcia A., Salazar-Casals A., Siebert U. (2020). An innovative approach for combining marking of phocid seals with biopsy sampling using a new type of livestock ear tags. J. Mar. Anim. their Ecol. 12, (1).
Vincent C., Ridoux V., Fedak M. A., Hassani S. (2002). Mark-recapture and satellite tracking of rehabilitated juvenile grey seals (Halichoerus grypus): dispersal and potential effects on wild populations. Aquat. Mammals 28, (2).
Vincent C., Ridoux V., Fedak M. A., McConnel B. J., Sparling C. E., Leaute J-P., et al. (2016). Foraging behaviour and prey consumption by grey seals (Halichoerus grypus) – spatial and trophic overlaps with fisheries in a marine protected area. ICES J. Mar. Sci. 73 (10). doi: 10.1093/icesjms/fsw102
Votier S. C., Fayet A. L., Bearhop S., Bodey T. W., Clark B. L., Grecian J., et al. (2017). Effects of age and reproductive status on individual foraging site fidelity in a long-lived marine predator. Proc. R. Soc. 284, 20171068. doi: 10.1098/rspb.2017.1068
Wimberger K., Downs C. S., Boyes R. S. (2010). A survey of wildlife rehabilitation in South Africa: is there a need for improved management? Anim. welfare 19, 481–499. doi: 10.1017/S0962728600001962
Keywords: Argos satellite tracking, marine top predator, wildlife rehabilitation, post-release monitoring, pinnipeds
Citation: Kuncienė L, Nachtsheim D, Siebert U and Kleiva Ž (2024) Ontogeny of horizontal movement patterns of rehabilitated grey seal juveniles (Halichoerus grypus) in the Baltic Sea. Front. Mar. Sci. 11:1449023. doi: 10.3389/fmars.2024.1449023
Received: 14 June 2024; Accepted: 08 August 2024;
Published: 02 September 2024.
Edited by:
Mark Jessopp, University College Cork, IrelandReviewed by:
Matt I. D. Carter, University of St Andrews, United KingdomCecile Vincent, Université de la Rochelle, France
Copyright © 2024 Kuncienė, Nachtsheim, Siebert and Kleiva. This is an open-access article distributed under the terms of the Creative Commons Attribution License (CC BY). The use, distribution or reproduction in other forums is permitted, provided the original author(s) and the copyright owner(s) are credited and that the original publication in this journal is cited, in accordance with accepted academic practice. No use, distribution or reproduction is permitted which does not comply with these terms.
*Correspondence: Laura Kuncienė, bC5sdXBlaWthaXRlQG11emllanVzLmx0