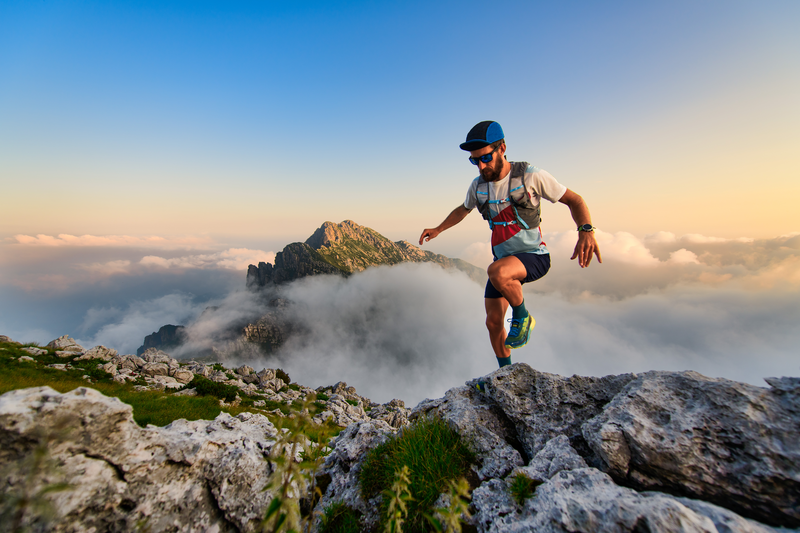
95% of researchers rate our articles as excellent or good
Learn more about the work of our research integrity team to safeguard the quality of each article we publish.
Find out more
ORIGINAL RESEARCH article
Front. Mar. Sci. , 09 August 2024
Sec. Aquatic Physiology
Volume 11 - 2024 | https://doi.org/10.3389/fmars.2024.1443710
This article is part of the Research Topic Endocrine Regulation and Physiological Adaptation of Stress Response in Aquatic Organisms, Volume II View all 13 articles
In this study, we explored the effects of chronic stress on lumpfish (Cyclopterus lumpus) physiological, immune response, health, and plasma free amino acids. 3 groups of lumpfish were exposed to 1-minute air exposure. 1 group was exposed to stress once per week, a second group exposed 2 times per week, and a third group exposed 4 times per week. The present study revealed significant alterations in immunity and increased nutritional demands, particularly the branched chain amino acids and lysine. Cortisol levels fluctuated, with significantly higher levels halfway through the experiment on the groups that were stressed more often. Though, by the end of the experiment, there were no significant differences in cortisol levels between groups. Regardless of stress exposure, cataract developed in virtually all sampled fish, pointing toward a potential dietary imbalance. A transient immunomodulation of stress was visible. While in early stages stress had an immune enhancing effect, as seen by the increase in plasma nitric oxide and peroxidase in the group most frequently exposed to stress, these differences were not apparent by the end of the experiment. Additionally, the worst health condition was found in this group. Our results underscore the complex interplay between stress, immunity and nutrition, highlighting the need for tailored dietary strategies and improved rearing practices.
Lumpfish, naturally occurring along the Norwegian shores, have been documented grazing on sea lice1. For this particular trait, lumpfish have garnered attention from the salmon farming industry (Imsland et al., 2018). The deployment of these fish in salmon farms has surged in response to escalating ectoparasitic infestations impacting salmonid aquaculture (Fiskeridirektoratet, 2021; Imsland et al., 2021). While the effectiveness of this species as delousing agents remains a subject of debate, one aspect is indisputable: the welfare of deployed lumpfish is concerning (Stien et al., 2020).
Beyond the economic benefits, the welfare of aquaculture animals has important ethical considerations. Our society is based on principles of justice and solidarity, and it is our responsibility to uphold these values not only in relation to humanity but also in regard to our environment. This responsibility extends to the animals and the finite natural resources our civilization relies upon (Charter of Fundamental Rights of the European Union, 2012; The European Green Deal, 2019). Consequently, sustainability and welfare have emerged as vital considerations, sparking extensive research and dialogue (Bovenkerk and Meijboom, 2013; Brown, 2015). As we delve in this paper on the physiological and immunological facets of lumpfish, it is imperative to underscore the ethical dimension of animal welfare.
Indeed, while production of lumpfish has surged during the past decade, the welfare standards did not follow suit (Garcia de Leaniz et al., 2022; Reynolds et al., 2022). This has culminated in disconcerting reports from the Norwegian Food Safety Authority regarding elevated mortality rates, disease outbreaks and unknown fate of a large portion of deployed lumpfish (Mattilsynet, 2020; Stien et al., 2020). It is crucial to deepen our understanding on the species physiology and requirements, as a cornerstone for improvement of welfare standards.
Stress is an inherent aspect of a teleost’s life in aquaculture. Common stressors encompass netting and transferring, transport, daily operations involving handling, sorting, crowding, disease outbreaks, social stress and aggression, among others, all of which can elicit a stress response (Sneddon et al., 2016; Afonso, 2020). Briefly, the nervous system regulates the immediate response, by altering hormonal activity (Reid et al., 1998). Endocrine alterations help prepare the organism for immunological challenges like infection and wound repair, essential for survival. This neural-endocrine-immune conversation involves two main stress axes, the brain-sympatho-chromaffin axis (BSC) and the hypothalamus-pituitary-interrenal axis (HPI) (Wendelaar Bonga, 1997; Tort, 2011). These axes play pivotal roles in the teleost stress response, which can be manifest along three stages. The BSC axis is instantly activated upon stress perception, resulting in the release of catecholamine hormones such as epinephrine and norepinephrine. The HPI axis, on the other hand, exhibits a slight latency, being prompted minutes after, resulting in the release of cortisol by the interrenal cells in the head-kidney. Both catecholamines and cortisol release orchestrate the fallout of the stress response, mediating energy mobilization and modulating immunity. The metabolic changes following the action of the stress hormones are part of the second stage in the stress response. The third stage ensues when the organism is unable to successfully cope and overcome stress, leaving lasting maladaptations such as alterations in behavior and immune suppression (Barton and Iwama, 1991; Wendelaar Bonga, 1997; Schreck and Tort, 2016).
The intertwining of teleost stress and immune responses is well documented (Tort, 2011; Urbinati et al., 2020). This link emerges from a crosstalk between the neuroendocrine and immune systems, being expressed through a network of nerves, hormones, cytokines, and neuropeptides (Tort et al., 2003; Verburg-Van Kemenade et al., 2009; Yada and Tort, 2016). Most immune cells express receptors for neuropeptides, neurotransmitters, and hormones that, upon binding, alter transcription and cell activity. On an initial stage, perception of stress can induce the release of pro-inflammatory cytokines, namely interleukin-1β, interleukin-6, interleukin-12, tumor necrosis factor-α (Mosser and Edwards, 2008; Tort, 2011; Verburg-van Kemenade et al., 2011; Khansari et al., 2017). Mounting evidence supports the role of pro-inflammatory cytokines as early molecular initiators of the host inflammatory response through interaction with peripheral nerve terminals (Molfino et al., 2009). These findings highlight the intricate interplay between neuronal, endocrine, and immune systems.
Although most stressors cannot be eliminated, they can however, be mitigated. Increasing our understanding on the lumpfish stress physiology and its interconnectedness with health, nutrient requirements and the immune response opens the door for various stress-mitigating strategies (Ashley, 2007; Andersen et al., 2016; Sneddon et al., 2016; Martos-Sitcha et al., 2020). In fact, stress mitigating dietary strategies have been a major area of research in aquaculture (Kaushik and Seiliez, 2010; Herrera et al., 2019; Ciji and Akhtar, 2021). While improving aquaculture standards and welfare measures is key, nutrition is arguably one of the most important aspects for ensuring good health. Missing nutrient needs leads to disorders, and in many cases, a total disruption of fish health. Amino acids (AAs), for instance, have received particular attention (Costas et al., 2011a; Azeredo et al., 2017). In addition to being the building blocks of proteins, some AAs play functional roles in several physiological processes including growth, immune functions and energy metabolism. Some are also necessary for the synthesis of non-protein molecules like neurotransmitters and hormones (Li et al., 2009; Andersen et al., 2016; Ahmad et al., 2021). Certain AAs are only available through diet, deemed essential amino acids (EAA), as the organism cannot produce them, and as such their requirements must be known (Li et al., 2009). Stress modulates the need for certain nutrients, including AAs (Aragão et al., 2008) which means that if a diet does not take this into consideration, there is a risk of falling short on the nutritional demands, leading to potential disorders (Li et al., 2007; Yoneda et al., 2009; Ramos-Pinto, 2020). A practical and accurate way of assessing the potential increased needs in certain AAs levels in a diet for a particular fish, is by analyzing the free AAs in plasma (Aragão et al., 2008; Costas et al., 2011a). In this study, we research the nexus between chronic stress, health and immune functions with a focus on the effects in plasma free AAs.
Artic Seafood Group (Mørkvedbukta, Bodø, Norway) supplied 408 lumpfish juveniles with a mean weight of 58.5 ± 1.9 g which were transferred to Mørkvedbukta research station (Nord University, Mørkvedbukta, Norway). Upon arrival, fish health was assessed for all individuals, following a well described system tailored for visual health inspection of lumpfish (Reynolds et al., 2022). Lumpfish remained undisturbed for three weeks in indoor 1000 L flowthrough seawater tanks at an initial stocking density of 2.84 g L-1. During this acclimation period and throughout the study, fish were fed 2% body weight with a commercial diet (Skretting’s Clean Assist, 1.8 mm pellet size, Skretting, Stavanger, Norway) using feeding automats. Six meals were given throughout the day, following a 12h light-dark photoperiod. The daily routine consisted of checking feed levels in the automats, water temperature (7.6 ± 0.3°C), salinity (34.3 ± 0.3 ‰) and dissolved oxygen (7.8 ± 0.2 mg L-1). In addition, fish behavior, such as feeding and hovering, was observed daily in each tank.
Four triplicate groups of lumpfish with an initial mean (± SD) weight of 89.8 ± 4.5 g (n = 34; N = 408) were established from the original population (groups 0C, 1S, 2S and 4S). Group numbers represent the number of times the groups were subject to stress per week, with letter “C” indicating control group and “S” stress group. This means that group 4S was exposed 4 times per week to the selected stressor, while group 0C was the control group, remaining undisturbed until sampling. The groups were distributed randomly across tanks. The stress exposure was scheduled randomly, occurring at different days of the week, at different times throughout the day, to avoid predictability. Disturbance of the fish was kept at absolute minimum, validated by the cortisol levels in the control group throughout the experiment. Netting the fish was followed with a chronometer to ensure consistency between tanks and was completed under 30 seconds. The air exposure was also timed with a chronometer and lasted exactly 1 minute. Air exposure was chosen as a stressor for its practical implication in aquaculture and it has been validated as stressor for lumpfish according to the procedures described by Lopes et al. (2023). This chronic stress experiment lasted for 10 weeks. On the day preceding sampling, lumpfish were fasted to minimize effects on biomarkers prompted by feeding (Arends et al., 1999). Sampling occurred always 1 h after stress exposure. Samplings were conducted on the 2nd, 6th and 10th week and the health assessments were recorded in each sampling point.
Lumpfish health was evaluated every two weeks. Sampled fish were visually inspected following the Lumpfish Health Scoring System guidelines (LHSS) (Reynolds et al., 2022) and the health score registered. Cataracts were scored for size and opacity using a portable slit lamp (Heine, Gilching, Germany). Cataract incidence was calculated as . Length and weight were measured and the specific growth rate (SGR) calculated37.
For sampling, 3 individuals from each tank were swiftly removed and euthanized with 1600 mg L-1 metacaine (tricaine methanesulphonate, Sigma Aldrich Co, St. Louis, Missouri, USA) (Skår et al., 2017).
Blood was drawn from the caudal vein in less than 3 min onto a heparinized vacutainer (BD, Plymouth, UK), and centrifuged at 2000 × g for 5 min at 4 °C. The resulting plasma was transferred to 1.8 ml cryotubes, snap-frozen in liquid nitrogen and stored at −80°C.
Liver biopsies (approximately 1 cm3) were promptly taken consistently from the same area of the liver, the inferior part of the right lobe, using a scalpel, with each sample being divided into two aliquots and snap-frozen in liquid nitrogen. Biopsies were stored into two separate 1.8 ml cryotubes designated for oxidative stress and metabolite assays. For preparation of liver samples for metabolite analyses, frozen liver was finely minced in a 50 ml Falcon tube. A high-performance dispersing instrument (SilentCrusher M, Heidolph Instruments, Schwabach, Germany) was employed for mechanical disruption, in 7.5 vol. ice-cold 6% (w/v) perchloric acid. The homogenate was neutralized with an equal volume of 1 M KHCO3, then centrifuged at 13,000 × g for 30 min at 4°C. Samples for oxidative stress analysis were prepared in a similar manner but using a different buffer: 1:10 volume K phosphate buffer (KPB) (0.1 M K2HPO4, 0.1 M KH2PO4, pH 7.4, Sigma Aldrich Co). To measure lipid peroxidation (LPO), 200 µL homogenate aliquots were retrieved. To each lipid peroxidation aliquot, 4 µL of 4% 3,5-di-tert-4-butylhydroxytoluene (BHT, in methanol, Sigma Aldrich Co.) were added before centrifugation to inhibit lipid peroxidation. The remaining homogenates were then centrifuged at 10,000 × g for 20 min at 4°C and the supernatants stored in separate aliquots at −80°C until use for oxidative stress assays.
Plasma cortisol levels were quantified utilizing a commercial ELISA kit (IBL International GMBH, Hamburg-Nord, Germany) that had been previously validated and adapted for lumpfish (da Santa Lopes et al., 2023). The procedure involved the extraction of cortisol in plasma using diethyl ether at a 1:20 proportion. Following extraction, phosphate buffer infused with 1 g L-1 gelatine (pH 7.6) was added in a 2:1 proportion, thoroughly mixed and added to the microtiter plates, where kit recommendations ensued. For the quantification of plasma glucose, lactate and triglycerides, alongside hepatic lactate and triglycerides we used Spinreact kits (Spinreact) adapted to 96-well micro-plates (Costas et al., 2011a). Following the protocol outlined by Costas et al (Costas et al., 2014), plasma total proteins were assessed in 1:50 (v/v) diluted samples using Pierce™ BCA Protein Assay Kit (Thermo Fisher Scientific; USA). The resulting microplates of each parameter were read with the use of Synergy HT Microplate Reader (BioTek Instruments, Winooski, VT USA).
Lysozyme was measured using a turbidimetric test following the adaptation by Costas et al. (2011a) (Costas et al., 2011a) from the assay described by Ellis (1990) (Ellis, 1990a). To determine the amount of lysozyme present in the samples, a standard curve was established, where lyophilized hen egg white lysozyme (Sigma Aldrich Co.) was successively diluted in sodium phosphate buffer (0.05 M, pH 6.2).
Total peroxidase activity was quantified following Quade and Roth’s colorimetric procedure (Quade and Roth, 1997), where one unit of peroxidase activity is defined by the quantity of peroxidase required to produce an absorbance change of 1 OD.
The protease levels in plasma were measured according to the methods described by Ellis (Ellis, 1990b) and Machado et al (MaChado et al., 2020). The principle behind the method is to evaluate the degradation of azocasein when incubated with plasma, using a trypsin solution (5 mg ml–1 in 0.5% NaHCO3, pH 8.3) instead of plasma as positive control.
Plasma bactericidal activity was investigated by determining the overall capacity of plasma in eliminating selected bacteria, following Machado et al.’s adaptations (MaChado et al., 2015) of the protocol described by Graham et al. (Graham and Secombes, 1988) which has been standardized and validated for several fish species (Azeredo et al., 2019; Reis et al., 2021; Magalhães et al., 2023). For this assay, 20 μL of each plasma sample were added to U-shaped 96-well plate. Then, 20 μL Vibrio anguillarum (1 x 106 cfu ml-1) were added in each well, and left incubating at 25°C for 2.5 h. Subsequently, 25 μL of 3- (4, 5-dimethyl-2-yl)-2,5-diphenyl tetrazolium bromide (1 mg mL−1; Sigma Aldrich Co) was added and incubated for an additional 10 min at the same temperature for formazan formation. After centrifugation at 2000 × g for 10 min, the precipitate was dissolved in 200 μL of dimethyl sulfoxide (Sigma Aldrich Co.). Absorbance of the dissolved formazan was measured at 560 nm. Bactericidal activity was expressed as a percentage, determined from the difference in surviving bacteria relative to the positive control (100%).
Total plasma nitrite and nitrate were assessed as proxy for nitric oxide (NO) production using a colorimetric kit (Roche Diagnostics GmbH, Mannheim, Germany), as these compounds are a product of NO degradation. A sodium nitrite standard curve was developed to allow calculation of total nitrite in the samples.
Hepatic enzymatic activity was studied to understand the extent of oxidative damage in liver. Homogenized liver samples were used to assess lipid peroxidation (LPO), superoxide dismutase (SOD) and catalase (CAT) activities in addition to total protein concentration. The methodology delineated by Claiborne (1985) (Claiborne, 1985) and adapted by Peixoto et al. (Peixoto et al., 2021) was used to determine CAT. This was done by measuring the decrement in H2O2 concentration. The observed data is translated into enzyme activity expressed as enzyme units per milligram of total protein (U mg−1 protein). To determine SOD, we used the protocol illustrated by Lima et al. (Lima et al., 2007) and modified by Almeida et al (Almeida et al., 2010). Enzymatic activity is calculated based on the amount of enzyme necessary to inhibit 50% of cytochrome c reduction rate, instigated by the presence of superoxide radicals. Lipid peroxidation was measured using thiobarbituric acid-reactive substances (TBARSs) (Bird and Draper, 1984; Aloísio Torres et al., 2002). The total protein concentration of liver samples was determined following the protocol adapted by Costas et al. (Costas et al., 2014) employing the Pierce™ BCA Protein Assay Kit (Thermo Fisher Scientific, USA).
The free amino acid profile from plasma samples was determined by ultra-high-performance liquid chromatography (UPLC) on a Waters Reversed-Phase Amino Acid Analysis System, using norvaline as an internal standard. All samples were deproteinized by centrifugal ultrafiltration (10 kDa cut-off, 2500 × g, 20 min, 4°C). After deproteinization, samples were pre-column derivatised with Waters AccQ Fluor Reagent (6-aminoquinolyl-N-hydroxysuccinimidyl carbamate) using the AccQ Tag method (Waters, USA). Amino acids were identified by retention times of standard mixtures and pure standards (Sigma-Aldrich). Instrument control, data acquisition and processing were achieved using Waters Empower software.
The ensuing results are expressed as mean ± standard error. All data were subject to statistical analysis by testing for homogeneity and normality of variances using Levene’s and Kolmogrov-Smirnov tests, respectively. To address skewed data on plasma cortisol, plasma and liver lactate and liver triglycerides, the data of these parameters was transformed logarithmically. Identification and comparison of significant differences on all parameters within each timepoint was performed by one-way ANOVA (p value< 0.05), followed by Tukey HSD post hoc test (Zar, 1984). Statistical analyses were carried out using IBM SPSS v25.0 0 (IBM Corp., Armonk, New York, USA).
Analysis of cortisol in lumpfish plasma revealed significant differences at 2 and 6 weeks of the chronic stress experiment (Figure 1). At two weeks, mean cortisol levels were highest on group 1s (17.12 ± 4.62 ng ml-1), being significantly higher than control group 0c (7.73 ± 1.50 ng ml-1). Cortisol levels at 6 weeks were significantly increased for groups 2s (28.90 ± 9.59 ng ml-1) and 4s (27.07 ± 6.53 ng ml-1), when compared to the control group 0c (12.22 ± 2.16 ng ml-1). By the end of the experiment, no significant differences were observed between groups.
Figure 1. Plot of cortisol levels (ng ml -1) in plasma (expressed as average ± SE) in undisturbed lumpfish (0c) and in lumpfish exposed to air for 1 minute (1s; 2s and 4s) on 2nd, 6th and 10th week samplings. Letters represent significant differences between groups within the same timepoint (ANOVA, Tukey, p< 0.05).
Glucose analysis revealed significant differences at week 2, where group 2s had higher glucose levels than control group and group 1s (Supplementary Figure 1). No significant differences were observed in the following sampling points.
Plasma levels of lactate were not altered by the exposure to air (Supplementary Figure 2), with no significant differences found in any timepoint.
Significant differences were found in triglyceride levels in both plasma and liver samples (Figure 2). In the 6th week sampling, groups 2s (2.70 ± 0.21 mM) and 4s (2.68 ± 0.14 mM) had lower triglyceride levels than control group 0c (3.35 ± 0.13 mM). In addition, liver samples from groups 2s (4.42 ± 0.81 mM) and 4s (3.39 ± 0.20 mM) at the 10th week sampling, had also the lowest levels, with a significant decrease when compared with control group 0c (6.84 ± 0.61 mM).
Figure 2. Plot of triglyceride levels (mM) in plasma (A) and liver (B) expressed as average ± SE in undisturbed lumpfish (0c) and in lumpfish exposed to air for 1 minute (1s; 2s and 4s) on 2nd, 6th and 10th week samplings. Letters represent significant differences for the same tissue between different groups within the same timepoint (ANOVA, Tukey, p< 0.05).
Immune parameters revealed significant differences between groups (Figure 3). Peroxidase activity was highest in group 1s at 6th week sampling point (9.4 ± 1.0 units ml-1), being significantly higher than peroxidase levels found in control group (4.5 ± 0.8 units ml-1).
Figure 3. Plots of immune parameters: peroxidase (A); protease activity (B); lysozyme (C); bactericidal activity (D) and nitric oxide (E) in plasma. Expressed as average ± SE in undisturbed lumpfish (0c) and in lumpfish exposed to air for 1 minute (1s; 2s and 4s) on weeks 2, 6 and 10. Letters represent significant differences between different groups within the same timepoint (ANOVA, Tukey, p< 0.05).
Significant differences were also found in protease activity both in 2nd and 10th week. On 2nd week, group 0c (2.0 ± 0.1%) and 1s (2.0 ± 0.1%) had lower protease activity when compared to groups 2s (2.4 ± 0.1%) and 4s (2.3 ± 0.1%). By week 10, group 1s had significantly higher protease activity (2.3 ± 0.1%) than group 2s (1.9 ± 0.1%).
Lysozyme levels were significantly lower in group 2s (36.8 ± 2.6 µg ml-1) than in control group 0c (48.0 ± 3.0 µg ml-1) at the 2nd week sampling, with no more significant differences found thereafter.
Bactericidal activity was highest in groups 2s (43.5 ± 2.7%) and 4s (45.7 ± 2.5%) when compared to group 0c (26.1 ± 4.1%) and 1s (21.5 ± 3.5%) during week 6.
All the other groups had significantly lower levels of nitric oxide than control group (0.12 ± 0.01 µM) in week 2. However, in week 6, group 2s (0.08 ± 0.004 µM) had highest levels of nitric oxide, being significantly higher than control group (0.03 ± 0.01 µM).
Plasma free AA analyses revealed significant differences on week 6, in all 3 branched-chain amino acids (BCAAs: isoleucine, leucine and valine), and lysine (Figure 4). Group 4s had significantly lower levels of the 4 AAs when compared to control group. Isoleucine and valine levels were also statistically lower in group 2s than group 0c. No differences were found between group 1s and control group in this timepoint. There were no significant differences in the remaining AA (Supplementary Table 1) between groups neither in the 6th nor at the 10th week.
Figure 4. Plasma free amino acid levels of 4 essential AAs (Lysine; Isoleucine; Leucine and Valine) expressed as average ± SE in undisturbed lumpfish (0c) and in lumpfish exposed to air for 1 minute (1s; 2s and 4s) on sampling at week 6. Letters represent significant differences between different groups within the same timepoint (ANOVA, Tukey, p< 0.05).
No mortalities occurred during the whole trial period. Lumpfish started with an overall mean weight of 89.8 ± 0.6 g. There were no significant differences in growth between any group, however by end of the study, the control group “0c” had the highest group average weight (226.1 ± 13.8 g), with the highest specific growth rate (SGR) of 1.3%, followed by 1s with a final mean weight of 211.0 ± 11.6 (SGR 1.2%). Group 2s had a final mean weight of 197.8 ± 10.8 g by the end of the experiment (SGR 1.1%), with group 4s having the lowest mean weight at the end sampling, with 184.5 ± 8.2 g (SGR 1.1%). The health score was measured following the LHSS, revealing an increase in health deterioration in all groups, with 100% of fish in group 4s entering concerning area (health score > 2) by week 6 (Figure 5). Cataract incidence was below 25% for all groups on the day of transfer, however, it quickly rose up to virtually 100% by week 6 (Figure 6). At the last sampling point, cataract incidence was 100% for all stressed groups, and 92.0 ± 8.3% for the control group. There were no significant differences both in health score and cataract incidence between the different groups.
Figure 5. Percentage of incidence of group health scores throughout the experiment for undisturbed lumpfish (0c) and lumpfish groups exposed to stress (1s; 2s and 4s). “s” means score; [s< 1] is the category of scores below; [1< s ≤2] is for scores above 1 and below or equal to 2; and [2< s ≤3] is for the scores above 2 and below or equal to 3. Chi square analysis revealed no significant differences. The results are presented as a percentage.
Figure 6. Plot of cataract incidence on undisturbed lumpfish (0c) and lumpfish exposed to stress (1s; 2s and 4s). Cataract incidence expressed as %.
The negative impacts of chronic stress on teleost health are undeniable, having been extensively demonstrated (Pickering and Pottinger, 1989; Barton and Iwama, 1991; Mommsen et al., 1999). In aquaculture rearing, fish frequently encounter prolonged or repeated stress, typically leading to immune suppression (Fast et al., 2008). The immunosuppressive effects of stress lead to impaired disease resistance, opening the door for opportunistic pathogens, and other health complications (Saeij et al., 2003). Lumpfish are no exception, even though lacking the evident reactive fight-or-flight response characteristic of many teleosts such as salmon (Hale, 2000). Several studies have confirmed the stress response of lumpfish to a variety of stress exposures, such as crowding, temperature, behavioral cues, and air exposure (Jørgensen et al., 2017; da Santa Lopes et al., 2023; Espmark et al., 2019; Noble et al., 2019; Staven et al., 2019; Remen et al., 2022). However, there is a lack of research connecting the lumpfish chronic stress exposure to immunity, overall health, and specific nutritional requirements. In this study, cortisol served as reliable indicator of HPI activation, with cortisol levels in the control group (0c) being kept under 15 ng ml-1 throughout the study. In comparison, the highest cortisol levels were found in groups stressed twice a week (2s) and 4 times per week (4s), in week 6. This coincided with a higher bactericidal activity also found at this time point. In addition, the proxy analysis of NO revealed its highest levels in all stressed groups, being significantly higher than control, in group 2s. Moreover, peroxidase activity also trended higher in stressed groups, with lowest levels on the undisturbed fish. In fact, peroxidase activity was statistically higher in the group stressed only once a week. The immune analysis of samples taken at week 6 hints at a potential immune-enhancing effect on stressed groups, as seen by the increased NO, bactericidal and peroxidase activities. This is not unheard of, with several studies depicting this occasionally paradoxical effect of stress on immunity (Tort, 2011). Acute stress can boost immunity by preparing the organism for immediate challenges. Cortisol released during a short-term stress adjusts leukocyte activity and cytokine levels, resulting in increased immunoprotection (Castillo et al., 2009; Yada and Tort, 2016; Khansari et al., 2017). Increased immunity can be beneficial, with an animal being more prepared to deal with immune challenge, but it can also be physiologically demanding. Under immune challenge, energy and resource allocations are diverted from certain physiological processes such as reproduction, and growth (Sadoul and Vijayan, 2016; Schreck and Tort, 2016; Yada and Tort, 2016). However, without a significant challenge, the energy budget might be sufficient to accommodate the increased energy demands of an immune enhancement caused by a mild stress. Accordingly, we have not seen any significant differences in weight between the different stress groups. Moreover, innate immune cell populations such as neutrophil and activated macrophages are mobilized, increasing in immune activation sites in response to acute stress, even without an initial immune challenge (Wojtaszek et al., 2002; Tort, 2011). Neutrophils are prime effectors of peroxidase activity, while macrophages are key NO releasers, and their increased numbers prepare the organism for a potential challenge, effectively enhancing immunoprotection. Despite not looking directly to leukocyte population dynamics in this study, we did find the highest levels in peroxidase activity and nitric oxide in stressed groups. Nonetheless, prolonged stressed exposure can bring the immune enhancing effects to a halt, leading to subsequent immunosuppression (Mommsen et al., 1999; Dhabhar, 2008; Yada and Tort, 2016). Indeed, in our findings, the immune enhancing effects faded, with immune analysis at the end sampling showing no significant differences between undisturbed and stressed fish in any parameter. Stress shifts how fish use their energy and resources, prioritizing immediate survival over, reproduction, growth, and even immune functions. In fact, in our study, despite not being statistically significant, we saw a trend for lower growth in the more frequently stressed group 4s, while scoring the worst health, which was mostly explained by worsened skin erosion. Fin erosion is one of the first conditions seen in chronically stressed fish, where skin barrier defenses and maintenance erode, giving an opportunity for pathogens to flourish (Latremouille, 2003; Ellis et al., 2008). Adequate nutrition is paramount to maintain the epithelial structure and integrity of this important defensive barrier, and in fact, lysine deficiency has been attributed as potential cause of fin erosion (Lall, 2010; Hardy, 2012).
The influence of stress on teleost nutritional requirements has been explored in several aquaculture species. Often, stress exposure triggers an elevation of nutritional needs, to fuel the stress response, causing an energy, nutrient and oxygen reallocation mediated by catecholamines and cortisol. As seen in this study, stressed groups 4s and 2s displayed modulation of hepatic energy mobilization, with lowered triglyceride levels by the end of the experiment. Modulation of energy substrate preference was also seen in a previous study of our group, on acute stress exposure with lumpfish (da Santa Lopes et al., 2023). Cortisol also modulates the AA metabolism (Aragão et al., 2008). Indeed, Costas et al. (2011a) demonstrated that exposing Senegalese sole (Solea senegalensis) to stress led to a modulation in AAs requirements (Costas et al., 2011a). Moreover, chronically stressed Senegalese sole dropped plasma free EAA concentrations, which according to authors can be due to either increased energetic demands or synthesis of important compounds needed to cope with stress (Costas et al., 2008). This corresponds to findings from the present study, where free plasma BCAAs and lysine levels were significantly lower in the most chronically stressed group, when compared to undisturbed fish. AAs such as BCAAs are ubiquitous, being used as building blocks of proteins, utilized as energy substrate and as signaling molecules (Van Waarde, 1988; Neinast et al., 2019; Ahmad et al., 2021). BCAAs integrate the AA chain composing virtually all proteins, including proteins relevant in the immune and antioxidant defense system. Oxidative stress is one of the outcomes of a stressed organism (Lushchak and Bagnyukova, 2007; Aschbacher et al., 2013; Sánchez-Muros et al., 2013). It increases nutritional demands, as the organism ups production of antioxidant defenses such as glutathione (GSH), superoxide dismutase and catalase to clear the reactive oxygen species (Iwasa et al., 2013; Espinosa-Diez et al., 2015). BCAA can be a key precursor of glutamate, which is necessary for GSH synthesis (Wu et al., 2004). In fact, dietary BCAA supplementation has proved effective in increasing expression of proteins involved in antioxidant defenses (Ichikawa et al., 2012; Iwasa et al., 2013). In addition, leucine is a potent activator of the mTOR pathway, which controls protein synthesis and cellular growth. Stress, through glucocorticoid action, has been shown to modulate mTOR activity, affecting protein synthesis and other metabolic processes (Valenzuela et al., 2018). Oxidative stress has been shown to alter mTOR in rats, being associated to retinal pathologies (Wang et al., 2022), a fact that should be better understood in fish.
Cataracts are a concerning disorder in cultured lumpfish (Imsland et al., 2021). Being an indicator of malnutrition and/or sub-optimal rearing conditions, cataracts can undermine the welfare of aquaculture species (Bjerkås et al., 2006; Peuhkuri et al., 2009). Lumpfish are deployed with the ultimate goal of preying on sea lice attached to salmons. If the eye health is undermined, this objective is most certainly unmet. In addition to this performance impairment from the eye of the farmer, there is also the welfare aspect, as lumpfish with poor eye health are also more likely to be ill adjusted for adequately feeding (Jonassen et al., 2017). It is reasonable to think that lumpfish, given the cataract severity and cohabiting with many considerably larger predatory teleosts such as salmon, will be less capable of adequately finding food. Naturally, as malnutrition progresses, the health of such fish will only erode.
In an epidemiological study of wild and farmed lumpfish, cataract incidence was significantly lower in wild mature lumpfish (Jonassen et al., 2017). The authors could not explain the cataract prevalence with an unbalance in histidine levels, as it has been previously reported in the salmonid aquaculture (Waagboø et al., 2010). The authors of the study suggested the cataract prevalence in lumpfish to be potentially related to disturbance in nutrient metabolism and malnutrition, via an AA imbalance, worsened by sub-optimal rearing. In addition, among the various AAs found with a significant positive correlation with cataract score, was lysine. In the present study, we could not observe significant differences in cataract prevalence between undisturbed and stressed fish, underpinning this urgent need for improved commercial diets and optimized rearing procedures. However, we did find an increase in BCAAs and lysine requirement on the group 4s. Lysine is both a precursor of polyamine synthesis and plays a pivotal role in collagen fiber integrity. Polyamines play an important role in cell growth and differentiation, while also providing stability for lens’ proteins such as crystallin and regulating osmotic stress (Wallace et al., 2003; Bloemendal et al., 2004; Mischiati et al., 2020; Munley et al., 2021; Han et al., 2022). Moreover, lysine is crucial for collagen synthesis which is of utmost importance for the structural integrity of the eye (Lythgoe, 1975; Bunce et al., 1990). Oxidative stress, which increases with stress, is highly correlated to caractogenesis, as the lens proteins and lipids suffer from the accumulation of reactive oxygen species (Varma et al., 1984; Berthoud and Beyer, 2009; Deering et al., 2023). An imbalance in dietary lysine can, thus, cripple the ability of the organism in, not only maintaining adequate antioxidant balance, but also, in tissue healing and maintenance, through impaired collagen and polyamine production.
Chronic stress will cause a sustained elevated release of cortisol until habituation or desensitization (Vijayan and Leatherland, 1990; Sopinka et al., 2016). In the early stages, the increase in cortisol will potentially result in enhanced immune functions, preparing the organism for challenge, as seen in this study, where bactericidal capacity was enhanced. With time, the sustained elevated cortisol levels will start having deleterious effects on the overall health of the fish (Opinion et al., 2023). The high energy and resource expenditure of maintaining an organism on this state of alertness will start to take its toll. The impact of the shift of resources from other biological functions to fuel the stress response will become increasingly noticeable (Schreck and Tort, 2016). Growth becomes impaired and immune functions suppressed as resources such as specific EAAs lack. If diet does not account for the increase expenditure, the building blocks will become scarce or insufficient (Winberg et al., 2016). As such, the oxidant–antioxidant balance will be disrupted, unleashing the cellular damage caused by high ROS levels (Sopinka et al., 2016). Disease resistance will be hampered, as immune suppression unfolds. Signs of health worsening will emerge, such as fin erosion and deteriorating eye health, exacerbated by sub-optimal nutrition. With the defenses lowered, opportunistic pathogens may carpe diem, exploiting this weakness (Pickering and Pottinger, 1989; Dhabhar, 2008; Yada and Tort, 2016). Thus, the absence of tailored aquafeeds for lumpfish that consider potentially elevated nutritional requirements, together with the lack of optimized rearing standards, results in a concerning puzzle that is the welfare status of lumpfish. If lumpfish are to be used as biological delousers in Atlantic salmon farming, there should be a focus on welfare improvement and stress mitigation research. To this end, it is important to consider dietary strategies that embrace increased nutritional demands together with improved aquacultures practices (Ashley, 2007; Costas, 2011; Costas et al., 2011b; Segner et al., 2012; Andersen et al., 2016; Sneddon et al., 2016; MaChado et al., 2018; Martos-Sitcha et al., 2020).
Lumpfish have been on the spotlight for their grim health and welfare situation. While they are assisting salmon farmers with their quest for mitigating the sea lice impacts, lumpfish welfare must be assured.
In this study, we shed light on the intricate modulatory effects of chronic stress on health, immunity, and potential nutritional requirements. The higher levels of cortisol in group 4s during the 6th week sampling subsided by the end of the experiment. Moreover, the immune-modulatory effects of cortisol were visible, with changes in plasma nitric oxide, bactericidal activity and peroxidase levels on stressed groups. Moreover, and although not significantly, the trend for lower growth and worse health score was seen in the most frequently stressed group. The cataract prevalence was high, nearly 100% among all groups, regardless of stress, pointing toward causes not just exclusive to stress experienced by lumpfish. There were clear modulatory effects of chronic stress on plasma free BCAAs and lysine levels, suggesting increased nutritional demands in lumpfish under chronic stressful conditions, which should be explored in future stress-immunonutrition research endeavors.
The raw data supporting the conclusions of this article will be made available by the authors, without undue reservation.
The animal study was approved by Norwegian Food Safety Authority (NFSA) under the application FOTS ID 26739. The study was conducted in accordance with the local legislation and institutional requirements.
TL: Conceptualization, Data curation, Formal Analysis, Funding acquisition, Investigation, Methodology, Project administration, Resources, Validation, Visualization, Writing – original draft, Writing – review & editing. BC: Conceptualization, Formal Analysis, Funding acquisition, Methodology, Resources, Supervision, Writing – review & editing. LR: Formal Analysis, Investigation, Methodology, Writing – review & editing. PR: Conceptualization, Funding acquisition, Investigation, Methodology, Project administration, Resources, Supervision, Writing – review & editing. AI: Conceptualization, Funding acquisition, Project administration, Resources, Supervision, Writing – review & editing. CA: Formal Analysis, Investigation, Methodology, Resources, Writing – review & editing. JF: Conceptualization, Funding acquisition, Investigation, Project administration, Resources, Supervision, Writing – review & editing.
The author(s) declare financial support was received for the research, authorship, and/or publication of this article. This study was financed by the Research Council of Norway, project number 317607; by the Gildeskål Forskningsstasjon AS; by Nord University, by Skretting AS; Lerøy Seafood Group, the Norwegian Seafood Research Fund (VEIEN, 901798) and the Icelandic Research Council (Rannís, 186971-0611). Gildeskål Forskningsstasjon AS, Skretting AS, and Lerøy Seafood Group were not involved in the study design, collection, analysis, interpretation of data, the writing of this article, or the decision to submit it for publication. The work presented in this paper was supported by national funds through the Foundation for Science and Technology (FCT, Portugal) within the scope of UIDB/04423/2020, UIDP/04423/2020, UIDB/04326/2020, UIDP/04326/2020, LA/P/0101/2020 and DL57/2016/CP1361/CT0033). JMOF acknowledges the grant ‘Severo Ochoa Centre of Excellence’ accreditation (CEX2019-000928-S) funded by AEI 10.13039/501100011033.
The authors thank the support and assistance provided by Mørkvedbukta Research Station’s staff and by CIIMAR’s A2S team.
Authors TL and PR were employed by company Gildeskål Forskningsstasjon AS.
The remaining authors declare that the research was conducted in the absence of any commercial or financial relationships that could be construed as a potential conflict of interest.
All claims expressed in this article are solely those of the authors and do not necessarily represent those of their affiliated organizations, or those of the publisher, the editors and the reviewers. Any product that may be evaluated in this article, or claim that may be made by its manufacturer, is not guaranteed or endorsed by the publisher.
The Supplementary Material for this article can be found online at: https://www.frontiersin.org/articles/10.3389/fmars.2024.1443710/full#supplementary-material
Afonso L. O. B. (2020). Identifying and managing maladaptive physiological responses to aquaculture stressors. Fish Physiol. 38, 163–191. doi: 10.1016/bs.fp.2020.10.002
Ahmad I., Ahmed I., Fatma S., Peres H. (2021). Role of branched-chain amino acids on growth, physiology and metabolism of different fish species: A review. Aquaculture Nutr. 27, 1270–1289. doi: 10.1111/anu.13267
Almeida J. R., Oliveira C., Gravato C., Guilhermino L. (2010). Linking behavioural alterations with biomarkers responses in the European seabass Dicentrarchus labrax L. exposed to the organophosphate pesticide fenitrothion. Ecotoxicology (London England) 19, 1369–1381. doi: 10.1007/s10646-010-0523-y
Aloísio Torres M., Pires Testa C., Gáspari C., Beatriz Masutti M., Maria Neves Panitz C., Curi-Pedrosa R., et al. (2002). Oxidative stress in the mussel Mytella guyanensis from polluted mangroves on Santa Catarina Island, Brazil. Mar. pollut. Bull. 44, 923–932. doi: 10.1016/S0025-326X(02)00142-X
Andersen S. M., Waagbø R., Espe M. (2016). Functional amino acids in fish nutrition, health and welfare. Front. Bioscience (Elite Edition) 8, 143–169. doi: 10.2741/757
Aragão C., Corte-Real J., Costas B., Dinis M. T., Conceição L. E. C. (2008). Stress response and changes in amino acid requirements in Senegalese sole (Solea Senegalensis Kaup 1858). Amino Acids 34, 143–148. doi: 10.1007/s00726-007-0495-2
Arends R. J., Mancera J. M., Muñoz J. L., Wendelaar Bonga S. E., Flik G. (1999). The stress response of the gilthead sea bream (Sparus aurata L.) to air exposure and confinement. J. Endocrinol. 163, 149–157. doi: 10.1677/joe.0.1630149
Aschbacher K., O’donovan A., Wolkowitz O. M., Dhabhar F. S., Su Y., Epel E. (2013). Good stress, bad stress and oxidative stress: insights from anticipatory cortisol reactivity. Psychoneuroendocrinology 38, 1698–1708. doi: 10.1016/j.psyneuen.2013.02.004
Ashley P. J. (2007). Fish welfare: Current issues in aquaculture. Appl. Anim. Behav. Sci. 104, 199–235. doi: 10.1016/j.applanim.2006.09.001
(2012). Charter of Fundamental Rights of the European Union. Available online at: https://eur-lex.europa.eu/legal-content/EN/TXT/?uri=celex%3AC2012%2F326%2F02.
(2019). The European Green Deal. Available online at: https://eur-lex.europa.eu/legal-content/EN/TXT/?uri=COM%3A2019%3A640%3AFIN.
Azeredo R., MaChado M., Martos-Sitcha J. A., Martínez-Rodríguez G., Moura J., Peres H., et al. (2019). Dietary Tryptophan Induces Opposite Health-Related Responses in the Senegalese Sole (Solea Senegalensis) Reared at Low or High Stocking Densities with Implications in Disease Resistance. Front. Physiol. 10. doi: 10.3389/FPHYS.2019.00508/BIBTEX
Azeredo R., Serra C. R., Oliva-Teles A., Costas B. (2017). Amino acids as modulators of the European seabass, Dicentrarchus labrax, innate immune response: An in vitro approach. Sci. Rep. 7, 18009. doi: 10.1038/s41598-017-18345-3
Barton B. A., Iwama G. K. (1991). Physiological changes in fish from stress in aquaculture with emphasis on the response and effects of corticosteroids. Annu. Rev. Fish Dis. 1, 3–26. doi: 10.1016/0959-8030(91)90019-G
Berthoud V. M., Beyer E. C. (2009). Oxidative stress, lens gap junctions, and cataracts. Antioxidants Redox Signaling 11, 339. doi: 10.1089/ars.2008.2119
Bird R. P., Draper H. H. (1984). Comparative studies on different methods of malonaldehyde determination. Methods Enzymology 105, 299–305. doi: 10.1016/S0076-6879(84)05038-2
Bjerkås E., Breck O., Waagbø R. (2006). The role of nutrition in cataract formation in farmed fish. CAB Reviews: Perspect. Agriculture Veterinary Science Nutr. Natural Resour. 1, 16–16. doi: 10.1079/PAVSNNR20061033
Bloemendal H., De Jong W., Jaenicke R., Lubsen N. H., Slingsby C., Tardieu A. (2004). Ageing and vision: Structure, stability and function of lens crystallins. Prog. Biophysics Mol. Biol. 86, 407–485. doi: 10.1016/j.pbiomolbio.2003.11.012
Bovenkerk B., Meijboom F. L. B. (2013). Fish welfare in aquaculture: explicating the chain of interactions between science and ethics. J. Agric. Environ. Ethics 26, 41–61. doi: 10.1007/S10806-012-9395-X/FIGURES/1
Brown C. (2015). “Fish intelligence, sentience and ethics,” in Animal Cognition, vol. 18. (New York, US: Springer Verlag), 1–17. doi: 10.1007/s10071-014-0761-0
Bunce G. E., Kinoshita J., Horwitz J. (1990). Nutritional factors in cataract. Annu. Rev. Nutr. 10, 233–254. doi: 10.1146/annurev.nu.10.070190.001313
Castillo J., Teles M., Mackenzie S., Tort L. (2009). Stress-related hormones modulate cytokine expression in the head kidney of gilthead seabream (Sparus aurata). Fish Shellfish Immunol. 27, 493–499. doi: 10.1016/j.fsi.2009.06.021
Ciji A., Akhtar M. S. (2021). Stress management in aquaculture: a review of dietary interventions. Rev. Aquaculture 13, 2190–2247. doi: 10.1111/raq.12565
Claiborne A. (1985). “Catalase activity,” in Handbook Methods for Oxygen Radical Research. Ed. Greenwald R. A. (Boca Raton, US: CRC Press), 283–284. doi: 10.1201/9781351072922
Costas B. (2011). Stress mitigation in sole (Solea Senegalensis) through improved nitrogen nutrition: amino acid utilization, disease resistance and immune status (Porto, Portugal: University of Porto).
Costas B., Aragão C., Mancera J. M., Dinis M. T., Conceição L. E. C., Refojos B. C. (2008). High stocking density induces crowding stress and affects amino acid metabolism in Senegalese sole Solea Senegalensis (Kaup 1858) juveniles. Aquaculture Res. 39, 1–9. doi: 10.1111/j.1365-2109.2007.01845.x
Costas B., Conceição L. E. C., Aragão C., Martos J. A., Ruiz-Jarabo I., Mancera J. M., et al. (2011a). Physiological responses of Senegalese sole (Solea Senegalensis Kaup 1858) after stress challenge: Effects on non-specific immune parameters, plasma free amino acids and energy metabolism. Aquaculture 316, 68–76. doi: 10.1016/j.aquaculture.2011.03.011
Costas B., Conceição L. E. C., Dias J., Novoa B., Figueras A., Afonso A. (2011b). Dietary arginine and repeated handling increase disease resistance and modulate innate immune mechanisms of Senegalese sole (Solea Senegalensis Kaup 1858). Fish Shellfish Immunol. 31, 838–847. doi: 10.1016/j.fsi.2011.07.024
Costas B., Simões I., Castro-Cunha M., Afonso A. (2014). Non-specific immune responses of Senegalese sole, Solea Senegalensis (Kaup), head-kidney leucocytes against Tenacibaculum maritimum. J. Fish Dis. 37, 765–769. doi: 10.1111/jfd.12171
da Santa Lopes T., Costas B., Ramos-Pinto L., Reynolds P., Imsland A. K. D., Fernandes J. M. O. (2023). Exploring the effects of acute stress exposure on lumpfish plasma and liver biomarkers. Animals 13, 3623. doi: 10.3390/ani13233623
Deering M. J., Paradis H., Ahmad R., Al-Mehiawi A. S., Gendron R. L. (2023). The role of dietary vitamin A in mechanisms of cataract development in the teleost lumpfish (Cyclopterus lumpus L). J. Fish Dis. 47, 1–15. doi: 10.1111/JFD.13899
Dhabhar F. S. (2008). Enhancing versus Suppressive Effects of Stress on Immune Function: Implications for Immunoprotection versus Immunopathology. Allergy Asthma Clin. Immunology 4, 2. doi: 10.1186/1710-1492-4-1-2
Ellis A. E. (1990a). “Lysozyme assays,” in Techniques in Fish Immunology. Eds. Stolen J. S., Fletcher T. C., Anderson D. P., Roberson B. S., van Muiswinkel W. B. (SOS Publications, Fair Haven), 101–103.
Ellis A. E. (1990b). Serum Antiproteases in Fish. In: Stolen J.S., Fletcher T.C., Anderson D.P., Roberson B.S., van Muiswinkel W.B. editors. Techniques in Fish Immunology. 1st ed. (Fair Haven, MA, USA: SOS Publications) pp. 95–99. Available at: https://cir.nii.ac.jp/crid/1573950399902395392.bib?lang=en.
Ellis T., Oidtmann B., St-Hilaire S., Turnbull J. F., North B. P., Macintyre C. M., et al. (2008). “Fin erosion in farmed fish,” in Fish Welfare (Oxford, UK: Blackwell Publishing Ltd), 121–149. doi: 10.1002/9780470697610.ch9
Espinosa-Diez C., Miguel V., Mennerich D., Kietzmann T., Sánchez-Pérez P., Cadenas S., et al. (2015). Antioxidant responses and cellular adjustments to oxidative stress. Redox Biol. 6, 183–197. doi: 10.1016/j.redox.2015.07.008
Espmark Å. M., Noble C., Kolarevic J., Marit Berge G., Hansen Aas G., Tuene S., et al. (2019). Welfare in cleaner fish – operational welfare indicators (OWI) - RENSVEL. Nofima Rapport, 12/2019. Available online at: www.nofima.no.
Fast M. D., Hosoya S., Johnson S. C., Afonso L. O. B. (2008). Cortisol response and immune-related effects of Atlantic salmon (Salmo salar Linnaeus) subjected to short- and long-term stress. Fish Shellfish Immunol. 24, 194–204. doi: 10.1016/j.fsi.2007.10.009
Fiskeridirektoratet (2021). Aquaculture statistics: Use of cleanerfish 1998-2021 (Oslo, Norway: Norwegian Directorate of Fisheries). Available at: https://www.fiskeridir.no/English/Aquaculture/Statistics/Cleanerfish-Lumpfish-and-Wrasse.
Garcia de Leaniz C., Gutierrez Rabadan C., Barrento S. I., Stringwell R., Howes P. N., Whittaker B. A., et al. (2022). Addressing the welfare needs of farmed lumpfish: Knowledge gaps, challenges and solutions. Reviews in Aquaculture, vol. 14, 139–155. doi: 10.1111/raq.12589
Graham S., Secombes C. J. (1988). The production of a macrophage-activating factor from rainbow trout Salmo gairdneri leucocytes. Immunology 65, 293.
Hale M. E. (2000). Startle responses of fish without Mauthner neurons: escape behavior of the lumpfish (Cyclopterus lumpus). Biol. Bull. 199, 180–182. doi: 10.2307/1542886
Han W., Li H., Chen B. (2022). Research progress and potential applications of spermidine in ocular diseases. Pharmaceutics 14, 1500. doi: 10.3390/pharmaceutics14071500
Hardy R. W. (2012). “The nutritional pathology of teleosts,” in Fish Pathology (New Jersey, US: Wiley-Blackwell), 402–424. doi: 10.1002/9781118222942.ch10
Herrera M., Mancera J. M., Costas B. (2019). The use of dietary additives in fish stress mitigation: Comparative endocrine and physiological responses. Front. Endocrinol. 10. doi: 10.3389/FENDO.2019.00447/BIBTEX
Ichikawa K., Okabayashi T., Shima Y., Iiyama T., Takezaki Y., Munekage M., et al. (2012). Branched-chain amino acid-enriched nutrients stimulate antioxidant DNA repair in a rat model of liver injury induced by carbon tetrachloride. Mol. Biol. Rep. 39, 10803–10810. doi: 10.1007/s11033-012-1974-4
Imsland A. K. D., Hanssen A., Nytrø A. V., Reynolds P., Jonassen T. M., Hangstad T. A., et al. (2018). ). It works! Lumpfish can significantly lower sea lice infestation in large-scale salmon farming. Biol. Open 7, bio036301. doi: 10.1242/bio.036301
Imsland A. K. D., Reynolds P., Hangstad T. A., Kapari L., Maduna S. N., Hagen S. B., et al. (2021). Quantification of grazing efficacy, growth and health score of different lumpfish (Cyclopterus lumpus L.) families: Possible size and gender effects. Aquaculture 530, 735925. doi: 10.1016/j.aquaculture.2020.735925
Iwasa M., Kobayashi Y., Mifuji-Moroka R., Hara N., Miyachi H., Sugimoto R., et al. (2013). Branched-chain amino acid supplementation reduces oxidative stress and prolongs survival in rats with advanced liver cirrhosis. PLoS One 8, e70309. doi: 10.1371/journal.pone.0070309
Jonassen T., Hamadi M., Remø S. C., Waagbø R. (2017). An epidemiological study of cataracts in wild and farmed lumpfish (Cyclopterus lumpus L.) and the relation to nutrition. J. Fish Dis. 40, 1903–1914. doi: 10.1111/jfd.12664
Jørgensen E. H., Haatuft A., Puvanendran V., Mortensen A. (2017). Effects of reduced water exchange rate and oxygen saturation on growth and stress indicators of juvenile lumpfish (Cyclopterus lumpus L.) in aquaculture. Aquaculture 474, 26–33. doi: 10.1016/j.aquaculture.2017.03.019
Kaushik S. J., Seiliez I. (2010). Protein and amino acid nutrition and metabolism in fish: current knowledge and future needs. Aquaculture Res. 41, 322–332. doi: 10.1111/are.2010.41.issue-3
Khansari A. R., Carles Balasch J., Reyes-López F. E., Tort L. (2017). Stressing the inflammatory network: Immuno-endocrine responses to allostatic load in fish NANOAu-Effects of gold nanoparticles to aquatic organisms View project. Article J. Mar. Sci. Technol. 8, 401.
Lall S. P. (2010). “Disorders of nutrition and metabolism,” in Fish diseases and disorders, vol. 2. (Oxfordshire, UK: CABI), 202–237. doi: 10.1079/9781845935535.0202
Latremouille D. N. (2003). Fin erosion in aquaculture and natural environments. Rev. Fisheries Sci. 11, 315–335. doi: 10.1080/10641260390255745
Li P., Mai K., Trushenski J., Wu G. (2009). New developments in fish amino acid nutrition: towards functional and environmentally oriented aquafeeds. Amino Acids 37, 43–53. doi: 10.1007/s00726-008-0171-1
Li P., Yin Y.-L., Li D., Woo Kim S., Wu G. (2007). Amino acids and immune function. Br. J. Nutr. 98, 237–252. doi: 10.1017/S000711450769936X
Lima I., Moreira S. M., Osten J. R., Soares A. M. V. M., Guilhermino L. (2007). Biochemical responses of the marine mussel Mytilus galloprovincialis to petrochemical environmental contamination along the North-western coast of Portugal. Chemosphere 66, 1230–1242. doi: 10.1016/j.chemosphere.2006.07.057
Lushchak V. I., Bagnyukova T. V. (2007). Hypoxia induces oxidative stress in tissues of a goby, the rotan Perccottus glenii. Comp. Biochem. Physiol. Part B: Biochem. Mol. Biol. 148, 390–397. doi: 10.1016/j.cbpb.2007.07.007
Lythgoe J. N. (1975). The structure and function of iridescent corneas in teleost fishes. Proc. R. Soc. London. Ser. B. Biol. Sci. 188, 437–457. doi: 10.1098/rspb.1975.0030
MaChado M., Arenas F., Svendsen J. C., Azeredo R., Pfeifer L. J., Wilson J. M., et al. (2020). Effects of water acidification on Senegalese sole Solea Senegalensis health status and metabolic rate: implications for immune responses and energy use. Front. Physiol. 11. doi: 10.3389/FPHYS.2020.00026/BIBTEX
MaChado M., Azeredo R., Díaz-Rosales P., Afonso A., Peres H., Oliva-Teles A., et al. (2015). Dietary tryptophan and methionine as modulators of European seabass (Dicentrarchus labrax) immune status and inflammatory response. Fish Shellfish Immunol. 42, 353–362. doi: 10.1016/j.fsi.2014.11.024
MaChado M., Azeredo R., Fontinha F., Fernández-Boo S., Conceição L. E. C., Dias J., et al. (2018). Dietary methionine improves the european seabass (Dicentrarchus labrax) immune status, inflammatory response, and disease resistance. Front. Immunol. 9. doi: 10.3389/fimmu.2018.02672
Magalhães R., Martins N., Fontinha F., Olsen R. E., Serra C. R., Peres H., et al. (2023). Dietary ARA, DHA, and carbohydrate ratios affect the immune status of gilthead sea bream juveniles upon bacterial challenge. Animals 13, 1770. doi: 10.3390/ani13111770
Martos-Sitcha J. A., Mancera J. M., Prunet P., Magnoni L. J. (2020). Editorial: welfare and stressors in fish: challenges facing aquaculture. Front. Physiol. 11. doi: 10.3389/fphys.2020.00162
Mattilsynet (2020). Nasjonal tilsynskampanje 2018/2019 Velferd Hos Rensefisk (Oslo, Norway: The Norwegian Food Safety Authority’s National Inspection Projects). Available at: https://www.mattilsynet.no/fisk_og_akvakultur/akvakultur/rensefisk/mattilsynet_sluttrapport_rensefiskkampanje_2018:2019.37769/binary/Mattilsynetsluttrapportrensefiskkampanje2018-2019.
Mischiati C., Feriotto G., Tabolacci C., Domenici F., Melino S., Borromeo I., et al. (2020). Polyamine oxidase is involved in spermidine reduction of transglutaminase type 2-catalyzed βH-crystallins polymerization in calcium-induced experimental cataract. Int. J. Mol. Sci. 21, 5427. doi: 10.3390/ijms21155427
Molfino A., Rossi-Fanelli F., Laviano A. (2009). The interaction between pro-inflammatory cytokines and the nervous system. Nat. Rev. Cancer 9, 224–224. doi: 10.1038/nrc2507-c1
Mommsen T. P., Vijayan M. M., Moon T. W. (1999). Cortisol in teleosts: Dynamics, mechanisms of action, and metabolic regulation. Rev. Fish Biol. Fisheries 9, 211–268. doi: 10.1023/A:1008924418720/METRICS
Mosser D. M., Edwards J. P. (2008). Exploring the full spectrum of macrophage activation. Nat. Rev. Immunol. 8, 958–969. doi: 10.1038/nri2448
Munley K. M., Liu D., Galvez F. (2021). Increased polyamine levels and maintenance of γ-aminobutyric acid (Gaba) homeostasis in the gills is indicative of osmotic plasticity in killifish. Comp. Biochem. Physiol. Part A: Mol. Integr. Physiol. 257, 110969. doi: 10.1016/j.cbpa.2021.110969
Neinast M., Murashige D., Arany Z. (2019). Branched chain amino acids. Annu. Rev. Physiol. 81, 139. doi: 10.1146/annurev-physiol-020518-114455
Noble C., Iversen M. H., Lein I., Kolarevic J., Johansen L.-H., Berge G. M., et al. (2019). Rensvel OWI fact sheet series: An introduction to Operational and Laboratory-based Welfare Indicators for lumpfish ( Cyclopterus lumpus L.) (Tromsø, Norway: Nofima). Available at: https://www.fhf.no/prosjekter/prosjektbasen/901136/.
Opinion A. G. R., Vanhomwegen M., De Boeck G., Aerts J. (2023). Long-term stress induced cortisol downregulation, growth reduction and cardiac remodeling in Atlantic salmon. J. Exp. Biol. 226, jeb246504. doi: 10.1242/jeb.246504
Peixoto D., Pinto W., Gonçalves A. T., MaChado M., Reis B., Silva J., et al. (2021). Microalgal biomasses have potential as ingredients in microdiets for Senegalese sole (Solea Senegalensis) post-larvae. J. Appl. Phycology 33, 2241–2250. doi: 10.1007/s10811-021-02431-1
Peuhkuri N., Bjerkås E., Brännäs E., Piironen J., Primmer C., Taskinen J. (2009). Looking fish in the eye- cataract as a problem in fish farming. Available online at: http://norden.diva-portal.org/smash/get/diva2:700809/FULLTEXT01.pdf.
Pickering A. D., Pottinger T. G. (1989). Stress responses and disease resistance in salmonid fish: Effects of chronic elevation of plasma cortisol. Fish Physiol. Biochem. 7, 253–258. doi: 10.1007/BF00004714
Quade M. J., Roth J. A. (1997). A rapid, direct assay to measure degranulation of bovine neutrophil primary granules. Veterinary Immunol. Immunopathology 58, 239–248. doi: 10.1016/S0165-2427(97)00048-2
Ramos-Pinto L. (2020). Amino acids as key mediators of immune status and nutritional condition in fish Vol. 93 (Porto, Portugal: University of Porto). University of Porto.
Reid S. G., Bernier N. J., Perry S. F. (1998). The adrenergic stress response in fish: Control of catecholamine storage and release. Comp. Biochem. Physiol. - C Pharmacol. Toxicol. Endocrinol. 120, 1–27. doi: 10.1016/S0742-8413(98)00037-1
Reis B., Ramos-Pinto L., Martos-Sitcha J. A., MaChado M., Azeredo R., Fernández-Boo S., et al. (2021). Health status in gilthead seabream (Sparus aurata) juveniles fed diets devoid of fishmeal and supplemented with Phaeodactylum tricornutum. J. Appl. Phycology 33, 979–996. doi: 10.1007/S10811-021-02377-4/METRICS
Remen M., Nes A. M., Hangstad T. A., Geraudie P., Reynolds P., Urskog T. C., et al. (2022). Temperature and size-dependency of lumpfish (Cyclopterus lumpus) oxygen requirement and tolerance. Aquaculture 548, 737576. doi: 10.1016/j.aquaculture.2021.737576
Reynolds P., Imsland A. K. D., Boissonnot L. (2022). Causes of mortality and loss of lumpfish Cyclopterus lumpus. Fishes 7, 328. doi: 10.3390/fishes7060328
Sadoul B., Vijayan M. M. (2016). Stress and growth. Fish Physiol. 35, 167–205. doi: 10.1016/B978-0-12-802728-8.00005-9
Saeij J. P. J., Verburg-Van Kemenade L. B. M., Van Muiswinkel W. B., Wiegertjes G. F. (2003). Daily handling stress reduces resistance of carp to Trypanoplasma borreli: In vitro modulatory effects of cortisol on leukocyte function and apoptosis. Dev. Comp. Immunol. 27, 233–245. doi: 10.1016/S0145-305X(02)00093-9
Sánchez-Muros M. J., Villacreces S., Miranda-de la Lama G., de Haro C., García-Barroso F. (2013). Effects of chemical and handling exposure on fatty acids, oxidative stress and morphological welfare indicators in gilt-head sea bream (Sparus aurata). Fish Physiol. Biochem. 39, 581–591. doi: 10.1007/S10695-012-9721-2/METRICS
Schreck C. B., Tort L. (2016). The concept of stress in fish. Fish Physiol. 35, 1–34. doi: 10.1016/B978-0-12-802728-8.00001-1
Segner H., Sundh H., Buchmann K., Douxfils J., Snuttan Sundell K., Mathieu C., et al. (2012). Health of farmed fish: its relation to fish welfare and its utility as welfare indicator. Fish Physiol. Biochem. 38, 85–105. doi: 10.1007/s10695-011-9517-9
Skår M. W., Haugland G. T., Powell M. D., Wergeland H. I., Samuelsen O. B. (2017). Development of anaesthetic protocols for lumpfish (Cyclopterus lumpus L.): Effect of anaesthetic concentrations, sea water temperature and body weight. PloS One 12, e0179344. doi: 10.1371/journal.pone.0179344
Sneddon L. U., Wolfenden D. C. C., Thomson J. S. (2016). Stress management and welfare. Fish Physiol. 35, 463–539. doi: 10.1016/B978-0-12-802728-8.00012-6
Sopinka N. M., Donaldson M. R., O’Connor C. M., Suski C. D., Cooke S. J. (2016). Stress indicators in fish. Fish Physiol. 35, 405–462. doi: 10.1016/B978-0-12-802728-8.00011-4
Staven F. R., Nordeide J. T., Imsland A. K., Andersen P., Iversen N. S., Kristensen T. (2019). Is habituation measurable in lumpfish Cyclopterus lumpus when used as cleaner fish in atlantic salmon Salmo salar aquaculture? Front. Veterinary Sci. 6. doi: 10.3389/FVETS.2019.00227/BIBTEX
Stien L. H., Størkersen K. V., Gåsnes S. K. (2020). Analyse av dødelighetsdata fra spørreundersøkelse om velferd hos rensefisk. Rapport fra havforskningen, 2020-6. Havforskningsinstituttet, Trondheim, Norway. Available at: https://www.hi.no/hi/nettrapporter/rapport-fra-havforskningen-2020-6.
Tort L. (2011). Stress and immune modulation in fish. Dev. Comp. Immunol. 35, 1366–1375. doi: 10.1016/j.dci.2011.07.002
Tort L., Balasch J. C., Mackenzie S. (2003). Fish immune system. A crossroads between innate and adaptive responses. Inmunología 22, 277–286.
Urbinati E. C., Zanuzzo F. S., Biller J. D. (2020). Stress and immune system in fish. Biol. Physiol. Freshw. Neotropical Fish, 93–114. doi: 10.1016/B978-0-12-815872-2.00005-1
Valenzuela C. A., Zuloaga R., Mercado L., Einarsdottir I. E., Björnsson B. T., Valdés J. A., et al. (2018). Chronic stress inhibits growth and induces proteolytic mechanisms through two different nonoverlapping pathways in the skeletal muscle of a teleost fish. Am. J. Physiol. - Regul. Integr. Comp. Physiol. 314, R102–R113. doi: 10.1152/AJPREGU.00009.2017/ASSET/IMAGES/LARGE/ZH60121793710008.JPEG
Van Waarde A. (1988). Biochemistry of non-protein nitrogenous compounds in fish including the use of amino acids for anaerobic energy production. Comp. Biochem. Physiol. Part B: Comp. Biochem. 91, 207–228. doi: 10.1016/0305-0491(88)90136-8
Varma S. D., Chand D., Sharma Y. R., Kuck J. F., Richards R. D. (1984). Oxidative stress on lens and cataract formation: role of light and oxygen. Curr. Eye Res. 3, 35–58. doi: 10.3109/02713688408997186
Verburg-van Kemenade B. M. L., Ribeiro C. M. S., Chadzinska M. (2011). Neuroendocrine–immune interaction in fish: Differential regulation of phagocyte activity by neuroendocrine factors. Gen. Comp. Endocrinol. 172, 31–38. doi: 10.1016/j.ygcen.2011.01.004
Verburg-Van Kemenade B. M. L., Stolte E. H., Metz J. R., Chadzinska M. (2009). Chapter 7 neuroendocrine–immune interactions in teleost fish. Fish Physiol. 28, 313–364. doi: 10.1016/S1546-5098(09)28007-1
Vijayan M. M., Leatherland J. F. (1990). High stocking density affects cortisol secretion and tissue distribution in brook charr, Salvelinus fontinalis. J. Endocrinol. 124, 311–318. doi: 10.1677/joe.0.1240311
Waagboø R., Tröße C., Koppe W., Fontanillas R., Breck O. (2010). Dietary histidine supplementation prevents cataract development in adult Atlantic salmon, Salmo salar L., in seawater. Br. J. Nutr. 104, 1460–1470. doi: 10.1017/S0007114510002485
Wallace H. M., Fraser A. V., Hughes A. (2003). A perspective of polyamine metabolism. Biochem. J. 376, 1. doi: 10.1042/bj20031327
Wang Y., Fung N. S. K., Lam W. C., Lo A. C. Y. (2022). mTOR signalling pathway: A potential therapeutic target for ocular neurodegenerative diseases. Antioxidants 11, 1304. doi: 10.3390/antiox11071304
Wendelaar Bonga S. E. (1997). The stress response in fish. Physiol. Rev. 77, 591–625. doi: 10.1152/physrev.1997.77.3.591
Winberg S., Höglund E., Øverli Ø. (2016). Variation in the neuroendocrine stress response. Fish Physiol. 35, 35–74. doi: 10.1016/B978-0-12-802728-8.00002-3
Wojtaszek J., Dziewulska-Szwajkowska D., Lozińska-Gabska M., Adamowicz A., Dugaj A. (2002). Hematological effects of high dose of cortisol on the carp (Cyprinus carpio L.): cortisol effect on the carp blood. Gen. Comp. Endocrinol. 125, 176–183. doi: 10.1006/gcen.2001.7725
Wu G., Fang Y. Z., Yang S., Lupton J. R., Turner N. D. (2004). Glutathione metabolism and its implications for health. J. Nutr. 134, 489–492. doi: 10.1093/jn/134.3.489
Yada T., Tort L. (2016). Stress and disease resistance: immune system and immunoendocrine interactions. Fish Physiol. 35, 365–403. doi: 10.1016/B978-0-12-802728-8.00010-2
Yoneda J., Andou A., Takehana K. (2009). Regulatory roles of amino acids in immune response. Curr. Rheumatol. Rev. 5, 252–258. doi: 10.2174/157339709790192567
Keywords: cleaner fish, lumpfish, salmon farming, stress, health, welfare, amino acids
Citation: Lopes TdS, Costas B, Ramos-Pinto L, Reynolds P, Imsland AKD, Aragão C and Fernandes JMO (2024) Lumpfish physiological response to chronic stress. Front. Mar. Sci. 11:1443710. doi: 10.3389/fmars.2024.1443710
Received: 04 June 2024; Accepted: 22 July 2024;
Published: 09 August 2024.
Edited by:
Yi-Feng Li, Shanghai Ocean University, ChinaReviewed by:
Carlo C. Lazado, Norwegian Institute of Food, Fisheries and Aquaculture Research (Nofima), NorwayCopyright © 2024 Lopes, Costas, Ramos-Pinto, Reynolds, Imsland, Aragão and Fernandes. This is an open-access article distributed under the terms of the Creative Commons Attribution License (CC BY). The use, distribution or reproduction in other forums is permitted, provided the original author(s) and the copyright owner(s) are credited and that the original publication in this journal is cited, in accordance with accepted academic practice. No use, distribution or reproduction is permitted which does not comply with these terms.
*Correspondence: Jorge M. O. Fernandes, am9yZ2UubS5mZXJuYW5kZXNAbm9yZC5ubw==
†Present address: Jorge M. O. Fernandes, Institute of Marine Sciences (ICM-CSIC), Barcelona, Spain
Disclaimer: All claims expressed in this article are solely those of the authors and do not necessarily represent those of their affiliated organizations, or those of the publisher, the editors and the reviewers. Any product that may be evaluated in this article or claim that may be made by its manufacturer is not guaranteed or endorsed by the publisher.
Research integrity at Frontiers
Learn more about the work of our research integrity team to safeguard the quality of each article we publish.