- 1State Key Laboratory of Marine Environmental Science, College of Ocean and Earth Sciences, Xiamen University, Xiamen, China
- 2Key Laboratory of Urban Environment and Health, Institute of Urban Environment, Chinese Academy of Sciences, Xiamen, China
- 3Department of Ocean Science, The Hong Kong University of Science and Technology, Kowloon, Hong Kong SAR, China
- 4School of Earth and Space Sciences, Peking University, Beijing, China
- 5Xiamen Key Laboratory of Urban Sea Ecological Conservation and Restoration (USER), Xiamen, China
As a response of the coastal oceans to more severe anthropogenic disturbance, increasing coastal primary production has been more frequently observed in recent years. This raises the question of how this progressive change reshapes the long-term carbon sequestration in the coastal oceans. With this question in mind, we investigated the sediment organic matter (OM) preserved on the shelf of the northern South China Sea (NSCS) where high primary production has been observed. Across this sediment core, relatively low total organic carbon content of around 0.60% was observed. Fatty acids with less carbon numbers exhibit exponential decreases downward with degradation rate constants ranging from 0.10 to 0.17 y-1, no matter they were from single sources (14:0 presumably from marine OM and ai-15:0, i-15:0, 15:0 from bacterial OM) or mixed sources (16:0). Meanwhile fatty acids with more carbon numbers, either 18:0 and 24:0 from mixed sources, or 26:0 and 28:0 presumably from terrestrial input, were less varied in concentrations with depth. This demonstrates a preferential decomposition of labile fatty acids during the early diagenesis of coastal sediment organic matter. A decrease of labile fatty acids δ13C values was observed with the decomposition, from which kinetic isotope fractionations were predicted ranging from 0.7 to 1.5‰. This provides an isotopic constraint of the mixing model to quantify labile organic matter from terrestrial input and local phytoplankton production, from which declined contribution of local phytoplankton production to labile fatty acids was identified in more recent sediment. This finding together with the low organic carbon content and rapid removal of fatty acids in the top sediment, demonstrates the poor preservation of labile organic matter on the shelf of NSCS, implying that the increasing primary production has not enhanced the coastal carbon sequestration yet, but rather leads to more intensified respiration with a potential risk to increase the CO2 outgassing from the coastal oceans.
Introduction
As the area most intensively influenced by anthropogenic disturbance, the coastal ocean has been found in the process toward a net sink of atmospheric CO2 (Bauer et al., 2013). Along with this process is a rapid decline of dissolved oxygen (DO) in coastal waters partially due to overloaded organic matter (Breitburg et al., 2018; Fennel and Testa, 2019). In the coastal ocean, shifting flux and origins of organic matter have been observed, which is considered a consequence of the changing river discharge, terrestrial input, and nutrient loading due to the alteration of land use and damming activities along the rivers (Canuel et al., 2012). For example, high nutrient loading in the Pearl River Estuary (PRE) from rapid urbanization and shift in agriculture activities fertilized the adjacent continental shelf of the northern South China Sea (NSCS) (Han et al., 2012). A predominant contribution of production from local phytoplankton has been suggested using the carbon isotope values of amino acids (Yang et al., 2020), demonstrating the potential of excessive growth of phytoplankton on the shelf of NSCS (Su et al., 2017; Li et al., 2023). It thus remains the question, whether the elevated labile organic matter in water column with increasing primary production can be preserved in the sediment underneath, which in turn influence the long-term carbon sequestration of the coastal ocean.
To trace the changing sources of organic matter in the coastal region, the mixing model based on the carbon isotope ratios (δ13C) of bulk organic carbon has been widely applied (Bianchi and Bauer, 2011 and the reference therein). The introduction of δ13C values of individual molecules further allows the identification of organic matter from specific origins (Close, 2019). Among those applications, the fatty acids δ13C were the most successful one. The n-alkyl fatty acids are a group of compounds with a span of decompositional reactivity and species-specific origins. It has been reported that individual fatty acids show substantially different reactivity against the decomposition during the early diagenesis (Sun et al., 1997; Arzayus and Canuel, 2004). It thus leads to dynamic compositional variations of those fatty acids even in the same samples (Sun and Wakeham, 1994; Wakeham et al., 1997). On the other hand, fatty acids provide clear origin information (Bianchi and Canuel, 2011), e.g. branched fatty acids (BRFA) e.g. i-15:0 and ai-15:0 come primarily from bacteria (Kaneda, 1991; Volkman et al., 1998), while long-chain fatty acids (LCFA) e.g. 26:0 and 28:0 are generally considered as tracers of the vascular plant (Eglinton and Hamilton, 1967; Volkman, 1986). The diversified origins give fatty acids characteristic stable carbon isotope values, which can be applied to identify the origin of sediment organic matter (Canuel et al., 1995; Bouillon and Boschker, 2006). Nevertheless, substantially different isotopic distributions among fatty acids was observed in sediment (Canuel and Martens, 1996; Boschker et al., 1999; Shi et al., 2001) and water column (Naraoka and Ishiwatari, 2000; Close et al., 2013), which potentially limits the application of fatty acid δ13C, particularly for those labile compounds (Bouillon et al., 2012). This diversity is partially a result of the kinetic isotopic effect (KIE) of fatty acids with varied reactivity during the decomposition, which has been poorly constrained at the molecular level.
The origin and geochemical behavior of sediment organic matter on the NSCS shelf have been relatively well documented. The sediment fatty acids were found to primarily originate from terrestrial input from PRE and locally produced phytoplankton (Hu et al., 2002, 2006; Chen et al., 2008). A combination of δ13C and Δ14C values of fatty acids from surface sediment on the NSCS shelf further demonstrates the highly dynamic distribution of organic matter from terrestrial input (Wei et al., 2021). Nevertheless, questions have remained whether fatty acid δ13C values without a constrain of KIE can be applied to identify the geochemical behavior of labile organic matter in sediment under the stress of intensified coastal eutrophication. With this in mind, we compared the abundance and δ13C distribution of fatty acids with varied lability in a short sediment core from the shelf of NSCS near the mouth of the PRE river during a cruise from June 1st, 2021 to June 29th, 2021 (Figure 1). We first used a G-model to determine which fatty acids were influenced by decomposition. Then a closed-system Rayleigh equation was applied to evaluate the KIE of individual fatty acids. The KIE of fatty acids with substantial decomposition was introduced into the mixing model to calibrate the relative contribution of marine and terrestrial input to labile organic matter on the shelf of NSCS.
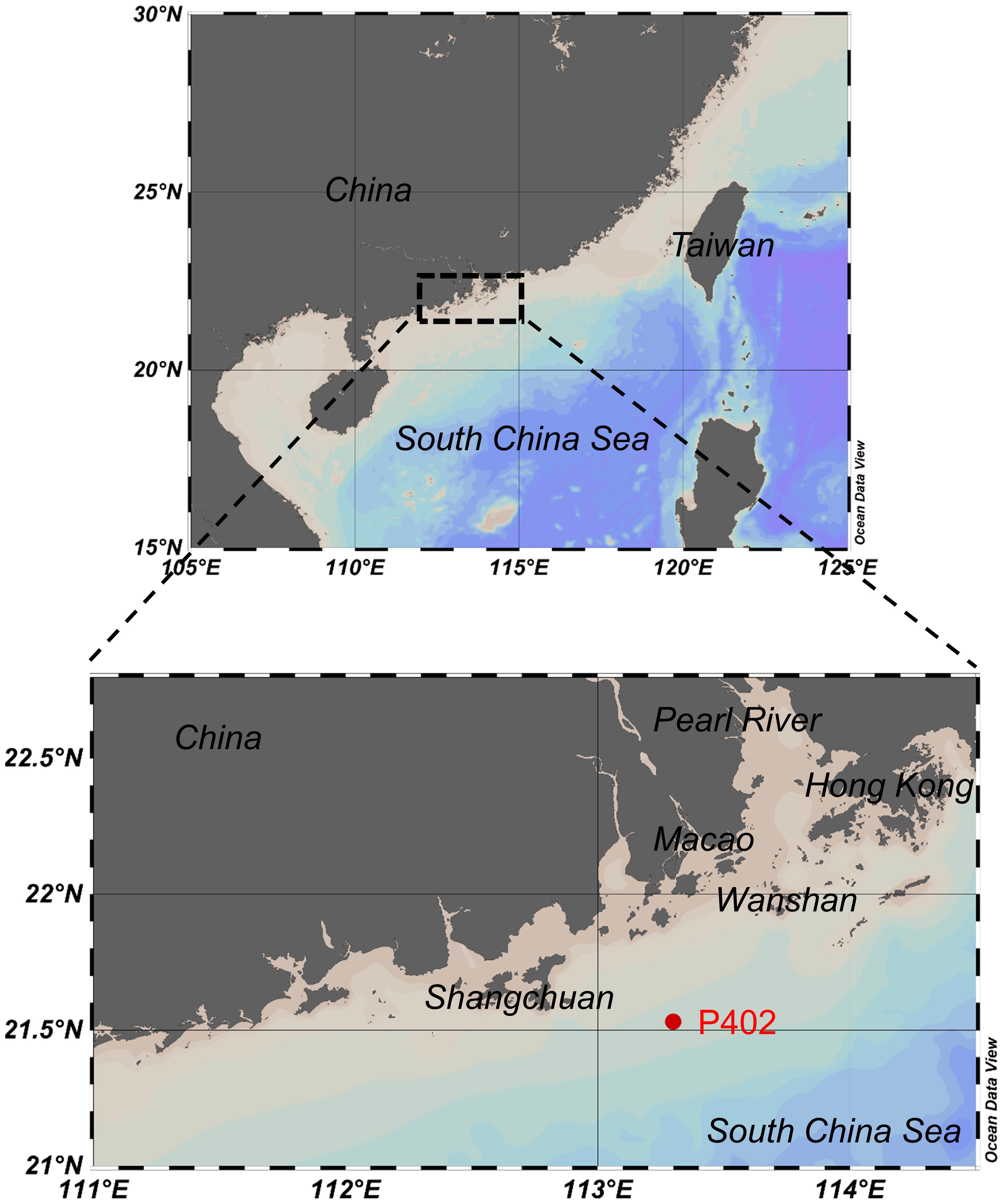
Figure 1 Location of the collected sediment samples at P402 (filled star) on the shelf of the northern South China Sea in June 2021. The figure was prepared using Ocean Data View.
Methods
Sample collections
Using the gravity coring device (UWITEC Sediment corer), sediment samples were collected at station P402 (21°53’N, 113°30’E) in June 2021 on the shelf of the northern South China Sea adjacent to the Pearl River Estuary (Figure 1). The sediment core is around 26 cm. Subsamples were segmented into pieces of 1 cm from the top 10 cm of the core, then into pieces of 2 cm for the rest. After freeze-drying, the samples were ground into powder in a mortar and pestle. Any large particles such as rocks and shells were removed, and the samples were sieved (200 mesh) before being stored at 4°C for further analysis.
Total organic carbon content and stable carbon isotope values
Subsamples from the sediment core were treated with drops of 1 mol L-1 HCl to remove any inorganic carbon before dried at 60°C for 48 hours (Kao et al., 2012). The concentrations and δ13C values of total organic carbon (TOC) and nitrogen (TN) were then analyzed from the acidified samples using an elemental analyzer - isotope ratio mass spectrometry (EA-IRMS). The concentrations and δ13C values were calibrated using isotope standards, acetanilide (Merck, -27.34‰) and glutamic acid (USGS-40, -26.24‰). The relative analytical standard deviations of concentrations were 2.9% for TOC and 2.3% for TN. The analytical standard deviation of δ13C values was 0.09‰.
Extraction and purification of fatty acids
The extraction of total lipids used a modified method by Bligh and Dyer (1959). Briefly, after quantitatively adding an internal standard (19:0, Sigma-Aldrich), total lipids were extracted from sediment samples of around 2 g by sonication in a mixture of chloroform, methanol, and 5% trichloroacetic acid in water. The extracted total lipids were saponified with alkaline methanol (3 mol L-1 KOH, pH ≥ 12) at 110°C for 2 hours (Canuel, 2001). After purification with hexane and dichloromethane (9:1, v/v), the solvent was acidified with 6 mol L-1 HCl. The fraction with fatty acids were extracted by hexane and dichloromethane (9:1, v/v). Then fatty acids were derivatized into fatty acid methyl esters (FAMEs) by heating in acidified methanol (5% HCl) at 90°C for 4 hours.
Determination of fatty acid concentrations and δ13C values
Fatty acid concentrations and δ13C values were measured following a method previously reported (Tang et al., 2017). First, individual FAMEs were separated on a column of gas chromatography (60m × 0.25mm, 0.25 µm, DB - 5MS), and identified by a quadrupole mass spectrometer (MSD, Agilent 5977B). Using the same GC program, the concentrations and δ13C of FAMEs were measured on gas chromatography - combustion - isotope ratio mass spectrometer (GC-IRMS, Trace1300 - Delta V Advantage), which combusted individual compounds into CO2 for the measurement of 13C/12C. The ramping program started at 130°C with a ramping rate of 5°C min-1 and was held at 180°C for 5 minutes. It was then ramped at 2.5°C min-1, followed by a ramping at 4°C min-1 from 230°C, and was held at 300°C for 12 mins. The baseline drifting and linearity of δ13C values relative to VPDB were calibrated with reference CO2 and n-alkane standards (B5, University of Indiana). Along with the sample analysis, a mixed fatty acid standard (Sigma-Aldrich) with known δ13C values was derivatized and measured in parallel to calibrate the concentrations of individual fatty acids as well as the δ13C of derived carbon from the methanol. The δ13C values of individual fatty acids in mixed standards were determined by EA-IRMS. The relative standard deviations of individual fatty acid concentrations varied from 2.2% to 29%, and the standard deviation of δ13C values ranged from 0.1‰ to 1.2‰.
Model analysis
(1) Decompositional reactivity by G-model
The first-order decomposition reactions of individual fatty acids were described by a G-model (Berner, 1964), which assumes that organic matter with the same molecular composition is buried at a constant rate from the upper water column and that the sedimentation rate of the studied area is 0.8 cm yr-1 as reported previously (Wei et al., 2020). Only degradable fatty acids can be fitted which is assumed as the fatty acid concentrations relative to that in the deepest depth studied (25 cm - 26 cm). In this model, fatty acid concentrations () are assumed to degrade at a rate proportional to their concentration at a given time as described in Equation 1 and Equation 2:
Here, is the concentration of a given fatty acid when the degradation time is 0, G∞ represents the final buried non-degradable fatty acids, which is equal to the concentration of this fatty acid at depth of 25cm - 26cm, and is the degradation rate constant. Transforming Equation 2 into natural logarithmic form yields Equation 3:
The slope of the regression between and sediment age, t, represents the degradation rate constant , while the intercept represents the logarithmic concentrations of this fatty acid deposited when t is 0.
(2) Kinetic isotope effect
The simplified closed-system KIE model was applied following a previous report (Mariotti et al., 1981), which was expressed as Equation 4:
Where represents the relative abundance of reactants to initial concentrations; represents the δ13C values of the reactant at any given ; represents the initial δ13C values of the reactant when is 1; represents the kinetic isotope fractionation between the product and reactant. By simplifying this equation, we can get Equation 5:
Using the δ13C of a given fatty acid at the surface 1 cm as , and dividing the concentrations of a fatty acid at a given depth by its concentration at 1 cm depth as , the δ13C of the fatty acid at depth as , then the can be fitted by Equation 5.
(3) Two-endmember mixing model
The relative contribution of marine and terrestrial input can be identified by a two-endmember mixing model as described by Equation 6 and Equation 7:
Where represents the contribution of terrestrial input to a given fatty acid with mixed origins, represents the contribution of locally produced marine phytoplankton, represents the δ13C of terrestrial origin fatty acids, and represents the measured δ13C of the given fatty acid.
Results
Distribution of total organic carbon content and δ13C values
The TOC content across the sediment record ranged from 0.43% to 0.63% (Figure 2A) with relatively small variations across the sediment core. The δ13C values of TOC ranged from -22.8‰ to -22.2‰ (-22.5 ± 0.2‰. Figure 2B), showing a characteristic marine origin as other works suggested in this region (Zhang et al., 2009; Yang et al., 2020). The TOC δ13C values exhibited an initial decrease in the top sediment, then slightly increased toward the bottom of this sediment core, suggesting the changing origins of sediment organic matter during its preservation on the NSCS shelf. The C/N ratios showed a progressive decrease from 9.50 at the surface to 10.8 at the bottom of 26 cm with an average of 10.1 ± 0.5 (Figure 2C). This is consistent with previous reports near this area (Zhang et al., 2009; He et al., 2010).
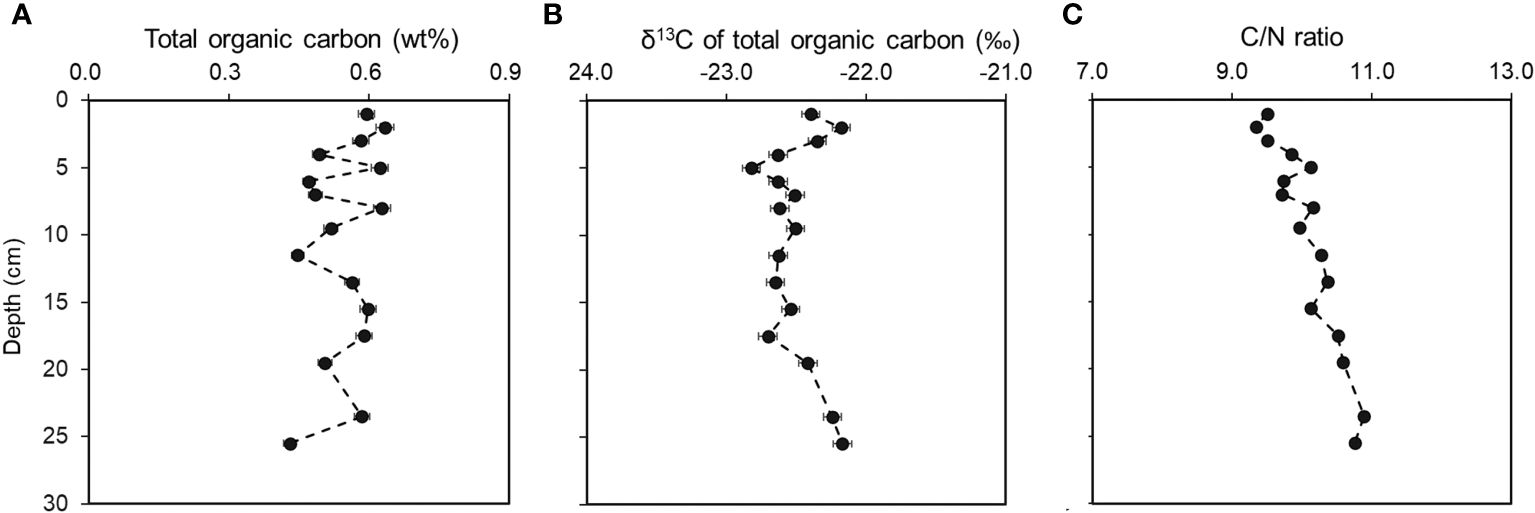
Figure 2 The vertical distributions of total organic carbon content (A), the δ13C values of total organic carbon (B) and the C/N ratios (C) with depth in sediment at P402 from the northern South China Sea in June 2021.
Distribution of fatty acid concentrations and their δ13C values
Three groups of fatty acids were identified including four short-chain fatty acids (SCFA, 14:0, 15:0, 16:0, and 18:0), two branched-chain fatty acids (BCFA, ai-15:0 and i-15:0), and three long-chain fatty acids (LCFA, 24:0, 26:0, 28:0) respectively. The total fatty acid (TFA) content decreased gradually from 66.1 µg g-1 DW at the surface to 17.5 µg g-1 DW at the bottom of 26 cm, exhibiting a rapid decline in the top sediment of 1-5 cm, followed by a slower decrease in the deeper sediment (Figure 3A). SCFAs were usually the dominant component with 16:0 as the most abundant, collectively accounting for around 20% of the total fatty acids (Figure 3B). This is consistent with previous reports of fatty acid composition in surface sediment of NSCS shelf (Hu et al., 2006) and Chesapeake Bay (Canuel and Martens, 1996). The decrease in concentrations with depth varies among individual SCFAs: the 14:0 decreased most rapidly followed by 16:0, the decrease of 18:0 is less significant in the studied sediment core. At the deeper layer below 7 cm, the concentrations of LCFAs exceeded SCFAs with relatively less decrease except for a peak of around 15.0 µg g-1 DW at the depth of 9 - 10 cm. In contrast, BCFAs accounted for a small component of less than 20% of the total fatty acids, with the highest concentrations observed at the surface layer (6.5 µg g-1 DW) followed by rapid decrease with depth to 0.9 µg g-1 DW at 26 cm.
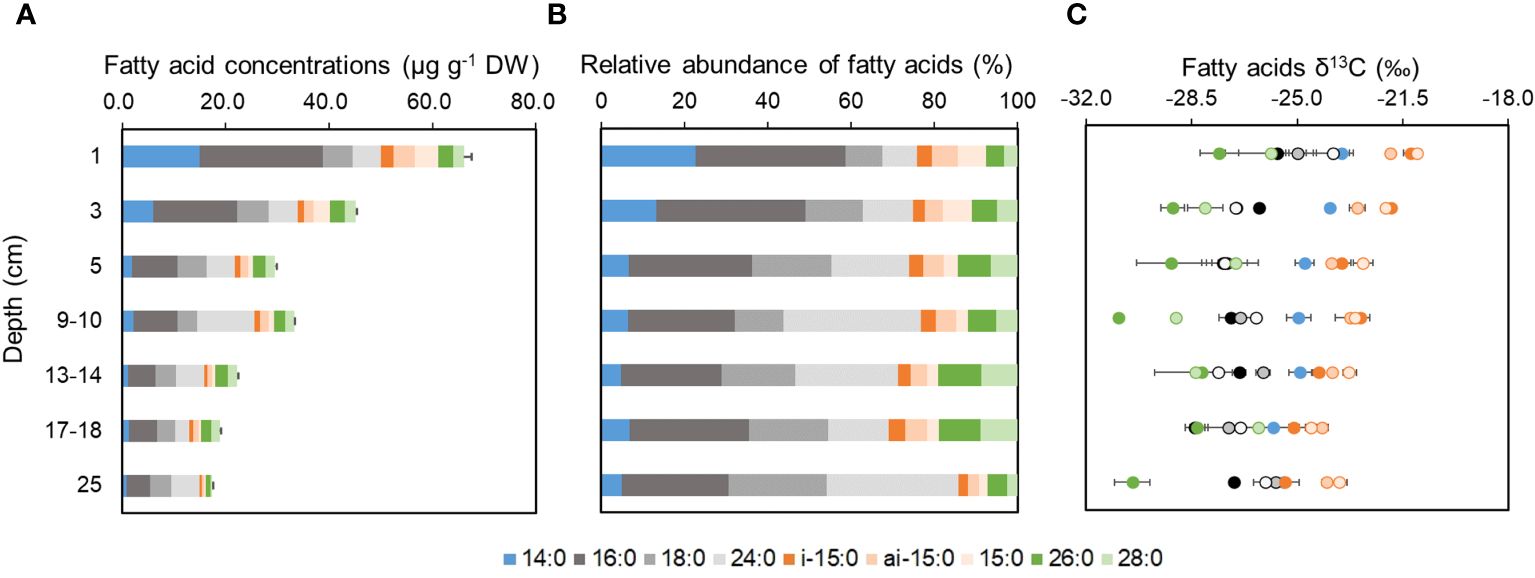
Figure 3 The vertical distributions of fatty acid concentrations (A), the relative abundance of fatty acids (B) and the δ13C values of fatty acids (C) with depth in sediment at P402 from the northern South China Sea in June 2021.
The δ13C values of individual fatty acids generally ranged from -30.9‰ to -21.0‰ (Figure 3C). The SCFA δ13C values (-28.5‰ ~ -23.5‰) were relatively more negative than BCFA (-25.1‰ ~ -21.2‰), and usually more positive than LCFA (-30.9‰ ~ -23.8‰). Exceptions can be observed in 15:0 δ13C, which is close to two BCFA. These δ13C distributions of major fatty acids were generally consistent with previous reports (Hu et al., 2006; Wei et al., 2021). Even among the three SCFAs, difference in δ13C values can be observed with enriched 13C in 14:0 compared to 16:0 and 18:0. Most fatty acids exhibited slight decrease in δ13C values in the top 5 cm. Below 5 cm, δ13C values of 14:0 and two BCFAs continued to decrease, while 16:0, 18:0 and LCFA increase slightly toward the bottom.
Discussion
Origins of fatty acids in the NSCS sediment
At the studied site, the high production of phytoplankton was found to be a major source of sediment organic matter on the shelf of NSCS (Yang et al., 2020). The organic matter from phytoplankton is characterized by high abundance of labile organic components like amino acids, pigment as well as short-chain fatty acids (Wakeham and Lee, 2019) and relatively enriched 13C due to the photosynthetic fixation of marine dissolved inorganic carbon (Bouillon et al., 2012). The terrestrial input from the adjacent Pearl River estuary also shows a substantial contribution to the NSCS sediment OM (Hu et al., 2006), which had experienced intensive decomposition before approaching the coast with relatively more refractory components from terrestrial materials like lignin, long-chain fatty acids, and humic substance. Isotopically lighter organic carbon from vascular plants was suggested to be an end-member of terrestrial OM (Hedges et al., 1997), though a wide range of δ13C was observed among different types of terrestrial organic matter (Bouillon et al., 2012; Tao et al., 2016). Moreover, the continued activity of bacteria at the sediment-water interface accumulates organic matter from bacterial cell content and detrital materials in sediment, of which the δ13C values were demonstrated to record their food source, thus the bacterial fatty acids usually show consistent δ13C values to bulk organic carbon (Bouillon and Boschker, 2006).
Looking back to the studied NSCS sediment, a span of δ13C values over 8‰ was observed among fatty acids from even the same sample, suggesting the varied contribution of diverse origins to individual fatty acids. The two LCFAs (26:0, 28:0) exhibited the most negative δ13C values, while fatty acids with fewer carbon numbers showed more positive δ13C than LCFA. The δ13C of 14:0 was relatively more positive than other fatty acids, e.g. 16:0, 18:0, and 24:0. Most positive δ13C values of up to -21.2‰ can be observed in 15:0 and two branched fatty acids, ai-15:0 and i-15:0, which suggests a shared origin of those three fatty acids from bacteria (Bouillon et al., 2012). This trend among fatty acids is different from that observed in riverbed sediment, in which short-chain fatty acids (-37‰ to -35‰) were more depleted in 13C than long-chain fatty acids (-33‰ to -31‰, Wakeham and Canuel, 2016). This discrepancy possibly results from the dominance of riverine phytoplankton (-32‰ to -27‰) in riverbed SCFA with lower δ13C values (Cloern et al., 2002).
Though the δ13C values of individual fatty acids varied slightly with depth, the isotopic difference among individual fatty acids can be continuously observed across the core. This trend together with the species-specific nature of certain fatty acids provides a reliable way to examine the contribution of diverse origins to fatty acids. Here we sort the fatty acids into single-origin fatty acids and mixed-origin fatty acids. There are three groups of single-origin fatty acids from distinct origins: Firstly, the two long-chain fatty acids 26:0 and 28:0 primarily originate from the vascular plant as a representative of terrestrial input; Secondly, the 15:0 and two branched fatty acids, ai-15:0 and i-15:0 are considered to be unique structures of bacterial cells; lastly, though potentially influenced by the bacterial contribution in the water column, the short-chain fatty acid 14:0 can be a good representative of local production by marine phytoplankton because of its rapid recycling and short resident time in the sediment. Meanwhile, mixed-origin fatty acids like 16:0, 18:0, and 24:0 are possibly from a mixture of terrestrial input and local phytoplankton production, while the quantitative contribution of bacteria can be ignored in this case due to their consumption of organic carbon from diverse origins and their recording of δ13C values of bulk organic carbon.
Reactivity of fatty acids in NSCS sediment
During the early diagenesis, sediment organic matter is subject to progressive degradation, which can be generally described by G model as a first-order decomposition reaction (Berner, 1964). Here the decomposition rate is a function of the organic carbon concentration at a given rate constant. Individual compounds exhibit varied degradation rates during the early diagenesis. Some labile compounds like amino acids, short-chain fatty acids can be more rapidly decomposed than others. Even among individual fatty acids, varied decomposition rates can be observed, which are usually lower in fatty acids with more carbon numbers (Caradec et al., 2004). Beyond the structural complexity, the depositional conditions were found to be another major factor regulating the reactivity of organic matter. Different degradation rates of the same fatty acids were observed in oxygen-replenished and -depleted sediment along the coast of Long Island Sound (Sun et al., 1997).
Comparing the three groups of fatty acids with distinct origins, terrestrial fatty acids show little changes in concentrations across the sediment (Figure 3A), while both marine and bacterial fatty acids exhibit exponential decrease with depths, suggesting faster degradation of marine and bacterial fatty acids during the deposition. Among the fatty acids with mixed origins, the exponential decrease in concentrations can also be observed in 16:0, while the decrease was much smaller in 18:0 and 24:0.
From the G model, the degradation rate constants of fatty acids in sediment ranged from 0.02 – 0.17 year-1 (Figure 4A; Table 1), which is consistent with the prediction from bulk organic carbon in the sediment core from Chesapeake Bay (0.0091~0.191 year-1 (Zimmerman and Canuel, 2000), but much smaller than those observed in lab-simulated decomposing phytoplankton ranging from 0.011 to 0.58 day-1 (Caradec et al., 2004; Ding and Sun, 2005; Lv et al., 2010). This discrepancy may be attributed to the limitation of organic matter supplied to the sediment as well as benthic processes reshaping the abundance and composition of organic matter supplied, such as bioturbation, oxygen availability, and diversified functions of microbial communities.
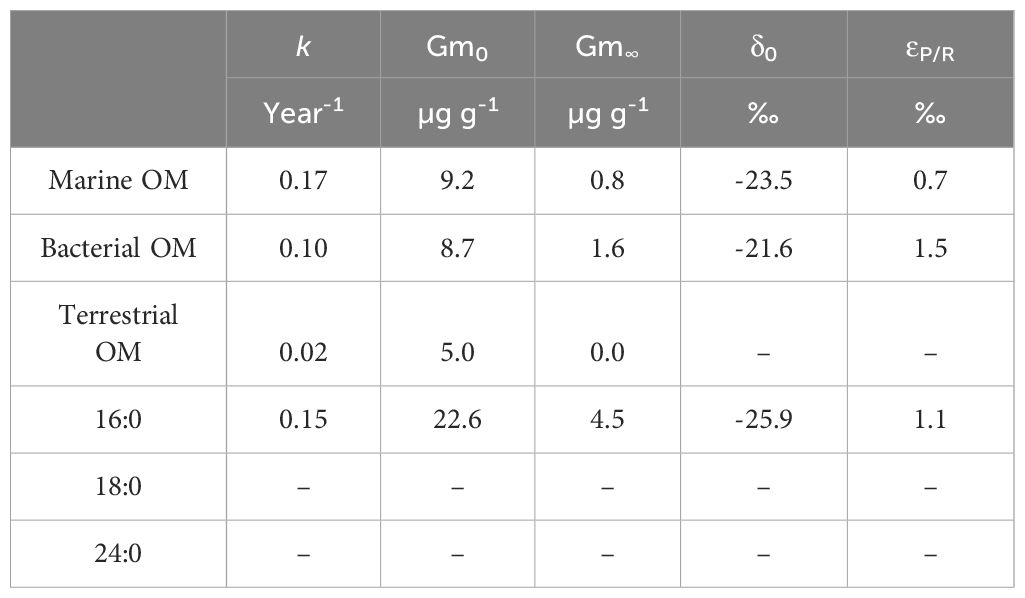
Table 1 The decomposition rate constants (k) and concentrations of initially deposited (Gm0) and undegradable (Gm∞) fatty acids to the sediment of northern South China Sea calculated from the G model; The δ13C values of initially deposited fatty acids (δ0) and the decompositional kinetic isotope fractionation (εP/R) of fatty acids in the sediment from NSCS calculated from a model with the assumption of closed-system kinetic isotope effect.
The single-origin fatty acids (marine, bacterial, and terrestrial fatty acids) and three mixed-origin fatty acids (16:0, 18:0, 24:0) show varied responses in degradation (Figure 4A). Better correlations between logarithmic concentrations and ages can be observed in short-chain fatty acids than those with more carbon numbers, e.g. 24:0 and terrestrial FA. The marine fatty acid 14:0 exhibit the highest degradation rate constant of 0.17 year-1, followed by bacterial fatty acids of 0.10 year-1. The lowest rate constant of 0.02 year-1 was observed in terrestrial fatty acids. For the fatty acids with mixed origins, the 16:0 degraded relatively faster with a rate constant of 0.15 year-1, which is close to marine and bacterial fatty acids. Whereas the decomposition of 18:0 and 24:0 were so low that the fittings with ages were under the confidence levels. The good fittings into first order degradation reactions for fatty acids from both single- and mixed- origins demonstrate that the degradation of fatty acids is independent of the origins. This finding together with the varied decompositional rate constants among fatty acids support the idea that labile compounds can be more rapidly removed regardless of their origins, so that the reactivity of individual fatty acids is more regulated by their chemical structure, while changes in origins and environmental conditions play less important roles in OM reactivity, at least in the settings of the studied environment.
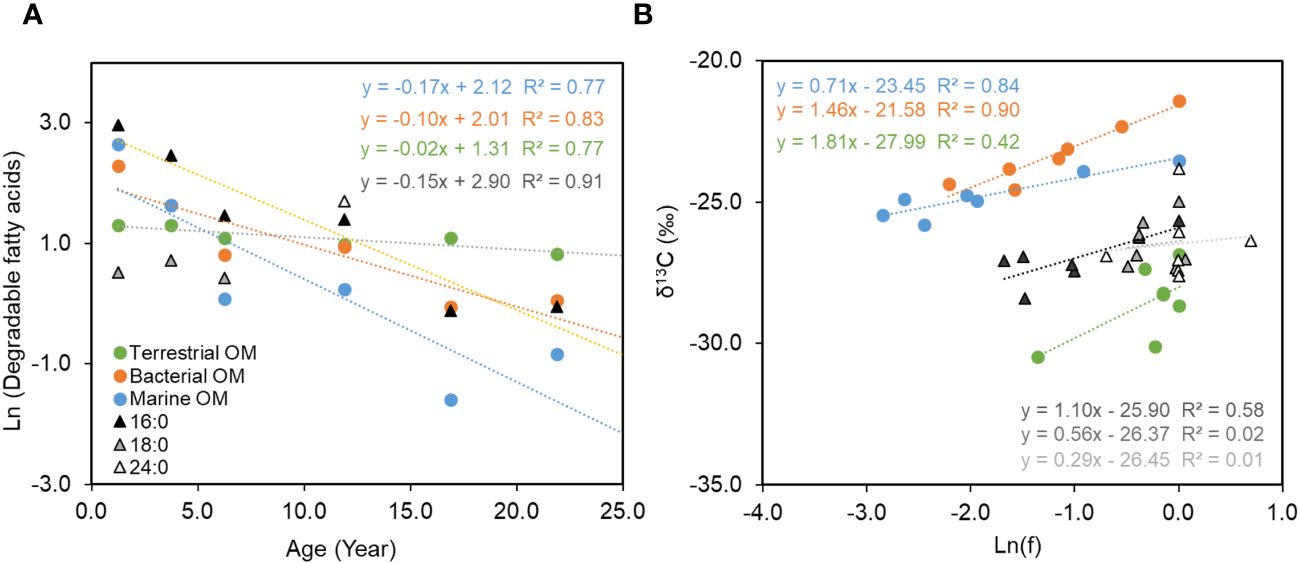
Figure 4 The linear relationship of logarithmic concentrations of fatty acids with single (marine, bacterial and terrestrial organic matter) and mixed sources (16:0, 18:0 and 24:0) verse the age of the sediment (A); the linear relationship of δ13C of fatty acids verse logarithmic ratios of remained fatty acids with single (marine, bacterial and terrestrial organic matter) and mixed sources (16:0, 18:0 and 24:0) (B).
Kinetic isotope effect of fatty acids during early diagenesis
Along with the exponential decomposition of fatty acids at varied rate constants, their δ13C values progressively decreased with depth (Figure 3C). To evaluate the kinetic isotope effect of fatty acids by decomposition, we first propose that the preserved fatty acids in sediment experienced the decompositional reactions with a kinetic isotope effect (KIE) in a closed system. At each depth, with the assumption of a constant supply of a given fatty acid, the decomposition reaction removes an amount of this fatty acid as a function of the sedimentary age. Under the kinetic isotope effect, the reactant as fatty acids were removed with a constant isotope fractionation from the pool δ13C, and then the δ13C value of the reactant remained in the sediment should have a relationship with the relative abundance of this reactant to the initial concentration as described previously (Hayes, 2001).
Linear positive relationship with ln(f) can be observed in δ13C of fatty acids with less carbon numbers like marine and bacterial fatty acids as well as 16:0 with mixed origins (Figure 4B). This relationship is less significant in terrestrial fatty acids and two other mixed-origin fatty acids, 18:0 and 24:0. The linear relationship with ln(f) demonstrates that the change of fatty acid δ13C values in sediment is primarily regulated by the closed-system KIE. Since the δ13C value of individual fatty acids is determined by both the δ13C values and the relative contribution of each origin, a dominant regulation of KIE to δ13C suggests no significant preferential removal of fatty acids from specific origins. The fatty acids with more carbon numbers experienced slower decompositions in the studied sediment, then their δ13C values are less influenced by KIE, instead more by the changes in their origins with varied δ13C end-members. This explains the less clear relationship in terrestrial fatty acids as well as 18:0 and 24:0.
Combining the evidence from the G model and the closed-system KIE, it can be concluded that sediment fatty acids, at least more labile ones with a primary regulation of decomposition, conform to the closed-system kinetic isotope effect with kinetic isotope fractionations () ranging from 0.7‰ to 1.5‰ (Figure 4B, Table 1). A positive isotope shifting of sediment organic carbon from primary production has been observed, which was considered to be a result from a combination of heterotrophic respiration and CH4 production in the sediment with an unclear influence from the change in molecular composition (Hayes, 1993). A slight enrichment in 13C was also suggested in the individual fatty acids from simulated decomposing phytoplankton debris (Sun et al., 2004). All these evidence may suggest the influence of multiple processes to decompositional KIE at varied time scales.
In addition to the varied response of individual fatty acids to decompositional KIE, the variation in values of fatty acids appear to be independent of reactivity. Though marine fatty acids show the highest degradation rate, its is relatively lower than bacterial fatty acids and 16:0. It is possibly related to the varied mechanisms that cause the isotopic effect between the product and reactant. For marine fatty acids, 14:0 originated primarily from the phytoplankton in the upper water column, its δ13C distribution is predominantly related to the decompositional removal in the sediment, while for bacterial fatty acids, a balance between in situ production by bacteria and decompositional removal should be expected in depths, giving a larger than other fatty acids.
Overall, this quantitative assessment of the effect of decomposition to δ13C values of individual compounds during the early diagenesis provides a reliable constraint of the potential variation in δ13C resulting from the decomposition.
Constrained two end-member mixing model
Using the closed-system KIE model, the by decomposition has been quantitatively assessed, it thus can be further applied to constrain the mixing model with two end-members, marine and terrestrial organic matter as Equation 6 and Equation 7:
The isotopic signature of terrestrial fatty acid end-member can be obtained from carbon weighted mean of 26:0 and 28:0 δ13C, which is less influenced by decomposition due to their recalcitrant nature as discussed above. Thus no constraints were applied for this end-member. For the δ13C of marine fatty acids, the representative fatty acid 14:0 is among the most rapidly decomposed components with an isotope fractionation of 0.7‰ during the early diagenesis. Since its δ13C distribution is primarily regulated by the decompositional KIE, the consistent end-member can be obtained as from the KIE model of 14:0 (-23.5‰, Table 1). This value is considered as the marine end member of the mixing model. Moreover, the decompositional KIE should also be considered for individual fatty acids with mixed origins: For 18:0 and 24:0, the fitting of KIE with low confidence level suggests negligible decompositional KIE of these two recalcitrant fatty acids, while it cannot be ignored for 16:0 δ13C as suggested by an of up to 1.1‰ (Table 1). We thus only constrained the δ13C of 16:0 and phytoplankton endmember with the following assumptions: first, the fatty acids 16:0, 18:0, and 24:0 have only two origins, marine and terrestrial respectively; Fatty acids from the same end-members are assumed to have the same δ13C values; Then a given fatty acids, from either endmembers, are assumed to decompose in the same fashion with a constant εP/R. With those assumptions, the relative abundance of fatty acids 16:0, 18:0, and 24:0 from terrestrial and marine origins can be obtained with and without the constraint of KIE by decomposition (Figure 5). A maximum difference of up to 22% can be observed in the relative abundance of fatty acids from marine phytoplankton.
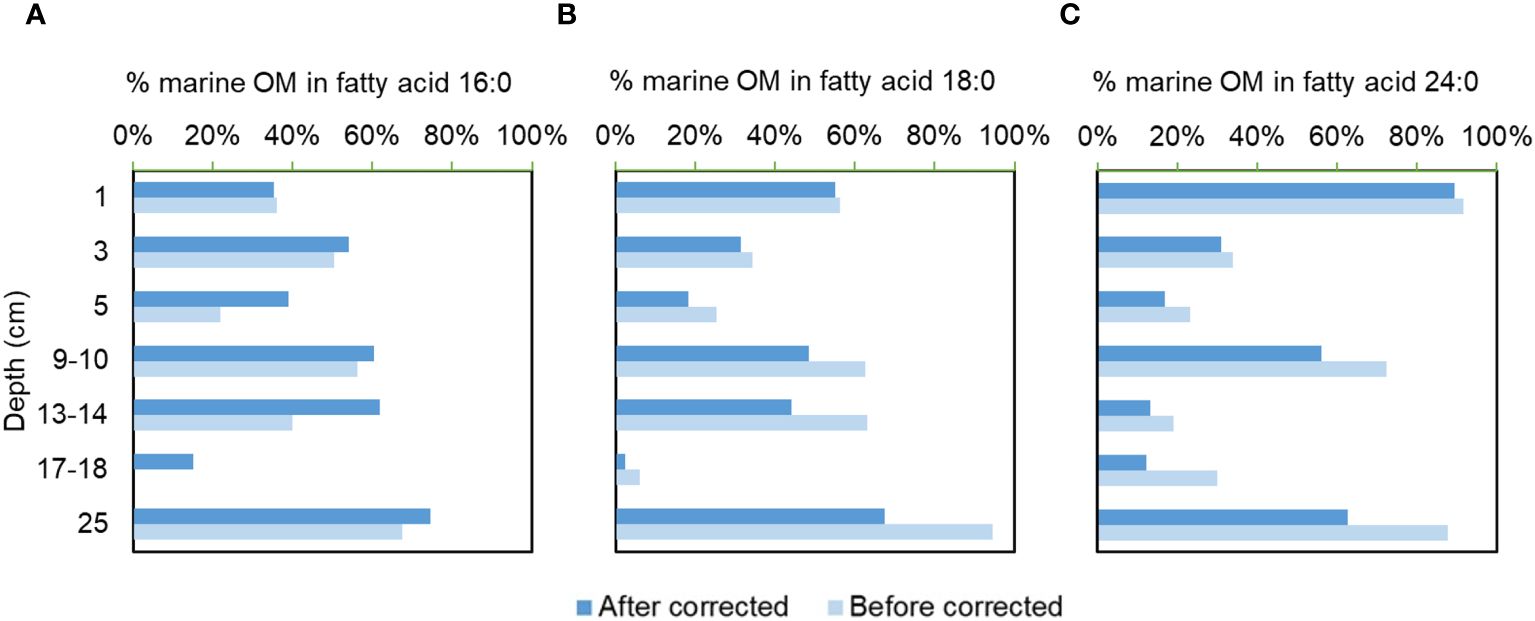
Figure 5 The relative abundance of fatty acids 16:0 (A), 18:0 (B) and 24:0 (C) from marine organic matter in sediment from NSCS based on a two end-member mixing model with and without the correction of kinetic isotope effect by the decomposition.
The three fatty acids with mixed origins, though varied in reactivity, have relatively consistent trends in the relative contribution of marine and terrestrial input with depth. The highest marine contribution to the three fatty acids appeared in the deepest sediment, which was generally decreased in shallower depth, though marine 24:0 was extraordinarily enriched in the topmost sediment. This source distribution of fatty acids indicates a decreasing contribution of local phytoplankton production to the preserved organic matter in NSCS sediment. This is contradictory to the observation a progressive elevation of coastal primary production due to enlarged nutrient input from the adjacent Pearl River Estuary (Su et al., 2017; Wei et al., 2023).
Implication to carbon cycling on the NSCS shelf
The preservation of fatty acids in NSCS sediment appears to be less influenced by the changing environmental conditions from the upper water column. The recent evidence of labile organic matter overwhelmed from local phytoplankton shows a response of coastal primary production to increased supply of nutrients from the PRE (Yang et al., 2020). However, the decreasing contribution of marine phytoplankton to preserved organic matter in sediment suggests no preferential accumulation of the over-growing phytoplankton on the shelf of NSCS. Moreover, the bulk organic carbon content and burial rate of bulk organic carbon in NSCS are generally lower than in many other coastal areas. For instance, the Sweden’s fjords contains total organic carbon of more than 2% in sediment (Watts et al., 2024), and the Chesapeake Bay has an organic carbon content of more than 1% (Zimmerman and Canuel, 2000). All those evidence pinpoints a poor preservation of organic matter on the shelf of NSCS, even though the primary production is increasing in the upper water column.
One possible reason is that the preservation of organic matter in sediment is more determined by the depositional environment, rather than the environmental conditions in the upper water column (Arzayus and Canuel, 2004; Yu et al., 2021). The strong disturbance from wind-driven coastal upwelling and flushing from freshwater discharge may enhance the remineralization of organic matter just reached the surface of the broad NSCS shelf. Thus contrasting to fjord and other coastal environments, the organic matter preservation is relatively lower on the continental shelf (Zimmerman and Canuel, 2000; Smith et al., 2015). Additionally, the threshold may exist for the low preservation of organic matter on NSCS shelf considering the long-term trend in the upper water column toward a more eutrophic and expanding hypoxic environment. Our findings of no enhanced OM preservation on NSCS implies that the elevated labile organic matter in the NSCS water column hasn’t promoted the coastal carbon sequestration yet, but rather leads to more intensified respiration in water column with a potential risk of increasing the CO2 outgassing from the coastal ocean (Dai et al., 2013).
Data availability statement
The original contributions presented in the study are included in the article/supplementary material. Further inquiries can be directed to the corresponding author.
Author contributions
TW: Conceptualization, Writing – original draft, Data curation, Formal analysis, Investigation, Methodology. CL: Methodology, Writing – original draft, Data curation. HZ: Methodology, Writing – original draft. JYL: Investigation, Writing – original draft. LW: Investigation, Writing – original draft. YL: Writing – original draft, Investigation. JL: Writing – original draft, Data curation, Formal analysis. JS: Writing – original draft, Methodology. TT: Conceptualization, Data curation, Writing – original draft, Writing – review & editing, Supervision, Validation.
Funding
The author(s) declare financial support was received for the research, authorship, and/or publication of this article. The work described in this paper was supported by National Natural Science Foundation of China (Project No. 41976035) and by the Research Grants Council of the Hong Kong Special Administrative Region, China (Project Reference Number: AoE/P-601/23-N).
Acknowledgments
We are thankful to Z.M. Lu for the CTD deployment, and X.H. Guo for DO analysis. Special thanks to the crew and captain of RV “Haike 68”. We also thank B.M. Liu, J.H. Chen, L. Tian and Y.Y. Li for their assistance in GC-IRMS, EA-IRMS and GC-MS.
Conflict of interest
The authors declare that the research was conducted in the absence of any commercial or financial relationships that could be construed as a potential conflict of interest.
Publisher’s note
All claims expressed in this article are solely those of the authors and do not necessarily represent those of their affiliated organizations, or those of the publisher, the editors and the reviewers. Any product that may be evaluated in this article, or claim that may be made by its manufacturer, is not guaranteed or endorsed by the publisher.
References
Arzayus K. M., Canuel E. A. (2004). Organic matter degradation in sediments of the York River estuary: Effects of biological vs. physical mixing. Geochim. Cosmochim. Acta 69, 455–464. doi: 10.1016/j.gca.2004.06.029
Bauer J. E., Cai W. J., Raymond P. A., Bianchi T. S., Hopkinson C. S., Regnier P. A. G. (2013). The changing carbon cycle of the coastal ocean. Nature 504, 61–70. doi: 10.1038/nature12857
Berner R. A. (1964). An idealized model of dissolved sulfate distribution in recent sediments. Geochim. Cosmochim. Acta 28, 1497–1503. doi: 10.1016/0016-7037(64)90164-4
Bianchi T. S., Bauer J. E. (2011). Particulate organic carbon cycling and transformation. Treatise Estuar. Coast. Sci. 5, 67–117. doi: 10.1016/B978-0-12-374711-2.00503-9
Bianchi T. S., Canuel E. A. (2011). Chemical biomarkers in aquatic ecosystems. Princeton Univ. Press. doi: 10.1515/9781400839100
Bligh E. G., Dyer W. J. (1959). A rapid method of total lipid extraction and purification. Can. J. Biochem. Physiol. 37, 911–917. doi: 10.1139/o59-099
Boschker H. T. S., De Brouwer J. F. C., Cappenberg T. E. (1999). The contribution of macrophyte-derived organic matter to microbial biomass in salt-marsh sediments: Stable carbon isotope analysis of microbial biomarkers. Limnol. Oceanogr. 44, 309–319. doi: 10.4319/lo.1999.44.2.0309
Bouillon S., Boschker H. T. S. (2006). Bacterial carbon sources in coastal sediments: a review based on stable isotope data of biomarkers. Biogeosciences 3, 175–185. doi: 10.5194/bg-3-175-2006
Bouillon S., Connolly R. M., Gillikin D. P. (2012). Use of stable isotopes to understand food webs and ecosystem functioning in estuaries. Treatise Estuar. Coast. Sci. 7, 143–173. doi: 10.1016/B978-0-12-374711-2.00711-7
Breitburg D., Levin L. A., Oschlies A., Grégoire M., Chavez F. P., Conley D. J., et al. (2018). Declining oxygen in the global ocean and coastal waters. Science 359, eaam7240. doi: 10.1126/science.aam7240
Canuel E. A., Cloern J. E., Ringelberg D. B., Guckert J. B., Rau G. H. (1995). Molecular and isotopic tracers used to examine sources of organic matter and its incorporation into the food webs of San Francisco Bay. Limnol. Oceanogr. 40, 67–81. doi: 10.4319/lo.1995.40.1.0067
Canuel E. A. (2001). Relations between river flow, primary production and fatty acid composition of particulate organic matter in San Francisco and Chesapeake bays: A multivariate approach. Org. Geochem. 32, 563–583. doi: 10.1016/S0146-6380(00)00195-9
Canuel E. A., Cammer S. S., McIntosh H. A., Pondell C. R. (2012). Climate change impacts on the organic carbon cycle at the land-ocean interface. Annu. Rev. Earth Planet. Sci. 40, 685–711. doi: 10.1146/annurev-earth-042711-105511
Canuel E. A., Martens C. S. (1996). Reactivity of recently deposited organic matter: Degradation of lipid compounds near the sediment-water interface. Geochim. Cosmochim. Acta 60, 1793–1806. doi: 10.1016/0016-7037(96)00045-2
Caradec S., Grossi V., Gilbert F., Guigue C., Goutx M. (2004). Influence of various redox conditions on the degradation of microalgal triacylglycerols and fatty acids in marine sediments. Org. Geochem. 35, 277–287. doi: 10.1016/j.orggeochem.2003.11.006
Chen F., Zhang L., Yang Y., Zhang D. (2008). Chemical and isotopic alteration of organic matter during early diagenesis: Evidence from the coastal area off-shore the Pearl River estuary, south China. J. Mar. Syst. 74, 372–380. doi: 10.1016/j.jmarsys.2008.02.004
Cloern J. E., Canuel E. A., Harris D. (2002). Stable carbon and nitrogen isotope composition of aquatic and terrestrial plants of the San Francisco Bay estuarine system. Limnol. Oceanogr. 47, 713–729. doi: 10.4319/lo.2002.47.3.0713
Close H. G. (2019). Compound-specific isotope geochemistry in the ocean. Ann. Rev. Mar. Sci. 11, 10.1–10.30. doi: 10.1146/annurev-marine-121916-063634
Close H. G., Shah S. R., Ingalls A. E., Diefendorf A. F., Brodie E. L., Hansman R. L., et al. (2013). Export of submicron particulate organic matter to mesopelagic depth in an oligotrophic gyre. Proc. Natl. Acad. Sci. U.S.A. 110, 12565–12570. doi: 10.1073/pnas.1217514110
Dai M., Cao Z., Guo X., Zhai W., Liu Z., Yin Z., et al. (2013). Why are some marginal seas sources of atmospheric CO2? Geophys. Res. Lett. 40, 2154–2158. doi: 10.1002/grl.50390
Ding H., Sun M. Y. (2005). Biochemical degradation of algal fatty acids in oxic and anoxic sediment-seawater interface systems: Effects of structural association and relative roles of aerobic and anaerobic bacteria. Mar. Chem. 93, 1–19. doi: 10.1016/j.marchem.2004.04.004
Eglinton G., Hamilton R. J. (1967). Leaf epicuticular waxes: The waxy outer surfaces of most plants display a wide diversity of fine structure and chemical constituents. Science 156, 1322–1335. doi: 10.1126/science.156.3780.1322
Fennel K., Testa J. M. (2019). Biogeochemical controls on coastal hypoxia. Ann. Rev. Mar. Sci. 11, 105–130. doi: 10.1146/annurev-marine-010318-095138
Han A., Dai M., Kao S. J., Gan J., Li Q., Wang L., et al. (2012). Nutrient dynamics and biological consumption in a large continental shelf system under the influence of both a river plume and coastal upwelling. Limnol. Oceanogr. 57, 486–502. doi: 10.4319/lo.2012.57.2.0486
Hayes J. M. (1993). Factors controlling 13C contents of sedimentary organic compounds: Principles and evidence. Mar. Geol. 113, 111–125. doi: 10.1016/0025-3227(93)90153-M
Hayes J. M. (2001). Fractionation of carbon and hydrogen isotopes in biosynthetic processes. Rev. Mineral. Geochem. 43, 225–277. doi: 10.2138/gsrmg.43.1.225
He B., Dai M., Huang W., Liu Q., Chen H., Xu L. (2010). Sources and accumulation of organic carbon in the Pearl River Estuary surface sediment as indicated by elemental, stable carbon isotopic, and carbohydrate compositions. Biogeosciences 7, 3343–3362. doi: 10.5194/bg-7-3343-2010
Hedges J. I., Keil R. G., Benner R. (1997). What happens to terrestrial organic matter in the ocean? Org. Geochem. 27, 195–212. doi: 10.1016/S0146-6380(97)00066-1
Hu J., Peng P., Jia G., Fang D., Zhang G., Fu J., et al. (2002). Biological markers and their carbon isotopes as an approach to the paleoenvironmental reconstruction of Nansha area, South China Sea, during the last 30 ka. Org. Geochem. 33, 1197–1204. doi: 10.1016/S0146-6380(02)00082-7
Hu J., Zhang H., Peng P. (2006). Fatty acid composition of surface sediments in the subtropical Pearl River estuary and adjacent shelf, Southern China. Estuar. Coast. Shelf. Sci. 66, 346–356. doi: 10.1016/j.ecss.2005.09.009
Kaneda T. (1991). Iso- and anteiso-fatty acids in bacteria: Biosynthesis, function, and taxonomic significance. Microbiol. Rev. 55, 288–302. doi: 10.1128/mr.55.2.288-302.1991
Kao S. J., Terence Yang J. Y., Liu K. K., Dai M., Chou W. C., Lin H. L., et al. (2012). Isotope constraints on particulate nitrogen source and dynamics in the upper water column of the oligotrophic South China Sea. Global Biogeochem. Cycles 26, GB2033. doi: 10.1029/2011GB004091
Li D., Gan J., Lu Z., Cheng W., Kung H., Li J. (2023). Hypoxia formation triggered by the organic matter from subsurface chlorophyll maximum in a large estuary-shelf system. Water Res. 240, 120063. doi: 10.1016/j.watres.2023.120063
Lv D., Song Q., Wang X. (2010). Decomposition of algal lipids in clay-enriched marine sediment under oxic and anoxic conditions. Chin. J. Oceanol. Limnol. 28, 131–143. doi: 10.1007/s00343-010-9226-8
Mariotti A., Germon J. C., Hubert Kaiser P. P., Letolle R., Tardieux A., Tardieux P. (1981). Experimental determination of nitrogen kinetic isotope fractionation: Some principles; illustration for the denitrification and nitrification processes. Plant Soil 62, 413–430. doi: 10.1007/BF02374138
Naraoka H., Ishiwatari R. (2000). Molecular and isotopic abundances of long-chain n-fatty acids in open marine sediments of the western North Pacific. Chem. Geol. 165, 23–36. doi: 10.1016/S0009-2541(99)00159-X
Shi W., Sun M. Y., Molina M., Hodson R. E. (2001). Variability in the distribution of lipid biomarkers and their molecular isotopic composition in Altamaha estuarine sediments: Implications for the relative contribution of organic matter from various sources. Org. Geochem. 32, 453–467. doi: 10.1016/S0146-6380(00)00189-3
Smith R. W., Bianchi T. S., Allison M., Savage C., Galy V. (2015). High rates of organic carbon burial in fjord sediments globally. Nat. Geosci. 8, 450–453. doi: 10.1038/ngeo2421
Su J., Dai M., He B., Wang L., Gan J., Guo X., et al. (2017). Tracing the origin of the oxygen-consuming organic matter in the hypoxic zone in a large eutrophic estuary: The lower reach of the Pearl River Estuary, China. Biogeosciences 14, 4085–4099. doi: 10.5194/bg-14-4085-2017
Sun M. Y., Wakeham S. G. (1994). Molecular evidence for degradation and preservation of organic matter in the anoxic Black Sea Basin. Geochim. Cosmochim. Acta 58, 3395–3406. doi: 10.1016/0016-7037(94)90094-9
Sun M. Y., Wakeham S. G., Lee C. (1997). Rates and mechanisms of fatty acid degradation in oxic and anoxic coastal marine sediments of Long Island Sound, New York, USA. Geochim. Cosmochim. Acta 61, 341–355. doi: 10.1016/S0016-7037(96)00315-8
Sun M. Y., Zou L., Dai J., Ding H., Culp R. A., Scranton M. I. (2004). Molecular carbon isotopic fractionation of algal lipids during decomposition in natural oxic and anoxic seawaters. Org. Geochem. 35, 895–908. doi: 10.1016/j.orggeochem.2004.04.001
Tang T., Mohr W., Sattin S. R., Rogers D. R., Girguis P. R., Pearson A. (2017). Geochemically distinct carbon isotope distributions in Allochromatium vinosum DSM 180T grown photoautotrophically and photoheterotrophically. Geobiology 15, 324–339. doi: 10.1111/gbi.12221
Tao S., Eglinton T. I., Montluçon D. B., McIntyre C., Zhao M. (2016). Diverse origins and pre-depositional histories of organic matter in contemporary Chinese marginal sea sediments. Geochim. Cosmochim. Acta 191, 70–88. doi: 10.1016/j.gca.2016.07.019
Volkman J. K. (1986). A review of sterol markers for marine and terrigenous organic matter. Org. Geochem. 9, 83–99. doi: 10.1016/0146-6380(86)90089-6
Volkman J. K., Barrett S. M., Blackburn S. I., Mansour M. P., Sikes E. L., Gelin F. (1998). Microalgal biomarkers: A review of recent research developments. Org. Geochem. 29, 1163–1179. doi: 10.1016/S0146-6380(98)00062-X
Wakeham S. G., Canuel E. A. (2016). The nature of organic carbon in density-fractionated sediments in the Sacramento-San Joaquin River Delta (California). Biogeosciences 13, 567–582. doi: 10.5194/bg-13-567-2016
Wakeham S. G., Lee C. (2019). Limits of our knowledge, part 2: Selected frontiers in marine organic biogeochemistry. Mar. Chem. 212, 16–46. doi: 10.1016/j.marchem.2019.02.005
Wakeham S. G., Lee C., Hedges J. I., Hernes P. J., Peterson M. L. (1997). Molecular indicators of diagenetic status in marine organic matter. Geochim. Cosmochim. Acta 61, 5363–5369. doi: 10.1016/S0016-7037(97)00312-8
Watts E. G., Hylén A., Hall P. O. J., Eriksson M., Robertson E. K., Kenney W. F., et al. (2024). Burial of organic carbon in Swedish fjord sediments: Highlighting the importance of sediment accumulation rate in relation to fjord redox conditions. J. Geophys. Res. Biogeosci. 129, 1–22. doi: 10.1029/2023JG007978
Wei B., Mollenhauer G., Hefter J., Grotheer H., Jia G. (2020). Dispersal and aging of terrigenous organic matter in the Pearl River Estuary and the northern South China Sea Shelf. Geochim. Cosmochim. Acta 282, 324–339. doi: 10.1016/j.gca.2020.04.032
Wei B., Mollenhauer G., Hefter J., Kusch S., Grotheer H., Schefuß E., et al. (2021). The nature, timescale, and efficiency of riverine export of terrestrial organic carbon in the (sub)tropics: Insights at the molecular level from the Pearl River and adjacent coastal sea. Earth. Planet. Sci. Lett. 565, 116934. doi: 10.1016/j.epsl.2021.116934
Wei B., Mollenhauer G., Kusch S., Hefter J., Grotheer H., Schefuß E., et al. (2023). Anthropogenic perturbations change the quality and quantity of terrestrial carbon flux to the coastal ocean. J. Geophys. Res. Biogeosci. 128, e2023JG007482. doi: 10.1029/2023JG007482
Yang Z., Zhang H., Kang P., Zhao Y., Tang T. (2020). Influence of algal production and decomposition on the carbon isotope signature of labile particulate organic matter on a productive continental shelf under the stress of coastal hypoxia. Front. Mar. Sci. 7. doi: 10.3389/fmars.2020.00675
Yu M., Eglinton T. I., Haghipour N., Montluçon D. B., Wacker L., Hou P., et al. (2021). Contrasting fates of terrestrial organic carbon pools in marginal sea sediments. Geochim. Cosmochim. Acta 309, 16–30. doi: 10.1016/j.gca.2021.06.018
Zhang L., Yin K., Wang L., Chen F., Zhang D., Yang Y. (2009). The sources and accumulation rate of sedimentary organic matter in the Pearl River Estuary and adjacent coastal area, Southern China. Estuar. Coast. Shelf. Sci. 85, 190–196. doi: 10.1016/j.ecss.2009.07.035
Keywords: fatty acids, compound specific isotope analysis, decomposition, coastal carbon sequestration, South China Sea, sediment
Citation: Wang T, Lin C, Zhang H, Li J, Wang L, Luo Y, Liu J, Shen J and Tang T (2024) Kinetic isotope effect of decomposing fatty acids in the continental shelf sediment of the northern South China Sea. Front. Mar. Sci. 11:1438092. doi: 10.3389/fmars.2024.1438092
Received: 25 May 2024; Accepted: 19 June 2024;
Published: 05 July 2024.
Edited by:
Guangchao Zhuang, Ocean University of China, ChinaReviewed by:
Xinxin Li, Southern University of Science and Technology, ChinaWeichao Wu, Shanghai Ocean University, China
Copyright © 2024 Wang, Lin, Zhang, Li, Wang, Luo, Liu, Shen and Tang. This is an open-access article distributed under the terms of the Creative Commons Attribution License (CC BY). The use, distribution or reproduction in other forums is permitted, provided the original author(s) and the copyright owner(s) are credited and that the original publication in this journal is cited, in accordance with accepted academic practice. No use, distribution or reproduction is permitted which does not comply with these terms.
*Correspondence: Tiantian Tang, dGlhbnRpYW4udGFuZ0B4bXUuZWR1LmNu