- Middle East Technical University, Institute of Marine Sciences, Erdemli, Türkiye
Advection of pelagic fish larvae through ocean currents is a source of dispersal that is known to structure marine populations by directly impacting population success and persistence. To be able to understand fish population dynamics and manage existing fisheries it is therefore of importance to assess the extent of exchange between populations and determine the factors that drive it, which is still a challenge in fisheries research today. In this study, the output of a high-resolution basin-scale circulation model is used to calculate the dispersal probability from spawning areas and the remaining Black Sea for a range of commercially important fish such as anchovy, sprat, turbot, red mullet, and bluefish under different environmental conditions. The common trait of many commercially important species in the Black Sea is that they have pelagic larval stages of different time scales which are simulated by tracking virtual drifters in the simulated surface circulation of the Black Sea. Simulation results show that the dynamic current structure of the Black Sea causes low local retention of larvae. During spring and summer lower offshore transport and higher local retention is observed than in fall and winter, which is directly related to the Rim Current strength. The lowest offshore transport is observed on the northern northwestern shelf, the main spawning area for pelagic fish with still 24-32% of larvae transported offshore. Simulations show that pelagic fish species such as anchovy and sprat adopted different reproductive strategies through their species-specific traits. For the summer spawning species anchovy, timing, and location of spawning together with its short pelagic larval stage is shown to enhance retention on the northwestern shelf. Sprat with its long pelagic larval stage and winter spawning is more adapted to high offshore transport, making it unlikely that sprat is recruited to the same area as where it is spawned. This modeling framework provides a basis for investigating recruitment variability of pelagic fish species in the Black Sea specifically considering the impact of climate variability and provides a useful guide to the potential connectivity of marine populations or the spread of invasive pests in the Black Sea.
1 Introduction
The dispersal of pelagic larvae in marine environments is one mechanism of connecting fish populations across large distances and structuring their population success and persistence. Ocean currents and behavioral patterns can affect the supply of larvae and hence determine the distribution and abundance of many marine species. It is mostly the planktonic stages that are affected by such ocean transport at a time when directional swimming is not yet developed. The importance of such transport has been shown in several studies (Cowen et al., 2006; Condie et al., 2011; Wood et al., 2014; Torri et al., 2018; Legrand et al., 2019; Patti et al., 2020) and is of importance to be able to understand population dynamics as well as manage fisheries today (Rossi et al., 2014; Dubois et al., 2016; Di Stefano et al., 2022). In addition, it has been increasingly recognized that general characterizations of marine connectivity via currents are of importance to interpret marine biogeographic distributions (Hamilton et al., 2010), assess risks of invasive species or contaminants (Jaspers et al., 2018; Johnston & Purkis, 2011; Johnston & Purkis, 2014), and help with the establishment and management of marine protected areas (Cowen et al., 2006; Lester et al., 2009; Moffitt et al., 2009; Moffitt et al., 2011; Clavel-Henry et al., 2024).
The Black Sea hosts a unique ecosystem that has undergone many transformations over the last 50 years and supports diverse multi-species fisheries dominated in recent decades by small pelagics (Scientific, Technical and Economic Committee for Fisheries (STECF), 2017). The general circulation is characterized by the Rim Current, which is a permanent, basin-wide cyclonic circulation situated over the continental slope zone (Oguz and Besiktepe, 1999). Currents associated with the Rim Current move at speeds of well exceeding 50 cm at the surface, decreasing to 10-20 cm/s between 150-300m depth (Oguz et al., 1993; Oguz and Besiktepe, 1999) and a series of quasi-permanent anticyclonic eddies and meanders located between the coast and the Rim Current (Blokhina and Afanasyev, 2003; Korotaev, 2003; Oguz et al., 2005; Capet et al., 2012). The interior of the basin is composed of two or more interconnected cyclonic gyres referred to as the western and eastern gyres. Apart from the anticyclonic eddies, transient mesoscale activities in the form of meanders, ubiquitous mesoscale eddies, dipoles and filaments are observed on both sides of the Rim Current (Blokhina and Afanasyev, 2003; Korotaev, 2003). These meanders and across-stream filaments or offshore jets may serve as drivers of exchange between the coastal region and the interior of the Black Sea (Oguz, 2017; Akpınar, 2024).
The most important fishery in the Black Sea is anchovy (Engraulis encrasicolus) with total landings of 161,584 tons in 2016 while sprat is the second most important commercial fish with total landings of 80,018 tons in 2016 (Scientific, Technical and Economic Committee for Fisheries (STECF), 2017). Following these species, red mullet (Mullus barbatus), turbot (Scophthalmus maeoticus), Bluefish (Pomatomus saltatrix) and horse mackerel (Trachurus mediterraneus) are of importance. All of these fish species are known to spawn preferably in shelf areas of the Black Sea (Figure 1) but differ significantly in their life history traits such as their spawning times (spring to winter) and pelagic larval stages (20 - 70 days), which are considered in this study.
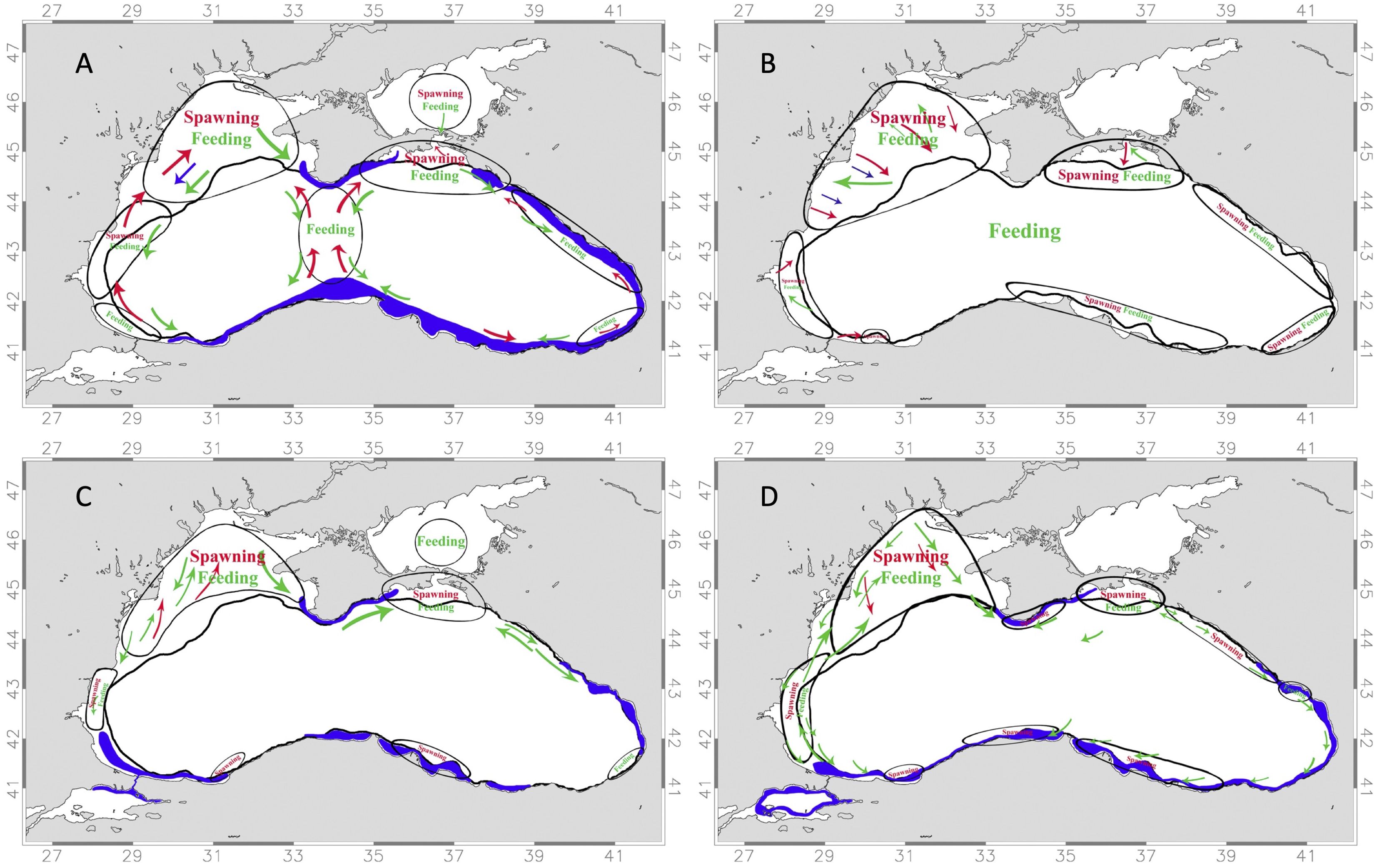
Figure 1. Maps of the Black Sea showing the spawning (red), feeding (green), and overwintering regions (blue) of different pelagic fish species considered in this study: (A) Black Sea anchovy (Engraulis encrasicolus ponticus) (B) Sprat (Sprattus sprattus) (C) Red mullet (Mullus barbatus) (D) Horse mackerel (Trachurus mediterraneus ponticus). Maps drawn based on information from the STECF Report (Scientific, Technical and Economic Committee for Fisheries (STECF), 2017).
One aspect of larval transport is the cross-shelf, onshore-offshore transport that determines if species are transported away from spawning sites and/or nursery grounds and how fast. Because commercially important fishes in the Black Sea most spawn on the northwestern shelf and other shelf areas (Figure 1), which are also the main feeding grounds for these fish (Ivanov and Beverton, 1985; Daskalov, 2003; Scientific, Technical and Economic Committee for Fisheries (STECF), 2017) cross-shelf transport is an important factor influencing larval survival. Hence offshore transport as well as local retention are the two key processes that may influence recruitment success of these fish species while onshore transport with currents may carry larvae inshore to nursery regions.
While there have been many studies that aimed on quantifying transport and connectivity patterns of larvae in different parts of the world oceans such as the Mediterranean Sea (Rossi et al., 2014; Torri et al., 2018; Legrand et al., 2019; Patti et al., 2020; Di Stefano et al., 2022), North Sea (Bolle et al., 2009; Erftemeijer et al., 2009; Romagnoni et al., 2020) or Australia (Condie et al., 2011, 2021; Condie, 2022; Roughan et al., 2011) to name only a few, to date only three studies have focused on the Black Sea connectivity (Fach, 2014; Ozturk et al., 2017; Guraslan et al., 2017). Initially, Fach (2014) tested the possibility of large-scale transport of anchovy larvae via surface currents computed from satellite data and found that the Rim Current is the main facilitator of basin-wide transport. Guraslan et al. (2017) then modeled anchovy overwintering migration in detail, while Ozturk et al. (2017) considered the large-scale connectivity between different regions of the Black Sea to help define suitable areas for the establishment of Marine Protective Areas. However, these Black Sea studies have focused on the transport and migration of only anchovy. It is however necessary to look at specific mechanisms that may facilitate transport or retention and also look at a much broader range of fish species that are of importance commercially.
In this study, an analysis of general connectivity in the Black Sea based on Lagrangian drifter pathways is presented that focuses on across-shore transport probabilities and local retention. These two characteristics are chosen to represent connectivity because the cross-shelf transport determines if larvae are transported away from the spawning sites that are in the case of the Black Sea also nursery areas, to more or less favorable habitats, and recruitment success is often influenced by retention (Almany et al., 2007; Condie et al., 2011; Chashchin et al., 2015; Monroy et al., 2017). Six pelagic fish species that are of commercial importance are identified and their life history traits, pelagic larval duration and spawning times, are considered. The study aims to characterize the seasonal and interannual variability of cross-shelf transport and local retention in the Black Sea in relation to the circulation patterns of the Black Sea. Further, the study aims to understand how currents associated with the Rim Current can facilitate cross-shelf transport or retention through mesoscale features in different regions and how these circulation features impact pelagic fish with different life traits and with it the recruitment potential of exploitable fish resources.
2 Methods
In this study, a hydrodynamic model is used to provide Black Sea surface velocity fields for the years 2001 to 2010 and Lagrangian particles are released in it and advected over time. The model used to generate an eddy-resolving velocity field of the Black Sea is the Stony Brook Parallel Ocean Model (sbPOM) (Jordi and Wang, 2012) based on the Princeton Ocean Model using the Mellor-Yamada level 2.5 turbulence parameterization (POM; Blumberg and Mellor, 1987). The model domain encompasses the entire Black Sea with a 5x5 km horizontal grid and a 35 level, sigma-coordinate, vertical grid. The model was forced using the atmospheric fields generated by the HIRHAM5 model of Danish Meteorological Institute (DMI) (Christensen et al., 2007) and river discharge rates obtained from Ludwig et al. (2009) and the Black Sea Commission’s database (http://www.blacksea-commission.org/Inf.%20and%20Resources/Data%20Links/). The model was initialized using World Ocean Atlas fields (WOA; Locarnini et al., 2013; Zweng et al., 2013), spun up for five years and then a simulation was performed for the period 1990-2010. The model results have been validated in Salihoglu et al. (2017) against available data from the SeaDataCloud Temperature and Salinity Historical Data Collection for the Black Sea V2 (https://sextant.ifremer.fr/geonetwork/srv/api/records/b90d7650-a0d7-409c-ba31-122a1f4f9c3f) and satellite derived circulation fields using the AVISO+ (Archiving, Validation and Interpretation of Satellite Oceanographic data) Sea Level Anomalies (SLA) regional product for the Black Sea. (http://www.aviso.altimetry.fr/en/data/products/sea-surface-height-products/regional/msla-black-sea.html). Model data comparisons showed that the model can reasonably well resolve the Black Sea circulation, including Rim Current dynamics and anti-cyclonic eddies, as well as interannual variability in temperature and salinity (Allen et al., 2014; Salihoglu et al., 2017).
2.1 Lagrangian particle tracking
Passive Lagrangian drifters were released four times a year (January, April, July and October) at 1 km intervals in surface circulation fields at 10 m depth (410478 drifters per simulation) during the years 2000 to 2010. Thereby it is assumed that larvae are homogeneously distributed in the surface layer. Drifters were moved individually at 1-hour intervals according to the locally interpolated current velocity. This choice is representing the surface mixed layer in which larvae are assumed to be homogeneously distributed. Neglecting vertical movements of larvae situated in the surface layer is a reasonable assumption here because most particles remain in the selected layer over short timescales (≤ 2 months) since horizontal velocities are several orders of magnitude higher than vertical ones (d’Ovidio et al., 2004).
Drifters were advected using a first-order accurate Lagrangian particle tracking scheme in which the 2D velocity field is interpolated to the particles position, which then carries the particle to a new position with each time step. However, processes smaller than the grid-spacing of the model cannot be resolved by such velocity fields and hence need to be added with a numerical technique simulating particle dispersion by sub-grid scale turbulence. In this study dispersion is superimposed on the advection of particles using a random walk process described and tested in detail in Xue et al. (2008) and Roughan et al. (2011) that scales the turbulent diffusion terms with a Gaussian random process that has a mean of 0 and a standard deviation of 1 between subsequent random events, resulting in a diffusivity coefficient of ca. 5*105 cm2s-1.
2.2 Trait-based parameters
In this study, pelagic fish species are not resolved individually in detail, but rather characteristic features of their life (traits) are modelled. Here two of the most important traits when investigating the ecological consequences of dispersal and connectivity are considered, which is the 1. release time, dependent on the spawning time of each species, and 2. pelagic larval duration (PLD), the length of time larvae of different species stays in the water column. Hence, larval dispersal in this study is dependent on duration of larvae in the surface water, the timing and location of spawning and the seasonal and interannual variation in oceanic circulation. We focus on six different fish species with different pelagic larval phases to represent a wide variety of commercially and/or ecologically important species in the Black Sea. These focus species are (see also Table 1):
● Anchovy (Engraulis encrasicolus ponticus): Spawning occurs most efficiently from June to August in water temperatures higher than 20°C (Niermann et al., 1994; Lisovenko and Andrianov, 1996; Sorokin, 2002). Pelagic larval duration is approximately 36 days, the typical time period of anchovy development into juveniles (Dulcic, 1997). Dominates ichtyoplankton in central south Black Sea in summer (Satilmis et al., 2003; Satilmis et al., 2014). Spawning takes place on the northwestern shelf in summer and anchovy overwinter in warmer coastal areas in the winter time, mainly along the Turkish coast (Chashchin et al., 2015; Gucu et al., 2016) (see Figure 1A). Anchovy is the most important commercial fish with total landings of 161,584 tons in 2016 (Scientific, Technical and Economic Committee for Fisheries (STECF), 2017).
● Sprat (Sprattus sprattus): Sprat have been observed to spawn in the Black Sea from autumn to spring (Satilmis et al., 2003; Satilmis et al., 2014), but peak spawning is between November and March (Ivanov and Beverton, 1985). Sprat spawn in surface waters of inshore areas throughout the Black Sea basin until ~100 km offshore, especially in river deltas and in the northwestern shelf area (Ivanov and Beverton, 1985) (see Figure 1B). Pelagic larval period until metamorphosis lasts ca. 70 days (Ojaveer, 1981; Houde, 1989). Sprat is the second most important commercial fish with total landings of 80,018 tons in 2016 (Scientific, Technical and Economic Committee for Fisheries (STECF), 2017) and dominate ichtyoplankton during fall, winter, and spring (Satilmis et al., 2003; Satilmis et al., 2014).
● Red mullet (Mullus barbatus ponticus): Spawning takes place in the Black Sea from May on mainly on the northwestern shelf and further selected shelf areas (Satilmis et al., 2003) (Figure 1C). Larvae stay pelagic for about 28-35 days in the Mediterranean (Macpherson and Raventós, 2006 and Galarza et al., 2009). According to Sabatés and Palomera (1987), larvae are found strictly in surface waters mainly between June and July in the Mediterranean Sea.
● Bluefish (Pomatomus saltatrix): This species has been observed to spawn in the Black Sea during spring (Satilmis et al., 2003) and summer (Satlimis et al., 2014; Ceyhan et al., 2007; Gordina and Klimova, 1996). Hare and Cowen (1997) found their pelagic larval duration to be on average 21.21 days (18-25 days). This species undergoes spawning migration to the Black Sea from the Mediterranean via the Aegean Sea in spring and then migrate south to return in early autumn. Bluefish are found all along the Turkish coast as well as on the Ukrainian and Bulgarian coasts.
● Turbot (Scophthalmus maeoticus): Spawning of this species has been observed from spring to summer (Satlimis et al., 2014) and their pelagic larval stage lasts a minimum of 4 weeks (Giragosov and Khanaychenko, 2012). Turbot is distributed all over the Black Sea continental shelf from 1 m depths to about 100 -110m and larvae are distributed in the surface waters (Giragosov and Khanaychenko, 2012).
● Horse mackerel (Trachurus mediterraneus ponticus): The Black Sea horse mackerel (Trachurus mediterraneus ponticus) exists as a subspecies of the Mediterranean horse mackerel (Trachurus mediterraneus) (Scientific, Technical and Economic Committee for Fisheries (STECF), 2017). This species is found in the entire Black Sea coastal regions and is known to spawn during summer in coastal areas mostly near the northwestern shelf and the Kerch Strait (Satilmis et al., 2003; Satilmis et al., 2014; Scientific, Technical and Economic Committee for Fisheries (STECF), 2017) and travels to the Crimean coast and the Marmara Sea for wintering (see Figure 1D). Larvae have a pelagic larval duration of ca. 25days.
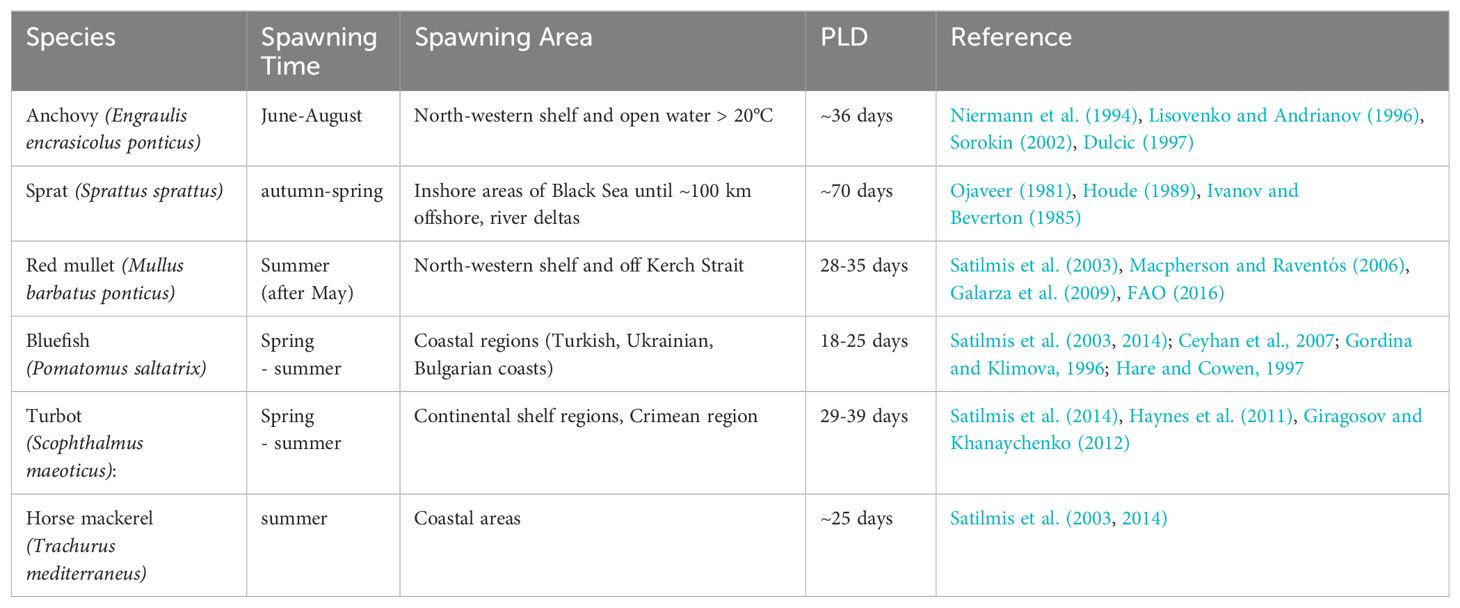
Table 1. Important commercial fish species used as target species in this study and their pelagic larval durations (PLD) in the Black Sea.
Black Sea spawning locations and nursery areas for the above listed species are defined as mainly the northwestern shelf region, but also other shelf areas around the Black Sea (Figure 1). To cover the traits of these species, simulations of winter, spring, summer and falls spawning times (1st of January, 1st of April, 1st of July, and 1st of October) as well as three different PLD times (20, 35, and 70 days) for the years 2001 to 2010 were chosen. At each spawning time and in each year a total of 410478 drifters at 1km spacing covering the entire Black Sea were released. The Lagrangian model moved particles individually at 1-hour intervals along the locally interpolated velocity fields combined with sub-grid scale processes and tracked for a total time of 90 days.
2.3 Statistical analysis
The connectivity in the Black Sea was evaluated via statistical analyses of the simulated particles trajectories acquired in the runs described above. To facilitate the statistical analysis, the Black Sea was divided into geographical subareas or grids the size of 10x10km. Different types of probability were calculated that represent measures of retention and across-shelf transport in the entire Black Sea:
2.3.1 Probability of cross-shelf transport
The probability of particles originating anywhere in the Black Sea to be transported into waters shallower or deeper than a specified depth (D) within a specified timeframe (T) corresponding to respective PLDs were calculated. This is a measure of the number of larvae (N) in a geographic grid of 10x10km that may get transported off-shelf (or on-shelf) from their respective spawning sites during their pelagic larval duration times. The specified depth (D) was chosen to be 200m as this is a good indicator of the edge of the northwestern shelf and translates into Equation 1 for offshore transport:
where t0 is the initial day of the calculation and Ni is the total number of released particles from grid cell i
2.3.2 Relative local retention of the particles in the Black Sea
The probability of local retention (LR) was computed separately from the above calculations as the number of particles released (Ninitialized) in a 10 x 10 km grid at the start of the simulation and remained (Nretained) within an area nine times as large (30 x 30 km) after each dispersal time (T). This dispersal time is directly related to the pelagic larval duration of the pelagic fish species of interest to this study (see Table 1).
This method was chosen because it provides a consistent relative measure of the percentage of larvae that remain close to their spawning areas within the pelagic larval duration period and is a widely used concept in larval transport studies of different seas (Condie et al., 2011; Rossi et al., 2014; Dubois et al., 2016; Monroy et al., 2017; Patti et al., 2020) because it is useful to identify the proportion of local larval production that is retained on a site (Carson et al., 2011; Condie et al., 2011; Monroy et al., 2017). The choice of initial release area to retention area was chosen with respect to the baroclinic Rossby Radius of deformation, which is 20-30km in the Black Sea (Oguz et al., 1994; Besiktepe et al., 2001). A choice of 10km grid cells was also large enough to have statistically significant numbers of particles in each grid cell (100 particles).
2.3.3 Particle dispersal percentage from the northwestern shelf
The dispersal percentage (DP) is quantified by dividing the number of particles ending in different model grid cells (Ngrid i) after each dispersal time (T) by the total number of particles released in the spawning area at the beginning of each simulation. This dispersal time is directly related to the pelagic larval duration of the pelagic fish species of interest to this study (see Table 1).
To reduce the uncertainties associated with the dispersal calculation and to account for the fact that many of the commercial marine fishes are multiple spawners, the cross-shelf transport (or dispersal) probability and local retention probability, was calculated based on a larger number of drifters created by an ensemble run. This means that not only the probability of 410478 drifters released at the beginning of each season and year were considered, but the probability distribution of all drifters during the entire simulation time (90 days) were calculated from day 1 of the simulation to day T, then from day 2 to day T+1, etc. until reaching the last day of each simulation, thereby creating an ensemble of probabilities (Condie et al., 2011). These probabilities were further averaged to give the probability distribution of each season (averaged over the 90-day period), each year (averaged over 365 days), or over the entire time (averaged across all years) or subsets thereof. That means for the simulation with 35-day PLD a total of 56 ensembles were averaged to calculate the probability distribution for that season instead of just one simulation.
3 Results
The circulation of the Black Sea is mainly driven by the wind stress curl and modulated by the steep bottom topography at the shelf edge in addition to seasonal variation of the surface thermohaline fluxes (Kubryakov et al., 2016). These factors form an intense, narrow Rim Current flowing along the shelf edge and a number of anticyclonic eddies along the coast (Oguz et al., 1993; Korotaev, 2003; Zatsepin, 2003) that is reproduced very well by the circulation model. The mean simulated circulation field in the Black Sea over the 10-year simulation shows the mean location of the major current of the Black Sea, the Rim Current, and the coastal anticyclonic eddies associated with its flow (Figure 2). Clearly visible is the bifurcation of the Rim Current on the northwestern shelf where the Rim Current divides into two branches, and the Sevastapol, Danube, Constanta and Kaliakra eddies as well as the Sakarya, Sinop, and Kizilirmak eddies. The seasonal variation of the Rim Current strength is reproduced by the model with the Rim Current weakening in spring and summer (Figures 2B, C) as a result of reduced wind forcing (Besiktepe et al., 2001) and a strengthening of the Rim Current in fall and winter (Figures 2A, D) related directly to increased wind stress curl (Korotaev et al., 2001; Kara et al., 2005). As discussed in Salihoglu et al. (2017) the model achieves high model skill for the physical variables, T and S. However, the Rim Current in the model is slightly faster in speed than the Rim Current derived from satellite data and there is a discrepancy between model and observations on the northern part of the shelf where the modelled currents flow northward and eastward near the northwestern coast instead of flowing south and west as in the satellite derived fields. This was found to be due to a discrepancy between the observed and modeled wind fields in the area and leads to an increase in model uncertainty on the northwestern shelf.
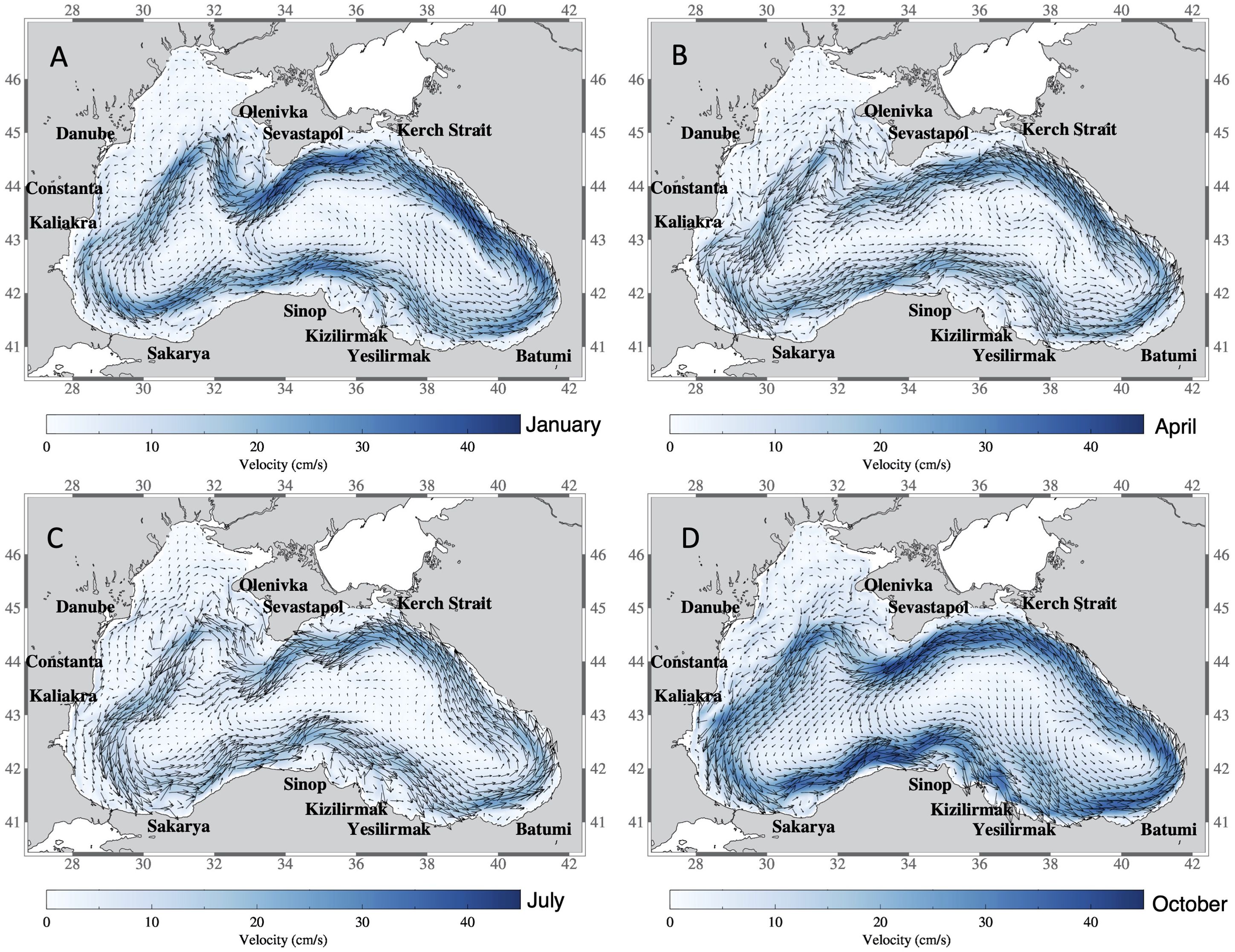
Figure 2. Simulated mean flow field of the model averaged over10 years (2001-2010) for (A) January, (B) April, (C) July and (D) October.
The results of the connectivity study are presented here as maps detailing the probability of cross-shore transport depending on the dispersal time (T), which is identical to the pelagic larval durations (PLD), the season and year. In addition, local retention maps are presented to elucidate the possibility for larvae to be retained in the local spawning area at different spawning times.
3.1 Mean cross-shelf transport
The maps of 10-year mean offshore transport show that fish larvae with short transport times of 20 days may face small transport probabilities on the shelf regions of the northwestern shelf and elsewhere (Figure 3A). There are however distinct exceptions, such as a considerable probability (>0.3, which translates to > 30%) of being transported offshore from areas on the continental shelf at the Kerch Strait region that are up to 40 km away from the shelf break at 200 m, as well as the northwestern shelf up to 80 km inland on in the Sevastopol/Crimea region (Figure 3A). This distance increases to up to 100 km on the northwestern shelf for transport times of 35 days (Figure 3B) and 120-140 km for simulations with 70-day transport times (Figure 3C). This suggests that the current dynamics at the shelf break downstream of Crimea is of importance for the offshore transport of larvae in this larger area.
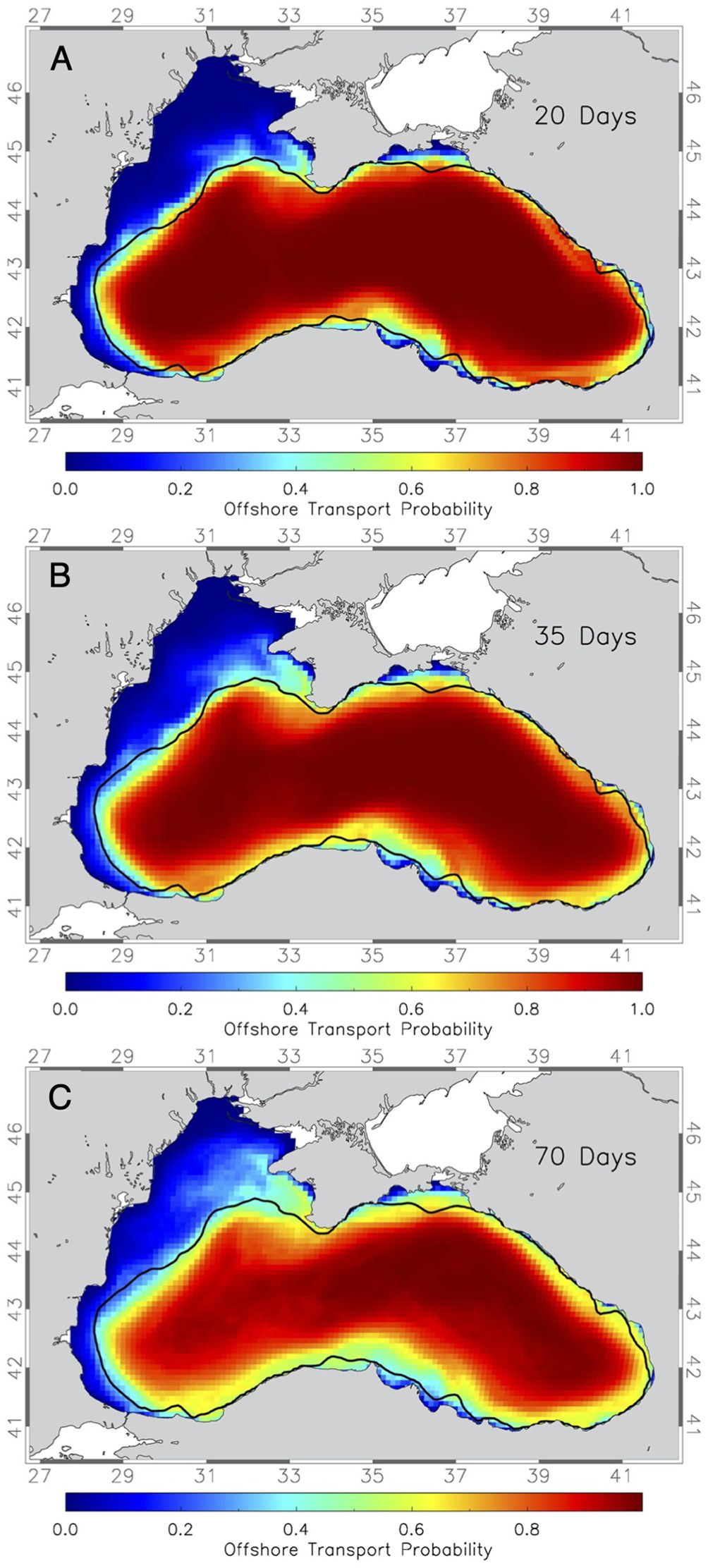
Figure 3. Maps of the average probability of drifter released at 1km spacing everywhere in the Black Sea being transported offshore (> 200m) in (A) 20 days, (B) 35 days and (C) 70 days Thick black line marks the 200m depth contour.
Similarly, most offshore, inner Black Sea regions experience low probabilities at 20-day onshore transport times (Supplementary Figure S1A). However, considerable onshore transport probability (> 0.3) from offshore regions up to 70 km away from the shelf break is observed in the 70-day simulations in the western gyre (Supplementary Figure S1C), while this transport is about 30 km less in the 35-day simulation (Supplementary Figure S1B) and even more reduced in the 20-day simulation (Supplementary Figure S1A). Similar changes in onshore transport can be observed in the eastern gyre as well. At the southern coast past Sinop, the area from where onshore transport from the open sea can be facilitated increases from about 50 km in the 20-day simulation to about 80 km in the 70-day simulation. In addition, the regions along the Kizilirmak and Yesilirmak rivers in the southern Black Sea show high probabilities of onshore transport for all simulation times (Supplementary Figures S1A–C) which indicates high cross-shelf exchange in the area. Because the onshore transport probabilities are invers to the offshore probabilities and offshore transport is of more interest concerning pelagic fish larvae, the following analysis will only consider offshore transport probabilities.
3.2 Seasonal variability of cross-shelf transport
Maps of seasonal transport averaged over 10 years show considerable differences in the offshore transport probabilities and reveal strong seasonal differences in transport patterns as well as considerable differences between different PLDs (Figure 4). In an effort to streamline the analysis of the multitude of simulations, only 35-day simulations, representing anchovy and possibly turbot or red mullet, and 70-day simulations, representing sprat, are considered in this analysis.
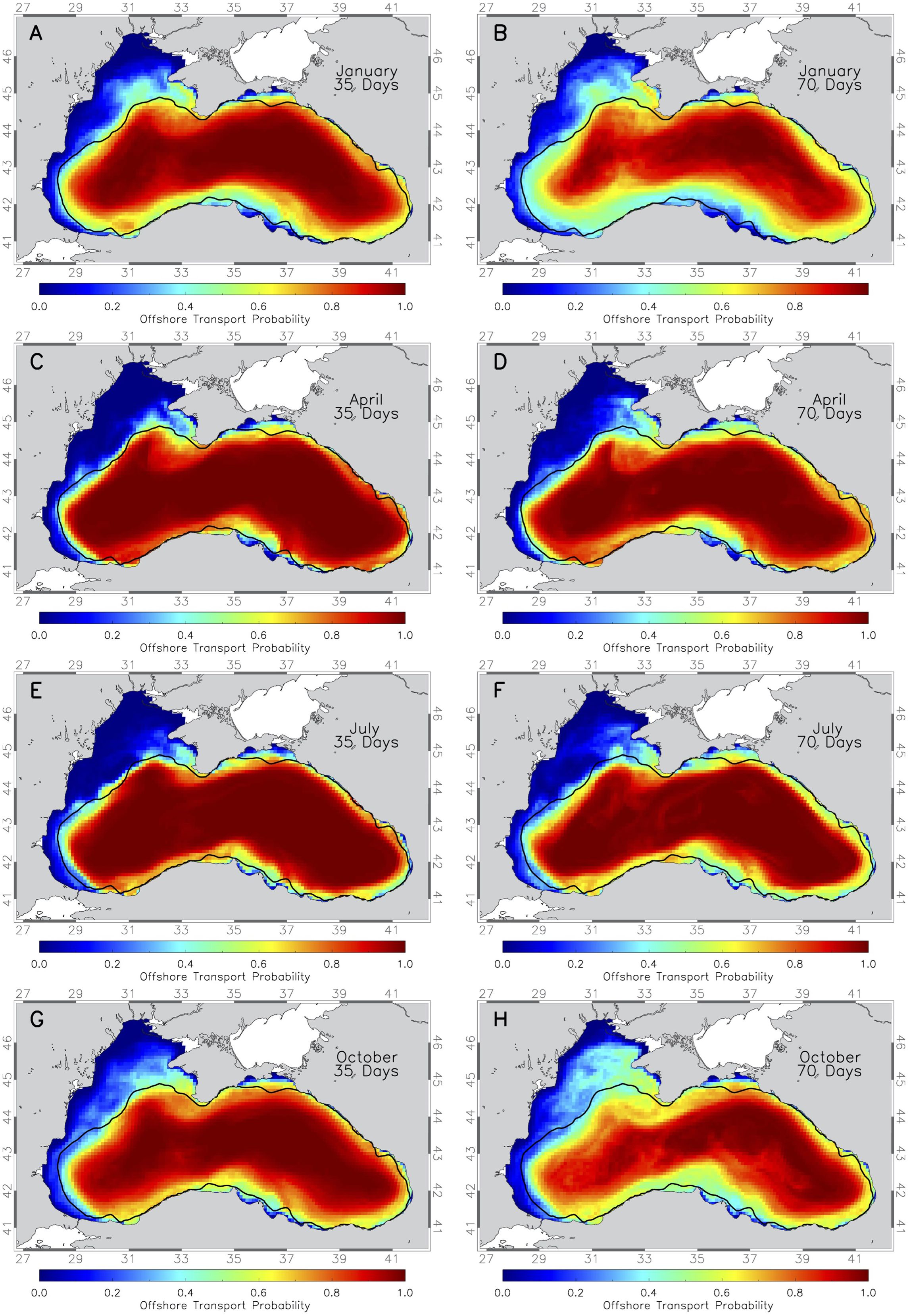
Figure 4. Maps of the average probability of drifter released at 1km spacing everywhere in the Black Sea being transported in 35 days offshore (> 200m) starting in (A) January, (C) April, (E) July, (G) October, and transported in 70 days offshore (> 200m) starting in (B) January, (D) April, (F) July, (H) October. Probability for each season is averaged over 10 years. Thick black line marks the 200m depth contour.
Simulations starting in January with a PLD of 35 days show a high probability of offshore transport from the northwestern shelf near the shelf edge, particularly near Sevastopol, due to the meandering Sevastopol eddy (Figure 4A). Further downstream following the Rim Current flow, areas up to 100 km from the shelf break have over 0.4 probability of offshore transport. This area increases when considering 70-day simulations, reaching up to 46 °N, well onto the shelf towards the Danube outflow. These regions overlap with spawning and nursery areas of key fish species. Along the south coast Rim Current location, a 0.65 probability band for offshore transport is observed, moving offshore in the western gyre and off Sinop, indicating the possibility for more offshore transport in winter away from the shelf break (Figure 4A). This band widens to 30 km and shifts 60 km offshore in 70-day simulations (Figure 4B), mainly in the western gyre and southern Black Sea.
In April, offshore transport probabilities are lower than in January for 35-day simulations (Figure 4C). A 0.4 probability extends 50 km onto the shelf and past Olenivka, and high offshore transport continues along the 200 m depth contour, only moving further offshore near the Kizilirmak and Yesilirmak deltas. In 70-day simulations, offshore transport from the northwestern shelf and Kerch Strait increases slightly (Figure 4D). Bluefish and turbot, with short to intermediate PLDs, are represented by the spring simulations.
In July, offshore transport probabilities near Sevastopol are lower than in April, but a high probability area exists downstream at 44°N (Figure 4E). For 70-day simulations, offshore transport affects larger regions on the northwestern shelf and Kerch Strait (Figure 4F). This impacts summer-spawning species like anchovy, red mullet, and horse mackerel. The 10-year averages suggest strong interannual differences (Figure 4F) that are explored in section 3.3.
In fall, offshore transport increases on the northwestern shelf, reaching up to 150 km from the shelf break in 35-day simulations (Figure 4G). This area expands in the 70-day simulations, affecting much of the northwestern shelf up to 46°N. The Rim Current’s interaction with Crimea causes it to bifurcate, increasing offshore transport from the northwestern shelf.
Similar seasonal differences for medium and long pelagic larval stages can be observed for 20-day pelagic larval stage simulations (not shown here), with general probabilities of offshore transport from the shelf being smaller due to the shorter simulation time.
3.3 Interannual variability of cross-shelf transport
To examine the interannual variability in cross-shelf transport, the following section focuses on the variability of the transport probabilities over 10 years in the form of the standard deviation over 10-year simulations. Of all possible seasons and PLDs the July release with 35-day PLD is chosen as this best represents anchovy (Figure 4E) and further the January release with 70-day PLD representing sprat (Figure 4B), the fish species of main commercial importance in the Black Sea.
The main areas of offshore transport variability in the 35-day simulation are the regions of the Sevastopol eddy and bifurcation of the Rim Current southwest of Crimea to the Kali-Akra anticyclonic eddy on the southern part of the northwestern shelf and a narrow band along southern Black Sea coast with the Yesilirmak eddy, as well as the north coast near Kerch Strait (Figure 5A). In the 70-day PLD winter spawning simulations, this variability (Figure 5B) is higher and covers more regions than in the July simulation. The northwestern shelf and the northern shelf show the highest variability, while in the southern Black Sea high variability is mainly seen in the open Black Sea. This is because the simulation time is longer but also because during winter a stronger, more developed Rim Current is circling the Black Sea which has been observed (Kubryakov and Stanichny, 2015) and reproduced in other modelling studies (Sadighrad et al., 2021).
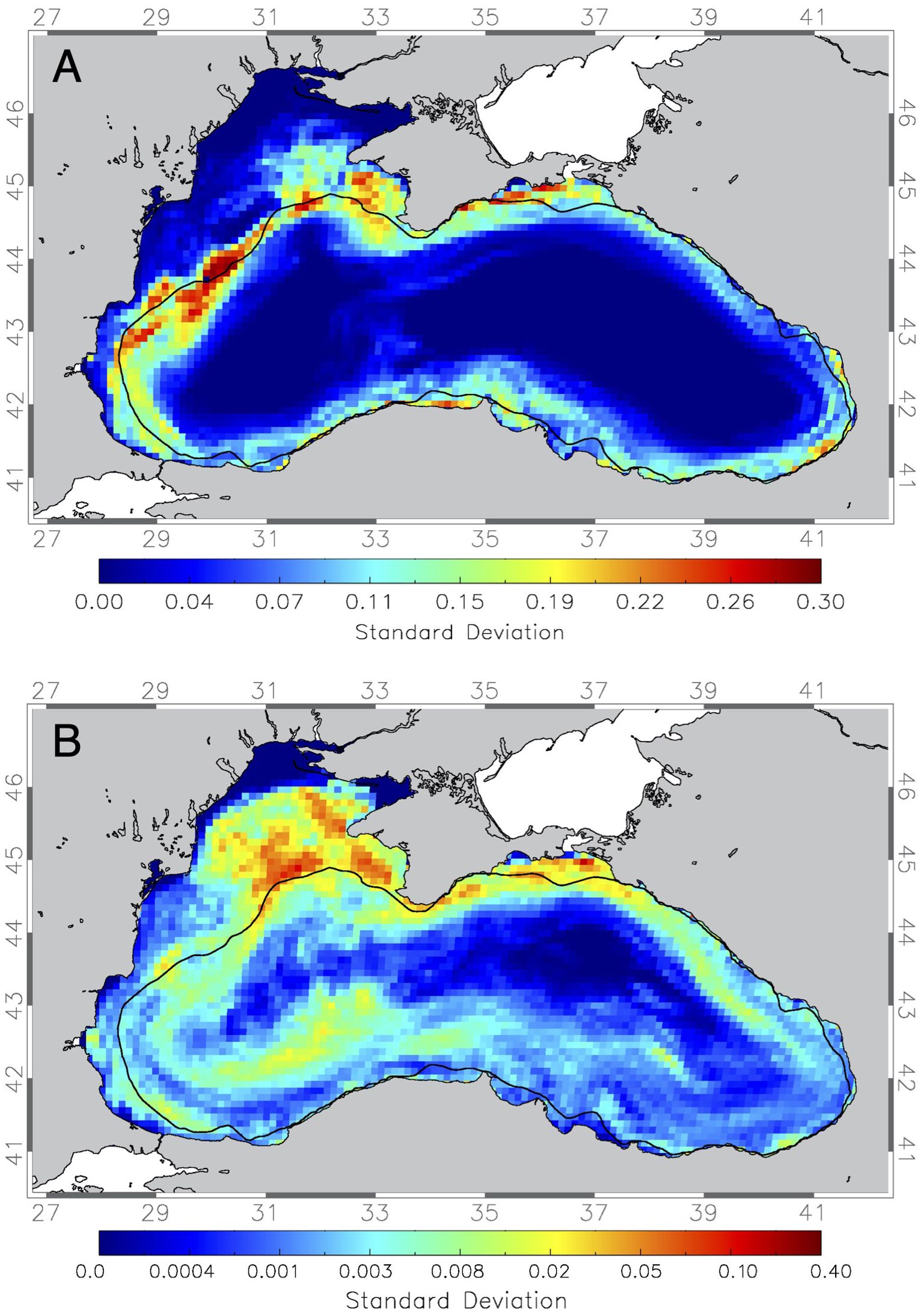
Figure 5. Maps of the standard deviation of offshore (> 200m) transport for drifter released at 1km spacing everywhere in the Black Sea in the years 2001-2010 in the (A) 35-day simulations starting in July of 2001-2010 and (B) 70-day simulations starting in January. Thick black line marks the 200m depth contour.
For a more detailed analysis in interannual variability the region-specific percentage of larvae transported offshore was calculated for each year (Tables 2, 3), whereby the Black Sea was divided into eleven regions (Figure 6). It should be noted that the northwestern shelf area here is defined to not encompass the entire shelf region down to 42S but encompasses the northern part of the shelf region only until 44.5S. The southern part of that shelf region was separated into another region (West Coast), because of the distinct difference in offshore transport probabilities between both regions. The open ocean was divided into the two gyres that exist in the Black Sea and the two regions are mentioned for completeness. They are however biologically not significant, as the analysis concerns fish larvae spawned on the shelf.
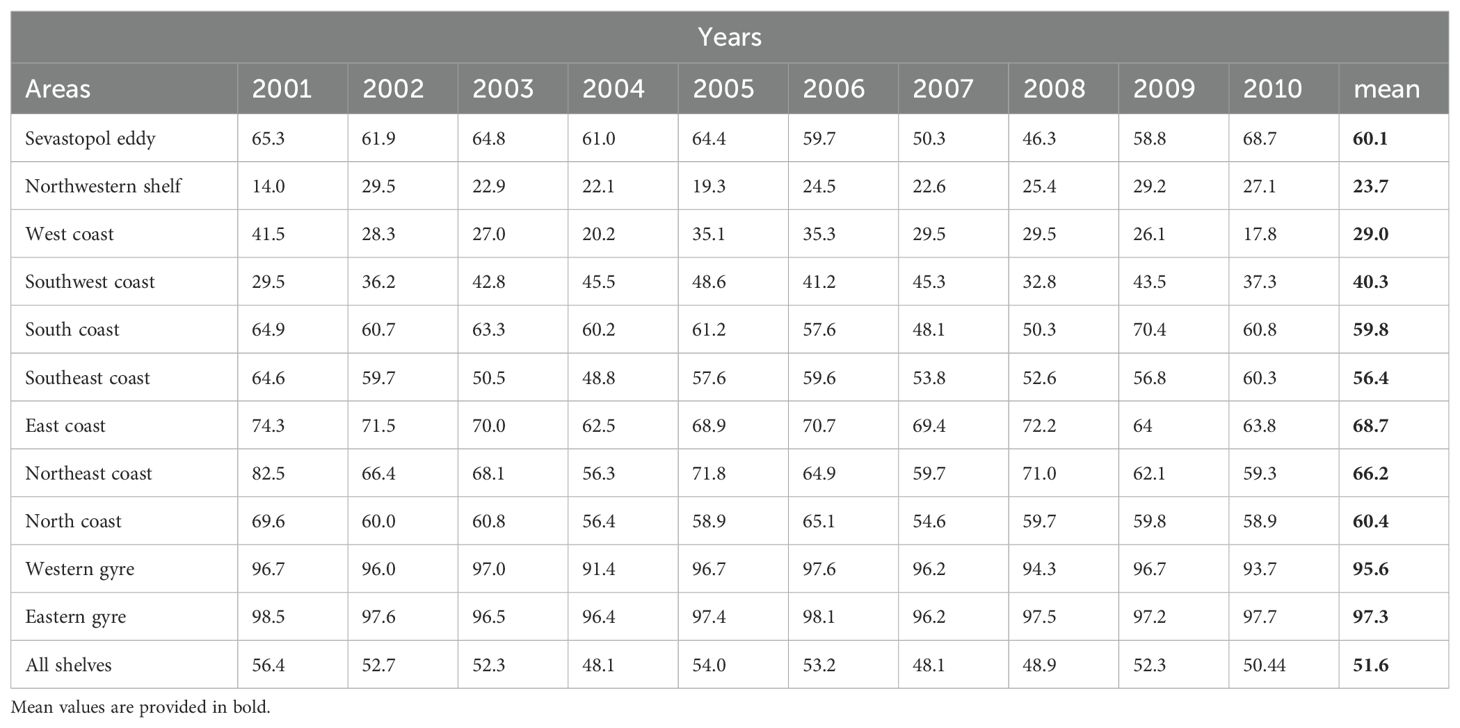
Table 2. Area specific (see Figure 6) analysis of the percentage of anchovy larvae spawned in July that are transported offshore each year of the simulations and on average.
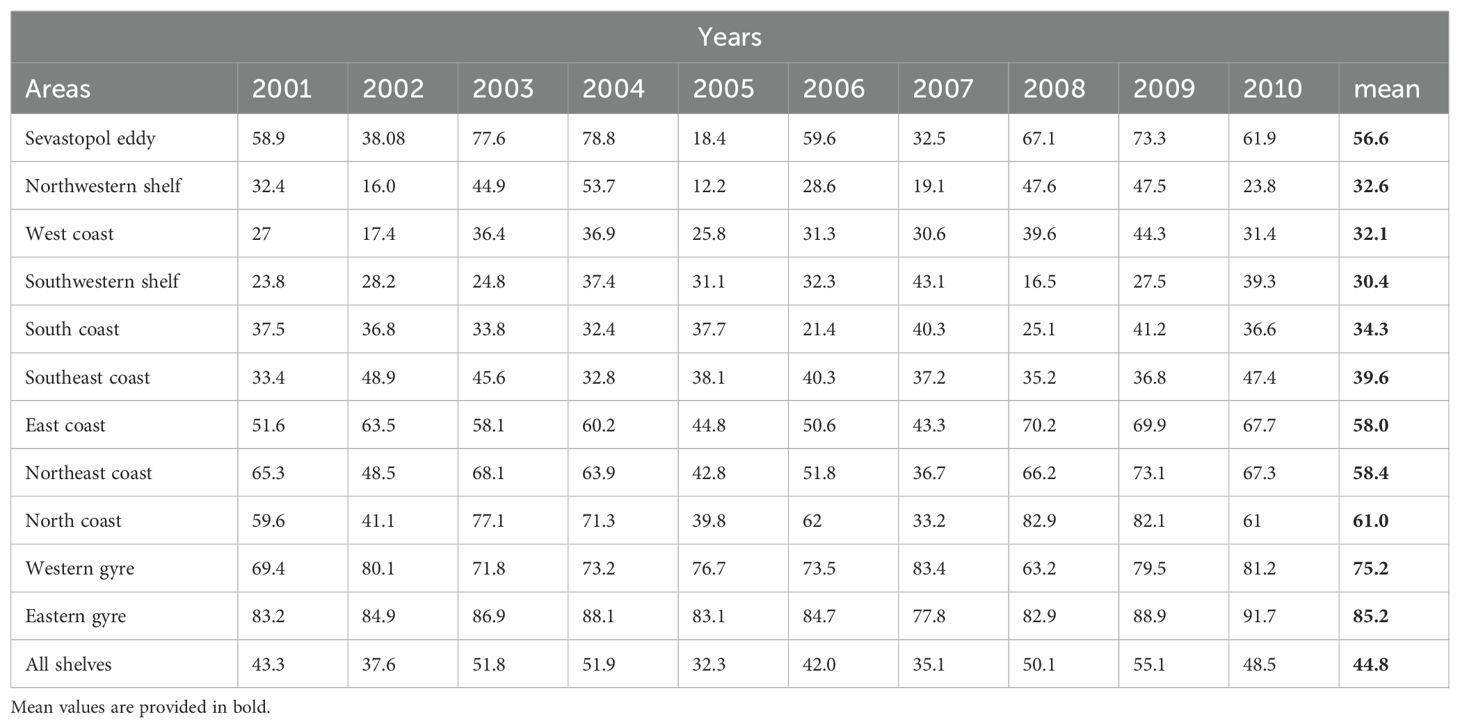
Table 3. Area specific (see Figure 6) analysis of the percentage of sprat larvae spawned in January that are transported offshore each year of the simulations and on average.
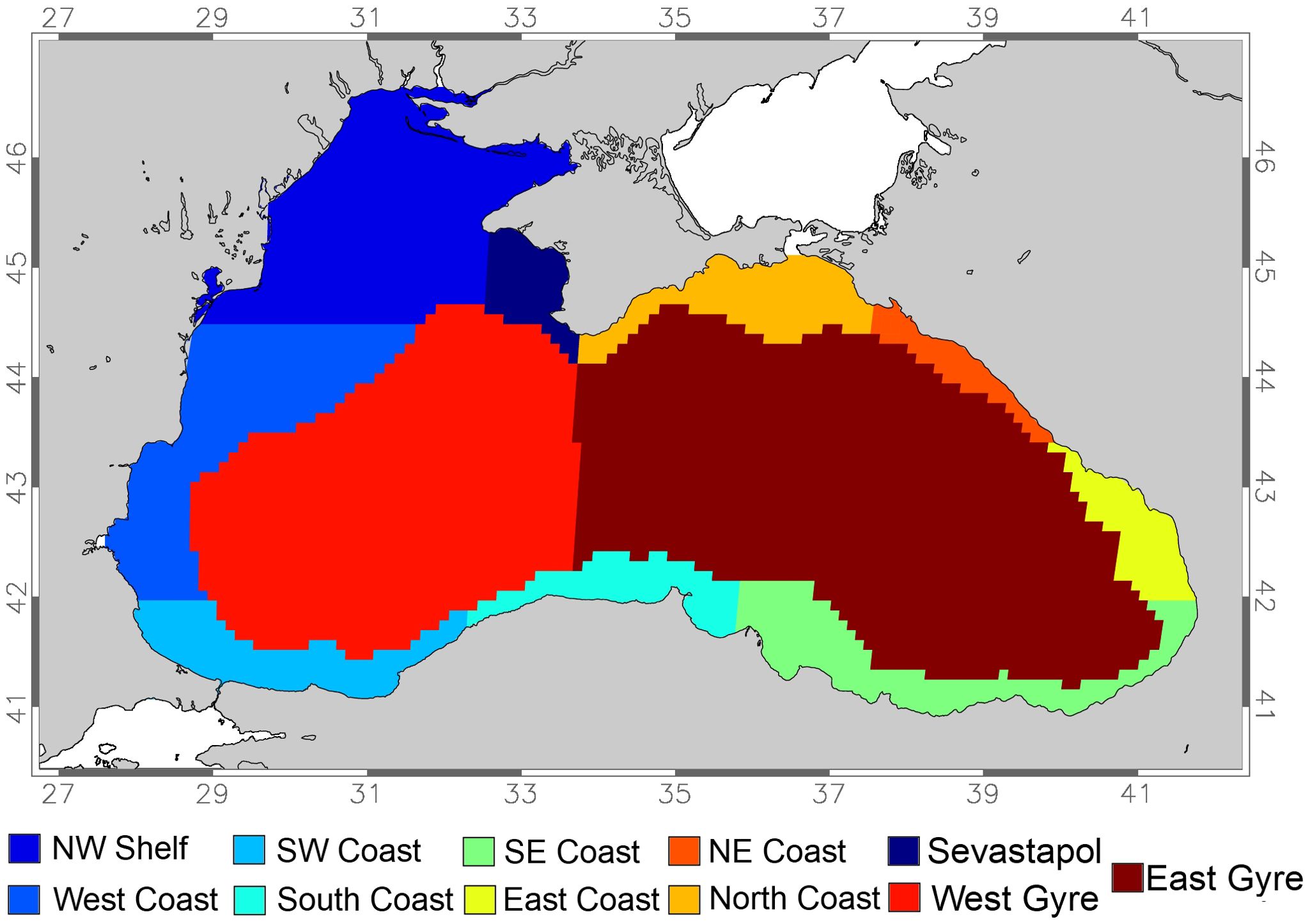
Figure 6. Map of the Black Sea divided into eleven areas used to calculate the percentage of different pelagic fish larvae to be advected offshore.
The northwestern shelf shows a mean transport with almost a quarter of the anchovy larvae (23.7%) being transported offshore in 35 days (Table 2). The area exhibits moderate variability in transport percentages, with values ranging from 14.0% in 2001 to 29.5% in 2009, while the Sevastopol eddy area exhibits significantly higher transport percentages than the northwestern shelf ranging from 46.3% in 2008 to 68.7% in 2010, which means values vary by up to 22%. Further downstream, the East coast has the highest and most consistent transport percentages of all shelf areas, ranging from 62.5% in 2004 to 74.3% in 2001. Overall, the regions in the east and north-east have the highest and most consistent offshore transport percentages (Table 2), indicating strong and reliable transport due to the narrow shelf and proximity of the Rim Current. In contrast, the West Coast has the lowest transport percentages after the northwestern shelf. The other regions show varying degrees of transport efficiency, influenced by their local environmental conditions, and currents dynamics. When analyzing transport variability between years, 2001 had the highest transport offshore transport overall, with a mean transport percentage of 56.2% (Table 2, see Supplementary Figure S2). This was followed closely by 2005 (54.0%). The year 2004 had the lowest transport percentage with 48.1% (Table 2; Supplementary Figure S2). The general trend shows fluctuations in transport efficiency from year to year, depending on the varying environmental conditions.
For the 70-day simulations, the percentage of transport from all shelf regions varied considerably between years (Table 3; Supplementary Figure S3), more so than in the 35-Day simulations. Transport from the Northwestern shelf varied strongly from a low of 12.2% in 2005 to a high of 53.7% in 2004, a 41.5% range. The mean value over the period is 32.6% (Table 3) which is more than in the 35-day simulations. The offshore transport percentages in the Sevastopol Eddy area varied between 18.4% in 2005 and 78.8% in 2004 with a mean value of 56.6%. Transport from the East Coast area was high, ranging from 36.7% in 2007 to 73.1% in 2009 with a mean value of 58.4% (Table 3) over the ten-year period, which is less than in the 35-day simulations. The Southwest Coast has shown the lowest transport percentages while the Northeast Coast and North Coast have the highest and most consistent offshore transport percentages (Table 3). For these longer simulations 2009 showed the highest offshore transport from shelf regions overall, with a mean transport percentage of 55.1%. This was followed closely by 2004 (51.9%). The year 2005 had the lowest transport percentage (32.3%). Interestingly, different years are of importance for high offshore percentages between the 35-day and the 70-day simulation.
3.4 Local retention
It is important to note that in areas with zero offshore transport probability found on the northwestern shelf and elsewhere (see Figure 4) drifters are not necessarily retained. This analysis shows they are not transported offshore into greater than 200m deep waters but may still be transported long distances ending up on the shelf elsewhere. Hence it is also necessary to look at the potential of fish larvae in different areas for retention, which is explored below.
Local retention is calculated as the percentage of particles released in a 10x10 km area at the start of the simulation and remained within an area nine times as large and retention maps use a logarithmic scale because of the extreme distribution of retention values. The main retention areas calculated over the entire 10-year simulations are present on the northwestern shelf, in the Sevastapol and Sakarya eddies, along the southeastern coast of Turkey in the Yesilirmak and Kizilirmak river region and near Kerch Strait (Figure 7A). An increase in simulation time due to longer PLDs results in an overall drop of local retention values but the areas of high retention remain the same (Figure 7B). Most notable is the fact that there are rather large areas of local retention inside the Eastern and Western Gyre, sometimes connected throughout the entire open sea (Figure 7). This is caused by the currents variability as discussed above.
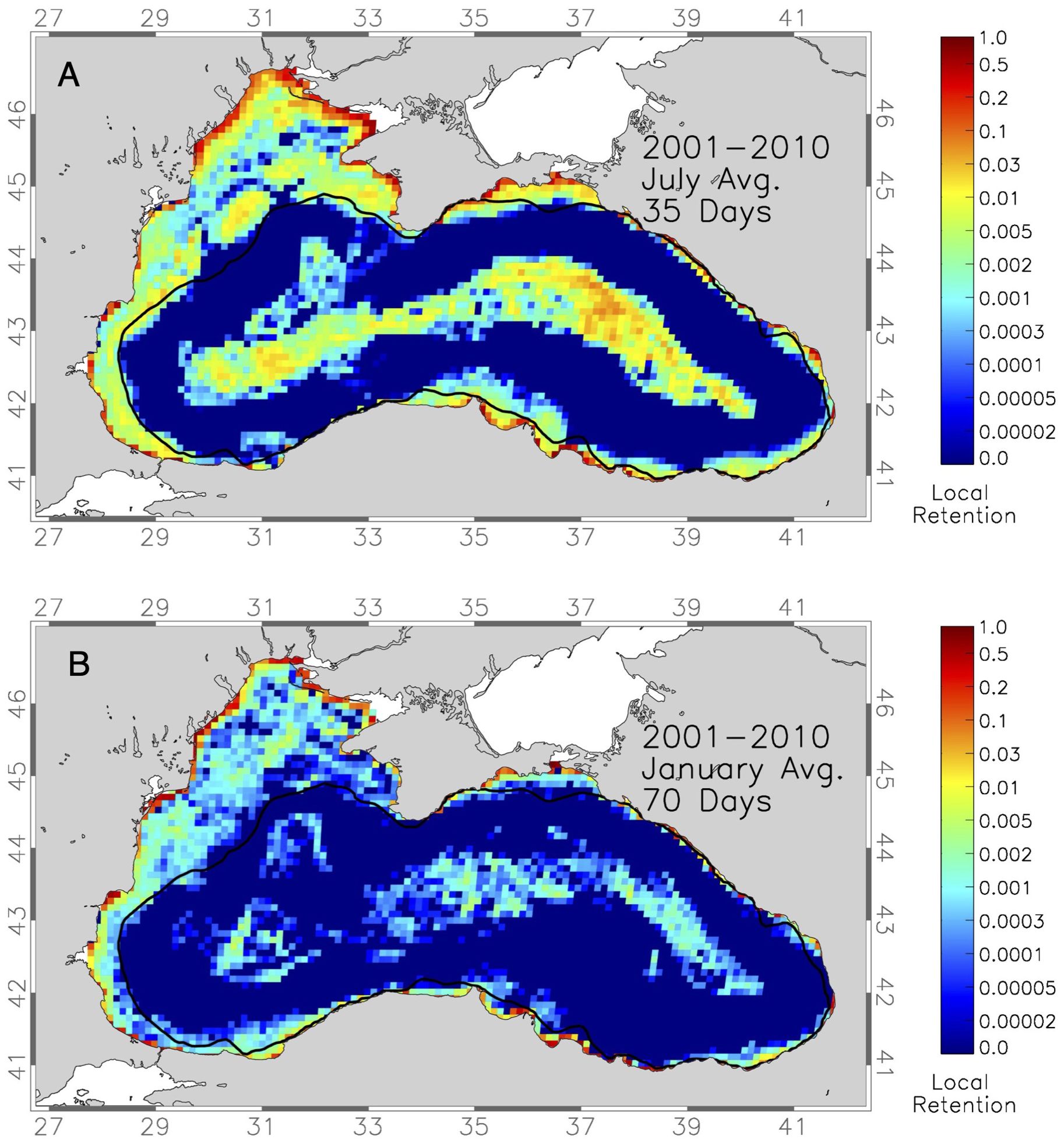
Figure 7. Maps of mean 10-year (2001-2010) local retention of drifter released at 1km spacing everywhere in the Black Sea in (A) the 35-day simulation starting in July and (B) the 70-day simulation starting in January. Thick black line marks the 200m depth contour.
Seasonal variability in local retention on the shelf regions of the Black Sea is lowest in January, reaches the highest values in April, slightly decreases in July and again reach low values in October for both 35 and 70day PLDs (Supplementary Figure S4). The interannual variability of local retention reflects the results from the above offshore transport probability analysis. There is considerable variability of local retention during the 35-day simulations between different years (Supplementary Figure S5). It is notable that the Sevastapol eddy region exhibits high retention rates in all years and a band of retention along the shelf break of the northwestern shelf is visible (Supplementary Figure S5). The Kizilirmak and Yesilirmak regions exhibit consistent local retention which is pronounced in 2005 (Supplementary Figure S5D) and 2009 (Supplementary Figure S5E). The western gyre has consistently high retention rates, while retention in the Eastern Gyre is more variable (Supplementary Figure S5). Considering that the main spawning and nursery areas of most of the five species considered in this study are on the shelf areas, the open ocean retention spots are not biologically significant.
3.5 Implications for small pelagic fish
In section 3.3 it was shown that 23.7% and 32.6% of larvae are transported offshore from the northwestern shelf in both 35-day and 70-day simulations, respectively. This transport rate is higher than anticipated but remains lower compared to all other regions. Retention regions were then identified in section 3.4. To further understand the extent of larval dispersion from the spawning areas based on life-history traits, finally the dispersal percentage of pelagic fish larvae is examined. The spawning area is defined as the combined Northwestern shelf and the Sevastopol eddy regions (see Figure 6). Particles are released considering different planktonic larval durations (PLDs).
Simulations results show that the final location of anchovy spawning in summer with a 35-day PLD can be quite different depending on the year. Overall, much of the larvae end up within the northern part of the northwestern shelf (Figures 8A, C, E) which is also the feeding or nursery area for anchovy larvae (see Figure 1A). However, part of the drifters are transported westward and south in 2001, 2003 and 2010 (Figures 8A, C, E) while also moving slightly offshore from the northwestern shelf in all three years, following the Rim Current path (Figure 2C). Offshore transport rates from the above defined spawning area for these specific years vary from 24.0% in 2001 to 28.6% in 2003 and 25.3% in 2007. In 2001 a wide band of the larvae that end up offshore is seen in the northern part, off the northwestern shelf as far south as the Bosporus (Figure 8A). In contrast, more larvae are reaching areas even further downstream in 2003 and a small percentage of larvae is moved directly offshore into the western gyre. In 2007 again a wide band of offshore larvae is observed while in this year currents also transported larvae much further south in the open Black Sea past the Bosporus (Figure 8E).
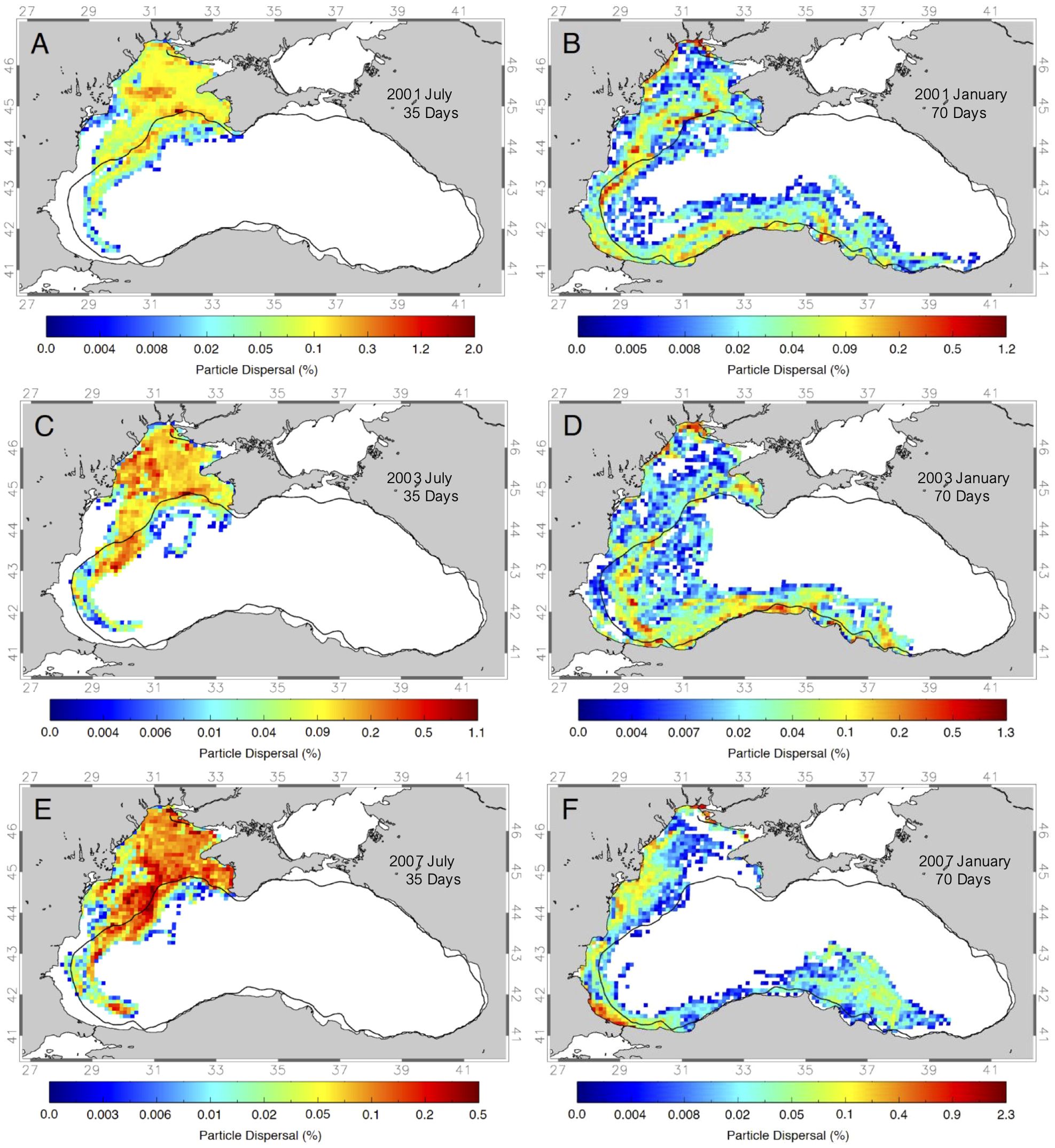
Figure 8. Maps of the dispersal probability (%) of drifter released in the combined northwestern shelf and Sevastopol eddy areas (see Figure 6) with 35-day PLD starting in July of the years (A) 2001, (C) 2003, (E) 2007, and with 70-day PLD’s in January of the years (B) 2001, (D) 2003, and (F) 2007. Thick black line marks the 200m depth contour.
Sprat larvae with a 70-day PLD and release in winter show marked differences in dispersal between years (Figures 8B, D, F). Offshore transport rates vary from 39.1% in 2001 to 51.0% in 2003 and 19.5% in 2007. As expected sprat larvae travel much farther distances than anchovy larvae with the main transport path each year being towards south from the northwestern shelf, following the western coast of the Black Sea, entering the Rim Current and moving as far as the southeastern coast like in all years 2001, 2003 and 2007 (Figures 8B, D, F). The farthest transport is possible in 2001, where larvae reach all the way to the Batumi region (Figure 8B). While in 2001 many regions along the northwestern shelf and along the west and south coasts retained larvae, the currents in 2003, the year with one of the highest offshore transports from the northwestern shelf (Table 3), transported more larvae offshore into the western gyre. In 2007, the year with low offshore transport, most larvae are transported towards the southern coast and into the eastern gyre.
Like the cross-shelf transport and local retention analysis this analysis shows that there is substantial interannual variability of how far and where to pelagic fish larvae can be dispersed during their individual pelagic larval duration time. It is also interesting to see that many 70-day simulation particles are swept from the northwestern shelf area but end up in different onshore regions downstream, such as along the narrow southern coast shelf regions. That explains the lower offshore transport percentages found for sprat larvae (Table 3) compared to anchovy larvae (Table 2) in different years.
4 Discussion
In this study a very high number of virtual particles (410 478 drifters) representing pelagic fish larvae were released across the entire Black Sea for every single simulation. These were dispersed by the ocean circulation fields, simulated with a hydrodynamic model over a 10-year duration. The pelagic fish larvae were tracked over different time frames according to species specific criteria. This has the benefit that it is possible to investigate species specific dispersal pathways and retention areas as a baseline for future change scenarios. This is of particular interest as the species studied are commercially important fish species for the Black Sea.
4.1 Cross-shelf transport and retention
The patterns of 10 year mean onshore and offshore transport indicate that the main driving force of this cross-shelf transport are currents associated with the Rim Current circling cyclonically around the Black Sea and that are interacting with the shelf break. Particularly the current dynamics at the shelf break downstream of Crimea, where the Sevastopol anticyclonic eddy is located is of importance for the cross-shelf transport of larvae in this larger area. Here, the interaction of the Rim Current with the tip of Crimea is causing a bifurcation of the Rim Current at times and increasing the offshore transport probabilities from the northwestern shelf as well as the onshore transport probabilities southwest of Crimea that has also been documented (Shapiro et al., 2010). However, the eddy itself is supporting the retention of pelagic fish larvae.
Various anticyclonic eddies such as the Sevastopol eddy spin off the Rim Current. In this study particularly the Sinop eddy has been seen to play a large role in facilitating local retention as drifters are caught up in the eddy and retained. In addition, large areas close to Sinop, Kizilirmak and Yesilirmak eddies simulate high probabilities of onshore transport indicating high cross-shelf exchange in the area associated with currents and filaments associated with the eddies. This mechanism of cross-shelf transport has been described in detail in previous studies (Akpinar et al., 2022).
Further, this study has shown the high seasonal and interannual variability of dispersal in the Black Sea. Such variability in cross-shelf transport has been observed in previous studies (Sur et al., 1994; Zatsepin, 2003; Kubryakov and Stanichny (2015); Akpinar et al., 2022). However, this study quantifies the variability of advective offshore transport to be 22.4% for larvae from the Sevastopol eddy region in contrast to 15.5% from the northwestern shelf region in the 35-day simulations representing anchovy larvae. These values are considerably higher for 70-day simulations (sprat) with 54.9% and 41.5% from the Sevastopol eddy regions and the northwestern shelf region, respectively.
Overall, simulations show that local retention in the Black Sea is low, even on the northwestern shelf, the main spawning and nursery area for fish. The 10-year mean of the simulations show 24% of anchovy larvae and 32 of sprat larvae are transported offshore. Fish spawned on the shelf may therefore not at all be able to stay in the area but be transported away and/or offshore. Further, the cross-shelf transport probability facilitated by currents associated with the Rim Current is rather variable. Such variability in cross-shelf transport has been observed in previous studies (Sur et al., 1994; Zatsepin, 2003; Kubryakov and Stanichny (2015); Akpinar et al., 2022).
In addition, simulation results show two different transport regimes in the model simulations. During spring and summer lower offshore transport is observed than in fall and winter. Similarly higher retention occurs during the summer months than in winter. This is directly related to Rim Current strength which has been shown to vary seasonally (Kubryakov and Stanichny, 2015) and has been reproduced in other modelling studies (Sadighrad et al., 2021). Studies have shown that seasonally changing winds have direct implications on the Black Sea circulation. In spring and summer, the Rim Current has been observed to weaken as a result of reduced wind forcing (Besiktepe et al., 2001), and a weakening of the Rim Current results in increased mesoscale eddy activity (Kubryakov and Stanichny, 2015; Kubryakov et al., 2016; Miladinova et al., 2020; Sadighrad et al., 2021). In addition, cross-shelf transport at the surface is under the direct influence of the wind leading to Ekman transport in addition to the eddy activity (Akpinar et al., 2022). It has been shown that the effect of Ekman transport is to increase the on-shelf transport (Akpinar et al., 2022). In this study the decreased Rim Current strength and increased anticyclonic activity in spring and summer led to more retention on the north-western shelf and in the anticyclonic eddies along the southern Black Sea coast.
However, at the same time increased meso-scale eddy activity in the simulations were observed to increase the cross-shelf transport in certain regions such as the south-east Black Sea coast. Meso-scale eddies, transient mesoscale features such as filaments, dipoles and meanders in the Black Sea are known to serve as sources of nutrients and plankton productivity (Oguz et al., 1993; Korotaev, 2003; Blokhina and Afanasyev, 2003). And besides the importance of wind stress curl (Kubryakov et al., 2016) the interaction of the currents with capes and peninsulas (Blokhina and Afanasyev, 2003; Staneva et al., 2001; Elkin and Zatsepin, 2013; Zatsepin et al., 2019) play a major role in the formation of eddies in the Black Sea. Analyses show that mesoscale features transport nutrients and biomass of shelf waters into the open sea (Sur et al., 1994; Özsoy and Ünlüata, 1997; Zatsepin, 2003; Kubryakov et al., 2016, Kubryakov et al., 2018; Sadighrad et al., 2021; Akpınar, 2024). Hence the results of this study that fish larvae experience such high rates of cross-shelf dispersal, with lowest rates only on the norther northwestern shelf are in line with these findings.
4.2 Implications for life histories of small pelagic fish in the Black Sea
The species-specific early life history of pelagic fish plays an important role in the recruitment of fish and population dynamics. In the Black Sea typical larval durations are between 20 days and 70 days (Satilmis et al., 2003; Chashchin et al., 2015; Gucu et al., 2016; Satilmis et al., 2014; Scientific, Technical and Economic Committee for Fisheries (STECF), 2017) and these larvae stages cannot yet perform directional swimming. Because the main circulation structure of the Black Sea, the Rim Current, features fast-moving currents of approximately 10-50 cm/s as well as several anticyclonic eddies on the coastal side of the Rim Current, it is highly likely that pelagic fish larvae are subject to advection. However, it should be noted that all the target fish species of this study have different attributes regarding their eggs and larvae, such as shape, size and density, as well as vertical migration, that has not taken into consideration in the simulation. This is a limitation of this study as such factors may introduce slight variations in the drifter paths.
Another limitation is that the circulation model at times does not correctly represent the currents on the northern northwestern shelf when compared to satellite data (Salihoglu et al., 2017). In particular, the simulated currents flow northward and eastward near the northwestern coast, favoring retention in the area, instead of flowing south and west as in the satellite derived fields that favor flow towards the open Black Sea (Salihoglu et al., 2017). Therefore, the offshore transport rates calculated for the northwestern shelf may actually be an underestimation of the actual dispersal, which should be kept in mind.
The statistics of cross-shelf transport, local retention and dispersal percentage presented here provide a useful proxy for the potential early-life dispersal of small pelagic fish spawning in the Black Sea. They show that fish larvae spawned on the northwestern shelf area (see Figure 6) experience cross-shelf transport of 23.7% versus 32.6% for 35-day and 70-day simulations, respectively. Similarly for the Sevastopol eddy region (see Figure 6) where the offshore transport is 60.1% and 56.6%. Further, low retention on the shelf areas of the Black Sea is observed.
The findings presented here suggest that anticyclonic eddies on the coastal side of the Rim Current provide mechanisms of retention and limiting offshore transport particularly during spring and summer, while the Rim Current and other mesoscale features occurring along the shelf break tend to facilitate cross-shelf transport (Sadighrad et al., 2021; Akpinar et al., 2022). Spawning during summer, such as anchovy, red mullet and horse mackerel exhibit, thereby favors retention on the shelf at that time vs fall or winter. Timing and location of anchovy spawning thereby is likely to enhance retention on the northwestern and northeastern shelf.
Phytoplankton concentration as measured with satellited chlorophyll concentration in the Black Sea show big differences between the chlorophyll-rich shelf and comparatively less productive open regions (Sorokin, 2002). The open Black Sea experiences its chlorophyll maximum during autumn and winter with minimum levels found during the summer months (Vinogradov et al., 1999; McQuatters-Gollop et al., 2008) while the Northwest Shelf undergoes two blooms, a spring bloom dependent upon river inflow, and a smaller autumn bloom (Cociasu and Popa, 2005; Yunev et al., 2007; McQuatters-Gollop et al., 2008). Therefore, the strategy of retention may be a good survival strategy as the chlorophyll-rich shelf likely provides more food to fish than the comparatively less productive open regions (Sorokin, 2002; McQuatters-Gollop et al., 2008). Fish species that spawn in spring, such as Bluefish and Turbot, that also have PLD’s between 30-40 days optimize the possibility to be retained in their spawning areas event more than summer spawners.
In contrast, sprat with its long 70-day PLD and winter spawning is more adapted to high offshore transport and low retention rates. Its winter spawning time subjects it to conditions when the Rim Current is strong and enhances cross-shelf transport. In addition, the very long dispersal times of this species makes it unlikely that sprat is recruited to the same area as where it is spawned. Sprat is known to spawn in all shelf regions of the Black Sea up to 100 km offshore (Ojaveer, 1981; Houde, 1989; Ivanov and Beverton, 1985) and as sprat is a cold loving fish this may be an ideal strategy to reach different feeding grounds at the beginning of spring and find suitable cold habitats.
It is suggested that Black Sea pelagic fish species such as anchovy and sprat adopted different reproduction strategies through their species-specific traits that are the spawning area, timing of spawning and pelagic larval duration. The larvae of spring and summer spawners then are able utilize summer production while winter spawners utilize winter and spring production further offshore (Daskalov, 1999). Considering the bloom dynamics in the Black Sea (Vinogradov et al., 1999; Cociasu and Popa, 2005; Yunev et al., 2007; McQuatters-Gollop et al., 2008), advection offshore may put larvae into a habitat with less food where they are more likely to face starvation (Chashchin et al., 2015). The retention of pelagic fish eggs and larvae (as well as their zooplankton prey) on the shelf and in anticyclonic eddies may therefore be an important factor influencing spawning success and recruitment for these pelagic fish that has been supported by observations (Melnikov et al., 2024). It may be directly impacting the pelagic fisheries that are in place by all Black Sea riparian countries.
This study further documents the high seasonal and interannual variability of dispersal in the Black Sea. This large variability may influence successful recruitment of small pelagic fish in different regions and hence may be one factor influencing ultimately populations dynamics of these species that lead to observed strong fluctuations in fish catch (Scientific, Technical and Economic Committee for Fisheries (STECF), 2017). This study demonstrates strong interannual variability in cross-shore transport (Figure 5; Supplementary Figures S2, S3) and retention (Figure 7) and it would be of interest to match this with equivalent data relating to variability in spawning success. The work presented here gives a baseline from which the impacts of future changes in the Black Sea fish recruitment can be assessed.
5 Conclusion
The modeling of dispersal via currents in the Black Sea revealed differences in the upper ocean transports in different regions of the Black Sea. The interaction of the cyclonic Rim Current circling the entire Black Sea basin with topographic features and the coastline causes formation of mesoscale features such as eddies and filaments that facilitate strong cross-shelf transport. This is particularly evident at the Crimean coast and downstream along the northwestern shelf break, where the Rim Current interacts with topography, and near Kerch Strait.
Anticyclonic eddies on the coastal side of the Rim Current and eddy induced mixing however tend to provide mechanisms of retention and limit offshore transport particularly in spring and summer. This leads to higher retention in regions such as Sinop, Kizilirmak and Yesilirmak eddies, as well as in the Sevastapol eddy. However, overall local retention, except on the northern part of the northwestern shelf, is surprisingly low in the Black Sea.
It is argued that this physical environment favors very different reproductive strategies by different pelagic fish that contribute to their successful recruitment. Thereby sprat favors a high dispersal strategy during winter while anchovy and other fish species with low PLD’s such as red mullet and horse mackerel are minimizing their dispersal to be retained in the spawning areas which can function as nurseries at the same time.
Theoretical modeling studies such as presented here should be considered when interpreting future recruitment variability and changes in population dynamics in the Black Sea and elsewhere. Results presented here can serve as a baseline when considering the impact of future changes on the Black Sea current system and its impact on pelagic fish species. It is anticipated that the 100-year return period wind speeds increase approximately 7% and 27% in the western and eastern Black Sea, respectively, compared to the historical period (Islek et al., 2021) likely increasing the strength of the wind-driven Rim Current as has been observed for short periods before (Capet et al., 2012). This will presumably increase cross-shelf transport and could result in less retention of pelagic fish larvae in the nursery areas located mainly on the shelf, decreasing successful recruitment.
Data availability statement
The raw data supporting the conclusions of this article will be made available by the authors, without undue reservation.
Author contributions
BF: Conceptualization, Funding acquisition, Project administration, Writing – original draft, Methodology. BC: Formal analysis, Investigation, Visualization, Writing – original draft, Methodology. SA: Investigation, Methodology, Supervision, Writing – review & editing. DT: Funding acquisition, Resources, Supervision, Writing – review & editing. BS: Funding acquisition, Resources, Supervision, Writing – review & editing.
Funding
The author(s) declare financial support was received for the research, authorship, and/or publication of this article. This study was partially supported by the 117Y396 project funded by the Turkish Scientific and Technical Council (TÜBİTAK) and DEKOSIM (BAP‐08‐11‐DPT2012K120880) funded by the Turkish Ministry of Development. The authors also acknowledge support by the Horizon 2020 framework program projects DOORS (contract no. 101000518) and BRIDGE-BS (contract no. 101000240).
Acknowledgments
This paper stems resulted from the MSc Thesis of BC. We thank Ekin Akoglu for fruitful discussions on the MSc. thesis.
Conflict of interest
The authors declare that the research was conducted in the absence of any commercial or financial relationships that could be construed as a potential conflict of interest.
Publisher’s note
All claims expressed in this article are solely those of the authors and do not necessarily represent those of their affiliated organizations, or those of the publisher, the editors and the reviewers. Any product that may be evaluated in this article, or claim that may be made by its manufacturer, is not guaranteed or endorsed by the publisher.
Supplementary material
The Supplementary Material for this article can be found online at: https://www.frontiersin.org/articles/10.3389/fmars.2024.1435556/full#supplementary-material
References
Akpinar A., Sadighrad E., Fach B. A., Arkın S. (2022). Eddy induced cross-shelf exchanges in the black sea. Remote Sens. 14, 4881. doi: 10.3390/rs14194881
Akpınar A. (2024). Seasonal and interannual variability in sea surface temperature fronts in the levantine basin, mediterranean sea. J. Mar. Sci. Eng.12 2024, 1249. doi: 10.3390/jmse12081249
Allen I., Arkin S., Cossarini G., Butenschoen M., Ciavatti S., Christensen A., et al. (2014). D2.6: Report on reanalysis hindcast skill. OPEC Rep., pp 66.
Almany G. R., Berumen M. L., Thorrold S. R., Planes S., Jones G. P. (2007). Local replenishment of coral reef fish populations in a marine reserve. Science 316, 742–744. doi: 10.1126/science.1140597
Besiktepe S., Lozano C. J., Robinson A. R. (2001). On the summer mesoscale variability of the black sea. J. Mar. Res. 59 (4), 475–515. doi: 10.1357/002224001762842163
Blokhina M. D., Afanasyev Y. D. (2003). Baroclinic instability and transient features of mesoscale surface circulation in the black sea: laboratory experiment. J. Geophys. Res. Oceans 108. doi: 10.1029/2003JC001979
Blumberg A. F., Mellor G. L. (1987). “A description of a three-dimensional coastal ocean circulation model,” in Three Dimensional Coastal Ocean Models. Eds. Heaps, Norman S. (Washington, DC: American Geophysical Union), 1–16.
Bolle L. J., Dickey-Collas M., van Beek J. K. L., Erftemeijer P. L. A., Witte J., van der Veer H. W., et al. (2009). Variability in transport of fish eggs and larvae. III. effects of hydrodynamics and larval behaviour on recruitment in plaice. Mar. Ecol. Prog. Ser. 390, 195–211. doi: 10.3354/meps08177
Capet A., Barth A., Beckers J. M., Marilaure G. (2012). Interannual variability of black sea's hydrodynamics and connection to atmospheric patterns. Deep-Sea Res. Part II 77-80, 128–142. doi: 10.1016/j.dsr2.2012.04.010
Carson H. S., Cook G. S., López-Duarte P. C., Levin L. A. (2011). Evaluating the importance of demographic connectivity in a marine metapopulation. Ecology 92 (10), 1972–1984.
Ceyhan T., Akyol O., Ayaz A., Juanes F. (2007). Age, growth, and reproductive season of bluefish (Pomatomus saltatrix) in the marmara region, turkey. ICES J. Mar. Sci. 64 (3), 531–536. doi: 10.1093/icesjms/fsm026
Chashchin A., Shlyakhov V. A., Dubovik V. E., Negoda S. (2015). “Stock assessment of anchovy (Engraulis encrasicolus L.) in Northern Black Sea and Sea of Azov,” in Progressive Engineering Practices in Marine Resource ManagementStock Assessment of Anchovy (Engraulis encrasicolus L.) in Northern Black Sea and Sea of Azov. Eds. Zlateva I., Raykov V., Nikolov N. (IGI Global, USA), 209–243.
Christensen O. B., Drews M., Christensen J. H., Dethloff K., Ketelsen K., Hebestadt I., et al. (2007). The HIRHAM Regional Climate Model. Version 5 (beta), DMI Technical Report No. 06-17. Danish Climate Centre, DMI.
Clavel-Henry M., Bahamon N., Aguzzi J., Navarro J., López M., Company J. B. (2024). Indicators to assess temporal variability in marine connectivity processes: A semi-theoretical approach. PloS One 19, e0297730. doi: 10.1371/journal.pone.0297730
Cociasu A., Popa L. (2005). Significant changes in Danube nutrient loads and their impact on the Romanian Black Sea coastal waters. Cercetari Mar. 35, 25–37.
Condie S. A. (2022). Changing the climate risk trajectory for coral reefs. Front. Clim. 4. doi: 10.3389/fclim.2022.980035
Condie S. A., Anthony K. R. N., Babcock R. C., Baird M. E., Beeden R., Fletcher C. S., et al. (2021). Large-scale interventions may delay decline of the Great Barrier Reef. R. Soc Open Sci. 8, 201296. doi: 10.1098/rsos.201296
Condie S. A., Mansbridge J. V., Cahill M. L. (2011). Contrasting local retention and cross-shore transports of the East Australian Current and the Leeuwin Current and their relative influences on the life histories of small pelagic fishes. Deep Sea Res. Part II: Topical Stud. Oceanogr. 58, 606–615. doi: 10.1016/j.dsr2.2010.06.003
Cowen R. K., Paris C. B., Srinivasan A. (2006). Scaling of connectivity in marine populations. Science. 311 (5760), 522–527. doi: 10.1126/science.1122039
d’Ovidio F., Fernández V., Hernández-García E., López C. (2004). Mixing structures in the mediterranean sea from finite-size lyapunov exponents. Geophys. Res. Lett. 31 (17). doi: 10.1029/2004gl020328
Daskalov G. (1999). Relating fish recruitment to stock biomass and physical environment in the Black Sea using generalized additive models. Fish. Res. 1, 1–23. doi: 10.1016/S0165-7836(99)00006-5
Daskalov G. M. (2003). Long-term changes in fish abundance and environmental indices in the Black Sea. Mar. Ecol. Prog. Ser. 255, 259–270. doi: 10.3354/meps255259
Di Stefano M., Legrand T., Di Franco A., Nerini D., Rossi V. (2022). Insights into the spatio-temporal variability of spawning in a territorial coastal fish by combining observations, modelling and literature review. Fish. Oceanogr. 32, 70–90. doi: 10.1111/fog.12609
Dubois M., Rossi V., Ser-Giacomi E., Arnaud-Haond S., López C., Hernández-García E. (2016). Large-scale connectivity and management of marine ecosystems. Global Ecol. Biogeogr. 25, 503–515. doi: 10.1111/geb.12431
Dulcic J. (1997). Growth of anchovy, engraulis encrasicolus (L.), larvae in the northern adriatic sea. Fisheries Res. 31 (3), 189–195. doi: 10.1016/s0165-7836(97)00030-1
Elkin D. N., Zatsepin A. G. (2013). Laboratory investigation of the mechanism of the periodic eddy formation behind capes in a coastal sea. Oceanology 53, 24–35. doi: 10.1134/S0001437012050062
Erftemeijer P. L. A., van Beek J. K. L., Bolle L. J., Dickey-Collas M., Los H. F. J. (2009). Variability in transport of fish eggs and larvae. i. modelling the effects of coastal reclamation. Mar. Ecol. Prog. Ser. 390, 167–181. doi: 10.3354/meps08173
Fach B. A. (2014). Modeling the influence of hydrodynamic processes on anchovy distribution and connectivity in the black sea. Turkish J. Fish. Aquat. Sci. 14, 353–365. doi: 10.4194/1303-2712-v14_2_06
Food and Agriculture Organization [FAO] (2016). The State of Mediterranean, and Black Sea Fisheries (Rome: FAO).
Galarza J. A., Boulay R., Cerdá X., Doums C., Federici P., Magalon H., et al. (2009). Development of single sequence repeat markers for the ant aphaenogaster senilis and cross-species amplification in a. iberica, a. gibbosa, a. subterranea and messor maroccanus. Conserv. Genetics 10, 519–521. doi: 10.1007/s10592-008-9554-9
Giragosov V., Khanaychenko A. (2012). The state-of-art of the black sea turbot spawning population off crimea, (1998-2010). Turkish J. Fish. Aquat. Sci. 12, 377–383. doi: 10.4194/1303-2712-v12_2_25
Gordina A. D., Klimova T. N. (1996). On bluefish (Pomatomus saltatrix l.) spawning in the black sea. Mar. Freshw. Res. 47 (2), 315. doi: 10.1071/mf9960315
Gucu A. C., Inanmaz O. E., Ok M., Sakinan S. (2016). Recent changes in the spawning grounds of Black Sea anchovy, Engraulis encrasicolus. Fish. Oceanogr. 25, 67–84. doi: 10.1111/fog.12135
Guraslan C., Fach B. A., Oguz T. (2017). Understanding the impact of environmental variability on anchovy overwintering migration in the black sea and its implications for the fishing industry. Front. Mar. Sci. 4. doi: 10.3389/fmars.2017.00275
Hamilton S., Caselle J., Malone D., Carr M. (2010). Incorporating biogeography into evaluations of the Channel Islands marine reserve network. Proc. Natl. Acad. Sci. U.S.A. 107, 18272–18277. doi: 10.1073/pnas.0908091107
Hare J. A., Cowen R. K. (1997). Size, growth, development, and survival of the planktonic larvae of pomatomus saltatrix (Pisces: Pomatomidae). Ecology. 78 (8), 2415–2431. doi: 10.2307/2265903
Haynes P. S., Brophy D., Mcgrath D. (2011). The early life history of turbot (Psetta maxima l.) on nursery grounds along the west coast of ireland: 2007–2009, as described by otolith microstructure. Fisheries Res. 110 (3), 478–482. doi: 10.1016/j.fishres.2011.05.014
Houde E. D. (1989). Comparative growth, mortality, and energetics of marine fish larvae –temperature and implied latitudinal effects. Fishery Bull. 87 (3), 471–495.
Islek F., Yuksel Y., Sahin C. (2021). Evaluation of regional climate models and future wind characteristics in the Black Sea. Int. J. Climatol. 42, 1877–1901. doi: 10.1002/joc.7341
Ivanov L., Beverton R. J. H. (1985). The fisheries resources of the Mediterranean. pt. 2: Black Sea (FAO).
Jaspers C., Huwer B., Antajan E., Hosia A., Hinrichsen H.-H., Biastoch A., et al. (2018). Ocean current connectivity propelling the secondary spread of a marine invasive comb jelly across western Eurasia. Global Ecol. Biogeogr. 27, 814–827. doi: 10.1111/geb.12742
Johnston M. W., Purkis S. J. (2011). Spatial analysis of the invasion of lionfish in the western atlantic and caribbean. Mar. pollut. Bull. 62 (6), 1218–1226. doi: 10.1016/j.marpolbul.2011.03.028
Johnston M. W., Purkis S. J. (2014). Are lionfish set for a mediterranean invasion? modelling explains why this is unlikely to occur. Mar. pollut. Bull. 88 (1), 138–147. doi: 10.1016/j.marpolbul.2014.09.013
Jordi A., Wang D. P. (2012). A parallel implementation of Princeton Ocean model. Environ. Model. Softw. 38, 59–61. doi: 10.1016/j.envsoft.2012.05.013
Kara A. B., Hurlburt H. E., Wallcraft A. J., Bourassa M. A. (2005). Black sea mixed layer sensitivity to various wind and thermal forcing products on climatological time scales. J. Climate 18 (24), 5266–5293. doi: 10.1175/jcli3573r2.1
Korotaev G. (2003). Seasonal, interannual, and mesoscale variability of the Black sea upper layer circulation derived from altimeter data. J. Geophys. Res. 108, 3122. doi: 10.1029/2002JC001508
Korotaev G. K., Saenko O. A., Koblinsky C. J. (2001). Satellite altimetry observations of the black sea level. J. Geophysical Research: Oceans 106 (C1), 917–933. doi: 10.1029/2000jc900120
Kubryakov A. A., Mizyuk A. I., Puzina O. S., Senderov M. V. (2018). Three-dimensional identification of the black sea mesoscale eddies according to NEMO numerical model calculations. Phys. Oceanography 25 (1). doi: 10.22449/1573-160x-2018-1-18-26
Kubryakov A. A., Stanichny S. V. (2015). Seasonal and interannual variability of the black sea eddies and its dependence on characteristics of the large-scale circulation. Deep sea research part i: Oceanographic research papers. 97, 80–91. doi: 10.1016/j.dsr.2014.12.002
Kubryakov A. A., Stanichny S. V., Zatsepin A. G., Kremenetskiy V. V. (2016). Long-term variations of the black sea dynamics and their impact on the marine ecosystem. J. Mar. Syst. 163, 80–94.
Legrand T., Di Franco A., Ser-Giacomi E., Caló A., Rossi V. (2019). A multidisciplinary analytical framework to delineate spawning areas and quantify larval dispersal in coastal fish. Mar. Environ. Res. 151, 1–13. doi: 10.1016/j.marenvres.2019.104761
Lester S., Halpern B., Grorud-Colvert K., Lubchenco J., Ruttenberg B., Gaines S., et al. (2009). Biological effects within no-take marine reserves: a global synthesis. Mar. Ecol. Prog. Ser. 384, 33–46.
Lisovenko L. A., Andrianov D. P. (1996). Reproductive biology of anchovy (Engraulis encrasicolus ponticus Alexandrov 1927) in the Black Sea. Scientia Marina 60, 209–218.
Locarnini R. A., Mishonov A. V., Antonov J. I., Boyer T. P., Garcia H. E., Baranova O. K., et al. (2013). World Ocean Atlas 2013, Volume 1: Temperature Vol. 73. Eds. Levitus S., Mishonov A. (NOAA Atlas NESDIS). 40 pp.
Ludwig W., Dumont E., Meybeck M., Heussner S. (2009). River discharges of water and nutrients to the mediterranean and black sea: Major drivers for ecosystem changes during past and future decades? Prog. Oceanography 80 (40271), 199–217.
Macpherson E., Raventos N. (2006). Relationship between pelagic larval duration and geographic distribution of mediterranean littoral fishes. Mar. Ecol. Prog. Ser. 327, 257–265. doi: 10.3354/meps327257
Miladinova S., Macias D., Stips A., Garcia-Gorriz E. (2020). Identifying distribution and accumulation patterns of floating marine debris in the black sea. Mar. pollut. Bull. 153, 110964. doi: 10.1016/j.marpolbul.2020.110964
McQuatters-Gollop A., Mee L. D., Raitsos D. E., Shapiro G. I. (2008). Non-linearities, regime shifts and recovery: The recent influence of climate on Black Sea chlorophyll. J. Mar. Syst. 74, 649–658. doi: 10.1016/j.jmarsys.2008.06.002
Melnikov V., Chudinovskih E., Silakov M., Fedirko A., Masevich A., Serebrennikov A. (2024). The influence of the Black Sea rim current and the coastal quasi-stationary anticyclonic Eddie on the distribution of ichthyoplankton and jellyfish. Regional Stud. Mar. Sci. 75, 103565. doi: 10.1016/j.rsma.2024.103565
Moffitt E. A., Botsford L. W., Kaplan D. M., O’Farrell M. R. (2009). Marine reserve networks for species that move within a home range. Ecol. Appl. 19, 1835–1847.
Moffitt E. A., White J. W., Botsford L. W. (2011). The utility and limitations of size and spacing guidelines for designing marine protected area networks. Biol. Conserv. 144, 306–318.
Monroy P., Rossi V., Ser-Giacomi E., Lopez C., Hernández-Garcia E. (2017). Sensitivity and robustness of larval connectivity diagnostics obtained from lagrangian flow networks. ICES J. Mar. Sci. 74 (6), 1763–1779. doi: 10.1093/icesjms/fsw235
Niermann U., Bingel F., Gorban A., Gordina A. D., Gucu A. C., Kideys A. E., et al. (1994). Distribution of anchovy eggs and larvae (Engraulis encrasicolus Cuv.) in the Black Sea in 1991–1992. ICES J. Mar. Sci.: J. du Conseil 51, 395–406. doi: 10.1006/jmsc.1994.1041
Oguz T., Aubrey D. G., Latun V. S., Demirov E., Koveshnikov L., Sur H. I., et al. (1994). Mesoscale circulation and thermohaline structure of the Black Sea observed during HydroBlack’91. Deep Sea Res. Part I: Oceanogr. Res. Papers 41, 603–628. doi: 10.1016/0967-0637(94)90045-0
Oguz T., Latun V. S., Latif M. A., Vladimirov V. V., Sur H. I., Markov A. A., et al. (1993). Circulation in the surface and intermediate layers of the Black sea. Deep Sea Res. Part Oceanogr. Res. Pap. 40, 1597–1612. doi: 10.1016/0967-0637(93)90018-X
Oguz T. (2005). Black sea ecosystem response to climatic teleconnections. Oceanography 18 (2), 122–133. doi: 10.5670/oceanog.2005.47
Oguz T. (2017). Controls of multiple stressors on the black sea fishery. Front. Mar. Sci. 4. doi: 10.3389/fmars.2017.00110
Oguz T., Besiktepe S. (1999). Observations on the rim current structure, CIW formation and transport in the western black sea. Deep-Sea Res. Part I: Oceanogr. Res. Pap. 46, 1733–1753. doi: 10.1016/S0967-0637(99)00028-X
Ojaveer E. A. (1981). The effect of temperature and salnitiy on the embryonic-development of the baltic herring clupea-harengus membras l. Biologiya Morya-Marine Biol. 5, 39–48.
Özsoy E., Ünlüata Ü. (1997). Oceanography of the Black sea: A review of some recent results. Earth-Sci. Rev. 42, 231–272. doi: 10.1016/S0012-8252(97)81859-4
Ozturk B., Fach B. A., Keskin C., Arkin S., Topaloglu B., Ozturk A. A. (2017). Prospects for marine protected areas in the Turkish Black sea. Manage. Mar. Protected Areas: A Netw. Perspect., 247.
Patti B., Torri M., Cuttitta A. (2020). General surface circulation controls the interannual fluctuations of anchovy stock biomass in the Central Mediterranean Sea. Sci. Rep. 10, 1554. doi: 10.1038/s41598-020-58028-0
Romagnoni G., Kvile K. Ø., Dagestad K.-F., Eikeset A.M., Kristiansen T., Stenseth N., et al. (2020). Influence of larval transport and temperature on recruitment dynamics of North Sea cod (Gadus morhua) across spatial scales of observation. Fish Oceanogr. 29, 324–339. doi: 10.1111/fog.12474
Rossi V., Ser-Giacomi E., Lopez C., Hernández-Garcia E. (2014). Hydrodynamic provinces and oceanic connectivity from a transport network help designing marine reserves. Geophys. Res. Lett. 41 (8), 2883–2891. doi: 10.1002/2014gl059540
Roughan M., Macdonald H. S., Baird M. E., Glasby T. M. (2011). Modelling coastal connectivity in a western boundary current: Seasonal and inter-annual variability. Deep Sea Res. Part II: Topical Stud. Oceanography 58 (5), 628–644. doi: 10.1016/j.dsr2.2010.06.004
Sabatés A., Palomera I. (1987). Repartition des larves du rouget de vase (Mullus barbatus, l) le long de la cote catalane (Méditerranée occidentale). Vie Milieu 37 (3/4) 207, – 214.
Sadighrad E., Fach B. A., Arkin S. S., Salihoğlu B., Hüsrevoğlu Y. S. (2021). Mesoscale eddies in the Black sea: characteristics and kinematic properties in a high-resolution ocean model. J. Mar. Syst. 223, 103613. doi: 10.1016/j.jmarsys.2021.103613
Salihoglu B., Arkin S. S., Akoglu E., Fach B. A. (2017). Evolution of future black sea fish stocks under changing environmental and climatic conditions. Front. Mar. Sci. 4. doi: 10.3389/fmars.2017.00339
Satilmis H. H., Sumer C., Ozdemir S., Bayrakli B. (2014). Length-weight relationships of the three most abundant pelagic fish species caught by mid-water trawls and purse seine in the black sea. Cahiers Biologie Mar. 55 (2), 259–265.
Satilmis H. H., Gordina A. D., Bat L., Bircan R., Culha M., Akbulut M., et al. (2003). Seasonal distribution of fish eggs and larvae off Sinop (the southern Black Sea) in 1999-2000. Acta Oecol. 24. doi: 10.1016/S1146-609X(03)00022-5
Scientific, Technical and Economic Committee for Fisheries (STECF). (2017). Assessment of Black Sea stocks (STECF 17-14), EUR 25309 EN, JRC 85367. 2017. (Luxembourg: Publications Office of the European Union), 498 pp.
Shapiro G. I., Stanichny S. V., Stanychna R. R. (2010). Anatomy of shelf–deep sea exchanges by a mesoscale eddy in the north west black sea as derived from remotely sensed data. Remote Sens. Environ. 114 (4), 867–875. doi: 10.1016/j.rse.2009.11.020
Sorokin Y. A. (2002). “The Black Sea: ecology and oceanography,” in Biology of Inland Waters (Backhuys, Leiden, The Netherlands).
Staneva J. V., Dietrich D. E., Stanev E. V., Bowman M. J. (2001). Rim current and coastal eddy mechanisms in an eddy-resolving black sea general circulation model. J. Mar. Syst. 31, 137–157. doi: 10.1016/S0924-7963(01)00050-1
Sur H.İ., Özsoy E., Ünlüata Ü. (1994). Boundary current instabilities, upwelling, shelf mixing and eutrophication processes in the Black sea. Prog. Oceanogr. 33, 249–302. doi: 10.1016/0079-6611(94)90020-5
Torri M., Corrado R., Falcini F., Cuttitta A., Palatella L., Lacorata G., et al. (2018). Planktonic stages of small pelagic fishes (Sardinella aurita and Engraulis encrasicolus) in the central Mediterranean Sea: The key role of physical forcings and implications for fisheries management. Prog. Oceanogr. 162, 25–39. doi: 10.1016/j.pocean.2018.02.009
Vinogradov M., Shushkina E., Mikaelyan A., Nezlin N. P. (1999). “Temporal (seasonal and interannual) changes of ecosystem of the open waters of the Black Sea,” in Environmental Degradation of the Black Sea: Challenges and Remedies. Ed. Beşiktepe S., et al (Springer: Kluwer Academic Publishers), 109–129.
Wood S., Paris C. B., Ridgwell A., Hendy E. J. (2014). Modelling dispersal and connectivity of broadcast spawning corals at the global scale. Global Ecol. Biogeogr. 23, 1–11. doi: 10.1111/geb.12101
Xue H., Incze L., Xu D., Wolff N., Pettigrew N. (2008). Connectivity of lobster populations in the coastal gulf of maine part i: Circulation and larval transport potential. Ecol. Model. 210 (1-2), 193–211. doi: 10.1016/j.ecolmodel.2007.07.024
Yunev O. A., Carstensen J., Moncheva S., Khaliulin A., Aertebjerg G., Nixon S. (2007). Nutrient and phytoplankton trends on the western Black Sea shelf in response to cultural eutrophication and climate changes. Estuarine Coast. Shelf Sci. 74, 63–76. doi: 10.1016/j.ecss.2007.03.030
Zatsepin A. G. (2003). Observations of Black sea mesoscale eddies and associated horizontal mixing. J. Geophys. Res. 108, 3246. doi: 10.1029/2002JC001390
Zatsepin A., Kubryakov A., Aleskerova A., Elkin D., Kukleva O. (2019). Physical mechanisms of submesoscale eddies generation: evidences from laboratory modeling and satellite data in the Black sea. Ocean Dyn. 69, 253–266. doi: 10.1007/s10236-018-1239-4
Keywords: connectivity, larval dispersal and retention, Black Sea, cross-shelf transport, anchovy (Engraulis encrasicolus), sprat (Sprat sprattus)
Citation: Fach BA, Cagdas B, Arkin SS, Salihoglu B and Tezcan D (2024) Investigating the impact of cross-shelf transport and local retention in the Black Sea Rim Current system on small pelagic fishes. Front. Mar. Sci. 11:1435556. doi: 10.3389/fmars.2024.1435556
Received: 20 May 2024; Accepted: 19 August 2024;
Published: 25 September 2024.
Edited by:
Stylianos (Stelios) Somarakis, Institute of Marine Biological Resources and Inland Waters, GreeceReviewed by:
Kostas Tsiaras, Hellenic Centre for Marine Research (HCMR), GreeceBernardo Patti, National Research Council (CNR), Italy
Copyright © 2024 Fach, Cagdas, Arkin, Salihoglu and Tezcan. This is an open-access article distributed under the terms of the Creative Commons Attribution License (CC BY). The use, distribution or reproduction in other forums is permitted, provided the original author(s) and the copyright owner(s) are credited and that the original publication in this journal is cited, in accordance with accepted academic practice. No use, distribution or reproduction is permitted which does not comply with these terms.
*Correspondence: Bettina A. Fach, bettina@metu.edu.tr