- 1Department of Life Science and Biotechnology, Soonchunhyang University, Asan, Republic of Korea
- 2Research Group of Tidal Flats, Gyeonggi-do Maritime and Fisheries Resources Research Institute, Ansan, Republic of Korea
Introduction: The construction of coastal industrial zones has significantly impacted the marine environment, with the extent of these effects varying based on the type of industrial activity. This study compares the environmental impact of two prevalent types of industrial zones along South Korea’s coast: ironworks and shipyards.
Methods: We assessed heavy metal pollution near these industrial zones using the Pollution Load Index (PLI) and Nemerow Pollution Index (Pn). To evaluate the impact of heavy metals and organic matter on macrobenthic communities, we employed redundancy analysis (RDA) and Spearman correlation analysis. Additionally, we used the AZTI’s Marine Biotic Index (AMBI), Multivariate AMBI (M-AMBI), and Benthic Pollution Index (BPI) to gauge the ecological quality of the affected marine environments.
Results and discussion: Our findings indicated that the biodiversity and abundance of species near shipyards were significantly lower than those near ironworks. Results of PLI and Pn revealed that the ecological risk posed by heavy metals from shipyard activities was significantly higher than from ironworks. The AZTI’s Marine Biotic Index (AMBI), Multivariate AMBI (M-AMBI), and Benthic Pollution Index (BPI) demonstrated that the ecological quality of Asan Bay (ironworks) is better than that of Dangdong Bay (shipyard). RDA analysis identified Lumbrineris longifolia, Ancistrosyllis hanaokai, and Theora fragilis as biological indicators for assessing heavy metal and organic matter pollution. Spearman correlation analysis indicated that BPI and species richness index are effective indicators for evaluating such pollution. Overall, the negative impact of shipyards on the marine environment was significantly more significant than that of ironworks. Our study provides valuable insights for the South Korean government in managing coastal industrial zones and formulating relevant policies.
1 Introduction
As the global population and economy grow, coastal environments are increasingly affected by various human activities, including water pollution, air pollution, heavy metal pollution, and noise pollution caused by coastal industrial zones (Gao et al., 2024; Xu et al., 2024; Yu et al., 2021). Among these, heavy metal pollution from coastal industrial zones to the surrounding environment has become a widely acknowledged issue (Li et al., 2012). Coastal areas often serve as hubs for significant industrial activity, where facilities may release heavy metals, such as As, Cd, and Pb, during their production processes (El Zrelli et al., 2015). These heavy metals can enter the environment through wastewater, exhaust gases, and solid waste. Despite extensive studies on heavy metal pollution caused by coastal industrial zones (Hu et al., 2017), few have focused on the varying impacts of different types of coastal industrial zones on the environment and macrobenthos.
Numerous methods, such as the utilization of plankton, seagrass, and fish populations (Danovaro et al., 2016; Montefalcone, 2009), have been developed to assess the status of marine ecosystems. Due to their limited mobility and long life cycles, Macrobenthos are often considered effective bioindicators for assessing human impacts on the marine environment as they reflect long-term environmental changes (Dong et al., 2021). Methods based on macrobenthos are widely applied in assessing coastal ecosystems worldwide, and their effectiveness has been extensively validated (Dong et al., 2023; Liang et al., 2024b). To effectively manage and protect the marine environment, accurately assessing the state of the marine ecosystem is crucial (Liang et al., 2024a; Mulik et al., 2020).
Shipyards and ironworks in South Korea primarily dominate coastal industrial zones. Heavy metal contamination is the primary pollution from shipyards and ironworks to the marine environment (Armiento et al., 2022; Sprovieri et al., 2020). Different types of coastal industrial zones have varied impacts on the marine environment (Zhai et al., 2020). This study addresses this gap by investigating the environmental characteristics of two industrial zones in South Korea, Asan Bay (ironworks) and Dangdong Bay (shipyard). Furthermore, heavy metals can bioaccumulate and undergo biomagnification through the food chain, intensifying their ecological consequences (Szynkowska et al., 2018; Stankovic et al., 2012). A historical example of such impact is the large-scale mercury poisoning incident in Minamata, Japan, in the 1950s. The local populace was affected by consuming mercury-containing seafood (Budnik and Casteleyn, 2019). Heavy metal pollution can significantly impact benthic communities, reducing biodiversity and inhibiting growth and reproductive capabilities of benthos (Zhou et al., 2008; Todd et al., 2010).
In South Korea, the steel and shipbuilding industries are pillars of the national economy (Khayyat, 2015). Although South Korea’s shipbuilding industry holds a significant position globally, the marine environment surrounding shipyards has suffered irreversible impacts (Shim et al., 2000). For example, sediments near South Korean shipyards contain high concentrations of tributyltin (Shim et al., 2002). In South Korea, most studies have indicated that the structure of benthic communities near coastal industrial zones is influenced by human activities (Bae et al., 2018; Kim et al., 2020). However, research using biotic and heavy metal indices to compare different types of coastal industrial zones is lacking. Although significant progress has been made in the study of benthic fauna near coastal industrial zones, particularly in shipyards (Chatzinikolaou et al., 2021; Rebai et al., 2022), limited research has compared benthic fauna in different types of coastal industrial zones (Borja et al., 2003).
The objectives of this study were to: (1) assess chemical oxygen demand (COD), ignition loss (IL), and heavy metal concentrations in Asan Bay (ironworks) and Dangdong Bay (shipyard), (2) analyse macrobenthic community structures in these two bays, (3) evaluate ecological quality of each bay using benthic indices and assess ecological risks using heavy metal indices, and (4) examine the relationship between macrobenthos and heavy metal pollution.
2 Materials and methods
2.1 Study area
Asan Bay is located in the central part of the West Sea in South Korea. It covers an area of approximately 100 km2. It is a crucial industrial and logistics hub for Gyeonggi Province, South Korea (Park et al., 2021). The average tidal range in Asan Bay is 6.1 m. Industrialisation and urbanisation proximate to Asan Bay have contributed to the degradation of its marine ecosystem (Kim et al., 2021). The study area is located in the northern part of Asan Bay, near Ironworks.
Dangdong Bay is located in the inner western part of Jinhae Bay. It is a semi-enclosed bay (Kim and Lee, 2009). An industrial complex has developed around the bay. There is a large-scale submersible aquaculture facility within the bay itself. As a result, there has been an increase in the influx of organic materials, which accumulate in the bottom sediments, causing severe organic pollution. Additionally, the formation of hypoxic water masses during the summer has raised concerns about their adverse impacts on this region’s marine environment and ecosystems (Park et al., 2000; Yang and Shin, 2020). The study area is on the western side of Dangdong Bay, adjacent to an industrial zone predominantly occupied by the shipyard. It is important to note that this region is primarily dominated by the shipbuilding industry.
2.2 Sample collection
Dangdong Bay, located in the South Sea of Korea, experiences significant environmental changes due to seasonal factors. In autumn, typhoons cause large influxes of freshwater into Dangdong Bay, and low temperatures alter the macrobenthic community structure in winter. In contrast, spring marks the period for macrobenthic reproduction and settlement, and the high temperatures in summer favour their growth. Consequently, spring and summer provide a more accurate reflection of the impact of industrial zones on the macrobenthic community compared to autumn and winter.
Sampling surveys were conducted at ten stations (A1-A10) in Asan Bay during the spring (February) and summer (Jun) of 2011, and at six stations (D1-D6) in Dangdong Bay during the spring (February) and summer (Jun) of 2016 (Figure 1). Two macrobenthic and one sediment samples were collected by Peterson grab sampler (0.1 m2) at each station. In the field, macrobenthic samples were processed using a 0.5 mm mesh size sieve and subsequently preserved in a 10% formalin solution. Sediment samples were preserved in a freezer at -20°C for transportation to the laboratory.
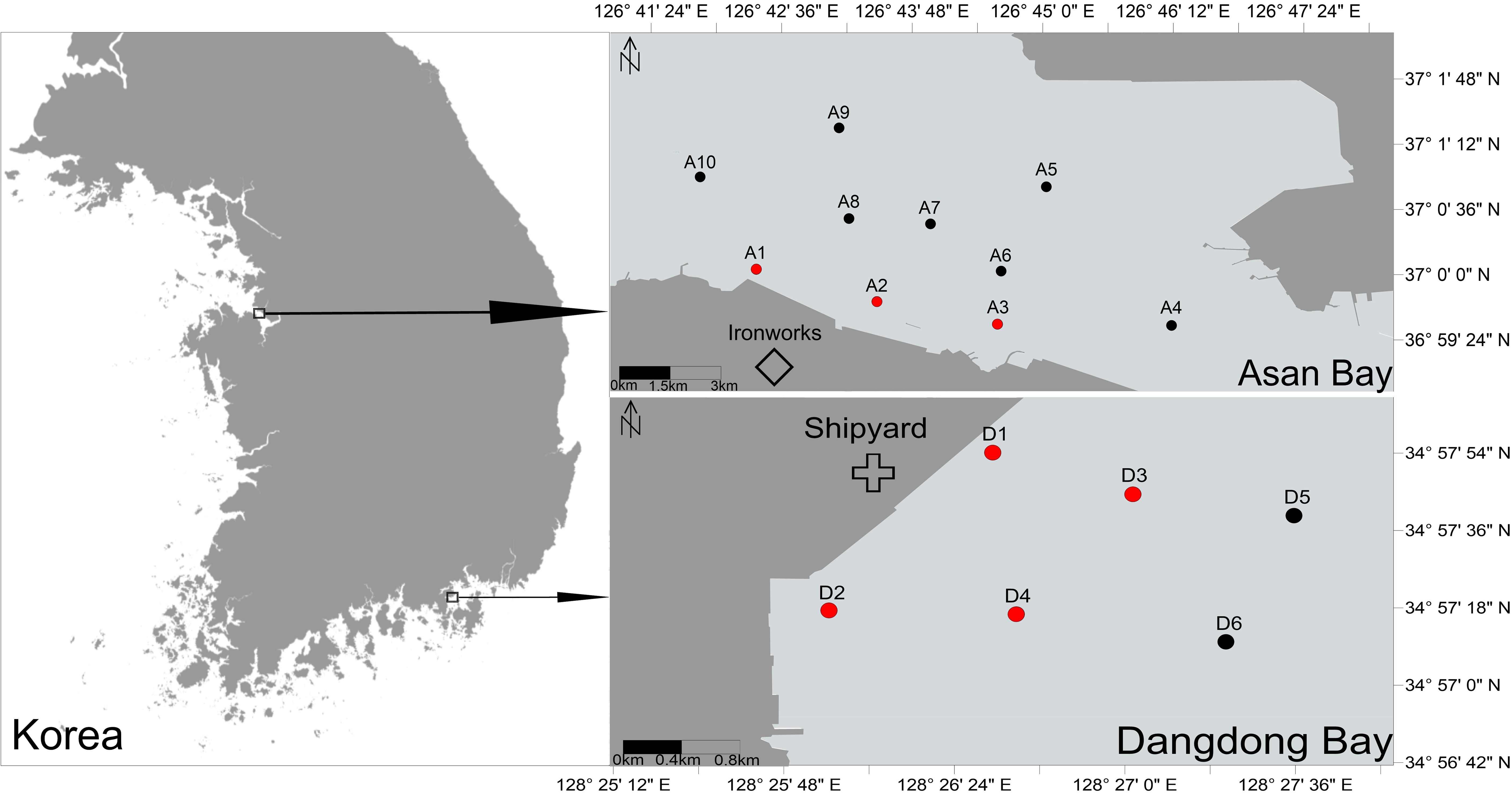
Figure 1. Sampling stations and study area in South Korea. Red stations are within a 1 km radius of industrial zones.
2.3 Sample processing
In the laboratory, macrobenthos were identified at the species level using an Olympus SZX-12 microscope. To measure the ignition loss, 10 g of sediment samples were dried for 48 hours at 60°C and heated in a muffle furnace at 550°C for 2 hours. To evaluate the COD content in sediment samples, the samples are mixed with a potassium permanganate and sodium hydroxide solution and then heated in a water bath for 1 hour. After cooling, potassium iodide and sodium azide are added to the mixture. The resulting solution is then filtered using filter papers. Sulfuric acid is added to the filtrate and titrated with sodium thiosulfate. The detection limits for IL and COD are 0.05% and 0.005 mg/g, respectively. To evaluate the concentrations of five heavy metals (As, Cd, Cu, Pb, Zn) in sediment samples, the samples were digested in a polytetrafluoroethylene (PTFE) container using a mixed acid solution (HNO3: HClO4: HF = 2:1:2) and heated to 130°C. The samples were then dissolved in a 2% HNO3 solution for analysis using an inductively coupled plasma mass spectrometer (JP-7900 mode, Agilent Technologies, USA). The typical detection limit for an ICP-MS instrument for five heavy metals is 1 μg/kg. All analyses were performed in triplicate for each sediment sample. All analytical methods followed the Marine Environmental Process Test Method (National Institute of Fisheries Science, 2010).
2.4 Dominance index
The dominance index (Y) quantifies the prevalence of dominant species within a specific sampling zone, assigning values of 0.02 or higher. It was calculated using the following formula: Y = (ni/N) × fi, where ‘N’ denoted the total number of individuals across all species, ‘ni’ was the number of individuals of the ith species, and ‘fi’ was the occurrence frequency of the ith species at study area (Xu and Chen, 1989).
2.5 Heavy metal indices
The pollution load index (PLI) and Nemerow pollution index (Pn) are widely used to assess the extent of heavy metal contamination in marine sediments (Liang et al., 2024c). Background concentrations are considered when calculating the two heavy metal indices (Table 1). However, when calculating the Nemerow pollution index (Pn), the highest ratio of heavy metal concentrations in the study area to the geochemical background values was also considered (Borah and Deka, 2023; Zhao et al., 2023). Determining geochemical background values for coastal areas of Korea was based on a study by Woo et al., 2019. Formulas and threshold values for the two heavy metal indices are shown in Table 1. Background concentrations for five heavy metals are shown in Table 2.
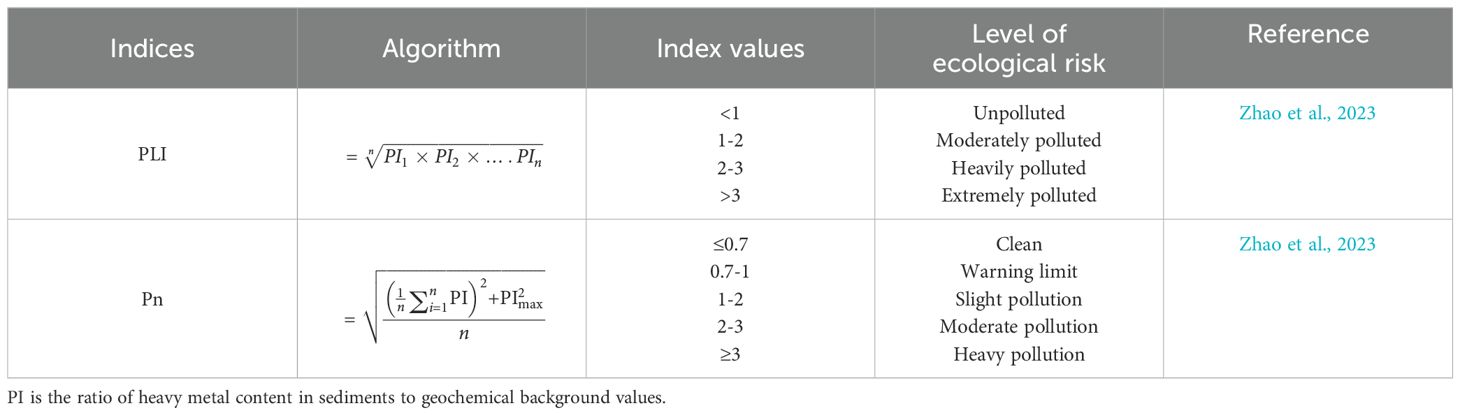
Table 1. Algorithmic methods for assessing heavy metals and categorizing ecological risk levels using heavy metal indices.
2.6 Benthic indices
The Azti’s Marine Biotic Index (AMBI) and Multivariate AMBI (MAMBI) are the most widely used benthic indices globally (Borja et al., 2019). They have also been applied to the Korean coast (Jung et al., 2014). The Benthic Pollution Index (BPI) was developed based the ecosystems of the West Sea in Korea. It has been widely applied along the Korean coast (Seo et al., 2014). The AMBI classifies macrobenthos into five ecological groups based on their tolerance to organic matter (Borja et al., 2000). Multivariate AMBI (M-AMBI) was calculated based on a factor analysis of AMBI, Shannon-wiener index, and species richness (Muxika et al., 2007). The BPI classifies macrobenthos into four functional groups based on their life history (Ryu et al., 2016).
Ecological groups in AMBI were assigned using the database of AMBI software version 6.0. For species not present in the database, we referred to the classification of similar species within the same genus or family (Liang et al., 2024c) (Supplementary Table S2). For M-AMBI, reference conditions enhanced the highest Shannon-wiener index and species richness by 15% for two bays (Borja et al., 2008). The assignment of functional groups in the BPI was based on a study of Seo et al., 2014 (Supplementary Table S2). When the number of species was less than four, a value of 6 was assigned to AMBI and a value of 0 was assigned to both MAMBI and BPI. Calculation formulas for the three benthic indices and the ecological quality status (EcoQs) threshold values are shown in Table 3.
2.6 Data analysis
Redundancy analysis (RDA) was employed to assess correlations between dominant species, number of species, and abundance of species with environmental factors using Canoco 5 software (http://www.canoco5.com). Spearman’s rank correlation analysis was used to evaluate correlations between benthic indices, heavy metal indices, species indices, and environmental factors using SPSS 29 software (SPSS Inc., USA). The species richness index (d), Pielou’s evenness index (J’), and Shannon-wiener index (H’) were calculated with Primer 7 software (PRIMER-E, NZL). The abundance of species data was transformed using the log(x+1) method before conducting cluster analyses. Analysis of similarities (ANOSIM) was used to evaluate differences in community composition among groups using PRIMER 7 software (PRIMER-E, NZL).
Independent samples t-test and Mann-Whitney U test were employed to evaluate differences in environmental factors, heavy metal indices, species indices, benthic indices, and species abundance between two bays and between areas near industrial zones versus those further away using SPSS 29 software. The Gidding method was used to create spatial distribution maps for heavy metal indices, species indices, benthic indices, species abundance, and the number of species using Surfer 14 software (Golden Software Inc., USA). In addition, principal component analysis (PCA) was employed to assess the environmental characteristics of the two bays. Prior to the analysis, the environmental data were log-transformed using log(x+1) and normalized. PRIMER 7 software (PRIMER-E, NZL). was utilized for this analysis.
3 Results
3.1 Environmental characteristic
Environmental factors at each station are shown in Supplementary Table S3. In the Mann-Whitney U test and the independent samples t-test, most environmental factors (except for As) exhibited significant differences between the two bays (P ≤ 0.05). In Dandong Bay, the ignition loss (IL) showed significant differences between stations near industrial zones and those situated farther away (t-test, P ≤ 0.05).
The range and mean values of environmental factors are shown in Table 4. The COD value exhibited the highest coefficient of variation in Asan Bay. Heavy metals in sediments were found in the following descending order of concentration: zinc (Zn), lead (Pb), copper (Cu), cadmium (Cd), and arsenic (As). In Dandong Bay, Pb concentrations at most stations exceeded effects range-low (Table 2; Supplementary Table S3).
In principal component analysis, the PC1 axis accounted for 78% of the total variance in environmental variables, while the PC2 axis explained 11% of the total variance (Figure 2). All stations in Dandong Bay were located on the right side of the principal component analysis plots (Figure 2), indicating that Dandong Bay had higher concentrations of COD, IL, and heavy metals than Asan Bay (Supplementary Table S4).
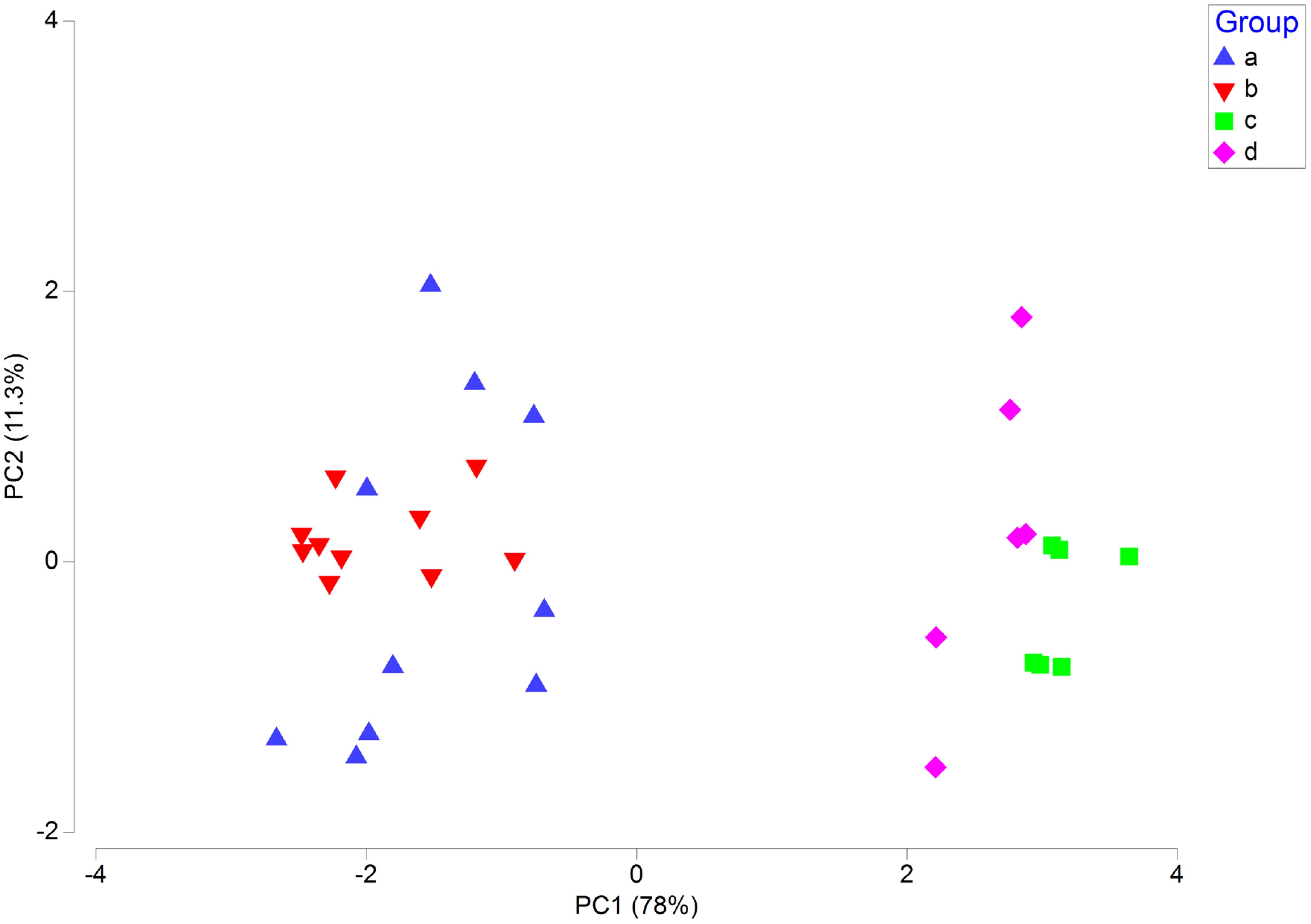
Figure 2. Principal component analysis for environmental factors in two bays. (A) station in Asan Bay in spring; (B) station in Asan Bay in summer (C) station in Dangdong Bay in spring; (D) station in Dangdong Bay in summer.
3.2 Macrobenthic community characteristics and species indices
A total of 141 macrobenthic species were identified from the two bays. Among these, species belonging to the Annelida phylum were the most numerous, totalling 80 species or 56.7% of the overall count. Following Annelida, Mollusca, Arthropoda, and Echinodermata were represented by 28 (19.9%), 23 (16.3%), and six (4.3%) species, respectively. There were four (2.8%) other animal species (Figure 3). Overall, Asan Bay exhibited the highest diversity during the spring, with up to 100 species. In contrast, in the summer, Dandong Bay recorded the lowest biodiversity, with only 16 species observed (Figure 3). There are four and three dominant species in Asan Bay and Dandong Bay, respectively. The highest dominance value of Amphiodia craterodmeta in Asan Bay was 0.2 and the highest dominance value of Lumbrineris longifolia in Dangdong Bay was 0.4 (Table 5). Cluster analysis results for macrobenthic assemblages in the two bays are depicted in Figure 4. At a 26% similarity level, the macrobenthic species were divided into five groups. Analysis of similarity (ANOSIM) showed differences in community composition among the five groups (R = 0.912, significance level of sample statistic = 0.1%).
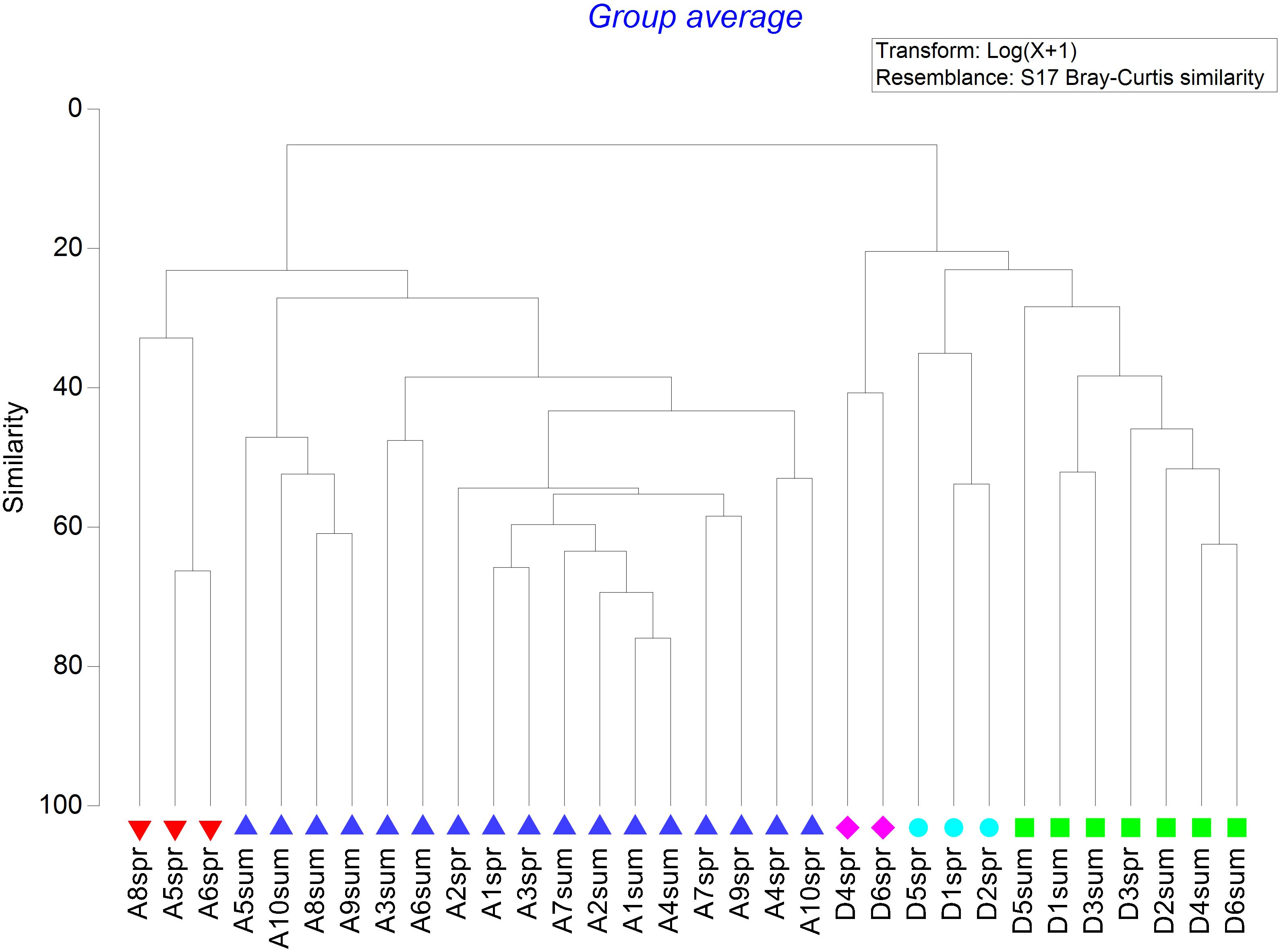
Figure 4. Cluster analyses of macrobenthic assemblages of sampling stations in two bays. (A) Asan Bay; (D) Dangdong Bay; Spr, spring; Sum, summer.
The average species abundance was 2225. 75 ± 1597.3 ind./m2 in Asan Bay and 237.1 ± 315.5 ind./m2 in Dangdong Bay. The highest species abundance was observed at Asan Station 6 in the summer, while the lowest was at Dandong Bay Station 1 in the summer (Supplementary Table S5). The species abundance significantly differed between the two bays (t-test, P ≤ 0.05). However, the abundance of species in Asan Bay had significant differences between stations near industrial zones and those situated farther away (t-test, P ≤ 0.05). Spatial distributions of abundance of species and number of species in two bays are shown in Figure 5.
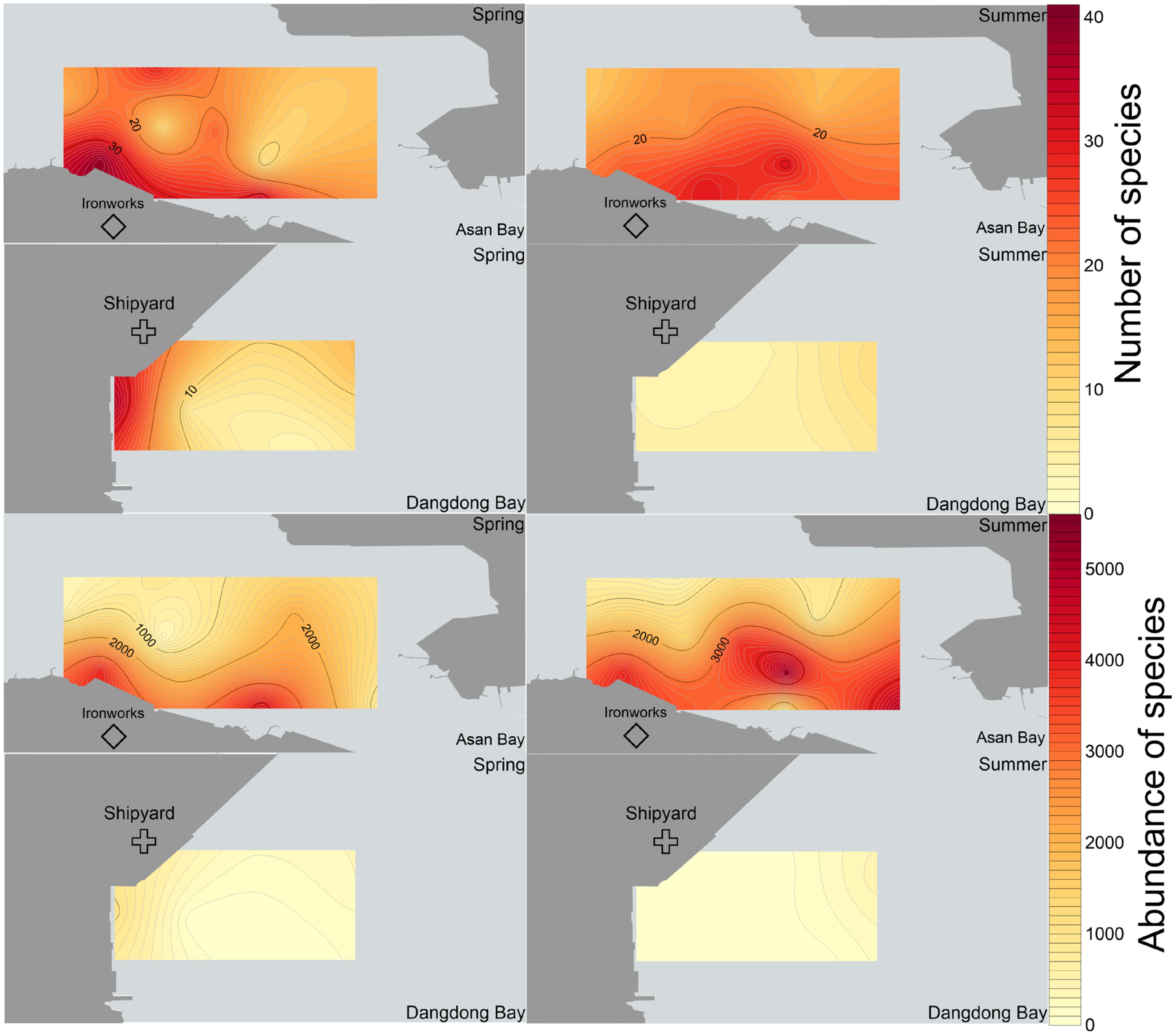
Figure 5. Spatial distribution of abundance of species, number of species, and species indices in two bays.
In Asan Bay, average values of the species richness index (d), Pielou’s evenness index (J’), and Shannon-wiener index (H’) were 2.72 ± 0.97, 0.63 ± 0.21, and 2.72 ± 0.94, respectively. In Dangdong Bay, average values of the Species richness index (d), Pielou’s evenness index (J’), and (H’) were 1.58 ± 1.28, 0.79 ± 0.14, and 2.12 ± 0.68, respectively. There were no significant differences in species indices between the two bays or between stations near industrial zones and those farther away. Spatial distributions of species indices in the two bays are shown in Figure 6. The values of species indices at each station are shown in Supplementary Table S5.
3.3 Heavy metal indices
Average values of PLI and Pn were 0.61 ± 0.07 and 6.5 ± 1.67, respectively, in Asan Bay and 1.24 ± 0.11 and 84.41 ± 34.1, respectively, in Dangdong Bay. PLI values of all stations showed that Asan Bay was unpolluted in spring and summer but moderately polluted in Dangdong Bay in spring and summer. Pn values of all stations showed heavy pollution in Asan Bay and Dangdong Bay in spring and summer. Values of PLI and Pn exhibited significant differences between the two bays (U-test, P ≤ 0.05). There were no significant differences in values of PLI or Pn between stations near industrial zones and those situated farther away in two bays. Overall, Dandong Bay exhibited the highest values of PLI and Pn in spring (Figure 7). The values of heavy metal indices at each station are shown in Supplementary Table S6.
3.4 Benthic biotic indices
In Asan Bay, average AMBI, MAMBI, and BPI values were 1.72 ± 1.03, 0.6 ± 0.15, and 64.39 ± 22.47, respectively. In Dangdong Bay, average AMBI, MAMBI, and BPI values were 2.82 ± 1.52, 0.49 ± 0.26, and 20.29 ± 15.01, respectively. According to AMBI assessment criteria, EcoQs were high for eight stations, good for twenty stations, moderate for two stations, and bad for two stations in the two bays. According to the MAMBI assessment criteria, EcoQs were high for three stations, good for seventeen stations, moderate for eight stations, poor for two stations, and bad for two stations in the two bays. According to the BPI assessment criteria, EcoQs were high for twelve stations, good for four stations, moderate for five stations, poor for four stations, and bad for seven stations in the two bays. Values of BPI exhibited significant differences between the two bays (U-test, P ≤ 0.05). Asan Bays, AMBI, MAMBI, and BPI values exhibited significant differences between stations near industrial zones and those farther away (U-test, P ≤ 0.05).
Despite variations in EcoQS assessments at certain stations based on three benthic indices, Asan Bay generally exhibited superior EcoQS to Dangdong Bay. In Asan Bay, areas closer to the industrial zone consistently showed better EcoQS than those farther away during spring and summer. In contrast, in Dangdong Bay, the EcoQS near the industrial zone was superior in spring, whereas regions further from the industrial zone demonstrated improved EcoQS in summer (Figure 8). The values of benthic indices and EcoQs at each station are shown in Supplementary Table S7.
3.5 Redundancy and correlation analysis
In redundancy analysis (RDA), contributions of IL, As, Pb, COD, Zn, Cu, and Cd were 68.7%, 10.7%, 5.6%, 6.5%, 2.7%, 3.9%, and 1.9%, respectively. Axes 1 and 2 accounted for 46.96% of the cumulative explained variation. Amphiodia craterodmeta, Heteromastus filiformis, Ampharete arctica, Corophium sp, number of species, and abundance of species exhibited negative correlations with the presence of IL, As, COD, Pb, Cu, and Zn. Conversely, Lumbrineris longifolia, Ancistrosyllis hanaokai, and Theora fragilis positively correlated with these environmental factors (Figure 9).
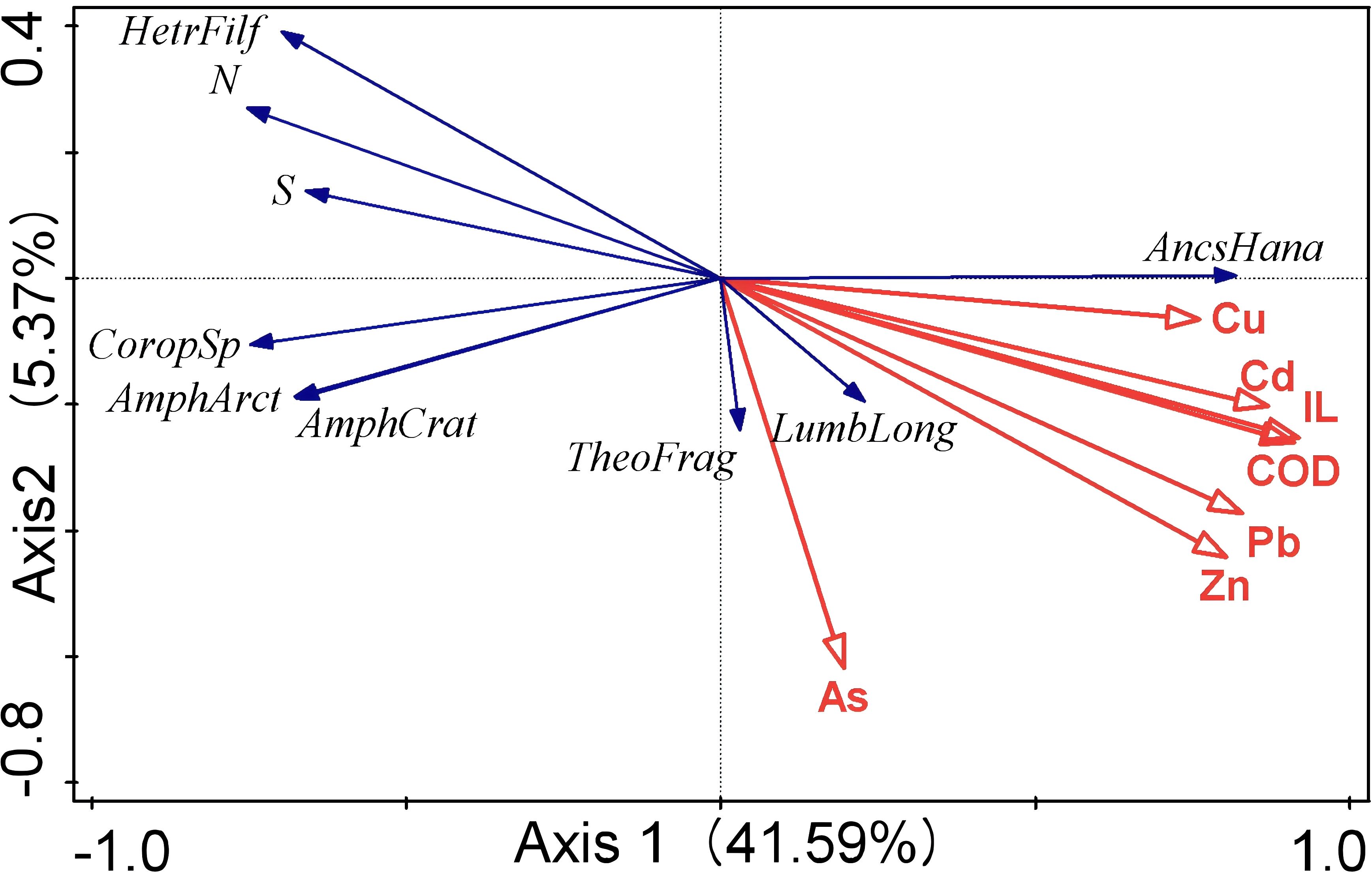
Figure 9. Redundancy analysis (RDA) of dominant species, number of species, abundance of species, species indices and environmental factors. AmphArct, Ampharete arctica; AmphCrat, Amphiodia craterodmeta; AncsHana, Ancistrosyllis hanaokai; CoropSp, Corophium sp; HetrFilf, Heteromastus filiformis; LumbLong, Lumbrineris longifolia; TheoFrag, Theora fragilis; S, number of species; N, abundance of species; COD, chemical oxygen demand; IL, ignition loss.
Four heavy metals (Pb, Zn, Cu, and Cd, excluding As) demonstrated positive correlations with IL and COD in the correlation analysis. AMBI was negatively correlated with MAMBI, BPI, and d. AMBI had positive correlations with J’. Conversely, the BPI positively correlated with MAMBI, d, and H’. BPI exhibited negative correlations with six environmental factors (COD, IL, Cd, Cu, Pu, and Zn), Pn, and PLI (Figure 10). d exhibited negative correlations with five environmental factors (COD, IL, Cd, Cu, and Pb) and Pn. J’ had a positive correlation with Zn and H’ had a negative correlation with COD. Additionally, d had a positive correlation with H’.
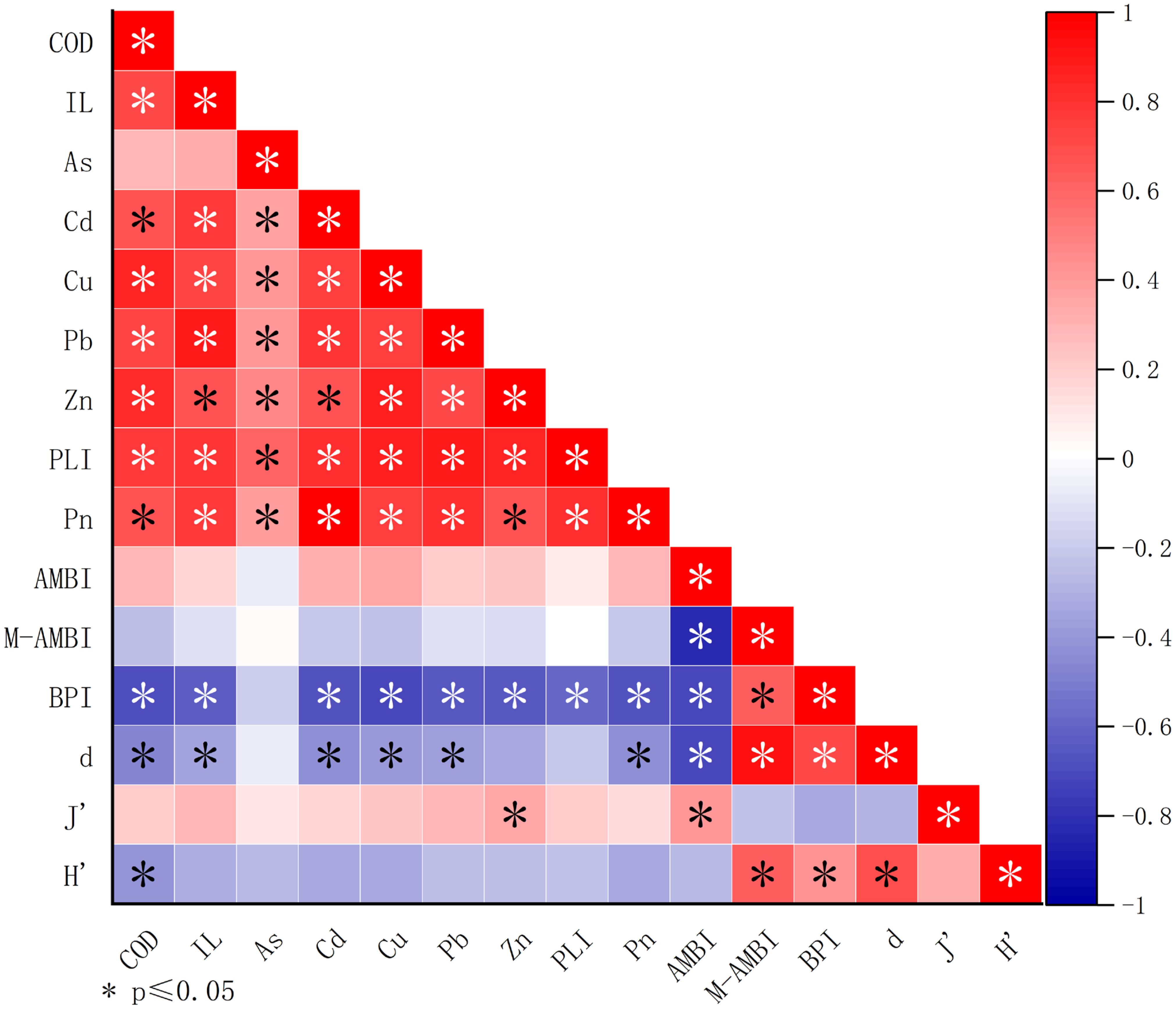
Figure 10. Correlation analysis of benthic indices, species indices, heavy metal indices, and environmental factors. COD, chemical oxygen demand; IL, ignition loss; d, species richness index; J’, pielou’s evenness index; H’, Shannon-wiener index.
4 Discussion
4.1 Environmental characteristics in Dangdong Bay and Asan Bay
In this study, we evaluated the concentrations of COD, IL, and five heavy metals (As, Cd, Cu, Pb, and Zn) in two bays. Previous research has indicated that the concentrations of these heavy metals in environments near industrial areas are higher than those in other regions (Taati et al., 2020; Wang et al., 2020), suggesting a strong association with industrial activities. Furthermore, these heavy metals are known to exhibit significant bioaccumulative properties (Trevizani et al., 2016). Specifically, Cd, Cu, Pb, and Zn have been shown to have significant biomagnification effects on aquatic invertebrates (Goodyear and McNeill, 1999).
The COD, IL, and five heavy metals concentrations were higher in Dangdong Bay compared to Asan Bay. Specifically, the average concentration of COD in Dangdong Bay (39.40 ± 0.25) was 7.7 times higher than in Asan Bay (5.11 ± 0.40), and the average concentration of IL (31.53 ± 0.17) was 8.7 times higher than in Asan Bay (3.62 ± 0.38). Regarding the five heavy metals, the average Cd concentration in Asan Bay (0.71 ± 0.12) was significantly lower than in Dangdong Bay (2.56 ± 0.22). Dangdong Bay’s coastline hosts many shipyards, which are significant sources of environmental contaminants. Various contaminants can impact sediments in the vicinity of shipyards. These contaminants typically include petroleum derivatives, antifouling paints, solvents, polycyclic aromatic hydrocarbons (PAHs), and metals, which are byproducts of vessel repair and maintenance processes (Pereira et al., 2018; Choi et al., 2014). Oyster farms have organic matter accumulation in Dandong Bay (Choi et al., 2017; Hyun et al., 2013; Bae et al., 2017). Additionally, Dangdong Bay, a semi-enclosed bay with slower water currents than Asan Bay, further contributes to organic matter accumulation. In Dangdong Bay, average concentrations of most heavy metals are higher than those found along the South Korean Coast but lower than those in Shenzhen Bay (Woo et al., 2019; Huang et al., 2018). However, average concentrations of Cd and Pb are several times higher than geochemical background values. Moreover, concentrations of Cd and Pb exceeded effects range-low values (Liang et al., 2024d) (Table 2).
In Asan Bay, the average concentrations of As (0.37 mg/kg), Cu (17.17 mg/kg), Pb (22.40 mg/kg), and Zn (44.40 mg/kg) were lower than those observed along the South Korean coast (As, 7.80 mg/kg; Cu, 14.90 mg/kg; Pb, 25.70 mg/kg; Zn, 73.8 mg/kg) and in Shenzhen Bay (Cu, 90.90 mg/kg; Pb, 58.94 mg/kg; Zn, 73.8 mg/kg) (Woo et al., 2019; Huang et al., 2018) (Supplementary Table S1). However, the average Cd concentration (0.71 mg/kg) exceeded the geochemical background concentration (0.131mg/kg) (Table 2). Despite this, concentrations of heavy metals remained below ERL values (Table 2). Trace amounts of heavy metals in emissions from ironworks will ultimately settle in the surrounding environment (Sponza and Karaoğlu, 2002; Timothy and Tagui Williams, 2019). Cho et al. (2009) have indicated that the air near the ironworks in Asan Bay contains Cr, Cd, and Pb. Additionally, there are multiple artificial lakes near Asan Bay, where a significant amount of domestic, agricultural, and industrial wastewater is discharged. Domestic, agricultural, and industrial wastewater often contain substantial amounts of heavy metals (Li et al., 2022; Shrestha et al., 2021; Saini et al., 2024), which might be one of the reasons for elevated Cd levels in Asan Bay.
Future research should aim to identify sources of Cd in sediments of Asan Bay. Average concentrations of Cd and Pb in Dangdong Bay exceeded ERL values, making it imperative to regulate shipyards in Dangdong Bay to protect the marine environment. Additionally, given the filter-feeding behaviour of oysters, they can accumulate heavy metals from their surrounding environment (Aslam et al., 2020; Chen et al., 2014; Liang et al., 2024e). Consequently, measuring heavy metal contents in oysters cultured in Dangdong Bay is necessary.
4.2 Macrobenthic community characteristics in Dangdong Bay and Asan Bay
In this survey, a total of 98 species of macrobenthos were identified in Asan Bay, while 56 were identified in Dangdong Bay. The macrobenthos in two bays was predominantly from the phylum Annelida, consistent with findings from other studies (Bae et al., 2018; Paik et al., 2008; Yu et al., 2011; Liang et al., 2024f). In Asan Bay, the echinoderm Amphiodia craterodmeta had the highest dominance value. Notably, this species was primarily found at coastal stations (A1, A2, and A4), indicating that the benthic environment of Asan Bay might be subject to influences from trawling disturbances (González-Irusta et al., 2012). Meanwhile, in Dangdong Bay, the annelid Lumbrineris longifolia was most dominant, reflecting the prevalence of opportunistic species likely due to organic and heavy metal pollution. Heavy metal pollution typically has a negative impact on most macrobenthos., while organic enrichment may enhance the growth and reproduction of certain opportunistic species (Culhane et al., 2019; Dong et al., 2021; Ryu et al., 2011). The cluster analysis and species indices revealed further deterioration of macrobenthic communities in Dandong Bay over the summer. In this region, a significant influx of nutrients from human activities and sluggish water circulation frequently leads to thermal stratification. This condition is responsible for a widespread hypoxia at the seabed during warmer months (Lee et al., 2018; Huang and An, 2022). However, due to the lack of dissolved oxygen and temperature data in this study, we believe that hypoxia in bottom seawater during summer could further contribute to the degradation of the macrobenthic community in Dangdong Bay.
Overall, the abundance and diversity of species in Dangdong Bay were significantly lower than those in Asan Bay. In Dangdong Bay, the dominant species were opportunistic species. This suggests that the benthic environment near Dangdong Bay has been severely damaged, primarily due to the impact of heavy metal and organic matter pollution from shipbuilding activities.
4.3 Ecological risk and ecological quality in Dangdong Bay and Asan Bay
PLI results indicated that Asan Bay was unpolluted concerning the ecological risk, while Dangdong Bay was assessed as moderately polluted. In contrast, PN results suggested heavy pollution in Asan Bay and Dangdong Bay. These discrepancies in ecological risk assessments of sediments in Asan Bay, as determined by PLI and PN, were attributable to differences in these indices’ calculation methods (Liang et al., 2024c, e). Dong et al. (2023) have highlighted challenges of assessing ecological risk using a single heavy metal index. Given that PLI and Pn calculations require considering geochemical background values, the absence of a unified standard in South Korea was a notable limitation. It is imperative to propose a unified standard or methodology for geochemical background values to enhance the comparability and accuracy of related research.
PLI and Pn values indicated that heavy metal pollution in Dangdong Bay was significantly higher than that in Asan Bay. Shipyards contribute to more severe heavy metal contamination in sediments than ironworks. The primary source of heavy metal pollution from ironworking activities is atmospheric deposition (Žibret and Rokavec, 2010; Li et al., 2024). However, shipbuilding operations typically involve discharging substantial amounts of heavy metals into the surrounding environment (Pereira et al., 2018; Kim et al., 2015).
Three benthic indices indicated that most stations in Asan Bay in spring and summer were classified as High and Good regarding their ecological quality status. However, in Dangdong Bay, the three benthic indices revealed contradictory classifications of ecological quality status. The BPI categorised most stations in Dangdong Bay as having a Poor or Bad ecological quality status, while AMBI and MAMBI classified most stations as High and Good. Ecological groups of AMBI are based on Europe’s marine ecology. However, life histories of macrobenthic organisms can vary across different geographical regions, which might affect the applicability or accuracy of the AMBI when used in areas outside of Europe (Wu et al., 2022). Lumbrineris longifolia is classified under EGII (disturbance-indifferent species) in the AMBI software, whereas it is classified as N4 (opportunistic species) in the BPI. This discrepancy in classification leads to differences in the assessment of the ecological quality status of Dangdong Bay by the three benthic indices. We recommend that when using the AMBI, it is necessary to recalibrate ecological groups to enhance its performance and applicability. Furthermore, other studies have indicated that setting reference conditions for the M-AMBI can also impact its applicability and performance (Dias et al., 2022; Santibañez-Aguascalientes et al., 2020; Liang et al., 2024f).
4.4 Indication of macrobenthos on heavy metal pollution
In the correlation analysis, AMBI and M-AMBI exhibited no correlation with heavy metals or heavy metal indices. In contrast, BPI correlated with most heavy metals and heavy metal indices. Lim et al. (2013) have shown that BPI yields results akin to those of the heavy metal index in assessing the ecological quality of Jinhae Bay. Similarly, in Garolim Bay and the East Sea of South Korea, AMBI and M-AMBI showed no correlation with heavy metals (Liang et al., 2024c, d). These studies suggest that AMBI and M-AMBI might not be adequate indicators of heavy metal pollution in South Korea. In contrast, BPI may serve as a more reliable indicator of heavy metal pollution.
Within species indices, d was correlated with three heavy metals and Pn, while J’ was only correlated with Zn. Despite some studies indicating that d is not a good indicator of heavy metal pollution (Zhang et al., 2023), d is a measure of biodiversity that considers the number of species and the number of individuals (Gamito, 2010). Different macrobenthos exhibit varying tolerance levels to heavy metal pollution (Hu et al., 2019; Ryu et al., 2011; Dauvin, 2008). Polychaetes typically exhibit higher tolerance to heavy metal pollution than other benthic fauna (Chen et al., 2010; Tang et al., 2021). Additionally, substantial organic matter accumulation can lead to a prolific reproduction of opportunistic species (Gray, 1989). In Dangdong Bay, d correlated with heavy metals and Pn possibly due to the abundant occurrence of opportunistic polychaete Lumbrineris longifolia. This result indicated that d could effectively indicate heavy metal pollution in areas polluted with heavy metals and organic matter.
Bivalves and polychaetes are extensively utilised to assess and detect heavy metal pollution (Giangrande et al., 2017; Kumar et al., 2022). In redundancy analysis, Lumbrineris longifolia, Ancistrosyllis hanaokai, and Theora fragilis positively correlated with heavy metals. This suggests that the three species could serve as promising bioindicators for assessing heavy metal pollution, particularly in areas surrounding shipyards.
5 Conclusions
1. COD, IL, and heavy metal contents were higher in Dangdong Bay than in Asan Bay, primarily because emissions of heavy metals and organic matter from shipyards exceeded those from ironworks. Additionally, Dangdong Bay, a semi-enclosed bay, further contributed to the accumulation of organic matter.
2. In this study, a total of 141 species of macrobenthos were identified across the two bays. Dangdong Bay exhibited a lower number of species and abundance than Asan Bay primarily due to pollution from heavy metals and organic matter. In Dangdong Bay, opportunistic species dominated the macrobenthic community. Additionally, hypoxic conditions in bottom waters during summer further contributed to the decline of benthos in this bay.
3. The three benthic indices used in this study indicate that the ecological quality of Asan Bay is superior to that of Dangdong Bay. Additionally, the two heavy metal indices suggest that the ecological risk associated with Asan Bay is lower than that of Dangdong Bay. Before using the AMBI, it is necessary to calibrate ecological groups to ensure accurate assessments of ecological quality based on specific characteristics of the local marine environment.
4. The BPI and d are effective indicators for assessing heavy metal pollution in the two bays. Lumbrineris longifolia, Ancistrosyllis hanaokai, and Theora fragilis positively correlated with heavy metals. This indicates that these species could be effective bioindicators for monitoring heavy metal pollution, especially near shipyards.
Data availability statement
The raw data supporting the conclusions of this article will be made available by the authors, without undue reservation.
Ethics statement
The manuscript presents research on animals that do not require ethical approval for their study.
Author contributions
JL: Writing – original draft, Writing – review & editing, Conceptualization, Formal analysis, Project administration, Software. C-WM: Writing – review & editing, Data curation, Funding acquisition, Project administration, Resources, Supervision, Validation, Visualization. K-BK: Conceptualization, Investigation, Writing – review & editing.
Funding
The author(s) declare financial support was received for the research, authorship, and/or publication of this article. This work was supported by the Soonchunhyang University Research Fund.
Acknowledgments
We used Grammarly software during the writing process to check the grammar and improve the manuscript's readability.
Conflict of interest
The authors declare that the research was conducted in the absence of any commercial or financial relationships that could be construed as a potential conflict of interest.
Publisher’s note
All claims expressed in this article are solely those of the authors and do not necessarily represent those of their affiliated organizations, or those of the publisher, the editors and the reviewers. Any product that may be evaluated in this article, or claim that may be made by its manufacturer, is not guaranteed or endorsed by the publisher.
Supplementary material
The Supplementary Material for this article can be found online at: https://www.frontiersin.org/articles/10.3389/fmars.2024.1433536/full#supplementary-material
References
Armiento G., Barsanti M., Caprioli R., Chiavarini S., Conte F., Crovato C., et al. (2022). Heavy metal background levels and pollution temporal trend assessment within the marine sediments facing a brownfield area (Gulf of Pozzuoli, Southern Italy). Environ. Monit. Assess. 194, 814. doi: 10.1007/s10661-022-10480-3
Aslam S., Chan M. W. H., Siddiqui G., Boczkaj G., Kazmi S. J. H., Kazmi M. R. (2020). A comprehensive assessment of environmental pollution by means of heavy metal analysis for oysters’ reefs at Hab River Delta, Balochistan, Pakistan. Mar. pollut. Bull. 153, 110970. doi: 10.1016/j.marpolbul.2020.110970
Bae H., Lee J.-H., Song S. J., Park J., Kwon B.-O., Hong S., et al. (2017). Impacts of environmental and anthropogenic stresses on macrozoobenthic communities in Jinhae Bay, Korea. Chemosphere 171, 681–691. doi: 10.1016/j.chemosphere.2016.12.112
Bae H., Lee J.-H., Song S. J., Ryu J., Noh J., Kwon B.-O., et al. (2018). Spatiotemporal variations in macrofaunal assemblages linked to site-specific environmental factors in two contrasting nearshore habitats. Environ. pollut. 241, 596–606. doi: 10.1016/j.envpol.2018.05.098
Borah G., Deka H. (2023). Crude oil associated heavy metals (HMs) contamination in agricultural land: Understanding risk factors and changes in soil biological properties. Chemosphere 310, 136890. doi: 10.1016/j.chemosphere.2022.136890
Borja A., Chust G., Muxika I. (2019). “Forever young: The successful story of a marine biotic index,” in Advances in marine biology (Elsevier, London), 93–127. doi: 10.1016/bs.amb.2019.05.001
Borja A., Dauer D. M., Díaz R., Llansó R. J., Muxika I., Rodríguez J. G., et al. (2008). Assessing estuarine benthic quality conditions in Chesapeake Bay: A comparison of three indices. Ecol. Indic. 8, 395–403. doi: 10.1016/j.ecolind.2007.05.003
Borja A., Franco J., Pérez V. (2000). A marine biotic index to establish the ecological quality of soft-bottom benthos within european estuarine and coastal environments. Mar. pollut. Bull. 40, 1100–1114. doi: 10.1016/S0025-326X(00)00061-8
Borja A., Muxika I., Franco J. (2003). The application of a Marine Biotic Index to different impact sources affecting soft-bottom benthic communities along European coasts. Mar. pollut. Bull. 46, 835–845. doi: 10.1016/S0025-326X(03)00090-0
Budnik L. T., Casteleyn L. (2019). Mercury pollution in modern times and its socio-medical consequences. Sci. Total Environ. 654, 720–734. doi: 10.1016/j.scitotenv.2018.10.408
Chatzinikolaou E., Damianidis P., Pavloudi C., Vasileiadou A., Faulwetter S., Keklikoglou K., et al. (2021). Benthic communities in three Mediterranean touristic ports: MAPMED project. Biodivers. Data. J. 9, e66420. doi: 10.3897/BDJ.9.e66420
Chen K., Tian S., Jiao J. J. (2010). Macrobenthic community in tolo harbour, hong kong and its relations with heavy metals. Estuaries. Coasts. 33, 600–608. doi: 10.1007/s12237-010-9271-8
Chen Y.-M., Li H.-C., Tsao T.-M., Wang L.-C., Chang Y. (2014). Some selected heavy metal concentrations in water, sediment, and oysters in the Er-Ren estuary, Taiwan: chemical fractions and the implications for biomonitoring. Environ. Monit. Assess. 186, 7023–7033. doi: 10.1007/s10661-014-3907-2
Cho T.-J., Jeong M.-H., Jeon J.-M., Son B.-S. (2009). A study on the concentration of fine particles and heavy metals in iron works. J. Environ. Sci. 18, 401–409. doi: 10.5322/JES.2009.18.4.401
Choi J. Y., Hong G. H., Ra K., Kim K.-T., Kim K. (2014). Magnetic characteristics of sediment grains concurrently contaminated with TBT and metals near a shipyard in Busan, Korea. Mar. pollut. Bull. 85, 679–685. doi: 10.1016/j.marpolbul.2014.03.029
Choi M., Lee I.-S., Hwang D.-W., Kim H. C., Yoon S.-P., Yun S., et al. (2017). Organic enrichment and pollution in surface sediments from jinhae and geoje-hansan bays with dense oyster farms. Korean J. Fish. Aquat. Sci. 50, 777–787. doi: 10.5657/KFAS.2017.0777
Culhane F. E., Briers R. A., Tett P., Fernandes T. F. (2019). Response of a marine benthic invertebrate community and biotic indices to organic enrichment from sewage disposal. J. Mar. Biol. Ass. 99, 1721–1734. doi: 10.1017/S0025315419000857
Danovaro R., Carugati L., Berzano M., Cahill A. E., Carvalho S., Chenuil A., et al. (2016). Implementing and innovating marine monitoring approaches for assessing marine environmental status. Front. Mar. Sci. 3. doi: 10.3389/fmars.2016.00213
Dauvin J.-C. (2008). Effects of heavy metal contamination on the macrobenthic fauna in estuaries: The case of the Seine estuary. Mar. pollut. Bull. 57, 160–169. doi: 10.1016/j.marpolbul.2007.10.012
Dias H. Q., Sukumaran S., Mulik J., Janakiram P. (2022). Ecological quality status assessment of tropical estuaries with benthic indices using differently derived reference conditions. Mar. pollut. Bull. 177, 113457. doi: 10.1016/j.marpolbul.2022.113457
Dong J.-Y., Wang X., Zhang X., Bidegain G., Zhao L. (2023). Integrating multiple indices based on heavy metals and macrobenthos to evaluate the benthic ecological quality status of Laoshan Bay, Shandong Peninsula, China. Ecol. Indic. 153, 110367. doi: 10.1016/j.ecolind.2023.110367
Dong J.-Y., Zhao L., Sun X., Hu C., Wang Y., Li W.-T., et al. (2021). Response of macrobenthic communities to heavy metal pollution in Laoshan Bay, China: A trait-based method. Mar. pollut. Bull. 167, 112292. doi: 10.1016/j.marpolbul.2021.112292
El Zrelli R., Courjault-Radé P., Rabaoui L., Castet S., Michel S., Bejaoui N. (2015). Heavy metal contamination and ecological risk assessment in the surface sediments of the coastal area surrounding the industrial complex of Gabes city, Gulf of Gabes, SE Tunisia. Mar. pollut. Bull. 101, 922–929. doi: 10.1016/j.marpolbul.2015.10.047
Gamito S. (2010). Caution is needed when applying Margalef diversity index. Ecol. Indic. 10, 550–551. doi: 10.1016/j.ecolind.2009.07.006
Gao W., Liu J., Xu Y., Li P. (2024). Evolution of sandy shores under the combined impact of global climate change and anthropogenic activities in Shandong Peninsula, East China. J. Asian Earth Sci. 259, 105887. doi: 10.1016/j.jseaes.2023.105887
Giangrande A., Licciano M., Del Pasqua M., Fanizzi F. P., Migoni D., Stabili L. (2017). Heavy metals in five Sabellidae species (Annelida, Polychaeta): ecological implications. Environ. Sci. pollut. Res. 24, 3759–3768. doi: 10.1007/s11356-016-8089-8
González-Irusta J. M., Punzón A., Serrano A. (2012). Environmental and fisheries effects on Gracilechinus acutus (Echinodermata: Echinoidea) distribution: is it a suitable bioindicator of trawling disturbance? ICES J. Mar. Sci. 69, 1457–1465. doi: 10.1093/icesjms/fss102
Goodyear K. L., McNeill S. (1999). Bioaccumulation of heavy metals by aquatic macro-invertebrates of different feeding guilds: a review. Sci. Total Environ. 229, 1–19. doi: 10.1016/S0048-9697(99)00051-0
Gray J. S. (1989). Effects of environmental stress on species rich assemblages. Biol. J. Linn. Soc Lond. 37, 19–32. doi: 10.1111/j.1095-8312.1989.tb02003.x
Hu B., Wang J., Jin B., Li Y., Shi Z. (2017). Assessment of the potential health risks of heavy metals in soils in a coastal industrial region of the Yangtze River Delta. Environ. Sci. pollut. Res. 24, 19816–19826. doi: 10.1007/s11356-017-9516-1
Hu C., Dong J., Gao L., Yang X., Wang Z., Zhang X. (2019). Macrobenthos functional trait responses to heavy metal pollution gradients in a temperate lagoon. Environ. pollut. 253, 1107–1116. doi: 10.1016/j.envpol.2019.06.117
Huang F., Xu Y., Tan Z., Wu Z., Xu H., Shen L., et al. (2018). Assessment of pollutions and identification of sources of heavy metals in sediments from west coast of Shenzhen, China. Environ. Sci. pollut. Res. 25, 3647–3656. doi: 10.1007/s11356-017-0362-y
Huang Y., An S. (2022). Weak hypoxia enhanced denitrification in a dissimilatory nitrate reduction to ammonium (DNRA)-dominated shallow and eutrophic coastal waterbody, jinhae bay, South Korea. Front. Mar. Sci. 9. doi: 10.3389/fmars.2022.897474
Hyun J.-H., Kim S.-H., Mok J.-S., Lee J. S., An S.-U., Lee W.-C., et al. (2013). Impacts of long-line aquaculture of Pacific oysters (Crassostrea gigas) on sulfate reduction and diffusive nutrient flux in the coastal sediments of Jinhae–Tongyeong, Korea. Mar. pollut. Bull. 74, 187–198. doi: 10.1016/j.marpolbul.2013.07.004
Jung R.-H., Seo I.-S., Choi M., Park S. R., Choi B.-M., Kim M. H., et al. (2014). Community structure and health assessment of macrobenthic assemblages during spring and summer in the shellfish farming ground of wonmun bay, on the southern coast of korea. Korean J. Fish. Aquat. Sci. 47, 908–926. doi: 10.5657/KFAS.2014.0908
Khayyat N. T. (2015). “History of economic development in South Korea,” in Energy demand in industry (Springer Netherlands, Dordrecht), 21–29. doi: 10.1007/978-94-017-9953-9_2
Kim B. O., Lee C. K. (2009). Estimation of historical shorelines on a coastal reclaimed land (II) : shoreline change analysis. J. Korean Soc Coast. Ocean Eng. 21, 380–390.
Kim H.-G., Song S. J., Bae H., Noh J., Lee C., Kwon B.-O., et al. (2020). Natural and anthropogenic impacts on long-term meiobenthic communities in two contrasting nearshore habitats. Environ. Int. 134, 105200. doi: 10.1016/j.envint.2019.105200
Kim K.-B., Jung Y.-J., Oh J.-K., Kang H., Son D.-S., Ma C.-W. (2021). Macrobenthic community and benthic health assessment of central area in asan bay. J. Korean Soc Fish. Mar. Sci. Educ. 33, 903–917. doi: 10.13000/JFMSE.2021.8.33.4.903
Kim N. S., Hong S. H., An J. G., Shin K.-H., Shim W. J. (2015). Distribution of butyltins and alternative antifouling biocides in sediments from shipping and shipbuilding areas in South Korea. Mar. pollut. Bull. 95, 484–490. doi: 10.1016/j.marpolbul.2015.03.010
Kumar M. R., Krishnan K. A., Vimexen V., Faisal A. K., Mohind M., Arun V. (2022). Heavy metal impression in surface sediments and factors governing the fate of macrobenthic communties in tropical estuarine ecosystem, India. Environ. Sci. pollut. Res. 29, 38567–38590. doi: 10.1007/s11356-021-18394-2
Lee J., Park K.-T., Lim J.-H., Yoon J.-E., Kim I.-N. (2018). Hypoxia in korean coastal waters: A case study of the natural jinhae bay and artificial shihwa bay. Front. Mar. Sci. 5. doi: 10.3389/fmars.2018.00070
Li Q., Liu G., Qi L., Wang H., Ye Z., Zhao Q. (2022). Heavy metal-contained wastewater in China: Discharge, management and treatment. Sci. Total. Environ. 808, 152091. doi: 10.1016/j.scitotenv.2021.152091
Li X., Liu L., Wang Y., Luo G., Chen X., Yang X., et al. (2012). Integrated assessment of heavy metal contamination in sediments from a coastal industrial basin, NE China. PLoS. One 7, e39690. doi: 10.1371/journal.pone.0039690
Li Y., Feng H., She X., Ren K., You X., Wang J., et al. (2024). Substance-flow analysis and emission-reduction strategies for thallium in the steel industry process. Process. Saf. Environ. Prot. 185, 116–126. doi: 10.1016/j.psep.2024.02.081
Liang J., Huang H.-R., Ma C.-W., Son D.-S., Kim S.-K. (2024d). Using the heavy metal indices and benthic indices to assess the ecological quality in the tidal flats of garolim bay, South Korea. Water 16, 736. doi: 10.3390/w16050736
Liang J., Ma C.-W., Kim K.-B. (2024e). Ecological risk assessment of heavy metals in surface sediments and their impact on macrobenthos in asan bay, South Korea. Front. Mar. Sci. 11. doi: 10.3389/fmars.2024.1450396
Liang J., Ma C.-W., Kim S.-K., Park S.-H. (2024a). Assessing the benthic ecological quality in the intertidal zone of cheonsu bay, korea, using multiple biotic indices. Water 16, 272. doi: 10.3390/w16020272
Liang J., Ma C.-W., Kim K.-B., Son D.-S. (2024f). Can the ecological quality of several bays in South Korea be accurately assessed using multiple benthic biotic indices? J. Mar. Sci. Eng. 12, 1179. doi: 10.3390/jmse12071179
Liang J., Ma C.-W., Son D.-S. (2024c). Using the heavy metal and biotic indices to assess ecological quality in the central area of the east sea, South Korea. Water 16, 1230. doi: 10.3390/w16091230
Liang J., Shu M.-Y., Huang H.-R., Ma C.-W., Kim S.-K. (2024b). Using benthic indices to assess the ecological quality of sandy beaches and the impact of urbanisation on sandy beach ecosystems. J. Mar. Sci. Eng. 12, 487. doi: 10.3390/jmse12030487
Lim D., Choi J.-W., Shin H. H., Jeong D. H., Jung H. S. (2013). Toxicological impact assessment of heavy metal contamination on macrobenthic communities in southern coastal sediments of Korea. Mar. pollut. Bull. 73, 362–368. doi: 10.1016/j.marpolbul.2013.05.037
Montefalcone M. (2009). Ecosystem health assessment using the Mediterranean seagrass Posidonia oceanica: A review. Ecol. Indic. 9, 595–604. doi: 10.1016/j.ecolind.2008.09.013
Mulik J., Sukumaran S., Dias H. Q. (2020). Can the ecological status of three differentially impacted monsoonal tropical estuaries in NW India, be adequately assessed by a common estuarine benthic index? Ecol. Indic. 119, 106807. doi: 10.1016/j.ecolind.2020.106807
Muxika I., Borja Á., Bald J. (2007). Using historical data, expert judgement and multivariate analysis in assessing reference conditions and benthic ecological status, according to the European Water Framework Directive. Mar. pollut. Bull. 55, 16–29. doi: 10.1016/j.marpolbul.2006.05.025
National Institute of Fisheries Science. (2010). Notification of marine environmental process test standards. Available online at: https://www.nifs.go.kr/board/actionBoard0052List.do?BBS_CL_CD=ALL (Accessed March 01, 2024).
Paik S. G., Yun S. G., Park H. S., Lee J. H., Ma C. W. (2008). Effects of sediment disturbance caused by bridge construction on macrobenthic communities in Asan Bay, Korea. J. Environ. Biol. 29, 559–566.
Park H.-S., Choi J.-W., Lee H.-G. (2000). Community structure of Macrobenthic Fauna under Marine Fish Culture Cages near Tong-yong, Southern Coast of Korea. J. Korean Fish. Soc 33, 1–8.
Park S. J., Kim K., Kim T. I. (2021). Vertical profiles of suspended sediment transport integrating bottom boundary layer in the channel and port of asan bay, korea. J. Korean Soc Mar. Environ. Energy 24, 236–248. doi: 10.7846/JKOSMEE.2021.24.4.236
Pereira T. L., Wallner-Kersanach M., Costa L. D. F., Costa D. P., Baisch P. R. M. (2018). Nickel, vanadium, and lead as indicators of sediment contamination of marina, refinery, and shipyard areas. Environ. Sci. pollut. Res. 25, 1719–1730. doi: 10.1007/s11356-017-0503-3
Rebai N., Mosbahi N., Dauvin J.-C., Neifar L. (2022). Ecological risk assessment of heavy metals and environmental quality of Tunisian harbours. J. Mar. Sci. Eng. 10, 1625. doi: 10.3390/jmse10111625
Ryu J., Hong S., Chang W. K., Khim J. S. (2016). Performance evaluation and validation of ecological indices toward site-specific application for varying benthic conditions in Korean coasts. Sci. Total Environ. 541, 1161–1171. doi: 10.1016/j.scitotenv.2015.10.016
Ryu J., Khim J. S., Kang S.-G., Kang D., Lee C., Koh C. (2011). The impact of heavy metal pollution gradients in sediments on benthic macrofauna at population and community levels. Environ. pollut. 159, 2622–2629. doi: 10.1016/j.envpol.2011.05.034
Saini K., Singh J., Malik S., Saharan Y., Goyat R., Umar A., et al. (2024). Metal-Organic Frameworks: A promising solution for efficient removal of heavy metal ions and organic pollutants from industrial wastewater. J. Mol. Liq. 399, 124365. doi: 10.1016/j.molliq.2024.124365
Santibañez-Aguascalientes N. A., Borja Á., Montero-Muñoz J. L., Herrera-Dorantes M. T., Ardisson P.-L. (2020). Setting reference conditions to assess the ecological status of the sublittoral and bathyal benthic communities of the southern Gulf of Mexico. Ecol. Indic 111, 105964. doi: 10.1016/j.ecolind.2019.105964
Seo J.-Y., Lim H.-S., Choi J.-W. (2014). Threshold value of Benthic Pollution Index (BPI) for a muddy healthy benthic faunal community and its application to Jinhae Bay in the southern coast of Korea. Ocean Sci. J. 49, 313–328. doi: 10.1007/s12601-014-0030-9
Shim W. J., Hong S. H., Yim U. H., Kim N. S., Oh J. R. (2002). Horizontal and vertical distribution of butyltin compounds in sediments from shipyards in korea. Arch. Environ. Contam. Toxicol. 43, 277–283. doi: 10.1007/s00244-002-0156-0
Shim W. J., Kahng S. H., Hong S. H., Kim N. S., Kim S. K., Shim J. H. (2000). Imposex in the rock shell, Thais clavigera, as evidence of organotin contamination in the marine environment of Korea. Mar. Environ. Res. 49, 435–451. doi: 10.1016/S0141-1136(99)00084-7
Shrestha R., Ban S., Devkota S., Sharma S., Joshi R., Tiwari A. P., et al. (2021). Technological trends in heavy metals removal from industrial wastewater: A review. J. Environ. Chem. Eng. 9, 105688. doi: 10.1016/j.jece.2021.105688
Sponza D., Karaoğlu N. (2002). Environmental geochemistry and pollution studies of Aliağa metal industry district. Environ. Int. 27, 541–553. doi: 10.1016/S0160-4120(01)00108-8
Sprovieri M., Passaro S., Ausili A., Bergamin L., Finoia M. G., Gherardi S., et al. (2020). Integrated approach of multiple environmental datasets for the assessment of sediment contamination in marine areas affected by long-lasting industrial activity: the case study of Bagnoli (southern Italy). J. Soils. Sediments. 20, 1692–1705. doi: 10.1007/s11368-019-02530-0
Stankovic S., Jovic M., Stankovic A. R., Katsikas L. (2012). “Heavy metals in seafood mussels. Risks for human health,” in Environmental chemistry for a sustainable world. Eds. Lichtfouse E., Schwarzbauer J., Robert D. (Springer Netherlands, Dordrecht), 311–373. doi: 10.1007/978-94-007-2442-6_9
Szynkowska M. I., Pawlaczyk A., Maćkiewicz E. (2018). “Bioaccumulation and biomagnification of trace elements in the environment,” in Recent advances in trace elements. Eds. Chojnacka K., Saeid A. (Hoboken: Wiley), 251–276. doi: 10.1002/9781119133780.ch13
Taati A., Salehi M. H., Mohammadi J., Mohajer R., Díez S. (2020). Pollution assessment and spatial distribution of trace elements in soils of Arak industrial area, Iran: Implications for human health. Enviro. Res. 187, 109577. doi: 10.1016/j.envres.2020.109577
Tang Y., Liu Q., Liao Y., Zhou K., Shou L. (2021). Responses of macrobenthic communities to patchy distributions of heavy metals and petroleum hydrocarbons in sediments: A study in China’s Zhoushan Archipelago. Acta Oceanol. Sin. 40, 117–125. doi: 10.1007/s13131-021-1892-1
Timothy N., Tagui Williams E. (2019). Environmental pollution by heavy metal: an overview. Int. J. Environ. Chem. 3, 72. doi: 10.11648/j.ijec.20190302.14
Todd P. A., Ong X., Chou L. M. (2010). Impacts of pollution on marine life in Southeast Asia. Biodivers. Conserv. 19, 1063–1082. doi: 10.1007/s10531-010-9778-0
Trevizani T. H., Figueira R. C. L., Ribeiro A. P., Theophilo C. Y. S., Majer A. P., Petti M. A. V., et al. (2016). Bioaccumulation of heavy metals in marine organisms and sediments from Admiralty Bay, King George Island, Antarctica. Mar. pollut. Bull. 106, 366–371. doi: 10.1016/j.marpolbul.2016.02.056
Wang Y., Duan X., Wang L. (2020). Spatial distribution and source analysis of heavy metals in soils influenced by industrial enterprise distribution: Case study in Jiangsu Province. Sci. Total Environ. 710, 134953. doi: 10.1016/j.scitotenv.2019.134953
Woo J., Lee H., Park J., Park K., Cho D., Jang D., et al. (2019). Background concentration and contamination assessment of heavy metals in korean coastal sediments. Sea: J. Korean Soc Oceanogr. 24, 64–78. doi: 10.7850/JKSO.2019.24.1.064
Wu H.-Y., Fu S.-F., Hu W.-J., Chen F.-G., Cai X.-Q., Chen Q.-H., et al. (2022). Response of different benthic biotic indices to eutrophication and sediment heavy metal pollution, in fujian coastal water, East China sea. Chemosphere 307, 135653. doi: 10.1016/j.chemosphere.2022.135653
Xu J., Gai M., Yan X., Xu Y., Yue P. (2024). Contradictions in human-nature relationships threaten coastal resilience and sustainability in the Bohai Rim Region, China. Environ. Sci. pollut. Res. 31, 22945–22961. doi: 10.1007/s11356-024-32485-w
Xu Z., Chen Y. (1989). Aggregated intensity of dominant species of zooplankton in autumn in the east China sea and yellow sea. Chin. J. Ecol. 4, 13–15.
Yang J. K., Shin H. C. (2020). The influence of the dissolved oxygen of bottom water on the temporal variation of the benthic polychaetous community structure in dangdong bay. Ocean Polar Res. 42, 233–247. doi: 10.4217/OPR.2020.42.3.233
Yu J., Zhou D., Yu M., Yang J., Li Y., Guan B., et al. (2021). Environmental threats induced heavy ecological burdens on the coastal zone of the Bohai Sea, China. Sci. Total Environ. 765, 142694. doi: 10.1016/j.scitotenv.2020.142694
Yu O. H., Lee H.-G., Lee J.-H. (2011). The influence of environmental variables on macrobenthic communities after dike construction in asan bay, korea. Korean. J. Environ. Biol. 29, 326–344.
Zhai T., Wang J., Fang Y., Qin Y., Huang L., Chen Y. (2020). Assessing ecological risks caused by human activities in rapid urbanization coastal areas: Towards an integrated approach to determining key areas of terrestrial-oceanic ecosystems preservation and restoration. Sci. Total Environ. 708, 135153. doi: 10.1016/j.scitotenv.2019.135153
Zhang M., Liu C., Zhang C., Zhu H., Wan J., Liu X. (2023). Response of macrofaunal assemblages to different pollution pressures of two types of ports. Ecol. Indic 146, 109858. doi: 10.1016/j.ecolind.2022.109858
Zhao Z., Liu Q., Liao Y., Yu P., Tang Y., Liu Q., et al. (2023). Ecological risk assessment of trace metals in sediments and their effect on benthic organisms from the south coast of Zhejiang province, China. Mar. pollut. Bull. 187, 114529. doi: 10.1016/j.marpolbul.2022.114529
Zhou Q., Zhang J., Fu J., Shi J., Jiang G. (2008). Biomonitoring: An appealing tool for assessment of metal pollution in the aquatic ecosystem. Anal. Chim. Acta 606, 135–150. doi: 10.1016/j.aca.2007.11.018
Keywords: macrobenthos, ironworks, shipyard, heavy metal, benthic index
Citation: Liang J, Ma C-W and Kim K-B (2024) Comparing the environmental impacts of pollution from two types of industrial zones on the coast. Front. Mar. Sci. 11:1433536. doi: 10.3389/fmars.2024.1433536
Received: 16 May 2024; Accepted: 12 August 2024;
Published: 03 September 2024.
Edited by:
Mario Barletta, Federal University of Pernambuco, BrazilReviewed by:
Ester Santos, Federal University of Pernambuco, BrazilYibo Liao, Ministry of Natural Resources, China
Copyright © 2024 Liang, Ma and Kim. This is an open-access article distributed under the terms of the Creative Commons Attribution License (CC BY). The use, distribution or reproduction in other forums is permitted, provided the original author(s) and the copyright owner(s) are credited and that the original publication in this journal is cited, in accordance with accepted academic practice. No use, distribution or reproduction is permitted which does not comply with these terms.
*Correspondence: Chae-Woo Ma, cwooma@sch.ac.kr