- 1Maurice-Lamontagne Institute, Fisheries and Oceans Canada, Mont-Joli, QC, Canada
- 2Centre d'Étude pour la Promotion des Activités Lagunaires Maritimes, Sète, France
Bivalve culture is know to affect some interactions between the water column and the benthic environment with the organic enrichment. An in situ mesocosms experiment was used to determine the influence of mussel biodeposition on the benthic ecosystem in St. Peters Bay, Prince Edward Island, eastern Canada. Eight mussel densities (0 to 2266 mussels m-2) were placed above mesocosms to reproduce the organic enrichment from a mussel farm over 12 weeks. The experimental procedure created an accumulation of organic matter on the seafloor with increasing mussel densities. Some benthic parameters had the predicted response to organic enrichment, with the presence of anoxic sediment in sediment profile images, increased water and organic matter content, and reduced infaunal abundance and species richness. Other parameters, including redox potential, total free sulfides, and the response of the biotic index AMBI, had inconsistent responses to mussel biodeposition-related organic enrichment. Given that other studies done in other locations have observed varying results, results suggest that measurement of various parameters is important to interpret the influence of deposition from mussel farms. The conditions of the site and the study duration are also parameters to consider when evaluating the results from mesocosm studies. Results from this study also suggest that St. Peters Bay is likely impacted by biodeposition from the mussels farmed there.
1 Introduction
Bivalve aquaculture is considered to have less impact on the environment relative to other types of aquaculture, e.g. finfish for which an addition of organic matter is required as food (Crawford et al., 2003; Newell, 2004; Dumbauld et al., 2009; Naylor et al., 2021). However, suspension-feeding bivalve culture plays a key role in several environmental processes due to the addition of physical structures (longlines, rafts, etc.) and the introduction of thousands or millions of individuals (cultivated species and biofouling organisms) to the environment. The effects of organic enrichment (biodeposition) from bivalve faeces and pseudofaeces are now relatively well known (Mattsson and Lindén, 1983; Weise et al., 2009; McKindsey et al., 2011; Sun et al., 2021), as is how this correlates with local current regimes and depth (Hartstein and Stevens, 2005; Giles et al., 2009; Gallardi, 2014; Forget et al., 2020).
Many studies have described the impacts from biodeposition on sediment biogeochemical parameters (Dahlbäck and Gunnarsson, 1981; Mattsson and Lindén, 1983; reviewed in McKindsey et al., 2011), nutrient exchanges at the sediment-water interface (Baudinet et al., 1990; Richard et al., 2006, 2007a; Richard et al., 2007b; Robert et al., 2013; Lacoste et al., 2020), and benthic infaunal communities (Callier et al., 2008, 2009; McKindsey et al., 2012; Lacoste et al., 2018). However, results vary by sites and culture systems, and while some studies noted little or no changes in sediments beneath farms (Grant et al., 1995; Crawford et al., 2003), other have shown organic enrichment and related alteration of infaunal communities (Chivilev and Ivanov, 1997; Stenton-Dozey et al., 1999). Significant accumulation of organic matter on the sea floor can promote hypoxic or even anoxic and sulfate reducing conditions due to bacteria that degrade the organic matter by consuming the available oxygen in benthic sediments (Hargrave et al., 2008a, 2008b; McKindsey et al., 2011). Individual infaunal taxa and community assemblages react to environments that are characterized by low oxygen or the accumulation of sulfide (Pearson and Rosenberg, 1978; Hargrave et al., 2008b; Elvines et al., 2023). Organic loading commonly leads to reduced macrobenthic infaunal abundances and promotes the dominance of small opportunistic deposit feeders relative to that of suspension feeders (Callier et al., 2009; Dumbauld et al., 2009; McKindsey et al., 2011). Some studies show that only species tolerant of low oxygen and relatively high total free sulfides (TFS) are found in sites characterized by organic enrichment (Hargrave et al., 2008b). Macrobenthic infauna plays a crucial role in benthic ecosystems due to bioturbation and bio-irrigation activities. By reworking sediment, infauna enhance oxygenation and influence nutrient exchanges between sediment and the water column (Michaud et al., 2009; Wrede et al., 2017). Decreased benthic infauna may therefore increase organic enrichment effects by reducing sediment reworking and the mineralization of the organic matter (McKindsey et al., 2011). Evaluation of benthic infauna is thus thought to be a good indicator of organic enrichment from bivalve farms.
Given that several past studies have reproduced organic loading from mussel biodeposition in Îles-de-la- Madeleine, Quebec (Callier et al., 2009; Robert et al., 2013; Lacoste et al., 2019), and that ecosystem responses vary by site location, this in-situ study, done in Prince Edward Island (PEI), evaluates the impact of biodeposition on various benthic indicators. Mussel culture occupies a large portion of PEI bays with a production of 17 440 tonnes in 2022, 75% of the total production in Canada, with a value of $30 million (Fisheries and Oceans Canada, 2022). This underlines the importance of better understanding the relationship between mussel farms and benthic condition in this part of the country.
This study evaluates the impact of biodepostion from mussel farms on various benthic indicators, including sediment parameters (total free sulfides – TFS, redox potentials normalized to hydrogen potential – EhNHE, and organic matter – OM) and infaunal community structure in St. Peters Bay, PEI. In situ benthic mesocosm were installed on the bottom with varying densities of mussels to create various levels of organic enrichment through biodeposition. This is a part of a larger program to provide a holistic description of benthic ecosystem responses to organic enrichment from mussels in various exploited areas in Canada (Callier et al., 2007, 2008; Robert et al., 2013; Lacoste et al., 2019). Also, the results may be use by the Canadian government for the aquaculture monitoring program.
2 Materials and methods
2.1 Study area
The study was conducted in St. Peters Bay (SPB, 46°26’16’’N, 62°40’9’’W) in Prince Edward Island, Canada, in summer 2012 (Figure 1A). SPB is a small (13 × 2 km) and shallow (mostly < 6 m) semi-enclosed embayment on the north shore of the island. A 4-5 m deep channel runs along its longitudinal axis. A description of the bay is also provided in Guyondet et al. (2015) and Sonier et al. (2016). The bay is used extensively for longline blue mussel (Mytilus edulis) culture, representing about 38% of its total surface area. Mussels are grown in suspension using 2 m individual polyethylene sleeves (‘socks’) that hang from submerged longlines (Sonier et al., 2016). SPB is characterized by slow water renewal and poor flushing with tidal currents of a few centimeters per second (Guyondet et al., 2015).
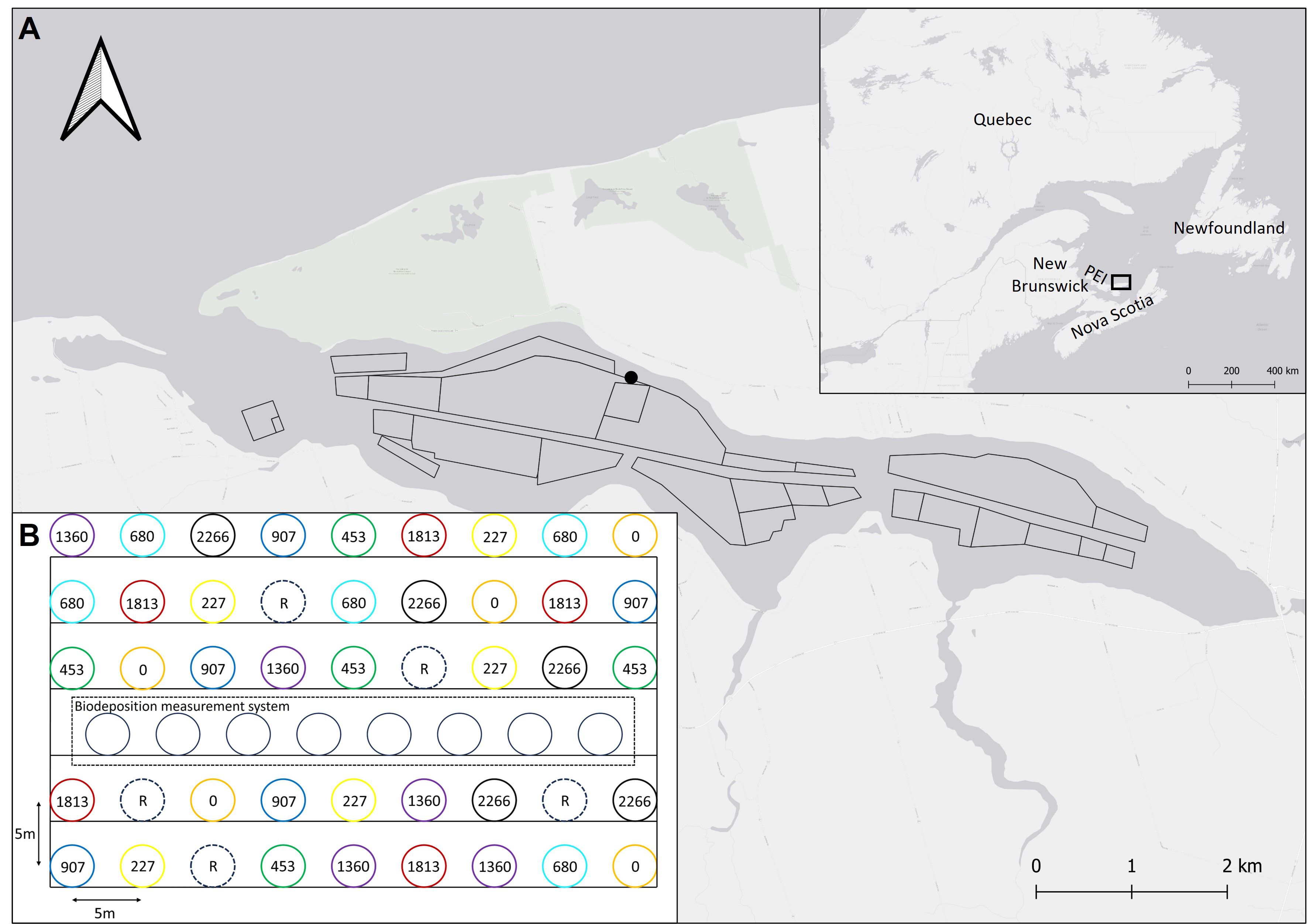
Figure 1. (A) Location of the experimental mesocosm (black dot) site in St. Peters Bay, Prince Edward Island (PEI), eastern Canada. Polygons in the bay represent the mussel lease sites. (B) Layout of experimental grid in St. Peters Bay (n = 5 mesocosms per each mussel density treatments and reference location). The numbers and colors refer to the different mussel densities.
2.2 Mesocosms
To examine the influence of mussel biodeposition on sediment biogeochemical parameters and benthic communities, 40 benthic mesocosms were installed on a 5 × 9 grid (including 5 reference locations) on a soft, muddy sand bottom approximately 500 m distant from the closest farm on the northwestern side of the bay with an extra 8 “mesocosms” installed in the middle of the array to evaluate sedimentation (Figure 1B). The site was judged to be sufficiently distant from farms to limit their influence (Weise et al., 2009). Methods to construct mesocosms were as those outlined in Lacoste et al. (2019) except that ¼” × ¼” wire mesh was used for mesocosms in this study to avoid invasion of cages by sea stars that consumed mussels in an earlier trial experiment. The top component of mesocosms, a 23.5 cm high by 57 cm diameter cage, was also constructed from ¼” × ¼” PVC coated wire mesh. A plastic 53 cm diameter sleeve formed the base of each mesocosm, against which the wire mesh abutted. Each mesocosm was spaced 5 m apart to avoid influence by adjacent ones. Divers removed fouling organisms from mesocosms two times each month for the duration of the experiment to ensure good water circulation to feed the mussels contained within.
For 12 weeks (June to September), mesocosms received continuous biodeposition from overlying mussels from one of eight pre-defined mussel densities (0, 50, 100, 150, 200, 300, 400, and 500 mussels). The eight mussel densities correspond to 0, 227, 453, 680, 907, 1360, 1813, and 2266 mussels m-2, respectively. Each mussel density was replicated five times, with five locations left undisturbed as reference sites (R). Each mesocosm received mussels from the same size range (i.e. 60.55 ± 0.18 mm, mean ± SE calculated from thirty random mussels per mesocosm at the end of the experiment). At the end of the experiment, divers took photographs of each mesocosm to assess seabed conditions. Divers then carefully collected two sediment cores (15 cm high × 10 cm diameter) from each mesocosm and the reference sites. Once returned to the surface, photographs of the top and side of each core were taken. The two cores were used to collect sub-samples for sediment geochemical analyses and were sieved for infaunal analyses (see sampling details in ‘Surficial sediment characteristics’ and ‘Infaunal communities’ below).
2.3 Deposition rates
In July (n = 38 samples) and August (n = 23 samples), mussel biodeposition rates were measured in situ using sediment traps as described in Lacoste et al. (2019). Sediment traps were placed on the bottom portion of mesocosms along the transect in the center of the grid (Figure 1B). Randomly chosen cages of each treatment (7 mussel densities and no mussels) were placed on the sediment traps. After 24 hours, each cage and sediment trap was gently shaken prior to removing them to allow the recently produced biodeposits to sink into the sampling bottle. Sampling bottles were kept frozen at -20°C until laboratory analyses to determine total suspended particulate matter (TSPM) and total organic matter (TOM).
In the laboratory, samples were thawed, the supernatant removed, and swimmers and detritus seen by the naked eye removed. Sampling bottle contents were then transferred to pre-burned and pre-weighed aluminium dishes and samples dried at 65°C for 72 h to constant weight and weighed. TSPM and TOM were expressed in terms of g m-2 d-1. Estimated biodeposition (organic matter, OM) for each individual mussel was calculated by subtracting the background sedimentation from density 0 (empty cage).
2.4 Sediment visual observation
Sediment profile images (SPI) was used to detect impacts of organic enrichment in bottom sediments, following Wildish et al. (2003). A picture of each macrofaunal core was taken (before being sieved) and these side-view images were visually analyzed to determine the region of sediments where oxidizing processes are replaced by reducing processes resulting their darkening. Only the presence or absence of darker sediment were recorded as data for each picture.
2.5 Surficial sediment characteristics (EhNHE, TFS, %WC and %OM)
Sediment sub-samples (n = 90) were collected by lateral insertion of a 5 mL cut-off plastic syringe through the side of cores via a pre-drilled hole covered by duct tape at the 0-2 cm sediment depth. Syringes were tightly capped with parafilm to avoid exposure to air and stored on ice until analysis (within 6 hours). Measurements of redox potentials (EhNHE), total free sulfides (TFS), water content (%WC), and organic matter (%OM) were made on each 5 mL sub-sample.
EhNHE and TFS in sediment porewater were measured using the same protocol as outlined in Lacoste et al. (2019) following the measurement method described in Wildish et al. (1999). EhNHE is an indirect indicator of aerobic vs. anaerobic conditions in sediments while TFS is a measure of the accumulation of soluble sulfides, a product of sulfate reduction that occurs under anaerobic conditions. EhNHE and TFS concentrations were expressed as µM based on sediment volume.
To calculate %WC and %OM in surficial sediments (0-2 cm), a further 1 cc of wet sediment was extruded from each syringe and kept frozen. In the laboratory, samples were weighed, dried to constant weight (65°C, 24 hours). %WC was determined as the percent weight loss between wet and dried material. %OM was determined by a two-step combustion (Kristensen, 1990). Dry samples were heated in a muffle furnace at 250°C for 2 hours to completely dry them and subsequently at 550°C for 18 hours to completely ash them. The samples were weighed after each step to give the total OM and the %OM calculated from weight loss as a percent of sample dry weight.
2.6 Infaunal communities
The two sediment cores collected in each mesocosm (n = 90) were gently sieved through a 500 µm mesh and the material retained preserved in a buffered (magnesium borate) 5% formaldehyde-saline solution. After sorting, infaunal specimens were stored in 70% ethanol. Identifications were made to the lowest taxonomic level possible. Samples were characterized in terms of total abundance (N), the number of species (S, species richness), Shannon-Wiener diversity (H’) and Pielou’s evenness index (J’). To evaluate the effect of organic enrichment on benthic communities, the AZTI Marine Biotic Index (AMBI; Borja et al., 2000) and a related multivariate index (M-AMBI, Muxika et al., 2007) that considers species richness and diversity were calculated. Species were classified into AMBI ecological groups based on their sensitivity to pollution (organic matter accumulation). AMBI scores were detailed in six groups: 0.0 to 1.2 indicate unpolluted, 1.2 to 3.3 slightly polluted, 3.3 to 5.0 moderately polluted, 5.0 to 6.0 heavily polluted and more than 6.0 extremely polluted (Borja et al., 2000). M-AMBI provides an ecological status based on these values: >0.82 ‘High’, 0.62-0.82 ‘Good’, 0.41-0.61 ‘Moderate’, 0.20-0.40 ‘Poor’, and <0.20 ‘Bad’ (Muxika et al., 2007). Reference values used in M-AMBI calculations are those observed at the nominally “least impacted” of the sites (i.e., the reference sites).
2.7 Data analysis
2.7.1 Univariate measurements
Variation among mussel densities for sediment characteristics (%WC, %OM, EhNHE and TFS) and infaunal communities (abundance, richness, biodiversity index) were evaluated using permutational analysis of variance (PERMANOVA, with 9999 permutations) using PRIMER 7 (Clarke and Gorley, 2015) and PERMANOVA+ (Anderson et al., 2008), as data transformations were unable to constrain the data to meet the assumptions of ANOVA (Anderson, 2001). Similarity matrices used for PERMANOVAs were constructed based on Euclidean distances. All analyses included 2 factors: Mussel density (9 levels: R, 0, 227, 453, 680, 907, 1360, 1813 and 2266) and Mesocosms (replicate mesocosms for each density) that were nested in Mussel density treatments. Differences among factors were determined using a posteriori pairwise comparisons using PERMANOVA. Homogeneity of multivariate dispersion was evaluated using PERMDISP (Anderson et al., 2008) and data transformed (square-root for sediment characteristics parameters and fourth-root for infaunal community parameters), as necessary. For sediment characteristics, one variable (EhNHE) included negative values and thus a constant was added to each data point so they could be analysed following the appropriate transformation.
Linear regressions were used to evaluate the relationship between infauna metrics (abundance and richness) and TFS concentrations. Correlation coefficients were calculated to see if there is an association between variables (0 = no linear relationship; less than 0.8 or greater than -0.8 = correlation not considered powerful).
2.7.2 Multivariate Measurements
PERMANOVA was used to evaluate variations in infaunal community structure between mussel densities. The matrix was constructed based on Bray-Curtis similarity on fourth-root transformed data. Species (17) that appeared only once were removed (Clarke and Warwick, 2001) from the data matrix. This analysis considered the same two factors used for the univariate measures and a post-hoc test evaluated variation among factors. SIMPER analysis was used to identify the infaunal species that were most responsible for the dissimilarity among mussel densities. Non-metric multi-dimensional scaling (nMDS) was used to show graphically the variation in benthic assemblages among the treatments.
3 Results
3.1 Sedimentation rates and biodeposition
Average sedimentation rates measured in mesocosms (in July and August) are presented in Table 1. TSPM and TOM varied greatly between mussel densities and between sampling dates. As expected, sedimentation rates generally increased with mussel density, likely due to biodeposition. Rates were higher in July, especially the background (treatment 0) for TSPM: 53.10 ± 7.60 in August compared to 176.14 ± 57.99 g m-2 d-1 in July (Table 1). The fraction of organic matter in the TSPM varied between 7.1 (treatment 0) and 14.3% (1360 mussels m-2) in July and 11.0 (453 mussels m-2) and 15.5% (680 mussels m-2) in August. Biodeposition (OM) seemed to be lower when mussel density was higher (Table 1). OM was higher in August at low density mussels, but similar between the two months at high density (Table 1).
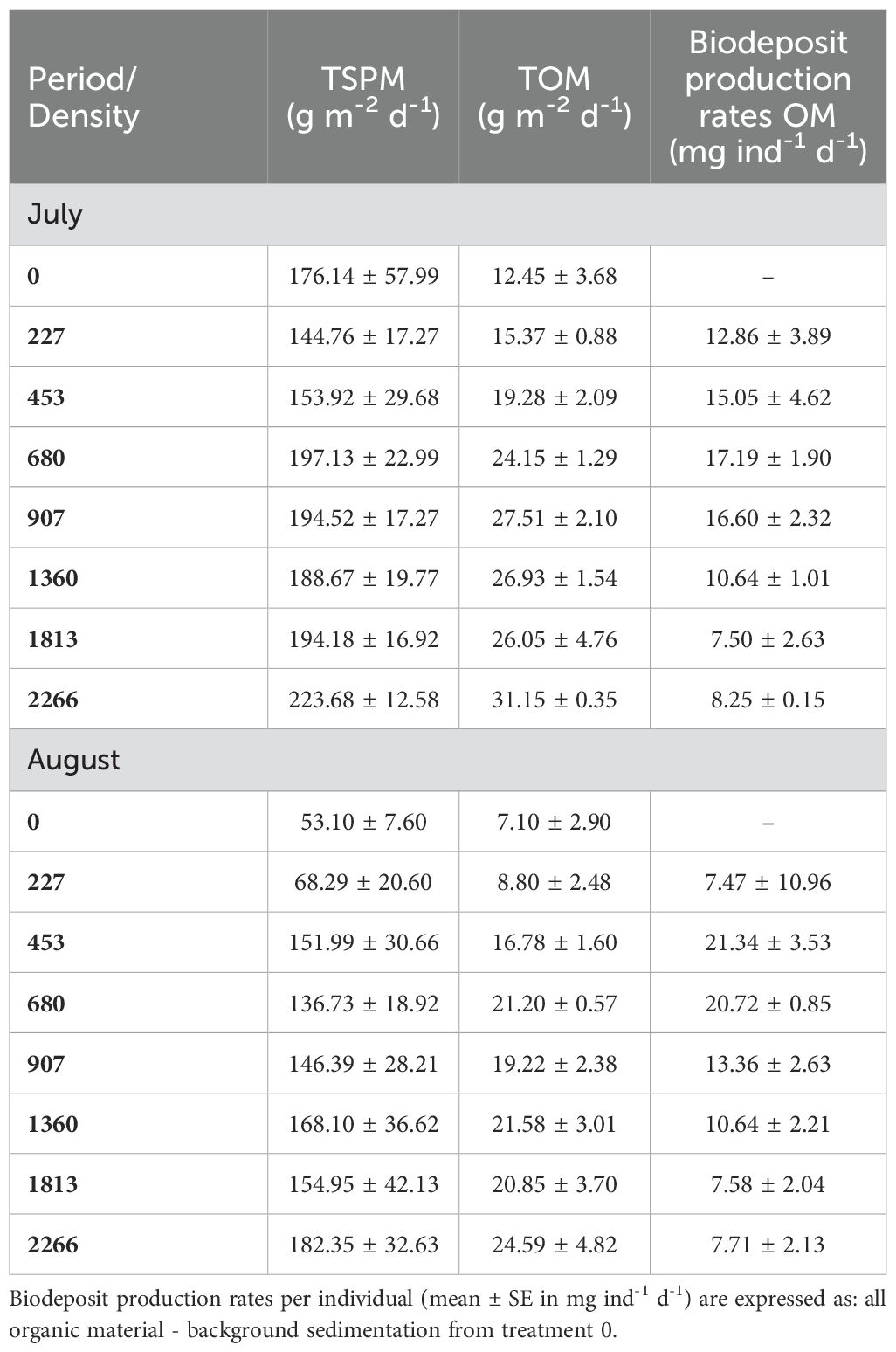
Table 1. Mean (± SE) sedimentation rates expressed as total suspended particulate matter (TSPM in g m-2 d-1) and total organic matter (TOM in g m-2 d-1) for each treatment during two periods (July and August).
3.2 Sediment visual observation
Side-view images of sediment cores provided visual information on benthic conditions in mesocosms (Figure 2). Unfortunately, some pictures were not enough good to be used to detect or not darker sediments and were discarded from the analyses (for densities with 1360 and 2266 mussels m-2). Surface sediments from the densities 0 and 227 mussel m-2 appeared similar to those at reference (R) sites with light brown sediments. In comparison, side-views of sediment cores from densities 453 mussels m-2 and more revealed a loose and flocculent brown layer of biodeposits mixed with sediments (ca. 0.5-1.0 cm thick) covering a large black layer of reduced sediments (ca. 5 cm thick).
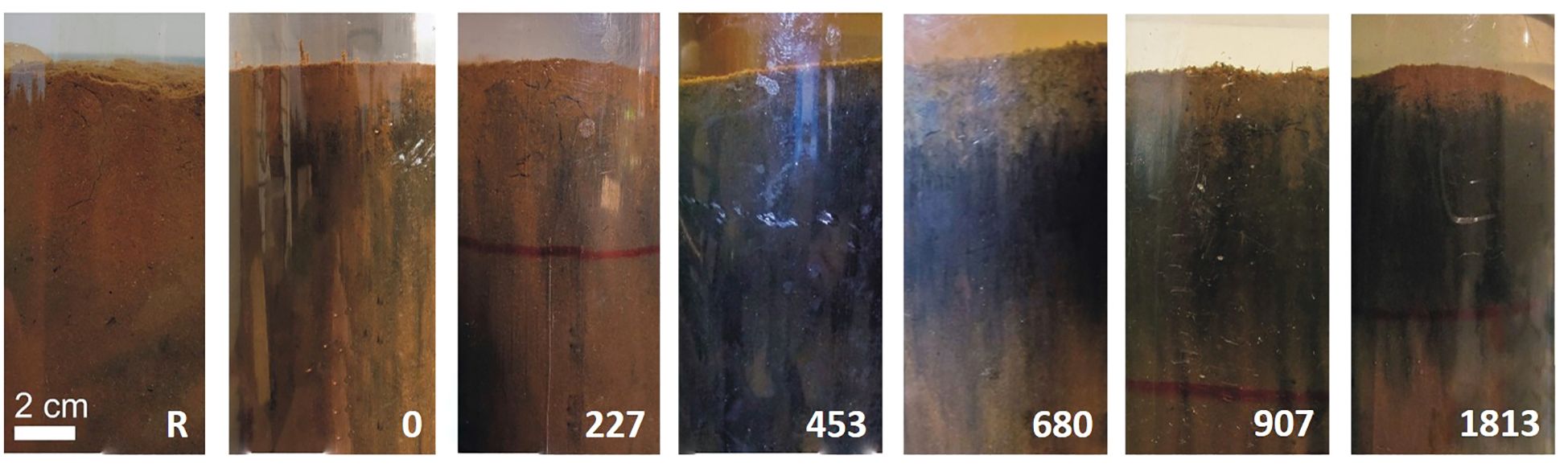
Figure 2. Side-view of sediment cores from benthic mesocosms after 12 weeks in-situ exposure to different mussel densities (R, 0, 227, 453, 680, 907, and 1813 mussels m-2). Photos were not available for densities with 1360 and 2266 mussels m-2 as explain in the section 2.4.
3.3 Sediment characteristics
Biogeochemical measures from surficial sediments (0-2 cm strata) are shown in Figure 3. Mussel density had a significant effect for all biogeochemical indicators (Table 2). %WC and %OM in surficial sediments tend to increase from the lowest density (0 mussel m-2) to the highest density (2266 mussels m-2) and values were higher relative to the R mesocosms (Figures 3A, B). %OM was almost two to ten times greater than at R sites with mean values ranging from 12.06 ± 1.99 to 20.69 ± 1.21% in densities with 453 and 2266 mussels m-2 respectively (Figure 3B).
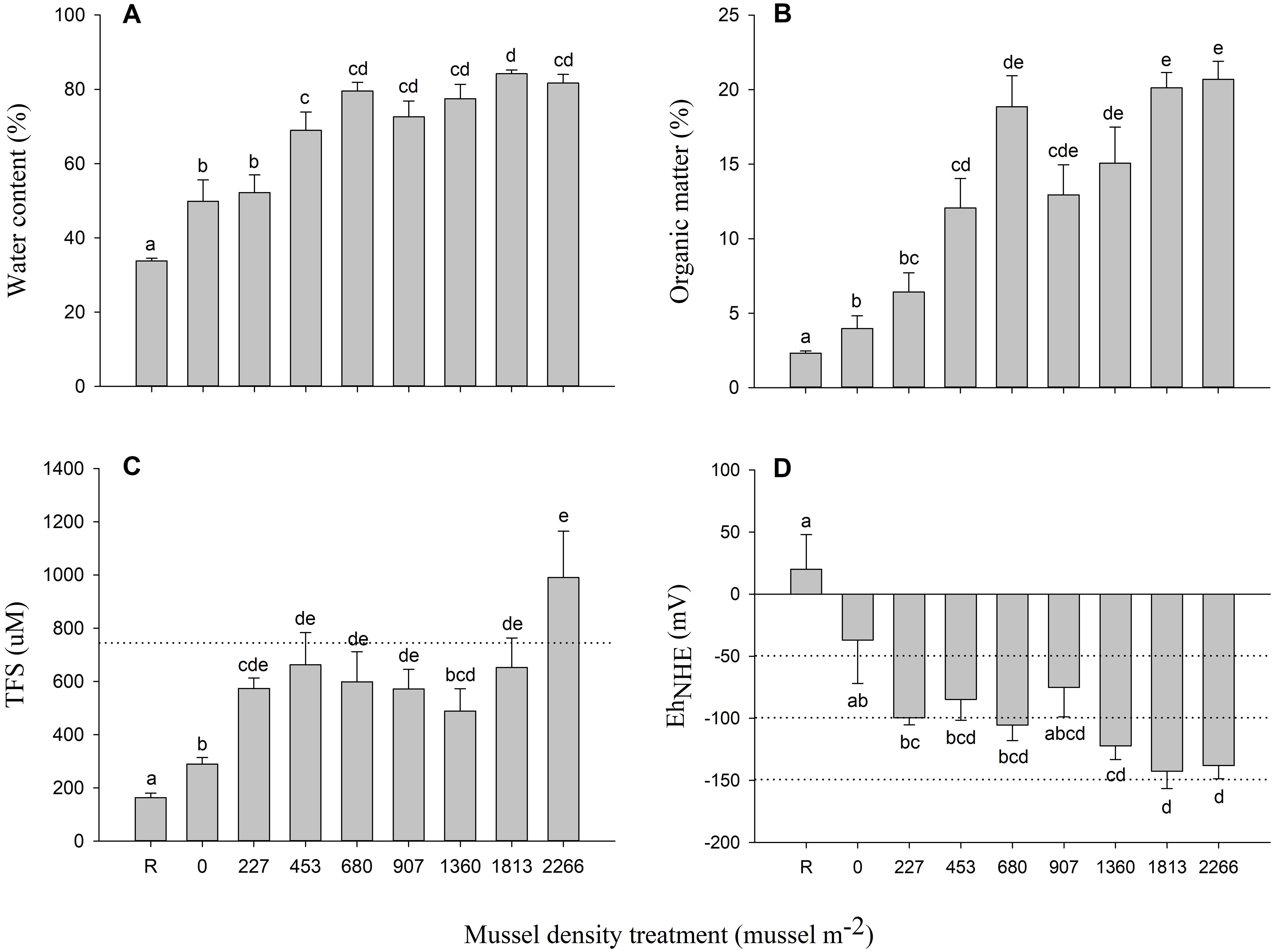
Figure 3. Mean (± SE) (A) % water content (WC), (B) % organic matter (OM), (C) sulfides (TFS, µM), and (D) redox potential (EhNHE, mV) measured in surficial (0-2 cm) sediments for each mussel density. The dashed line in (C) represent sulfide concentrations for “normal” (oxic A, < 750 µM) and values above represent “transitory” condition (oxic B, 750-1500 µM) based on Hargrave et al. (2008b). The dashed lines in (D) represent the redox thresholds for transitions between oxic A (> 100 mV), oxic B (100 to -50 mV), hypoxic A (-50 to -100 mV), hypoxic B (-100 to -150 mV), and anoxic (< -150 mV) categories based on Hargrave et al. (2008b). Differences between mussel density means are indicated by different letters (p < 0.05).
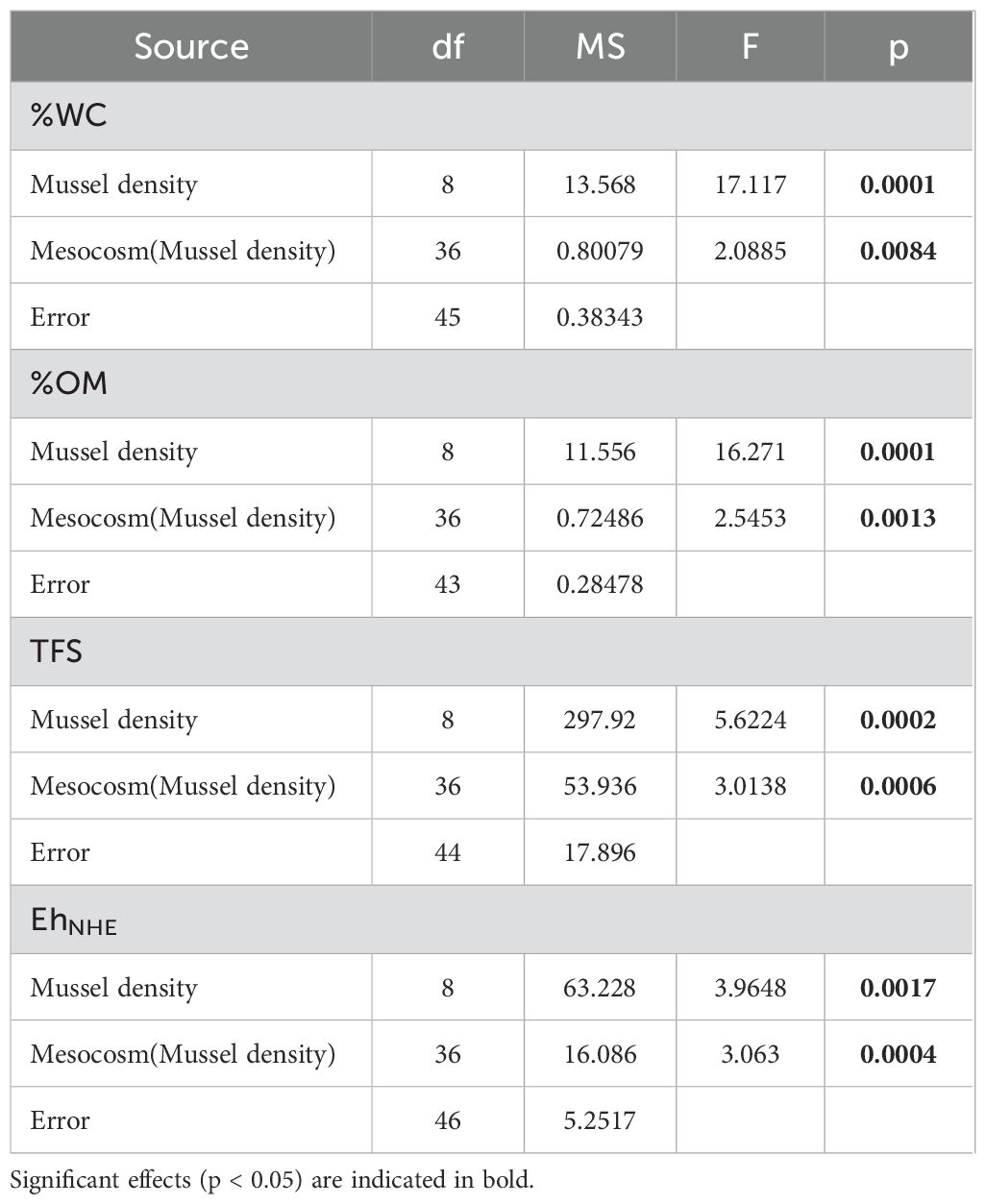
Table 2. Results of PERMANOVA evaluating the effect of Mussel density and Mesocosm(Mussel density) on sediment characteristics (%WC, %OM, TFS and EhNHE).
Mean TFS concentration was significantly lower in R mesocosms than all other mussel densities and generally increased with organic loading (Figure 3C). TFS mean (± SE) values varied between 162.74 ± 17.17 and 990.25 ± 174.79 μM (Figure 3C). EhNHE mean (± SE) averaged 19.90 ± 28.08 mV at R sites and shifted to increasingly negative values along the mussel density gradient (Figure 3D).
3.4 Infaunal community
3.4.1 Community structure
A total of 6602 individuals from 65 taxa was found. Polychaetes were the most common organisms, representing 55.4% (35 taxa) of the total sample followed by bivalves with 34.5% (11 taxa) and gastropods with 5.9% (6 taxa). No clear pattern was observed for the proportion of these groups between the mussel density treatments. Mussel density impacted all infaunal community parameters (Table 3). Abundance varied between 26 ± 4 (2266 mussels m-2) to a maximum of 116 ± 16 individuals per core (227 mussels m-2) and peak richness was observed with a mean of about 19 species per core for the reference treatment (Figures 4A, B). Species diversity (Shannon-Wiener index) was lowest in the 1813 mussels m-2 treatment and highest at the reference location, at 1.53 ± 0.06 and 2.57 ± 0.07, respectively (Figure 4C). Densities R, 1360 and 2266 mussels m-2 had the greatest species uniformity as determined by the Pielou eveness index compared to the 1813 mussel m-2 treatment that had the lowest index (Figure 4D).
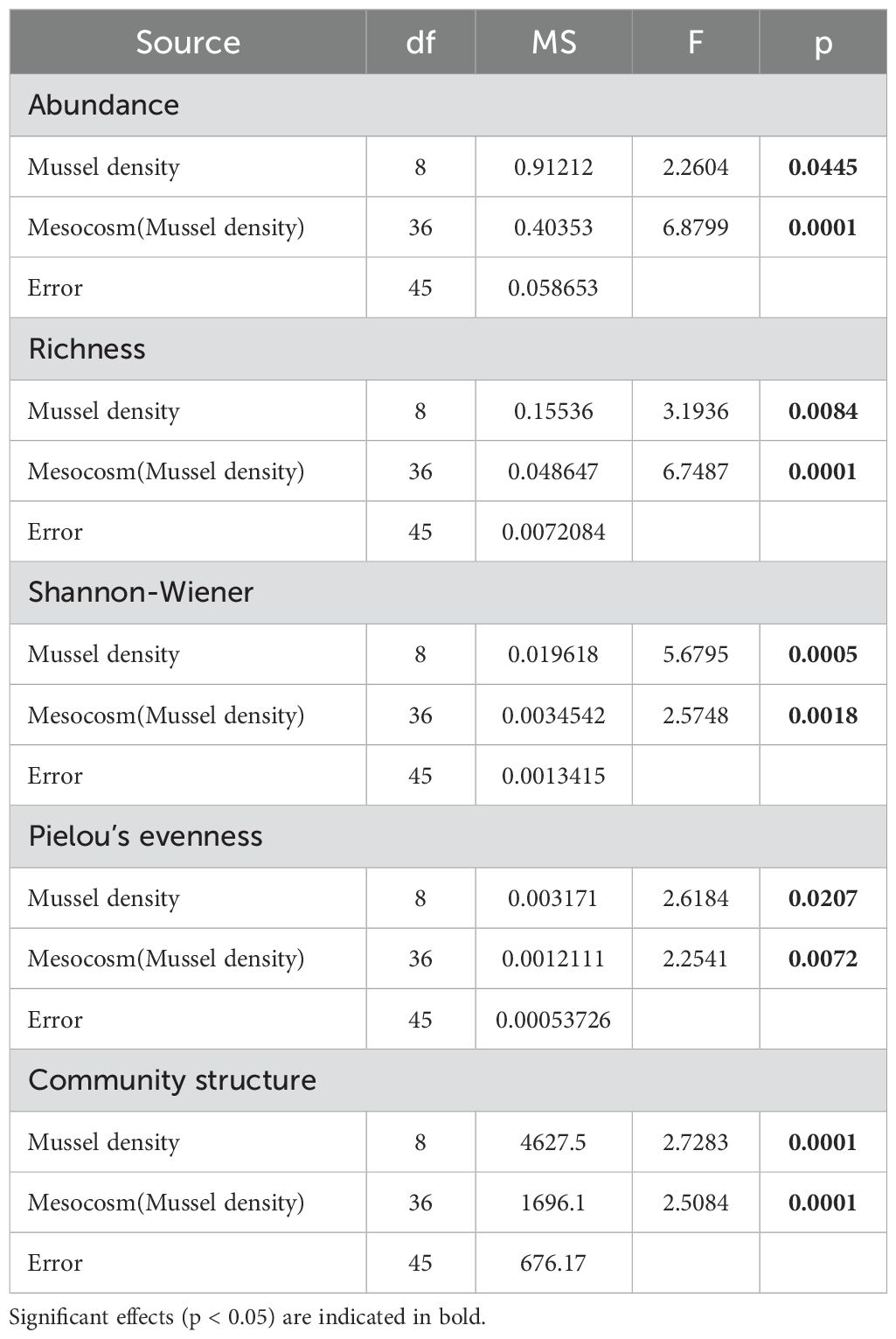
Table 3. Results of PERMANOVA evaluating the effect of Mussel density and Mesocosm(Mussel density) on sediment infaunal community abundance, species richness, Shannon-Wiener diversity, Pielou’s evenness index and infaunal community structure.
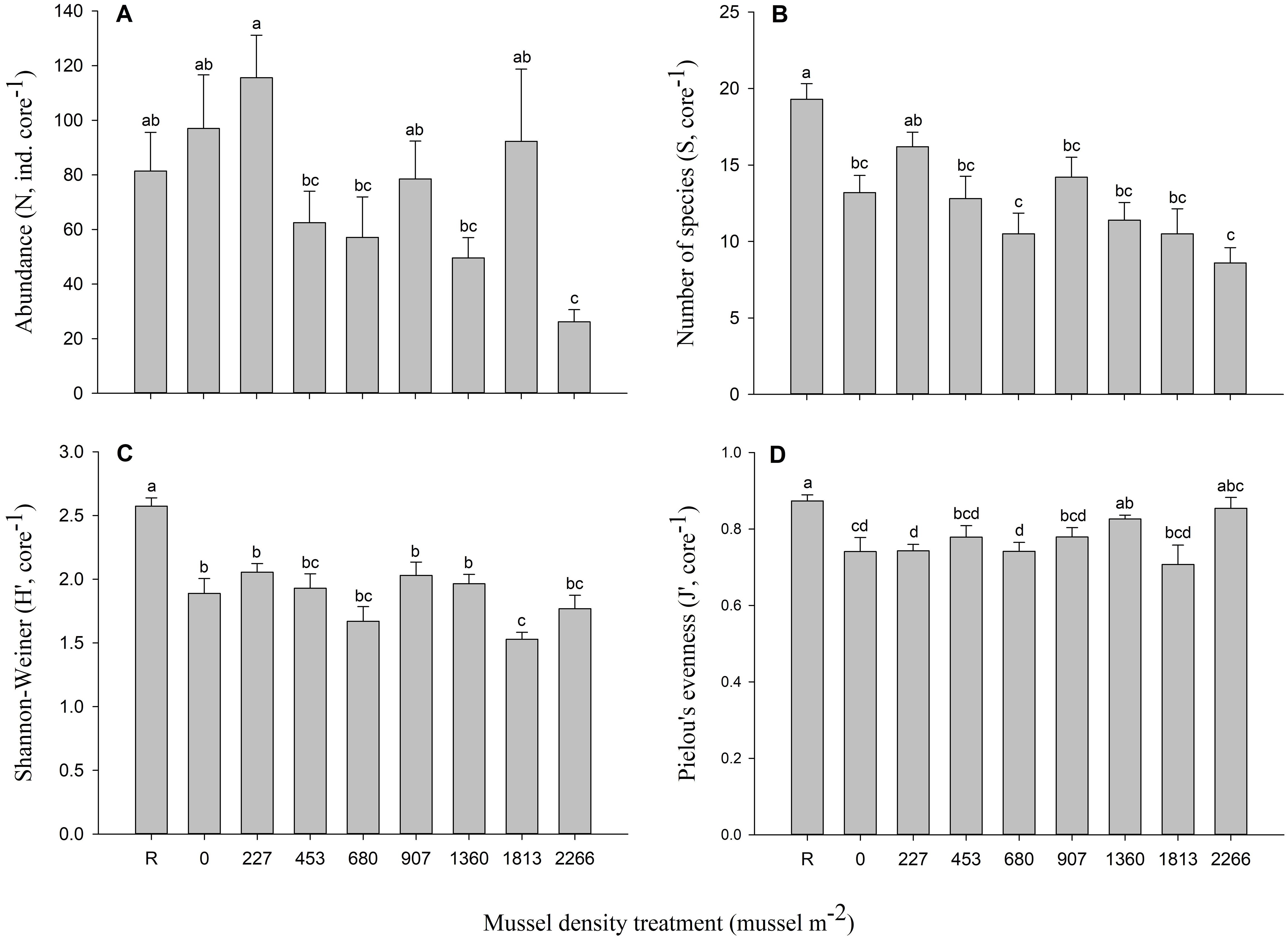
Figure 4. Mean (± SE) (A) abundance, (B) number of species (species richness), (C) Shannon-Wiener diversity, and (D) Pielou’s evenness index of infauna from reference (R) and mesocosms receiving biodeposition from eight different mussel densities. For each density, n = 10 (five mesocosms with two replicate cores each). Differences between mussel density means are indicated by different letters (p < 0.05).
Infaunal community structure varied significantly with mussel density (Table 3). Densities R, 0 and 227 mussels m-2 differed significantly from all other treatments and each other, except for the 227 and 907 mussels m-2 that were similar. Densities 453 through 2266 mussels did not differ significantly. The nMDS (Figure 5) showed the separation between densities R, 0, and 227 from all the others. As the stress is quite high (0.2), the projection in the figure represents only the general relationship among data points. SIMPER analysis reveals that eight species explained ca. 30 to 35% of the dissimilarity between the mussel density treatments; seven polychaetes: Dipolydora quadrilobata, Hypereteone heteropoda, Leitoscoloplos fragilis, Mediomastus ambiseta, Pectinaria gouldii, Scolelepis (Parascolelepis) bousfieldi, Streblospio benedicti, and one bivalve: Mysella planulata (Figure 6). All species abundances differ significantly among densities (Table 4). The dissimilarity between mussel density treatments varied from 44.92% (treatments 227-907) to 64.89% (treatments 0-2266).
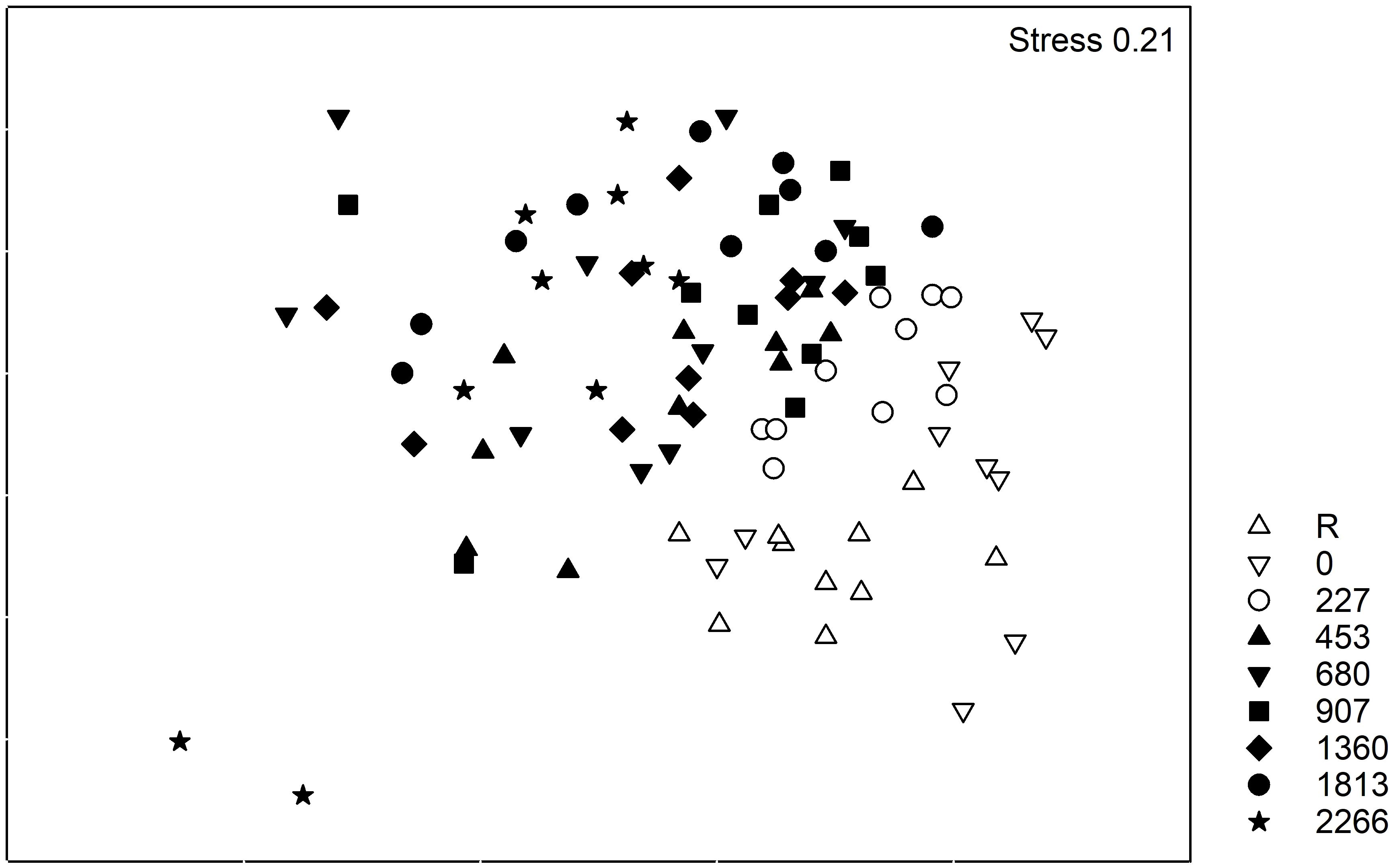
Figure 5. Non-metric multi-dimensional scaling (nMDS) of benthic assemblage structure in mesocosms (n = 10).
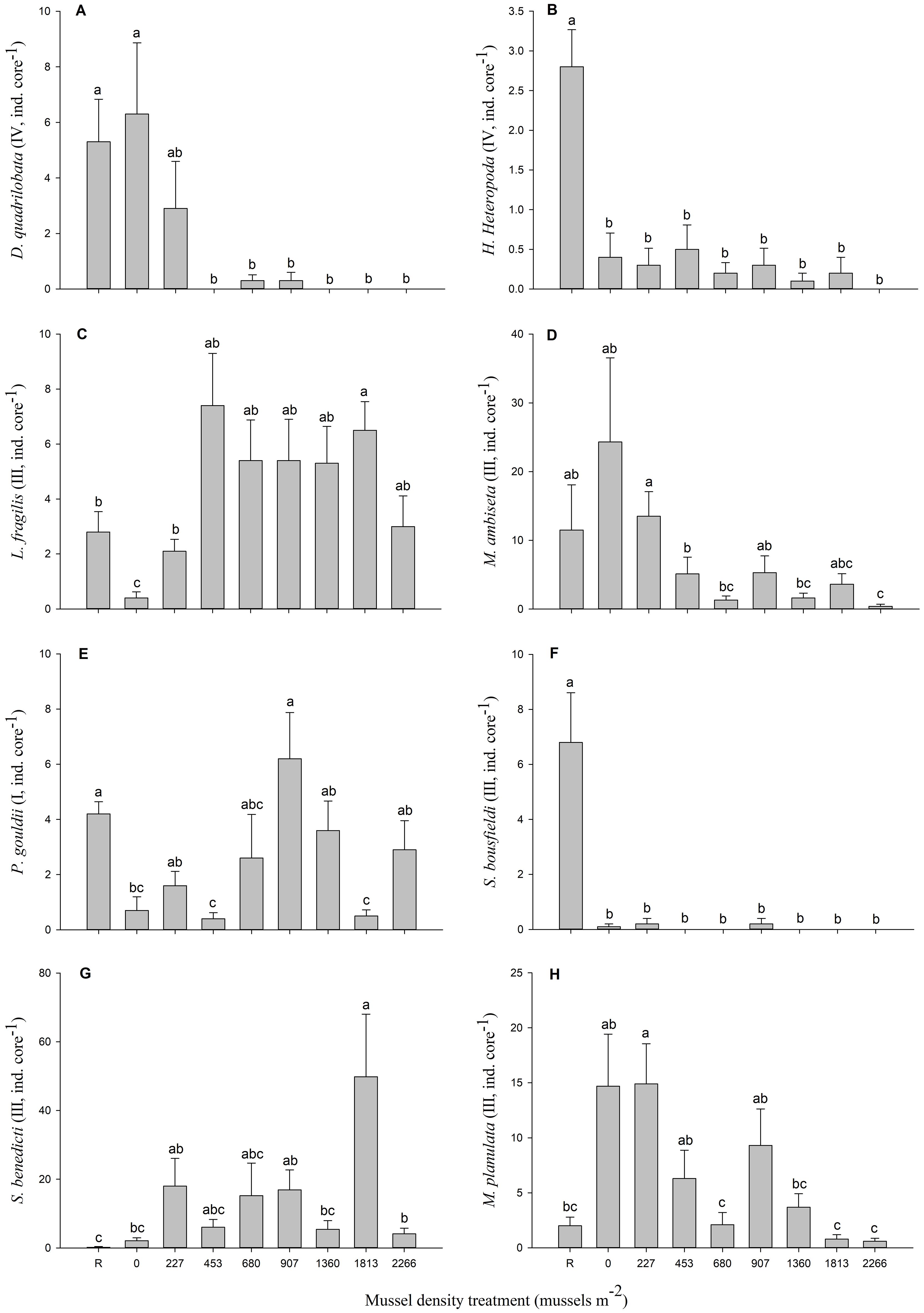
Figure 6. Mean (± SE) abundance per core of eight species that explain 30 to 35% of dissimilarity (SIMPER analysis) between mussel density treatments: (A) Dipolydora quadrilobata, (B) Hypereteone heteropoda, (C) Leitoscoloplos fragilis, (D) Mediomastus ambiseta, (E) Pectinaria gouldii, (F) Scolelepis (Parascolelepis) bousfieldi, (G) Streblospio benedicti and (H) Mysella planulata. For each mussel density, n = 10 (five mesocosms with two replicate cores each). Differences between mussel density means are indicated by different letters (p < 0.05).
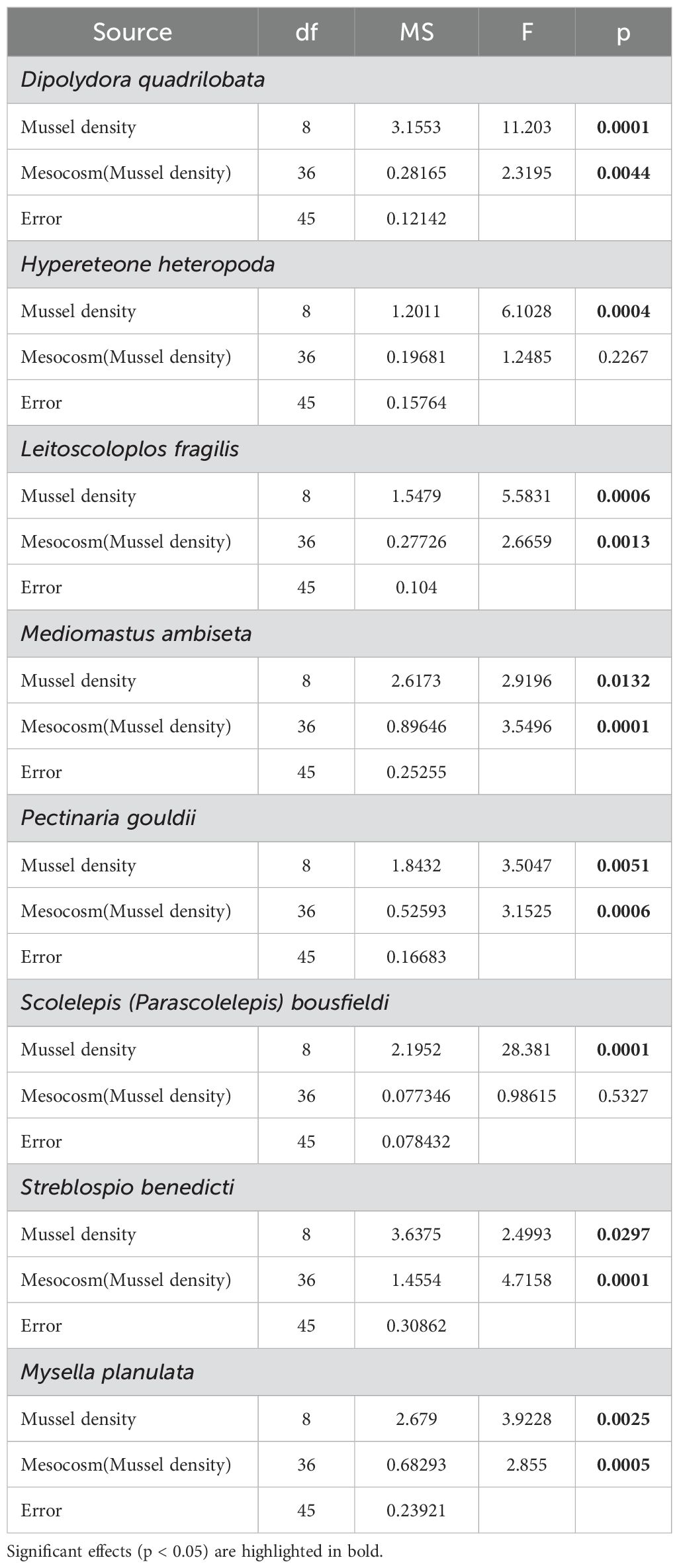
Table 4. Results of PERMANOVA evaluating the effect of Mussel density and Mesocosm(Mussel density) on eight species that explain about 30 to 35% of dissimilarity between treatments.
Community condition evaluated using AMBI shows all treatments to be only slightly disturbed (mean biotic index = 2). Species from Group III (those tolerant to organic enrichment) were in general more abundant than the other groups, especially for mussel density treatment 1813 (Figure 7). No species from group V (first-order opportunistic species) were observed at any mussel density treatment. AMBI groupings identified eight species that play a role in the dissimilarity between treatments (Group I: P. gouldii; Group III: L. fragilis, M. ambiseta, S. bousfieldi, S. benedicti and M. planulata; and Group IV: D. quadrilobata and H. heteropoda). Ecological status estimated by M-AMBI indicated a ‘High’ ecological status (0.8402 to 0.9785) for all the treatments except for the 1813 mussel density that received a ‘Good’ ecological status mention (0.7560).
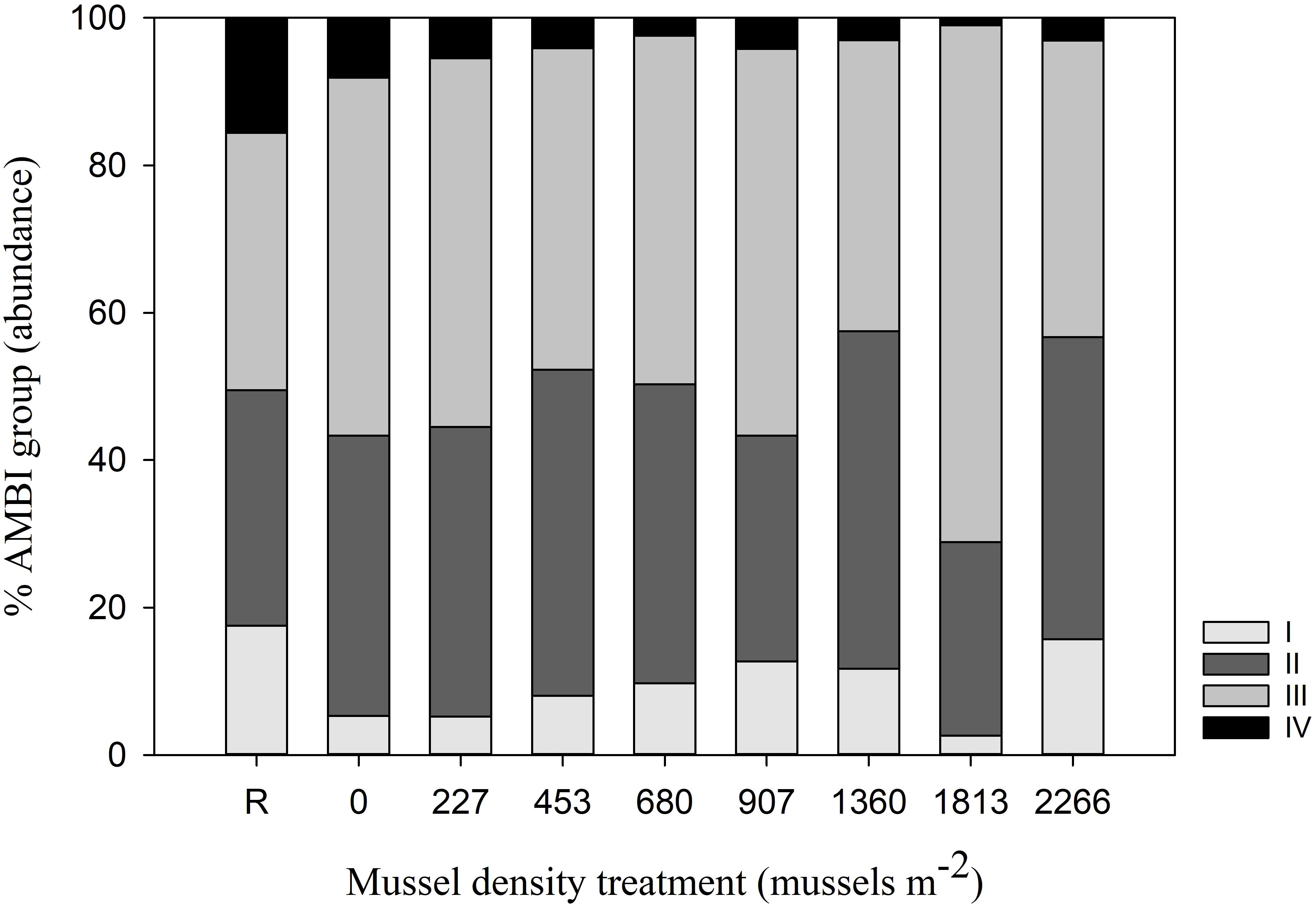
Figure 7. Mean relative abundance (%) of infauna classified in ecological groups according to their sensitivity to an increasing pollution gradient (organic enrichment): I, species very sensitive; II, species indifferent; III, species tolerant; IV, second-order opportunistic species; and V, first-order opportunistic species (none identified) (Borja et al., 2000).
3.4.2 Relationship with the total free sulfides
The correlation between TFS and abundance (coefficient correlation = -0.37) and richness (coefficient correlation = -0.57) were not very strong (Figure 8), suggesting that the level of sulfides failed to explain a great proportion of the total variation in infauna abundance (r2 = 0.13) and richness (r2 = 0.33) although these relationships are statistically significant (p < 0.05). A 50% reduction in abundance and richness are represented by 1376 and 1538 μM TFS, respectively. Considerable variation is present in the dataset, both between treatments and between samples in the same treatment.
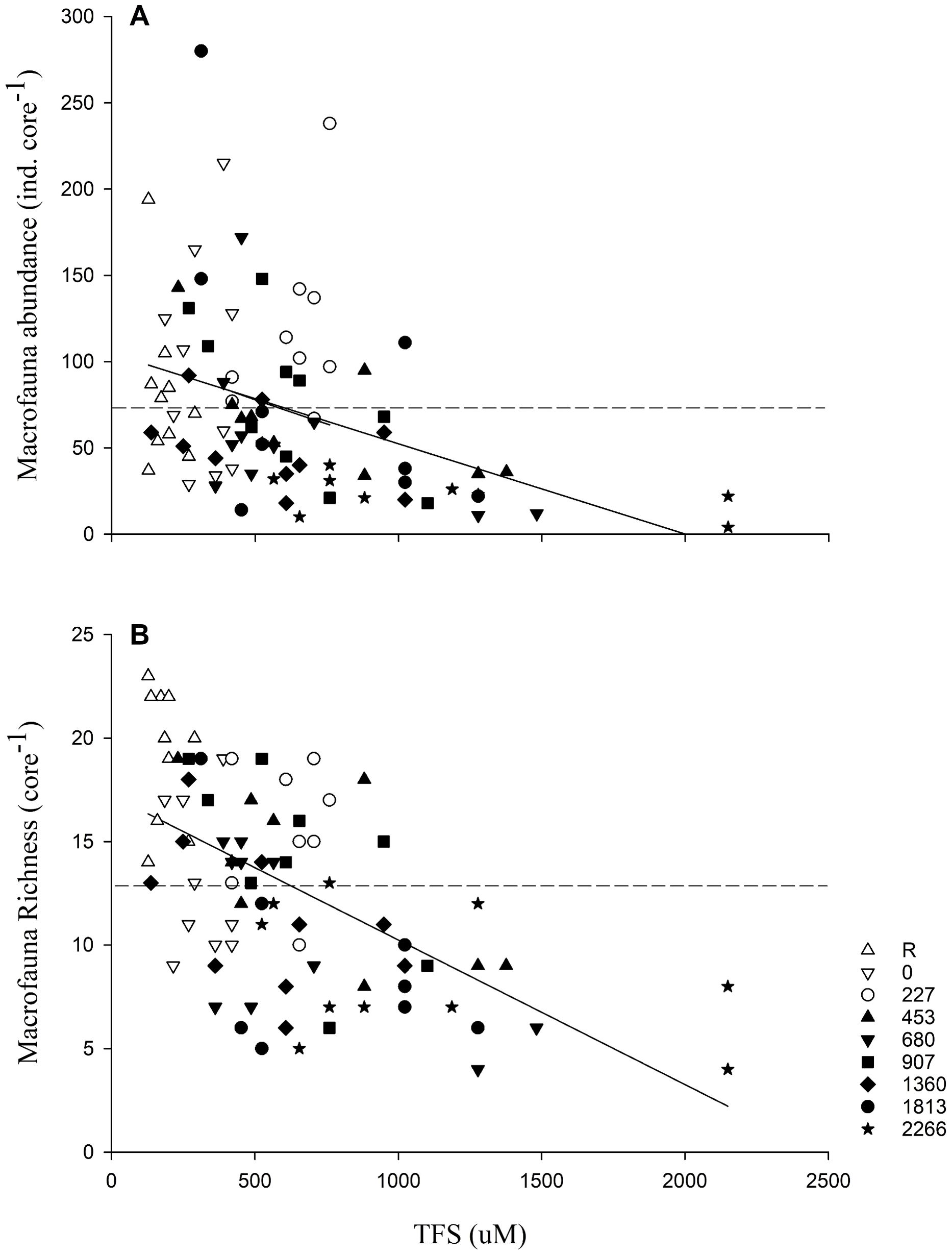
Figure 8. Relationship between TFS (µM) and infauna (A) abundance (y = 103.94 – 0.049x; r2 = 0.13; p = 0.0005) and (B) richness (y = 17.27 – 0.007x; r2 = 0.33; p < 0.0001) for each mussel treatment density (n = 10). The dashed line in each graph represents the mean (N = 73 individuals and S = 13 species) for all the data.
4 Discussion
The use of mesocosms that are open to the natural environment allows for recruitment and migration of animals into them while allowing modification of biodeposition rates from mussels to mimic various levels of farm-related biodeposition. In this study, the experiment was, in general, a success in that organic loading increased with higher mussel densities. The results from this study suggest that much of St. Peters Bay is likely impacted due to biodeposition from the mussels farmed there. Many other studies have noted this (see reviews in McKindsey et al., 2011 and Filgueira et al., 2015).
4.1 Sedimentation conditions in St. Peters Bay
Background sedimentation from the water column in SPB had an impact on mesocosms as the 0 mussel treatment m-2 received considerable sedimentation (especially in July). In July, all treatments, except the 0 mussel m-2 one, received a TOM value judged to be sufficient to affect sediment characteristics and create transitory hypoxic conditions (ca. 15 g m-2 d-1; Hargrave et al., 2008b; Callier et al., 2009; Weise et al., 2009). In August, 453 mussels m-2 were needed to alter sediment characteristics. Other mesocosm studies found organic enrichment greater than 15 g m-2 d-1 at various densities of mussels: 764 mussels m-2 (Callier et al., 2009) and 200 to 1600 mussels m-2 (Lacoste et al., 2019). Based on a theoretical biodeposition rates of 0.022 g mussel-1 d-1 calculated by Callier et al. (2009); Robert et al. (2013) suggested that between 600 and 800 mussels m-2 would be needed to attain the 15 g m-2 d-1 threshold. Organic enrichment from mussels seems to depend on other factors in addition to local current regimes and depth. The time (month, day) when biodeposition was measured greatly impacted the density of mussels needed to obtain the organic sedimentation threshold. Hydrodynamic regimes within sites (Hartstein and Stevens, 2005; Giles et al., 2009; Keeley et al., 2013; Guyondet et al., 2015), quality of sedimenting material (Martinez-Garcia et al., 2015), presence of epibionts (McKindsey et al., 2009; Lacoste and Gaertner-Mazouni, 2015), and temperature (Jansen et al., 2012) also likely influence organic enrichment and decomposition, thus affecting benthic impacts under farms. Water renewal time in SPB is relatively low and characterized by poor flushing with aquaculture development (expressed as total bay area cover) among the highest in the world relative to other bivalve production areas (Guyondet et al., 2015) and by organic enrichment from agriculture (Cranford et al., 2009), potentially explaining the high sedimentation in the bay.
4.2 Sediment response to organic enrichment
This study clearly shows what was expected that biodeposition from high densities of mussels induces organic enrichment and impacts several sediment parameters in SPB. Increased %OM and %WC in sediments receiving biodeposition from high densities of mussels suggest that the seafloor received more organic loading that it can mineralize (Robert et al., 2013). In SPB, %OM in surficial sediments increased six to ten times that observed in reference sites with high mussel densities, going from 2.2% in reference sites to 12.01% for 453 mussels m-2 and to 20.69% for 2266 mussels m-2, which is greater than the value observed by McKindsey and Weise (unpublished data) for the same density of mussels (12% at 1813 and 2266 mussels m-2) but at a different region. Moreover, only 227 mussels m-2 was needed to obtain 5% OM in surficial sediments compared to other mesocosm studies (5.5%: 764 mussels m-2, Callier et al., 2009; 5%: 1200 mussels m-2, Robert et al., 2013; and 5%: 400 mussels m-2, Lacoste et al., 2019). However, levels measured in the present study (from 12 to 21% for 453 to 2266 mussels m-2, respectively) were more similar to levels typically observed under shellfish farms (24%: Dahlbäck and Gunnarsson, 1981; 13-22%: Mattsson and Lindén, 1983; 15-20%: Deslous-Paoli et al., 1998; 12-13%: Christensen et al., 2003; 11-13%: Cranford et al., 2009). This study was done during summer, when mussels produce more biodeposits (Hatcher et al., 1994), potentially explaining the high %OM observed in benthic sediments. As also mentioned in above section, PEI embayments are also known to be subject to anthropogenic organic enrichment from agriculture (Cranford et al., 2009), which also likely contributes to the observed high %OM.
The side-view images of sediment cores show a large black layer indicative of reduced sediments in treatments with 453 to 1813 mussels m-2, contrasting with the lighter oxidized sediments of the other treatments and indicating the degree of sediment impact due to biodeposition from mussel farms. The black layers observed with 453 mussels m-2 and more correspond at a TOM ≥ 15 g m-2 d-1 (see Table 1). McKindsey and Weise (unpublished data) also observed a larger black layer of reduced sediments when 453 mussels m-2 added biodeposits to the mesocosms in their study with 10.0 ± 4.7 g TOM m-2 d-1 compared to 19.28 ± 2.09 g TOM m-2 d-1 in July and 16.78 ± 1.60 g TOM m-2 d-1 in August observed in this study. Even if the threshold of 15 g TOM m-2 d-1 was not reached, McKindsey and Weise (unpublished data) started to observe changes in sediments at this density of mussels.
Measurements of EhNHE and TFS are considered good indicators to evaluate the impact of benthic enrichment from suspended mussels (Hargrave et al., 2008a; Cranford et al., 2009). However, this study found that EhNHE and TFS concentrations were inconsistent, indicating different sediment enrichment classes for a given mussel density. Hargrave et al. (2008a) shows that these indicators have a negative linear relationship with increasing biodeposition. In this study, TFS indicated that sediment under almost all mussel densities was oxic whereas EhNHE indicated hypoxic conditions from 227 mussels m-2. These parameters were also found to not be sensitive enough to detect the effect of biodeposition by mussel farms (Anderson et al., 2005; Miron et al., 2005; Callier et al., 2007), especially in environments where organic matter content is naturally high (Anderson et al., 2005). A new approach to analyze TFS was determined (Cranford et al., 2020; Cranford, 2024) because the standard ion selective electrode (ISE) method (used in this study) can give biased results. Wildish et al. (2004) reported that ion analytical methods for EhNHE can give false results if the probes become “poisoned” and if a reconditioning is not done. The same study also suggest that EhNHE measurements can be only used semi-quantitatively to validate TFS where sediments are hypoxic or anoxic. Interpretation of these parameters using ISE methods warrant attention to ensure they may detect the organic loading.
4.3 Infaunal community reactions: presence of a “disturbed balance”
In general, organic enrichment can stress benthic infaunal communities and change their structure with a concomitant loss of organism abundances and richness (Pearson and Rosenberg, 1978) and an increase of opportunistic species (Pearson and Rosenberg, 1978; Norkko et al., 2006). Changes in benthic communities due to organic loading from mussel farms is well known (see McKindsey et al., 2011 and references therein). In SPB, the benthic environment was hypoxic under some loading conditions, as indicated by some sediment parameters (sediment profile images and %OM), and biodeposition influenced benthic community structure, up to a certain level, as described in Pearson and Rosenberg (1978). Thise trend was evident for infaunal abundance, which decreased for mesocosms receiving biodeposition from greater than 453 mussels m-2, the density at which organic matter increased significantly. At a density of 1813 mussels m-2, a more tolerant species (S. benedicti) was much more abundant. In contrast, the infaunal community indices AMBI and M-AMBI did not respond at this same level of organic enrichment. M-AMBI results showed the ecological status of the infaunal communities for the various mussel density treatments to be “High,” although organic enrichment was elevated at high mussel densities. AMBI indicated that species from group III (tolerant species) were dominant in each treatment, closely followed by group II species (indifferent to enrichment). Given that a preponderance of group III species was observed, it may be concluded that the benthic community has adapted to SPB conditions: low renewal water time (80 days at the head of the bay and 30 to 40 days where the experiment was done, Guyondet et al., 2015), high aquaculture development (Guyondet et al., 2015), and organic enrichment from agriculture (Cranford et al., 2009). Other studies (Callier et al., 2007; Lacoste et al., 2019) have also observed inconsistencies between the benthic ecosystem responses and the organic enrichment. Lacoste et al. (2019) observed that abundance and richness were greater with organic loading, whereas no effect was detected on sediment indicators (%OM, sediment composition, and number of bacteria per sample), suggesting a potential reset of the benthic condition due to autumn storms and related resuspension of benthic sediments. Valdemarsen et al. (2010) also suggest that well established infaunal communities may counteract the effects of surface enrichment by stimulating the transport of O2 into sediments.
Of the eight species that played an important role in defining the dissimilarity between mussel treatments, one was from AMBI group I (very sensitive species), five were from group III (tolerant species), and two from the group IV (second-order opportunistic species). The species from group I, P. gouldii, was not only present in mesocosms subjected to biodeposition from low mussel densities in mesocosms but also in mesocosms where organic enrichment was higher. P. gouldii is a polychaete that builds and lives in cone-shaped tubes of sand grains. Settlement is reduced in muddy sediments because it can be easily buried and die under organic-rich sediments (Fuller et al., 2021). Accordingly, it’s curious that this polychaete was also found in treatments with abundant organic matter, although Lacoste et al. (2019) also found this worm associated with higher mussel densities. A related species, Cistenides granulata, was found in abundance under and around mussel farms of nearby Îles-de-la-Madeleine (Callier et al., 2008). Of the five species in AMBI group III, only two polychaetes (L. fragilis and S. benedicti) had increased abundance with organic enrichment. Abundances of the three others (polychaetes M. ambiseta and S. bousfieldi and the mollusc M. planulata) decreased with increasing mussel densities. Tolerant (group III) species are found under normal conditions, although their abundance may be stimulated by organic enrichment and second-order opportunistic species (group IV) are found under slightly to pronounced enriched conditions (Borja et al., 2000). The abundance of the two polychaetes from group IV (D. quadrilobata and H. heteropoda) decreased with organic enrichment. Khaitov et al. (1999) observed that D. quadrilobata was associated with strong organic enrichment and particularly by organic matter produced by wild mussels.
Hargrave et al. (2008b) observed a correlation between benthic infauna parameters and TFS such that diversity under or close the fish pens decreased with increasing TFS with a decrease of 50% in abundance and richness occurring when TFS reached 300 μM and 1000 μM, respectively. In the present study, a TFS near 1376 μM and 1538 μM, respectively, was needed to decrease abundance and richness by 50%, although the correlation between TFS and infaunal parameters was not great (low r2). Other parameters, including those mentioned above, as current regimes, depth, etc., likely account for changes in benthic communities, or the community has reached a balanced state (Aguado-Giménez et al., 2012). The study was done over 12 weeks and results suggest that this was sufficient to create benthic organic loading responses in SPB. However, some macrofaunal parameters mentioned above did not react as expected with increasing organic loading suggesting that maybe a longer study was needed to see the changes in benthic communities as suggested by Aguado-Giménez et al. (2012).
5 Conclusion
Mesocosms may be used to create various levels of biodeposition from mussels even if conclusions may not be generalized and extended to predict outcomes in all mussel farms. In this study, the results suggest that St. Peters Bay is impacted by the biodeposition from mussel farms even if twelve weeks was insufficient to completely ‘switch’ infaunal communities and related sediment parameters. This study demonstrates that measurement of multiple parameters is important to interpret benthic responses to organic enrichment due to mussel biodeposition.
Data availability statement
The raw data supporting the conclusions of this article will be made available by the authors, without undue reservation.
Ethics statement
The manuscript presents research on animals that do not require ethical approval for their study.
Author contributions
M-FL: Writing – review & editing, Writing – original draft, Visualization, Formal analysis. ÉL: Writing – review & editing, Formal analysis. AW: Writing – review & editing, Methodology, Conceptualization. CM: Writing – review & editing, Methodology, Funding acquisition, Conceptualization.
Funding
The author(s) declare financial support was received for the research, authorship, and/or publication of this article. This work was funded by the DFO Program for Aquaculture Regulatory Research (PARR-2010-QC-05) to CM.
Acknowledgments
We thank the mussel growers for their cooperation and Stephen Fortune for allowing us to work in his mussel lease. Gwenaëlle Chaillou, Matthieu Huot, Anaïs Lacoursière, Lysandre Landry, François Roy, Martin Protat, and Geneviève Perrin provided invaluable technical assistance during the field work.
Conflict of interest
The authors declare that the research was conducted in the absence of any commercial or financial relationships that could be construed as a potential conflict of interest.
Publisher’s note
All claims expressed in this article are solely those of the authors and do not necessarily represent those of their affiliated organizations, or those of the publisher, the editors and the reviewers. Any product that may be evaluated in this article, or claim that may be made by its manufacturer, is not guaranteed or endorsed by the publisher.
References
Aguado-Giménez F., Piedecausa M. A., Gutierrez J. M., Garcia-Charton J. A., Belmonte A., Garcia-Garcia B. (2012). Benthic recovery after fish farming cessation: a “beyond-BACI” approach. Mar. pollut. Bull. 64, 729–738. doi: 10.1016/j.marpolbul.2012.02.012
Anderson M. J. (2001). A new method for non-parametric multivariate analysis of variance. Austral. Ecol. 26, 32–45. doi: 10.1111/j.1442-9993.2001.01070.pp.x
Anderson M. J., Gorley R. N., Clarke K. R. (2008). PERMANOVA+ for PRIMER: Guide to software and statistical methods (Plymouth, UK: PRIMER-E).
Anderson M. R., Tlusty M. F., Pepper V. A. (2005). Organic enrichment at cold water aquaculture sites – the case of coastal Newfoundland. Hdb. Env. Chem. 5, 99–113. doi: 10.1007/b136006
Baudinet D., Alliot E., Berland B., Grenz C., Plante-Cuny M., Plante R., et al. (1990). Incidence of mussel culture on biogeochemical fluxes at the sediment-water interface. Hydrobiologia 207, 187–196. doi: 10.1007/BF00041456
Borja A., Franco J., Pérez V. (2000). A marine biotic index to establish the ecological quality of soft-bottom benthos within European estuarine and coastal environments. Mar. pollut. Bull. 40, 1100–1114. doi: 10.1016/S0025-326X(00)00061-8
Callier M. D., McKindsey C. W., Desrosiers G. (2007). Multi-scale spatial variations in benthic sediment geochemistry and macrofaunal communities under a suspended mussel culture. Mar. Ecol. Prog. Ser. 348, 103–115. doi: 10.3354/meps07034
Callier M. D., McKindsey C. W., Desrosiers G. (2008). Evaluation of indicators used to detect mussel farm influence on the benthos: two case studies in the Magdalen Islands, Eastern Canada. Aquaculture 278, 77–88. doi: 10.1016/j.aquaculture.2008.03.026
Callier M. D., Richard M., McKindsey C. W., Archambault P., Desrosiers G. (2009). Responses of benthic macrofauna and biogeochemical fluxes to various levels of mussel biodeposition: an in situ “benthocosm” experiment. Mar. pollut. Bull. 58, 1544–1553. doi: 10.1016/j.marpolbul.2009.05.010
Chivilev S., Ivanov M. (1997). Response of the arctic benthic community to excessive amounts of nontoxic organic matter. Mar. pollut. Bull. 35, 280–286. doi: 10.1016/S0025-326X(97)00094-5
Christensen P. B., Glud R. N., Dalsgaard T., Gillespie P. (2003). Impacts of longline mussel farming on oxygen and nitrogen dynamics and biological communities of coastal sediments. Aquaculture 218, 567–588. doi: 10.1016/S0044-8486(02)00587-2
Clarke K. R., Warwick R. M. (2001). Change in marine communities: an approach to statistical and interpretation, 2nd edition (Plymouth: PRIMER-E).
Cranford P., Brager L., Elvines D., Wong D., Law B. (2020). A revised classification system describing the ecological quality status of organically enriched marine sediments based on total dissolved sulfides. Mar. Pollut. Bull. 154, 111088. doi: 10.1016/j.marpolbul.2020.111088
Cranford P. J. (2024). A simple and rapid method for measuring total free sulfides in marine sediments. Limnol. Oceanogr. 22, 608–617. doi: 10.1002/lom3.10619
Cranford P. J., Hargrave B. T., Doucette L. I. (2009). Benthic organic enrichment from suspended mussel (Mytilus edulis) culture in Prince Edward Island, Canada. Aquaculture 292, 189–196. doi: 10.1016/J.AQUACULTURE.2009.04.039
Crawford C., Macleod C. K. A., Mitchell I. M. (2003). Effects of shellfish farming on the benthic environment. Aquaculture 224, 117–140. doi: 10.1016/S0044-8486(03)00210-2
Dahlbäck B., Gunnarsson L.Å.H. (1981). Sedimentation and sulfate reduction under a mussel culture. Mar. Biol. 63, 269–275. doi: 10.1007/BF00395996
Deslous-Paoli J. M., Souchu P., Mazouni N., Juge C., Dagault F. (1998). Relations milieu-ressources: impact de la conchyliculture sur un environnement launaire mediterraneen (Thau). Oceanol. Acta 21, 831–843. doi: 10.1016/S0399-1784(99)80010-3
Dumbauld B. R., Ruesink J. L., Rumrill S. S. (2009). The ecological role of bivalve shellfish aquaculture in the estuarine environment: a review with application to oyster and clam culture in West Coast (USA) estuaries. Aquaculture 290, 196–223. doi: 10.1016/j.aquaculture.2009.02.033
Elvines D. M., MacLeod C. K., Ross D. J., Hopkins G. A., White C. A. (2023). Fate and effects of fish farm organic waste in marine systems: Advances in understanding using biochemical approaches with implications for environmental management. Rev. Aquacult 16, 66–85. doi: 10.1111/raq.12821
Filgueira R., Comeau L. A., Guyondet T., McKindsey C. W., Byron C. (2015). “Modelling carrying capacity of bivalve aquaculture: a review of definitions and methods,” in Encyclopedia of Sustainability Science and Technology. Ed. Meyers R. A. (Reference Editorial Office, Springer, New York), 33. doi: 10.1007/978-1-4939-2493-6_945-1
Fisheries and Oceans Canada (2022). Aquaculture production and value. Available online at: https://www.dfo-mpo.gc.ca/stats/aqua/aqua21-eng.htm (Accessed May 14, 2024).
Forget N., Duplisea D. E., Sardenne F., McKindsey C. W. (2020). Using qualitative network models to assess the influence of mussel culture on benthic ecosystem dynamics. Ecol. Model. 430, 109070. doi: 10.1016/j.ecolmodel.2020.109070
Fuller K. M., Fox A. L., Jacoby C. A., Trefry J. H. (2021). Biological abundance and diversity in organic-rich sediments from a Florida barrier island lagoon. Front. Mar. Sci. 8. doi: 10.3389/fmars.2021.768083
Gallardi D. (2014). Effects of bivalve aquaculture on the environment and their possible mitigation: A review. Fish. Aquacult. J. 5, 1–8. doi: 10.4172/2150-3508.1000105
Giles H., Broekhuizen N., Bryan K. R., Pilditch C. A. (2009). Modelling the dispersal of biodeposits from mussel farms: The importance of simulating biodeposit erosion and decay. Aquaculture 291, 168–178. doi: 10.1016/j.aquaculture.2009.03.010
Grant J., Hatcher A., Scott D. B., Pocklington P., Schafer C. T., Winters G. V. (1995). A multidisciplinary approach to evaluating impacts of shellfish aquaculture on benthic communities. Estuaries 18, 124–144. doi: 10.2307/1352288
Guyondet T., Comeau L. A., Bacher C., Grant J., Rosland R., Sonier R., et al. (2015). Climate change influences carrying capacity in a coastal embayment dedicated to shellfish aquaculture. Estuar. Coasts 38, 1593–1618. doi: 10.1007/s12237-014-9899-x
Hargrave B. T., Doucette L. I., Cranford P. J., Law B. A., Milligan T. G. (2008a). Influence of mussel aquaculture on sediment organic enrichment in a nutrient-rich coastal embayment. Mar. Ecol. Prog. Ser. 365, 137–149. doi: 10.3354/MEPS07636
Hargrave B. T., Holmer M., Newcombe C. P. (2008b). Towards a classification of organic enrichment in marine sediments based on biogeochemical indicators. Mar. pollut. Bull. 56, 810–824. doi: 10.1016/j.marpolbul.2008.02.006
Hartstein N. D., Stevens C. L. (2005). Deposition beneath long-line mussel farms. Aquacult. Eng. 33, 192–213. doi: 10.1016/j.aquaeng.2005.01.002
Hatcher A., Grant J., Schofield B. (1994). Effects of suspended mussel culture (Mytilus spp.) on sedimentation, benthic respiration and sediment nutrient dynamics in a coastal bay. Mar. Ecol. Prog. Ser. 115, 219–235. doi: 10.3354/meps115219
Jansen H., Verdegem M., Strand Ø., Smaal A. (2012). Seasonal variation in mineralization rates (C-N-P-Si) of mussel Mytilus edulis biodeposits. Mar. Biol. 159, 1567–1580. doi: 10.1007/s00227-012-1944-3
Keeley N. B., Forrest B. M., Macleod C. K. (2013). Novel observations of benthic enrichment in contrasting flow regimes with implications for marine farm monitoring and management. Mar. pollut. Bull. 66, 105–116. doi: 10.1016/j.marpolbul.2012.10.024
Khaitov V. M., Fokin M. V., Nicolaeva A. M. (1999). “Structure of communities associated with dense assemblages of the tube-dwelling polychaete Polydora quadrilobata Jacobi (Spionidae) in the White Sea,” in Biological, Physical and Geochemical Features of Enclosed and Semi-enclosed Marine Systems: Proceedings of the Joint BMB 15 and ECSA 27 Symposium, 9–13 June 1997. Eds. Maria E., Blomqvist E., Bonsdorff K. E. (Springer, Åland Islands, Finland), 221–226.
Kristensen E. (1990). Characterization of biogenic organic matter by stepwise thermogravimetry (STG). Biogeochemistry 9, 139–159. doi: 10.1007/BF00692169
Lacoste É., Gaertner-Mazouni N. (2015). Biofouling impact on production and ecosystem functioning: a review for bivalve aquaculture. Rev. Aquacult. 7, 187–196. doi: 10.1111/raq.12063
Lacoste É., Drouin A., Weise A. M., Archambault P., McKindsey C. W. (2018). Low benthic impact of an offshore mussel farm in Îles-de-la-Madeleine, eastern Canada. Aquacult. Environ. Interact. 10, 473–485. doi: 10.3354/aei00283
Lacoste É., McKindsey C. W., Archambault P. (2020). Biodiversity–Ecosystem Functioning (BEF) approach to further understanding aquaculture–environment interactions with application to bivalve culture and benthic ecosystems. Rev. Aquacult. 12, 2027–2041. doi: 10.1111/raq.12420
Lacoste É., Weise A. M., Lavoie M.-F., Archambault P., McKindsey C. W. (2019). Changes in infaunal assemblage structure influence nutrient fluxes in sediment enriched by mussel biodeposition. Sci. Total Environ. 692, 39–48. doi: 10.1016/j.scitotenv.2019.07.235
Martinez-Garcia E., Carlsson M. S., Sanchez-Jerez P., Sánchez-Lizaso J. L., Sanz-Lazaro C., Holmer M. (2015). Effect of sediment grain size and bioturbation on decomposition of organic matter from aquaculture. Biogeochemistry 125, 133–148. doi: 10.1007/s10533-015-0119-y
Mattsson J., Lindén O. (1983). Benthic macrofauna succession under mussels, Mytilus edulis L. (Bivalvia), cultured on hanging long-lines. Sarsia 68, 97–102. doi: 10.1080/00364827.1983.10420561
McKindsey C. W., Archambault P., Callier M. D., Olivier F. (2011). Influence of suspended and off-bottom mussel culture on the sea bottom and benthic habitats: a review. Can. J. Zool. 89, 622–646. doi: 10.1139/z11-037
McKindsey C. W., Archambault P., Simard N. (2012). Spatial variation of benthic infaunal communities in baie de Gaspé (eastern Canada) – influence of mussel aquaculture. Aquaculture 356–357, 48–54. doi: 10.1016/j.aquaculture.2012.05.037
McKindsey C. W., Lecuona M., Huot M., Weise A. M. (2009). Biodeposit production and benthic loading by farmed mussels and associated tunicate epifauna in Prince Edward Island. Aquaculture 295, 44–51. doi: 10.1016/j.aquaculture.2009.06.022
Michaud E., Desrosiers G., Aller R. C., Mermillod-Blondin F., Sundby B., Stora G. (2009). Spatial interactions in the Macoma balthica community control biogeochemical fluxes at the sediment-water interface and microbial abundances. J. Mar. Res. 67, 43–70. doi: 10.1357/002224009788597926
Miron G., Landry T., Archambault P., Frenette B. (2005). Effects of mussel culture husbandry practices on various benthic characteristics. Aquaculture 250, 138–154. doi: 10.1016/j.aquaculture.2005.01.030
Muxika I., Borja A., Bald J. (2007). Using historical data, expert judgement and multivariate analysis in assessing reference conditions and benthic ecological status, according to the European Water Framework Directive. Mar. pollut. Bull. 55, 16–29. doi: 10.1016/j.marpolbul.2006.05.025
Naylor R. L., Hardy R. W., Buschmann A. H., Bush S. R., Cao L., Klinger D. H., et al. (2021). A 20-year retrospective review of global aquaculture. Nature 591, 551–563. doi: 10.1038/s41586-021-03308-6
Newell R. I. E. (2004). Ecosystem influences of natural and cultivated populations of suspension-feeding bivalve molluscs: a review. J. Shellfish Res. 23, 51–61.
Norkko A., Rosenberg R., Thrush S. F., Whitlatch R. B. (2006). Scale-and intensity-dependent disturbance determines the magnitude of opportunistic response. J. Exp. Mar. Biol. Ecol. 330, 195–207. doi: 10.1016/j.jembe.2005.12.027
Pearson T. H., Rosenberg R. (1978). Macrobenthic succession in relation to organic enrichment and pollution of the marine environment. Oceanogr. Mar. Biol. Annu. Rev. 16, 229–311. doi: 10.2983/035.034.0121u1.10
Richard M., Archambault P., Thouzeau G., Desrosiers G. (2006). Influence of suspended mussel lines on the biogeochemical fluxes in adjacent water in the Îles-de-la-Madeleine (Quebec, Canada). Can. J. Fish. Aquat. Sci. 63, 1198–1213. doi: 10.1139/F06-030
Richard M., Archambault P., Thouzeau G., Desrosiers G. (2007a). Summer influence of 1 and 2 yr old mussel cultures on benthic fluxes in Grande-Entrée lagoon, Îles-de-la-Madeleine (Québec, Canada). Mar. Ecol. Prog. Ser. 338, 131–143. doi: 10.3354/meps338131
Richard M., Archambault P., Thouzeau G., McKindsey C. W., Desrosiers G. (2007b). Influence of suspended scallop cages and mussel lines on pelagic and benthic biogeochemical fluxes in Havre-aux-Maisons Lagoon, Îles-de-la-Madeleine (Quebec, Canada). Can. J. Fish. Aquat. Sci. 64, 1491–1505. doi: 10.1139/F07-116
Robert P., McKindsey C. W., Chaillou G., Archambault P. (2013). Dose-dependent response of a benthic system to biodeposition from suspended blue mussel (Mytilus edulis) culture. Mar. pollut. Bull. 66, 92–104. doi: 10.1016/j.marpolbul.2012.11.003
Sonier R., Filgueira R., Guyondet T., Tremblay R., Olivier F., Meziane T., et al. (2016). Picophytoplankton contribution to Mytilus edulis growth in an intensive culture environment. Mar. Biol. 163, 73. doi: 10.1007/s00227-016-2845-7
Stenton-Dozey J. M. E., Jackson L. F., Busby A. J. (1999). Impact of mussel culture on macrobenthic community structure in Saldanha Bay, South Africa. Mar. pollut. Bull. 39, 357–366. doi: 10.1016/S0025-326X(98)00180-5
Sun X., Dong J., Hu C., Zhang Y., Chen Y., Zhang X. (2021). Use of macrofaunal assemblage indices and biological trait analysis to assess the ecological impacts of coastal bivalve aquaculture. Ecol. Indic. 127, 107713. doi: 10.1016/j.ecolind.2021.107713
Valdemarsen T., Kristensen E., Holmer M. (2010). Sulfur, carbon, and nitrogen cycling in faunated marine sediments impacted by repeated organic enrichment. Mar. Ecol. Prog. Ser. 400, 37–53. doi: 10.3354/meps08400
Weise A. M., Cromey C. J., Callier M. D., Archambault P., Chamberlain J., McKindsey C. W. (2009). Shellfish-DEPOMOD: Modelling the biodeposition from suspended shellfish aquaculture and assessing benthic effects. Aquaculture 288, 239–253. doi: 10.1016/j.aquaculture.2008.12.001
Wildish D., Akagi H. M., Hargrave B. T., Strain P. M. (2004). Inter-laboratory calibration of redox potential and total sulfide measurements in interfacial marine sediments and the implications for organic enrichment assessment. Can. Tech. Rep. Fish. Aquat. Sci. 2546, iii + 25 p. doi: 10.1080/00288330.2010.550628.
Wildish D. J., Akagi H. M., Hamilton N., Hargrave B. T. (1999). A recommended method for monitoring sediments to detect organic enrichment from mariculture in the Bay of Fundy. Can. Tech Rep. Fish. Aquat. Sci. 2286, iii + 31 p.
Wildish D. J., Hargrave B. T., Macleod C., Crawford C. (2003). Detection of organic enrichment near finfish net-pens by sediment profile imaging at SCUBA-accessible depths. J. Exp. Mar. Biol. Ecol. 285-286, 403–413. doi: 10.1016/S0022-0981(02)00540-3
Keywords: biodeposition, organic enrichment, mussel aquaculture, infaunal macrobenthic communities, Mytilus edilus
Citation: Lavoie M-F, Lacoste É, Weise AM and McKindsey CW (2024) Benthic responses to organic enrichment under a mussel (Mytilus edulis) farm. Front. Mar. Sci. 11:1433365. doi: 10.3389/fmars.2024.1433365
Received: 15 May 2024; Accepted: 05 August 2024;
Published: 23 August 2024.
Edited by:
Nafsika Papageorgiou, National and Kapodistrian University of Athens, GreeceReviewed by:
Pablo Muniz, University of the Republic, UruguayMauricio Shimabukuro, Universidade Federal do Rio Grande, Brazil
Copyright © 2024 Lavoie, Lacoste, Weise and McKindsey. This is an open-access article distributed under the terms of the Creative Commons Attribution License (CC BY). The use, distribution or reproduction in other forums is permitted, provided the original author(s) and the copyright owner(s) are credited and that the original publication in this journal is cited, in accordance with accepted academic practice. No use, distribution or reproduction is permitted which does not comply with these terms.
*Correspondence: Christopher W. McKindsey, Y2hyaXMubWNraW5kc2V5QGRmby1tcG8uZ2MuY2E=