- 1College of Environmental Science and Engineering, Guilin University of Technology, Guilin, China
- 2Guangxi Key Laboratory of Environmental Pollution Control Theory and Technology, Guilin, China
- 3Guangxi Collaborative Innovation Center for Water Pollution Control and Water Safety in Karst Areas, Guilin, China
- 4College of Fisheries, Ocean University of China, Qingdao, China
- 5College of Fisheries, Guangdong Ocean University, Zhanjiang, China
Invertebrates can store carbon (C), nitrogen (N), phosphorus (P), and other elements in their body tissues at theoretically homeostatic rates, thus playing an important role in the biogeochemical cycle of aquatic ecosystems. To sustain homeostasis, consumers must either balance their resource supply or adjust their stoichiometric features in response to environmental changes. However, there is limited understanding regarding potential differences in the ecological stoichiometric characteristics of marine invertebrates. To explore the ecological stoichiometric characteristics of marine invertebrates, the C, N, P, calcium (Ca), δ13C, and δ15N contents of 18 invertebrate species were analyzed from the Beibu Gulf. The results revealed that the ranges of elemental variations (C, N, and P) in invertebrates were 25.17%-47.34%, 6.14%-14.13%, and 0.26%-1.31%, respectively. The content of P in invertebrates exhibited the most significant variation, leading to alterations in C:P and N:P ratios. A significant negative correlation was observed between P content and body weight in invertebrates (p < 0.01). Furthermore, the C and N content of invertebrates were significantly negatively correlated with δ13C (p < 0.01), suggesting that the variations in C content and N content in invertebrates are influenced by different food sources, while P content varies according to body size. Our results also indicated significant interspecific differences in the ecological stoichiometry of invertebrates from the Beibu Gulf (p < 0.05). Invertebrate growth may be inhibited by P, and they do not maintain strict homeostasis, with stable homeostasis observed in higher trophic levels.
Introduction
Ecological stoichiometry offers a unifying conceptual framework that integrates various facets of aquatic biology, encompassing fish physiology, population ecology, community dynamics, ecosystem functions, and evolutionary processes (Bogotá-Gregory et al., 2020). This theory explores the balance of energy and diverse elements within ecological processes, proposing that the elemental composition of organisms reflects their chemical element requirements and the extent of elemental homeostasis (Sterner and Elser, 2002; Fernández-Martínez, 2022). All living organisms are composed of a series of fundamental chemical elements, especially carbon (C), nitrogen (N), phosphorus (P), among others (Sterner and Elser, 2002), and their ecological stoichiometric characteristics serve as crucial indicators for growth and development, life history changes, and biological characteristics of organisms (Peñuelas et al., 2019). However, the proportions of these elements in organisms exhibit significant variation, both across taxa and trophic groups (Elser et al., 2000; Peñuelas et al., 2019; May and El-Sabaawi, 2022).
The primary focus of current ecological stoichiometry research is on nutrient dynamics (Laspoumaderes et al., 2022), microbial nutrition (Wang Z. et al., 2022), host-pathogen relationships (Price et al., 2021), consumer-driven nutrient cycles (Leroux et al., 2020), biogeography of organic matter stoichiometry (Martiny et al., 2013), population dynamics and elementome diversity (Williamson and Ozersky, 2019; Fernández-Martínez, 2022), forest succession and recession (Bin et al., 2022), nutrient limitation (Hessen et al., 2004), food webs (Pacioglu et al., 2021), biological evolution (Jeyasingh et al., 2014), biological invasions (González et al., 2010; Williamson and Ozersky, 2019), and biogeochemical niches (Sardans et al., 2021). However, the ecological stoichiometry of marine invertebrates remains largely unexplored. Invertebrates, as the main consumers within marine ecosystems, play a pivotal role in the material cycle of ecosystems. The C:N:P contents of these consumers serve as an indicator of their respective elemental demand ratios. Any imbalance in these nutritional ratios between consumers and their prey can significantly affect the growth, reproduction, metabolism, and ultimately the ecological processes of the entire population (Sterner et al., 1992; Regnier et al., 2013).
Inter- and intraspecific differences in organismal stoichiometry have been confirmed across various animal taxa, offering significant insights into evolutionary patterns within this domain (Vanni et al., 2002). The ecological stoichiometry of fishery catches exhibits both intra- and interspecific variations, primarily influenced by ontogeny and environmental conditions such as food quality, nutritional status, resources, and habitat (Benstead et al., 2014; Sullam et al., 2015). Some organisms undergo substantial shifts in their elemental composition during development, specifically vertebrates. For instance, the formation of phosphorus-rich bones results in a significant increase in whole-body P content in species like fish, amphibians, and reptiles (Sterrett et al., 2015; Tiegs et al., 2016). Furthermore, the distribution and proportion of carbon-rich lipids, nitrogen-rich muscles, and carbon-rich invertebrate chitin shell materials also significantly affect the ecological stoichiometric characteristics of organisms during development (Pilati and Vanni, 2007; Boros et al., 2015). These changes are also affected by biological genetic characteristics, with different organisms exhibiting unique morphological structures. Additionally, interspecific differences in ecological stoichiometry may arise from variations in food sources (Naddafi et al., 2009; Sun et al., 2014). Food stoichiometry can affect nutrient acquisition and growth rates in consumers, as well as the rate and proportion of the release of nutrient waste into the environment (Elser and Urabe, 1999). For example, daphniids consuming phosphorus-deficient food will experience alterations in their growth rate and body P content (DeMott et al., 2004). Changes in the stoichiometric characteristics of C, N, and P are inherently linked to material circulation and energy flow, which regulate the relationship between the trophic levels within the food web by affecting the biodiversity and species abundance of the community, exerting a decisive influence on the structure and function of ecosystems (Sardans et al., 2012; Wang et al., 2018a). Conversely, the structure of food webs and nutrient cycling can also influence the stoichiometry of C, N, and P.
The Beibu Gulf (105°40′~110°10′ E, 17°00′~21°45′ N) is a semi-enclosed bay in the northern South China Sea, characterized by its tropical and subtropical climate. This unique geographical environment contributes to its high productivity and abundant fishery resources (Qiao and Li, 2007). However, significant declines have been observed in the primary high-quality fishery resources and their communities in the Beibu Gulf due to factors such as overfishing, environmental pollution, and global climate change (Lao et al., 2021; Xu et al., 2022). Current studies on fishery catches in the Beibu Gulf mainly focus on the biological characteristics of economic fish species (Li et al., 2009; Wang J. et al., 2022), biotoxicology (Koongolla et al., 2022; Lu and Wang, 2023), as well as fishery resources investigations and dynamic analysis (Hou et al., 2021). However, systematic research data on the ecological stoichiometry of species within marine ecosystems are conspicuously lacking.
The examination of the ecological stoichiometry of invertebrates is essential for understanding the cycling of C, N, and P nutrients within the increasingly impacted coastal marine ecosystem. This study aimed to explore the ecological stoichiometric characteristics of invertebrates and their influencing factors by analyzing the contents of C, N, P, calcium (Ca), δ13C, and δ15N in invertebrates from the Beibu Gulf. We hypothesized that variations in elemental stoichiometry among invertebrates could be attributed to changes in body size, genetic relationships, and available food sources. We further explored interspecific variations in the C:N:P contents of invertebrates and the drivers responsible for its homeostasis changes. The results will provide evidence to reveal the balance of C, N, and P elements among consumers, thereby guiding for biogeochemical cycle, conservation, and management of fishery resources in the Beibu Gulf.
Materials and methods
Sampling
In April and August 2022, a fishing vessel equipped with a 441-kilowatt main engine was deployed in the Beibu Gulf to collect marine invertebrates via bottom trawling (Figure 1). The trawl net was 20 meters in length, 8 meters in width, and 3 meters in height, with mesh sizes ranging between 1 and 5 centimeters. All samples were stored at -20 °C before being transported to the laboratory for further analysis.
Sample preparation and element determination
All invertebrates were thawed and weighed to the nearest ± 0.1 g, with total length measured using vernier calipers to an accuracy of ± 1 mm. The invertebrates underwent gutting and freeze-drying processes, followed by grinding into fine powder using a grinding mill and sieving through a 60-mesh sieve to ensure homogeneity. Smaller individuals from both shellfish and shrimp samples were combined into a single sample. A 2.5-4.0 mg dry sample was embedded in tin capsules and analyzed for C and N using an elemental analyzer (EA2400II). For the determination of δ13C and δ15N, 0.40-0.45 mg of each sample was weighed and embedded in tin capsules and burned at high temperature in an EA Isolink Elemental Analyzer (Thermo Fisher Scientific, Waltham, MA, USA) to produce CO2 and N2. The gas was analyzed by a 253 Plus Isotope Mass Spectrometer (Thermo Fisher Scientific, Waltham, MA, USA) for stable isotope analysis. P and Ca contents were determined by ICP-OES (Optima 7000DV). Prior to analysis, approximately 0.2 g of dried sample was digested in 7 mL of mixed acid (HNO3:H2O2 = 5:2) using microwave digestion. The mixture was then heated on a hot plate at 100 °C for 30 minutes to evaporate excess acid, diluted to 50 mL with ultrapure water, and subsequently analyzed by ICP-OES. The recovery rates of Ca and P were 91.67 ± 6.76% and 92.86 ± 6.37%, respectively. The results of C, N, P, and Ca contents are expressed as dry mass percentages (%), while the C:N, C:P, and N:P ratios are molar ratios of elements.
Statistical analyses
The basic statistical analysis of the C, N, and P contents and their ratios was initially carried out for all invertebrate samples. The normal distribution was tested by Kolmogorov-Smirnov test. Differences in the ecological stoichiometric characteristics among invertebrate taxa were analyzed by principal coordinate analysis (PCoA) and analysis of similarities (ANOSIM). One-way analysis of variance (ANOVA) was utilized to compare the C, N, and P contents and δ13C and δ15N values across different taxa. Pearson correlation analysis was conducted to determine the relationship between elemental contents and ratios, body weight, δ13C and δ15N. All statistical analyses were performed using SPSS 23.0, Excel 2016, and graphing was done with R (Version 4.0.3) and OriginPro 2021.
Results
Elemental composition of invertebrates
A total of 18 species of invertebrates (n = 130) were collected in this study, revealing significant variations in their elemental contents (Table 1). Specifically, the ranges for C, N, and P contents were 25.17%-47.34%, 6.14%-14.13%, and 0.26%-1.31%, respectively. The ratios of C:N, C:P, and N:P varied between 3.67-6.87, 55.72-348.08, and 4.67-42.72, respectively. Among these elements, the coefficient of variation (CV) was highest for P, followed by N and C. Notably, larger variations in P content corresponded to more significant changes in both C:P and N:P ratios, whereas the C:N ratio demonstrated relatively minor variations.
Relationship of ecological stoichiometry characteristics of invertebrates
Correlation analysis revealed that the C:N and N content, C:P and P content, and N:P and P content of invertebrates in the Beibu Gulf were all significantly negatively correlated. Conversely, there was a significant positive correlation between C:P and C content, N:P and C content, N:P and N content, N content and C content (p < 0.01) (Figure 2). Notably, the correlation between the C:P and P content, as well as the N:P and P content of invertebrates, was the strongest (r > 0.8) (Figure 2), indicating that the C:P and N:P ratios were mainly influenced by the change in P content. Furthermore, the correlation analysis indicated no significant correlation between the P content of the invertebrates and the Ca content (p > 0.05) (Figure 2).
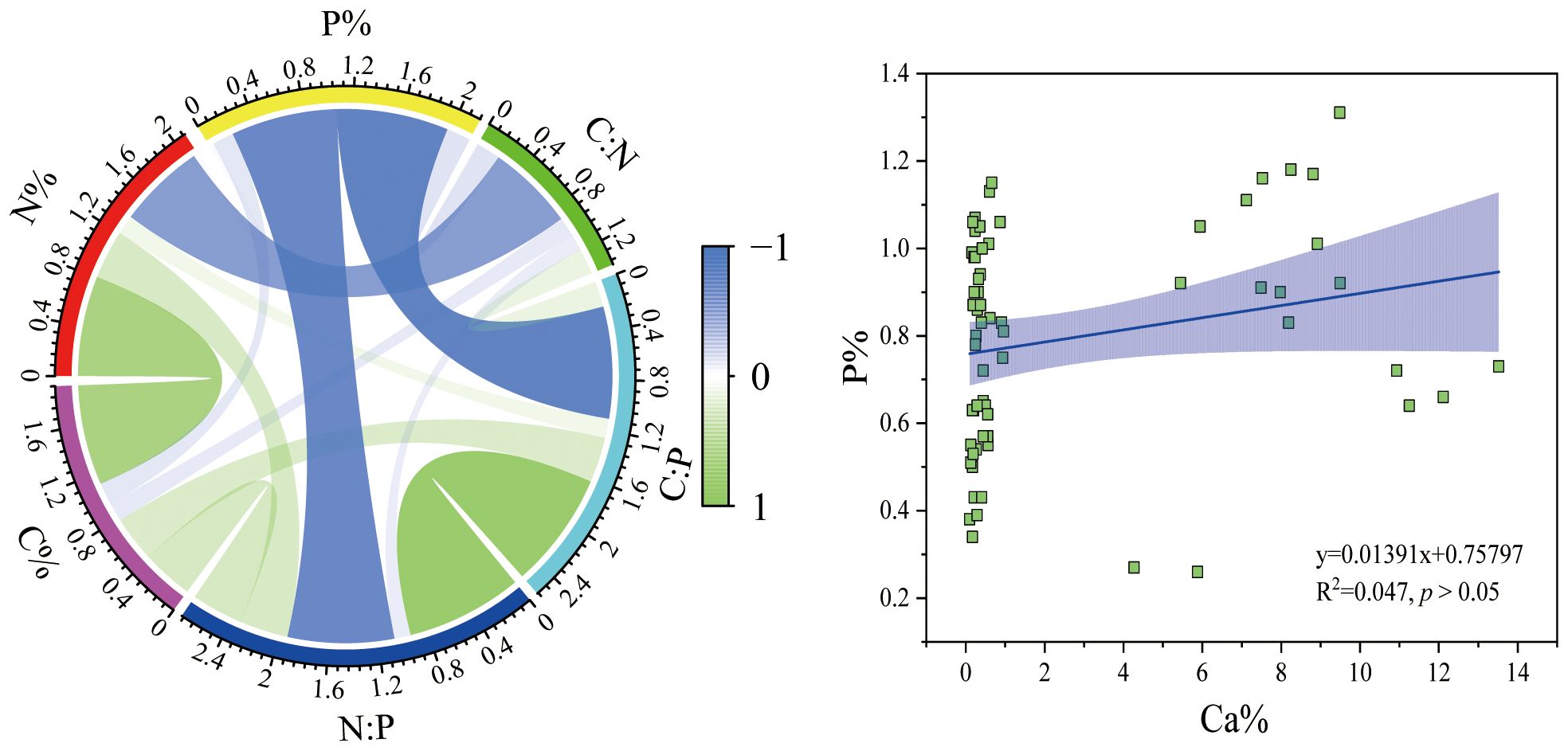
Figure 2 Analysis of the correlation relationships between elements in invertebrates from the Beibu Gulf.
Variations in the ecological stoichiometric characteristics of invertebrates
A total of 18 invertebrate species were categorized into 5 groups: cephalopods, crabs, sea cucumber, shellfish, and shrimps. The results from the ANOSIM showed that the ecological stoichiometric characteristics varied significantly among these different categories of invertebrates. Furthermore, it was observed that invertebrates from different taxa consisted of different elements (R = 0.535, p = 0.001) (Figure 3).
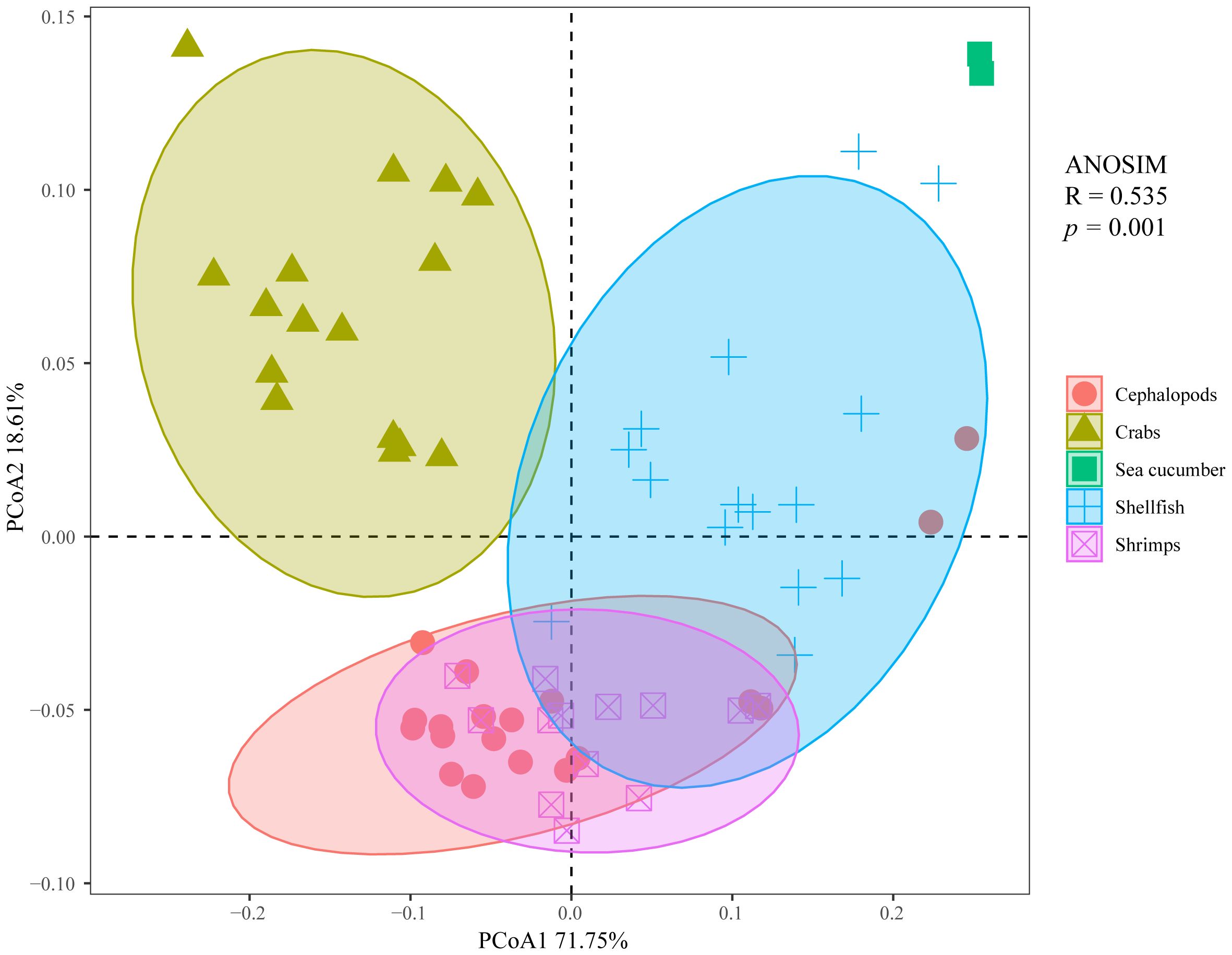
Figure 3 Principal coordinate analysis (PCoA)+analysis of similarities (ANOSIM) diagram of the contents and ratios of C, N, and P among different categories of invertebrates from the Beibu Gulf.
The one-way ANOVA results indicated significant difference in the C, N, and P content, as well as the C:N, C:P, and N:P ratios among different invertebrates (p < 0.05) (Figure 4). Cephalopods, shellfish, and shrimps exhibited significantly higher C content than sea cucumber and crabs, with crabs having the lowest C content. Shrimps had the highest N content, followed by cephalopods, shellfish, sea cucumber, and crabs, respectively. Cephalopods, crabs, and shrimps had significantly higher P content compared to shellfish and sea cucumber, with the least in sea cucumber. The C:N ratios of crabs, sea cucumber, and shellfish were significantly greater than those of shrimps. The C:P and N:P ratios were the highest in sea cucumber, followed by shellfish, cephalopods, shrimps, and crabs, respectively.
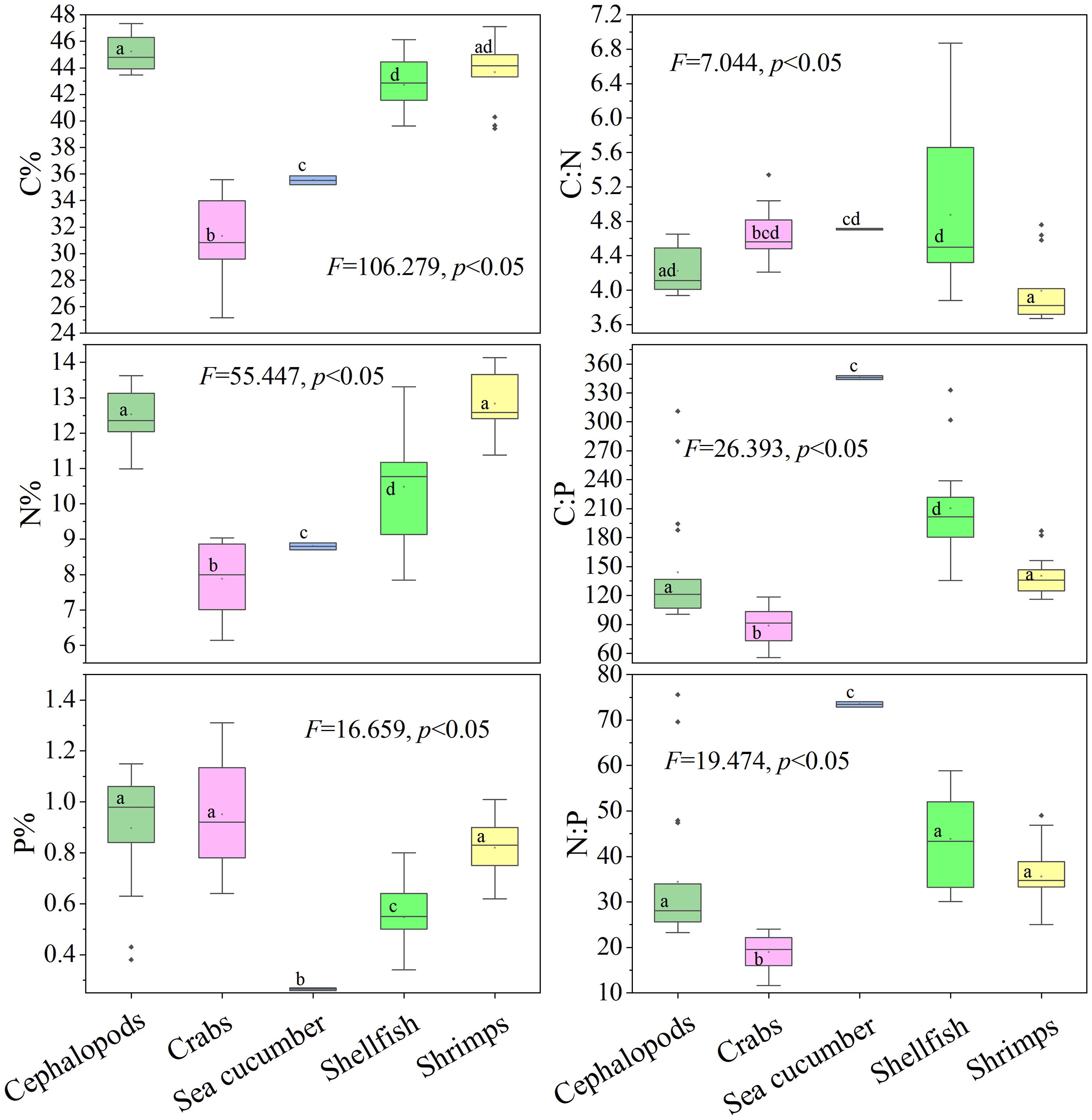
Figure 4 Box diagram of the contents and ratios of C, N, and P in different categories of invertebrates from the Beibu Gulf Significant differences are designated by different lower-case letters (p < 0.05).
The relationship between ecological stoichiometry characteristics of invertebrates and δ15N, δ13C and body weight
The δ15N values in the studied invertebrates ranged from 7.99‰ to 13.81‰, while the δ13C values ranged from -13.01‰ to -22.73‰. Significant differences were observed in the δ15N and δ13C values across different invertebrate taxa (p < 0.05) (Figure 5). Specifically, the δ15N values of cephalopods and shrimps were significantly higher than those of other invertebrates. Furthermore, the δ13C values of shellfish, shrimps, and cephalopods were significantly higher than those of crabs and sea cucumber.
The correlation analysis revealed significant negative correlations between the C and N content and δ13C of these invertebrates in the Beibu Gulf. Additionally, a significant negative correlation was observed between the P content of these invertebrates and their body weight. Conversely, the ratios of C:P and N:P demonstrated a significant positive correlation with body weight (p < 0.01) (Table 2).

Table 2 Analysis of the correlation between C, N, and P contents and their ratios with δ15N, δ13C, and body weight of invertebrates from the Beibu Gulf.
Discussion
Element composition and differences of different invertebrates
The results show P content of invertebrates in the Beibu Gulf changes the most, with coefficients of variation in the order of P > N > C, which is consistent with other species (Vanni et al., 2002; Sun et al., 2014). The growth rate hypothesis presumes that P is a primary determinant of population dynamics, and the variations in P content within organisms influence changes in the C:N:P ratios (Elser et al., 2003). In this study, the most notable change was observed in P content, resulting in substantial alternations in both C:P and N:P ratios. Hendrixson et al. (2007) proposed a strong correlation between P content and phylogeny, along with a broad spectrum of interspecific variation in P content. Phylogenetic imprinting is evident in the ecological stoichiometry of invertebrates, and patterns of nutrient composition across species introduce additional, potentially phylogenetically-based variations to these patterns (González et al., 2018; Allgeier et al., 2020). The concentrations of elements such as C, N, and P in the tissues of aquatic organisms were influenced by their environmental abundance and bioavailability, as well as the type, developmental stage, and physiological condition of the organism (Pinto et al., 2022). Analyzing elemental correlations can elucidate the interconnectedness of these elements. For example, negative correlations between elements may highlight elemental mass balance, where an increase in one element leads to a decrease in another (Allgeier et al., 2020). A significant positive correlation was observed between the N and C contents of invertebrates in this study. The muscles and shells of invertebrates are abundant in C and N elements, which suggests that C and N contents concurrently increase with the individual development of invertebrates, indicating a synergistic effect between these elements that promote growth and metabolism (Small and Pringle, 2010). However, there was no significant correlation between P content and Ca content in this study, indicating that the development of invertebrates’ bones did not influence P content. Invertebrates’ bones contain minimal amounts of P, and many invertebrates have a high proportion of chitin, a polysaccharide rich in nitrogen and carbon (Small and Pringle, 2010), which is primarily associated with their exoskeletons. Therefore, it is speculated that changes in P content of invertebrates are mainly related to the growth rate hypothesis trend and that P synthesis transporters are necessary to support organismal growth during development.
The availability of nutrients plays a pivotal role in determining organism growth, population structure, species interaction, and ecosystem stability (Allgeier et al., 2020). Therefore, identifying the type of nutrient that is insufficiently supplied becomes essential for maintaining system stability. Ecological stoichiometry defines the element with the least supply relative to demand as the “limiting” element (Sterner and Elser, 2002). The C:P and N:P ratios in invertebrates were high in this study, while the C:N ratio was low. Specifically, among the elements required by invertebrates, P content was relatively the smallest. Furthermore, a significant negative correlation was observed between the P content of invertebrates and body weight, indicating that P may constrain invertebrate growth. This is attributed to the insufficient P available to produce the RNA necessary for protein synthesis, resulting in developmental growth retardation (González et al., 2018).
The ecological stoichiometric characteristics of different invertebrates exhibited significant differences (Figure 3). Many invertebrates have a high proportion of chitin, which is a nitrogen- and carbon-rich polysaccharide that is mainly found in their exoskeletons (Small and Pringle, 2010). Therefore, organisms with larger surface area/volume ratios due to chitinous structures should have higher C and N contents (Allgeier et al., 2020). This is consistent with the findings of Allgeier et al. (2020) that shrimp had higher C and N contents. However, crabs have a larger surface area-to-volume ratio and a chitinous shell but had the lowest C and N contents in this study. Crabs have larger and thicker hard shells. Although they contain nitrogen- and carbon-rich chitin-which also contributes to their lower P content, the proportion of more carbon- and nitrogen-rich muscle is less, accounting for approximately half of their wet weight, resulting in a lower overall body C and N contents. In addition, the P content of sea cucumber was found to be lower than that in other species, with both C and N contents also relatively low, reflecting the findings of Allgeier et al. (2020). This may be attributed to the feeding habits of sea cucumbers, which are detritivores that feed on ocean sediments (Sales et al., 2023). Since the C, N, and P contents of the sediments are all low (Chen et al., 2021), sea cucumber absorbs fewer C, N and P contents.
Factors affecting ecological stoichiometry of invertebrates
Carbon and nitrogen stable isotopes (δ13C and δ15N) serve as effective tools for studying the nutrient cycle and energy flow within ecosystems (Wang et al., 2018b, 2020). In aquatic organisms, the δ13C value reflects the primary food sources of the consumer, with different δ13C values indicating different food sources, while the δ15N values can determine the trophic level of organisms (Liu et al., 2018; Wang et al., 2019, 2020). The C and N contents in invertebrates were found to be correlated with δ13C in this study, indicating that feeding habit significantly influences variations in C and N contents within these organisms. Given the highly variable availability of food resources in nature and the diverse range of foods consumed by marine organisms, their food stoichiometry also varies widely. Interspecific variations in ecological stoichiometry among invertebrates may be associated with variations in food sources (Cross et al., 2003; Lemoine et al., 2014). The water quality characteristics of the Beibu Gulf are complex and diverse, with an abundance of bait sources (Lao et al., 2021). Changes in the nutrient level of the water body can alter the composition of basic resources and bait, thereby directly or indirectly affecting the element content of consumers. Studies have shown that changes in the quality of basic resources limit the elemental content within higher trophic levels, with implications for not only primary consumers but across multiple trophic levels (Small and Pringle, 2010; Wang et al., 2021). For instance, the eutrophication of aquatic ecosystems can affect the stoichiometric properties of omnivorous fish (Mäkelin and Villnäs, 2022). The stoichiometry of omnivorous fish is influenced by the quantity and quality of phytoplankton, which are regulated by nutrients and light (Dickman et al., 2008). Therefore, changes in bioecological stoichiometry are more likely to reflect changes in environmental conditions that affect the availability of elements in consumer food or basic resources than modifications in biological traits (McIntyre and Flecker, 2010). The elemental composition of animals is, in fact, more flexible and less homeostatic than previously assumed. While certain species may exhibit homeostatic mechanisms for self-regulating stoichiometry, it is important to note that not all fishery catches exhibit identical elemental compositions. Instead, the contents of various elements can greatly differ among species.
The key life history and ecological traits of an organism, including its nutrient uptake, storage, and transfer mechanisms, are significantly affected by body size (Amundrud and Srivastava, 2016). A meta-analysis of all individual species based on body weight revealed a negative correlation between P content and body weight, which finding is consistent with the results of the study by González et al. (2018). The decrease in P content with increasing body size is due to the lower growth rate of large organisms compared to small organisms (Sterner and Elser, 2002; Wang et al., 2023). Both growth rate and RNA content decrease with age. The growth rate hypothesis presumes that organisms with higher growth rates have higher phosphorus contents due to the increased synthesis of phosphorus-rich rRNA required for their rapid protein synthesis (Elser et al., 2003). Furthermore, genetic relationships also play an important role in shaping the ecological stoichiometric characteristics of C, N, and P in invertebrates. Our analysis identified distinct clusters of closely related invertebrate taxa and clear separations between distantly related taxa (Figure 3), suggesting significant differences among different invertebrate groups. Variations in elemental composition and proportions among consumers are mainly associated with phylogenetic differences that influence factors such as body size, growth rate, and resource allocation to structural components within the body. Cross et al. (2005) suggested that phylogeny may be more important than local environmental conditions or species composition within higher taxonomic groups in determining the elemental composition of consumers at least at the class or higher level.
Homeostasis in organisms
A key challenge in ecological stoichiometry is to explore the capacity of living organisms to sustain homeostasis in elemental composition. The demand for chemical elements by organisms is largely determined by individual investments in structural resources, such as vertebrates’ investment in phosphorus-rich bones, arthropods’ investment in carbon- and nitrogen-rich chitin and muscles, and plants’ investment in carbon-rich cellulose and lignin (Leal et al., 2017). Additionally, physiological processes such as growth and reproduction also influence the elemental requirements of organisms (Leal et al., 2017). Variations in the steady-state levels of elements between different organisms, such as between plants and animals, can lead to significant differences in the organism’s stoichiometry (Sterner and Elser, 2002; Persson et al., 2010). Therefore, each species should maintain an optimal balance of biological elemental composition, with different taxa exhibiting different elements and increasing differences as taxonomic distance and evolutionary time increase (Peñuelas et al., 2019). Furthermore, species demonstrate a degree of flexibility and adaptability, altering their elemental stoichiometry in response to changes in the composition and/or environmental conditions of neighboring species (Sardans and Peñuelas, 2014). In light of this, the size of the area of the principal coordinate analysis (PCoA) confidence interval (i.e., the size of the variation in element composition) were applied to represent the degrees of homeostasis of species or taxa (Figure 3). A smaller PCoA area indicates a smaller change in the content and ratio of C, N, and P, indicating species with stricter homeostasis. On the contrary, a larger area on PCoA indicates a greater change in the content and ratio of C, N, and P, indicating species with less strict homeostasis. In addition, species or taxa with relatively larger PCoA areas had lower δ15N values, while those with smaller PCoA areas had higher δ15N values. A correlation between homeostasis and trophic level was observed in this study, with species or taxa occupying higher trophic levels maintaining stricter homeostasis. This finding aligns with the research results of Feng et al. (2023), which indicated that the stoichiometric stability of aquatic organisms increased with their trophic level.
Conclusion
Our research has indicated significant interspecific differences in the ecological stoichiometric traits of invertebrates within the Beibu Gulf. The growth of these invertebrates appears to be constrained by P, and they do not exhibit strict homeostasis, with higher trophic levels displaying greater stability. In future studies, we plan to integrate our results with recent data on marine biological resources in the Beibu Gulf, including total biomass, fishing amount, and natural mortality rates. We also plan to explore the total content and ratios of C, N, and P across various marine ecosystem sizes, as well as their origins and contributions. Furthermore, we intend to assess its impact on the succession of fishery catch communities, thereby enhancing the application of ecological stoichiometry in marine ecology research.
Data availability statement
The raw data supporting the conclusions of this article will be made available by the authors, without undue reservation.
Ethics statement
The animal study was approved by Guilin University of Technology, Guilin China. The study was conducted in accordance with the local legislation and institutional requirements.
Author contributions
CW: Conceptualization, Data curation, Formal Analysis, Investigation, Software, Visualization, Writing – original draft, Writing – review & editing. LH: Conceptualization, Data curation, Investigation, Methodology, Writing – original draft, Writing – review & editing. BK: Methodology, Writing – review & editing, Supervision. LZ: Investigation, Visualization, Writing – review & editing. HL: Investigation, Visualization, Writing – review & editing. SZ: Data curation, Visualization, Writing – review & editing. YC: Data curation, Visualization, Writing – review & editing. AS: Writing – review & editing, Validation. YY: Writing – review & editing, Investigation, Supervision.
Funding
The author(s) declare financial support was received for the research, authorship, and/or publication of this article. This study was financially supported by the National Natural Science Foundation of China (U20A2087), the Key Research and Development Program of Guangxi (Guike AB22035050).
Acknowledgments
We thank all the staff of Guibei Fishing Boat No. 98388 for their sampling assistance, and the reviewers for the revision of the manuscript.
Conflict of interest
The authors declare that the research was conducted in the absence of any commercial or financial relationships that could be construed as a potential conflict of interest.
Publisher’s note
All claims expressed in this article are solely those of the authors and do not necessarily represent those of their affiliated organizations, or those of the publisher, the editors and the reviewers. Any product that may be evaluated in this article, or claim that may be made by its manufacturer, is not guaranteed or endorsed by the publisher.
References
Allgeier J. E., Wenger S., Layman C. A. (2020). Taxonomic identity best explains variation in body nutrient stoichiometry in a diverse marine animal community. Sci. Rep. 10, 13718. doi: 10.1038/s41598-020-67881-y
Amundrud S. L., Srivastava D. S. (2016). Trophic interactions determine the effects of drought on an aquatic ecosystem. Ecology 97, 1475–1483. doi: 10.1890/15-1638.1
Benstead J. P., Hood J. M., Whelan N. V., Kendrick M. R., Nelson D., Hanninen A. F., et al. (2014). Coupling of dietary phosphorus and growth across diverse fish taxa: a meta-analysis of experimental aquaculture studies. Ecology 95, 2768–2777. doi: 10.1890/13-1859.1
Bin H., Li Q., Zhang P., Li W., Xue X., Zou S., et al. (2022). Effects of Elevation on Ecological Stoichiometry of Plant Leaves, Litter, and Soils in Pseudotsuga sinensis Forest in the Karst Mountain region, Southwest China. J. Soil Sci. Plant Nutr. 22, 3582–3597. doi: 10.1007/s42729-022-00911-y
Bogotá-Gregory J. D., Lima F. C. T., Correa S. B., Silva-Oliveira C., Jenkins D. J., Ribeiro F. R., et al. (2020). Biogeochemical water type influences community composition, species richness, and biomass in megadiverse Amazonian fish assemblages. Sci. Rep. 10, 15349. doi: 10.1038/s41598-020-72349-0
Boros G., Sály P., Vanni M. J. (2015). Ontogenetic variation in the body stoichiometry of two fish species. Oecologia 179, 329–341. doi: 10.1007/s00442-015-3349-8
Chen D., Ke Z., Tan Y. (2021). Distribution of C/N/P stoichiometry in suspended particulate matter and surface sediment in a bay under serious anthropogenic influence: Daya Bay, China. Environ. Sci. pollut. Res. 28, 29177–29187. doi: 10.1007/s11356-021-12812-1
Cross W. F., Benstead J. P., Frost P. C., Thomas S. A. (2005). Ecological stoichiometry in freshwater benthic systems: recent progress and perspectives. Freshw. Biol. 50, 1895–1912. doi: 10.1046/j.1461-0248.2003.00481.x
Cross W. F., Benstead J. P., Rosemond A. D., Wallace J. B. (2003). Consumer-resource stoichiometry in detritus-based streams. Ecol. Lett. 6, 721–732. doi: 10.1046/j.1461-0248.2003.00481.x
DeMott W. R., Pape B. J., Tessier A. J. (2004). Patterns and sources of variation in Daphnia phosphorus content in nature. Aquat. Ecol. 38, 433–440. doi: 10.1023/B:Aeco.0000035183.53389.66
Dickman E., Newell M. J. M., González M. J., Vanni M. J. (2008). Light, nutrients, and food-chain length constrain planktonic energy transfer efficiency across multiple trophic levels. Proc. Natl. Acad. Sci. United. States America 105, 18408–18412. doi: 10.1073/pnas.0805566105
Elser J. J., Acharya K., Kyle M., Cotner J., Makino W., Markow T., et al. (2003). Growth rate-stoichiometry couplings in diverse biota. Ecol. Lett. 6, 936–943. doi: 10.1046/j.1461-0248.2003.00518.x
Elser J. J., Fagan W. F., Denno R. F., Dobberfuhl D. R., Folarin A., Huberty A., et al. (2000). Nutritional constraints in terrestrial and freshwater food webs. Nature 408, 578–580. doi: 10.1038/35046058
Elser J. J., Urabe J. (1999). The stoichiometry of consumer-driven nutrient recycling: Theory, observations, and consequences. Ecology. 80, 735–751. doi: 10.1890/0012-9658(1999)080[0735:TSOCDN]2.0.CO;2
Feng M. J., Cheng H. W., Zhang P. Y., Wang K., Wang T., Zhang H., et al. (2023). Stoichiometric stability of aquatic organisms increases with trophic level under warming and eutrophication. Sci. Total. Environ. 856, 160106. doi: 10.1016/j.scitotenv.2022.160106
Fernández-Martínez M. (2022). From atoms to ecosystems: elementome diversity meets ecosystem functioning. New Phytol. 234, 35–42. doi: 10.1111/nph.17864
González A. L., Céréghino R., Dézerald O., Farjalla V. F., Leroy C., Richardson B. A., et al. (2018). Ecological mechanisms and phylogeny shape invertebrate stoichiometry: A test using detritus-based communities across Central and South America. Dryad. Digital. Repository. 32, 2448–2463. doi: 10.1111/1365-2435.13197
González A. L., Kominoski J. S., Danger M., Ishida S., Iwai N., Rubach A. (2010). Can ecological stoichiometry help explain patterns of biological invasions? Oikos 119, 779–790. doi: 10.1111/j.1600-0706.2009.18549.x
Hendrixson H. A., Sterner R. W., Kay A. D. (2007). Elemental stoichiometry of freshwater fishes in relation to phylogeny, allometry and ecology. J. Fish. Biol. 70, 121–140. doi: 10.1111/j.1095-8649.2006.01280.x
Hessen D. O., Ågren G. I., Anderson T. R., Elser J. J., de Ruiter P. C. (2004). Carbon sequestration in ecosystems: The role of stoichiometry. Ecology 85, 1179–1192. doi: 10.1890/02-0251
Hou G., Zhang H., Wang J., Chen Y., Lin J. (2021). Stock assessment of 19 perciformes in the beibu gulf, China, using a length-based bayesian biomass method. Front. Mar. Sci. 8. doi: 10.3389/fmars.2021.731837
Jeyasingh P. D., Cothran R. D., Tobler M. (2014). Testing the ecological consequences of evolutionary change using elements. Ecol. Evol. 4, 528–538. doi: 10.1002/ece3.950
Koongolla J. B., Lin L., Yang C. P., Pan Y. F., Li H. X., Liu S., et al. (2022). Microplastic prevalence in marine fish from onshore Beibu Gulf, South China Sea. Front. Mar. Sci. 9. doi: 10.3389/fmars.2022.964461
Lao Q., Liu G., Shen Y., Su Q., Lei X. (2021). Biogeochemical processes and eutrophication status of nutrients in the northern Beibu Gulf, South China. J. Earth Syst. Sci. 130, 199. doi: 10.1007/s12040-021-01706-y
Laspoumaderes C., Meunier C. L., Magnin A., Berlinghof J., Elser J. J., Balseiro E., et al. (2022). A common temperature dependence of nutritional demands in ectotherms. Ecol. Lett. 25, 2189–2202. doi: 10.1111/ele.14093
Leal M. C., Seehausen O., Matthews B. (2017). The ecology and evolution of stoichiometric phenotypes. Trends Ecol. Evol. 32, 108–117. doi: 10.1016/j.tree.2016.11.006
Lemoine N. P., Giery S. T., Burkepile D. E. (2014). Differing nutritional constraints of consumers across ecosystems. Oecologia 174, 1367–1376. doi: 10.1007/s00442-013-2860-z
Leroux S. J., Wiersma Y. F., Vander E. W. (2020). Herbivore impacts on carbon cycling in boreal forests. Trends Ecol. Evol. 35, 1001–1010. doi: 10.1016/j.tree.2020.07.009
Li Z., Lu H., Gan X., Jin X. (2009). Growth and mortality of bottom threadfin bream nemipterus bathybius in the mouth of beibu gulf,South China sea. Fisheries. Sci. 28, 556–562. doi: 10.16378/j.cnki.1003-1111.2009.10.004
Liu Y., Liu G., Yuan Z., Liu H., Lam P. K. S. (2018). Heavy metals (As, Hg and V) and stable isotope ratios (δ13C and δ15N) in fish from Yellow River Estuary, China. Sci. Total. Environ. 613-614, 462–471. doi: 10.1016/j.scitotenv.2017.09.088
Lu G. Y., Wang W. X. (2023). Tissue-based trace element pollution of clam Ruditapes philippinarum in China: Hotspot identification and multiple nonlinear analysis. Sci. Total. Environ. 868, 161598. doi: 10.1016/j.scitotenv.2023.161598
Mäkelin S., Villnäs A. (2022). Food sources drive temporal variation in elemental stoichiometry of benthic consumers. Limnol. Oceanogr. 67, 784–799. doi: 10.1002/lno.12034
Martiny A. C., Pham C. T. A., Primeau F. W., Vrugt J. A., Moore J. K., Levin S. A., et al. (2013). Strong latitudinal patterns in the elemental ratios of marine plankton and organic matter. Nat. Geosci. 6, 279–283. doi: 10.1038/ngeo1757
May E. M., El-Sabaawi R. W. (2022). Life stage and taxonomy the most important factors determining vertebrate stoichiometry: A meta-analysis. Ecol. Evol. 12, e9354. doi: 10.1002/ece3.9354
McIntyre P. B., Flecker A. S. (2010). Ecological stoichiometry as an integrative framework in stream fish ecology. Am. Fisheries. Soc. Symposium. 73, 539–558.
Naddafi R., Eklov P., Pettersson K. (2009). Stoichiometric constraints do not limit successful invaders: zebra mussels in swedish lakes. PloS One 4, e5345. doi: 10.1371/journal.pone.0005345
Pacioglu O., Amărioarei A., Duţu L. T., Plăvan G., Iţcuş C., Plăvan O., et al. (2021). The structure and functionality of communities and food webs in streams along the epigean–hypogean continuum: unifying ecological stoichiometry and metabolic theory of ecology. Aquat. Sci. 83, 63. doi: 10.1007/s00027-021-00815-6
Peñuelas J., Fernández-Martínez M., Ciais P., Jou D., Piao S., Obersteiner M. (2019). The bioelements, the elementome, and the biogeochemical niche. Ecology 100, e02652. doi: 10.1002/ecy.2652
Persson J., Fink P., Goto A., Hood J. M., Jonas J., Kato S. (2010). To be or not to be what you eat: regulation of stoichiometric homeostasis among autotrophs and heterotrophs. Oikos 119, 741–751. doi: 10.1111/j.1600-0706.2010.18545.x
Pilati A., Vanni M. J. (2007). Ontogeny, diet shifts, and nutrient stoichiometry in fish. Oikos 116, 1663–1674. doi: 10.1111/j.2007.0030-1299.15970.x
Pinto F. R., Duarte A. M., Silva F., Barroso S., Mendes S., Pinto E., et al. (2022). Annual, variations in the mineral element content of five fish species from the Portuguese coast. Food Res. Int. 158, 111482. doi: 10.1016/j.foodres.2022.111482
Price T. L., Harper J., Francoeur S. N., Halvorson H. M., Kuehn K. A. (2021). Brown meets green: light and nutrients alter detritivore assimilation of microbial nutrients from leaf litter. Ecology 102, e03358. doi: 10.1002/ecy.3358
Qiao Y., Li Z. (2007). The relationship between the main features of land form, the distritubton of bottom sediment and fishery distribution. Trans. Oceanol. Limnol. S1), 232–238. doi: 10.13984/j.cnki.cn37-1141.2007.s1.030
Regnier P., Friedlingstein P., Ciais P., Mackenzie F. T., Gruber N., Janssens I. A., et al. (2013). Anthropogenic perturbation of the carbon fluxes from land to ocean. Nat. Geosci. 6, 597–607. doi: 10.1038/ngeo1830
Sales S. S., Lourenço H. M., Bandarra N. M., Cardoso C., Brito P., Botelho M. J., et al. (2023). Elemental composition and in vitro bioaccessibility assessment of holothuroids. J. Food Composition. Anal. 115, 104986. doi: 10.1016/j.jfca.2022.104986
Sardans J., Janssens I. A., Ciais P., Obersteiner M., Peñuelas J. (2021). Recent advances and future research in ecological stoichiometry. Perspect. Plant Ecol. Evol. Syst. 50, 125611. doi: 10.1016/j.ppees.2021.125611
Sardans J., Peñuelas J. (2014). Climate and taxonomy underlie different elemental concentrations and stoichiometries of forest species: the optimum “biogeochemical niche. Plant Ecol. 215, 441–455. doi: 10.1007/s11258-014-0314-2
Sardans J., Rivas-Ubach A., Peñuelas J. (2012). The elemental stoichiometry of aquatic and terrestrial ecosystems and its relationships with organismic lifestyle and ecosystem structure and function: a review and perspectives. Biogeochemistry 111, 1–39. doi: 10.1007/s10533-011-9640-9
Small G. E., Pringle C. M. (2010). Deviation from strict homeostasis across multiple trophic levels in an invertebrate consumer assemblage exposed to high chronic phosphorus enrichment in a Neotropical stream. Oecologia 162, 581–590. doi: 10.1007/s00442-009-1489-4
Sterner R. W., Elser J. J. (2002). Ecological stoichiometry: the biology of elements from molecules to the biosphere (Press, Princeton, NJ: Princeton Univ).
Sterner R. W., Elser J. J., Hessen D. O. (1992). Stoichiometric relationships among producers, consumers and nutrient cycling in pelagic ecosystems. Biogeochemistry 17, 49–67. doi: 10.1007/BF00002759
Sterrett S. C., Maerz J. C., Katz R. A. (2015). What can turtles teach us about the theory of ecological stoichiometry? Freshw. Biol. 60, 443–455. doi: 10.1111/fwb.12516
Sullam K. E., Dalton C. M., Russell J. A., Kilham S. S., El-Sabaawi R., German D. P., et al. (2015). Changes in digestive traits and body nutritional composition accommodate a trophic niche shift in Trinidadian guppies. Oecologia 177, 245–257. doi: 10.1007/s00442-014-3158-5
Sun P., Li X., Gong X., Liu Y., Zhang X., Wang L. (2014). Carbon, nitrogen and phosphorus ecological stoichiometry of Lateolabrax macultus and Acanthogobius ommaturus in the Estuary of Yangtze River, China. Acta Ecol. Sin. 34, 196–203. doi: 10.1016/j.chnaes.2013.06.009
Tiegs S. D., Berven K. A., Carmack D. J., Capps K. A. (2016). Stoichiometric implications of a biphasic life cycle. Oecologia 180, 853–863. doi: 10.1007/s00442-015-3504-2
Vanni M. J., Flecker A. S., Hood J. M., Headworth J. L. (2002). Stoichiometry of nutrient recycling by vertebrates in a tropical stream: linking species identity and ecosystem processes. Ecol. Lett. 5, 285–293. doi: 10.1046/j.1461-0248.2002.00314.x
Wang Z., Cébron A., Baillard V., Danger M. (2022). Nitrogen to phosphorus ratio shapes the bacterial communities involved in cellulose decomposition and copper contamination alters their stoichiometric demands. FEMS Microbiol. Ecol. 98, fiac107. doi: 10.1093/femsec/fiac107
Wang J., Luo K., Fan Y., Deng Y., He X., Yan Y. (2022). Feeding ecology of Nemipterus japonicus in Beibu Gulf. Prog. Fishery. Sci. 44 (1), 47–57. doi: 10.19663/j.issn2095-9869.20210606001
Wang S., Luo B. K., Qin Y. J., Su L. H., Stewart S. D., Wang T. T., et al. (2020b). Consumer-diet discrimination of δ13C and δ15N: Source- and feeding-oriented patterns based on gut content analysis in a large subtropical river of China. River. Res. Appl. 36, 1124–1136. doi: 10.1002/rra.3644
Wang S., Luo B. K., Qin Y. J., Zhao J. G., Wang T. T., Stewart S. D., et al. (2020a). Fish isotopic niches associated with environmental indicators and human disturbance along a disturbed large subtropical river in China. Sci. Total. Environ. 750, 141667. doi: 10.1016/j.scitotenv.2020.141667
Wang S., Tang J. P., Su L. H., Fan J. J., Chang H. Y., Wang T. T., et al. (2019). Fish feeding groups, food selectivity, and diet shifts associated with environmental factors and prey availability along a large subtropical river, China. Aquat. Sci. 81, 31. doi: 10.1007/s00027-019-0628-1
Wang S., Wang L., Chang H. Y., Li F., Tang J. P., Zhou X. A., et al. (2018b). Longitudinal variation in energy flow networks along a large subtropical river, China. Ecol. Model. 387, 83–95. doi: 10.1016/j.ecolmodel.2018.08.019
Wang S., Wang T. T., Tang J. P., Wang L., Yang Y., Lin H. J., et al. (2018a). Longitudinal variation in fish prey utilization, trophic guilds, and indicator species along a large subtropical river, China. Ecol. Evol. 8, 11467–11483. doi: 10.1002/ece3.4577
Wang S., Wang T. T., Xia W. T., Chen Z. B., Stewart S. D., Yang F. J., et al. (2021). Longitudinal pattern of resource utilization by aquatic consumers along a disturbed subtropical urban river: Estimating the relative contribution of resources with stable isotope analysis. Ecol. Evol. 11, 16763–16775. doi: 10.1002/ece3.8304
Wang C. G., Zhu L., Huang L. L., Xu H., Xu P., He X. B., et al. (2023). Ecological stoichiometric characteristics of three dominant fish species from the Beibu Gulf: inter- and intraspecific variations. Front. Ecol. Evol. 11. doi: 10.3389/fevo.2023.1176052
Williamson F., Ozersky T. (2019). Lake characteristics, population properties and invasion history determine impact of invasive bivalves on lake nutrient dynamics. Ecosystems 22, 1721–1735. doi: 10.1007/s10021-019-00371-z
Keywords: ecological stoichiometry, stable isotope, homeostatic, marine invertebrates, Beibu Gulf
Citation: Wang C, Huang L, Kang B, Zhu L, Liu H, Zhao S, Cheng Y, Shahab A and Yan Y (2024) Interspecific differences in ecological stoichiometric characteristics of invertebrates and their influencing factors from the Beibu Gulf, China. Front. Mar. Sci. 11:1433305. doi: 10.3389/fmars.2024.1433305
Received: 15 May 2024; Accepted: 24 June 2024;
Published: 08 July 2024.
Edited by:
Junfu Dong, Shandong University, ChinaReviewed by:
Sai Wang, Hainan University, ChinaHu He, Chinese Academy of Sciences (CAS), China
Naicheng Wu, Ningbo University, China
Copyright © 2024 Wang, Huang, Kang, Zhu, Liu, Zhao, Cheng, Shahab and Yan. This is an open-access article distributed under the terms of the Creative Commons Attribution License (CC BY). The use, distribution or reproduction in other forums is permitted, provided the original author(s) and the copyright owner(s) are credited and that the original publication in this journal is cited, in accordance with accepted academic practice. No use, distribution or reproduction is permitted which does not comply with these terms.
*Correspondence: Liangliang Huang, llhuang@glut.edu.cn