- 1State Key Laboratory for Managing Biotic and Chemical Threats to the Quality and Safety of Agro-products, Ningbo University, Ningbo, China
- 2Laboratory of Biochemistry and Molecular Biology, School of Marine Sciences, Ningbo University, Ningbo, China
- 3Key Laboratory of Applied Marine Biotechnology of Ministry of Education, Ningbo University, Ningbo, China
- 4Nanjing Silphium Biotechnology Company Limited, Nanjing, China
The present study was conducted to evaluate the effect of dietary Silphium perfoliatum L. on growth performance, immunity, intestinal health, and resistance against Nocardia seriolae of largemouth bass. Four dietary concentrations of S. perfoliatum L. were prepared: 0% (SPL0), 1% (SPL1), 2.5% (SPL2.5), and 5% (SPL5). After 60 d feeding, a challenge test was conducted by injecting N. seriolae intraperitoneally. The results showed that supplementing the diets with S. perfoliatum L. could promote the weight gain rate, specific growth rate, and feed intake. Meantime, S. perfoliatum L. could also enhance the activity of immune-related enzymes and improve the expression of immune-related genes in the liver. Compared to the control group, S. perfoliatum L. improved intestinal morphology and reduced the abundance of harmful bacteria in intestinal microbiota. Importantly, supplementing the diets with S. perfoliatum L. protected largemouth bass from N. seriolae infection, resulting in a relative percent survival (RPS) of 24% (SPL1), 31.99% (SPL2.5), and 43.99% (SPL5). In conclusion, the present study showed that S. perfoliatum L. could boost growth performance, enhance immunity, promote intestinal health, and improve the ability of largemouth bass against N. seriolae infection.
1 Introduction
Largemouth bass (Micropterus salmoides) is a freshwater carnivorous teleost species, which is one of the main aquaculture species in China (Habte-Tsion et al., 2020). The production of largemouth bass reached about 0.8 million tons in 2022 (Hu et al., 2023). In recent years, the disease of largemouth bass has become increasingly serious, which brings huge economic losses to farmers (Hoang et al., 2020; Lu et al., 2023).
Nocardia seriolae is a pathogeny of fish nocardiosis, causing substantial morbidity in a wide range of fish species (Wang et al., 2017). It was first discovered in cultured yellowtail (Seriola quinqueradiata) in Japan (Kariya et al., 1968) and is known to cause substantial morbidity across a wide range of fish species, including Japanese sea bass (Lateolabrax japonicus) (Chen et al., 2000) and orange-spotted grouper (Epinephelus coioides) (Nguyen et al., 2023). The clinical signs of nocardiosis include white nodules in viscera, skin hyperemia, focal dermal ulcers. Nocardiosis has a long course of the disease, and the disease is asymptomatic or insignificant in initial stage, with a high morbidity and mortality, affecting the economic viability of aquaculture (Nawaz et al., 2022). There is an urgent need for safe and efficient strategies to control disease caused by N. seriolae.
Natural plants are rich in active ingredients, including alkaloids, flavonoids, pigments, phenols, terpenoids, steroids, and essential oils (Citarasu, 2009). Adding plant additives to fish feed has been proven to have a positive effect on fish farming, such as promoting growth, stimulating appetite, stimulating immunity, regulating intestinal microbiota, and antibacterial properties (Talpur, 2014; Du et al., 2023). For instance, Artemisia annua extract was used as a feed additive to improve health and promote the growth performance of fish (He et al., 2022). 2% fermented tea residue addition enhances the resistance to bacteria, growth, and antioxidant capacity of largemouth bass (Jiang et al., 2022). Dietary supplementation of astaxanthin improved growth performance, antioxidant capacity, and immunity of largemouth bass (Xie et al., 2020). Dietary banana peel promotes the growth performance and enhances the immunity of Labeo rohita (Giri et al., 2016). Dietary nutmeg enhances the antioxidant and immune response of carp and also protects carp from the attack of Aeromonas hydrophila (Rashidian et al., 2022). Therefore, supplementing natural plant feed additives to the feed might be beneficial for largemouth bass.
Silphium perfoliatum L. is native to North America and has been through numerous generations of cultivation and development. It has a wide range of adaptations, great resilience to adversity, high grass yield, and high nutrition (Peni et al., 2022). S. perfoliatum L. is rich in protein, amino acids, flavonoids, phenols, polysaccharides, carotene, vitamin C, vitamin E, and superoxide dismutase (Guo et al., 2020). Due to its high crude protein content, which can reach 10% to 18% of dry weight, S. perfoliatum L. is recommended as a potential source of livestock feed (Gansberger et al., 2015). In aquaculture, adding S. perfoliatum L. to the feed improves the antioxidant capacity of juvenile Megalobrama amblycephala (Hu et al., 2024). In addition, plants can also be used to directly inhibit bacteria. For example, the leaf and root extract of S. perfoliatum L. exhibits properties against both Gram-positive bacteria (Enterococcus faecalis, Staphylococcus aureus) and Gram-negative bacteria (Escherichia coli, Pseudomonas aeruginosa) (Kowalski and Kedzia, 2007). Therefore, S. perfoliatum L. as an ideal product to reduce and replace antibiotics is very suitable for plant feed additives.
This study aims to evaluate the effects of S. perfoliatum L. as a plant feed additive on the immunity, intestinal health, and disease resistance of largemouth bass. After adding 1%, 2.5%, and 5% S. perfoliatum L. to the basic feed of largemouth bass for 60 days, we investigated the immune-related enzyme activity, immune-related gene expression, intestinal morphology, intestinal microbiota, and resistance to N. seriolae infection in largemouth bass.
2 Materials and methods
2.1 Experimental diets and fish experiment
The basic feed was purchased from Wuxi Tongwei Biotechnology (Jiangsu, China), which is consists of fish meal, flour, soybean oil, soybean meal, minerals, trace elements, and vitamins. Different proportions of S. perfoliatum L. (0, 1%, 2.5%, and 5%) were respectively added to the basic feed, named SPL0, SPL1, SPL2.5, and SPL5.
Experimental fishes were purchased from a largemouth bass farm (Huzhou, Zhejiang, China). A total of 720 healthy and uniform-sized fish (20.09 ± 0.87 g) were distributed into 12 tanks (300 L) with 60 fish per tank (three tanks per treatment). Feed twice a day with apparent satiety (8:30 and 17:30), and the next feeding amount was adjusted according to the feeding and growth of the fish. Change 1/5-2/5 of the water every 2-3 days, the bottom feces were removed by using the pump. Water condition: temperature 23 ± 2°C, ammonia nitrogen ≤ 0.02 mg/L, dissolved oxygen content ≥ 6.0 mg/L, pH 7.0 ± 0.2. The experimental procedure is shown in Figure 1A.
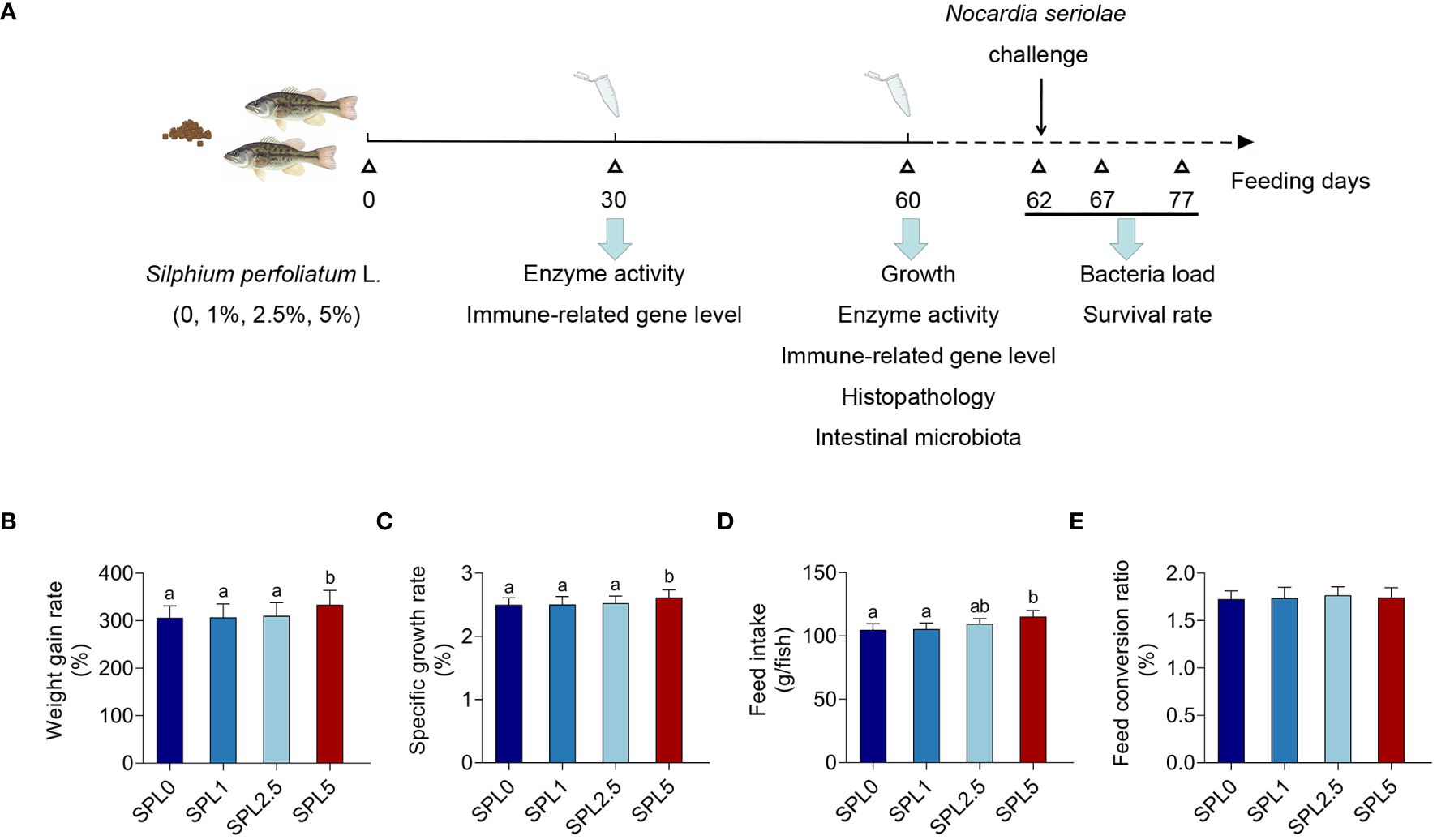
Figure 1 Effects of S. perfoliatum L. diet on growth performance of largemouth bass. (A) Schematic illustration of the experiment. (B) The weight gain rate (WGR), (C) specific growth rate (SGR), (D) feed intake (FI) and (E) feed conversion rate (FCR). Bars with different letters were significantly different according to the Duncan’s multiple range, n = 3, P< 0.05.
All animal experiments were conducted in accordance with the Experimental Animal Management Law of China and approved by the Animal Ethics Committee of Ningbo University.
2.2 Sampling
Samples were collected on days 30 and 60 respectively. Before sacrificed, fish was anesthetized by tricaine methanesulfonate (MS-222) in 0.1 g/kg. The liver was separated and immediately frozen in liquid nitrogen and stored at -80°C before use.
Select 9 fish from each of the SPL0 and SPL5 groups. After anesthetized by MS-222, cut open the intestines and remove the contents. Mix the contents of every 3 fish into a sample and store it in a sterile centrifuge tube at -80°C for subsequent sequencing.
2.3 Growth performance
Growth performance was assessed under different conditions using standard formulas to calculate weight gain rate, specific growth rate, feed intake, and feed conversion ratio (Xu et al., 2021). The growth performance was calculated as follows:
2.4 Enzyme activity
The activity of lysozyme (LZM), catalase (CAT), superoxide dismutase (SOD), and glutathione s-transferase (GST) were measured in the previous study (Lu et al., 2018). Briefly, tissues were homogenized with saline, and the homogenate was separated by centrifuge at 4°C for 20 min. Subsequently, the enzyme activities were measured using a spectrophotometer or microplate reader using commercial kits (Jiancheng, Nanjing, China).
2.5 RNA extraction and cDNA synthesis
Total tissue RNA was extracted using TRIzol reagent (Takara, Dalian, China). The quality and concentration of total RNA were detected by Nanodrop. Subsequently, RNA was reverse transcribed to cDNA using PrimeScript™ II 1st Strand cDNA Synthesis Kit (Takara) according to the manufacturer’s instructions.
2.6 Real-Time quantitative PCR
The expression of immune-related genes was assessed, including tumor necrosis factor-α (TNF-α), interleukin-1β (IL-1β), interleukin-10 (IL-10), transforming growth factor-β (TGF-β), kelch-like ech-associated protein 1 (Keap1), nuclear factor erythroid 2-related factor 2 (Nrf2), complement 3 (C3), and c-type lysozyme (LZM-c). 18s rRNA served as the internal control. The cDNA was used as a template for RT-qPCR analysis with TB Green Premix Ex Taq™ (Takara) on ABI QuantStudio 3 System (ABI, Foster City, USA), following a protocol of 95°C for 30 s, 40 cycles at 95°C for 5 s, and 60°C for 30 s, followed by 95°C for 15 s, 60°C for 60 s, 95°C for 15 s, and 50°C for 30 s. The 2−∆∆CT method was used to calculate the relative expression levels of the different genes. All primers were shown in Table 1.
2.7 Histological
Hindgut tissues of largemouth bass were fixed at room temperature with 4% paraformaldehyde for 48 h. Next, the fixed hindgut tissues were dehydrated within the series concentration of ethanol and embedded with paraffin. The embedded hindgut tissues were cut transversely into 5 μm and dyed with eosin and hematoxylin. Finally, intestinal sections were observed by optical microscopy (Nikon, Japan).
2.8 Intestinal microbiota analysis
DNA of bacteria in intestinal contents was separated by DNeasy PowerSoil kit (Qiagen, Hilden, Germany). PCR amplification of the V3-V4 hypervariable regions of the bacterial 16S rRNA gene was carried out by universal primer pairs (343F: 5′-TACGGRAGGCAGCAG-3′; 798R: 5′-AGGGTATCTAATCCT-3′). The PCR products were purified with Agencourt AMPure XP beads and quantified using a Qubit dsDNA assay kit (Beckman, Miami, USA). Sequencing was performed with Illumina NovaSeq6000. Then, cluster the high-quality sequences obtained by sequencing into operational classification units (OTUs). Using the alpha diversity, which incorporates the Chao1 index, Ace index, Simpson index, and Shannon index, the microbial diversity of intestinal contents was calculated. Phylogenetic tree construction and unweighted Unifrac Principal coordinates analysis (PCoA) were both done using the QIIME software’s Unifrac distance matrix.
2.9 Challenge test
N. seriolae was kindly provided by Xiaoli Huang, College of Veterinary Medicine, Sichuan Agricultural University, Wenjiang, Sichuan, China. The culture conditions of N. seriolae have been described in previous studies (Lei et al., 2020).
After 60 days of feeding, 40 fish per tank were used for challenge test. Fish were anesthetized by MS-222 in 0.1 g/kg, and 0.2 mL N. seriolae was intraperitoneally injected with a concentration of 1 × 108 CFU/mL. The mortality was monitored in the next 15 days. The RPS was calculated as RPS = (mortality of control group% - mortality of immunized group%)/mortality of control group%.
2.10 Bacterial quantification analysis
Bacteria were counted as previously described (Lu et al., 2018). Briefly, bacterial DNA in tissues was extracted by using a genomic DNA extraction Kit (Tiangen, Beijing, China). The bacteria load was determined by qPCR using the standard curve method. Primers used for bacterial quantification were listed in Table 1.
2.11 Calculations and statistical analysis
All data are presented as the mean ± S.E.M. (standard error of the mean) and analyzed by SPSS 23.0. Differences among groups were evaluated by two-way ANOVA analysis followed by Tukey’s multiple comparisons. The intestinal bacterial diversity and OTU richness were analyzed by the Wilcoxon-Mann-Whitney test.
3 Results
3.1 Supplementation of S. perfoliatum L. promoted the growth performance
WGR, SGR, FI, and FCR were evaluated post 60 days of feeding. As shown in Figures 1B-E, WGR, SGR, and FI showed no significant difference in the SPL1 and SPL2.5 groups compared with the SPL0 group. However, WGR, SGR, and FI increased in group SPL5, with increases of 8.97% in WGR, 4.4% in SGR, and 7.27% in FI. In addition, no differences were found in FCR among the groups.
3.2 Supplementation of S. perfoliatum L. enhanced the activity of LZM, SOD, CAT, and GST
To investigate the effects of S. perfoliatum L. on the enzyme activity, LZM, SOD, CAT, and GST in liver were monitored. As shown in Figures 2A–D, the activity of four enzymes in SPL1, SPL2.5, and SPL5 was significantly increased compared with SPL0, and this promotion was concentration- and time-dependent. The enzyme activity in 60 days was higher than that of 30 days. Meanwhile, the higher concentration of S. perfoliatum L., the higher activity of enzyme activity.
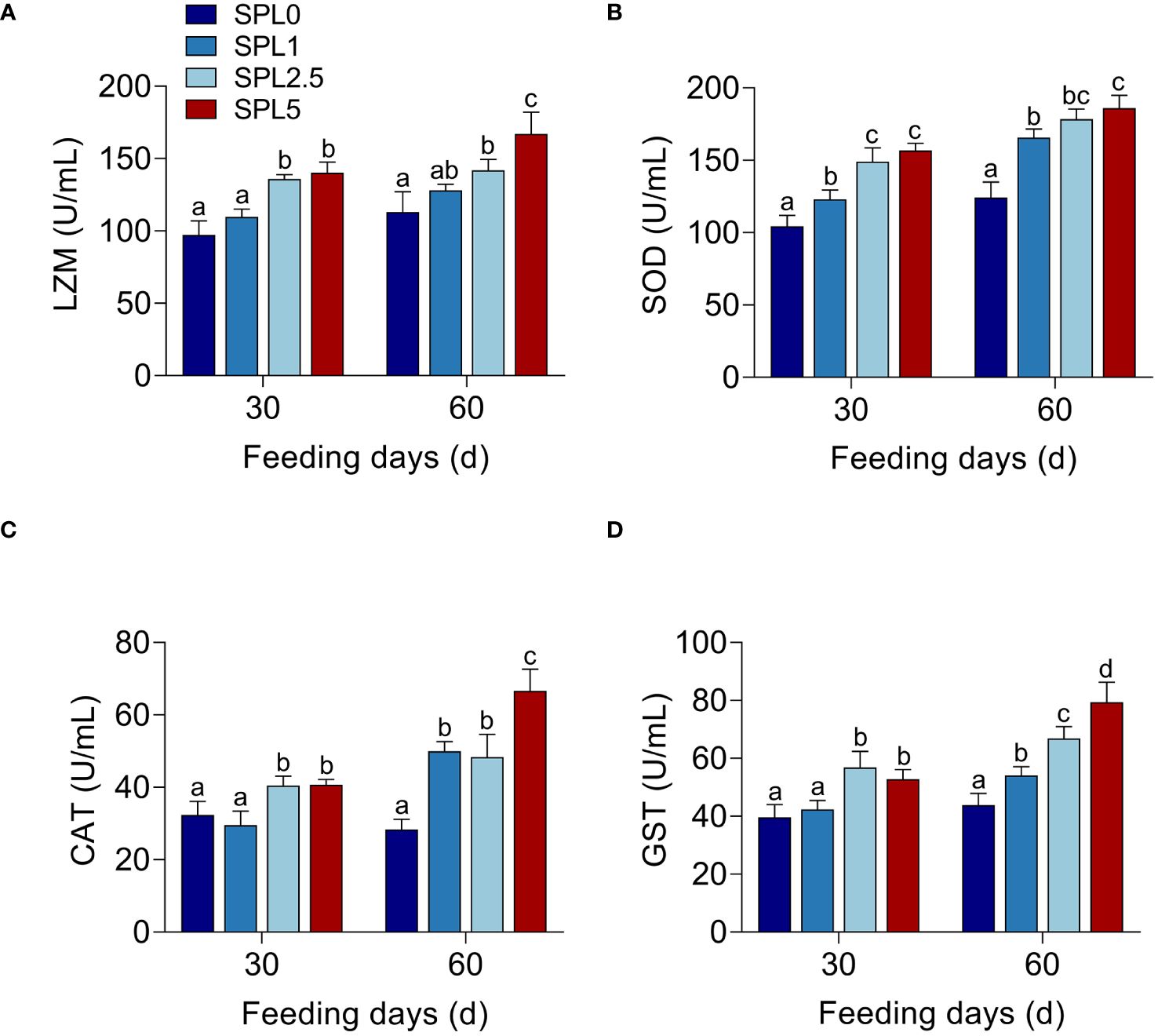
Figure 2 Enzyme activity of LZM (A), SOD (B), CAT (C), and GST (D) in the liver. Bars with different letters were significantly different according to the Duncan’s multiple range, n = 3, P< 0.05.
3.3 Effects of S. perfoliatum L. diet on the expression of the immune-relate genes
TGF-β, IL-10, TNF-α, and IL-1β are inflammatory cytokines (Lu et al., 2021a). Nrf2 and Keap1 have potential regulatory effects on the transcription of antioxidant-related genes (Chen et al., 2022). C3 and LZM-c are components of innate immunity to defend against bacteria (Gomez et al., 2013). Therefore, the mRNA expression of IL-10, TGF-β, TNF-α, IL-1β, C3, LZM-c, Nrf2, and keap1 was used to evaluate the effects of S. perfoliatum L. diet on innate immunity. All the qPCR products were determined in Supplementary Figure S1. RT-qPCR analysis showed that the higher mRNA levels of IL-10, TGF-β, Nrf2, C3, and LZM-c at S. perfoliatum L. added group than the SPL0 group (Figures 3A, B, E, F, G), while significantly lower mRNA of TNF-α, IL-1β, and Keap1 was observed (Figures 3C, D, H).
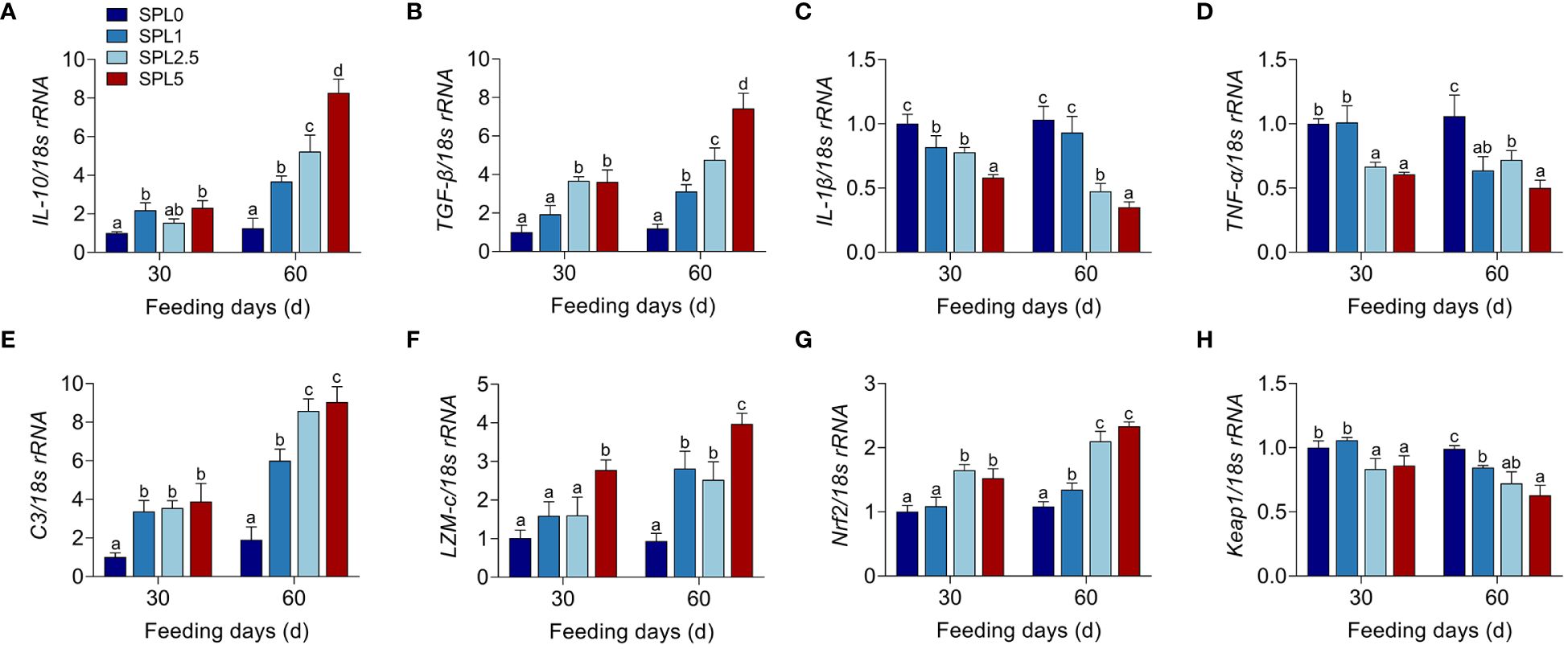
Figure 3 mRNA expression of IL-10 (A), TGF-β (B), IL-1β (C), TNF-α (D), C3 (E), LZM-c (F), Nrf2 (G), and keap1 (H) in the liver. Bars with different letters were significantly different according to the Duncan’s multiple range, n = 3, P< 0.05.
3.4 Effects of S. perfoliatum L. diet on the intestinal morphology
The effects of S. perfoliatum L. on the intestinal morphology were shown in Figure 4. No significant pathogenic changes were observed in intestinal tissues in all groups (Figure 4A). The length of the villus in the SPL5 was longer than that in the SPL0 (P< 0.05), while no significant difference was founded in the SPL1 and SPL2.5 groups compared with SPL0 group (Figure 4B). Additionally, there was no difference among SPL0, SPL1, SPL2.5, and SPL5 groups in the width of the intestinal villus, the thickness of intestinal muscle layer, and the goblet cell number (Figures 4C–E).
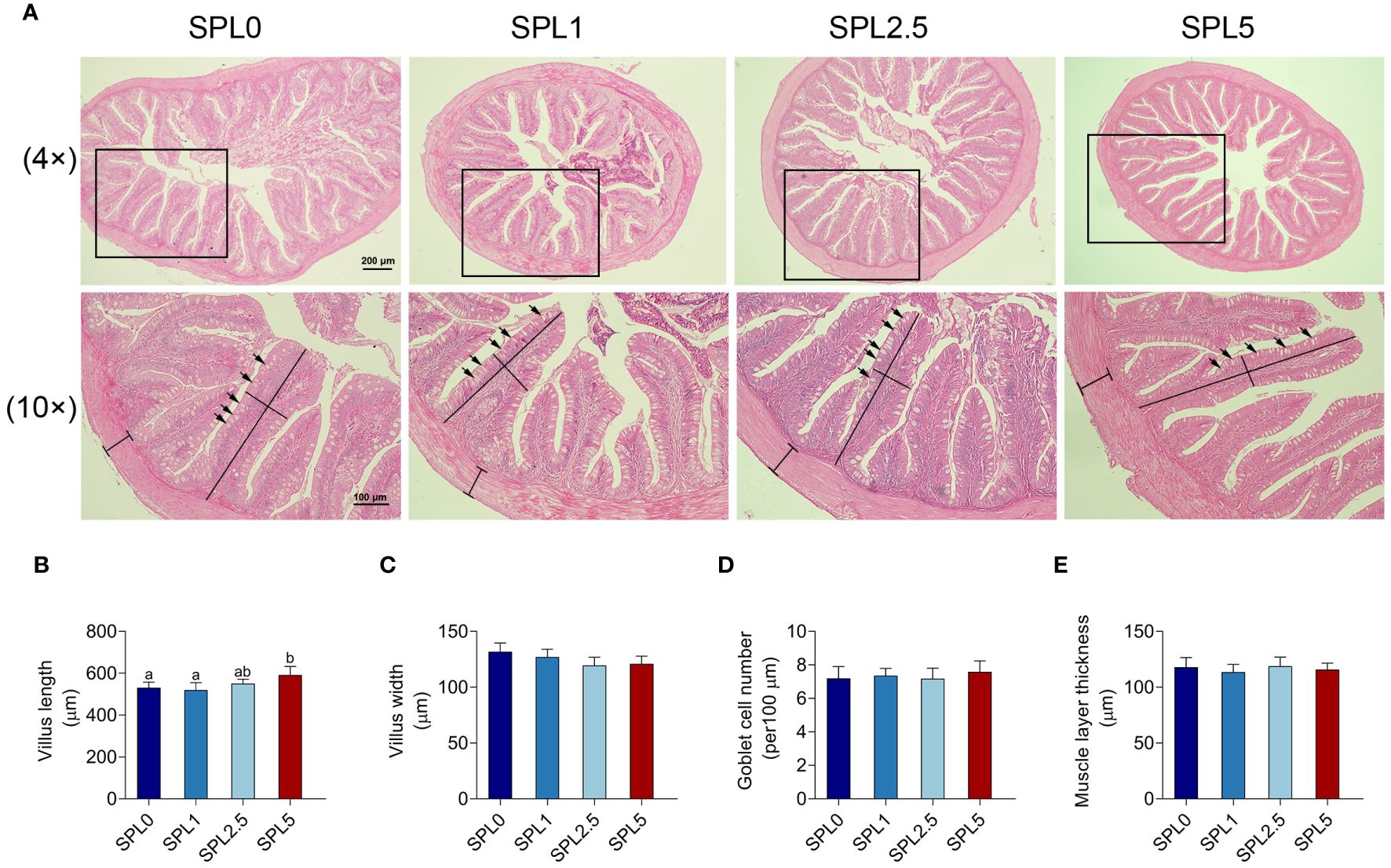
Figure 4 Histopathology analyses of intestinal section of largemouth bass. Samples were taken 60 days post the feeding experiment. (A) The hindgut tissues of SPL0, SPL1, SPL2.5, and SPL5 were performed for histopathology analysis. Villus length (B), villus width (C), goblet cell number (D), and muscular layer thickness (E) were statistically analyzed in different groups of largemouth bass. Black lines indicate villus length, width, and muscular layer thickness. Black arrows indicate goblet cells. Bars with different letters were significantly different according to the Duncan’s multiple range, n = 3, P< 0.05.
3.5 Effects of S. perfoliatum L. diet on the intestinal microbiota
The addition of 5% of S. perfoliatum L. to the feed produced the most significant results. As a consequence, SPL0 and SPL5 groups were selected to detect the effects of S. perfoliatum L. diet on the intestinal microbiota. The raw reads were stored in the NCBI Serial Read Archive (SRA) database under the login number PRJNA1000223.
The average OTUs of gut microbiota in SPL0 group was 416, and the average OTUs of gut microbiota in SPL5 group was 399. There were 132 OTUs shared by both groups (Figure 5A). Besides, the Simpson, Shannon, Ace, and Chao index of the intestinal microbiota alpha diversity index showed no significant differences in the SPL5 and SPL0 groups (Table 2). The rank abundance curves showed a high degree of fit between the shape and width of the two curves, indicating that species richness and evenness were similar in the SPL0 and SPL5 groups (Figure 5B).
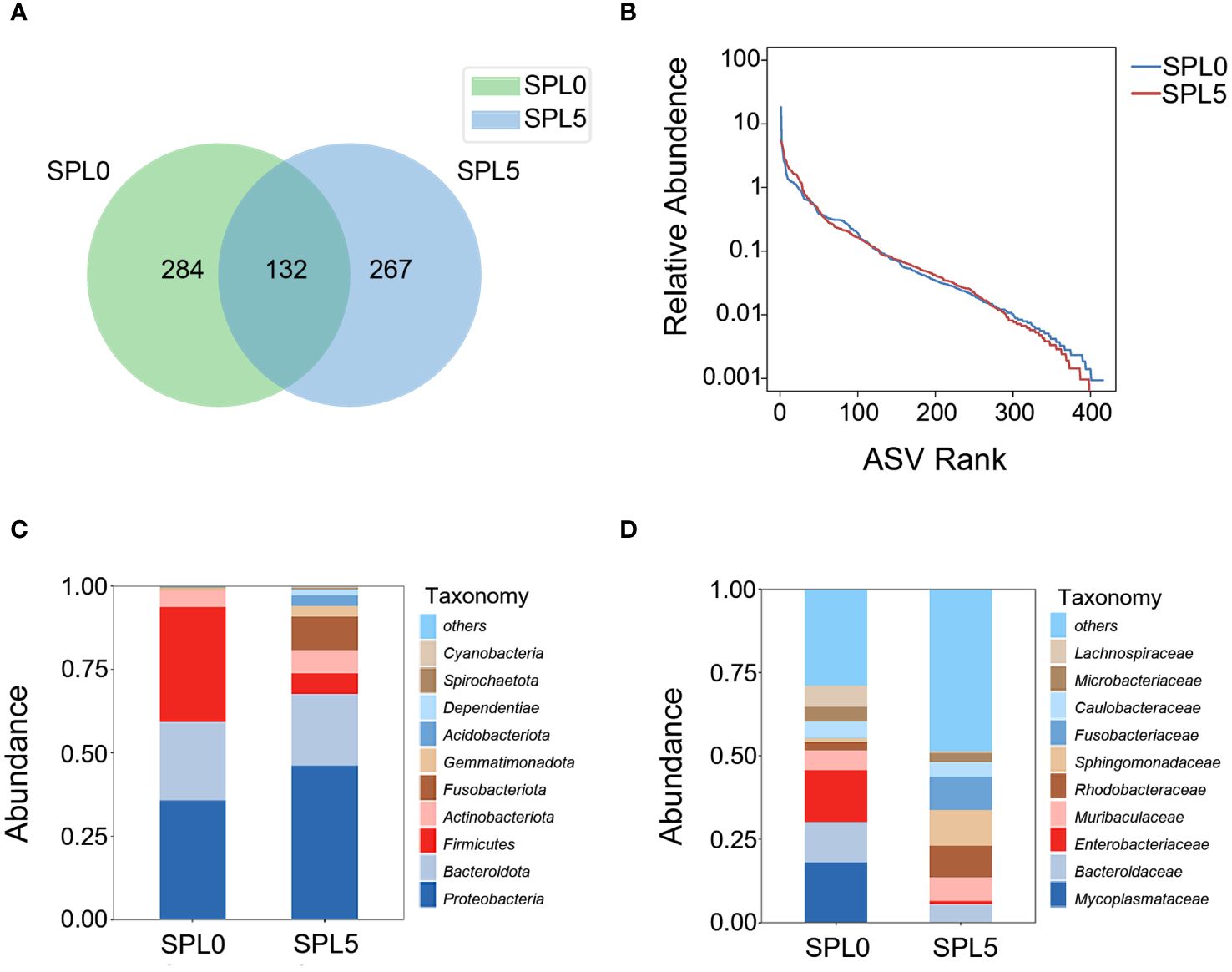
Figure 5 Effects of S. perfoliatum L. diet on the intestinal microbiota. (A) OTUs of intestinal microbiota communities in SPL0 and SPL5 groups. (B) The rank abundance curve of SPL0 and SPL5 groups. Intestinal microbiota composition in SPL0 and SPL5 at the phylum (C) and genus (D) levels. Only the top 10 most abundant (based on relative abundance) microbiota phyla and genera were shown.
Subsequently, we analyzed the composition and relative abundance of the intestinal microbial community at the phylum and family levels. The dominant microbiota at the phylum level were Proteobacteria, Bacteroidota, and Firmicutes (Figure 5C). While the dominant microbiota at the family level were Mycoplasmataceae, Bacteroidaceae, and Enterobacteriaceae (Figure 5D). The Wilcoxon-Mann-Whitney test revealed that S. perfoliatum L. supplementation elevated the relative abundance of Sphingomonadaceae and decreased the abundance of Mycoplasmataceae.
LEfSe analysis was employed to evaluate variations in the composition of intestinal microbiota communities between the SPL0 and SPL5 groups across various taxonomic levels, from phyla to genera. The SPL0 group exhibited higher relative abundances of Firmicutes, Gammaproteobacteria, Mycoplasma (from order to genus), and Enterobacteriaceae (from order to genus). However, the SPL5 group showed an increased relative abundance of Fusobacteriaceae (from phylum to family), Acidobacteriae (from phylum to class), Sphingomonas (from order to genus), and Cetobacterium (Figure 6).
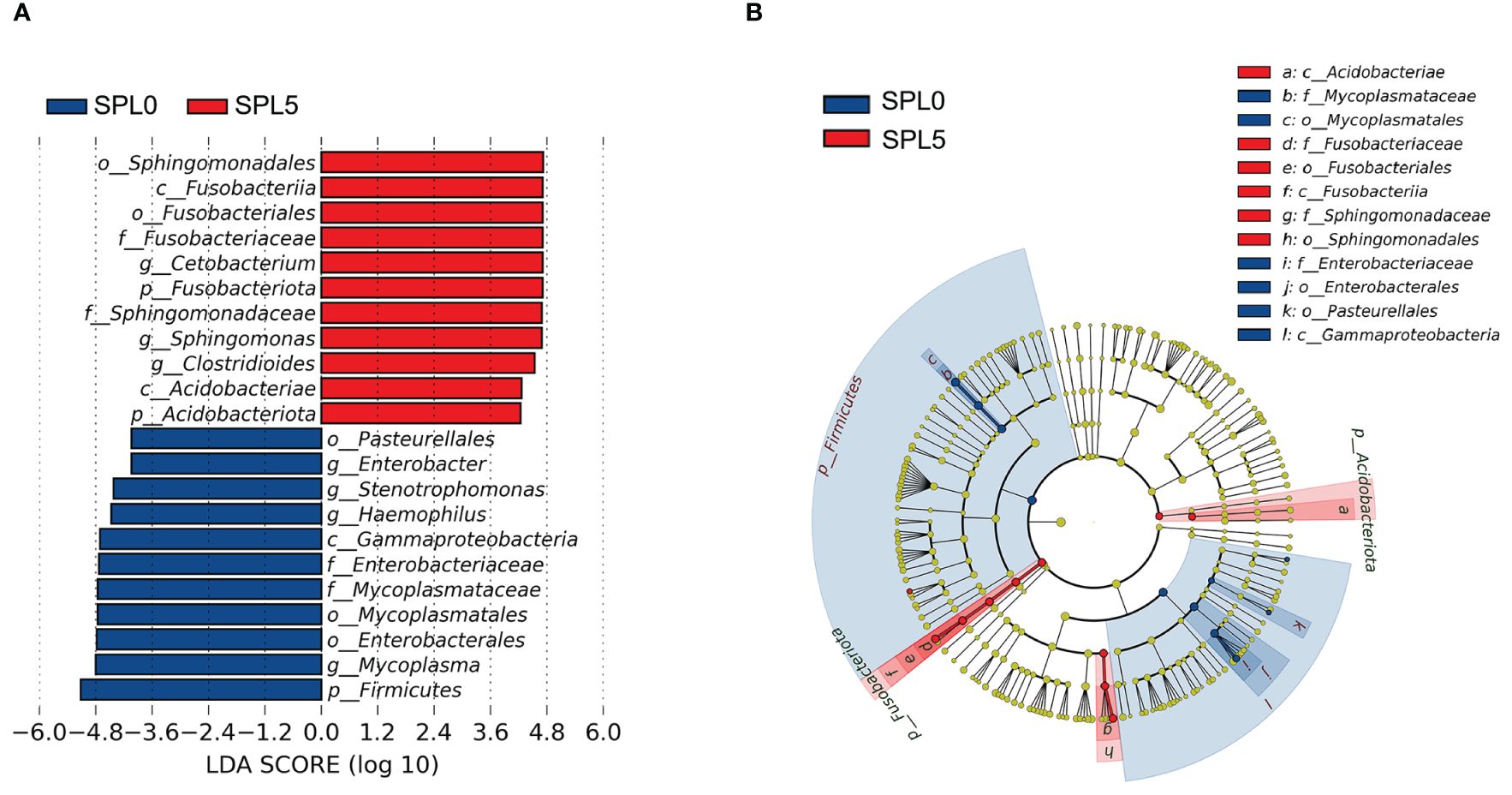
Figure 6 LEfSe analysis of intestinal microflora in largemouth bass. (A) Histogram of the distribution of LDA values for different species, with different colors indicating different groups (blue indicates SPL0 group; red indicates SPL5 group). (B) Evolutionary branching diagram. From the center outward, they represent the kingdom, phylum, class, order, family, genus, and species level, respectively. Blue and red nodes represent groups with significant differences in relative abundance in the SPL0 and SPL5 groups, respectively, and yellow dots indicate groups that are not significantly different in the comparison of the two groups.
3.6 Protective effect of S. perfoliatum L. on N. seriolae-infected largemouth bass
Next, we examined largemouth bass mortality within 15 days after N. seriolae infection. As shown in Figure 7, the survival rate of the SPL0 group was 16.67%, the SPL1 group was 36.67%, the SPL2.5 group was 43.33%, and the SPL5 group was 53.33%. Thus, the RPS of SPL1, SPL2.5, and SPL5 were 24%, 31.99%, and 43.99%, respectively. Overall, supplementation of S. perfoliatum L. protects largemouth bass from N. seriolae infection.
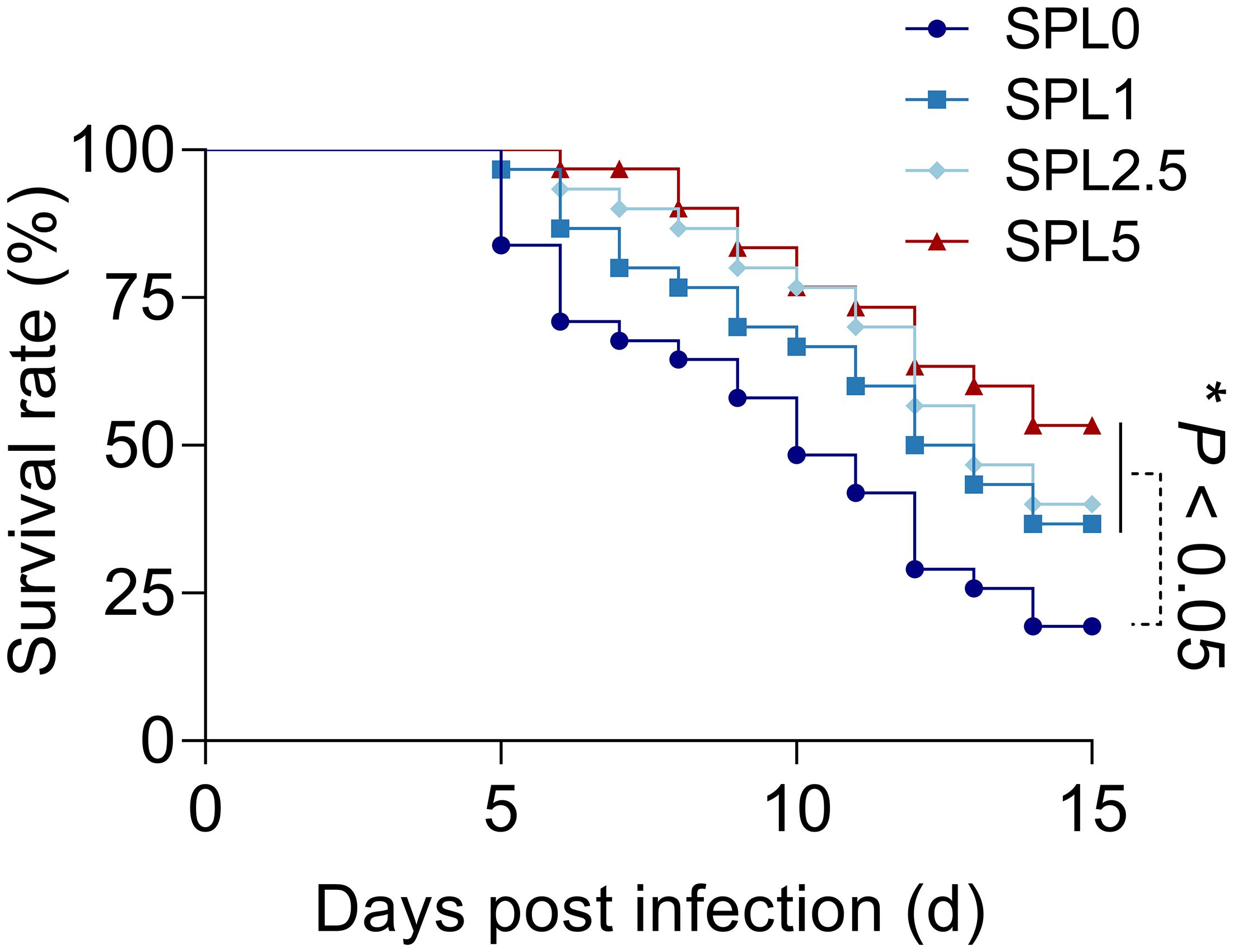
Figure 7 The cumulative mortality of largemouth bass infected with N. seriolae. Mortality was recorded daily and analyzed by the Kaplan-Meier survival curves. n = 3, *P< 0.05.
3.7 Supplementation of S. perfoliatum L. decreased the bacteria load in N. seriolae-infected largemouth bass
To further investigate the effect of S. perfoliatum L. supplementation on bacteria load, we analyzed the copy of N. seriolae in the liver, spleen, head kidney, and intestinal at 5 days post-infection. To confirm the CFU of N. seriolae, a standard curve was established using gradient diluted N. seriolae DNA (Figure 8A). The results showed that N. seriolae was detected in all selected tissues and the bacterial load was higher in the head kidney than in the liver, spleen, and intestinal. Compared with the SPL0 group, the N. seriolae load in SPL1, SPL2.5, and SPL5 decreased in a concentration-dependent manner in all detected tissues (Figure 8).
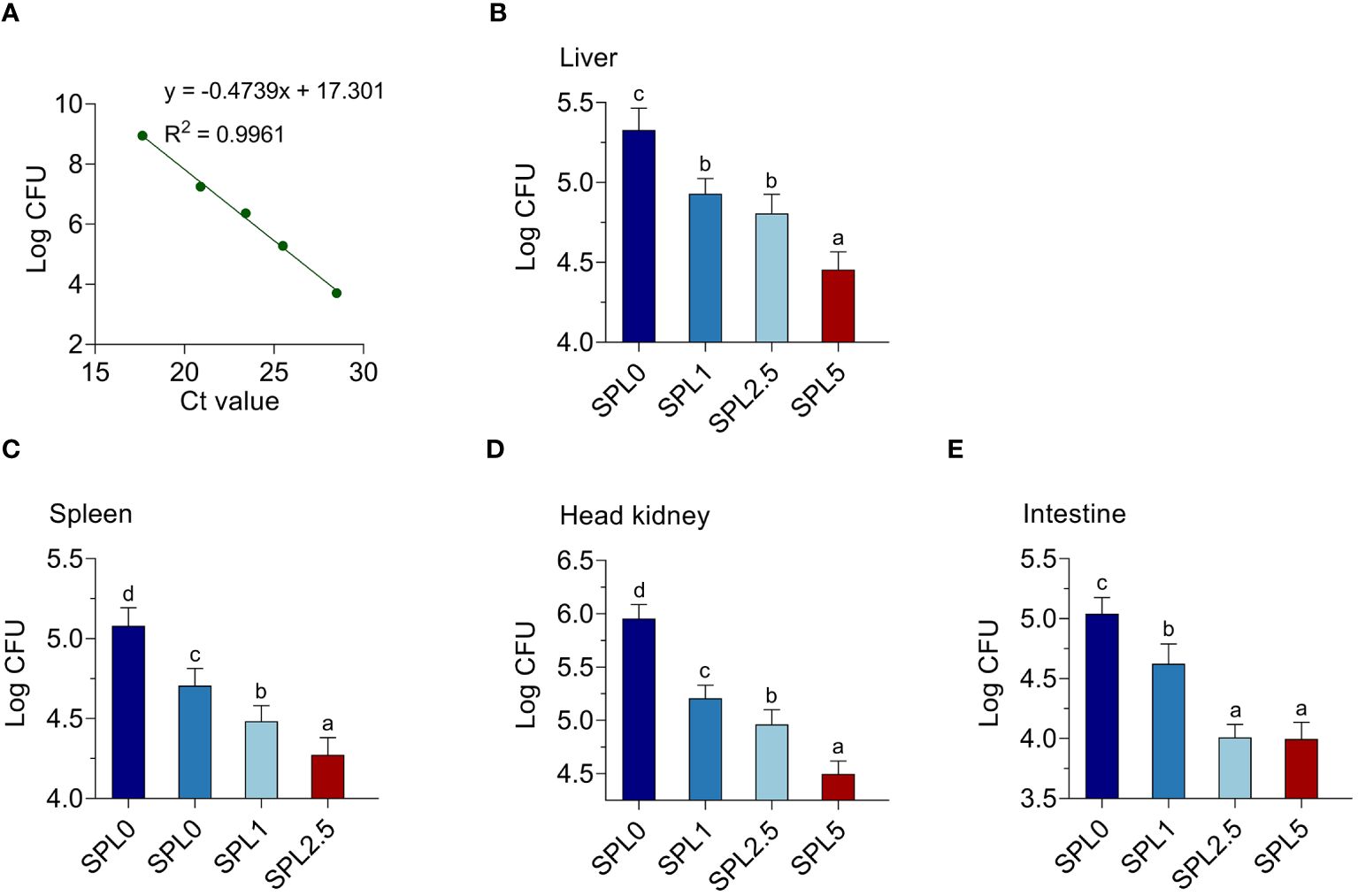
Figure 8 Supplementation of S. perfoliatum L. decreased the bacterial load in N. seriolae-infected largemouth bass. (A) A standard curve was established by determining the mean cycle threshold values. The bacteria load in the liver (B), spleen (C), head kidney (D), and intestinal (E) at 5 days post N. seriolae infection. Bars with different letters were significantly different according to the Duncan’s multiple range, n = 3, P< 0.05.
4 Discussion
Natural plant contains a variety of natural nutrients and bioactive substances, which have been demonstrated to have beneficial effects on animal metabolism, the utilization of nutrients, growth promotion, and disease resistance of animals (Tadese, 2009; Dawood et al., 2018). Nowadays, natural plants is widely used as feed additives in aquaculture (He et al., 2022; Rashidian et al., 2022; Du et al., 2023). In this study, the role of S. perfoliatum L. as a plant feed additive was evaluated. Our results showed that the addition of 5% of S. perfoliatum L. to the basal feed positively affects the immunity, intestinal health and disease resistance of largemouth bass.
Plant additives have the function of promoting metabolism, improving nutrient utilization, and promoting the growth of animals (Tadese, 2009; Dawood et al., 2018). Tan et al. proved that the addition of Taraxacum officinale extract to the diet could improve the feeding utilization rate of golden pompon and promote growth (Tan and Sun, 2020). S. perfoliatum L. is rich in flavonoids, phenols, protein, vitamin C, vitamin E, polysaccharides, carotene, and superoxide dismutase (Guo et al., 2020). In our previous study, S. perfoliatum L. was added to the feed of Penaeus vannamei, then we found that addition of S. perfoliatum L. promotes the growth performance of shrimp (Du et al., 2023). Here, we found that addition of S. perfoliatum L. promoted the feeding utilization rate and the growth performance of largemouth bass. The above dates indicated that S. perfoliatum L. as a plant additive could promote the growth performance and feed utilization of largemouth bass.
Teleost has evolved to employ its antioxidant pathways to scavenge excess oxygen radicals (Wang et al., 2021). GST is a crucial enzyme in glutathione binding reaction, which catalyzes the first step of glutathione binding reaction (Board and Menon, 2013). SOD and CAT are scavengers of reactive oxygen species and important antioxidant enzymes, which are important components of resisting oxidative stress (Ray and Husain, 2002). We demonstrated that the activity of SOD, CAT, and GST of largemouth bass increased significantly after 60 days of supplementation with 5% S. perfoliatum L. in the basal diet, indicating that S. perfoliatum L. could improve the antioxidant activity of largemouth bass. Furthermore, we analyzed the effect of S. perfoliatum L. on antioxidant enzyme regulated genes expression. Nrf2 regulates the expression of various antioxidant-related genes, while it is restricted by the Nrf2-Keap1 complex (Yuan et al., 2018). It was demonstrated that dietary supplementation with Atractylodes polysaccharides upregulated Nrf2 and downregulated Keap1 in largemouth bass, resulting in enhancing activity of the antioxidant enzyme (Dong et al., 2022). Here, we found S. perfoliatum L. induced the expression level of Nrf2 but inhibited the expression of Keap1. Therefore, S. perfoliatum L. might promote the antioxidant capacity through the Nrf2/Keap1 pathway in largemouth bass.
Cytokines play an important role in the immune defense response of teleost (Pan et al., 2023). TNF-α and IL-1β are crucial pro-inflammatory cytokines that can serve as markers of inflammatory response (Jin et al., 2022). IL-10 and TGF-β are crucial anti-inflammatory cytokines involved in host defense against microbial pathogens (Lu et al., 2021b). It was reported that natural plants could mediate teleost innate immunity by improving the expression of anti-inflammatory factors and suppressing pro-inflammatory factors (Jiang et al., 2022; Zhou et al., 2022). The extract of Eupatorium (Asteraceae) contains anti-inflammatory properties and can be used to treat inflammation (Clavin et al., 2007). Here, the pro-inflammatory cytokines expression was reduced, and the anti-inflammatory cytokines expression was induced by the supplementation of S. perfoliatum L. to the basal diet. The results showed that S. perfoliatum L. as plant additive could reduce the inflammation of largemouth bass.
Intestine is the main site of nutrient digestion and absorption, and its health is crucial for the growth and development of animals (Jiang et al., 2022). He et al. proved that supplementation the extract of 0.05% Artemisia annua promotes the length of intestinal villus in largemouth bass, but there are no significant differences in villus width, muscle thickness, and the goblet cell numbers (He et al., 2022). Similar results were obtained in our study, adding S. perfoliatum L. to the basic diet improved the intestinal villus length of largemouth bass. The increase in intestinal villus length implies an enhancement in intestinal nutrient absorption area, allowing for better absorption of available nutrients. The data suggested that S. perfoliatum L. could be used as a feed additive to improve intestinal morphology.
The intestinal-associated microbiota has co-evolved with their respective hosts and plays an important role in immune function and protection against pathogens (Wu et al., 2022). Proteobacteria, Bacteroidota, and Firmicutes dominate the intestinal microbiota of various fish (Pardesi et al., 2022; Cai et al., 2023). Similarly, in our study, Proteobacteria, Bacteroidota, and Firmicutes were the dominant intestinal microbiota in intestinal contents of largemouth bass. Mycoplasma has been recognized as highly probable cause of intestinal inflammatory diseases in mammals (Macfarlane et al., 2009; Gnanadurai and Fifer, 2020). Parshukov et al. found that Mycoplasma enrichment leads to changes in the fish gut microbiome which could negatively affect fish (Parshukov et al., 2022). It is noteworthy that the addition of S. perfoliatum L. led to a significant decrease in the abundance of Mycoplasma in the gut microbiota of largemouth bass. Sphingomonas and Cetobacterium, potential aquaculture probiotics, exhibited increased abundance post S. perfoliatum L. supplementation. Sphingomonas contributes to nutrient metabolism, detoxification, and prebiotic production, inhibiting Vibrio growth (Zhao et al., 2017; He et al., 2018). Cetobacterium promotes intestinal and liver health, synthesizing vitamin B12 and acetate, enhancing nutrient absorption, and maintaining gut microbiota stability (Finegold et al., 2003; Tsuchiya et al., 2008). Thus, our study demonstrates the beneficial effects of S. perfoliatum L. supplementation on largemouth bass intestinal microbiota.
The bacterial challenge test provides an opportunity to evaluate the protective effect of dietary treatments on pathogens and is usually used as the final indicator of fish health after nutritional testing (Li et al., 2020). Our results showed that the addition of S. perfoliatum L. to the basal feed positively affected the growth performance, immunity and intestinal health of largemouth bass. Therefore, it is not difficult to understand that S. perfoliatum L. increases the survival rate of largemouth bass post N. seriolae infection assay. In addition, the bacterial load decrease in tissues usually leads to lower mortality rates. S. perfoliatum L. is rich in flavonoids and phenolic compounds, which have the ability to destroy bacterial membrane structures (Williams et al., 2009). As a result, the extract of S. perfoliatum L. containing flavonoids and phenols has antibacterial activity (Kanatt et al., 2010). For instance, S. perfoliatum L. extract has antibacterial effects, including Enterococcus faecalis, Escherichia coli, Pseudomonas aeruginosa, and Staphylococcus aureus (Kowalski and Kedzia, 2007). Taken together, S. perfoliatum L. has a positive effect on the prevention of N. seriolae infection in largemouth bass.
5 Conclusion
In this study, it was found that the addition of S. perfoliatum L. to feed for 60 days could enhance the growth performance, boost immunity, promote intestinal health, and have an active role in the prevention of N. seriolae infection in largemouth bass. In summary, the study shows that S. perfoliatum L. is a potential green environmental feed additive.
Data availability statement
The datasets presented in this study can be found in online repositories. The names of the repository/repositories and accession number(s) can be found in the article/Supplementary Material.
Ethics statement
The animal study was approved by Animal Ethics Committee of Ningbo University. The study was conducted in accordance with the local legislation and institutional requirements.
Author contributions
HT: Investigation, Methodology, Software, Writing – original draft. SL: Investigation, Methodology, Software, Writing – original draft. JFC: Methodology, Writing – original draft, Conceptualization. QZ: Conceptualization, Methodology, Writing – original draft. GY: Conceptualization, Methodology, Writing – original draft. JL: Funding acquisition, Project administration, Writing – review & editing. JC: Project administration, Writing – review & editing.
Funding
The author(s) declare financial support was received for the research, authorship, and/or publication of this article. The project was supported by the Natural Science Foundation of Zhejiang Province (LY23C190001), the Program of Science and Technology Department of Ningbo City (2022S156), and the One Health Interdisciplinary Research Project of Ningbo University (HZ202201).
Conflict of interest
Author JFC was employed by Nanjing Silphium Biotechnology Company Limited.
The remaining authors declare that the research was conducted in the absence of any commercial or financial relationships that could be construed as a potential conflict of interest.
The author(s) declared that they were an editorial board member of Frontiers, at the time of submission. This had no impact on the peer review process and the final decision.
Publisher’s note
All claims expressed in this article are solely those of the authors and do not necessarily represent those of their affiliated organizations, or those of the publisher, the editors and the reviewers. Any product that may be evaluated in this article, or claim that may be made by its manufacturer, is not guaranteed or endorsed by the publisher.
Supplementary material
The Supplementary Material for this article can be found online at: https://www.frontiersin.org/articles/10.3389/fmars.2024.1432379/full#supplementary-material
References
Board P. G., Menon D. (2013). Glutathione transferases, regulators of cellular metabolism and physiology. Biochim. Biophys. Acta Biomembr. 1830, 3267–3288. doi: 10.1016/j.bbagen.2012.11.019
Cai M. C., Deng H., Sun H. C., Si W. T., Li X. Y., Hu J., et al. (2023). Changes of intestinal microbiota in the giant salamander (Andrias davidianus) during growth based on high-throughput sequencing. Front. Microbiol. 14. doi: 10.3389/fmicb.2023.1052824
Chen S. C., Lee J. L., Lai C. C., Gu Y. W., Wang C. T., Chang H. Y., et al. (2000). Nocardiosis in sea bass, Lateolabrax japonicus, in Taiwan. J. Fish Dis. 23, 299–307. doi: 10.1046/j.1365-2761.2000.00217.x
Chen S. W., Jiang X. L., Liu N., Ren M. C., Wang Z. J., Li M. K., et al. (2022). Effects of dietary berberine hydrochloride inclusion on growth, antioxidant capacity, glucose metabolism and intestinal microbiome of largemouth bass (Micropterus salmoides). Aquaculture 552, 738023. doi: 10.1016/j.aquaculture.2022.738023
Citarasu T. (2009). Herbal biomedicines: a new opportunity for aquaculture industry. Aquac. Int. 18, 403–414. doi: 10.1007/s10499-009-9253-7
Clavin M., Gorzalczany S., Macho A., Munoz E., Ferraro G., Acevedo C., et al. (2007). Anti-inflammatory activity of flavonoids from Eupatorium arnottianum. J. Ethnopharmacol. 112, 585–589. doi: 10.1016/j.jep.2007.04.007
Dawood M. A. O., Koshio S., Angeles Esteban M. (2018). Beneficial roles of feed additives as immunostimulants in aquaculture: a review. Rev. Aquac. 10, 950–974. doi: 10.1111/raq.12209
Dong B., Wu L. Y., Chen Q. Z., Xu W. J., Li D. G., Han D., et al. (2022). Tolerance assessment of Atractylodes macrocephala polysaccharide in the diet of largemouth bass (Micropterus salmoides). Antioxidants 11, 1581. doi: 10.3390/antiox11081581
Du Y., Hu X. M., Chen J. F., Xu W. L., Li H., Chen J. (2023). Investigation of the effects of cup plant (Silphium perfoliatum L.) on the growth, immunity, gut microbiota and disease resistance of Penaeus vannamei. Fish Shellfish Immunol. 135, 108631. doi: 10.1016/j.fsi.2023.108631
Finegold S. M., Vaisanen M. L., Molitoris D. R., Tomzynski T. J., Song Y., Liu C., et al. (2003). Cetobacterium somerae sp. nov. from human feces and emended description of the genus Cetobacterium. Syst. Appl. Microbiol. 26, 177–181. doi: 10.1078/072320203322346010
Gansberger M., Montgomery L. F. R., Liebhard P. (2015). Botanical characteristics, crop management and potential of Silphium perfoliatum L.as a renewable resource for biogas production: a review. Ind. Crop Prod. 63, 362–372. doi: 10.1016/j.indcrop.2014.09.047
Giri S. S., Jun J. W., Sukumaran V., Park S. C. (2016). Dietary administration of banana (Musa acuminata) peel flour affects the growth, antioxidant status, cytokine responses, and disease susceptibility of rohu, Labeo rohita. J. Immunol. Res. 2016, 4086591. doi: 10.1155/2016/4086591
Gnanadurai R., Fifer H. (2020). Mycoplasma genitalium: a review. Microbiology-Sgm 166, 21–29. doi: 10.1099/mic.0.000830
Gomez D., Sunyer J. O., Salinas I. (2013). The mucosal immune system of fish: the evolution of tolerating commensals while fighting pathogens. Fish Shellfish Immunol. 35, 1729–1739. doi: 10.1016/j.fsi.2013.09.032
Guo Y., Shang H. M., Zhao J. C., Zhang H. X., Chen S. L. (2020). Enzyme-assisted extraction of a cup plant (Silphium perfoliatum L.) polysaccharide and its antioxidant and hypoglycemic activities. Process Biochem. 92, 17–28. doi: 10.1016/j.procbio.2020.03.005
Habte-Tsion H. M., Kolimadu G. D., Rossi W. Jr., Filer K., Kumar V. (2020). Effects of Schizochytrium and micro-minerals on immune, antioxidant, inflammatory and lipid-metabolism status of Micropterus salmoides fed high- and low-fishmeal diets. Sci. Rep. 10, 7457. doi: 10.1038/s41598-020-64286-9
He G. L., Sun H., Liao R. S., Wei Y. X., Zhang T. T., Chen Y. J., et al. (2022). Effects of herbal extracts (Foeniculum vulgare and Artemisia annua) on growth, liver antioxidant capacity, intestinal morphology and microorganism of juvenile largemouth bass, Micropterus salmoides. Aquac. Rep. 23, 101081. doi: 10.1016/j.aqrep.2022.101081
He M. M., Guo M., Zhang X., Chen K. K., Yan J. P., Irbis C. (2018). Purification and characterization of alginate lyase from Sphingomonas sp. ZH0. J. Biosci. Bioeng. 126, 310–316. doi: 10.1016/j.jbiosc.2018.01.017
Hoang H. H., Wang P. C., Chen S. C. (2020). Interleukin 34 serves as a novel molecular adjuvant against Nocardia seriolae infection in largemouth bass (Micropterus salmoides). Vaccines 8, 151. doi: 10.3390/vaccines8020151
Hu C. H., Bie H. Q., Lu Z. Y., Ding Y., Guan H. H., Geng L. H., et al. (2023). Out-of-season spawning of largemouth bass in a controllable recirculating system. Front. Physiol. 14. doi: 10.3389/fphys.2023.1175075
Hu S. Q., Lin Y., Shi X. L., Chi C. H., Yan Y., Miao L. H., et al. (2024). Effects of dietary supplementation of Silphium perfoliatum L. @ on growth performance, antioxidant capacity and lipid metabolism of juvenile Megalobrama amblycephala. J. Fisheries China 48, 323–336. doi: 10.11964/jfc.20230814122
Jiang L., Zhou X. H., Yu J., Bao S. S., Li J., Wu Q., et al. (2022). Fermented tea residue improved growth performance, liver antioxidant capacity, intestinal morphology and resistance to Aeromonas hydrophila infection in juvenile largemouth bass (Micropterus salmoides). Front. Mar. Sci. 9. doi: 10.3389/fmars.2022.999947
Jin T. C., Lu J. F., Luo S., Wang L. C., Lu X. J., Chen J. (2022). Characterization of large yellow croaker (Larimichthys crocea) osteoprotegerin and its role in the innate immune response against to Vibrio alginolyticus. Comp. Biochem. Physiol. B Biochem. Mol. Biol. 258, 110680. doi: 10.1016/j.cbpb.2021.110680
Kanatt S. R., Chander R., Sharma A. (2010). Antioxidant and antimicrobial activity of pomegranate peel extract improves the shelf life of chicken products. Int. J. Food Sci. Technol. 45, 216–222. doi: 10.1111/j.1365-2621.2009.02124.x
Kariya T., Kubota S., Nakamura Y., Kira K. (1968). Nocardial infection in cultured yellowtails (Seriola quinqueruiata and S. purpurascens)- I. bacteriological study. Fish Pathol. 3, 16–23. doi: 10.3147/jsfp.3.16
Kowalski R., Kedzia B. (2007). Antibacterial activity of Silphium perfoliatum extracts. Pharm. Biol. 45, 494–500. doi: 10.1080/13880200701389409
Lei X. P., Zhao R. X., Geng Y., Wang K. Y., Yang P. O., Chen D. F., et al. (2020). Nocardia seriolae: a serious threat to the largemouth bass Micropterus salmoides industry in Southwest China. Dis. Aquat. Org. 142, 13–21. doi: 10.3354/dao03517
Li X., Wei X., Guo X., Mi S. C., Hua X. M., Li N. Y., et al. (2020). Enhanced growth performance, muscle quality and liver health of largemouth bass (Micropterus salmoides) were related to dietary small peptides supplementation. Aquac. Nutr. 26, 2169–2177. doi: 10.1111/anu.13155
Liu Q., Cheng L. S., Wang M. Z., Shen L. F., Zhang C. X., Mu J., et al. (2024). Dietary sodium acetate and sodium butyrate improve high-carbohydrate diet utilization by regulating gut microbiota, liver lipid metabolism, oxidative stress, and inflammation in largemouth bass (Micropterus salmoides). J. Anim. Sci. Biotechno. 15, 50. doi: 10.1186/s40104-024-01009-4
Lu J. F., Luo S., Jin T. C., Lu X. J., Chen J. (2021a). Nonstructural protein NS26 of grass carp reovirus is a principal regulator for viral replication and infection. J. Fish. Dis. 44, 661–664. doi: 10.1016/j.aquaculture.2021.736482
Lu J. F., Luo S., Jin T. C., Wang L. C., Yang G. J., Lu X. J., et al. (2021b). Betaine protects ayu (Plecoglossus altivelis) against Vibrio Anguillarum infection in salinity by regulating the immunomodulatory activity of monocytes/macrophages. Aquaculture 536, 736482. doi: 10.1016/j.aquaculture.2021.736482
Lu J. F., Luo S., Tang H., Liang J. H., Zhao Y. F., Hu Y., et al. (2023). Micropterus salmoides rhabdovirus enters cells via clathrin-mediated endocytosis pathway in a pH-, dynamin-, microtubule-, rab5-, and rab7-dependent manner. J. Virol. 97, e00714–e00723. doi: 10.1128/jvi.00714-23
Lu J. F., Zhang M. L., Lu L. Q. (2018). Tissue metabolism, hematotoxicity, and hepatotoxicity of trichlorfon in Carassius auratus gibelio after a single oral administration. Front. Physiol. 9. doi: 10.3389/fphys.2018.00551
Macfarlane S., Steed H., Macfarlane G. T. (2009). Intestinal bacteria and inflammatory bowel disease. Crit. Rev. Clin. Lab. Sci. 46, 25–54. doi: 10.1080/10408360802485792
Nawaz M., Gao T. T., Huang K. J., Gouife M., Chen S. Y., Zhu S. W., et al. (2022). Pathogenicity, diagnosis, prevention strategies and immune response of bacterium Nocardia seriolae: a critical review. Aquac. Res. 53, 4901–4918. doi: 10.1111/are.15988
Nguyen P., Giovanni A., Cheng L. W., Wang P. C., Chen S. C. (2023). Pathogenicity characterization of nocardiosis in orange-spotted grouper (Epinephelus coioides) via exposure to nine distinct isolates of Nocardia seriolae and four administrative routes. J. Fish Dis. 46, 1257–1268. doi: 10.1111/jfd.13845
Pan J., Fei C. J., Hu Y., Wu X. Y., Nie L., Chen J. (2023). Current understanding of the cGAS-STING signaling pathway: structure, regulatory mechanisms, and related diseases. Zool. Res. 44, 183–218. doi: 10.24272/j.issn.2095-8137.2022.464
Pardesi B., Roberton A. M., Lee K. C., Angert E. R., Rosendale D. I., Boycheva S., et al. (2022). Distinct microbiota composition and fermentation products indicate functional compartmentalization in the hindgut of a marine herbivorous fish. Mol. Ecol. 31, 2494–2509. doi: 10.1111/mec.16394
Parshukov A. N., Fokina N. N., Sukhovskaya I. V., Kantserova N. P., Lysenko L. A. (2022). Infection and antibiotic treatment have prolonged effects on gut microbiota, muscle and hepatic fatty acids in rainbow trout (Oncorhynchus mykiss). J. Appl. Microbiol. 133, 1709–1724. doi: 10.1111/jam.15674
Peni D., Stolarski M. J., Bordiean A., Krzyżaniak M., Dębowski M. (2022). Silphium perfoliatum—a herbaceous crop with increased interest in recent years for multi-purpose use. Agriculture 10, 640. doi: 10.3390/agriculture10120640
Rashidian G., Shahin K., Elshopakey G. E., Mahboub H. H., Fahim A., Elabd H., et al. (2022). The dietary effects of nutmeg (Myristica fragrans) extract on growth, hematological parameters, immunity, antioxidant status, and disease resistance of common carp (Cyprinus carpio) against Aeromonas hydrophila. J. Mar. Sci. Eng. 10, 325. doi: 10.3390/jmse10030325
Ray G., Husain S. A. (2002). Oxidants, antioxidants and carcinogenesis. Indian. J. Exp. Biol. 40, 1213–1232. doi: 10.1016/j.elecom.2007.06.034
Sun Y. X., Wen C. Y., Ma Z., Huang W. M., Liu C., Li W., et al. (2023). Development of TaqMan quantitative PCR assay for detection of Nocardia seriolae in fish and the environment. J. Microbiol. Methods 205, 106650. doi: 10.1016/j.mimet.2022.106650
Tadese C. T. (2009). Herbal biomedicines: a new opportunity for aquaculture industry. Aquac. Int. 18, 403–414. doi: 10.1007/s10499-009-9253-7
Talpur A. D. (2014). Mentha piperita (Peppermint) as feed additive enhanced growth performance, survival, immune response and disease resistance of Asian seabass, Lates calcarifer (Bloch) against Vibrio harveyi infection. Aquaculture 420, 71–78. doi: 10.1016/j.aquaculture.2013.10.039
Tan X. H., Sun Z. Z. (2020). Dietary dandelion extract improved growth performance, immunity, intestinal morphology and microbiota composition of golden pompano Trachinotus ovatus. Aquac. Rep. 18, 100491. doi: 10.1016/j.aqrep.2020.100491
Tsuchiya C., Sakata T., Sugita H. (2008). Novel ecological niche of Cetobacterium somerae, an anaerobic bacterium in the intestinal tracts of freshwater fish. Lett. Appl. Microbiol. 46, 43–48. doi: 10.1111/j.1472-765X.2007.02258.x
Wang F., Wang X. G., Liu C., Chang O. Q., Feng Y. Y., Jiang L., et al. (2017). Nocardia seriolae infection in cultured jade perch, Scortum barcoo. Aquac. Int. 25, 2201–2212. doi: 10.1007/s10499-017-0184-4
Wang H., Qiu T. X., Lu J. F., Liu H. W., Hu L., Liu L., et al. (2021). Potential aquatic environmental risks of trifloxystrobin: enhancement of virus susceptibility in zebrafish through initiation of autophagy. Zool. Res. 42, 339–349. doi: 10.24272/j.issn.2095-8137.2021.056
Williams J. D., Wojcinska M., Calabria L. M., Linse K., Clevinger J. A., Mabry T. J. (2009). The flavonoids and phenolic acids of the genus Silphium and their chemosystematic value. Nat. Prod. Commun. 4, 435–446. doi: 10.1177/1934578X0900400325
Wu X. Y., Xiong J. B., Fei C. J., Dai T., Zhu T. F., Zhao Z. Y., et al. (2022). Prior exposure to ciprofloxacin disrupts intestinal homeostasis and predisposes ayu (Plecoglossus altivelis) to subsequent Pseudomonas plecoglossicida-induced infection. Zool. Res. 43, 648–665. doi: 10.24272/j.issn.2095-8137.2022.159
Xie S. W., Yin P., Tian L. X., Yu Y. Y., Liu Y. J., Niu J. (2020). Dietary supplementation of astaxanthin improved the growth performance, antioxidant ability and immune response of juvenile largemouth bass (Micropterus salmoides) fed high-fat diet. Mar. Drugs 18, 642. doi: 10.3390/md18120642
Xu X. Y., Li X. Q., Xu Z., Yao W. X., Leng X. (2021). Dietary Azomite, a natural trace mineral complex, improved the growth, immunity response, intestine health and resistance against bacterial infection in largemouth bass (Micropterus salmoides). Fish Shellfish Immunol. 108, 53–62. doi: 10.1016/j.fsi.2020.11.016
Yu L. L., Yu H. H., Liang X. F., Li N., Wang X., Li F. H., et al. (2018). Dietary butylated hydroxytoluene improves lipid metabolism, antioxidant and anti-apoptotic response of largemouth bass (Micropterus salmoides). Fish Shellfish Immunol. 72, 220–229. doi: 10.1016/j.fsi.2017.10.054
Yuan J., Wang D. F., Liu Y., Chen X. J., Zhang H. L., Shen F., et al. (2018). Hydrogen-rich water attenuates oxidative stress in rats with traumatic brain injury via Nrf2 pathway. J. Surg. Res. 228, 238–246. doi: 10.1016/j.jss.2018.03.024
Zhao Q., Yue S. J., Bilal M., Hu H. B., Wang W., Zhang X. H. (2017). Comparative genomic analysis of 26 Sphingomonas and Sphingobium strains: dissemination of bioremediation capabilities, biodegradation potential and horizontal gene transfer. Sci. Total Environ. 609, 1238–1247. doi: 10.1016/j.scitotenv.2017.07.249
Zhou X. H., Wang Y. C., Yu J., Li J., Wu Q. H., Bao S. S., et al. (2022). Effects of dietary fermented Chinese herbal medicines on growth performance, digestive enzyme activity, liver antioxidant capacity, and intestinal inflammatory gene expression of juvenile largemouth bass (Micropterus salmoides). Aquac. Rep. 25, 101269. doi: 10.1016/j.aqrep.2022.101269
Keywords: largemouth bass, Silphium perfoliatum L., immunity, intestinal microbiota, disease resistance
Citation: Tang H, Luo S, Chen J, Zhou Q, Yang G, Lu J and Chen J (2024) Effects of dietary Silphium perfoliatum L. on the growth performance, immunity, intestinal health, and resistance against Nocardia seriolae of largemouth bass (Micropterus salmoides). Front. Mar. Sci. 11:1432379. doi: 10.3389/fmars.2024.1432379
Received: 14 May 2024; Accepted: 05 July 2024;
Published: 23 July 2024.
Edited by:
Songlin Li, Shanghai Ocean University, ChinaReviewed by:
Xueshan Li, Jimei University, ChinaBasanta Kumar Das, Central Inland Fisheries Research Institute (ICAR), India
Dan Xu, Shanghai Ocean University, China
Copyright © 2024 Tang, Luo, Chen, Zhou, Yang, Lu and Chen. This is an open-access article distributed under the terms of the Creative Commons Attribution License (CC BY). The use, distribution or reproduction in other forums is permitted, provided the original author(s) and the copyright owner(s) are credited and that the original publication in this journal is cited, in accordance with accepted academic practice. No use, distribution or reproduction is permitted which does not comply with these terms.
*Correspondence: Jianfei Lu, jianfeilu1@163.com; Jiong Chen, jchen1975@163.com