- 1REEFS Lab, Department of Earth Sciences, Indian Institute of Technology - Gandhinagar, Palaj, Gandhinagar, Gujarat, India
- 2Geo-Ocean, Univ Brest, CNRS, Ifremer, UMR6538, Plouzané, France
Low–lying coral islands are susceptible to rising sea levels and climate change, posing risks to island habitability over the next century. The decadal timescale evolution of the islands can provide an understanding of the governing controls of change and island response. This study investigates variations in the shoreline morphology of the Lakshadweep coral islands (Northern Indian Ocean/Arabian Sea) using satellite datasets (2003–2022; CNES/Airbus;0.3m – 0.7m resolution) and sedimentological data. Of the thirty islands (ten inhabited), six islands (Bangaram, Thinnakara, Suheli, Minicoy, Androth and Bitra) have been studied. Most of these islands are less than 1 km2 in size except Androth and Minicoy (>4 km2). While the data is discontinuous for the islands due to cloud obscuring, the shoreline morphology depicts variations for all the islands’ studied, irrespective of habitation and size. Larger islands have undergone consistent erosion since 2007 (total land loss is approximately 3 - 5% cumulatively). Smaller islands have undergone lateral migration, with sediment erosion usually along the southern edge and sediment migration and accumulation northwards, however, overall, their size remains consistently stable. The migration of sediments is observed only from 2007–2017, which coincides with severe El–Nino Southern Oscillation (ENSO) and low amplitude positive Indian Ocean Dipole (IOD) events. Furthermore, sedimentological analysis along Bangaram’s (small island) accreting margin reveals unconsolidated bio-detrital grainstones with predominant sand-sized fractions (2 – 0.25mm). Corals (43%), molluscs (33%), forams (13%), and algae (8%) are the major sediment producers (with 3% unidentified bioclasts). The ongoing climate warming and ocean acidification will influence the carbonate sediment budget in addition to the changing hydrodynamics owing to monsoons, cyclones, and coupled ocean-atmospheric regional phenomenon, altering the sediment production, transport and accumulation on these islands. Furthermore, rising sea levels and consequent erosion by wave action might lead to rapid drowning in the next few centuries. Our study identifies the current status of the island size variability in the Lakshadweep Archipelago and how site-specific and global factors influence them, thus providing insights into assessing the habitability within these low-lying coral islands.
1 Introduction
Sea-level rise and global climatic changes present significant challenges to low-lying coastal environments. The current sea-level rise from 2006 is about 4.2 ± 0.4 mm/year (Fox-Kemper et al., 2021), with rates projected to increase in the coming decades. The subsequent coastal inundation and saline intrusion would lead to loss of life and property (Mimura, 1999; Woodroffe, 2008; Oppenheimer et al., 2019; Almar et al., 2021), posing risks to approximately 11% of the world’s population that resides on islands, and 3% of the urban population that live in regions below elevations of 5m (Neumann et al., 2015; Magnan et al., 2019). In particular, coral islands are vulnerable to sea-level rise and climatic changes (Roy and Connell, 1989, 1991; Nicholls et al., 2007). Sea level rise results in wave interactions that involve the generation of infragravity waves over the reef flats and subsequent run-up at the shoreline, leading to coastal inundation (Beetham et al., 2017; Kench et al., 2022), and this is dependent on the island height and reef length (Amores et al., 2022). Global climate change has also aggravated ocean warming and acidification, directly impacting reef productivity and sediment supply (Anthony et al., 2008; Narayan et al., 2021). However, studies have indicated varying responses of carbonate islands to sea-level rise and climate change (Kench et al., 2018; Fellowes et al., 2023). The island’s response to inundation is governed by its physical and biological characteristics, which are largely site-specific and can vary even within island groups. The interplay of several factors, such as hydrodynamics (Homewood et al., 2007; Scheffers et al., 2011; Tamura, 2012; David and Schlurmann, 2020; Jinoj et al., 2020; Shimozono et al., 2022), sediment producers (Hallock, 1981; Perry et al., 2011) and antecedent topography (Hosking, 1991; Carter and Woodroffe, 1995; Barry et al., 2007; Woodroffe, 2008; Shah-Hosseini et al., 2018; Amores et al., 2022; Shimozono et al., 2022) needs to be taken into consideration while modeling the response of the islands to climate change (Roy and Connell, 1991; McLean and Kench, 2015). Understanding the factors leading to island morphological variations can lead to adopting adequate coastal management strategies.
Satellite geomorphological studies provide critical data on the decadal timescale variations and can shed light on the dynamics of the coral islands in the context of climate change and sea level rise (Prat et al., 2016; Kench et al., 2018). In decadal scales, the response to the local and global factors might translate to the islands’ erosion, accretion or rotation (Fellowes et al., 2023). Erosion and submergence for low-lying islands present a considerable risk to island habitability, particularly in terms of land (loss), which forms one of the five central pillars of the habitability index (Duvat et al., 2021a). The countries with the most significant risk include Indonesia, India, Philippines, USA, and Brazil due to their high population density in low-lying coastal regions (Ferrario et al., 2014). Particularly in these regions, assessment of shoreline behavior through satellite studies is necessary to understand the vulnerability of the low-lying islands in the coming decades. A large number of studies have been conducted on islands from the Pacific (n = 666) and the Indian Ocean (n = 186) (Kench and Mann, 2017; Duvat, 2018). Aerial photographic satellite studies have been conducted for the islands in the Pacific, mainly Tuvalu, Kiribati and the islands in the central and western Pacific, while the Maldives have been investigated in detail in the Indian Ocean (Kench and Nichol, 2005; Liang et al, 2022). While quantitative data from the Pacific Islands have shown island stability or expansion (Rankey, 2011; McLean and Kench, 2015; Rankey et al., 2023), the islands in the Indian Ocean (Maldives) have been shrinking over time (Duvat, 2018), with an increase in land attributed to anthropogenic interventions (Holdaway et al., 2021; Carruthers et al., 2023; Fellowes et al., 2023) and seasonal variations in the local wind conditions (Duvat, 2018; Dawson, 2021).
Additionally, the Indian Ocean is significant in that, modeling studies predict a rise in sea level of 0.76m (Arabian Sea; Jyoti et al., 2023), as well as a maximum rise of up to 3.4°C in sea surface temperature (Sein et al., 2024), by the end of the 21st century in the Northern Indian Ocean. Studies have also found sustained, anomalous warming over the Northern and Western Indian Ocean compared to the other global oceans (Roxy et al., 2014), rendering these regions as critical zones. Thus, discerning the decadal-scale variations and the underlying mechanisms of change for the coral islands in the Indian Ocean (particularly in the Northern Indian Ocean) can provide insights into the response (and resilience) of the islands to global and local climate change. Further, these can help add to the database of coral island stability in the Indo-Pacific. The Lakshadweep Islands are the northernmost coral islands in the Indian Ocean, thus representing a critical zone to understand the growth/decline of coral islands in response to global and local effects.
The Lakshadweep Archipelago includes 30 islands and 12 atolls, of which 10 islands are inhabited with a population of 64,429, according to the 2011 Census, residing in an area of 32 km2 (Prakash et al., 2015). The average elevation is 2m mean sea level (MSL), which makes the islands especially vulnerable to rising sea levels and coastal erosion (Prakash et al., 2015). The islands are also susceptible to storm surges and cyclones, as evidenced by the large-scale destruction due to the Okhi cyclone in 2019 (Riyas et al., 2019; Sanap et al., 2020; Mukherjee and Ramakrishnan, 2022). Furthermore, bleaching events due to ENSO could influence the subsequent sediment production (Arthur, 2000; Arthur et al., 2006; Yadav et al., 2018; McClanahan et al., 2019); affecting sediment supply. Previous studies have attested to erosion being a grave issue in most islands (Prakash et al., 2014), especially for the inhabited islands that have been attributed to human stress (Suganya et al., 2019). Although erosion zones have been defined (Prakash et al., 2015), variations in decadal timescales have not been estimated for these areas.
This study investigates the shoreline morphological variations and the various factors influencing the land gain vs. loss vs. stability of four small (Bangaram, Bitra, Thinnakara and Suheli; <1 km2) and two large (Minicoy and Androth; >4 km2) islands in Lakshadweep. The objectives of the study are, 1) to identify regions of erosion v/s accretion from 2003–2022, 2) to identify and quantify the relative abundance of the carbonate sediment producers and evaluate their adaptability with respect to variations in ocean parameters (ocean warming), and 3) to identify various causal mechanisms responsible for shoreline morphological variations. The islands for this study are chosen based on their size and habitability. The uninhabited islands have been chosen to discern whether the decadal timescale evolution of islands is determined solely by anthropogenic interventions (infrastructure/coastal management structures) or if local factors (ocean warming) can play a significant role in island stability. Besides, the smallest island, Bitra, is densely populated, with 300 people in 0.17 km2 area (Prakash et al., 2015). The large islands, Minicoy and Androth are densely populated with coastal infrastructures (Prakash et al., 2015; Government of India, UT of Lakshadweep); rendering these islands important socially and economically.
2 Regional settings
Located between 8° and 12° 30’ N and 71° and 74° E, the Lakshadweep Archipelago are coral islands in the Arabian Sea (Figure 1), off the western coast of the Indian Peninsula (Prakash et al., 2015; Mallik, 2017). The islands are the northernmost extent of the Laccadives-Maldives-Chagos Ridge and have been established atop a volcanic plateau formed as part of the Reunion hotspot (Duncan and Hargraves, 1990; Ashalatha et al., 1991; Belopolsky and Droxler, 2003; Droxler and Jorry, 2020). Most islands have lagoons on the western shores and islands on the eastern edge. The lagoons are usually 2 - 4 m water depth, sometimes exceeding >10 m for Bitra, Bangaram, Minicoy, and Suheli (Prakash et al., 2015). The elevation of the islands ranges between 0.5m to 6m (Jinoj et al., 2020).
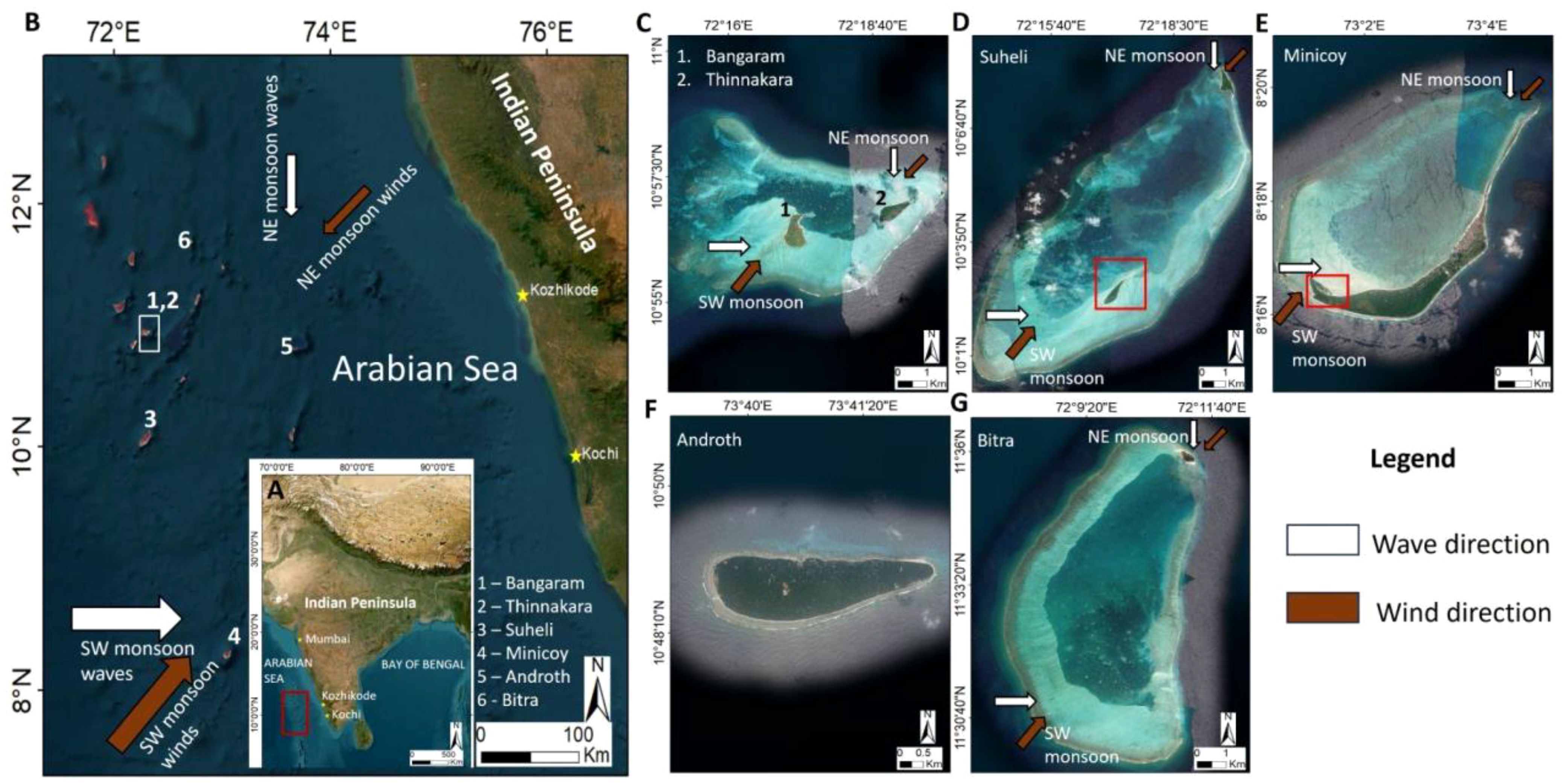
Figure 1 (A) Map of India with the location of the Lakshadweep Archipelago marked in red. (B) Map of Lakshadweep with the sites in the present study marked from 1–6. The arrow marks depict the local prevailing wind directions during the SW and NE monsoon (Brown arrows depict the wind direction while white arrows represent prevailing wave direction during each season). (C–G) Zoomed in satellite images of the islands in this study along with the hydrodynamic conditions depicted for each island during the SW and NE monsoon seasons. (C) Bangaram (1) and Thinnakara (2). (D) Suheli. The red rectangle depicts the location of Suheli. (E) Minicoy (red box depicts the region of migration of sediments for Minicoy). (F) Androth. (G) Bitra.
2.1 Hydrodynamics
Previous studies conducted by deploying Acoustic Velocimeters (Jinoj et al., 2020) and directional wave rider buoys (from 1990; Prakash et al., 2015) indicate that waves and wind magnitudes and directions are governed by the southwest (SW) monsoon. The monsoons control the wind direction, with a prominent NE direction observed during the NE monsoon (September – December), with a monthly average value of 4 m/s. In the months leading to the SW monsoon (June – August), wind velocities increase to about 18 m/s and majorly blow from the SW direction (Prakash et al., 2014, 2015; Jinoj et al., 2020).
The maximum wave height in each recording period averages 1.5 m, with the highest values from June-August during the SW monsoon (Dinakaran et al., 2022). Moreover, the wave direction is SW to NW (106° – 316°) during the year. During the SW monsoon, the wave distribution is dominantly westerly, with a maximum amplitude of 3.4 m. The tides are mixed semi-diurnal type with a maximum range of 1.4 m (Prakash et al., 2015).
Modeling studies have demonstrated that the currents are induced by waves breaking near the reefs (Prakash et al., 2014; Jinoj et al., 2020). The magnitude of the longshore currents ranges from 0.1 to 0.15 m/s during the NE monsoon, while during the SW monsoon, the magnitude varies from 0.2 to 0.4 m/s. The longshore currents in the eastern part of the island are stronger (0.50 m/s) than the western (0.15 m/s) due to the proximity of the reef flats to the islands (Prakash et al., 2014; Jinoj et al., 2020).
3 Methodology
3.1 Remote sensing studies and shoreline interpretation
Satellite geomorphological studies were carried out on six islands of the Lakshadweep Archipelago (Figure 1) based on inhabitation (inhabited vs. uninhabited) (Table 1). Additionally, the islands have been categorized based on size as small islands (<1 km2) and large islands (>4 km2) (the largest one being 4.9 km2). There were no islands in size between 1–4 km2 among the islands investigated in this study. Inhabited islands include Bangaram, Minicoy, Androth, and Bitra. The uninhabited islands are Thinnakara and Suheli.
Satellite imagery from Google Earth (CNES/Airbus/) (0.3m – 0.7m resolution) available from 2003 to 2022 was used to assess the shoreline changes. However, the data was discontinuous, with time gaps between 2008 – 2010 and 2012 – 2013 (for all islands) (See Supplementary Data 1) between each dataset, as these islands are remote and have sparse collections. Some data were obscured by clouds or were unclear. All the satellite images for the islands were collected post-monsoon (October – January). The images were then georeferenced using the latest satellite imagery (2022) and transformed using a second-order polynomial function. The toe of the beach was used to demarcate the islands’ shoreline (Webb and Kench, 2010; Rankey et al., 2023), following the methodology adopted by Dawson (2021). This also removes any influence from tides (Talavera et al., 2020) and is identifiable in all the image sources and environmental conditions. The digitization was carried out manually in QGIS 3.2.6, considering the uncertainties pertaining to the spatial resolution of satellite images and digitizing errors. Due to the variations in accuracies of the satellite images as well as extensive variations in shoreline over the time period studied, changes in shoreline positions (in terms of absolute area) of up to ± 3% were not considered significant (stable) (Webb and Kench, 2010).
3.2 Sedimentological studies
Based on satellite studies and the subsequent morphological variations, field investigations were conducted in Bangaram in March 2022. Surface sediment samples were collected from marked transects along the shoreline. The samples were freeze-dried, after which subsamples (20g) were dried in an oven at 40°C for two-three days; these were weighed and wet–sieved to separate each grain fraction (>4, 4 – 2, 2 – 0.5, 0.5 – 0.25, 0.25 – 0.125, 0.125 – 0.063, <0.063mm; Wentworth, 1922). After drying the samples (oven at 40°C for two days), they were weighed again. The samples were then classified according to Dunham (1962) classification, with the following ranges with respect to mud content – <10%: grainstone, 10 – 26%: packstone to grainstone, 26 – 42%: packstone, 42 – 58%: wackestone to packstone, 58 – 74%: wackestone, 74 – 90% mudstone to wackestone and >90% mudstone (Petrovic et al., 2022).
For petrography, the grains (2 – 0.5mm and 0.5mm – 0.25mm size fractions) were embedded in epoxy resin, made into thin sections, and then analyzed with a LEICA DM750 polarizing microscope. The quantitative estimation of the sediment components was carried out using the JMicroVision software. Point counting was conducted with 200 counts per section. The major components are coral fragments, foraminifera, molluscs and algae (green/red algae). Halimeda has been considered separate due to its relevance in producing carbonate sediments.
4 Results
4.1 Satellite geomorphology
4.1.1 Small islands (<1km2)
4.1.1.1 Thinnakara (Uninhabited island)
Time series satellite datasets for Thinnakara include imagery from 2003, 2007, 2011, 2014, 2016, 2017 and 2022 (Figure 2A). From 2003, the absolute area decreased from 0.56 km2 to 0.54 km2 in 2007. The island gradually grew in area from 2007 till 2011 (0.66 km2), then decreased by 2014 to 0.58 km2. An increase in area was observed thereafter, from 2014 to 2022 (approx. 0.63 km2). Overall, the island is migrating westwards, i.e., the island is migrating towards the lagoon. Up to 25% variation in island area has been observed over the timescales investigated in this study.
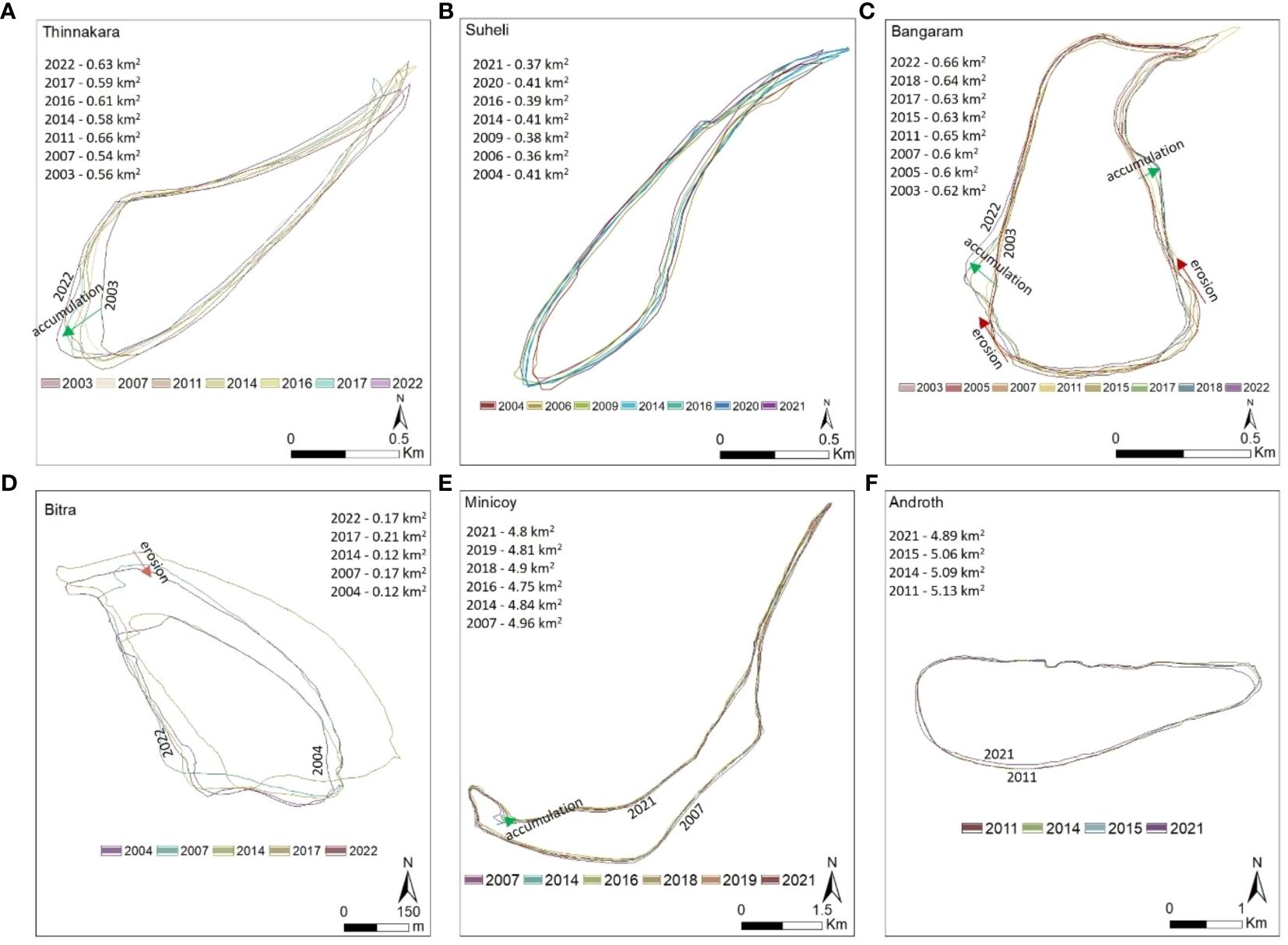
Figure 2 Shoreline morphological variations of the islands from 2003 – 2022. (A) Thinnakara. (B) Suheli. (C) Bangaram. (D) Bitra. (E) Minicoy. (F) Androth. The regions of extensive erosion and accretion have been depicted in red (erosion) and green arrows (accretion). The area of each island for the years surveyed has been shown for each island, depicting the overall variation for each year.
4.1.1.2 Suheli (Uninhabited island)
The datasets for this region are few or obscured by the clouds; the datasets included in this study are from 2004, 2006, 2009, 2014, 2016, 2020 and 2021 (Figure 2B). The island area decreased gradually from 2004 (0.41 km2) till 2016 (0.39 km2), after which an increase in area was observed until 2020 (0.41 km2), which decreases abruptly within a year by 2021 (0.37 km2). Mapping of the shoreline reveals that the island is migrating westwards towards the lagoon. Spit movement from the southern end to the island’s southwestern end was observed from 2004 to 2009, and subsequent migration of the sediments back to the south was seen from 2014 to 2022. The island is relatively stable, with less than 10% variation in absolute area.
4.1.1.3 Bangaram (Inhabited island)
Satellite studies have been undertaken for 2003, 2005, 2007, 2011, 2015, 2018 and 2022 (Figure 2C). The area of the island reduced from 0.62 km2 in 2003 to 0.60 km2 in 2007. A significant increase in area was observed from 2007 to 2011 (0.65 km2), after which the island gradually reduced in area in 2015 (0.63 km2). After 2015, the island increased in size until 2022 (0.66 km2). Moreover, in the period studied, substantial migration of sediments was observed in both the western and eastern parts of the island. On the western part, the sediments migrated from the south to approx. 100m north, whereas in the east, the sediments migrated from the south to about 400m north, accumulating as parallel - semi parallel ridge complexes. The island exhibits net accumulation through time, with less than a 10% change in absolute area over the period studied.
4.1.1.4 Bitra (Inhabited island)
The datasets are from 2004, 2007, 2014, 2017 and 2022 (Figure 2D). Unlike other islands, extensive variations in the island size are evidenced through satellite studies. The island size increased from 0.12 km2 in 2004 to 0.17 km2 in 2007, before reducing to 0.12 km2 in 2014. A sudden increase in area was observed until 2017 (0.21 km2), after which the island area decreased to 0.17 km2 by 2022. Net erosion was observed in the northern edge of the island over the studied time period. Further, extensive migration of sediments from the south to the southwest was also observed, indicating lateral migration in the south, over the time period studied. Bitra also exhibits the most significant variation of change in island area (30%) among all the islands in the study.
4.1.2 Large islands (4–5 km2)
4.1.2.1 Minicoy (Inhabited island)
The time series satellite dataset includes imagery from 2007, 2014, 2016, 2018, 2019 and 2021 (Figure 2E). It is observed that the island area decreased from 2007 (4.96 km2) to 2016 (4.75 km2). An increase in area was observed from 2016 to 2018 (4.9 km2), after which the island appears to have stabilized at about 4.8 km2, from 2019 to 2021 Spit growth was observed along the southwestern part of the island. Overall, the total change in the percentage of island area is calculated to be less than 5%.
4.1.2.2 Androth (Inhabited island)
Datasets for 2011, 2014, 2015 and 2021 show variabilities in the absolute area over time (Figure 2F). A general decreasing trend in the area was observed for the island from 2011 (5.13 km2) to 2021 (4.89 km2). Satellite studies indicate the migration of sediments in the eastern part of the island. Largely, the island has experienced net erosion of approximately 5%.
4.2 Sedimentological studies (Bangaram Island)
4.2.1 Grain size fractions
Grain size and texture analysis have been conducted along the Bangaram coastline (Figure 3) in particular, because the satellite studies indicate substantial transport of sediments. Extensive erosion is observed in the south and the sediments are observed to accumulate as parallel-semi parallel ridge complex systems towards the north (Figure 2C). Sediments have been collected from four locations along the coastline (Figure 3). First, at location A (Figure 3), a single sediment sample has been collected from a spit (exposed in low tide) approx. 200m north of Bangaram island. Sediments have also been collected from the accumulated ridges in the southwest, (n=8; Figure 3, transect from location B to C), and the eroded region in the south of the island (n=1; Figure 3, location D).
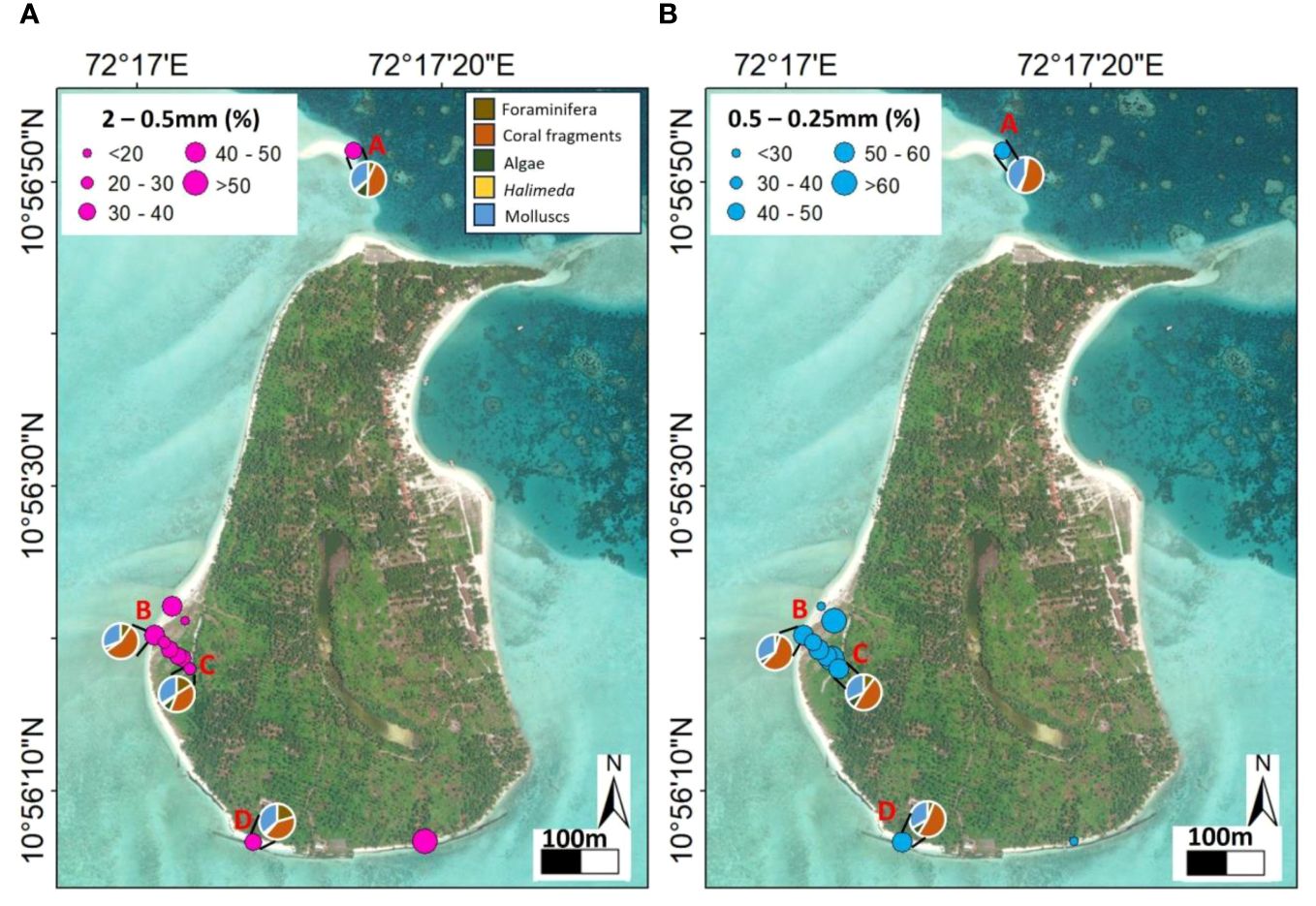
Figure 3 Map of Bangaram depicting the dominant grain size of the sediments collected along marked transects. (A) The size of the pink circles corresponds to the percentage of 2 – 0.5mm grain fraction present in each site. The pie charts represent the relative abundance of each sediment component (see legend) for each transect sampled. (B) The size of the blue circles represents the abundance (in percentage) of 0.5 – 0.25mm grained fraction in each site, and the pie chart corresponds to the relative abundance (in percentage) of each sediment component.
All the sediments (n=10) collected along the shoreline are unconsolidated bio-detrital grainstone, with grain size ranging from 2 – 0.5mm (38%), 0.5 – 0.25 mm (49%) and 0.25 – 0.125 mm (10%). There was no mud fraction. The spit in the north comprises dominantly, 2 – 0.5 mm (approx. 50%) and 0.5 – 0.25 mm (30%), grain size fraction (Figure 3). In the zone of accumulation, the dominant grain size fraction varies when moving inland. The coarser grain fraction (2- 0.5 mm) decreases progressively inland (from 33 to 20%). Consequently, the finer grain size (0.5 – 0.25 mm) increases (59 to 63%). The zones of erosion (southern end) have 0.5 – 0.25 mm (50%) and 2–0.5 mm (40%) grain size fractions (at location D in Figure 3).
4.2.2 Petrographic studies
Petrographic analysis of the 2–0.5 mm and 0.5–0.25 mm grain size fractions have been carried out for four samples, one from each location A, B, C, and D in Figure 3.
For 2–0.5 mm grain size fraction: The sediments collected from the sand spit exposed during low tide in the north of the island (Figure 3; location A) has coral fragments (44%), molluscs (36%), algae (12%; without Halimeda), forams (7%), and Halimeda (2%), respectively. The sediments collected from locations B (nearshore; Figure 3) and C (relatively inland; Figure 3) have coral fragments (56% and 41%), molluscs (31% and 34%), forams (9% and 16%), algae (3% and 8%), and Halimeda (<1% and 1%), respectively. Thus, coral fragments dominate in the sediments sampled near the coastline and their proportion reduces significantly (approx. 15%) moving inland. Furthermore, the abundance of molluscs, forams, algae and Halimeda increases marginally inland from B to C (Figure 3). In the zone of erosion (D in Figure 3A), corals are the major contributors (41%), followed by molluscs (36%) and forams (20%). Algae and Halimeda are minor components (less than 1%). On average, from these four locations, the dominant components for the 2–0.5 mm fraction include coral fragments (41%), molluscs (34%), foraminifera (13%), algae (6%; excluding Halimeda) and Halimeda (1%).
For 0.5–0.25 mm grain size fraction: The sediments collected from the sand spit exposed during low tide in the north of the island (Figure 3; location A) has coral fragments (53%), molluscs (40%), forams and algae (excluding Halimeda) constituting up to 3% each, and no Halimeda. The sediments collected from locations B (nearshore; Figure 3) and C (relatively inland; Figure 3) have coral fragments (58% and 48%), molluscs (approx. 32% each at B and C), foams (5% and 10%), and algae (<4% and 4%), respectively. Thus, similar to the coarse grain size fraction (2–0.5 mm), within the 0.5–0.25 grain size fraction the coral fragments dominate in the sediments sampled near the coastline and their proportion reduces moving inland. Additionally, the abundance of forams and algae increases marginally inland from B to C (Figure 3), with relatively no change in mollusc abundance. In the zone of erosion (D in Figure 3A), corals are the major contributors (53%), followed by molluscs (33%), forams (6%), and algae (7%; without Halimeda). On average, from these four locations, the dominant components for the 0.5–0.25 mm fraction include coral fragments (53%), molluscs (35%), forams and algae (6% each). Halimeda does not form a significant component in this particular grain size fraction (less than 1%).
Additionally, while detailed quantitative studies have been conducted for Bangaram, it has been noted that the major sediment producers for other islands are similar, with coral fragments, molluscs, forams and algae forming major components (Jinoj et al., 2020). However, quantitative analysis for the other islands has not been carried out. Thus, the sedimentological analysis for Bangaram can provide pertinent insights into the major sediment producers and their impact on sediment supply in the context of ongoing climate change.
5 Interpretation
5.1 Satellite geomorphology
The larger islands (4–5 km2) have been continuously losing land since 2007 (no data between 2003–2007), however, the smaller islands (<1 km2) have been relatively stable between 2003–2022 with a period (2007–2017) of large scale (up to approx. 30% of land area) sediment migration (erosion, transport, and accumulation). The migration of sediments in smaller islands thus suggests local controls on the processes influencing the smaller islands, however, the same processes do not influence the larger islands the same way.
5.1.1 Small islands
It has been observed that sediment migration is from the southern tip of the islands towards the north (for most of the islands). The wind and wave currents governed by the southwest (SW) monsoon scour sediments from the south, transporting them to the north (Jinoj et al., 2020). Thus, fluctuations in the intensity and magnitude of the SW monsoon could bring about significant variations in the shoreline morphology of the islands. Ocean-atmospheric interactions, mainly El-Nino Southern Oscillation (ENSO) and Indian Ocean Dipole (IOD) affect the monsoon (Ashok et al., 2001; Hameed and Yamagata, 2003; Kumar et al., 2006; Todmal et al., 2022; Ocean Surface Topography from Space; Open Government Data (OGD) Platform India; WEB Cyclone ATLAS: AboutEAtlas; COBE Sea Surface Temperature; NOAA, National Weather Services), leading to changes in the intensity and magnitude of the local wave and wind velocities and amplitudes. ENSO events are calculated using the sea surface temperature anomaly in the east-central Pacific Ocean, wherein the onset of El – Nino is declared when the anomaly exceeds 0.5°C for three months (Oceanic Nino Index (ONI); El Niño and La Niña (El Niño-Southern Oscillation); Trenberth, 1997; Hamlington et al., 2019). Severe El-Nino events have corresponded to low rainfall intensities while La-Nina events are characterized by higher rainfall over Lakshadweep (Ashok et al., 2001). IOD is calculated as the monthly difference of the sea surface temperatures between the western and eastern Indian Ocean (IOD index; Australian Government-Bureau of Meteorology Indian Ocean climate influences; Kumar et al., 2020). A positive IOD index leads to anomalous warming of the western Indian Ocean, leading to more rainfall in these areas, especially during the post-monsoon (Hameed and Yamagata, 2003; Cherchi and Navarra, 2013; Ratna et al., 2021). Negative IOD usually leads to a dearth in rainfall intensities over the western Indian Ocean (Kumar et al., 2006; Ashok and Saji, 2007; Ummenhofer et al., 2011; Rahaman et al., 2022).
ENSO and IOD records between 2003 – 2022 indicate several positive and negative phases (Figure 4), that have also been identified from local isotopic studies (corals) from Lakshadweep (Rahman et al., 2022). Within the ENSO and IOD record between 2003 to 2007, the severity of the ENSO is not high (SST Index <1) and the IOD is consistently negative with small periods of positive IOD. From 2007, severe ENSO events as well as an extended positive IOD phase are identified until 2016 (Figure 4). The resulting rainfall owing to the influence of the regional ocean-atmospheric phenomena has decreased between 2007–2016. This is the same period during which the migration of sediments along the smaller islands’ edges is observed. After 2017, the rainfall intensities increase significantly, potentially leading to the stabilization of the islands. Thus, we interpret that the local hydrodynamics are the primary reason responsible for small island shoreline modifications/stabilization, which is governed by ocean-atmospheric interactions.
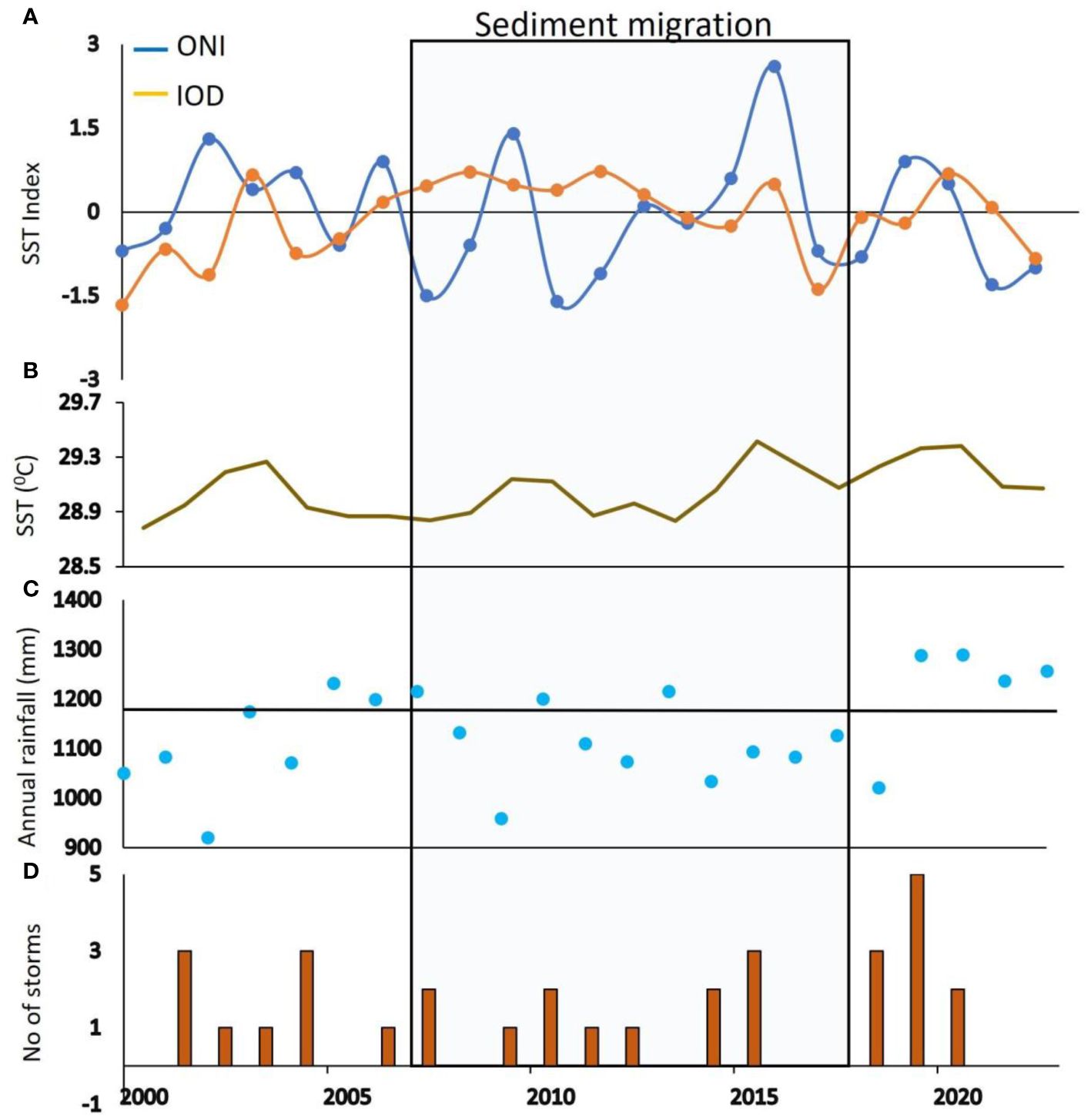
Figure 4 (A) Graph illustrating the El Nino-La Nina Index (ONI) (blue) and the Indian Ocean Dipole Index (ODI) (orange). Data for the Oni and IOD Index has been derived from Ocean Surface Topography from Space. (B) Graph of average annual sea surface temperatures over the Arabian Sea (near Lakshadweep). The data has been sourced from COBE Sea Surface Temperature. (C) Annual rainfall over India from 2000 – 2022. The black line indicates the average annual rainfall over India. The data has been derived from the Open Government Data Portal, Government of India and WEB Cyclone eAtlas. (D) Histogram of the number of storms in the Arabian Sea (near Lakshadweep) from 2000 – 2022. The light blue shaded rectangle corresponds to the years with the greatest variation in shoreline morphology (migration of sediments). Data presented in Supplementary Material 1.
5.1.2 Large islands
The two islands, Minicoy and Androth are very different in terms of their island geometry as well as overall configuration. Minicoy is an island on the southern margin of the Minicoy Atoll, however, Androth is an island surrounded by fringing reefs. From 2007–2022 (no data from 2003–2007) Minicoy reduced in size, however, an event of island growth is observed from 2016 - 2018 (Figures 2E, 5). It coincides with a transition from a severe El-Nino to La Nina event and a transition from positive to negative IOD. The influence of these ocean-atmosphere interactions indicates the strengthening of monsoon (Figure 4C), which would have influenced the wave intensity from W to E on the western margin of Minicoy island and transported the sediments from the nearby reef margin. However, for Androth, no such phenomenon is observed (that would increase the size of the island), indicating the island morphology, orientation, and accommodation (space) would play a key role in defining whether an island will grow or not.
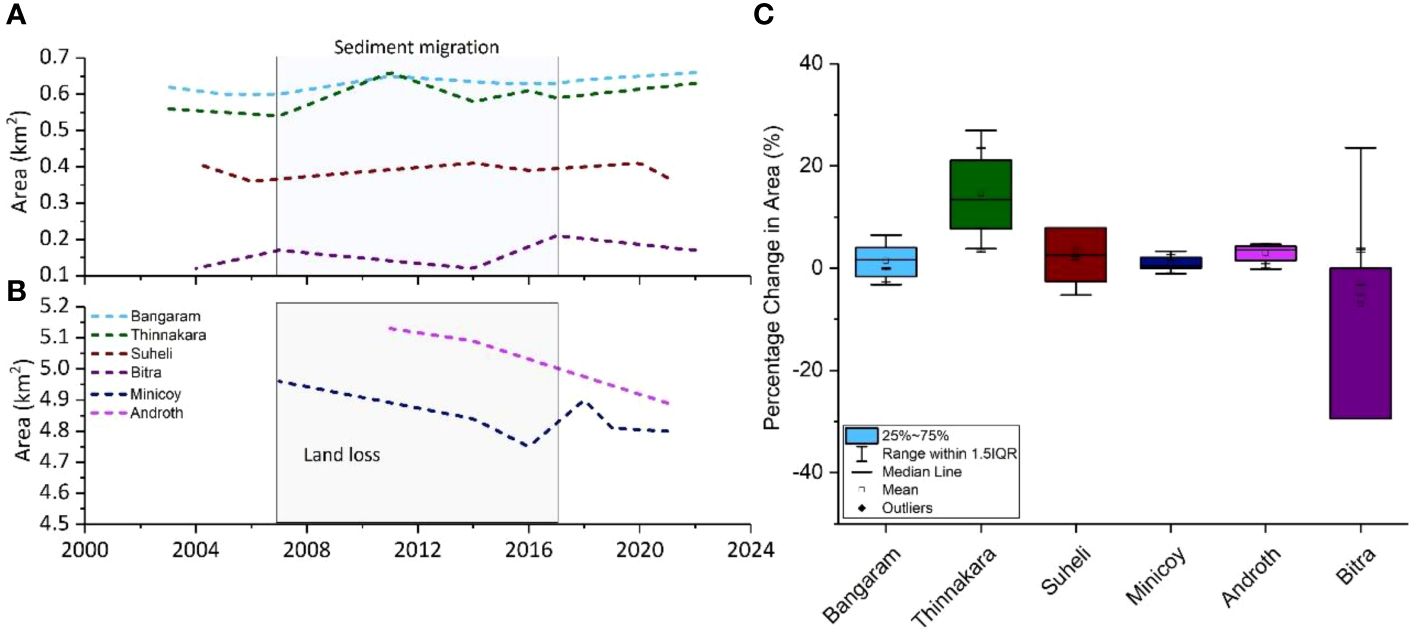
Figure 5 Graph illustrating the variations in total area of each island from 2003 to 2022. The area (km2) represents the absolute area of the islands for each timescale. (A) The variation in absolute area with time for the small islands. The light blue rectangle corresponds to the years with the largest extent of change in absolute area (migration of sediments). (B) Shoreline morphological variation over time for the large islands. The grey rectangle depicts the overall decrease in absolute area (land loss) over time. (C) Percentage change with respect to island area for each island. The error bars correspond to the standard deviation for each island. The standard deviation is largely less than 1. Data presented in Supplementary Data 1.
5.2 Sediment producers
Sedimentological studies have shown that the sediments are unconsolidated bio-detrital grainstone of sand-sized fractions (2 – 0.25mm). The component analysis has shown that, on average across all the locations sampled, coral fragments (43%) and molluscs (33%) form the dominant sediment producers, followed by forams (13%) and algae (8%) (cumulative for both grain size fractions). Halimeda comprises less than 1% of the total sediment components on average. The sediments are primarily constituted by eroded reef structures and skeletal organisms, and consequently, the sediment supply is determined by the abundance of these organisms and the rates of sediment transport (Browne et al., 2021; Ainesi et al., 2024). Corals are environmentally fragile and widespread bleaching has impacted the recovery rates and growth during high sea surface temperatures during the 2016 El–Nino in Lakshadweep (Yadav et al., 2018). There has also been a significant decline in coral cover and a shift in species from branching (less heat resistant) to stony corals (heat resistant) has been observed in the Lakshadweep Islands, which could affect sediment production rates (Yadav et al., 2018; Divan Patel et al., 2023). Climate change-induced habitability variations have also led to coral diseases, affecting coral growth (Das et al., 2023). Since the bulk of the sediment is composed of coral fragments, changes in environmental conditions could lead to a decrease in sediment supply, impacting the shoreline morphology. Molluscs are the other major sediment producers, with a broader range of habitability in terms of environmental conditions. However, increasing warming and acidification due to human-induced climate change exacerbate mollusc mortality (Rodolfo-Metalpa et al., 2011).
Anthropogenic interventions in terms of coastal infrastructure have led to the destruction of reef structures, adversely affecting sediment production (Lokier and Fiorini, 2016). Besides, in Lakshadweep, a decrease in the abundance of foraminifera has also been observed, which has been attributed to anthropogenic interventions (Panchang et al., 2023). Thus, for the inhabited islands, sediment production can be severely impacted by the declining reef health induced by human stresses, notably due to the decrease in the contribution of coral fragments. Therefore, quantitative studies of the various sediment producers for both Minicoy,Androth and Bitra can provide further evidence on the sediment production for these islands and the possible impact of anthropogenic activities influencing sediment production. In the future, the ability of each sediment producer (corals, forams, invertebrates, and algae) to withstand ongoing seasonal as well as long-term ocean warming and human stresses can dictate the sediment production and resilience of the carbonate system.
6 Discussion
The decadal timescale variations of the islands’ shoreline morphology provide insights into the island modification by local and global factors and how they might be influenced due to ongoing climate warming. Subsequently, this could lead to the critical reevaluation of the current practices in coastal management and, eventually, adopt better practices.
Results from satellite geomorphological studies present a different perspective from the narrative of island loss due to sea level rise. Irrespective of the habitability of the islands, these have undergone variable changes over time. Smaller islands (<1 km2), such as Bitra (0.17 km2), Suheli (0.38 km2) and Thinnakara (0.52 km2) have undergone up to 30% lateral migration while being relatively stable in size. However, the larger islands, i.e., Androth and Minicoy, have undergone net land loss. Thus, sediment production and redistribution of sediments impact the evolution of the islands in decadal timescales which are in turn affected by the ocean parameters over the Northern Indian Ocean (especially the Arabian Sea) as well as the monsoon.
6.1 ENSO and IOD impact
Besides influencing the region’s hydrodynamics, ocean-atmospheric interactions (ENSO and IOD) affect sediment supply (new sediment production + redistribution of sediments). El-Nino events lead to widespread coral mortality and low recovery of the corals (DeCarlo et al., 2017). Further, anomalous ocean warming also drives the monsoon, affecting the hydrodynamics (wind and wave amplitudes and velocities) surrounding Lakshadweep. However, the variations in amplitudes of the IOD events impact the sea surface temperatures and intensities of the Indian monsoon to a greater extent as compared to ENSO (Ashok and Saji, 2007). The IOD serves as the modulator of the Indian monsoon and based on the amplitude of IOD, the monsoon circulation induced by ENSO is either supported or countered (Ashok et al., 2001; Cherchi and Navarra, 2013). With ENSO and IOD events touted to become more severe and frequent due to climate change (Cai et al., 2009, Cai et al., 2021), this could lead to anomalous ocean warming (Abram et al., 2008; Roxy et al., 2014; Swapna et al., 2014). The strengthening of the ENSO and IOD is predicted to result in higher rainfall for India (Todmal et al., 2022). In this study, it has been observed that the migration of sediments for the small islands are determined by the amplitude of the ENSO and IOD events. Extensive migration occurs during phases of high variability (severity) in the ocean-atmospheric interactions. The strengthening of the monsoons due to human-induced climate change, combined with ocean-atmospheric interactions, can lead to both accretion and erosion in the future, particularly for the large islands.
6.2 Indian monsoon and cyclones
Satellite geomorphological studies of the islands suggest the role of the monsoon in controlling shoreline behavior. Studies have shown an increase in the probable number of days with heavy rainfall and cyclonic storms have increased after 2011 in the Arabian Sea (Hazard Atlas of India, Indian Meteorological Department (IMD), Pune). The frequency of days with heavy rainfall has increased from 3- 5 days before 2011 to 6 – 10 days after 2011, with the magnitude rising from 15.5 mm/day to 115.5 mm/day (Hazard Atlas of India, Indian Meteorological Department (IMD), Pune). Moreover, the monsoon is influenced by submarine volcanism, as evidenced in 2008, when the increased volcanic activity in the northwest of Lakshadweep led to anomalous rainfall over the islands (Akilan et al., 2023). The increase in island area for Minicoy (large island) during periods of strengthened monsoon implies that the monsoon is a potential tipping point for exacerbating the current trend in accretion by funneling the sand from the forereef/reef flats to the island; however, in few cases, it might also lead to erosion, for example, Androth, where the island is currently being eroded. For small islands (Bitra, Thinnakara, Bangaram, and Suheli), the monsoon could play a crucial role in sediment migration and redistribution.
Increasing storms have been observed since 2000 over the Northern Indian Ocean/Arabian Sea (Baburaj et al., 2020; Deshpande et al., 2021; Baburaj et al., 2022). The recent cyclone that wreaked havoc in the Lakshadweep Islands was the Okhi cyclone in 2017, wherein wind velocities of 160km/hr led to widespread coral mortality and redistribution of sediments (Riyas et al., 2019). Moreover, climate-change induced changes in sea surface temperatures have resulted in the increased probability of storms during the monsoon (Murakami et al., 2017). The cyclonic perturbations have also induced variations in sea surface temperatures, increasing the intensity of normal rainfall, especially during the SW monsoon (Baburaj et al., 2020), leading to large–scale redistribution of sediments. Moreover, storms and cyclones have led to extensive coral mortality in the islands (up to 100% for some species; Divan Patel et al., 2023), which can have significant impacts on sediment production (sediment supply). Sediment production in fore reef/reef flats determines the production rates and sediment supply to the island (Kench et al., 2022), with estimated rates of delivery of 0.1m3/m/yr to the islands in the Indo-Pacific coral islands (Ainesi et al., 2024). Modeling studies have indicated a decrease in sediment input from the reef flat to the islands (Bramante et al., 2020), which is potentially what is observed in the long-term trend for the larger islands (Minicoy and Androth) in the Lakshadweep as well. For the smaller islands, cyclones could induce large-scale redistribution of sediments, effectively eroding the islands. The results, thus, emphasize the role of both sediment production and redistribution to the islands being governed by the monsoon (and/or storms), which dictate shoreline morphology in decadal timescales.
6.3 Vulnerability of islands
6.3.1 Vulnerability index
Inspecting the eco-morphological attributes would suggest that size, sediment supply and response to environmental conditions (Fellowes et al., 2023) influence island morphology over time. This portends considerable risk to densely populated small islands wherein adequate mitigation strategies are necessary to combat land loss. Based on the vulnerability index that looks into the variables of robustness, i.e., island size, human disturbances, island elevation, shape and vegetation (Duvat et al., 2021a, Duvat et al., 2021b; Fellowes et al., 2023), low-risk islands have large areas, high maximum elevations (>2.5 m) and are vegetated. The mean tidal range should be less than 2 m with moderate wave heights (<1.5 m) and have low histories of tropical storms and marine heatwaves. Considering similar climatic conditions for all the islands in the Lakshadweep Archipelago (mean tidal range – 1 m; wave heights <1.5 m), the island’s vulnerability would be determined by its size and vegetation cover. Although almost all the islands are <1 km2 (high risk, as per Fellowes et al., 2023), the small islands largely display extensive migration of sediments, with overall stable shorelines over decadal timescales. However, widespread migration of sediments could entail potential social and resource access risks. Additionally, for the uninhabited islands (Suheli and Thinnakara) studied, the shoreline morphology has varied in decadal scales, irrespective of human activities (Figure 6). This implies greater control of natural and local factors in determining the island’s stability for smaller islands. The larger islands, exhibiting land loss are more at risk in terms of habitability. These islands have a high population and several infrastructure projects (Prakash et al., 2015). Consequently, these pose a high risk to habitability, even though the total variation in island areas is less than 5%.
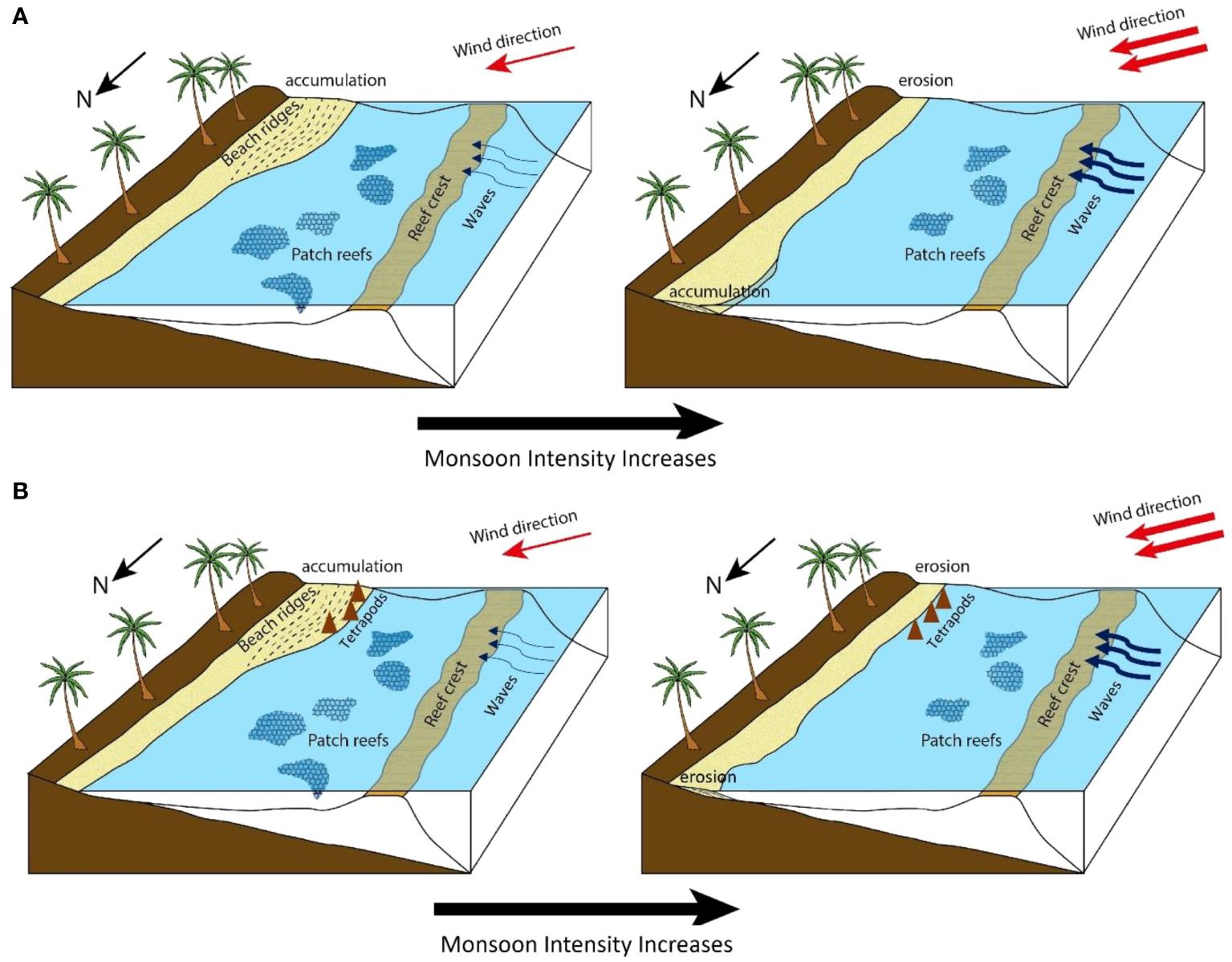
Figure 6 Schematic representation of future island morphology with increased rainfall intensities. The increase in monsoon intensities (both in terms of rainfall and cyclones) leads to the intensification of wind and wave velocities, depicted by arrow marks. (A) The shoreline morphological variations for smaller islands. As the wind and wave velocities intensify in the future and the coral abundance decreases, preferential erosion (south) and accumulation (north) could be observed in the islands. (B) For larger islands, greater monsoon intensities and reduced rates of sediment supply due to a decrease in coral abundance translated to erosion and land loss. Additionally, the tetrapods/breakwaters have had no significant impact in protecting the coastline.
Hence, the Lakshadweep Archipelago provides an excellent case study to understand the factors determining the stability of islands of similar size and distribution over decadal timescales. Local factors such as ocean-atmospheric interactions and the configuration/orientation of islands dictate the shoreline morphology. The strengthening of monsoons can lead to accretion (as seen in Minicoy) or erosion (Androth), depending on the configuration. Thus, this study highlights the importance of various local factors in determining island stability in decadal timescales for coral islands of similar size and distribution in the Indo-Pacific. Studies on decadal timescale variations can provide light on the regions requiring rapid protective measures and aid in adopting alternative strategies.
6.3.2 Manual coastal management structures and island vulnerability
Although both the uninhabited and inhabited islands depict variations in island area over decadal timescales, the large (incidentally, inhabited) islands have undergone net erosion over time. It has been observed that the coastal management structures have not had any significant impact in protecting the coastline of the islands in Lakshadweep. Tetrapods/breakwaters and hard concrete structures have been set up in the south of Bangaram and Minicoy and in the eastern and western parts of Androth in the early 2000s (local reports). However, these regions exhibit extensive erosion (Figure 2), thereby indicating the ineffectiveness of these coastal management structures. In Bangaram and Androth, unconsolidated sand has been scoured away from behind the structures (Figure 7). This demonstrates the necessity to take into consideration the local hydrodynamics and sediment migration trends to adequately adopt sustainable, practical coastal management structures. With increased intensities of rainfall and storms, the present coastal management structures could prove ineffectual in protecting the coastlines (Figure 6), particularly for Androth wherein local natural factors (configuration) play against island accretion in decadal timescales. Therefore, the adoption of proper coastal management strategies entails the understanding of the sediment migration or erosion/accretion trends and the underlying mechanisms influencing change. In several Indo-Pacific atolls, seawalls and other coastal engineering structures (tetrapods/breakwaters) have led to the exacerbation of coastline erosion (Nunn et al., 2021). This could mean scrutinizing the variations in shoreline morphology and adopting adequate coastal management practices that do not compromise on the existing geomorphology and stability of the coral islands.
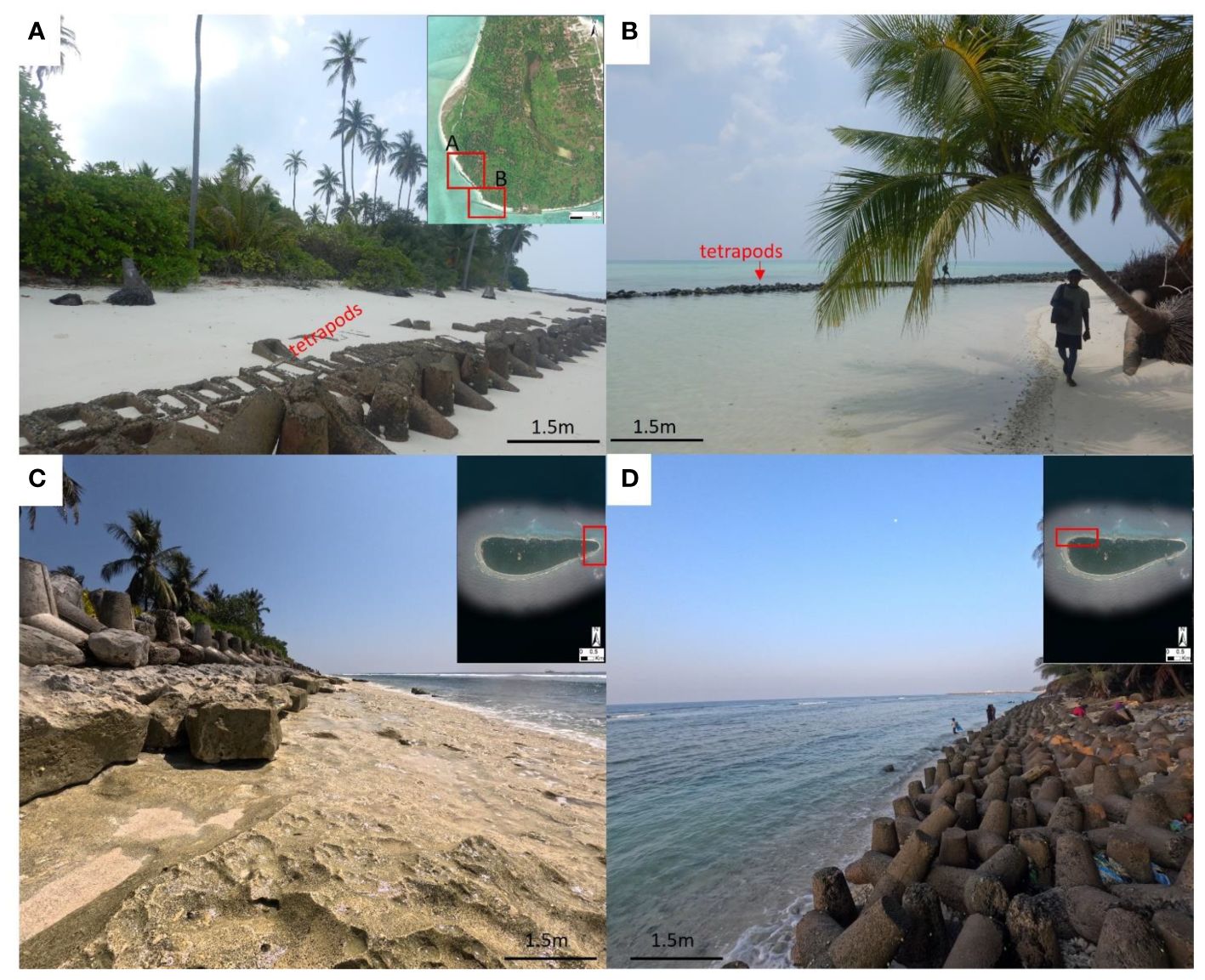
Figure 7 Field photographs from Bangaram and Androth depicting the coastal management structures (tetrapods/breakwaters and concrete blocks) put in place. Panels (A, B) show the coastal management structures in the south of Bangaram (location in inset map), while (C, D) correspond to the structures placed in Androth to combat coastal erosion. Note in panel (B) the structures are not along the present-day coastline, indicating extensive erosion and the futility of placing these structures.
6.3.3 Sea level and island morphology - the way forward
The current sea-level rise from 2006 is about 4.2 ± 0.4 mm/year (Fox-Temper et al., 2021), with rates projected to increase in the coming decades. It has also been predicted that a rise in sea levels could inundate the islands, albeit not as much as the coral islands in the Pacific (Jennath et al., 2021). Predictions have indicated overtopping at 0.1 to 0.4 m sea level rise, with variabilities attributed to local characteristics (Beetham and Kench, 2018). However, local factors can imprint over overtopping due to sea level rise. Consequently, these factors need to be taken into consideration while assessing island stability.
Additionally, shoreline changes have also been shown to substantially affect groundwater availability, crucial to coral island habitability (Duvat, 2018; Carruthers et al., 2023). Studies from Maldives have shown that accreting islands could lead to an increase in the volume of freshwater lens, although this is dependent on other factors such as island shape and sea level rise (Carruthers et al., 2023). More intensive studies on the freshwater lens need to be looked upon in the Lakshadweep Islands to understand the relationship between shoreline morphology and the volume of the freshwater lens. This, in turn, can provide crucial inputs to the island’s vulnerability in terms of habitability.
7 Conclusion
The study compiles the decadal timescale variations in the islands of Lakshadweep to discern the governing factors of change and the overall stability of the islands in response to climate change. The salient points have been summarized as follows:
1. The small islands (area <1 km2) undergo sediment erosion, usually along the southern edge and sediment migration and accumulation northwards; however, overall, their size remains consistently stable. In contrast, the larger islands (area >4 km2) are undergoing net erosion over time.
2. All the sediments collected along the Bangaram shoreline are unconsolidated bio-detrital grainstone, with grain size ranging from 2 – 0.5 mm (38%), 0.5 – 0.25 mm (49%) and 0.25 – 0.125 mm (10%). The dominant components include coral fragments (49%), molluscs (34%) and forams (9%). With ongoing ocean warming adversely impacting coral growth, sediment production will be determined by the abundance and sediment production rates of molluscs. This would entail erosion/accretion of the islands in the future.
3. The ocean-atmospheric interactions, particularly the amplitudes of El-Nino Southern Oscillation (ENSO) and Indian Ocean Dipole (IOD), determine the migration/redistribution of sediments in smaller islands. For the larger islands, the strengthening of monsoons due to the ocean-atmospheric interaction has led to accretion in Minicoy and erosion in Androth. This indicates that other factors such as configuration, orientation, and accommodation (space) of islands are also important in determining island stability. The larger islands show erosion over time and variations in monsoon can either lead to erosion or accretion in these large islands.
4. Although small islands do not depict land loss over time, the migration of sediments possesses considerable risk in terms of societal and resource risks. Although larger islands show less than 5% variation in area over time, these are eroding over time, posing significant risks to human habitability and resource access.
The Lakshadweep Archipelago also provides an analogue for island habitability risk in the Indian Ocean. This study emphasizes the governing role of ocean-atmospheric interactions in determining a host of local factors, such as sea surface temperatures, sediment supply and hydrodynamics and configuration of the islands. These site-specific factors, in turn, affect island stability. Thus, the interplay of global v/s local factors must be considered to discern decadal timescale variations in island morphology.
Data availability statement
The original contributions presented in the study are included in the article/Supplementary Material. Further inquiries can be directed to the corresponding author.
Author contributions
SM: Writing – original draft, Investigation, Formal analysis, Data curation. PK: Writing – review & editing, Supervision, Resources, Project administration, Methodology, Funding acquisition, Conceptualization. SKM: Writing – review & editing, Formal analysis, Data curation. SJ: Visualization, Writing – review & editing, Supervision.
Funding
The author(s) declare financial support was received for the research, authorship, and/or publication of this article. The authors would like to acknowledge financial support from IIT Gandhinagar grant number: IP/IITGN/ES/PK/2122/31 and SCIENCE & ENGINEERING RESEARCH BOARD (SERB) project number SRG/2022/ 000514 for undertaking this research. Without their support this research wouldn't have been possible.
Acknowledgments
We thank the Ministry of Forests and Environment, UT of Lakshadweep, the Department of Science and Technology, UT of Lakshadweep, Dr. Idrees Babu and Divetime, Agatti, for all the technical support and logistics for the fieldwork. Continental Labs is acknowledged for the preparation of thin-section samples.
Conflict of interest
The authors declare that the research was conducted in the absence of any commercial or financial relationships that could be construed as a potential conflict of interest.
Publisher’s note
All claims expressed in this article are solely those of the authors and do not necessarily represent those of their affiliated organizations, or those of the publisher, the editors and the reviewers. Any product that may be evaluated in this article, or claim that may be made by its manufacturer, is not guaranteed or endorsed by the publisher.
Supplementary material
The Supplementary Material for this article can be found online at: https://www.frontiersin.org/articles/10.3389/fmars.2024.1431655/full#supplementary-material
References
Abram N. J., Gagan M. K., Cole J. E., Hantoro W. S., Mudelsee M. (2008). Recent intensification of tropical climate variability in the Indian Ocean. Nat. Geosci. 1, 849–853. doi: 10.1038/ngeo357
Ainési B., Masselink G., Kench P. (2024). Meta-study of carbonate sediment delivery rates to indo-pacific coral reef islands. Geophysical Res. Lett. 51, e2023GL105610. doi: 10.1029/2023GL105610
Akilan A., Abdul Azeez K. K., Schuh H., Balaji S., Teja G. K., Satyanaryana H. V. S. (2023). Possible link between the rainfall and ridge seismicity from episodic GPS data at Lakshadweep islands in the Arabian Sea. Pure Appl. Geophysics 180, 4355–4370. doi: 10.1007/s00024-023-03380-1
Almar R., Ranasinghe R., Bergsma E. W., Diaz H., Melet A., Papa F., et al. (2021). A global analysis of extreme coastal water levels with implications for potential coastal overtopping. Nat. Commun. 12, 1–9. doi: 10.1038/s41467-021-24008-9
Amores A., Marcos M., Le Cozannet G., Hinkel J. (2022). Coastal flooding and mean sea-level rise allowances in atoll island. Sci. Rep. 12, 1281. doi: 10.1038/s41598-022-05329-1
Anthony K. R., Kline D. I., Diaz-Pulido G., Dove S., Hoegh-Guldberg O. (2008). Ocean acidification causes bleaching and productivity loss in coral reef builders. Proc. Natl. Acad. Sci. 105 (45), 17442–17446. doi: 10.1073/pnas.0804478105
Arthur R. (2000). Coral bleaching and mortality in three Indian reef regions during an El Niño southern oscillation event. Curr. Sci. 79, 1723–1729.
Arthur R., Done T. J., Marsh H., Harriott V. (2006). Local processes strongly influence post-bleaching benthic recovery in the Lakshadweep Islands. Coral Reefs 25, 427–440. doi: 10.1007/s00338-006-0127-4
Ashalatha B., Subrahmanyam C., Singh R. (1991). Origin and compensation of Chagos-Laccadive ridge, Indian ocean, from admittance analysis of gravity and bathymetry data. Earth Planetary Sci. Lett. 105, 47–54. doi: 10.1016/0012-821X(91)90119-3
Ashok K., Guan Z., Yamagata T. (2001). Impact of the Indian Ocean dipole on the relationship between the Indian monsoon rainfall and ENSO. Geophysical Res. Lett. 28, 4499–4502. doi: 10.1029/2001GL013294
Ashok K., Saji N. H. (2007). On the impacts of ENSO and Indian Ocean dipole events on sub-regional Indian summer monsoon rainfall. Natural Hazards 42, 273–285. doi: 10.1007/s11069-006-9091-0
Australian Government-Bureau of Meteorology Indian Ocean climate influences. Available at: http://www.bom.gov.au/climate/iod/.
Baburaj P. P., Abhilash S., Mohankumar K., Sahai A. K. (2020). On the Epochal Variability in the Frequency of Cyclones during the Pre-Onset and Onset Phases of the Monsoon over the North Indian Ocean. Adv. Atmos. Sci. 37, 634–651. doi: 10.1007/s00376-020-9070-5
Baburaj P. P., Abhilash S., Nirmal C. A., Sreenath A. V., Mohankumar K., Sahai A. K. (2022). Increasing incidence of Arabian Sea cyclones during the monsoon onset phase: Its impact on the robustness and advancement of Indian summer monsoon. Atmospheric Res. 267, 105915. doi: 10.1016/j.atmosres.2021.105915
Barry S., Cowell P., Woodroffe C. (2007). A morphodynamic model of reef-island development on atolls. Sedimentary Geology 197, 47–63. doi: 10.1016/j.sedgeo.2006.08.006
Beetham E., Kench P. S. (2018). Predicting wave overtopping thresholds on coral reef-island shorelines with future sea-level rise. Nat. Commun. 9, 1–8. doi: 10.1038/s41467-018-06550-1
Beetham E., Kench P. S., Popinet S. (2017). Future reef growth can mitigate physical impacts of sea-level rise on atoll islands. Earth’s Future 5, 1002–1014. doi: 10.1002/2017EF000589
Belopolsky A., Droxler A. (2003). Imaging Tertiary carbonate system—the Maldives, Indian Ocean: Insights into carbonate sequence interpretation. Leading Edge 22, 646–652. doi: 10.1190/1.1599690
Bramante J. F., Ashton A. D., Storlazzi C. D., Cheriton O. M., Donnelly J. P. (2020). Sea level rise will drive divergent sediment transport patterns on fore reefs and reef flats, potentially causing erosion on atoll islands. J. Geophysical Research: Earth Surface 125, e2019JF005446. doi: 10.1029/2019JF005446
Browne N. K., Cuttler M. V., Moon K., Morgan K. M., Ross C. L., Castro-Sanguino C., et al. (2021). Predicting responses of geo-ecological carbonate reef systems to climate change: a conceptual model and review. Oceanography Mar. Biol. 59, 229–370. doi: 10.1201/9781003138846-4
Cai W., Santoso A., Collins M., Dewitte B., Karamperidou C., Kug J., et al. (2021). Changing El Niño–Southern Oscillation in a warming climate. Nat. Rev. Earth Environ. 2, 628–644. doi: 10.1038/s43017-021-00199-z
Cai W., Sullivan A., Cowan T. (2009). Climate change contributes to more frequent consecutive positive Indian Ocean Dipole events. Geophysical Res. Lett. 36 (23). doi: 10.1029/2009GL040163
Carruthers L., East H. K., Ersek V., Suggitt A. J., Campbell M., Lee K. J., et al. (2023). Coral reef island shoreline change and the dynamic response of the freshwater lens, Huvadhoo Atoll, Maldives. Front. Mar. Sci. 10, 1070217. doi: 10.3389/fmars.2023.1070217
Carter R. W. G., Woodroffe C. D. (1995). Coastal evolution: Late Quaternary shoreline morphodynamics. Eds. Carter R. W. G., Woodroffe C. D. (Cambridge University Press Cambridge: New York). doi: 10.1017/CBO9780511564420
Cherchi A., Navarra A. (2013). Influence of ENSO and of the Indian Ocean Dipole on the Indian summer monsoon variability. Climate dynamics 41, 81–103. doi: 10.1007/s00382-012-1602-y
COBE Sea Surface Temperature. Available online at: https://psl.noaa.gov/data/gridded/data.cobe.html.
Das R. R., Sreeraj C. R., Mohan G., Simon N. T., Ramachandran P., Ramachandran R., et al. (2023). Evidence of coral diseases, phase shift, and stressors in the atolls of Lakshadweep islands, Arabian Sea—with geographical notes on their occurrence within the Indian EEZ and Contiguous International Waters. Diversity 15, 382. doi: 10.3390/d15030382
David C. G., Schlurmann T. (2020). Hydrodynamic drivers and morphological responses on small coral islands—The thoondu spit on Fuvahmulah, the Maldives. Front. Mar. Sci. 7. doi: 10.3389/fmars.2020.538675
Dawson J. L. (2021). Multi-decadal shoreline morphodynamics of a shelf-edge reef island, Great Barrier Reef: Implications for future island persistence. Geomorphology 392, 107920. doi: 10.1016/j.geomorph.2021.107920
DeCarlo T. M., Cohen A. L., Wong G. T., Davis K. A., Lohmann P., Soong K. (2017). Mass coral mortality under local amplification of 2 °C ocean warming. Sci. Rep. 7, 1–9. doi: 10.1038/srep44586
Deshpande M., Singh V. K., Ganadhi M. K., Roxy M. K., Emmanuel R., Kumar U. (2021). Changing status of tropical cyclones over the north Indian Ocean. Clim Dyn 57, 3545–3567. doi: 10.1007/s00382-021-05880-z
Dinakaran S. V., Alluri K. R., Joseph K. J., Murthy M. R., Venkatesan R. (2022). Modelling and simulation of extreme wave heights around agatti island of lakshadweep, west coast of India. Front. Built Environ. 8, 991768. doi: 10.3389/fbuil.2022.991768
Divan Patel F., Pinto W., Dey M., Alcoverro T., Arthur R. (2023). Carbonate budgets in Lakshadweep Archipelago bear the signature of local impacts and global climate disturbances. Coral Reefs 42, 729–742. doi: 10.1007/s00338-023-02374-8
Droxler A. W., Jorry S. J. (2020). The origin of modern atolls: challenging Darwin’s deeply ingrained theory. Annu. Rev. Mar. Sci. 13, 537–573. doi: 10.1146/annurev-marine-122414-034137
Duncan R. A., Hargraves R. B. (1990). “40Ar/39Ar geochronology of basement rocks from the Mascarene Plateau, the Chagos Bank, and the Maldives Ridge,” in Proceedings of the Ocean Drilling Program, Scientific Results, vol. 11543–51. (Ocean Drill. Program, College Station, TX).
Dunham R. J. (1962). Classification of carbonate rocks according to depositional textures. In: Classification of Carbonate Rocks—A Symposium. (Ed. Ham W. E.) (Tulsa, Oklahoma, USA: AAPG Memoir) 1, 108–121.
Duvat V. K. (2018). A global assessment of atoll island planform changes over the past decades. Wiley Interdiscip. Reviews: Climate Change 10, e557. doi: 10.1002/wcc.557
Duvat V. K., Magnan A. K., Perry C. T., Spencer T., Bell J. D., C. Wabnitz C. C., et al. (2021a). Risks to future atoll habitability from climate-driven environmental changes. Wiley Interdiscip. Reviews: Climate Change 12, e700. doi: 10.1002/wcc.700
Duvat V. K., Volto N., Costa S., Maquaire O., Pignon-Mussaud C., Davidson R. (2021b). Assessing atoll island physical robustness: application to Rangiroa Atoll, French Polynesia. Geomorphology 390, 107871. doi: 10.1016/j.geomorph.2021.107871
El Niño and La Niña (El Niño-Southern Oscillation). NOAA Climate.gov. Available at: http://www.climate.gov/enso.
Fellowes T., Vila-Concejo A., Bruce E., Byrne M., Baker E. (2023). Decadal evolution of coral islands in A changing ocean and climate. Coast. Eng. Proc. 37. doi: 10.9753/icce.v37.management.124
Ferrario F., Beck M. W., Storlazzi C. D., Micheli F., Shepard C. C., Airoldi L. (2014). The effectiveness of coral reefs for coastal hazard risk reduction and adaptation. Nat. Commun. 5, 1–9. doi: 10.1038/ncomms4794
Fox-Kemper B., Hewitt H. T., Xiao C., Aðalgeirsdóttir G., Drijfhout S. S., Edwards T. L., et al. (2021). “Ocean, cryosphere and sea level change. In climate change 2021: the physical science basis,” in Contribution of Working Group I to the Sixth Assessment Report of the Intergovernmental Panel on Climate Change. Eds. Masson-Delmotte V., Zhai P., Pirani A., Connors S. L., Péan C., Berger S., Caud N., Chen Y., Goldfarb L., Gomis M. I., Huang M., Leitzell K., Lonnoy E., Matthews J. B. R., Maycock T. K., Waterfield T., Yelekçi O., Yu R., Zhou B. (Cambridge University Press, Cambridge, United Kingdom and New York, NY, USA), 1211–1362. doi: 10.1017/9781009157896.011
Government of India: UT of Lakshadweep. Available online at: https://lakshadweep.gov.in/.
Hallock P. (1981). Production of carbonate sediments by selected large benthic foraminifera on two pacific coral reefs. J. Sediment. Petrol. 51, 467–474. doi: 10.1306/212F7CB1-2B24-11D7-8648000102C1865D
Hameed S., Yamagata T. (2003). Possible impacts of Indian Ocean Dipole Mode events on global climate. Clim. Res. 25, 151–169. doi: 10.3354/cr025151
Hamlington B. D., Cheon S. H., Piecuch C. G., Karnauskas K. B., Thompson P. R., Kim Y., et al. (2019). The dominant global modes of recent internal sea level variability. J. Geophysical Research: Oceans 124, 2750–2768. doi: 10.1029/2018JC014635
Hazard Atlas of India. Available online at: https://imdpune.gov.in/hazardatlas/index.html.
Holdaway A., Ford M., Owen S. (2021). Global-scale changes in the area of atoll islands during the 21st century. Anthropocene 33, 100282. doi: 10.1016/j.ancene.2021.100282
Homewood P., Vahrenkamp V. C., Mettraux M., Mattner J., Vlaswinkel B., Droste H. J., et al. (2007). Bar Al Hikman: a modern carbonate and outcrop analogue in Oman for Middle East Cretaceous fields. First Break 25, 55–61. doi: 10.3997/1365-2397.25.1113.27711
Hosking P. L. (1991). Geomorphology of reef islands and atoll motu in Tuvalu. Pac J. Nat. Sci. 11, 167–189.
Jennath A., Krishnan A., Paul S. K., Bhaskaran P. K. (2021). Climate projections of sea level rise and associated coastal inundation in atoll islands: Case of Lakshadweep Islands in the Arabian Sea. Regional Stud. Mar. Sci. 44, 101793. doi: 10.1016/j.rsma.2021.101793
Jinoj T. P., Bonthu S., Robin R. S., Babu K. K., Purvaja R., Ramesh R. (2020). Nearshore sediment dynamics of Kavaratti Island, Lakshadweep archipelago using integrated modelling system. Indian J. Geo-Marine Sci. 49, 845–857.
Jyoti J., Swapna P., Krishnan R. (2023). North Indian Ocean sea level rise in the past and future: the role of climate change and variability. Global Planetary Change 228, 104205. doi: 10.1016/j.gloplacha.2023.104205
Kench P. S., Beetham E. P., Turner T., Morgan K. M., Owen S. D., McLean R. F. (2022). Sustained coral reef growth in the critical wave dissipation zone of a Maldivian atoll. Commun. Earth Environ. 3, 9. doi: 10.1038/s43247-021-00338-w
Kench P. S., Ford M. R., Owen S. D. (2018). Patterns of island change and persistence offer alternate adaptation pathways for atoll nations. Nat. Commun. 9, 1–7. doi: 10.1038/s41467-018-02954-1
Kench P. S., Mann T. (2017). Reef island evolution and dynamics: insights from the Indian and Pacific oceans and perspectives for the spermonde archipelago. Front. Mar. Sci. 4. doi: 10.3389/fmars.2017.00145
Kench P., Nichol S. (2005). New Model of reef-island evolution: Maldives, Indian Ocean. Geology 33, 145–148. doi: 10.1130/G21066.1
Kumar P., Hamlington B., Cheon H., Han W., Thompson P. (2020). 20th century multivariate Indian ocean regional sea level reconstruction. J. Geophysical Research: Oceans 125, e2020JC016270. doi: 10.1029/2020JC016270
Kumar K. K., Rajagopalan B., Hoerling M., Bates G., Cane M. (2006). Unraveling the mystery of Indian monsoon failure during El Niño. Science 314, 115–119. doi: 10.1126/science.1131152
Liang C. Y., Kench P. S., Ford M. R., East H. K. (2022). Lagoonal reef island formation in Huvadhoo atoll, Maldives, highlights marked temporal variations in island building across the archipelago. Geomorphology 414, 108395. doi: 10.1016/j.geomorph.2022.108395
Lokier S. W., Fiorini F. (2016). Temporal evolution of a carbonate coastal system, Abu Dhabi, United Arab Emirates. Mar. Geology 381, 102–113. doi: 10.1016/j.margeo.2016.09.001
Magnan A. K., Garschagen M., Gattuso J., Hay J., Hilmi N., Holland E., et al. (2019). “Low - lying islands and coasts,” in IPCC Special Report on the Ocean and Cryosphere in a Changing Climate. Eds. Pörtner H.-O., Roberts D. C., Masson-Delmotte V., Zhai P., Tignor M., Poloczanska E., Mintenbeck K., Alegria A., Nicolai M., Okem A., Petzold J., Rama B., Weyer N. M. (Cambridge University Press, Cambridge, UK and New York, NY, USA).
Mallik T. K. (2017). Coral atolls of Lakshadweep. Arab. Sea Indian ocean. MOJ Ecol. Environ. Sci. 2, 68–83. doi: 10.15406/mojes.2017.02.00021
McClanahan T. R., Darling E. S., Maina J. M., Muthiga N. A., Jupiter S. D., Arthur R., et al. (2019). Temperature patterns and mechanisms influencing coral bleaching during the 2016 El Niño. Nat. Climate Change 9, 845–851. doi: 10.1038/s41558-019-0576-8
McLean R. F., Kench P. S. (2015). Destruction or persistence of coral atoll islands in the face of 20th and 21st century sea-level rise? WIREs Climate Change 6, 445–463. doi: 10.1002/wcc.350
Mimura N. (1999). Vulnerability of island countries in the South Pacific to sea level rise and climate change. Clim. Res. 12, 137–143. doi: 10.3354/cr012137
Mukherjee P., Ramakrishnan B. (2022). On the understanding of very severe cyclone storm Ockhi with the WRF-ARW model. Environ. Res. Clim. 1, 015002. doi: 10.1088/2752-5295/ac6adb
Murakami H., Vecchi G. A., Underwood S. (2017). Increasing frequency of extremely severe cyclonic storms over the Arabian Sea. Nat. Climate Change 7, 885–889. doi: 10.1038/s41558-017-0008-6
Narayan G. R., Reymond C. E., Stuhr M., Doo S., Schmidt C., Mann T., et al. (2021). Response of large benthic foraminifera to climate and local changes: implications for future carbonate production. Sedimentology 69 (1), 121–161. doi: 10.1111/sed.12858
Neumann B., Vafeidis A. T., Zimmermann J., Nicholls R. J. (2015). Future coastal population growth and exposure to sea-level rise and coastal flooding - A global assessment. PLOSONE 10, e0118571. doi: 10.1371/journal.pone.0118571
Nicholls R. J., Wong P. P., Burkett V. R., Codignotto J. O., Hay J. E., McLean R. F., et al. (2007). “Coastal systems and low-lying areas.” in Climate change 2007: impacts, adaptation and vulnerability. Contribution of working group II to the fourth assessment report of the intergovernmental panel on climate change, eds. Parry M. L., Canziani O. F., Palutikof J. P., Linden der van P. J., Hanson C. E. (Cambridge, UnitedKingdom: Cambridge University Press), 315–356.
NOAA, National Weather Services. Available online at: https://www.cpc.ncep.noaa.gov/data/indices/.
Nunn P. D., Klöck C., Duvat V. (2021). Seawalls as maladaptations along island coasts. Ocean Coast. Manage. 205, 105554. doi: 10.1016/j.ocecoaman.2021.105554
Ocean Surface Topography from Space. Available online at: https://sealevel.jpl.nasa.gov/overlay-iod/.
Open Government Data (OGD) Platform India. Available online at: https://data.gov.in/.
Oppenheimer M., Glavovic B. C., Hinkel J., van de Wal R., Magnan A. K., Abd-EIgawad A., et al. (2019). “Sea level rise and implications for low-lying islands, coasts and communities” in IPCC special report on the ocean and cryosphere in a changing climate, eds. Pö̈rtner H.-O. D., Roberts C., Masson-Delmotte V., Zhai P., Tignor M., Poloczanska E., Mintenbeck K., Alegría A., Nicolai M., Okem A., Petzold J., Rama B., N. M., Weyer , et al. (Cambridge, United Kingdom and New York, NY, United States: Cambridge University Press), 321–445. doi: 10.1017/9781009157964.006
Panchang R., N L. V., Padate V., Nigam R. (2023). Environmental monitoring of the Kavaratti lagoon, Lakshadweep Islands, Arabian Sea using larger foraminiferal distribution. J. Palaeontological Soc. India 68, 302–308. doi: 10.1177/05529360231224496
Perry C. T., Kench P. S., Smithers S. G., Riegl B., Yamano H. (2011). Implications of reef ecosystem change for the stability and maintenance of coral reef islands. Global Change Biol. 17, 3679–3696. doi: 10.1111/j.1365-2486.2011.02523.x
Petrovic A., Fuentes M. A., Putri I., Yahaya L. N., Khanna P., Purkis S. J., et al. (2022). Holocene sediment distribution in the Al Wajh platform lagoon (northern Red Sea, Saudi Arabia), a modern analogue for large rift basin carbonate platforms. Sedimentology 69, 1365–1398. doi: 10.1111/sed.12955
Prakash T. N., Nair L. S., Shahul Hameed T. S. (2015). Geomorphology and Physical Oceanography of the Lakshadweep Coral Islands in the Indian Ocean (New York, USA: Springer Briefs in Earth Sci). doi: 10.1007/978-3-319-12367-7
Prakash T. N., Nair L. S., Varghese T. I. (2014). Shoreline changes and reef strengthening at Kavaratti island in Lakshadweep Archipelago - a case study. Indian J. Mar. Sci. 43.
Prat S., Jorry S. J., Jouet G., Camoin G., Vella C., Le Roy P., et al. (2016). Geomorphology and sedimentology of a modern isolated carbonate platform: The Glorieuses archipelago, SW Indian Ocean. Mar. Geology 380, 272–283. doi: 10.1016/j.margeo.2016.04.009
Rahaman W., Tarique M., Fousiya A. A., Prabhat P., Achyuthan H. (2022). Tracing impact of El Niño Southern Oscillation on coastal hydrology using coral 87Sr/86Sr record from Lakshadweep, South-Eastern Arabian Sea. Sci. Total Environ. 843, 157035. doi: 10.1016/j.scitotenv.2022.157035
Rankey E. C. (2011). Nature and stability of atoll island shorelines: Gilbert Island chain, Kiribati, equatorial Pacific. Sedimentology 58, 1831–1859. doi: 10.1111/j.1365-3091.2011.01241.x
Rankey E. C., Uriam T., Perez M. (2023). Spatio-temporal dynamics of sediment transport pathways: sand apron bars and islands of Tokelau and Kiribati, Central Pacific. Earth Science Syst. Soc. 3, 10077. doi: 10.3389/esss.2023.10077
Ratna S. B., Cherchi A., Osborn T. J., Joshi M., Uppara U. (2021). The extreme positive Indian Ocean dipole of 2019 and associated Indian summer monsoon rainfall response. Geophysical Res. Lett. 48, e2020GL091497. doi: 10.1029/2020GL091497
Riyas C., Idreesbabu K., Marimuthu N., Sureshkumar S. (2019). Impact of the tropical cyclone Ockhi on ecological and geomorphological structures of the small low-lying Islands in the Central Indian Ocean. Regional Stud. Mar. Sci. 33, 100963. doi: 10.1016/j.rsma.2019.100963
Rodolfo-Metalpa R., Houlbrèque F., Tambutté É., Boisson F., Baggini C., Patti F. P., et al. (2011). Coral and mollusc resistance to ocean acidification adversely affected by warming. Nat. Climate Change 1, 308–312. doi: 10.1038/nclimate1200
Roxy M. K., Ritika K., Terray P., Masson S. (2014). The curious case of Indian ocean warming. J. Clim. 27, 8501–8509. doi: 10.1175/JCLI-D-14-00471.1
Roy P., Connell J. (1989). Greenhouse: the impact of sea level rise on low coral islands in the South Pacific (Sydney: Research Institute for Asia and the Pacific).
Roy P., Connell J. (1991). Climatic change and the future of atoll states. J. Coast. Res. 7, 1057–1075.
Sanap S. D., Mohapatra M., Ali M. M., Priya P., Varaprasad D. (2020). On the dynamics of cyclogenesis, rapid intensification and recurvature of the very severe cyclonic storm, Ockhi. J. Earth Syst. Sci. 129, 194. doi: 10.1007/s12040-020-01457-2
Scheffers A., Engel M., Scheffers S., Squire P., Kelletat D. (2011). Beach ridge systems – archives for Holocene coastal events? Prog. Phys. Geogr: Earth Environ. 36 (1), 5–37. doi: 10.1177/0309133311419549
Sein D. V., Martyanov S. D., Dvornikov A. Y., Cabos W., Ryabchenko V. A., Mishra A. K., et al. (2024). Future climate change in the Northern Indian Ocean as simulated with a high-resolution regional earth system model. Clim Dyn 62, 911–932. doi: 10.1007/s00382-023-06939-9
Shah-Hosseini M., Ghanavati E., Morhange C., Naderi Beni A., Lahijani H. A., Hamzeh M. A. (2018). The evolution of Chabahar beach ridge system in SE Iran in response to Holocene relative sea level changes. Geomorphology 318, 139–147. doi: 10.1016/j.geomorph.2018.06.009
Shimozono T., Shibata R., Onaka S., Mori T., Awazu Y. (2022). Variability of shoreline water levels on an atoll in the Maldives. J. Geophysical Research: Oceans 127, e2021JC017880. doi: 10.1029/2021JC017880
Suganya G. M., Deepika B., Madhumitha R., Rajakumari S., Purvaja R., Ramesh R. (2019). Planform Island change assessment for inhabited Lakshadweep Islands. Natural Hazards 98, 735. doi: 10.1007/s11069-019-03727-9
Swapna P., Krishnan R., Wallace J. M. (2014). Indian Ocean and monsoon coupled interactions in a warming environment. Clim. Dyn. 42, 2439–2454. doi: 10.1007/s00382-013-1787-8
Talavera L., Webster J. M., Smith C., Duce S., Fellowes T. E., Salles T., et al. (2020). Morphodynamic controls for growth and evolution of a rubble coral island. Remote Sens. 13, 1582. doi: 10.3390/rs13081582
Tamura T. (2012). Beach ridges and prograded beach deposits as palaeoenvironment records. Earth-Sci. Rev. 114, 279–297. doi: 10.1016/j.earscirev.2012.06.004
Todmal R. S., Koteswara Rao K., Ingle S., Korade M. S. (2022). Impact of Southern Oscillation and Indian Ocean Dipole on rainfall variability over India: trends and interlinkages during 1871–2017. Meteorology Atmospheric Phys. 134, 101. doi: 10.1007/s00703-022-00936-z
Trenberth K. E. (1997). The definition of El Niño. Bull. Am. Meteorological Soc. 78, 2771–2777. doi: 10.1175/1520-0477(1997)078<2771:TDOENO>2.0.CO;2
Ummenhofer C., Sen Gupta A., Li Y., Taschetto A., England M. (2011). Multi-decadal modulation of the El Nĩno-Indian monsoon relationship by Indian Ocean variability. Env. Res. Lett. 6. doi: 10.1088/1748-9326/6/3/034006
WEB Cyclone ATLAS::AboutEAtlas. Available online at: http://14.139.191.203/AboutEAtlas.aspx.
Webb A. P., Kench P. S. (2010). The dynamic response of reef islands to sea-level rise: Evidence from multi-decadal analysis of island change in the Central Pacific. Global Planetary Change 72, 234–246. doi: 10.1016/j.gloplacha.2010.05.003
Wentworth C. K. (1922). A scale of grade and class terms for clastic sediments. J. Geol. 30, 377–392. doi: 10.1086/622910
Woodroffe C. (2008). Reef-island topography and the vulnerability of atolls to sea-level rise. Glob. Planet. Change 62, 77–96. doi: 10.1016/j.gloplacha.2007.11.001
Keywords: Indian Ocean, Laccadive, coral reefs and islands, island vulnerability, climate change
Citation: Menon S, Khanna P, Misra SK and Jorry SJ (2024) Decadal timescale evolution of coral islands: insights from Lakshadweep Archipelago. Front. Mar. Sci. 11:1431655. doi: 10.3389/fmars.2024.1431655
Received: 12 May 2024; Accepted: 02 July 2024;
Published: 24 July 2024.
Edited by:
Wei Jiang, Guangxi University, ChinaReviewed by:
Weidong Zhu, Shanghai Ocean University, ChinaChuki Hongo, Wakayama Prefectural Nanki Kumano Geopark Center, Japan
Lucy Carruthers, East Carolina University, United States
Copyright © 2024 Menon, Khanna, Misra and Jorry. This is an open-access article distributed under the terms of the Creative Commons Attribution License (CC BY). The use, distribution or reproduction in other forums is permitted, provided the original author(s) and the copyright owner(s) are credited and that the original publication in this journal is cited, in accordance with accepted academic practice. No use, distribution or reproduction is permitted which does not comply with these terms.
*Correspondence: Pankaj Khanna, cGFua2FqLmtoYW5uYUBpaXRnbi5hYy5pbg==