- 1Coastal Oregon Marine Experiment Station, Oregon State University, Newport, OR, United States
- 2Fisheries, Wildlife and Conservations Sciences, Oregon State University, Corvallis, OR, United States
- 3Oceans, Hopkins Marine Station, Stanford University, Monterey, CA, United States
- 4Irish Whale and Dolphin Group, Ventry, County Kerry, Ireland
- 5School of Natural Sciences, Trinity College Dublin, Dublin, Ireland
- 6Irish Basking Shark Group, Ireland
Overlap between marine megafauna and maritime activities is a topic of global concern. Basking sharks (Cetorhinus maximus; CM) are listed as Globally Endangered under the IUCN, though reported sightings appear to be increasing in Ireland. While such trends in the region are welcome, increasing spatiotemporal overlap between CM and numerous water users poses an increased risk of boat strikes to the animals. To demonstrate the risk and impact of boat strikes on marine megafauna, we present camera-enabled animal-borne inertial measurement unit (IMU) data from a non-lethal boat strike on a CM within a proposed National Marine Park in Ireland. We tagged a ~7-m female CM in County Kerry, Ireland, which was struck by a boat ~6 h after tag deployment. Comparison of pre-strike data with 4 h of video and ~7.5 h of IMU data following the boat strike provides critical insight into the animal’s response. While the CM reacted momentarily with an increase in activity and swam to the seafloor, it quickly reduced its overall activity (i.e., overall dynamic body acceleration, tailbeat cycles, tailbeat amplitude, and vertical velocity) for the remainder of the deployment. Notably, the animal also ceased feeding for the duration of the video and headed towards deep offshore waters, which is in stark contrast to the pre-strike period where the animal was consistently observed feeding along the surface in shallow coastal water. This work provides insight into a CM’s response to acute injury and highlights the need for appropriate protections to mitigate risks for marine megafauna.
Introduction
Overlap between marine megafauna and maritime activities has long been, and is increasingly, a topic of concern, especially given the increase in maritime traffic and the continued declines of some species. It has been demonstrated that phenomena such as the formation of “marine roads” (i.e., shipping routes) pose significant risk to large surface-active marine megafauna (Pirotta et al., 2019). For example, Womersley et al. (2022) found that >90% of whale shark (Rhincodon typus) horizontal space use overlaps with persistent large vessel traffic and presents a significant mortality risk to these endangered sharks and Kite-Powell et al. (2007) estimated that collisions with large ships likely caused ~75% of known anthropogenic mortalities of critically endangered North Atlantic Right Whale (Eubalaena glacialis). While many studies focus on direct mortality caused by vessels, not all injuries cause direct mortality and non-lethal injuries can have significant short- and long-term consequences for animal physiology and behavior (Rennolds and Bely, 2023). For example, an initial flight response can negatively affect energy budgets if it either requires increased muscle recruitment or reduces energy intake (i.e., if the behavior results in the loss of foraging opportunities; Rennolds and Bely, 2023). Additionally, the injury itself could have longer-term negative impacts through a sustained altered behavioral state to compensate for the injury or due to tissue recovery and/or immune responses [see Rennolds and Bely (2023) for a review of sublethal mechanical injury biology]. There is also the possibility that injuries that appear non-lethal initially can eventually lead to mortality long after the event. It is therefore important to understand the responses and recovery periods of animals impacted by non-lethal strikes, especially given the high probability of strikes to marine megafauna that spend significant amounts of time near the surface. To date, most research efforts have relied on scarring quantity and recovery over time to understand these impacts (e.g., Bradford et al., 2009; Lester et al., 2020; Penketh et al., 2020), as behavioral observations directly surrounding a strike are incredibly rare. Here, we describe the immediate impacts of a non-lethal vessel strike on the behavior of a female basking shark (Cetorhinus maximus; CM) that was captured with a camera-enabled inertial measurement unit (IMU).
The basking shark is the world’s second largest fish and was historically abundant worldwide. Overfishing and culling efforts up until the late 20th century led to global declines (e.g., McFarlane et al., 2009; Finucci et al., 2021; McInturf et al., 2022) from which CMs have been slow to recover, leading to listing as Globally Endangered by the IUCN (Rigby et al., 2019). In Ireland, however, reported sightings appear to be increasing (Irish Basking Shark Group, personal communication); during certain months, individuals aggregate in shallow coastal hotspots, feeding at the surface on calanoid copepods (Calanus spp.; Baduini, 1995; Sims and Quayle, 1998; Miller et al., 2015) and potentially mating (Sims et al., 2022). Following public support, CM became the first fish to be listed under Section 23 of Ireland’s Wildlife Act of 1976 in 2022, rendering it illegal to injure or willfully interfere with the breeding or resting place of CM. Simultaneously, the Irish Basking Shark Group (IBSG), a local organization of researchers, conservationists, and educators, drafted a voluntary Code of Conduct for CM to limit anthropogenic impacts from human activities (i.e., swimming, drones, and vessel strikes), with the intention to guide binding protective legislation. Similar science-based plans for other megafauna such as whale sharks (Jones, 2021) and manta rays (Mobula alfredi; Murray et al., 2020) have been proposed and successfully adopted in other regions. In April 2024, Ireland announced the creation of its first National Marine Park, protecting 70,000 acres of land and sea, including seasonal CM habitat. However, though these collective actions represent a promising opportunity to protect the species in one of the few locations where CM sightings persist, specific management plans have yet to be developed let alone implemented; a lack of deliberate and enforced guidelines to ensure the intended protections can result in negative consequences for marine megafauna (for example, Legaspi et al., 2020). Thus, while an increase in both sightings and public interest are encouraging signs for the recovery of the species as a whole, the increasing spatiotemporal overlap between CM and numerous water users (e.g., fishing and commerce)—even the ecotourism industry itself—poses an increased risk to the animals without appropriate protections in place.
To demonstrate the risk and impact of boat strikes on marine megafauna, we present camera-enabled animal-borne IMU data from a non-lethal boat strike on a CM within the proposed National Marine Park in Ireland. We describe the immediate response and the first few hours of the subsequent recovery period of the animal. To our knowledge, these are the first published IMU data and direct observation of such an event on any marine species and has important implications for our understanding of the non-lethal impact of anthropogenic interactions with CM and marine megafauna more broadly.
Methods
In April 2024, we deployed a Customized Animal Tracking Solutions (CATS; Australia) camera-enabled IMU package on a CM within Ireland’s newly proposed National Marine Park near the Blasket Islands, County Kerry (Supplementary Figure 1). The IMU consisted of inertial sensors (12 channels of data: tri-axial accelerometers, magnetometers and gyroscopes, depth, temperature, and light; see Chapple et al., 2015) with a towed camera. The package was affixed to the body using two 12-cm monofilament leaders inserted into the dorsal musculature with a tagging pole and was set to release from the animal after ~12–18 h. The animal was sexed using underwater video and length was estimated based on comparison with the tagging vessel.
Data processing
Tag accelerometers were sampled at 200 Hz, gyroscopes and magnetometers at 50 Hz, and depth, pressure, light, and temperature at 10 Hz. We used custom-written scripts in MATLAB, 2023 following Cade et al. (2021) to decimate all data to 10 Hz, correct tag orientation for animal orientation (pitch, roll, and heading), and synchronize the video data from a towed float with the inertial sensors. Animal location was estimated for the duration of each deployment by interpolating pseudotracks of the animal derived from animal heading and speed, estimated from the amplitude of tag vibrations (Cade et al., 2018), between the tagging location, with four resight locations over the first 5 h of the deployment and the tag pop-off position.
Following Andrzejaczek et al. (2018); Andrzejaczek et al. (2020), we calculated vertical velocity (VV; the rate of change in depth over a 1-s period) and split the depth record into vertical swimming phases (ascending, descending, and level swimming). The depth data were smoothed using a 10-s running mean, and we calculated the average VV by taking the difference of this smoothed depth between successive points at 1-s intervals. Swimming phases were defined as VV > 0.05 m s-1 (ascents), VV<-0.05 m s-1 (descents) or 0.05 m s-1 >VV> -0.05 m s-1 (level). Note VV is presented below as the absolute value of VV but labeled as ascent or descent.
As a proxy of relative energetic expenditure, overall dynamic body acceleration (ODBA) was calculated by summing the absolute value of dynamic acceleration from all three axes (Wilson et al., 2006). The dynamic component of acceleration was calculated by subtracting the gravitational component from the raw acceleration for each axis. The gravitational component of acceleration (static acceleration) was determined using a 3-s box smoothing window on the raw acceleration data (Shepard et al., 2008).
Finally, we used a continuous wavelet transformation of the dynamic component of angular acceleration (i.e., sway) in Ethographer (Igor Pro 9, WaveMetrics, USA) to calculate tailbeat cycle (TBC; the time to complete a full tailbeat) and tailbeat amplitude (TBA; a proxy for the acceleration of the tailbeat wave) following Sakamoto et al. (2009). Note that the amplitude here is not a direct measurement of the intensity of the CM’s dynamic movement, but rather a proxy for the relative intensity using a Morlet wavelet function to decompose the acceleration signal. This allows a relative measure of TBA to be compared across the deployment.
We grouped the calculated metrics into 30-min bins to compare response and recovery following the boat strike to baseline behavior (similar to Whitney et al., 2016). Because the distributions were not normal, we also assessed parameter values before and after the strike using a Wilcoxon non-parametric comparison of means.
Video was reviewed for evidence of feeding (i.e., gills open, Figure 1A) before and after the strike as a proxy of resumption to pre-strike behavior.
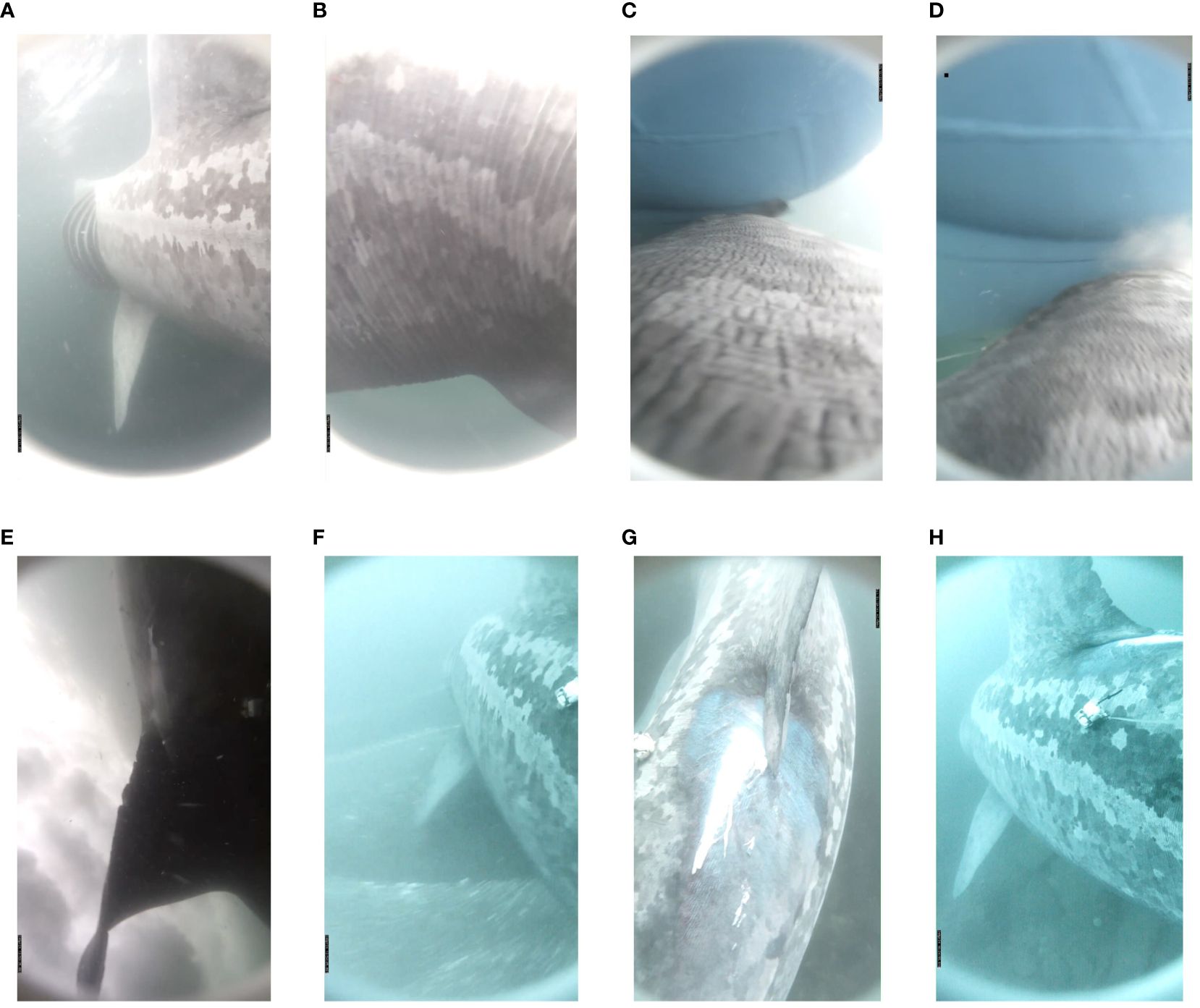
Figure 1 Still photos taken from the animal-borne camera show the basking shark (A) feeding at the surface prior to the boat strike. At 13:53:30, (B) the shark attempted to make a large and quick evasive movement. However, (C, D) within a second, a large boat keel cut across the back of the shark, just behind the dorsal fin and (E) the shark was tumbled through the prop wake. (F) The shark immediately increased tailbeat frequency and powered down to the seafloor for 30 s. (G) Anti-fouling paint (blue), damage to the dermis, and a red abrasion were evident posterior to the dorsal fin where the keel struck the shark. (H) The shark remained associated with the seafloor as it swam in a more directed route into deeper waters for the next 7 h 27 min without feeding.
Results
We tagged a ~7-m female CM at 08:02 local time (UTC+1) on 24 April 2024 while it was feeding on the surface near the Blasket Islands, Ireland (52.089°N, 10.420°W). Immediately following tagging, the shark dove to ~40 m for 7 min and then returned to the surface (Figures 2A, B) and began actively feeding. It swam in tortuous routes east of the Blasket Sound, mostly on the surface and feeding (Figures 1A, 2A, C), with an occasional dive to ~40 m for the next 5 h 50 min. At 13:53:30, the shark was actively feeding at <1 m depth when it attempted to make a large and quick evasive movement (Figure 1B). Within a second, a large boat keel cut across the back of the shark, just behind the dorsal fin (Figures 1C, D), and the shark was tumbled through the water (Figure 1E). Once righted, the shark immediately increased tailbeat frequency and powered down to the seafloor for 30 s (Figures 1F, 2D; Supplementary Videos 1, 2). There was immediate damage to the dermis, anti-fouling paint, and a red abrasion, posterior to the dorsal fin where the keel struck the shark, but no apparent bleeding or open wound (Figure 1G). The shark remained deeper than 10 m, consistently along the seafloor (Figure 1H), as it swam in a more directed route, interspersed with periods of near motionless movement near the sea bottom (Figure 2E; Supplementary Video 3) for the next 7 h 27 min without feeding, until the tag released at 21:22 (Figure 2).
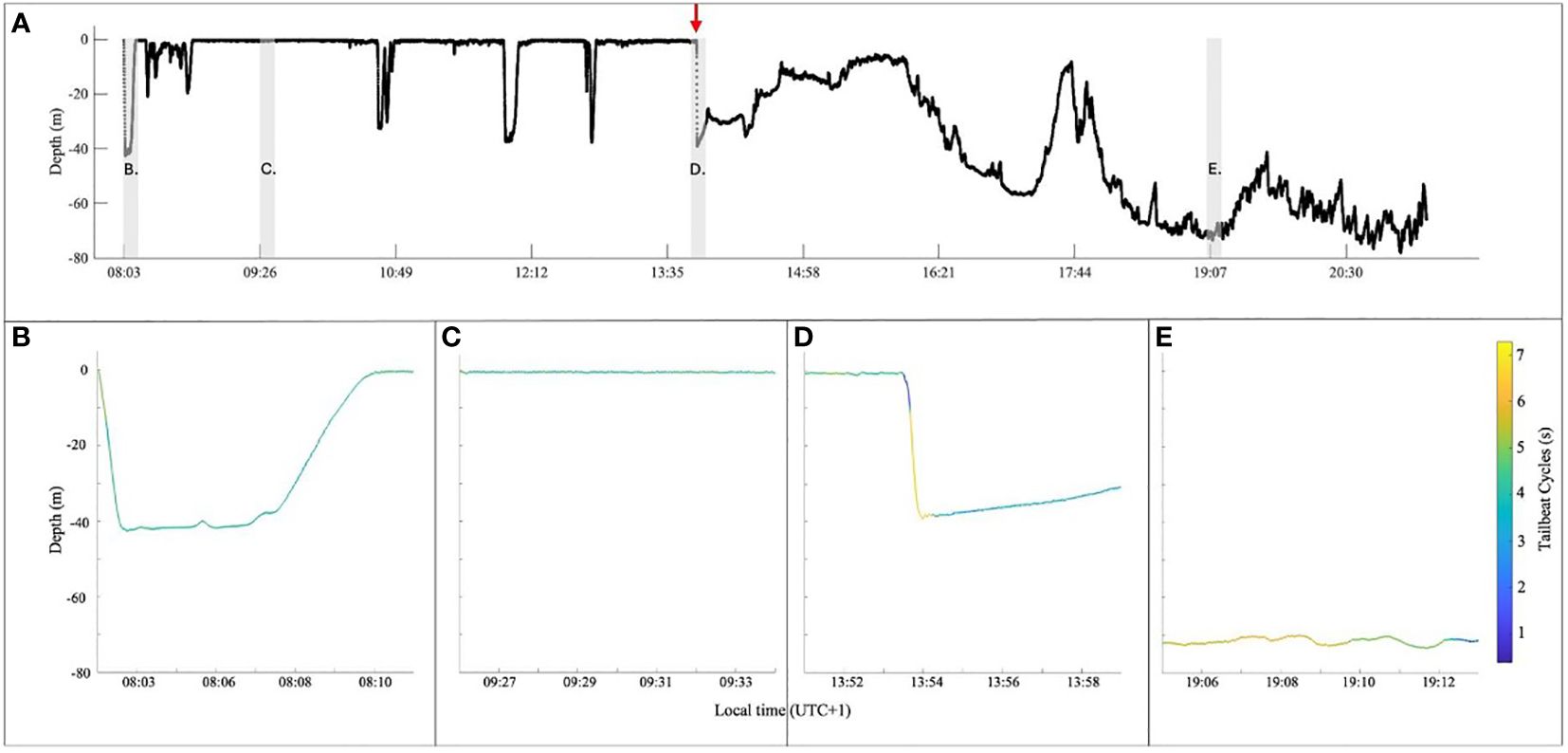
Figure 2 (A) Depth data (black) over the course of the deployment show the surface-associated foraging prior to the strike event (red arrow). Each point is a depth s−1. The spacing of the points immediately following the event indicates relatively how quickly the basking shark swam down, which can be compared to the response to tagging (first descent). The shark did not return to the surface after the boat strike but stayed along the bottom for the duration of the videos. (B–E) present 8-min sections of the entire depth plot (indicated by the shaded sections in (A)) with the line colored by Tailbeat Cycle, including (B) immediately post-tagging, (C) feeding at the surface, (D) the boat strike, and (E) the period where the animal was near motionless on the bottom.
Inertial sensors revealed a response in the behavior of the shark immediately following the strike and for the remainder of the deployment. Once the animal righted itself, there was an immediate burst of speed to 5.6 m s−1 with an average of 3.1 m s−1 ± 0.52 (mean ± SD) during the 30-s descent. This coincided with an increase in downward vertical velocity (2.3 m s−1 ± 0.26) as it swam toward the seafloor, but once the animal reached the seafloor, the vertical velocity (0.12 m s−1 ± 0.085) stayed below pre-strike level (0.22 m s−1 ± 0.21; p < 0.0001) with little variability (Table 1; Supplementary Figure 2). Positive vertical velocity (i.e., ascent; 0.088 m s−1 ± 0.038) also remained below pre-strike levels (0.16 m s−1 ± 0.098; p < 0.0001), suggesting an overall decrease in vertical activity (Table 1; Supplementary Figure 3). Similarly, ODBA initially spiked (0.37 g s−1 ± 0.20) during the 10 s following the boat strike, which was ~8× greater than the mean pre-strike value (0.047 g s−1 ± 0.02). Then, ODBA fell below the pre-strike value for the remainder of the deployment (0.027 g s−1 ± 0.016; p < 0.0001; Table 1; Supplementary Figure 4). Similar to ODBA, TBC and TBA initially peaked in activity (i.e., lower TBC and higher TBA) immediately after the strike (1.9 s ± 0.63 and 5.7 ± 0.82), but then decreased (5.2 s ± 1.1 and 1.6 ± 0.76) below pre-strike levels (4.4 s ± 0.42 and 2.5 ± 0.95; p < 0.0001 for both) for the remainder of the deployment (Table 1; Supplementary Figures 5, 6, respectively).
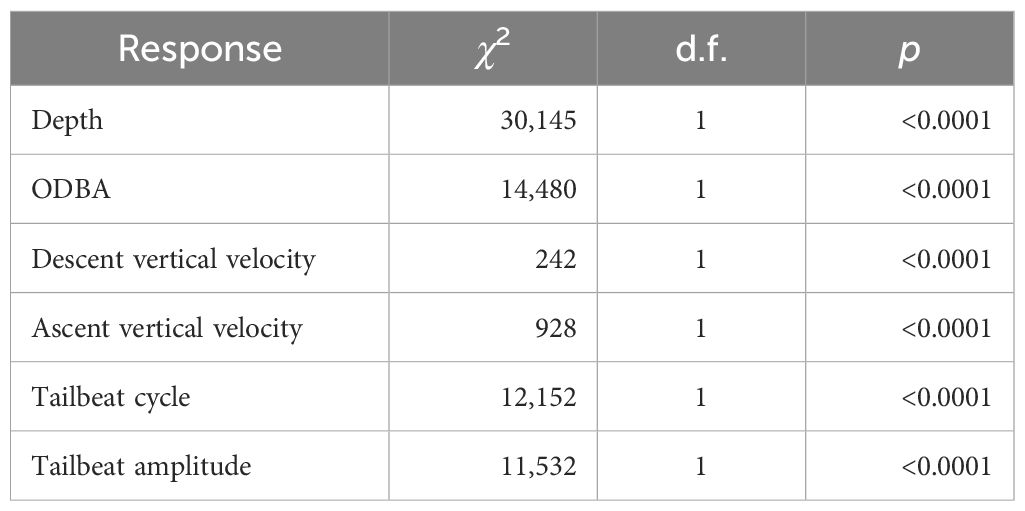
Table 1 Results from a Wilcoxon non-parametric comparison of means before and after the strike for the six different metrics analyzed from the IMU.
Discussion
We present data showing an immediate response by a CM to a boat strike in the newly delineated National Marine Park. While the CM reacted to the strike with highly energetic movement away from the event, it quickly reduced its overall activity (ODBA, TBC, TBA, and vertical velocity) for the remainder of the deployment. Notably, the animal also ceased to feed at any point across the remaining ~7.5 h and headed towards deep offshore waters. This is in stark contrast to the pre-strike period where the animal was observed feeding along the surface in shallow coastal water for 84% of the first ~6 h. It is common for animals to increase activity during recovery periods after handling (e.g., fish, Dolton et al., 2022; sharks, Iosilevskii et al., 2022; and mammals, Shuert et al., 2021). However, the CM exhibited flight behavior and a reduction in activity, such that it appeared to be resting on the bottom at times (Figure 2E; Supplementary Video 3). This difference likely stems from the difference in the stressor and required response; capture stress (i.e., fight time, handling) initiates changes in blood chemistry (e.g., buildup of lactate; Gallagher et al., 2014) and increased activity can aid in the removal of lactate via oxidation (Iosilevskii et al., 2022). Because the CM was responding to a brief acute stressor instead of capture, it likely did not require increased activity to drive oxidation, but instead responded to traumatic event/injury with reduced activity (Mercier et al., 2003). We note that species- and individual-specific differences do occur in acute stress response; thus, we cannot make a broader prediction of post-strike behavior across CM.
Animals in studies of post-release mortality and recovery likely employ very different behavioral and physiological pathways to cope with capture stress than an acute event/injury such as observed here. As these are, to our knowledge, the first direct data collected during a boat strike and the extent of any internal injury is unknown, the expected time to mortality or recovery of the CM is uncertain. While the initial strike was apparently non-lethal and CM did remain active for the 7.5 h following the event, the deployment duration was not sufficient to confirm a recovery back to pre-strike behaviors or determine any long-term consequences (e.g., effects of missed feeding opportunity; Rennolds and Bely, 2023) or eventual mortality. More work is needed to understand the long-term implications and recovery from such events.
It is important to note that while this interaction induced significant behavioral changes and cost to the animal and apparent abrasions, it did not leave obvious external injury definitively indicative of a boat strike (e.g., deep gashes or deformations). Thus, while physical evidence of boat strikes has been opportunistically observed in the area (Massett, personal observation; Supplementary Figure 7), observations of physical damage likely underestimate the actual prevalence of interactions. This finding is also informative for work in other areas where research efforts have relied on scarring quantity and recovery over time to understand the risks and impacts of boat strikes (e.g., Bradford et al., 2009; Lester et al., 2020; Strike et al., 2022).
These data emerge at a critical moment in the protection of CM, with the opportunity to justify and inform imminent management efforts for the species. With the increasing numbers of sightings in the region, and throughout Ireland (IBSG, personal communication), it is very likely that CM–vessel interactions will continue to increase with the recovery of the population. While observations of physical damage are imperfect, as mentioned above, a systematic recording of apparent physical damage could nonetheless provide a relative or minimum measure of interaction rates. Thus, we recommend prioritizing a systematic catalog of physical injuries to provide a conservative baseline of boat strike interactions and inform management actions. Additionally, with CM’s recent listing under Ireland’s Wildlife Act of 1976, which increased levels of protection from injury and disturbance in critical breeding or resting habitats, the newly delineated National Marine Park in County Kerry represents an opportunity to establish stronger protection for CM and other marine species. These protections further justify a systematic catalog of injuries and could mitigate threats, not only of these boat strikes, but also other anthropogenic risks encountered by marine species (e.g., ground lines connecting lobster traps; Supplementary Figure 8) within a defined marine area.
There have also been community-led efforts to mitigate such harmful human–wildlife encounters, such as through the Code of Conduct proposed by the IBSG. This document (available in English and Irish; https://www.baskingshark.ie/downloads) specifically identifies vessel strikes as a potential CM threat and offers guidelines for reducing these risks. However, neither this nor any other version of the Code of Conduct has been formally adopted as enforceable policy by any regulatory body. Within this conservation context, the data we present therefore offer further support for both a legally binding Code of Conduct and a formal management plan within the marine park, and throughout Ireland, to enhance protection measures for the species here.
Boat strikes have been reported as an ongoing global threat to CM (e.g., McInturf et al., 2022) and other marine megafauna (e.g., Kite-Powell et al., 2007; Womersley et al., 2022), but defining their scope and impact is challenging given the lack of reporting and opportunities to record behavioral responses immediately before and after events. Though overlap with vessel activity is likely to be highest in locations such as Ireland, where CM are still sighted regularly at the surface, we recommend that research and conservation efforts throughout the species’ range consider the likelihood that boat strikes, and their subsequent non-lethal effects, may be more common than previously considered. More work needs to be done to assess their impact on the short- and long-term recovery of this species.
Data availability statement
The raw data supporting the conclusions of this article will be made available by the authors, without undue reservation.
Ethics statement
The animal study was approved by Oregon State University-Institutional Animal Care and Use Committee #IACUC-2023-0373 Health Products Regulatory Authority of Ireland (#AE19136/P127). The study was conducted in accordance with the local legislation and institutional requirements.
Author contributions
TC: Conceptualization, Formal analysis, Funding acquisition, Investigation, Methodology, Project administration, Resources, Supervision, Visualization, Writing – original draft. DC: Conceptualization, Funding acquisition, Investigation, Methodology, Project administration, Writing – review & editing, Data curation, Formal analysis, Supervision, Visualization. NM: Investigation, Methodology, Resources, Writing – review & editing. JG: Conceptualization, Funding acquisition, Supervision, Writing – review & editing. NP: Conceptualization, Funding acquisition, Investigation, Methodology, Project administration, Supervision, Writing – review & editing, Resources. AM: Conceptualization, Funding acquisition, Supervision, Writing – review & editing, Investigation, Methodology, Project administration, Resources, Data curation, Visualization.
Funding
The author(s) declare financial support was received for the research, authorship, and/or publication of this article. Funding for this project was provided by Future Legend Films, Oregon State University, and National Geographic and Human Frontiers Science Project (HFSP - RGP0010/2020).
Acknowledgments
The authors would like to thank Jamie Cornelius, CHR Cornapple, Evan Batzer, Emmett Johnston, Simon Berrow, Donal Griffin and other members of the Irish Basking Shark Group, Niko Liebsch at Customized Animal Tracking Solutions, Captain Michael (“Mick”) Sheeran, and Vincent Brown with Blasket Island Eco Marine Tours and Future Legend Films.
Conflict of interest
The authors declare that the research was conducted in the absence of any commercial or financial relationships that could be construed as a potential conflict of interest.
Publisher’s note
All claims expressed in this article are solely those of the authors and do not necessarily represent those of their affiliated organizations, or those of the publisher, the editors and the reviewers. Any product that may be evaluated in this article, or claim that may be made by its manufacturer, is not guaranteed or endorsed by the publisher.
Supplementary material
The Supplementary Material for this article can be found online at: https://www.frontiersin.org/articles/10.3389/fmars.2024.1430961/full#supplementary-material
References
Andrzejaczek S., Gleiss A. C., Lear K. O., Pattiaratchi C., Chapple T. K., Meekan M. G. (2020). Depth-dependent dive kinematics suggest cost-efficient foraging strategies by tiger sharks. R. Soc Open Sci. 7, 200789. doi: 10.1098/rsos.200789
Andrzejaczek S., Gleiss A. C., Pattiaratchi C. B., Meekan M. G. (2018). First insights into the fine-scale movements of the sandbar shark, carcharhinus plumbeus. Front. Mar. Sci. 5. doi: 10.3389/fmars.2018.00483
Baduini C. L. (1995). Feeding ecology of the basking shark (Cetorhinus maximus) relative to distribution and abundance of prey. (San Jose State University). Available online at: https://search.proquest.com/openview/68d8ef44cdec14cbf26c8c4dd3c5f80d/1?pq-origsite=gscholar&cbl=18750&diss=y (Accessed May 8, 2024).
Bradford A. L., Weller D. W., Ivashchenko Y. V., Burdin A. M., Brownell R. L. Jr (2009). Anthropogenic scarring of western gray whales (Eschrichtius robustus). Mar. Mammal Sci. 25, 161–175. doi: 10.1111/j.1748-7692.2008.00253.x
Cade D. E., Barr K. R., Calambokidis J., Friedlaender A. S., Goldbogen J. A. (2018). Determining forward speed from accelerometer jiggle in aquatic environments. Journal of Experimental Biology 221 (2), jeb170449. doi: 10.1242/jeb.170449
Cade D. E., Gough W. T., Czapanskiy M. F., Fahlbusch J. A., Kahane-Rapport S. R., Linsky J. M. J., et al. (2021). Tools for integrating inertial sensor data with video bio-loggers, including estimation of animal orientation, motion, and position. Anim. Biotelemetry 9, 34. doi: 10.1186/s40317-021-00256-w
Chapple T. K., Gleiss A. C., Jewell O. J. D., Wikelski M., Block B. A. (2015). Tracking sharks without teeth: a non-invasive rigid tag attachment for large predatory sharks. Anim. Biotelemetry 3, 14. doi: 10.1186/s40317-015-0044-9
Dolton H. R., Jackson A. L., Drumm A., Harding L., Ó Maoiléidigh N., Maxwell H., et al. (2022). Short-term behavioural responses of Atlantic bluefin tuna to catch-and-release fishing. Conserv. Physiol. 10, coac060. doi: 10.1093/conphys/coac060
Finucci B., Duffy C. A. J., Brough T., Francis M. P., Milardi M., Pinkerton M. H., et al. (2021). Drivers of spatial distributions of basking shark (Cetorhinus maximus) in the Southwest Pacific. Front. Mar. Sci. 8. doi: 10.3389/fmars.2021.665337
Gallagher A. J., Serafy J. E., Cooke S. J., Hammerschlag N. (2014). Physiological stress response, reflex impairment, and survival of five sympatric shark species following experimental capture and release. Mar. Ecol. Prog. Ser. 496, 207–218. doi: 10.3354/meps10490
Iosilevskii G., Kong J. D., Meyer C. G., Watanabe Y. Y., Papastamatiou Y. P., Royer M. A., et al. (2022). A general swimming response in exhausted obligate swimming fish. R. Soc Open Sci. 9, 211869. doi: 10.1098/rsos.211869
Jones P. J. S. (2021). A governance analysis of Ningaloo and Shark Bay Marine Parks, Western Australia: Putting the ‘eco’ in tourism to build resilience but threatened in long-term by climate change? Mar. Policy 127, 103636. doi: 10.1016/j.marpol.2019.103636
Kite-Powell H. L., Knowlton A., Brown M. (2007). Modeling the effect of vessel speed on right whale ship strike risk. Proj. Rep. NOAANMFS Proj. NA04NMF47202394 2, 1–8.
Legaspi C., Miranda J., Labaja J., Snow S., Ponzo A., Araujo G. (2020). In-water observations highlight the effects of provisioning on whale shark behaviour at the world’s largest whale shark tourism destination. R. Soc Open Sci. 7, 200392. doi: 10.1098/rsos.200392
Lester E., Meekan M. G., Barnes P., Raudino H., Rob D., Waples K., et al. (2020). Multi-year patterns in scarring, survival and residency of whale sharks in Ningaloo Marine Park, Western Australia. Mar. Ecol. Prog. Ser. 634, 115–125. doi: 10.3354/meps13173
McFarlane G., King J., Leask K., Christensen L. B. (2009). Assessment of information used to develop a recovery potential assessment for basking shark Cetorhinus maximus (Pacific population) in Canada (Ottawa, ON(Canada: DFO). Available online at: https://www.researchgate.net/profile/Jackie-King-8/publication/305489595_Assessment_of_information_used_to_develop_a_Recovery_Potential_assessment_for_basking_shark_Cetorhinus_maximus_Pacific_population_in_Canada/links/5791179408ae0831552f95fb/Assessment-of-information-used-to-develop-a-Recovery-Potential-assessment-for-basking-shark-Cetorhinus-maximus-Pacific-population-in-Canada.pdf (Accessed May 8, 2024). Fisheries, D. of, and Oceans, O.
McInturf A. G., Muhling B., Bizzarro J. J., Fangue N. A., Ebert D. A., Caillaud D., et al. (2022). Spatial distribution, temporal changes, and knowledge gaps in basking shark (Cetorhinus maximus) sightings in the California Current Ecosystem. Front. Mar. Sci. 9, 818670. doi: 10.3389/fmars.2022.818670
Mercier S., Frédéric, Canini, Buguet A., Cespuglio R., Martin S., et al. (2003). Behavioural changes after an acute stress: stressor and test types influences. Behav. Brain Res. 139, 167–175. doi: 10.1016/S0166-4328(02)00265-6
Miller P. I., Scales K. L., Ingram S. N., Southall E. J., Sims D. W. (2015). Basking sharks and oceanographic fronts: quantifying associations in the north-east Atlantic. Funct. Ecol. 29, 1099–1109. doi: 10.1111/1365-2435.12423
Murray A., Garrud E., Ender I., Lee-Brooks K., Atkins R., Lynam R., et al. (2020). Protecting the million-dollar mantas; creating an evidence-based code of conduct for manta ray tourism interactions. J. Ecotourism 19, 132–147. doi: 10.1080/14724049.2019.1659802
Penketh L., Schleimer A., Labaja J., Snow S., Ponzo A., Araujo G. (2020). Scarring patterns of whale sharks, Rhincodon typus, at a provisioning site in the Philippines. Aquat. Conserv.: Mar. Freshw. Ecosyst. 31, 99–111. doi: 10.1002/aqc.3437
Pirotta V., Grech A., Jonsen I. D., Laurance W. F., Harcourt R. G. (2019). Consequences of global shipping traffic for marine giants. Front. Ecol. Environ. 17, 39–47. doi: 10.1002/fee.1987
Rennolds C. W., Bely A. E. (2023). Integrative biology of injury in animals. Biol. Rev. 98, 34–62. doi: 10.1111/brv.12894
Rigby C. L., Barreto R., Carlson J., Fernando D., Fordham S., Francis M. P., et al. (2019). Cetorhinus maximus (errata version published in 2020). The IUCN Red List of Threatened Species 2019, e.T4292A166822294. Available at: https://dx.doi.org/10.2305/IUCN.UK.2019-3.RLTS.T4292A166822294.en. (Accessed on 14 July 2024).
Sakamoto K. Q., Sato K., Ishizuka M., Watanuki Y., Takahashi A., Daunt F., et al. (2009). Can ethograms be automatically generated using body acceleration data from free-ranging birds? PLoS One 4, e5379. doi: 10.1371/journal.pone.0005379
Shepard E. L., Wilson R. P., Liebsch N., Quintana F., Gómez Laich A., Lucke K. (2008). Flexible paddle sheds new light on speed: a novel method for the remote measurement of swim speed in aquatic animals. Endanger. Species Res. 4, 157–164. doi: 10.3354/esr00052
Shuert C. R., Marcoux M., Hussey N. E., Watt C. A., Auger-Méthé M. (2021). Assessing the post-release effects of capture, handling and placement of satellite telemetry devices on narwhal (Monodon monoceros) movement behaviour. Conserv. Physiol. 9, coaa128. doi: 10.1093/conphys/coaa128
Sims D. W., Berrow S. D., O’Sullivan K. M., Pfeiffer N. J., Collins R., Smith K. L., et al. (2022). Circles in the sea: annual courtship “torus“ behaviour of basking sharks Cetorhinus maximus identified in the eastern North Atlantic Ocean. J. Fish Biol. 101, 1160–1181. doi: 10.1111/jfb.15187
Sims D. W., Quayle V. A. (1998). Selective foraging behaviour of basking sharks on zooplankton in a small-scale front. Nature 393, 460–464. doi: 10.1038/30959
Strike E. M., Harris J. L., Ballard K. L., Hawkins J. P., Crockett J., Stevens G. M. (2022). Sublethal injuries and physical abnormalities in Maldives manta rays, Mobula alfredi and Mobula birostris. Front. Mar. Sci. 9, 773897. doi: 10.3389/fmars.2022.773897
Whitney N. M., White C. F., Gleiss A. C., Schwieterman G. D., Anderson P., Hueter R. E., et al. (2016). A novel method for determining post-release mortality, behavior, and recovery period using acceleration data loggers. Fish. Res. 183, 210–221. doi: 10.1016/j.fishres.2016.06.003
Wilson R. P., White C. R., Quintana F., Halsey L. G., Liebsch N., Martin G. R., et al. (2006). Moving towards acceleration for estimates of activity-specific metabolic rate in free-living animals: the case of the cormorant. J. Anim. Ecol. 75, 1081–1090. doi: 10.1111/j.1365-2656.2006.01127.x
Keywords: boat strike, basking shark, inertial measurement unit, non-lethal injury, marine megafauna, behavior
Citation: Chapple TK, Cade DE, Goldbogen J, Massett N, Payne N and McInturf AG (2024) Behavioral response of megafauna to boat collision measured via animal-borne camera and IMU. Front. Mar. Sci. 11:1430961. doi: 10.3389/fmars.2024.1430961
Received: 05 June 2024; Accepted: 28 June 2024;
Published: 24 July 2024.
Edited by:
Emily Lester, Australian Institute of Marine Science (AIMS), AustraliaReviewed by:
Christine Barry, Murdoch University, AustraliaVanessa Pirotta, Macquarie University, Australia
Copyright © 2024 Chapple, Cade, Goldbogen, Massett, Payne and McInturf. This is an open-access article distributed under the terms of the Creative Commons Attribution License (CC BY). The use, distribution or reproduction in other forums is permitted, provided the original author(s) and the copyright owner(s) are credited and that the original publication in this journal is cited, in accordance with accepted academic practice. No use, distribution or reproduction is permitted which does not comply with these terms.
*Correspondence: Taylor K. Chapple, Y2hhcHBsZXRAb3JlZ29uc3RhdGUuZWR1