- 1School of Fishery, Zhejiang Ocean University, Zhoushan, China
- 2Key Laboratory for Coastal and Wetland Ecosystems, Ministry of Education, College of the Environment and Ecology, Xiamen University, Xiamen, China
- 3National Observation and Research Station for the Taiwan Strait Marine Ecosystem, Xiamen University, Xiamen, China
- 4Guangxi Key Lab of Mangrove Conservation and Utilization, Guangxi Academy of Marine Sciences, Guangxi Mangrove Research Center, Guangxi Academy of Sciences, Beihai, China
Vivipary is common in several mangrove species and is generally considered an adaptation to the intertidal saline environment. However, the coexistence of many nonviviparous mangroves makes this view controversial. This study investigated the propagule development of two nonviviparous mangrove species, Sonneratia alba and S. caseolaris, with marked differences in salt tolerance and distribution. Changes in the density, water content, and concentrations of the five main osmoregulatory elements (Cl, Na, K, Ca, and Mg) were determined. As the propagules of S. alba and S. caseolaris mature, the element concentrations (mg/g) in the propagules gradually decrease, indicating a desalination process. Moreover, the Cl, Na, Ca, and Mg content in the propagules were lower than in the mature leaves and calyx. Similar to viviparous mangroves, the development of the propagules of nonviviparous mangroves is also a desalination process. Although both viviparous and nonviviparous mangrove species undergo a desalination process during propagule development, our findings suggest that viviparity may not be solely defined by desalination, but rather by the extended period of low-salinity protection during early development on the maternal tree, which represents a key adaptation for survival in high-salinity environments. In contrast, nonviviparous mangroves, which rely on seed germination and early development in saline seawater, face additional challenges in high-salinity habitats, highlighting their distinct adaptive strategies.
1 Introduction
Mangroves, unique ecosystems located at the interface of land and sea, play vital roles in coastal protection, biodiversity conservation, and carbon sequestration (Duke, 2006). Mangrove plants employ distinct reproductive strategies, namely vivipary (including true-vivipary and crypto-vivipary) and nonvivipary, which are considered to contribute significantly to their ecological success in the intertidal environment (Tomlinson, 2016). Vivipary is the phenomenon whereby the offspring produced via sexual reproduction germinate directly on the maternal body (Elmqvist and Cox, 1996), which in the plant kingdom mainly occurs in mangroves. True-vivipary is when the propagule (mainly the hypocotyl) breaks through the seed coat and fruit coat and extends outside the fruit, mainly in the genus Rhizophora, Kandelia, Ceriops, and Bruguiera of Rhizophoracea. Crypto-vivipary is a phenomenon in which the seeds break through the seed coat but remain enclosed within the fruit, as in the genus Avicennia, Candelabra, Aegiceras, Nypa, and Pelliciera. The mangrove species that use seeds as propagules (nonvivipary) mainly include Sonneratia, Lumnitzera, Acanthus, and some associated mangrove species (Wang and Wang, 2007).
The previous studies on mangrove reproduction have mainly focused on viviparous species, as this trait is considered to be an important characteristic of mangrove plants (Tomlinson, 2016; Zhou et al., 2016; Zhou et al., 2022). The salient adaptive value of vivipary in mangroves is of great interest to many plant biologists, who have discussed the viviparous phenomenon from morphological, physiological, and evolutionary perspectives. Early germination on the mother tree may facilitate rooting (Zhou et al., 2016), buoyancy during sea dispersal (Van der Stocken et al., 2019), salt regulation (Joshi, 1933; Liang et al., 2008), ionic balance (Wang et al., 2002), and establishment in coarse-grained environments (Elmqvist and Cox, 1996). Because mangroves originated in freshwater habitats (Dawes, 1998), vivipary may facilitate the germination of mangrove propagules and avoid salt stress. Studies of the development stages of viviparous mangrove species and genome analysis have revealed that mangrove plants have reverted, suggesting that mangrove plants evolved from terrestrial plants (Zheng et al., 1999; Wang et al., 2002; Zhang et al., 2006; Guo et al., 2022). However, the developmental inferences are only for viviparous mangrove species, and proper evidence supporting such a suggestion is lacking on the counterpart nonviviparous species.
Mangrove ecosystems are characterized by their unique environmental conditions, where species must cope with fluctuating salinity (Xu et al., 2020), tidal inundation (Crase et al., 2013; Leong et al., 2018), and poor oxygen availability in the soil (Krauss et al., 2006). The reproductive strategies of mangrove species, including vivipary and nonvivipary, are essential adaptations that enable these plants to thrive in such challenging conditions. Vivipary has long been regarded as an adaptation to saltwater environments, providing several advantages such as reduced desiccation risk, early root establishment, and improved dispersal potential (Tomlinson, 2016). This strategy also allows seedlings to grow in the relative protection of the maternal plant, where they can complete their early developmental stages in a low-salinity environment before exposure to full seawater conditions (Liang et al., 2008; Zhou et al., 2022).
In contrast, non-viviparous mangroves, such as those in the genus Sonneratia, release seeds that germinate upon contact with suitable substrates, often facing higher salinity and harsher environmental conditions during early development. Although these species do not exhibit the same early-stage protection from salinity, they have evolved other mechanisms, such as salt excretion, osmotic regulation, and the ability to tolerate desiccation, which enable their survival in saline environments (Duke, 2006; Wang and Wang, 2007). The different reproductive strategies in mangroves highlight the diversity of adaptive mechanisms to cope with salinity stress, yet both viviparous and non-viviparous species share key features in their capacity to regulate osmotic pressure and enhance salt tolerance (Wang et al., 2002).
Most mangroves are nonviviparous, and only about one-third of all mangrove species exhibit vivipary (Dawes, 1998). To date, vivipary has always been studied as an isolated phenomenon, and the adaptive and evolutionary value of vivipary still needs to be further discussed through comparisons of viviparous and nonviviparous mangrove species. Comparative studies must address the mechanisms by which vivipary and recalcitrance have converged in so many unrelated taxa (Farnsworth, 2000). We agree with Tomlinson (2016), and Farnsworth and Farrant (1998) that integrative physiological and ecological studies of vivipary and nonvivipary are critical to devising seed-handling strategies, predicting seedling performance, and understanding the evolution of reproductive traits in general.
To improve our understanding of the significance of vivipary, we investigated the dynamics of the chemical elements involved in osmoregulation during the propagule development of two non-viviparous mangrove species of Sonneratia (S. alba and S. caseolaris) with different salinity adaptations and compared with viviparous mangrove. Sonneratia is naturally distributed in China on Hainan Island and includes five species, S. alba, S. caseolaris, S. ovata, S. gulngai, and S. hainanensis (Wang and Wang, 2007). This study analyzed S. alba and S. caseolaris from the same estuary. S. alba is the most salt-tolerant species in the genus Sonneratia and occurs in the downstream region of the estuary, while S. caseolaris is the least salt-tolerant species and is distributed in the upstream region (Duke, 2006; Wang and Wang, 2007; Hasegawa et al., 2014). By comparing the development process of viviparous mangrove plants, this study aims to explore whether vivipary is a key characteristic for mangrove species to adapt to saltwater environments, or if non-viviparous mangroves also undergo a similar desalination process during the development of their propagules. The comparative analysis of these strategies, focusing on aspects such as propagule development, osmotic regulation, and salinity tolerance, will shed light on the relative advantages and limitations of each strategy, offering insights into the evolution of mangrove reproductive traits and their broader ecological significance.
2 Material and methods
2.1 Collection of samples
The propagules/fruits S. alba and S. caseolaris were collected from the mangrove forest in Qinglan Harbor (19°22′-19°35′N, 110°40′-110°48′E), Hainan Island, China, in July of 2007 (Figure 1A). The bay has a tropical monsoon climate, with an average annual temperature of 24°C and an average annual precipitation of 1974 mm (Wang et al., 2019). The tidal pattern in the bay is irregular all-day tide, with an average tidal range of 0.75 m and a maximum tidal range of 2.06 m. Bamen Bay contains the highest biodiversity of mangrove species in China (Wang and Wang, 2007). Of the 25 true mangrove species found in China, 23 species are present in the bay. Bamen Bay is also home to 11 mangrove associates (Wang and Wang, 2007). With the Wenchang and Wenjiao rivers flowing into it, the salinity of the water in the Harbor fluctuates widely (0-25‰). The salinity of the seawater in the main distribution areas of S. alba and S. caseolaris was monitored (HOBO U24-002-C, Onset, USA) every two hours throughout July (Figure 1A). The salinity of the water in the habitat was measured in July, aligning with the peak production period of the propagules, to ensure accurate and contextually relevant data. Convert seawater salinity into density through the density formula of NaCl gradient concentration (25°C). The density of seawater in the study area ranges from 1.00-1.02 g/cm3. The distribution range of S. alba (Figure 1B) and S. caseolaris (Figure 1C) along the river was obtained through field surveys and both S. alba and S. caseolaris grow along the coastline at the outer edge of the mangrove forest (Figure 1A).
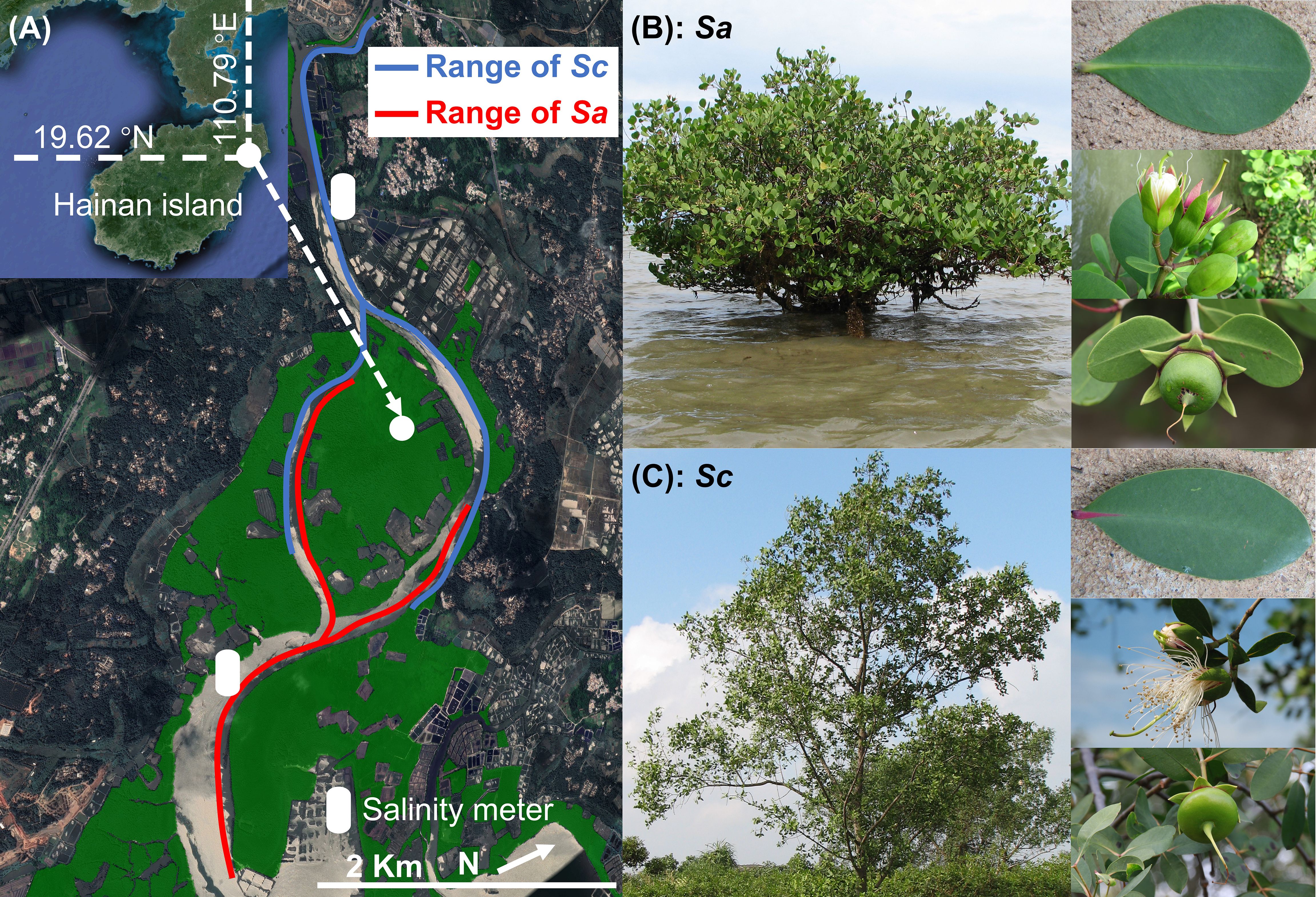
Figure 1. Study area and sampled Sonneratia species. (A) Distribution of Sonneratia alba (Sa) and Sonneratia caseolaris (Sc) along the Wenchang River; (B) Representative image of Sa; (C) Representative image of Sc.
Three adult trees of each species with similar heights and healthy with no obvious damage were chosen to be sampled in 2007. The development of the fruit was divided into four stages according to their weight and diameter (Zheng et al., 1999, Table 1). The last grade was mature fruit, collected from adult trees by gently shaking the branches. 5-9 propagules (fruits) were collected from each stage of each species, and mature leaves and calyxes from fruits were also collected. The experiment was conducted using fruits as propagules.
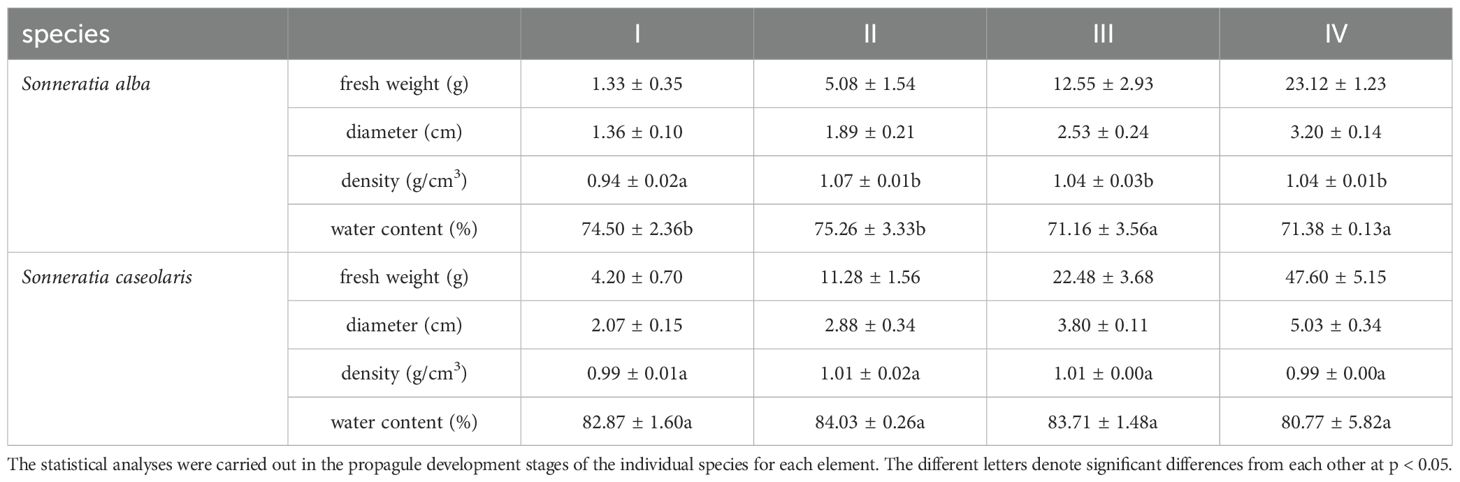
Table 1. Morphological characteristics ± standard deviation (SD)of the development stages of propagules of Sonneratia alba and S. caseolaris in Qinglan Harbor, Hainan Island, China.
2.2 Measurements and analysis
We immediately measured the diameter and fresh weight of the propagules using a vernier caliper (0.01 cm resolution) and an electronic balance (0.01 g resolution) respectively. Afterwards, we employed the water-displacement method to measure the volume of the propagules. Then we calculate the propagule density with the following formula
The osmotic potential of propagule tissues was measured using a model 5520 vapor pressure osmometer (WESCOR, USA). The propagule is then oven-dried at 60°C until a constant weight is reached to obtain the dry weight. The oven-dried propagule is stored in a glass desiccator and utilized for ion contents measurements.
The content of Cl, K, Na, Ca, and Mg was analyzed. Three propagules were randomly selected for measurements at each stage. The Cl content was determined via AgNO3 titration. The subsamples (about 0.2 g each) were heated at 550°C for 3 hours. To avoid the loss of Cl, about 0.05 g of calcium oxide was added and mixed with the sample beforehand. The ash was dissolved in distilled water and then filtered, and the filtrate was used to determine the Cl- content.
To determine the concentrations of K, Na, Ca, and Mg, subsample (about 0.1 g each) was incinerated at 550°C for 3 hours. The ash was dissolved in 1 ml of nitric acid (GR) for 45–60 minutes, and then, the solution was diluted using 2% nitric acid. The diluted solution was analyzed using inductively coupled mass spectrometry (ICP-MS) (ELANDRC-e, Perkin Elmer Inc., USA).
The element content data of viviparous mangrove propagules were obtained through literature sources. Data for Kandelia obovata and Bruguiera sexangula were extracted from Zheng et al., 1999, while data for Bruguiera gymnorhiza were obtained from Zhang et al., 2006. K. obovata, B. sexangula and B. gymnorhiza are typical viviparous mangrove species. The element content measurement methods both in this study and Zhang et al., 2006 followed those of Zheng et al., 1999.
The mean and standard deviation of triplicate sample analyses were calculated. Linear fitting was used to analyze the change of osmotic potential and elemental concentrations. Then we determined relationships between each element content and developmental stages (size) of mangrove propagules. In order to better compare different sized propagules, we normalized the data to a range of 0 to 1. All tests were conducted, and figures were created using R v.4.0.2 (R Core Team, 2020).
3 Results
3.1 Density, water content and osmotic potential
The density of the S. alba fruits increased markedly from Stage I to Stage II (p<0.05), with an increase of 13.8%, and thereafter, almost no change was recorded (Table 1). There were almost no changes for the density of S. caseolaris during the development of the fruits. In the later stages of development, the density of the S. alba propagules was consistently greater than 1 g/cm3 and was greater than that of S. caseolaris. The density of the mature fruits was close to that of seawater (1.00-1.02 g/cm3), which is consistent with their ability to drift and disperse via seawater.
The water content of the S. alba propagules significantly declined during development, decreasing by only 4.2% compared to that in Stage I (Table 1). In contrast, the water content of S. caseolaris showed no significant change throughout development. The propagules of both species maintained high (>70%) in each stage of development.
The salinity in the main distribution areas of S. alba and S. caseolaris fluctuates significantly (Figure 2A). The average salinity in the S. caseolaris distribution area is 5.69 ± 3.49‰, while in the S. alba distribution area, the average salinity is 19.45 ± 2.49‰. There are significant differences between S. alba and S. caseolaris in their tolerance to seawater salinity. During the development of propagules, osmotic potential increases significantly with the influx of water (Figure 2B), and the osmotic potential of S. caseolaris propagules is higher than that of S. alba.
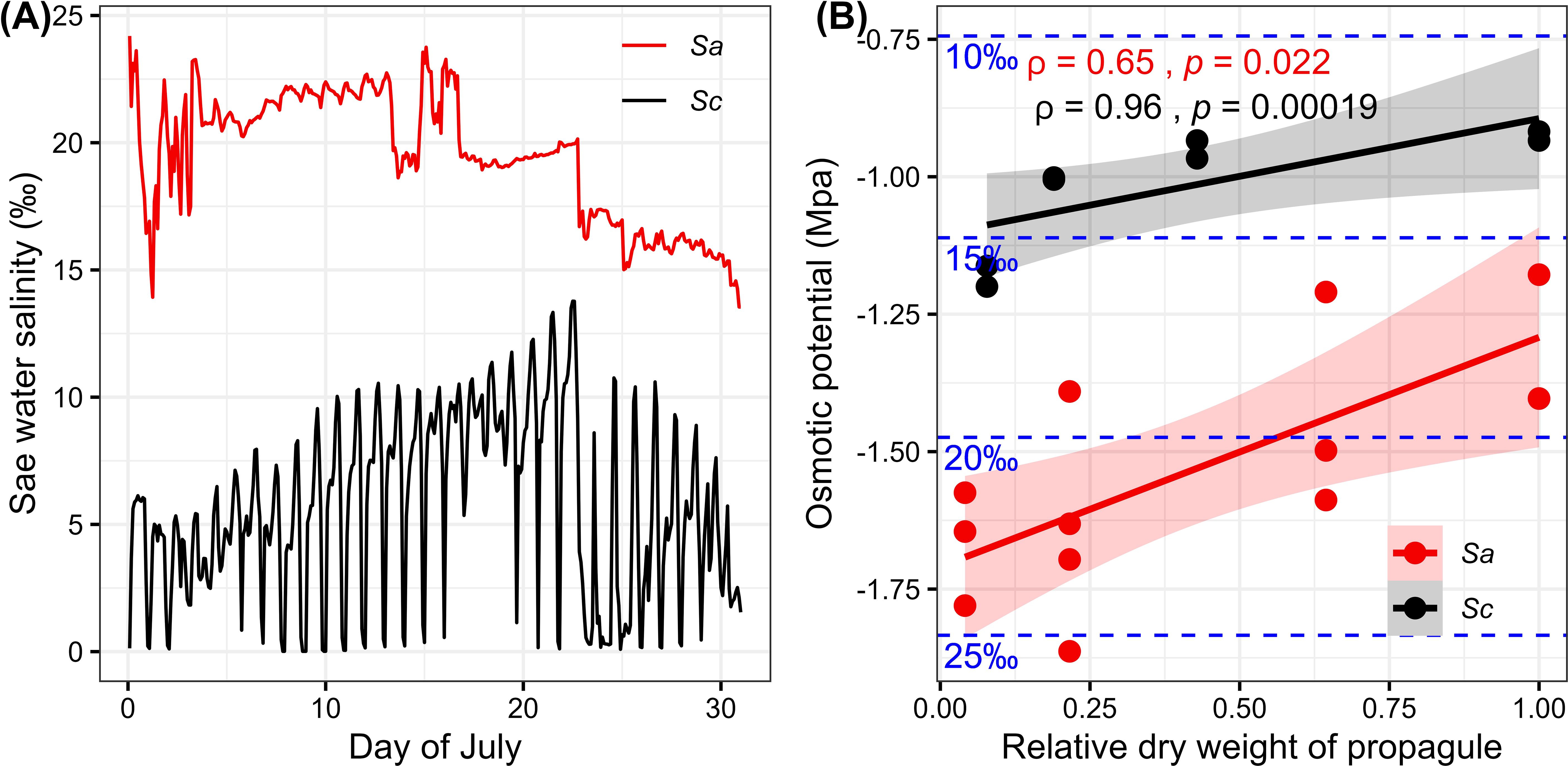
Figure 2. (A) July seawater salinity in the main distribution areas of Sonneratia alba (Sa) and Sonneratia caseolaris (Sc); (B) osmotic potential changes at different developmental stages of Sa and Sc propagules.
3.2 Cl, Na, K, Ca, and Mg contents
Part of the Cl, K, Na, Ca, and Mg contents of the propagules decreased with increasing development of the fruits on the maternal plant (Figure 3). From Stage I to maturation, for the fruits of S. alba and S. caseolaris, the Cl content decreased by 52.1% and 44.1%, the Na content decreased by 16.9% and 36.1%, the K content decreased by 21.8% and 34.4%, the Ca content decreased by 70.9% and 69.4%, and the Mg content decreased by 26.5% and 59.7%, respectively. The decrease in the Na content was the smallest (p>0.05), and the decrease in the Ca content was the largest. All data indicate a desalination process during the development of the propagules. In particular, Cl is a vital element correlated with the salt-resistance mechanism, and it decreased significantly (p<0.05). These results suggest that the propagules must develop in a low salt environment.
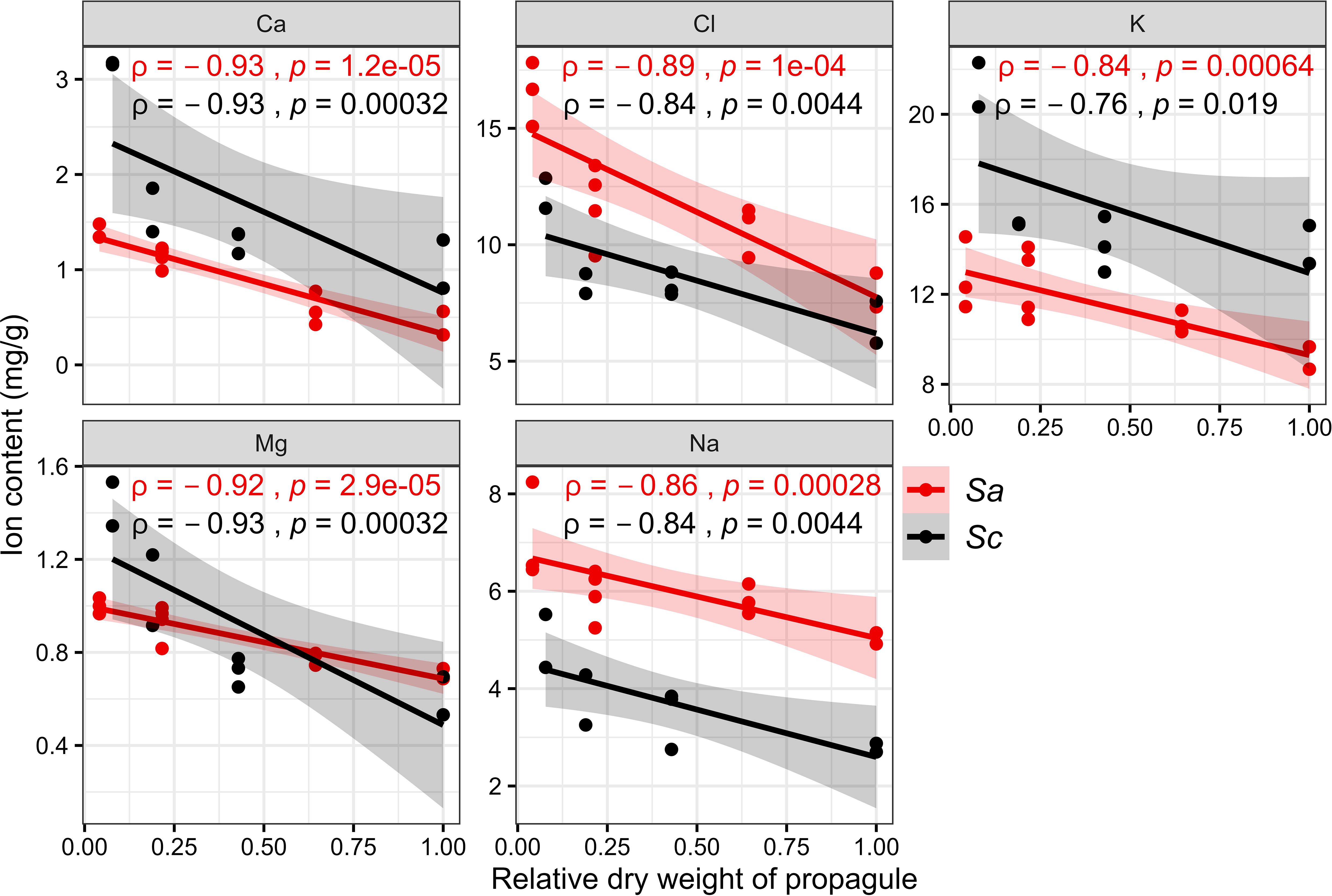
Figure 3. Elemental content changes in different developmental stages of Sonneratia alba (Sa) and Sonneratia caseolaris (Sc) propagules.
There were significant negative linear relationships (Figure 4, p<0.05) between the content of five elements and the development of propagule. Both viviparous (data details in Table 2) and non-viviparous mangrove species exhibited desalination process.
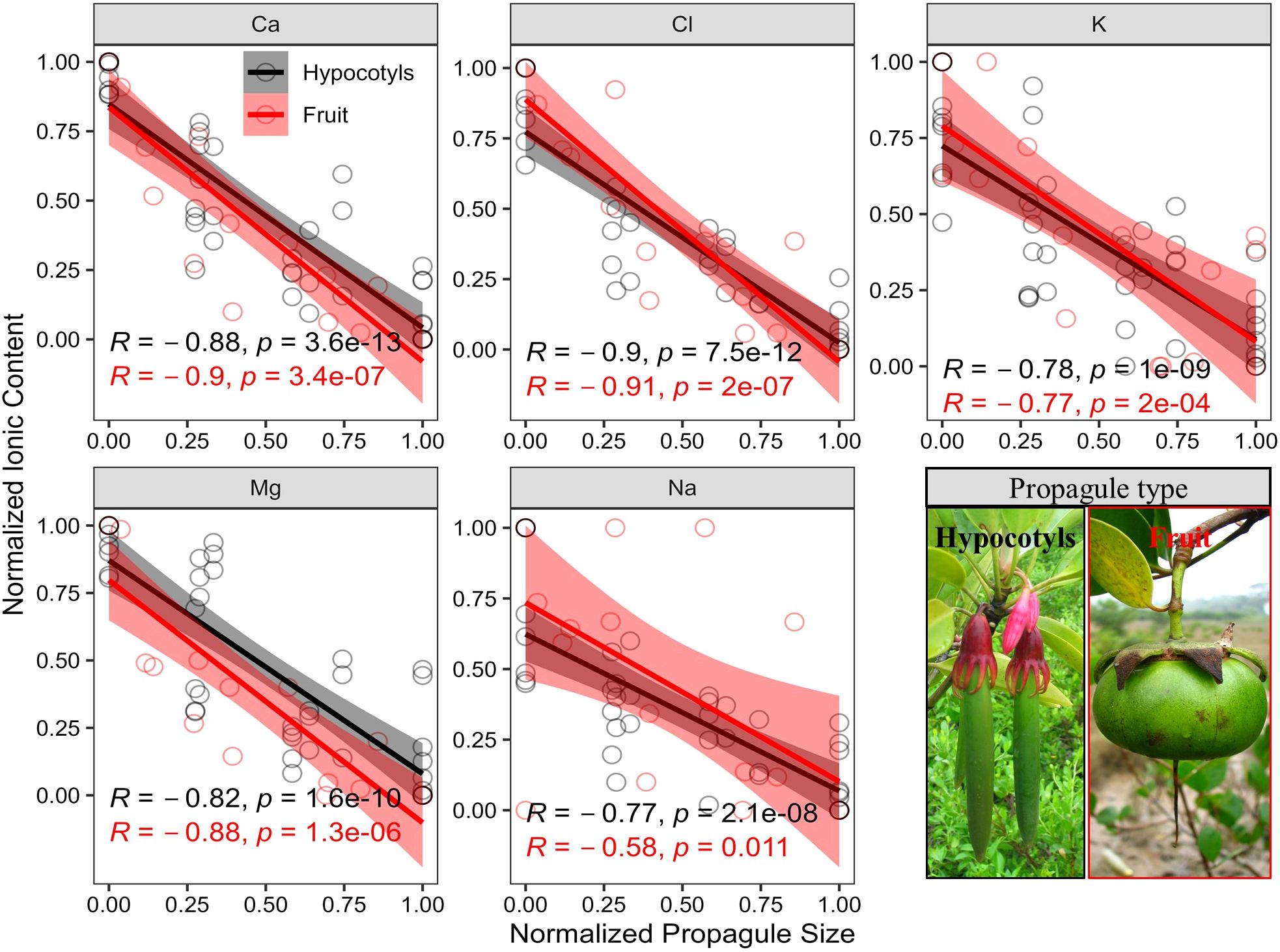
Figure 4. Relationship between elemental content and developmental processes (normalized propagule size) of mangrove propagules. Hypocotyls represent viviparous mangrove propagules, while fruits represent nonviviparous propagules. The bottom right corner shows representative images of hypocotyls and fruits.
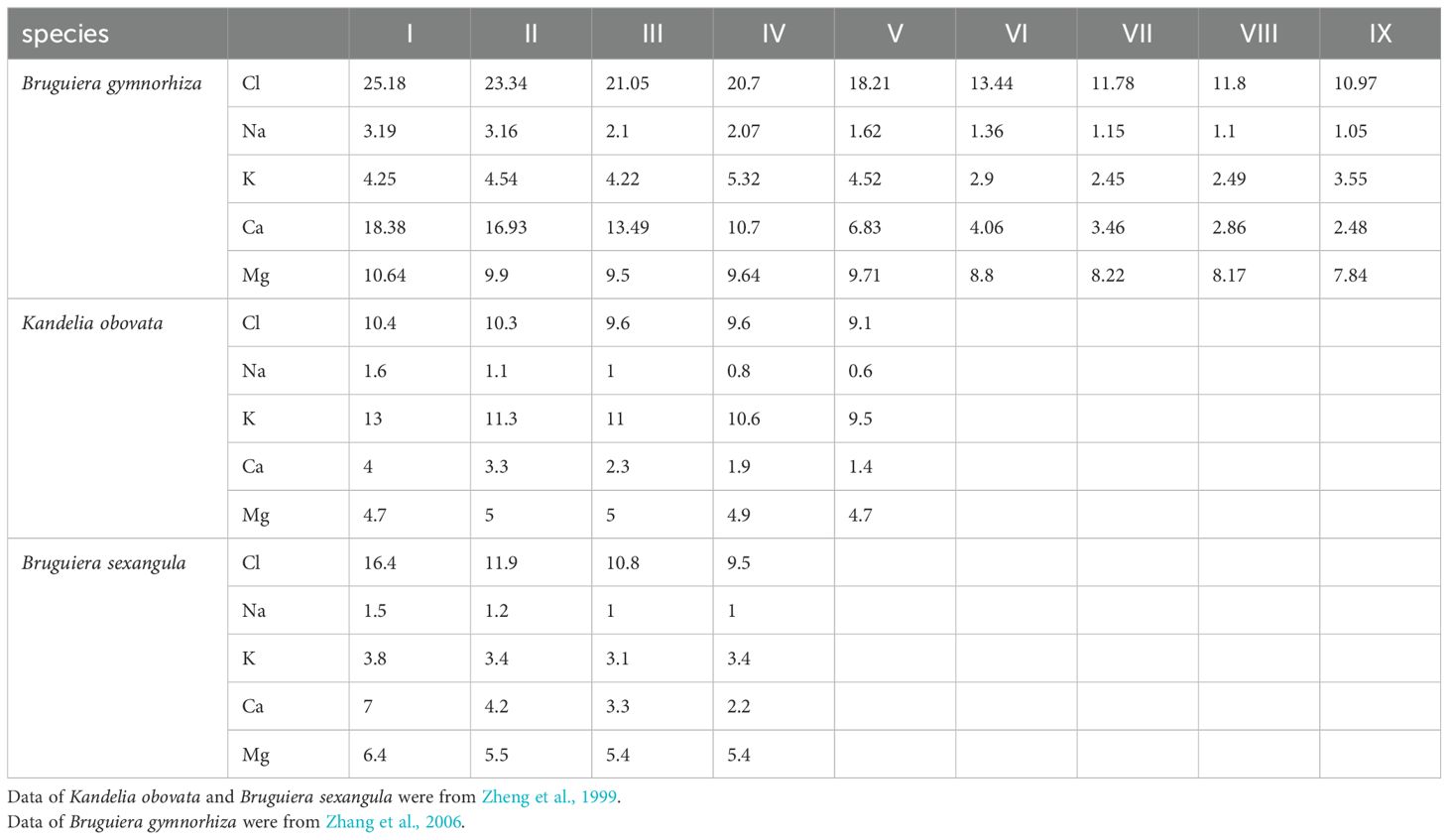
Table 2. Changes in the element contents (mg/g dry weight) of the development stages of propagules of Bruguiera gymnorhiza, Kandelia obovata and Bruguiera sexangula.
4 Discussion
Our findings suggest a rethinking of viviparity as a unique adaptive strategy for mangroves in saltwater environments. While viviparity is typically considered a key mechanism for mangrove adaptation to saline conditions, this study shows that the desalination process is not the sole adaptive factor. The advantage of viviparity lies in prolonging the period of low-salinity protection, providing a new perspective for further understanding the mechanisms by which mangroves adapt to saltwater environments.
One limitation of this study is that it focuses solely on two species of Sonneratia, and therefore the generalizability of the findings to other mangrove species is limited. While S. alba and S. caseolaris represent a range of salinity tolerances, the study does not account for the potential genetic and environmental variations within other mangrove species or populations. Additionally, although we analyzed osmotic potential and elemental composition, other physiological factors, such as antioxidant defense or ion transport, may also contribute to salinity tolerance and warrant further investigation.
The propagule densities of S. alba and S. caseolaris did not vary much during development and were both close to 1 g/cm−3 in the mature stage. Compared with the seawater in the habitat, the denser propagules were able to drift with the current and were mainly distributed downstream, where the water salinity is higher. On the other hand, the propagules of mangrove species with lower density, although capable of floating in seawater, could not remain in the downstream intertidal areas due to their buoyancy. These propagules were more likely to be transported further upstream, where the water salinity was lower and conditions were more favorable for their establishment. This is consistent with the propagule density-dependent distribution (Wang et al., 2019). In this study, Cl- and Na+ of the S. alba propagules were higher than those of the S. caseolaris propagules, indicating that S. alba are better adapted to high salt environments. The differences in the dispersal and physiological adaptations of S. alba and S. caseolaris led to different distribution along the estuary. This has been previously reported by Ball and Pidsley (1995) for the same species in northern Australia.
As mangrove propagule matures, their water content decreases. The water contents of S. alba and S. caseolaris remain at a high level (greater than 70%) during development and are similar to those of viviparous mangrove species such as water content of the mature propagules was greater than 65.08% for B. gymnorhiza (Zhang et al., 2006) and 63.7% for K. obovata (Wang et al., 2002). Regarding the changes in the water content during propagule development, the mangrove Sonneratia did not significantly differ from viviparous plants. Figure 2 shows that the osmotic potential of mature propagules is slightly lower than the osmotic potential of the surrounding seawater. This difference facilitates water absorption from the environment during establishment after detachment from the parent plant, or helps retain moisture during floating. Furthermore, the osmotic potential of mature S. alba propagules is lower than that of S. caseolaris, suggesting that S. alba propagules have a greater tolerance to salinity, which contributes to the distribution differences between the two species.
Vivipary in mangroves has long been recognized as an adaptation to salinity (Joshi, 1933; Hogarth, 1999; Zhou et al., 2016) and a reproductive strategy that facilitates germination on halophytic substrates. It has been proposed that viviparous seedlings exhibit lower salt concentrations than the parent shoot, particularly the leaves (Hogarth, 1999; Zheng et al., 1999; Zhang et al., 2006). This reduction in salt concentration, regardless of its origin, is often interpreted as a mechanism to protect the developing embryo from the harmful effects of high salinity until it matures (Tomlinson, 2016). However, for many halophytes, the salt concentration in propagules is generally lower than in the shoots and leaves (Ungar, 1991). For instance, seedlings of the non-viviparous mangrove Acanthus have been shown to have lower Na and Cl concentrations than adult plants (Hogarth, 1999), which is consistent with our findings that the Na and Cl concentrations in Sonneratia propagules are lower than in the leaves and calyxes. Therefore, the protection of seedlings from premature exposure to high salinity does not appear to be exclusive to viviparous mangroves, suggesting that similar desalination processes may occur in both viviparous and non-viviparous mangroves.
Our results further challenge the traditional view that vivipary is the primary adaptation to high salinity environments in mangroves. While vivipary has been considered a key mechanism for mangrove adaptation to saline habitats, this study shows that non-viviparous mangroves (such as Sonneratia) also undergo a similar desalination process during propagule development, indicating that salt regulation and tolerance are not exclusive to viviparous species. This suggests that vivipary should not be seen as the sole reproductive strategy for coping with saline environments, but rather as part of a broader suite of adaptive mechanisms. This finding compels us to reconsider the role of vivipary in the ecological success of mangroves, as it may function alongside other strategies to promote survival in coastal habitats.
By analyzing the element concentrations (on a dry weight basis) of three viviparous mangrove species, Zheng et al. (1999) concluded that the development of hypocotyls was not a salt accumulation process but rather a desalination process, which may be a sign of atavism, because the salt concentration on a dry weight basis declined with hypocotyl development. Because the Cl- concentration in mature propagules is much lower than that of seawater, it is inferred that the high salinity of mangrove plants is acquired in the environment after detachment from the maternal body. Wang et al. (2002) and Zhang et al. (2006) also reported desalination in propagule development in K. obovata and B. gymnorhiza. The above results are consonant with our observations of the element content dynamics in Sonneratia, of which also indicate a desalination process. In both viviparous and non-viviparous mangrove plants, the propagules desire a low salinity environment seem to favor the propagules development.
In addition, the viviparous species of the genus Avicennia are generally considered the most tolerant of high salinity conditions (Duke, 2006). However, all species of Avicennia exhibit cryptovivipary, and S. alba, one of the most tolerant of high salinity conditions among mangroves (Wang and Wang, 2007; Tomlinson, 2016), exhibits nonvivipary. Smith and Snedaker (1995) suggested that viviparous development under high salinity conditions may afford a higher level of salt tolerance in Rhizophora mangle. It seems that salt-resistance is related to the mangroves’ habitat, but the manner of reproduction is not. Following changes in osmoregulation during the development of K. obovata hypocotyls, Wang et al. (2002) reported no direct correlation between salt tolerance and vivipary. Therefore, the significance of osmotic changes during propagule development to salt tolerance remains debatable.
Assigning viviparous seedling adaptation to a single factor, such as salinity, has proven challenging. Halophytes and species of tropical swamp forests, which do not exhibit vivipary, suggest that this condition is not solely a response to either salinity or wet soil (Tomlinson, 2016). Juncosa (1982) proposed that vivipary is a consequence of the amplification of the normal torpedo stage of embryo development due to the extended growth of the hypocotyl. Clarke et al. (2001) examined the dispersal potential and early growth traits of 14 tropical mangrove species and found that viviparous and non-viviparous species exhibited similar buoyancy, seed weight, root and shoot initiation rates, early growth, and salinity tolerance. Furthermore, Sousa et al. (2007) observed that viviparous propagules were more easily obstructed by marker replenishment and did not disperse as widely as non-viviparous propagules, which limited their dispersal. These findings suggest that the hypothesis of vivipary being a specific adaptation to salinity among mangroves is not well-supported. Instead, vivipary should not be considered a distinct reproductive strategy to cope with saline habitats. Our results indicate that the development processes of both viviparous and non-viviparous mangrove propagules show convergent evolution in terms of salt tolerance and osmotic regulation, challenging the traditional view of vivipary as the primary adaptation to saltwater environments. Thus, the significance of vivipary in mangroves warrants a reconsideration, as it may involve a broader set of adaptive strategies beyond salinity tolerance.
Future research should focus on investigating the prolonged low-salinity protection provided by vivipary during early development and explore other ecological factors influencing propagule survival and dispersal. Additionally, comparative studies on the genetic and physiological basis of reproductive strategies in both viviparous and non-viviparous species could provide deeper insights into the evolutionary significance of these adaptations in mangroves.
In summary, our findings suggest that vivipary may not be solely defined as a definitive adaptation for mangroves to survive in saltwater habitats. While vivipary has traditionally been viewed as providing an advantage in high salinity conditions, our results, along with previous studies, indicate that other factors such as propagule density, osmotic potential, and elemental composition also play significant roles in mangrove survival and distribution. The convergent evolution between viviparous and non-viviparous mangrove propagules in terms of osmotic regulation and salinity tolerance challenges the traditional view of vivipary as the primary adaptation to saltwater habitats. This raises important questions about the broader ecological and evolutionary significance of vivipary in mangroves. Further research focusing on the prolonged low-salinity protection provided by vivipary during early development, as well as other ecological and physiological factors, could offer deeper insights into the adaptive strategies of mangrove species and potentially reshape our understanding of the role of vivipary in mangrove ecosystems.
Data availability statement
The original contributions presented in the study are publicly available. This data can be found here: https://doi.org/10.5281/zenodo.10260188.
Author contributions
CL: Conceptualization, Data curation, Formal analysis, Methodology, Project administration, Software, Visualization, Writing – original draft. LZ: Conceptualization, Methodology, Software, Writing – review & editing. XS: Conceptualization, Investigation, Methodology, Writing – review & editing. YT: Conceptualization, Investigation, Methodology, Writing – review & editing. MW: Conceptualization, Funding acquisition, Supervision, Validation, Writing – review & editing. WW: Conceptualization, Funding acquisition, Methodology, Project administration, Resources, Supervision, Validation, Writing – review & editing.
Funding
The author(s) declare financial support was received for the research, authorship, and/or publication of this article. This study was supported by the National Natural Science Foundation of China (30200031, 40776046).
Conflict of interest
The authors declare that the research was conducted in the absence of any commercial or financial relationships that could be construed as a potential conflict of interest.
Publisher’s note
All claims expressed in this article are solely those of the authors and do not necessarily represent those of their affiliated organizations, or those of the publisher, the editors and the reviewers. Any product that may be evaluated in this article, or claim that may be made by its manufacturer, is not guaranteed or endorsed by the publisher.
References
Ball M. C., Pidsley S. M. (1995). Growth responses to salinity in relation to distribution of two mangrove species, Sonneratia alba and S. lanceolata in northern Australia. Funct. Ecol. 9, 77–85. doi: 10.2307/2390093
Clarke P. J., Kerrigan R. A., Westphal C. J. (2001). Dispersal potential and early growth in 14 tropical mangroves: do early life history traits correlate with patterns of adult distribution? J. Ecol. 89, 648–659. doi: 10.1046/j.0022-0477.2001.00584.x
Crase B., Liedloff A., Vesk P. A., Burgman M. A., Wintle B. A. (2013). Hydroperiod is the main driver of the spatial pattern of dominance in mangrove communities. Glob. Ecol. Biogeogr. 22, 806–817. doi: 10.1111/geb.12063
Elmqvist T., Cox P. A. (1996). The evolution of vivipary in flowering plants. Oikos 77, 3–9. doi: 10.2307/3545579
Farnsworth E. J. (2000). The ecology and physiology of viviparous and recalcitrant seeds. Annu. Rev. Ecol. Syst. 31, 107–138. doi: 10.1146/annurev.ecolsys.31.1.107
Farnsworth E. J., Farrant J. M. (1998). Reductions in abscisic acid are linked with viviparous reproduction in mangroves. Am. J. Bot. 85, 760–769. doi: 10.2307/2446410
Guo Z., Xu S., Xie W., Shao S., Feng X., He Z., et al. (2022). Adaptation to a new environment with pre-adaptive genomic features - Evidence from woody plants colonizing the land–sea interface. Plant J. 111, 1411–1424. doi: 10.1111/tpj.15899
Hasegawa A., Oyanagi T., Minagawa R., Fujii Y., Sasamoto H. (2014). An inverse relationship between allelopathic activity and salt tolerance in suspension cultures of three mangrove species, Sonneratia alba, S. caseolaris and S. ovata: development of a bioassay method for allelopathy, the protoplast co-culture method. J. Plant Res. 127, 755–761. doi: 10.1007/s10265-014-0651-1
Joshi A. C. (1933). A suggested explanation of the prevalence of vivipary on the sea–shore. J. Ecol. 21, 209–212. doi: 10.2307/2255879
Juncosa A. M. (1982). Developmental morphology of the embryo and seedling of Rhizophora mangle L. (Rhizophoraceae). Am. J. Bot. 69, 1599–1611. doi: 10.2307/2442915
Krauss K. W., Doyle T. W., Twilley R. R., Rivera-Monroy V. H., Sullivan J. K. (2006). Evaluating the relative contributions of hydroperiod and soil fertility on growth of south Florida mangroves. Hydrobiologia 569, 311–324. doi: 10.1007/s10750-006-0139-7
Leong R. C., Friess D. A., Crase B., Lee W. K., Webb E. L. (2018). High-resolution pattern of mangrove species distribution is controlled by surface elevation. Estuar. Coast. Shelf. S. 202, 185–192. doi: 10.1016/j.ecss.2017.12.015
Liang S., Zhou R., Dong S., Shi S. (2008). Adaptation to salinity in mangroves: implication on the evolution of salt-tolerance. Sci. Bull. 53, 1708–1715. doi: 10.1007/s11434-008-0221-9
R Core Team (2020). R: a language and environment for statistical computing (Vienna: R for Statistical Computing). Available at: https://www.r-project.org (Accessed December 01, 2023)
Smith S. M., Snedaker S. C. (1995). Salinity response in two populations of viviparous Rhizophora mangle L. seedlings. Biotropica 27, 435–440. doi: 10.2307/2388955
Sousa W. P., Kennedy P. G., Mitchell B. J., Ordóñez L. M. O. (2007). Supply-side ecology in mangroves: Do propagule dispersal and seedling establishment explain forest structure? Ecol. Monogr. 77, 53–76. doi: 10.1890/05-1935
Van der Stocken T., Wee A. K. S., De Ryck D. J. R., Vanschoenwinkel B., Friess D. A., Dahdouh-Guebas F., et al. (2019). A general framework for propagule dispersal in mangroves. Biol. Rev. 94, 1547–1575. doi: 10.1111/brv.12514
Wang W., Li X., Wang M. (2019). Propagule dispersal determines mangrove zonation at intertidal and estuarine scales. Forests 10, 245. doi: 10.3390/f10030245
Wang W. Q., Ke L., Tam N. E. Y., Wong Y. S. (20022002). Changes in the main osmotica during the development of Kandelia candel hypocotyls and after mature hypocotyls were transplanted in solutions with different salinities. Mar. Biol. 141, 1029–1034. doi: 10.1007/s00227-002-0951-1
Xu L., Wang M., Xin C., Liu C., Wang W. (2020). Mangrove distribution in relation to seasonal water salinity and ion compartmentation: a field study along a freshwaterdominated river. Hydrobiologia 847, 549–561. doi: 10.1007/s10750-019-04119-7
Zhang Y. H., Wang W. Q., Chi M. J., Lin P. (2006). Development of viviparous hypocotyls of Bruguiera gymnorrhiza. Acta Oceanol. Sin. 28, 121–127.
Zheng W. J., Wang W. Q., Lin P. (1999). Dynamics of element contents during the development of hypocotyls and leaves of certain mangrove species. J. Exp. Mar. Biol. Ecol. 233, 247–257. doi: 10.1016/S0022-0981(98)00131-2
Zhou X. X., Cai L. L., Fu M. P., Hong L. W., Shen Y. J., Li Q. Q. (2016). Progress in the studies of vivipary in mangrove plants. Chin. J. Plant Ecol. 40, 1328–1343. doi: 10.17521/cjpe.2016.0087
Keywords: Sonneratia, nonvivipary, vivipary, developmental process, propagules, osmoregulation
Citation: Liu C, Zhang L, Shi X, Tang Y, Wang M and Wang W (2025) Element contents changes during the propagule development of two Sonneratia species. Front. Mar. Sci. 11:1430782. doi: 10.3389/fmars.2024.1430782
Received: 10 May 2024; Accepted: 29 November 2024;
Published: 07 January 2025.
Edited by:
Renato Crespo Pereira, Fluminense Federal University, BrazilReviewed by:
Yelin Huang, Sun Yat-sen University, ChinaSuhua Shi, Sun Yat-sen University, China
Jinwang Wang, Zhejiang Institute of Subtropical Crops, China
Copyright © 2025 Liu, Zhang, Shi, Tang, Wang and Wang. This is an open-access article distributed under the terms of the Creative Commons Attribution License (CC BY). The use, distribution or reproduction in other forums is permitted, provided the original author(s) and the copyright owner(s) are credited and that the original publication in this journal is cited, in accordance with accepted academic practice. No use, distribution or reproduction is permitted which does not comply with these terms.
*Correspondence: Wenqing Wang, bWFuZ3JvdmVzQHhtdS5lZHUuY24=