- 1School of Art and Design, Henan University of Science and Technology, Luoyang, China
- 2College of Horticulture and Plant Protection, Henan University of Science and Technology, Luoyang, China
- 3Department of Material Science and Engineering, School of Engineering and Applied Science, University of Pennsylvania, Philadelphia, PA, United States
Blue carbon refers to the carbon fixed in marine ecosystems such as mangroves, salt marshes, and seagrass beds. Considered a treasure house for capturing and storing carbon dioxide, it can alleviate environmental issues linked to climate change and positively influence the environments where people live. Thus, to clarify the hotspots and development trends of blue carbon research, bibliometric analysis incorporating ScientoPy and VOSviewer software were used to quantitatively analyze 4,604 blue carbon publications from Web of Science and Scopus databases between 1993 and 2023. The results indicate a rapidly growing number of published studies on blue carbon, with blue carbon research being multifaceted and gradually becoming an interdisciplinary and international topic. This study on blue carbon, which is based on keyword clustering analysis, comprises three stages. The analysis of the strength of the cooperative connections between scholars in various countries who have published work on blue carbon. found that the cooperation networks of developed countries are strong and those of developing countries are relatively weak. Quantitative trend analysis reveals a growing focus on the restoration and conservation of blue carbon ecosystems, with remote sensing being the predominant technology used in the blue carbon research field in recent years. In blue carbon research, increasing carbon sequestration capacity, climate change mitigation, and carbon sequestration in macroalgae remain potential hotspots for research and development.
1 Introduction
Blue carbon sequestration refers to the absorption and storage of atmospheric carbon dioxide by marine and coastal ecosystems, including marine organisms. Blue carbon ecosystems (BCEs), specifically mangroves, salt marshes, and seagrasses, capture and store carbon in marine sediments and contribute to climate change mitigation (Thomas, 2014). Furthermore, they provide vital ecosystem services, including food supply, coastal protection, biodiversity maintenance, water filtration, and cultural values (Bano et al., 1997; Young et al., 2021; Lester et al., 2020). However, the aforementioned ecosystems are facing various threats due to pollution, sea level rise, ocean acidification owing to climate change, overexploitation of coastal areas, overfishing, and land use change. These threats may cause BCEs to lose their ability to absorb and store carbon; they could even release a large amount of stored carbon due to BCE disturbances and losses, which could significantly affect the global carbon cycle and climate system. The release of this carbon would exacerbate global warming, thus triggering a series of chain reactions such as sea level rise and climate model changes (Cameron et al., 2021; Chuan et al., 2020; Perera et al., 2018; Duarte et al., 2005). Despite numerous efforts to conserve and manage BCEs including greenhouse gas emission reductions, water quality improvements, restoration of mangroves, salt marshes and seagrass beds, and the development of supportive policies and legislation (Ullman et al., 2012; Choi et al., 2022) these measures remain insufficient to counter the threats they face. Consequently, blue carbon research is attracting global attention.
The value of BCEs is becoming increasingly prominent in the context of global climate change. Although “blue carbon” as a unified concept is a rather recent conception, research on carbon storage in coastal wetlands and seagrass beds began as early as the middle or late 20th century. In the 1970s and 1980s, scientists began to study the role of mangroves, salt marshes, and seagrass beds in the carbon cycle, including their levels of productivity and organic carbon storage capacity. For example, in 1977, Beer, S. and other scientists considered HCO-3 to be the major carbon source for photosynthesis in seagrasses (Beer et al., 1977). In 1993, Pollard, P. C et al. identified seagrasses as the most important primary producer, providing most of the organic carbon for higher trophic groups (Pollard and Kogure, 1993; Mukai and Iijima, 1995). The presence of organic matter in mangroves, seagrass beds, and salt marshes makes these coastal ecosystems a important component of the global carbon cycle (Farnsworth et al., 1996; Clough, 1995; Middelburg et al., 1996; Bouillon et al., 2003; Bouillon and Connolly, 2009; Reef and Lovelock, 2014). In 2009, blue carbon was first proposed in a joint report of the United Nations Environment Program; Food and Agriculture Organization of the United Nations (FAO); and Intergovernmental Oceanographic Commission of the United Nations Educational, Scientific and Cultural Organization (IOC–UNESCO). Blue carbon research has steadily advanced, with studies focusing on assessing the sequestration value of BCEs and enhancing scientific knowledge to optimize carbon storage (Mcleod et al., 2011). Moreover, some studies have investigated blue carbon–climate change relationships for climate change mitigation (Buditama, 2016; Kelleway et al., 2016). Studies exploring factors influencing BCE conservation and management, such as biological invasion, habitat characteristics, and human activities, exist (Theuerkauf et al., 2017; Atwood et al., 2018). Furthermore, researchers have pursued more precise data on blue carbon to improve monitoring and support targeted conservation and management strategies (Mitra and Zaman, 2015), including innovations in ecosystem restoration technologies and research on policy and economic incentives (Howard et al., 2017; Renzi et al., 2019; Macreadie et al., 2019). The multifaceted nature of the blue carbon concept has also led to unprecedented interdisciplinary collaborative research to advance the goal of blue carbon codevelopment (Lovelock and Duarte, 2019; Krause-Jensen et al., 2018). In March 2023, according to the meeting summary of the “Earth Negotiations Bulletin” on solutions to marine challenges, the role of marine ecosystem restoration in achieving sustainable development goals was emphasized, and the current global marine ecological environment was discussed. Challenges such as biodiversity loss, climate change, land-based pollution, marine debris, and microplastic pollution were identified (Eyre et al., 2023; Pétillon et al., 2023). Despite active involvement from scientists, policymakers, and research institutions domestically and internationally, the complex and unique nature of blue carbon has left various areas under-researched, thus necessitating further study and breakthroughs (Coleman, 2023; Mack et al., 2022; Locatelli et al., 2014). Therefore, a thorough analysis and review of the existing literature on blue carbon research is necessary.
A bibliometric review is a methodology-based literature review. It uses bibliometrics to quantitatively describe, evaluate, and monitor data and can provide reasonable objective results through technical and scientific mapping analyses (Pallis, 2019). Technical analysis reveals contributions categorized by region, discipline, journal, publication, and author information (Lyu et al., 2023; Ninkov et al., 2022; Yu et al., 2024). Meanwhile, scientific mapping clarifies the links between topics in a research field, thereby revealing the knowledge structure and development process of blue carbon; it analyzes published literature to assess key topics and trends in the research field (Quevedo et al., 2023; Costa and Macreadie, 2022; Jiang et al., 2022). In this study, ScientoPy (V.2.1.3) and VOSviewer (version 1.6.17) were used to collect 4,604 valid blue carbon studies published in the two databases of Web of Science and Scopus over the past 30 years. Thus, bibliometric scores were obtained, and reasonable objective results were provided. The specific objectives are as follows: 1) to study the spatiotemporal distribution of blue carbon and the contributions of journals, disciplines, and authors; 2) to reveal the theme network and knowledge framework of blue carbon research in the past 30 years; and 3) to explain the evolution and trend of regions researching blue carbon hotspots and keywords of hotspots. The research findings offer a comprehensive and deep understanding of development trends in the field of blue carbon research. Expected to inform future studies and decision making, this analysis offers robust support and guidance by focusing on the evolution of research priorities and hotspots.
2 Methods
2.1 Data retrieval and processing
We used the Web of Science and Scopus databases as data sources for the literature search. To obtain accurate records and retrieve the search, we set the following parameters in the Web of Science Core journal (hereinafter referred to as WOS). ((TS=(“blue carbon”)) OR TS=(“mangrove*” OR “salt marsh*” OR “saltmarsh*” OR “seagrass*” OR “sea grass*”)) AND TS=(“carbon sink” OR “carbon cycle*” OR “carbon emission*” OR “blue carbon*” OR “carbon budget*” OR “carbon sequest*” OR “carbon storage*” OR “carbon pool*” OR “carbon burial” OR “carbon stock*” OR “carbon accumulation*” OR “carbon source”). The date range was set from “1993-01-01” to “2023-11-30,” and 3,301 related papers were retrieved by the index date. The following parameters were set in the Scopus database to retrieve the search: title, abstract, keyword search (“blue carbon”) OR (“mangrove*” OR “salt marsh*” OR “saltmarsh*” OR “sea grass*” OR “seagrass*”) AND (“carbon sink” OR “carbon cycle*” OR “carbon emission*” OR “blue carbon*” OR “carbon budget*” OR “carbon sequest*” OR “carbon storage*” OR “carbon pool*” OR “carbon burial” OR “carbon stock*” OR “carbon accumulation*” OR “carbon source”). The date range was set from “1993” to date, and 4,291 related papers were retrieved. In total, 7,592 articles were collected from WOS and Scopus databases.
To obtain scientific and effective data, ScientoPy preprocessing step technology was used to improve the readability and accuracy of the literature analysis (Table 1, Ruiz-Rosero et al., 2019). Preprocessing eliminated 395 unusable articles, leaving 7,197 viable ones 3,232 and 3,965 from the WOS and Scopus databases, respectively. Given the overlap between the two databases, ScientoPy’s duplicate removal filter reduced the total to 4,604 available documents 3,066 from WOS and 1,538 from Scopus. Subsequently, we conducted further analysis on these 4,604 documents.
To verify data accuracy, we further placed the data in EndNote X9 and performed a deduplication process. The data obtained are consistent with the ScientoPy data.
2.2 Technical performance analysis
In this study, the growth and growth trends of blue carbon literature published from 1993 to 2023 were analyzed using the ScientoPy (2.1.3) version to calculate the annual number of published documents. Similar methods were used to analyze the contributions of journals, disciplines, and authors to blue carbon.
2.3 Scientific mapping analysis
This study employed keyword and term co-occurrence analysis to reveal the evolution of hot topics in blue carbon research and their correlation with subfields. Specifically, keyword co-occurrence analysis aims to identify the most popular blue carbon and its internal relations, while term co-occurrence analysis aims to show the evolution of research priorities in blue carbon research by creating a bibliometric map of frequently used terms in the text.
2.3.1 Keyword preprocessing and term extraction
The keywords of the collected 4,604 papers were preprocessed. To accurately identify the content of each publication, we selected author keywords as the analysis target. Furthermore, all keywords were extracted using VOSviewer software (van Eck and Waltman, 2017). Keywords appearing more than 25 times were chosen for subsequent analysis. Of these, 509 keywords related to blue carbon were manually selected and retained for further analysis.
2.3.2 Co-occurrence analysis of keywords and terms
This study used VOSviewer software to calculate and collect 4,604 papers, extract the number and average time of author keywords, and analyze the co-occurrence of keywords. Co-occurrence is the number of occurrences of two terms in the same paper, and the average occurrence time of a term is the average publication time of the document containing the term. For keyword co-occurrence analysis, VOSviewer was used to calculate the number and average time of author keywords extracted from the 4,604 papers collected. Moreover, a bibliometric network of selected terms based on term co-occurrence analysis was created. Through network visualization, the relationship between selected keywords and research hotspots over time was demonstrated and clarified.
2.4 Trend analysis
2.4.1 Average growth rate
To elucidate the development trends in blue carbon research across countries, journals, disciplines, and authors, we used an equation to calculate the average growth rate (AGR) of publication.
where Ys and Ye denote the beginning and ending years of the selected period, respectively, and Pi represents the publication number of year i. For one object, the rising trend attribute must have a higher release growth rate, which can be reflected by the AGR.
2.4.2 Percentage of literature in recent years
Through the study of recent literature percentage, the hot journals, disciplines, and keywords of blue carbon-related research in recent years are calculated using the following equation.
where PDLY is the percentage of recent literature. Ye = end of year; Ys = the beginning of the year, the calculation method is the equation Ys = Ye−(WindowWidth + 1); = number of publications on year 1; and TND = total number of literatures. During the calculation, we set the WindowWidth to 5.
ScientoPy is used to calculate the yearly number of published documents and analyze the attention and contribution of each country to blue carbon. Taking the time axis as the main line, the trend analysis was performed based on keywords to reveal the evolution of the theme of blue carbon research.
3 Results and discussion
3.1 Overview of the blue carbon research field
3.1.1 Analysis of the number of published papers on blue carbon
We found that the total number of blue carbon-related studies in the two databases of WOS and Scopus increased rapidly, from 3 in 1993 to 734 in 2023 (Figure 1). In 2009, after the United Nations released “Blue Carbon”: An Assessment Report on the Carbon Sequestration Effect of a Healthy Ocean,” the academic term “blue carbon” officially appeared in the public eye. Subsequently, in August 2011, the systematic research literature on blue carbon first appeared in the collection of WOS core journals, and the research heat on blue carbon continued to rise. In 2012, the 18th United Nations Climate Change Conference, the United Nations Conference on Sustainable Development, and other gatherings by international organizations were held to promote the further development of the “blue carbon “ policy plan. These summits emphasized the importance of marine and wetland ecosystems for the absorption and storage of atmospheric carbon dioxide and promoted the increase in blue carbon stocks through its conservation and restoration. The following year, the number of blue carbon papers increased significantly, with a total of 130 papers, a year-on-year (Yoy) growth rate of 64.47%. In 2018, the Intergovernmental Panel on Climate Change “Special Report on Global Warming of 1.5°C” highlighted the need for zero global CO2 emissions by the mid-21st century. In the same year, the literature on blue carbon research surged once again, with 365 articles published, and the (Yoy) growth rate was 54.66%. By 2023, the number of blue carbon studies reached 734, with the popularity and importance of blue carbon research on a global scale increasing.
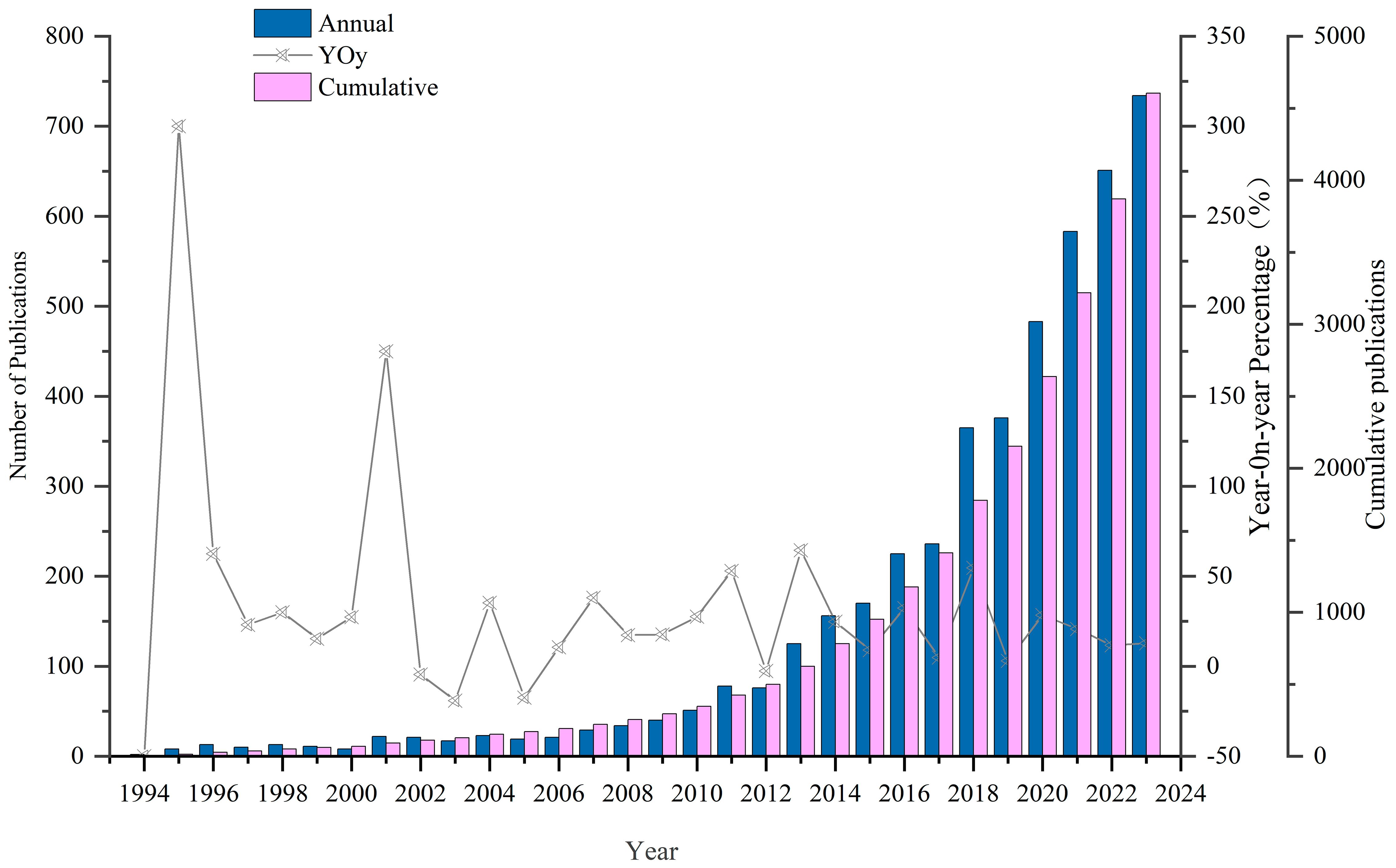
Figure 1. Evolution of blue carbon-related publications in Web of Science and Scopus database (Year-on-year percentage= (current value-the same period of last year)/the same period of last year) * 100%).
3.1.2 Contributions by journal
The contributions of journals related to blue carbon were evaluated using the publication count. Over the past 30 years, the number of journals publishing blue carbon-related content has expanded significantly, increasing from only 2 journals to 995. Figure 2 depicts the top 15 journals with blue carbon publications. Science of the Total Environment has published the most relevant blue carbon studies, totaling 197 articles, followed by Frontiers in Marine Science with 158 articles, Estuarine Coastal and Shelf Science with 139 articles, Limnology and Oceanography with 82 articles, and Marine Ecology Progress Series with 80 articles. Among them, approximately 90% of the blue carbon literature in the Remote Sensing magazine was published within the past five years, underscoring the active application of remote sensing technology in blue carbon research. Although the total number of blue carbon-related literature in the two journals of Forests and Ecological Indicators is not large, approximately 85% of this literature was published within the past five years, suggesting a growing emphasis on blue carbon research within these journals in recent years. Furthermore, notable contributions to recent blue carbon studies have emerged in prestigious publications such as Science and Nature journals, which played a leading role in advancing the blue carbon research field to some extent. The number of research journals dedicated to blue carbon continues to increase, providing an expanding platform for researchers to disseminate their latest findings and engage in academic discourse with their global counterparts. These journals contribute markedly to promoting scientific development and practical applications in the blue carbon field by promoting research cooperation, knowledge dissemination, and policy formulation.
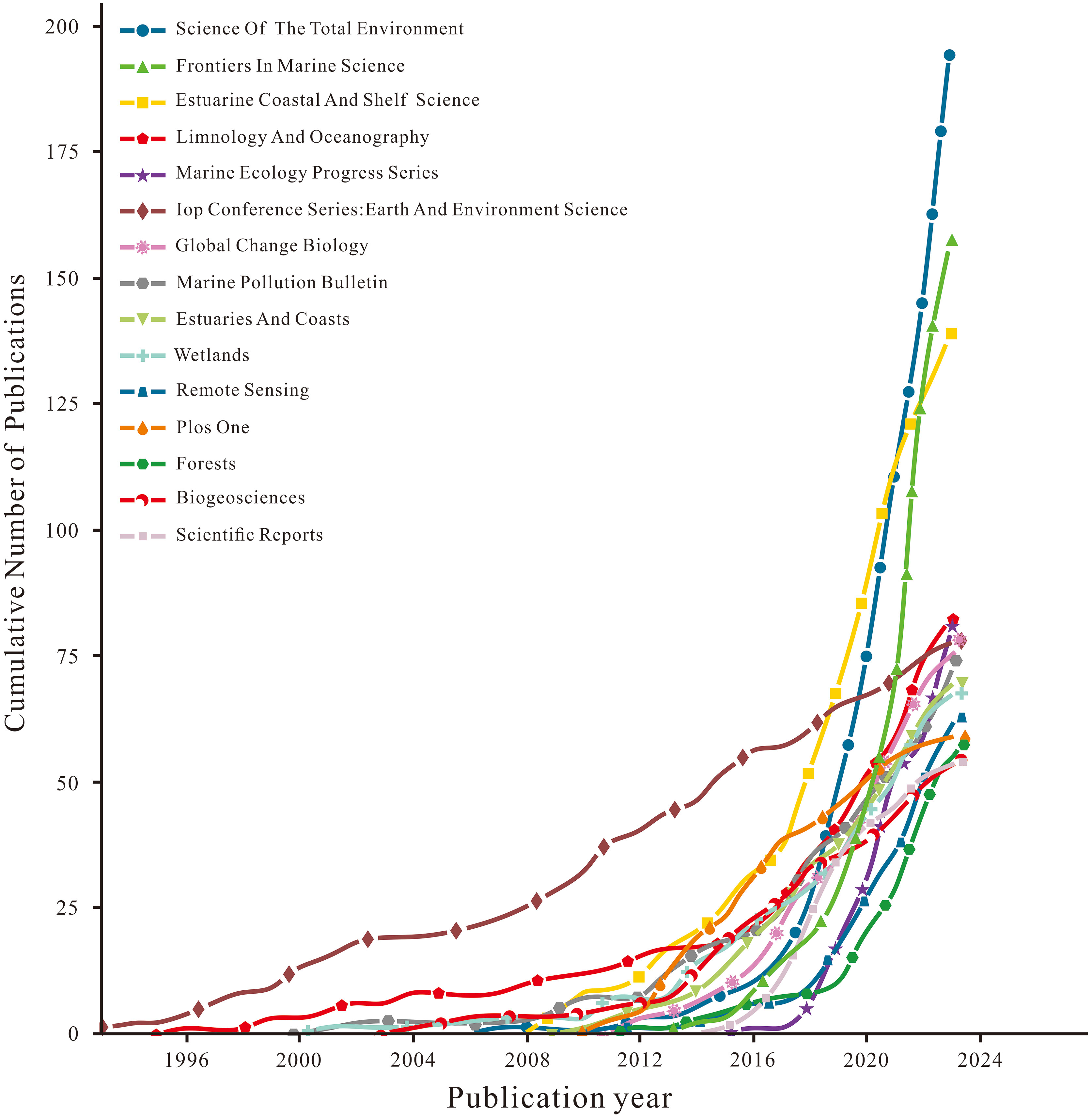
Figure 2. Cumulative number of BCE publications in the top 15 journals publishing BCE manuscripts between 1993 and 2023.
3.1.3 Contribution by discipline
The research disciplines involved in blue carbon have developed from 10 disciplines in 2009 to 51 disciplines in 2023, covering different aspects, including Environmental Sciences & Ecology; Marine & Freshwater Biology; Oceanography Geology (Donato et al., 2011; Ouyang et al., 2017; Zhu et al., 2020); Geology, Remote Sensing (Pham et al., 2019a; Zheng et al., 2019; Hawman et al., 2023; Navarro et al., 2021); Public, Environmental & Occupational Health (Tong et al., 2011; Ray et al., 2012; Lai et al., 2022; Miyamoto et al., 2023); Energy & Fuels (Yong et al., 2022; Zhao et al., 2022); Optics (Munir and Wicaksono, 2019; Svane et al., 2022; Stovall et al., 2020); and Business & Economics (Jakovac et al., 2020; Riegel et al., 2023; Tanner et al., 2019). This trend suggests that blue carbon research is diversifying across disciplines, evolving into a multidisciplinary topic of study. The number of papers published in the top 15 disciplines related to blue carbon in the past 30 years is shown in Figure 3. The most prominent subject concerning blue carbon is Environmental Sciences & Ecology, with 1,780 blue carbon publications. Notably, 69.2% of these papers were published in the past five years. Following closely are Marine & Freshwater Biology with 709 papers and Oceanography with 383 papers, and 66% and 61% of these papers, respectively, were published in the past five years. Furthermore, the observed 74% of blue carbon literature in Remote Sensing and Imaging Science & Photographic Technology published in the past five years indicates the great potential of these disciplines in advancing blue carbon research (Barenblitt et al., 2023). These two disciplines have contributed markedly to the effective evaluation of blue carbon research (Tran et al., 2022). Simultaneously, from the extensive performance of the discipline of blue carbon research and with the deepening and expansion of blue carbon research, its interdisciplinary nature has become increasingly significant. Moreover, increased disciplines will be involved in blue carbon research, which has become an interdisciplinary and multidisciplinary research topic.
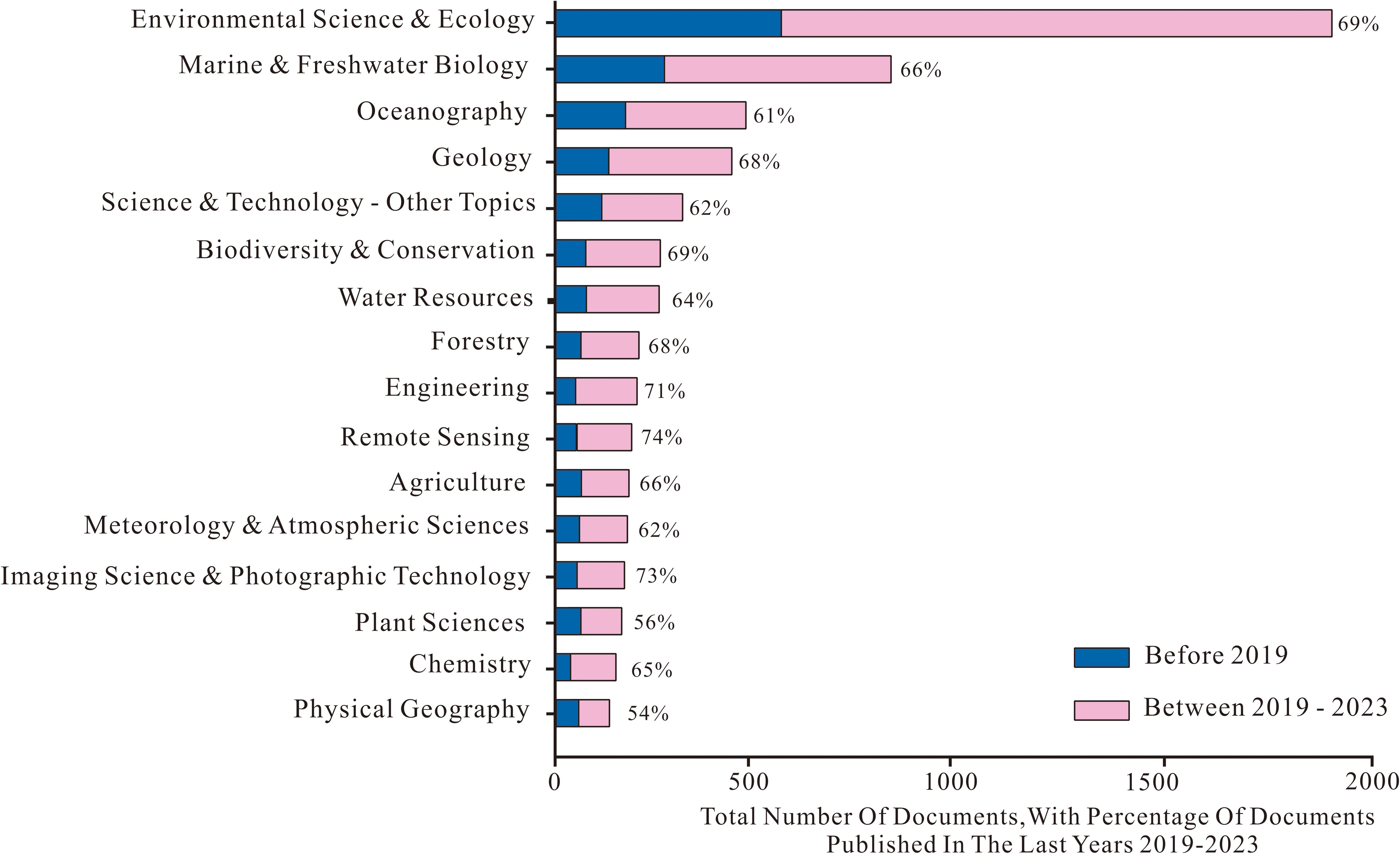
Figure 3. Total number of documents and percentage of documents of the top 15 disciplines in blue carbon-related research.
3.1.4 Contribution by author
The cooperation networks of the most effective researchers and authors were further analyzed. Duarte, C.M. has the most published and coauthored literature, with 85 papers. The second is Macreadie, P.I., with 81 papers, and the third is Lovelock, C.E., with 72 papers. Macreadie, P.I and Lovelock, C.E. from Australia have studied BCEs in various aspects, such as the climate solutions of blue carbon, ecosystem restoration, and the value and management measures of blue carbon (Macreadie et al., 2022; Serrano et al., 2019; Macreadie et al., 2017b). Duarte, C.M. from Spain studied and evaluated seagrass carbon sink capacity, blue carbon accounting, and climate change impacts on seagrass meadows and macroalgal forests (Duarte, 2017; Duarte et al., 2013). The author cooperation network diagram shows that 7 of the top 10 authors are from Australia, consistent with Australia’s high research output.
By using VOSviewer, the cooperative connection strength of all authors in the blue carbon literature was analyzed. The selection criterion was set to include authors who had published 20 or more articles. The findings showed that 60 out of 14,023 authors met this criterion and were subsequently analyzed (Figure 4). The strongest collaborator was found to be Duarte, C. M. of Spain, with the largest number of connections and wide strength. The author’s cooperative relationship diagram shows a close cooperative relationship between Macreadie, P. I., Duarte, C. M., Serrano, O., Lovelock, C. E., and Friess, D. A. The analysis revealed a strong collaboration network among developed countries (e.g., Australia, the United States, and Spain). Although Chinese authors had a large number of researchers and published literature in the field of blue carbon and close domestic cooperation, the number and intensity of international cooperation networks were limited. In addition, two authors in Brazil and one author in Indonesia, which were located on the upper left, also had this problem. Through in-depth analysis of these cooperation networks, it was found that the cooperation networks of developed countries were strong, and those of developing countries were relatively weak. Developing countries should take active measures to enhance blue carbon’s international cooperation opportunities. Blue carbon development requires global exchange and cooperation. Countries can share experience and technological progress and strengthen international cooperation in blue carbon projects to promote the sustainable development of global blue carbon.
3.2 Research process of blue carbon: three typical stages
A total of 406 most relevant terms were collected from the titles, keywords, and abstracts of the publications, thus forming a co-occurrence network. The research focused on dense areas in the network. Further analysis of these areas found that the dense areas are primarily centered on three clusters in Figure 5A. The occurrence time is indicated by the color in Figure 5B. Furthermore, the analysis and interpretation of the co-occurrence network revealed that blue carbon is divided into three stages.
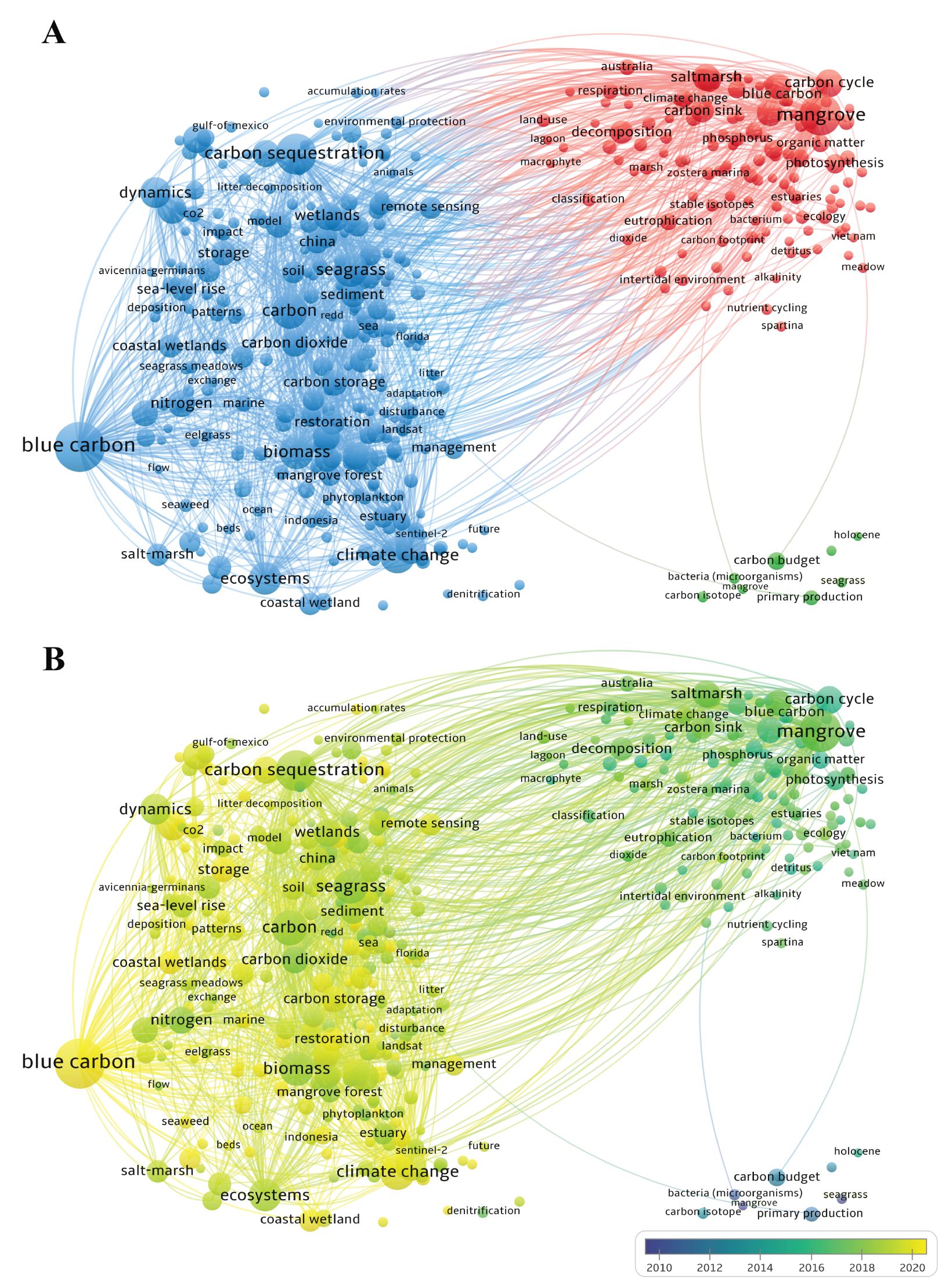
Figure 5. Co-occurrence network map of frequent terms in titles, keywords, and abstracts (A) Diagram of keyword sharing(the size of the nodes reflects the number of occurrences and the color represents different clusters classified according to co-occurrence analysis). (B) Graph of co-occurrence of keywords with respect to time.
3.2.1 Stage 1
In the first stage (the blue cluster of Figure 5B), primarily from 1993 to 2009, the co-occurrence network shows that the terms “carbon budget,” and “carbon isotope” appear quite early and are closely related to terms such as “mangrove” and “seagrass.” During this period, the research content was mainly to evaluate the carbon storage in marine ecosystems, such as mangroves and seagrass beds; estimate the carbon absorption and storage of these ecosystems through measurement and monitoring; and understand the carbon cycle mechanism in marine ecosystems, including carbon input, transformation, and output processes, and the role of ecosystems in them. For example, the relationships between photosynthetic rate data and irradiance, stand structure, and stable isotope analysis are used to understand and evaluate the storage and flow of blue carbon in ecosystems (Clough, 1998; Peña et al., 1999). In terms of research methodology, scientific methods, such as field observation and monitoring, biological sample analysis, and ecological models, are often used (Smit et al., 2005; Bouillon and Boschker, 2006).
During this period, the content and methods of blue carbon research were relatively simple, primarily focusing on the preliminary understanding of carbon storage and ecosystem functions of mangroves and seagrass beds (Duarte et al., 2005; Lovelock, 2008). With the deepening of the understanding of blue carbon, the attention gradually increased in subsequent research.
3.2.2 Stage 2
The second stage is shown in the green clustering in Figure 5B. From 2009 to 2017, the co-occurrence network reveals that the most frequently used terms in this stage include “mangrove,” “blue carbon,” “carbon cycle,” “carbon sink,” and “salt marsh.” As shown in the light green and green project node groups in Figure 5B, the most studied term is “mangrove” in BCEs. As the concept of “blue carbon” was put forward in 2009, the content and technology of blue carbon-related research are also gradually expanding.
As a key BCE component, mangroves are forests with high carbon sequestration efficiency. Compared with the first stage, the number, content breadth, and depth of mangrove literature research in this stage have obvious changes. The frequencies of keywords such as “carbon sequestration,” “carbon sink,” and “carbon storage” related to mangroves in BCEs exceed those of other keywords (the cluster in Figure 5B). Increasing carbon sinks, estimating carbon storage, and understanding the carbon cycle remain the focus of research during this period (Lunstrum and Chen, 2014; Peng et al., 2016). For example, due to the high carbon sequestration potential of mangroves, several countries have launched mangrove reforestation and afforestation plans to balance the needs of human development and the conservation of mangrove ecosystems (Richards and Friess, 2017). Studying the adaptability of mangrove ecosystems to climate change and the effects of climate change on their distribution, growth, and function can aid in formulating strategies for protecting and managing mangroves (Castillo et al., 2017; Estrada et al., 2015; Escobedo et al., 2010; Beaumont et al., 2014). Furthermore, studies have shown that mangroves provide habitats for a variety of animals and plants and participate in complex food webs. The maintenance of biodiversity plays an important role in ecosystem stability and climate regulation (Kulawardhana et al., 2015; Tu et al., 2015; Tokoro et al., 2014). Mangroves play a key role in biodiversity conservation, and their ecosystem services are critical to ecosystem stability, including climate regulation. The research results produced at this stage deepen the scientific community’s understanding of mangrove ecosystems and provide important scientific information for the next stage of research (Jiao et al., 2015; Alongi and Mukhopadhyay, 2015).
During this period, the research methods in this field changed significantly, including the use of stable isotopes, remote sensing, biomass models, land measurements, spectrometer data, and other technical means (Abdul Aziz et al., 2015; Dantas et al., 2011; Byrd et al., 2014; Jardine and Siikamäki, 2014; Friess et al., 2016; Xiong et al., 2018; Vo et al., 2015). The application of these new technologies enables scientists to effectively monitor the growth status, trends, and ecosystem functions associated with climate change in mangrove forests. Moreover, it offers deeper insights into the role of mangroves in biodiversity conservation, ecosystem stability, and climate regulation, as well as a more reliable basis for future climate change adaptation and conservation plans. For example, Hutchison J. and other researchers drew a spatial distribution map of the global mangrove potential aboveground biomass model of the global mangrove and estimated the global mangrove potential biomass, confirming the importance of mangroves in carbon accounting (Hutchison et al., 2014; Shapiro et al., 2015; Ya et al., 2015; Leopold et al., 2013).
As an extensible and comprehensive concept, blue carbon first appeared in the research literature in 2011 (Mcleod et al., 2011), and the follow-up research content and research methods continue to increase. Through systematic investigation and measurement, researchers have calculated and evaluated carbon storage in different BCE types and the effects of related factors on carbon storage (Miteva et al., 2015; McOwen et al., 2017; Serrano et al., 2014). Numerous studies have explored the effects of climate change, human disturbance, sea level rise, and other factors on BCEs, along with their potential impacts on blue carbon storage and ecosystem stability (Ewers Lewis et al., 2019; Schile et al., 2017; Serrano et al., 2016). The 2015 Paris Climate Agreement first mentioned BCEs, such as mangroves, salt marshes, and seagrass meadows, as important means of mitigating climate change. Thus, people began prioritizing the potential role of blue carbon conservation in climate change response, and some countries and international organizations began formulating relevant policies and support BCE conservation and restoration (Herrmann et al., 2015; Canu et al., 2015; Sutton-Grier et al., 2014). As the concept of blue carbon is gradually proposed and recognized, people began to realize the potential role of BCEs in the carbon cycle and climate change mitigation. This improvement in cognition has proliferated blue carbon research.
3.2.3 Stage 3
The third stage (the yellow-green clustering in Figure 5B) is the deepening research stage of blue carbon from 2018 to the present. For example, in yellow clustering, the keyword with the largest node is blue carbon. During this stage, blue carbon emerges as a focal point. From the perspective of research breadth and depth, keywords such as “carbon sequestration,” “carbon,” “climate change,” “ecosystem,” “biomass,” and “remote sensing” involve cross-cooperation in multiple disciplines such as marine ecology, biogeochemistry, and environmental economics. The focus of this phase is to deeply understand the role of BCEs in the global carbon balance and begin the prioritization of the potential impact of blue carbon on the environment, economy, and policy.
BCE conservation has been prioritized in marine management, garnering significant interest from scientists and coastal communities (Cuellar-Martinez et al., 2020). In the yellow cluster of Figure 5B, the frequencies of keywords such as “carbon sequestration,” “carbon,” and “carbon storage” related to blue carbon still exceed those of other keywords. Studying BCE’s role in carbon sequestration and sinks and estimating blue carbon biomass and carbon storage remain a research hotspot at this stage. For example, Orth et al. published a 20-year large-scale, successful seagrass restoration project, demonstrating that seagrass restoration increases blue carbon stocks (Orth et al., 2020). Sasmito et al. (2019) believed that the restoration of damaged BCEs can increase their carbon sequestration capacity. Moreover, the restoration of damaged seagrass beds or mangroves can increase the carbon storage of these ecosystems, thus indirectly promoting carbon sequestration. Lovelock et al. found that macroalgae may be considered as a blue carbon ecosystem in the future (Lin et al., 2021; Lovelock and Duarte, 2019; Krause-Jensen et al., 2018). Macroalgae, through photosynthesis, can accumulate substantial amounts of carbon within their bodies. Additionally, upon death and deposition, they facilitate the long-term storage of organic carbon in sediments. The restoration and expansion of natural macroalgae farms can increase the impact of carbon sinks. Research on the promotion of blue carbon storage needs to strengthen conservation by restoring and conserving lost coastal habitats. Blue carbon is intricately linked to carbon sequestration, cycling, and storage, as it collectively addresses the mechanisms and impacts of carbon retention and circulation within marine and coastal environments. By studying blue carbon, we can better understand and use the role of marine ecosystems in the global carbon balance. As the core themes of blue carbon research, “carbon sequestration,” “carbon,” and “carbon storage” continue to influence people’s understanding and attention to the carbon cycle of marine and coastal ecosystems. Furthermore, the keyword “biomass” with larger nodes directly affects the carbon sequestration capacity of the blue carbon ecosystem. Plants absorb carbon dioxide through photosynthesis and convert it into organic matter, which is stored in plants and deposited in soil or water after death, thus forming long-term carbon sequestration. Maintaining and increasing the biomass of ecosystems can effectively promote carbon sequestration and mitigate the effects of climate change (Saintilan et al., 2013; Christianson et al., 2022; Gao et al., 2022; Carnell et al., 2022).
As a prominent keyword for this stage, “remote sensing” plays an important role in monitoring and maintaining BCEs. With the development of “remote sensing” image accuracy, blue carbon research methods and technologies have been developed and innovated. For example, it is an innovative and efficient method to map, monitor, and predict the blue carbon ecosystem by using satellite images and remote sensing technology, multi-spectral sensors, synthetic aperture radar, drones, and new machine technologies such as the Logistic model, the InVEST coastal Vulnerability, and the coastal blue carbon biogeochemical digital model. This comprehensive use of technical means and models for blue carbon monitoring and assessment can effectively help to understand important information such as greenhouse gas emissions, organic carbon sequestration, and inventory changes (Mack et al., 2023; Hernández-Blanco et al., 2022; Yang, 2017; Poulter et al., 2023; Samper-Villarreal et al., 2018; Vázquez-Lule et al., 2022; Carlson et al., 2023; Pham et al., 2023; Doodee et al., 2023). Remote sensing, when combined with new technologies, enables the accurate discovery, monitoring, and evaluation of areas rich in carbon reserves. Moreover, it provides critical data on the distribution and trends of blue carbon resources, offering broader perspectives and more in-depth research methodologies for blue carbon studies. This approach further supports environmental conservation and promotes sustainable development. Remote sensing as a hot topic in this period will be increasingly used.
As an important keyword at this stage, “climate change” has attracted increased attention in blue carbon research (Chefaoui et al., 2018; Doughty et al., 2016). For example, in addition to providing ecosystem services in traditional marine wetland ecosystems, such as salt marshes and mangroves, studies have shown that carbon neutrality can also be achieved by planting large algae (e.g., kelp and seaweed) in offshore areas, thereby mitigating the impact of climate change (Guimond et al., 2020; Queirós et al., 2023, 2019; Oueslati et al., 2019; Duarte et al., 2021). Concurrently, climate change can markedly alter the carbon storage capacity of coastal wetlands. Under extreme weather conditions, mangroves may perish, with degraded mangroves resulting in a substantial reduction of blue carbon and markedly decreased carbon storage capacity (Senger et al., 2021; Chatting et al., 2022; Han et al., 2022). Recent in-depth research has underscored the significant role of blue carbon in regulating climate change and its substantial impact on global climate dynamics (Wang et al., 2021). Studying blue carbon is crucial for coping with climate change. Climate change is a hot topic in blue carbon research. Ecosystem services form a multidimensional concept closely related to climate, ecology, people, and culture (Hilmi et al., 2021; Osland et al., 2022). In recent years, marine ecosystem degradation, biodiversity decline, habitat degradation, loss, and fragmentation have become prominent problems, resulting in the loss of ecosystem services supporting the Earth (Hagger et al., 2022; Temmink et al., 2022). Countries are committed to conserving and restoring valuable ecosystem services. Supporting the absorption and storage of blue carbon from CO2 through the conservation of natural ecosystems with high carbon sequestration capacity has become an international consensus. Therefore, ecosystem services, another hot topic of blue carbon during this period, contribute markedly to ocean and coastline management (Quiros et al., 2021; Yadav et al., 2023).
In addition, we can clearly see “conservation,” “management,” “ restoration,” and other keywords from Figure 5B. As research into blue carbon deepens, its significant environmental, economic, and policy values are being progressively recognized and explored. For example, blue carbon conservation and utilization are essential for maintaining marine and wetland ecosystem health, as well as biodiversity and ecological balance (Wedding et al., 2021); the rational development and use of blue carbon resources creates a carbon trading market, provides enterprises with opportunities for carbon emission trading, and promotes carbon emission reduction and the development of a low-carbon economy (Luisetti et al., 2019). The international community has begun to gain a deeper understanding of the importance of conserving and restoring blue carbon and marine ecosystems (Wang et al., 2023). By conserving, planting, and restoring BCEs, countries directly offset their carbon emissions, restore and conserve isolated ecosystems, and promote other ecosystem services (e.g., coastal protection, timber, and fisheries; Tyllianakis et al., 2019). Some countries and regions have begun to develop policies and conservation frameworks to promote the conservation and sustainable management of blue carbon, including the establishment of marine protected areas, restoration of wetlands, and development of relevant management plans (Macreadie et al., 2017a; Mossman et al., 2022; Vanderklift et al., 2022; Duncan et al., 2022; Akhand et al., 2023).
These three typical stages can guide researchers in understanding blue carbon research advancements. In the future, the research direction and future research hotspots can be determined according to the development stage of blue carbon analysis.
3.3 Analysis of blue carbon-related trends
3.3.1 Trend analysis of blue carbon countries
As shown in Figure 6A, the geographical distribution of blue carbon research by correspondent author or author country reveals that the correspondent authors of the publication are from 143 countries. Among them, the United States (1237 articles), China (1127 articles), and Australia (717 articles) are the leading three countries in blue carbon research. The top ten countries list in blue carbon research is completed by the United Kingdom, Indonesia, Spain, India, Japan, Germany, and Brazil. Among them, countries with coastlines and large areas of marine wetlands, such as Australia, the United States, Indonesia, and Brazil, have made important progress in the study of blue carbon and have taken some measures to conserve and manage marine wetlands for carbon dioxide emissions reduction and carbon storage promotion. Mitigating climate change, promoting blue carbon research, and promoting sustainable development is crucial. Moreover, recently, as one of the world’s largest developing countries, China has made continuous progress in the study of blue carbon, demonstrating its concern for marine ecosystems and the carbon cycle.
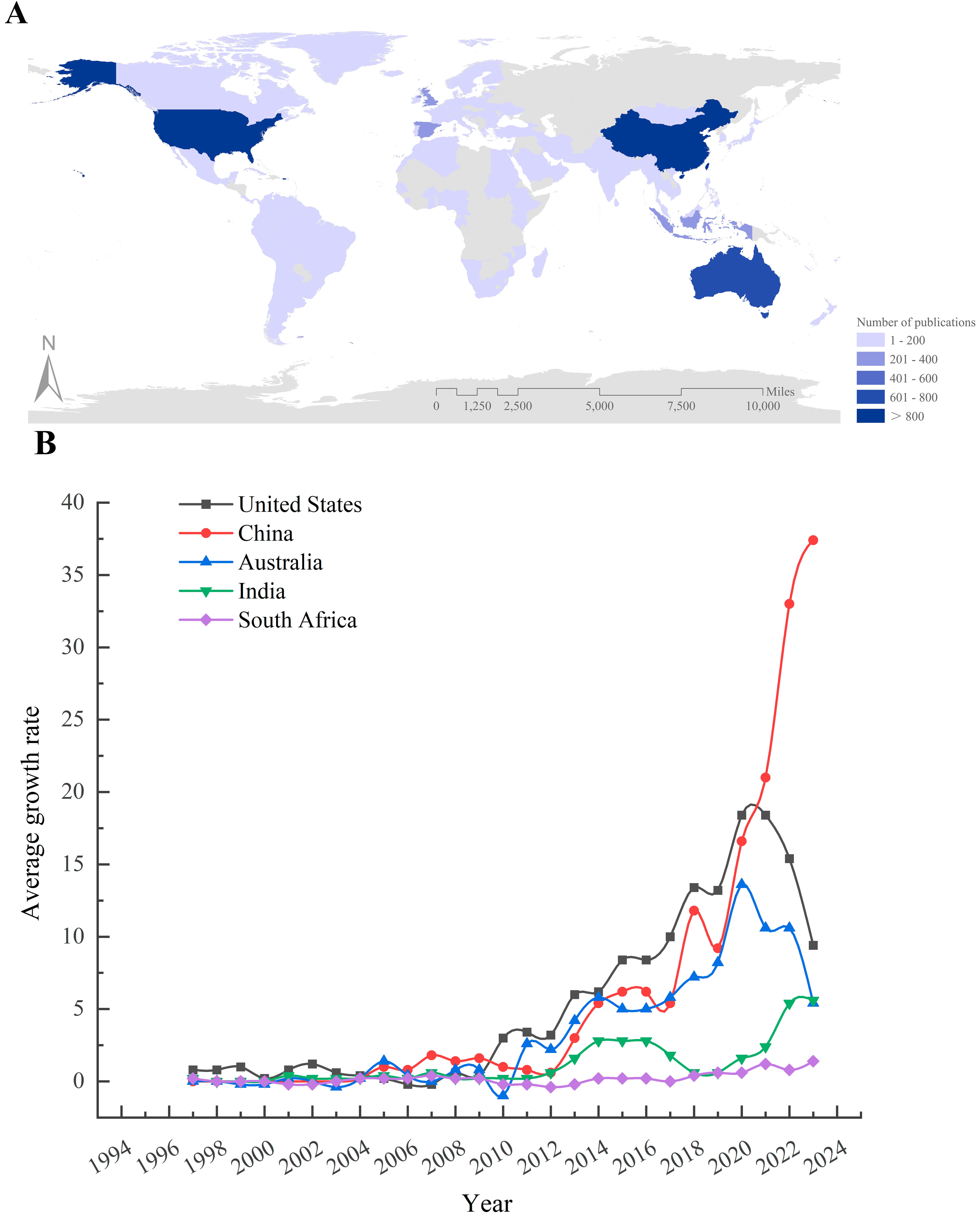
Figure 6. National trend analysis chart of blue carbon: (A) Geographical distribution of studies on BCEs by country. (B) Trends in the growth rate of BCE research in the United States, Australia, China, India, and South Africa.
As shown in Figure 6B, we applied the AGR method to calculate the number of papers published by different countries and derived the AGR values for blue carbon literature from the past 30 years by segmenting it into five-year intervals. Five typical countries were selected for analysis, and similarities in the trends of publications were observed between the United States and Australia, both of which decreased after the peak. To a certain extent, this observation reflects that the developed countries represented by the United States and Australia have slowed the speed of blue carbon research in recent years, possibly due to the upgrading of research methods and research contents. As the country with the second largest number of publications, China’s AGR has demonstrated an upward trend in the past three years, which is similar to India’s trend. As a developing country, attention to blue carbon has gradually increased in recent years. As a third-world country, South Africa has less literature on blue carbon, and the trend is not significant. However, to a certain extent, this finding reflects that some third-world countries are gradually paying attention to and investing in blue carbon research.
3.3.2 Analysis of the evolution of blue carbon keywords
The total number of publications, time of appearance, and connection with other topics have affected the evolution trend of hotspots in blue carbon research. In this paper, ScientoPy software was used to extract the author keywords in the literature with the time axis as the main line and analyze the number of keywords in different years and percentage of literature in recent years, revealing the evolution of research hotspots for blue carbon. As shown in Figure 7, in recent years, the related research on keywords such as “blue carbon,” “mangroves,” and “climate change” has increased linearly, indicating that increased attention and research on these topics. The specific data showed that blue carbon research has risen from 2 instances in 2011 to 775 presently, and publications from the past five years account for more than 74.7% of the literature. These data suggest that interest in and demand for blue carbon are growing rapidly, indicating that blue carbon research has become one of the mainstream topics in the fields of ecology, environmental science, and climate change. Furthermore, the more prominent keywords are mangroves and climate change. The number of mangroves was 716, and the percentage of literature published in the past five years was 57.3%. As a key BCE component, mangroves are a hot topic in blue carbon research. Furthermore, “climate change” appeared 375 times since 2008, and the percentage of literature published in the past five years is 70%. This trend reflects the increasing attention and concern of the global community on environmental issues, climate change, and related ecosystems. In addition, other mentioned terms include carbon sequestration, seagrass, salt marsh, ecosystem services, remote sensing, restoration, and hotspots (Orth and Heck, 2023; Rogers et al., 2019). Among them, the percentage of literature on restoration in the past five years exceeds 80%, thereby suggesting that BCE restoration has been a hot topic in blue carbon research in recent years. Moreover, the percentage of literature on remote sensing in the past five years surpasses 75%, indicating that the research and application of remote sensing technology in this field is becoming increasingly extensive and mature. The aforementioned development trends reflect the increasing attention that the global community is paying to environmental issues, climate change, and related ecosystems. Blue carbon (e.g., mangroves, seagrasses, and salt marshes) plays a key role in climate change mitigation, carbon storage, ecosystem conservation, and marine health, and it has attracted broad attention from researchers, policy makers, and the public (Pham et al., 2019b; Conrad et al., 2019).
Deepening the analysis of the development stage of specific blue carbon (i.e., the three typical stages in Section 3.2) can reveal information on the evolution of the popularity of blue carbon research content and future development trends. The analysis revealed a rapidly increasing number of publications on blue carbon literature in the past five years. We further analyze the author keywords of the blue carbon literature in the past five years (Figure 8A). In the selection of the first 20 keywords, the annual average increment rate of keywords was calculated in 3-year intervals. Figure 8B reveals that the trend factors of 18 keywords are positive, one average growth trend factor is 0, and the remaining one keyword trend factor is negative. In addition to “blue carbon,” “mangrove,” “climate change,” “seagrass,” “carbon sequestration,” “remote sensing,” “restoration,” “conservation,” “methane,” “macroalgae,” and other keywords have gradually attracted attention. For example, with the serious loss and functional degradation of BCEs, the restoration and conservation of blue carbon resources has become a hot issue of widespread concern in the international community. Methane and nitrous oxide emissions reduce the value of habitats acting as carbon sinks. Furthermore, in the blue carbon system, the value of stored organic carbon in mitigating climate change may become a research hotspot. Recent years have seen growing interest in macroalgae as a potential hotspot for blue carbon research (Ross et al., 2023; Schultz et al., 2023). Conversely, despite a negative AGR in salt marsh publications over the past three years, the volume of research remains substantial, likely suggesting the significant progress in related studies.
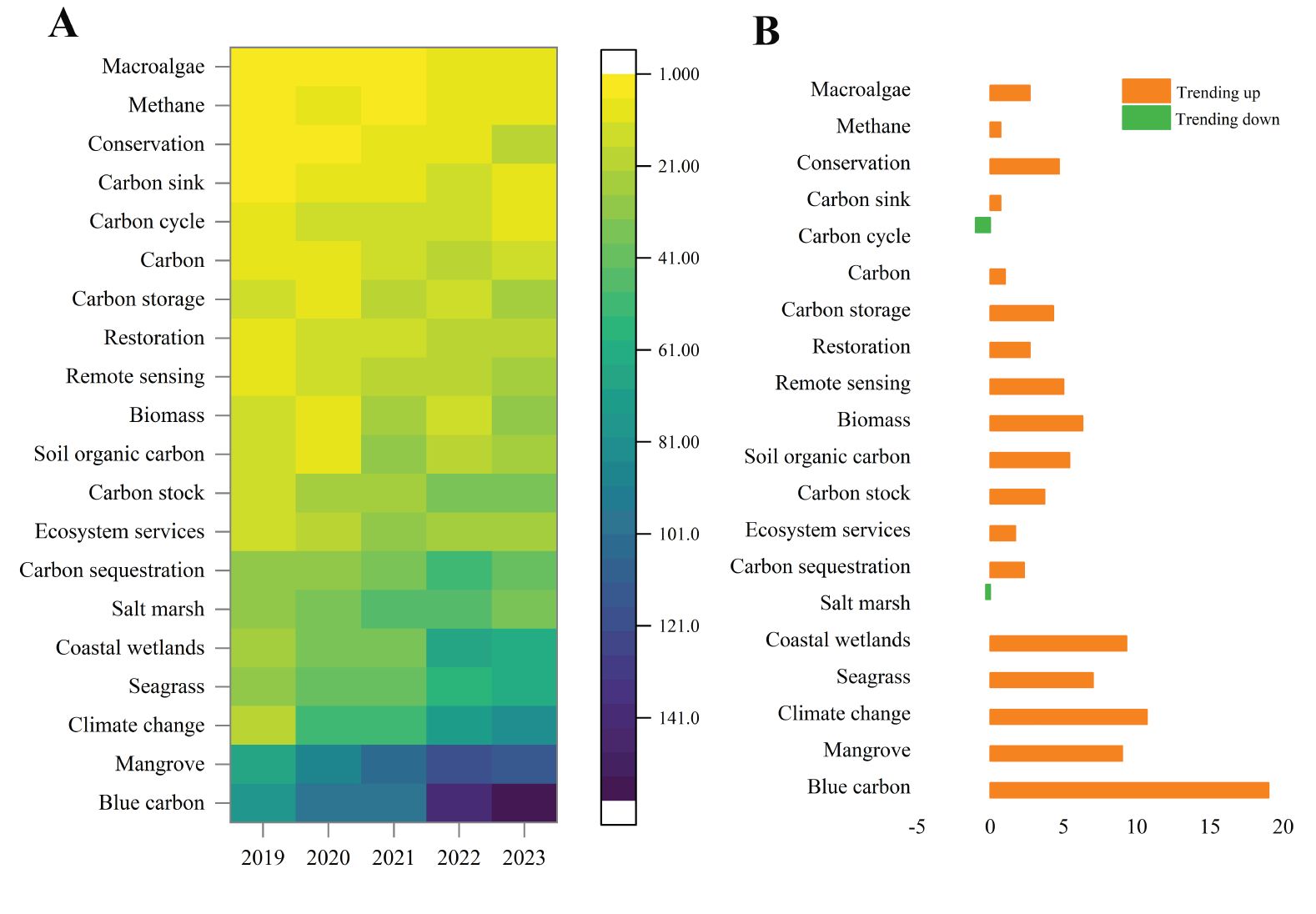
Figure 8. The evolution trend of the top 20 trending research topics related to BCE in the past five years: (A) BCEs are arranged in descending order of frequency. (B) Trends of cumulative keyword frequency.
4 Conclusion
Employing bibliometric-integrated ScientoPy and VOSviewer software, we conducted a quantitative analysis of 4,604 blue carbon-related studies from the WOS and Scopus databases spanning 1993 to 2023. The literature on blue carbon exhibits a rapid growth trend, with an increasing number of journals focusing on this area. This expansion allows more related research to be published across various journals, thereby promoting the further development and deepening of blue carbon studies. Furthermore, blue carbon research involves multiple disciplines and interdisciplinary cooperation, promoting exchanges and cooperation in more fields. Increased researchers are engaging in blue carbon research, and this area has gradually become an international cooperation research topic.
As a concept and research field, blue carbon has gone through three stages of development involving different focuses and research directions. (1) Blue carbon budding stage: From 1993 to 2009, scientists began examining the carbon storage and ecosystem functions of seagrasses, salt marshes, and mangroves. This stage witnessed a lack of in-depth understanding of the role of marine vegetation, especially mangroves and seagrass beds, in absorbing and fixing carbon, with carbon storage and ecosystem services in marine ecosystems receiving limited attention. (2) The concept of blue carbon: In 2009, the concept of blue carbon was proposed, and mangroves in BCEs emerged as a hot topic during this period. With the in-depth study of mangroves as a key BCE and the warming of climate change issues, people began realizing the potential role of BCEs in the carbon cycle and climate change mitigation. To a certain extent, increased research on blue carbon has been spawned. (3) Blue carbon deepening research: During 2018–2023, the focus is to deeply understand the role of BCEs in the global carbon balance and begin prioritizing the potential impact of blue carbon on the environment, economy, and policy. With much research supporting the potential response of blue carbon to climate change and its importance to ecosystem services, blue carbon has gradually become part of the international environmental policy and sustainable development agenda. People began to explore how to conserve and manage marine ecosystems to maximize their carbon storage capacity. Blue carbon research involves the cooperation of marine ecology, biogeochemistry, environmental economics, and other disciplines. Moreover, blue carbon research has gradually developed from being a single, simple, and decentralized area to becoming a comprehensive, complex, and networked area.
The trend analysis of blue carbon research shows that developed countries prioritize climate change and environmental conservation; hence, the attention to blue carbon is earlier and more prominent, but the research has reached a certain peak. Meanwhile, in developing countries, attention to blue carbon is gradually increasing. Third-world countries face economic, social, and environmental challenges to a large extent; therefore, their attention to blue carbon may be relatively low. The trend analysis of keywords revealed that BCE restoration and conservation are receiving increasing attention. Remote sensing, the most commonly used technology in the field of blue carbon research in recent years, has been actively applied. In blue carbon studies, increasing carbon sink capacity and mitigating climate change, as well as carbon sequestration of macroalgae have consistently remained potential hotspots in blue carbon research advancements.
Author contributions
SW: Conceptualization, Data curation, Methodology, Validation, Visualization, Writing – original draft, Writing – review & editing. DY: Conceptualization, Supervision, Visualization, Writing – review & editing. CW: Supervision, Writing – review & editing. LW: Investigation, Methodology, Resources, Software, Writing – review & editing. YH: Investigation, Methodology, Resources, Software, Writing – review & editing.
Funding
The author(s) declare financial support was received for the research, authorship, and/or publication of this article. This research was supported by Henan Province Soft Science Research Project (232400410015), PhD Research Startup Foundation of Henan University of Science and Technology (13480078), National College Student Innovation and Entrepreneurship Training Program (202310464058), and Henan University of Science and Technology College Student Innovation and Entrepreneurship Training Program (2023250).
Acknowledgments
We thank Silvester G (www.enago.cn) for editing the English text of a draft of this manuscript.
Conflict of interest
The authors declare that the research was conducted in the absence of any commercial or financial relationships that could be construed as a potential conflict of interest.
Publisher’s note
All claims expressed in this article are solely those of the authors and do not necessarily represent those of their affiliated organizations, or those of the publisher, the editors and the reviewers. Any product that may be evaluated in this article, or claim that may be made by its manufacturer, is not guaranteed or endorsed by the publisher.
References
Abdul Aziz A., Dargusch P., Phinn S., Ward A. (2015). Using REDD+ to balance timber production with conservation objectives in a mangrove forest in Malaysia. Ecol. Economics 120, 108–116. doi: 10.1016/j.ecolecon.2015.10.014
Akhand A., Chanda A., Jameel Y., Dasgupta R. (2023). The present state-of-the-art of blue carbon repository in India: a meta-analysis. Sustainability Sci. 18, 1031–1042. doi: 10.1007/s11625-022-01181-4
Alongi D. M., Mukhopadhyay S. K. (2015). Contribution of mangroves to coastal carbon cycling in low latitude seas. Agric. For. Meteorol. 213, 266–272. doi: 10.1016/j.agrformet.2014.10.005
Atwood T. B., Madin E. M. P., Harborne A. R., Hammill E., Luiz O. J., Ollivier Q. R., et al. (2018). Predators Shape sedimentary organic carbon storage in a coral reef ecosystem. Front. Ecol. Evolution 6. doi: 10.3389/fevo.2018.00110
Bano N., Nisa M. U., Khan N., Saleem M., Harrison P. J., Ahmed S. I., et al. (1997). Significance of bacteria in the flux of organic matter in the tidal creeks of the mangrove ecosystem of the Indus River delta, Pakistan. Mar. Ecol. Prog. Series 157, 1–12. doi: 10.3354/meps157001
Barenblitt A., Fatoyinbo L., Thomas N., Stovall A., de Sousa C., Nwobi C., et al. (2023). Invasion in the Niger Delta: remote sensing of mangrove conversion to invasive Nypa fruticans from 2015 to 2020. Remote Sens. Ecol. Conserv. 10, 5–23. doi: 10.1002/rse2.353
Beaumont N. J., Jones L., Garbutt A., Hansom J. D., Toberman M. (2014). The value of carbon sequestration and storage in coastal habitats. Estuar. Coast. Shelf Sci. 137, 32–40. doi: 10.1016/j.ecss.2013.11.022
Beer S., Eshel A., Waisel Y. (1977). Carbon Metabolism in Seagrasses: I. The utilization of exogenous inorganic carbon species in photosynthesis. J. Exp. Botany 28, 1180–1189. doi: 10.1093/jxb/28.5.1180
Bouillon S., Boschker H. T. S. (2006). Bacterial carbon sources in coastal sediments: A cross-system analysis based on stable isotope data of biomarkers. Biogeosciences 3, 175–185. doi: 10.5194/bg-3-175-2006
Bouillon S., Connolly R. M. (2009). “Carbon exchange among tropical coastal ecosystems,” in Ecological Connectivity among Tropical Coastal Ecosystems (Dordrecht, Netherlands: Springer Netherlands), 45–70. doi: 10.1007/978-90-481-2406-03
Bouillon S., Frankignoulle M., Dehairs F., Velimirov B., Eiler A., Abril G., et al. (2003). Inorganic and organic carbon biogeochemistry in the Gautami Godavari estuary (Andhra Pradesh, India) during pre-monsoon: The local impact of extensive mangrove forests. Global Biogeochemical Cycles 17, 25–21. doi: 10.1029/2002gb002026
Buditama A. (2016). Blue carbon for reducing the impacts of climate change: An Indonesian case study. Environmental Planning Law Joural 33, 68–88.
Byrd K. B., O’Connell J. L., Di Tommaso S., Kelly M. (2014). Evaluation of sensor types and environmental controls on mapping biomass of coastal marsh emergent vegetation. Remote Sens. Environment 149, 166–180. doi: 10.1016/j.rse.2014.04.003
Cameron C., Maharaj A., Kennedy B., Tuiwawa S., Goldwater N., Soapi K., et al. (2021). Landcover change in mangroves of Fiji: Implications for climate change mitigation and adaptation in the Pacific. Environ. Challenges 2, 100018. doi: 10.1016/j.envc.2020.100018
Canu D. M., Ghermandi A., Nunes P., Lazzari P., Cossarini G., Solidoro C. (2015). Estimating the value of carbon sequestration ecosystem services in the Mediterranean Sea: An ecological economics approach. Global Environ. Change-Human Policy Dimensions 32, 87–95. doi: 10.1016/j.gloenvcha.2015.02.008
Carlson D. F., Vivó-Pons A., Treier U. A., Mätzler E., Meire L., Sejr M. (2023). Krause-Jensen, D. Mapping intertidal macrophytes in fjords in Southwest Greenland using Sentinel-2 imagery. Sci. Total Environment 865, 161213. doi: 10.1016/j.scitotenv.2022.161213
Carnell P. E., Palacios M. M., Waryszak P., Trevathan-Tackett S. M., Masque P., Macreadie P. I. (2022). Blue carbon drawdown by restored mangrove forests improves with age. J. Environ. Management 306, 114301. doi: 10.1016/j.jenvman.2021.114301
Castillo J. A. A., Apan A. A., Maraseni T. N., Salmo S. G. (2017). Soil C quantities of mangrove forests, their competing land uses, and their spatial distribution in the coast of Honda Bay, Philippines. Geoderma 293, 82–90. doi: 10.1016/j.geoderma.2017.01.025
Chatting M., Al-Maslamani I., Walton M., Skov M. W., Kennedy H., Husrevoglu Y. S., et al. (2022). Future Mangrove carbon storage under climate change and deforestation. Front. Mar. Sci. 9. doi: 10.3389/fmars.2022.781876
Chefaoui R. M., Duarte C. M., Serrão E. A. (2018). Dramatic loss of seagrass habitat under projected climate change in the Mediterranean Sea. Global Change Biol. 24, 4919–4928. doi: 10.1111/gcb.14401
Choi C. Y., Xiao H., Jia M. M., Jackson M. V., Lai Y. C., Murray N. J., et al. (2022). An emerging coastal wetland management dilemma between mangrove expansion and shorebird conservation. Conserv. Biol. 36, e13905. doi: 10.1111/cobi.13905
Christianson A. B., Cabré A., Bernal B., Baez S. K., Leung S., Pérez-Porro A., et al. (2022). The promise of blue carbon climate solutions: where the science supports ocean-climate policy. Front. Mar. Sci. 9. doi: 10.3389/fmars.2022.851448
Chuan C. H., Gallagher J. B., Chew S. T., Norlaila Binti M. Z. (2020). Blue carbon sequestration dynamics within tropical seagrass sediments: Long-term incubations for changes over climatic scales. Mar. Freshw. Res. 71, 892–904. doi: 10.1071/MF19119
Clough B. F. (1995). Structure and productivity of a 20-yr-old stand of Rhizophora apiculata Bl. mangrove forest. J. Biogeogr. 22, 417–424. doi: 10.2307/2845938
Clough B. (1998). Mangrove forest productivity and biomass accumulation in Hinchinbrook Channel, Australia. Mangroves Salt Marshes 2, 191–198. doi: 10.1023/A:1009979610871
Coleman M. A. (2023). Algae in the Anthropocene: Managing, conserving, and utilizing algae in an era of rapid environmental change. J. Phycol. 60, 1–3. doi: 10.1111/jpy.13409
Conrad S., Brown D. R., Alvarez P. G., Bates B., Ibrahim N., Reid A., et al. (2019). Does regional development influence sedimentary blue carbon stocks? A case study from three Australian estuaries. Front. Mar. Sci. 5. doi: 10.3389/fmars.2018.00518
Costa M. D. D., Macreadie P. I. (2022). The evolution of blue carbon science. Wetlands 42, 127. doi: 10.1007/s13157-022-01648-1
Cuellar-Martinez T., Carolina Ruiz-Fernandez A., Sanchez-Cabeza J. A., Perez-Bernal L., Guadalupe Lopez-Mendoza P., Carnero-Bravo V., et al. (2020). Temporal records of organic carbon stocks and burial rates in Mexican blue carbon coastal ecosystems throughout the Anthropocene. Global Planetary Change 192, 103215. doi: 10.1016/j.gloplacha.2020.103215
Dantas S. T. P. L., Amaro V. E., Costa B. C. P. (2011). In Mangrove reforestation as a mesotidal coastal protection and Clean Development Mechanism on Macau-serra oil field, Potiguar Basin, Northeast Brazil. J. Coast. Res. 64, 1268–1271.
Donato D. C., Kauffman J. B., Murdiyarso D., Kurnianto S., Stidham M., Kanninen M. (2011). Mangroves among the most carbon-rich forests in the tropics. Nat. Geosci. 4, 293–297. doi: 10.1038/NGEO1123
Doodee M. D. D., Rughooputh S. D. D. V., Jawaheer S. (2023). Remote sensing monitoring of mangrove growth rate at selected planted sites in Mauritius. South Afr. J. Sci. 119, 13716. doi: 10.17159/sajs.2023/13716
Doughty C. L., Langley J. A., Walker W. S., Feller I. C., Schaub R., Chapman S. K. (2016). Mangrove range expansion rapidly increases coastal wetland carbon storage. Estuaries Coasts 39, 385–396. doi: 10.1007/s12237-015-9993-8
Duarte C. M. (2017). Reviews and syntheses: Hidden forests, the role of vegetated coastal habitats in the ocean carbon budget. Biogeosciences 14, 301–310. doi: 10.5194/bg-14-301-2017
Duarte B., Carreiras J., Caçador I. (2021). Climate change impacts on salt marsh blue carbon, nitrogen and phosphorous stocks and ecosystem services. Appl. Sci. 11, 1969. doi: 10.3390/app11041969
Duarte C. M., Kennedy H., Marbà N., Hendriks I. (2013). Assessing the capacity of seagrass meadows for carbon burial: Current limitations and future strategies. Ocean Coast. Management 83, 32–38. doi: 10.1016/j.ocecoaman.2011.09.001
Duarte C. M., Middelburg J. J., Caraco N. (2005). Major role of marine vegetation on the oceanic carbon cycle. Biogeosciences 2, 1–8. doi: 10.5194/bg-2-1-2005
Duncan C., Primavera J. H., Hill N. A. O., Wodehouse D. C. J., Koldewey H. J. (2022). Potential for return on investment in rehabilitation-oriented blue carbon projects: accounting methodologies and project strategies. Front. Forests Global Change 4. doi: 10.3389/ffgc.2021.775341
Escobedo F., Varela S., Zhao M., Wagner J. E., Zipperer W. (2010). Analyzing the efficacy of subtropical urban forests in offsetting carbon emissions from cities. Environ. Sci. Policy 13, 362–372. doi: 10.1016/j.envsci.2010.03.009
Estrada G. C. D., Soares M. L. G., Fernadez V., De Almeida P. M. M. (2015). The economic evaluation of carbon storage and sequestration as ecosystem services of mangroves: A case study from southeastern Brazil. Int. J. Biodiversity Sci. Ecosystem Serv. Management 11, 29–35. doi: 10.1080/21513732.2014.963676
Ewers Lewis C. J., Baldock J. A., Hawke B., Gadd P. S., Zawadzki A., Heijnis H., et al. (2019). Impacts of land reclamation on tidal marsh ‘blue carbon’ stocks. Sci. Total Environment 672, 427–437. doi: 10.1016/j.scitotenv.2019.03.345
Eyre B. D., Camillini N., Glud R. N., Rosentreter J. A. (2023). The climate benefit of seagrass blue carbon is reduced by methane fluxes and enhanced by nitrous oxide fluxes. Commun. Earth Environment 4, 374. doi: 10.1038/s43247-023-01022-x
Farnsworth E. J., Ellison A. M., Gong W. K. (1996). Elevated CO2 altersanatomy, physiology, growth, and reproduction of red mangrove (Rhizophora mangle L.). Oecologia 108, 599–609. doi: 10.1007/BF00329032
Friess D. A., Richards D. R., Phang V. X. H. (2016). Mangrove forests store high densities of carbon across the tropical urban landscape of Singapore. Urban Ecosystems 19, 795–810. doi: 10.1007/s11252-015-0511-3
Gao G., Beardall J., Jin P., Gao L., Xie S. Y., Gao K. S. (2022). A review of existing and potential blue carbon contributions to climate change mitigation in the Anthropocene. J. Appl. Ecol. 59, 1686–1699. doi: 10.1111/1365-2664.14173
Guimond J. A., Seyfferth A. L., Moffett K. B., Michael H. A. (2020). A physical-biogeochemical mechanism for negative feedback between marsh crabs and carbon storage. Environ. Res. Letters 15, 034024. doi: 10.1088/1748-9326/ab60e2
Hagger V., Waltham N. J., Lovelock C. E. (2022). Opportunities for coastal wetland restoration for blue carbon with co-benefits for biodiversity, coastal fisheries, and water quality. Ecosystem Services 55, 101423. doi: 10.1016/j.ecoser.2022.101423
Han G. X., Wang F. M., Ma J., Xiao L. L., Chu X. J., Zhao M. L. (2022). Blue carbon sink function, formation mechanism and sequestration potential of coastal salt marshes. Chin. J. Plant Ecol. 46, 373–382. doi: 10.17521/cjpe.2021.0264
Hawman P. A., Mishra D. R., O’Connell J. L. (2023). Dynamic emergent leaf area in tidal wetlands: Implications for satellite-derived regional and global blue carbon estimates. Remote Sens. Environment 290, 113553. doi: 10.1016/j.rse.2023.113553
Hernández-Blanco M., Moritsch M., Manrow M., Raes L. (2022). Coastal ecosystem services modeling in Latin America to guide conservation and restoration strategies: The case of mangroves in Guatemala and El Salvador. Front. Ecol. Evolution 10. doi: 10.3389/fevo.2022.843145
Herrmann M., Najjar R. G., Kemp W. M., Alexander R. B., Boyer E. W., Cai W. J., et al. (2015). Net ecosystem production and organic carbon balance of U.S. East Coast estuaries: A synthesis approach. Global Biogeochemical Cycles 29, 96–111. doi: 10.1002/2013GB004736
Hilmi N., Chami R., Sutherland M. D., Hall-Spencer J. M., Lebleu L., Benitez M. B., et al. (2021). The role of blue carbon in climate change mitigation and carbon stock conservation. Front. Climate 3. doi: 10.3389/fclim.2021.710546
Howard J., McLeod E., Thomas S., Eastwood E., Fox M., Wenzel L., et al. (2017). The potential to integrate blue carbon into MPA design and management. Aquat. Conservation-marine Freshw. Ecosystems 27, 100–115. doi: 10.1002/aqc.2809
Hutchison J., Manica A., Swetnam R., Balmford A., Spalding M. (2014). Predicting global patterns in mangrove forest biomass. Conserv. Letters 7, 233–240. doi: 10.1111/conl.12060
Jakovac C. C., Latawiec A. E., Lacerda E., Lucas I. L., Korys K. A., Iribarrem A., et al. (2020). Costs and carbon benefits of mangrove conservation and restoration: A global analysis. Ecol. Economics 176, 106792. doi: 10.1016/j.ecolecon.2020.106792
Jardine S. L., Siikamäki J. V. (2014). A global predictive model of carbon in mangrove soils. Environ. Res. Letters 9, 104013. doi: 10.1088/1748-9326/9/10/104013
Jiang L., Yang T., Yu J. (2022). Global trends and prospects of blue carbon sinks: a bibliometric analysis. Environ. Sci. pollut. Res. 29, 65924–65939. doi: 10.1007/s11356-022-22216-4
Jiao N. Z., Chen D. K., Luo Y. M., Huang X. P., Zhang R., Zhang H. B., et al. (2015). Climate change and anthropogenic impacts on marine ecosystems and countermeasures in China. Adv. Climate Change Res. 6, 118–125. doi: 10.1016/j.accre.2015.09.010
Kelleway J. J., Saintilan N., Macreadie P. I., Skilbeck C. G., Zawadzki A., Ralph P. J. (2016). Seventy years of continuous encroachment substantially increases “blue carbon” capacity as mangroves replace intertidal salt marshes. Global Change Biol. 22, 1097–1109. doi: 10.1111/gcb.13158
Krause-Jensen D., Lavery P., Serrano O., Marbà N., Masque P., Duarte C. M. (2018). Sequestration of macroalgal carbon: the elephant in the Blue Carbon room. Biol. Letters 14, 20180236. doi: 10.1098/rsbl.2018.0236
Kulawardhana R. W., Feagin R. A., Popescu S. C., Boutton T. W., Yeager K. M., Bianchi T. S. (2015). The role of elevation, relative sea-level history and vegetation transition in determining carbon distribution in Spartina alterniflora dominated salt marshes. Estuar. Coast. Shelf Sci. 154, 48–57. doi: 10.1016/j.ecss.2014.12.032
Lai Q. Y., Ma J., He F., Zhang A. G., Pei D. Y., Wei G., et al. (2022). Research development, current hotspots, and future directions of blue carbon: A bibliometric analysis. Water 14, 1193. doi: 10.3390/w14081193
Leopold A., Marchand C., Deborde J., Chaduteau C., Allenbach M. (2013). Influence of mangrove zonation on CO2 fluxes at the sediment-air interface (New Caledonia). Geoderma 202, 62–70. doi: 10.1016/j.geoderma.2013.03.008
Lester S. E., Dubel A. K., Hernán G., McHenry J., Rassweiler A. (2020). spatial planning principles for marine ecosystem restoration. Front. Mar. Sci. 7. doi: 10.3389/fmars.2020.00328
Lin Q. Q., Zeng D. Q., Guo T. Y., Peng L. (2021). Filter-feeding fish (Hypophthalmichthys molitrix) mediated phosphorus recycling versus grazing pressure as drivers of the trophic cascade in large enclosures subsidized by allochthonous detritus. Water Res. 204, 117579. doi: 10.1016/j.watres.2021.117579
Locatelli T., Binet T., Kairo J. G., King L., Madden S., Patenaude G. (2014). Turning the tide: How blue carbon and payments for ecosystem services (PES) might help save mangrove forests. Ambio 43, 981–995. doi: 10.1007/s13280-014-0530-y
Lovelock C. E. (2008). Soil respiration and belowground carbon allocation in mangrove forests. Ecosystems 11, 342–354. doi: 10.1007/s10021-008-9125-4
Lovelock C. E., Duarte C. M. (2019). Dimensions of blue carbon and emerging perspectives. Biol. Letters 15, 20180781. doi: 10.1098/rsbl.2018.0781
Luisetti T., Turner R. K., Andrews J. E., Jickells T. D., Kröger S., Diesing M., et al. (2019). Quantifying and valuing carbon flows and stores in coastal and shelf ecosystems in the UK. Ecosystem Services 35, 67–76. doi: 10.1016/j.ecoser.2018.10.013
Lunstrum A., Chen L. Z. (2014). Soil carbon stocks and accumulation in young mangrove forests. Soil Biol. Biochem. 75, 223–232. doi: 10.1016/j.soilbio.2014.04.008
Lyu P., Liu X., Yao T. (2023). A bibliometric analysis of literature on bibliometrics in recent half-century. J. Inf. Sci. doi: 10.1177/01655515231191233
Mack S. K., Lane R. R., Deng J., Morris J. T., Bauer J. J. (2023). Wetland carbon models: Applications for wetland carbon commercialization. Ecol. Modelling 476, 110228. doi: 10.1016/j.ecolmodel.2022.110228
Mack S. K., Lane R. R., Holland K., Bauer J., Cole J., Cowan R. A. (2022). blue carbon pilot project: Lessons learned. Carbon Management 13, 420–434. doi: 10.1080/17583004.2022.2112292
Macreadie P. I., Atwood T. B., Seymour J. R., Fontes M. L. S., Sanderman J., Nielsen D. A. (2019). Vulnerability of seagrass blue carbon to microbial attack following exposure to warming and oxygen. Sci. Total Environment 686, 264–275. doi: 10.1016/j.scitotenv.2019.05.462
Macreadie P. I., Nielsen D. A., Kelleway J. J., Atwood T. B., Seymour J. R., Petrou K., et al. (2017a). Can we manage coastal ecosystems to sequester more blue carbon? Front. Ecol. Environment 15, 206–213. doi: 10.1002/fee.1484
Macreadie P. I., Robertson A. I., Spinks B., Adams M. P., Atchison J. M., Bell-James J., et al. (2022). Operationalizing marketable blue carbon. One Earth 5, 485–492. doi: 10.1016/j.oneear.2022.04.005
Macreadie P. I., Serrano O., Maher D. T., Duarte C. M., Beardall J. (2017b). Addressing calcium carbonate cycling in blue carbon accounting. Limnol. Oceanogr. Letters 2, 195–201. doi: 10.1002/lol2.10052
Mcleod E., Chmura G. L., Bouillon S., Salm R., Björk M., Duarte C. M., et al. (2011). A blueprint for blue carbon: toward an improved understanding of the role of vegetated coastal habitats in sequestering CO2. Front. Ecol. Environment 9, 552–560. doi: 10.1890/110004
McOwen C. J., Weatherdon L. V., Van Bochove J. W., Sullivan E., Blyth S., Zockler C., et al. (2017). A global map of saltmarshes. Biodiversity Data J. 5, e11764. doi: 10.3897/BDJ.5.e11764
Middelburg J. J., Nieuwenhuize J., Slim F. J., Ohowa B. (1996). Sediment biogeochemistry in an east African mangrove forest (Gazi Bay, Kenya). Biogeochemistry 34, 133–155. doi: 10.1007/BF00000899
Miteva D. A., Murray B. C., Pattanayak S. K. (2015). Do protected areas reduce blue carbon emissions? A quasi-experimental evaluation of mangroves in Indonesia. Ecol. Economics 119, 127–135. doi: 10.1016/j.ecolecon.2015.08.005
Mitra A., Zaman S. (2015). Blue carbon reservoir of the blue planet (New Delhi, India: Springer India), 1–299.
Miyamoto H., Kawachi N., Kurotani A., Moriya S., Suda W., Suzuki K., et al. (2023). Computational estimation of sediment symbiotic bacterial structures of seagrasses overgrowing downstream of onshore aquaculture. Environ. Res. 219, 115130. doi: 10.1016/j.envres.2022.115130
Mossman H. L., Pontee N., Born K., Hill C., Lawrence P. J., Rae S., et al. (2022). Rapid carbon accumulation at a saltmarsh restored by managed realignment exceeded carbon emitted in direct site construction. PloS One 17, e0259033. doi: 10.1371/journal.pone.0259033
Mukai H., Iijima A. (1995). Grazing effects of a gammaridean Amphipoda, Ampithoe sp., on the seagrass, Syringodium isoetifolium, and epiphytes in a tropical seagrass bed of Fiji. Ecol. Res. 10, 243–257. doi: 10.1007/BF02347850
Munir M., Wicaksono P. (2019). Support Vector machine for seagrass percent cover mapping using planetscope image in Labuan Bajo, East Nusa Tenggara. Sixth International Symposium on LAPAN-IPB Satellite, Bogor, Indonesia, 11372. doi: 10.1117/12.2541849
Navarro A., Young M., Macreadie P. I., Nicholson E., Ierodiaconou D. (2021). Mangrove and saltmarsh distribution mapping and land cover change assessment for South-Eastern Australia from 1991 to 2015. Remote Sensing 13, 1450. doi: 10.3390/rs13081450
Ninkov A., Frank J. R., Maggio L. (2022). A Bibliometrics: Methods for studying academic publishing. Perspect. Med. Education 11, 173–176. doi: 10.1007/s40037-021-00695-4
Orth R. J., Heck K. L. Jr. (2023). The dynamics of seagrass ecosystems: History, past accomplishments, and future prospects. Estuaries Coasts 46, 1653–1676. doi: 10.1007/s12237-023-01252-4
Orth R. J., Lefcheck J. S., McGlathery K. S., Aoki L., Luckenbach M. W., Moore K. A., et al. (2020). Restoration of seagrass habitat leads to rapid recovery of coastal ecosystem services. Sci. Adv. 6, eabc6434. doi: 10.1126/sciadv.abc643
Osland M. J., Hughes A. R., Armitage A. R., Scyphers S. B., Cebrian J., Swinea S. H., et al. (2022). The impacts of mangrove range expansion on wetland ecosystem services in the southeastern United States: Current understanding, knowledge gaps, and emerging research needs. Global Change Biol. 28, 3163–3187. doi: 10.1111/gcb.16111
Oueslati W., van de Velde S., Helali M. A., Added A., Aleya L., Meysman F. J. R. (2019). Carbon, iron and sulphur cycling in the sediments of a Mediterranean lagoon (Ghar El Melh, Tunisia). Estuar. Coast. Shelf Sci. 221, 156–169. doi: 10.1016/j.ecss.2019.03.008
Ouyang X. G., Lee S. Y., Connolly R. M. (2017). The role of root decomposition in global mangrove and saltmarsh carbon budgets. Earth-Science Rev. 166, 53–63. doi: 10.1016/j.earscirev.2017.01.004
Pallis G. (2019). A look at the bibliometrics. IEEE Internet Computing 23, 5–7. doi: 10.1109/MIC.2019.2931020
Peña E. J., Zingmark R., Nietch C. (1999). Comparative photosynthesis of two species of intertidal epiphytic macroalgae on mangrove roots during submersion and emersion. J. Phycol. 35, 1206–1214. doi: 10.1046/j.1529-8817.1999.3561206.x
Peng C. J., Qian J. W., Guo X. D., Zhao H. W., Hu N. X., Yang Q., et al. (2016). Vegetation carbon stocks and net primary productivity of the mangrove forests in Shenzhen, China. Chin. J. Appl. Ecol. 27, 2059–2065. doi: 10.1007/s10021-008-9125-4
Perera K. A. R. S., De Silva K. H. W. L., Amarasinghe M. D. (2018). Potential impact of predicted sea level rise on carbon sink function of mangrove ecosystems with special reference to Negombo estuary, Sri Lanka. Global Planetary Change 161, 162–171. doi: 10.1016/j.gloplacha.2017.12.016
Pétillon J., McKinley E., Alexander M., Adams J. B., Angelini C., Balke T., et al. (2023). Top ten priorities for global saltmarsh restoration, conservation and ecosystem service research. Sci. Total Environ. 898, 165544. doi: 10.1016/j.scitotenv.2023.165544
Pham T. D., Ha N. T., Saintilan N., Skidmore A., Phan D. C., Le N. N., et al. (2023). Advances in Earth observation and machine learning for quantifying blue carbon. Earth-science Rev. 243, 104501. doi: 10.1016/j.earscirev.2023.104501
Pham T. D., Xia J. S., Ha N. T., Bui D. T., Le N. N., Takeuchi W. (2019a). A review of remote sensing approaches for monitoring blue carbon ecosystems: mangroves, seagrasses and salt marshes during 2010-2018. Sensors 19, 1933. doi: 10.3390/s19081933
Pham T. D., Yokoya N., Bui D. T., Yoshino K., Friess D. A. (2019b). Remote Sensing approaches for monitoring mangrove species, structure, and biomass: Opportunities and challenges. Remote Sensing 11, 230. doi: 10.3390/rs11030230
Pollard P. C., Kogure K. (1993). The role of epiphytic and epibenthic algal productivity in a tropical seagrass, Syringodium isoetifolium (Aschers.) Dandy, community. Mar. Freshw. Res. 44, 141–154. doi: 10.1071/MF9930141
Poulter B., Adams-Metayer F. M., Amaral C., Barenblitt A., Campbell A., Charles S. P., et al. (2023). Multi-scale observations of mangrove blue carbon ecosystem fluxes: The NASA Carbon Monitoring System BlueFlux field campaign. Environ. Res. Letters 18, 075009. doi: 10.1088/1748-9326/acdae6
Queirós A. M., Stephens N., Widdicombe S., Tait K., McCoy S. J., Ingels J., et al. (2019). Connected macroalgal-sediment systems: blue carbon and food webs in the deep coastal ocean. Ecol. Monographs 89, e01366. doi: 10.1002/ecm.1366
Queirós A. M., Tait K., Clark J. R., Bedington M., Pascoe C., Torres R., et al. (2023). Identifying and protecting macroalgae detritus sinks toward climate change mitigation. Ecol. Applications 33, e2798. doi: 10.1002/eap.2798
Quevedo J. M. D., Uchiyama Y., Kohsaka R. (2023). Progress of blue carbon research: 12 years of global trends based on content analysis of peer-reviewed and ‘gray literature’ documents. Ocean Coast. Manage. 236, 106495. doi: 10.1016/j.ocecoaman.2023.106495
Quiros T., Sudo K., Ramilo R. V., Garay H. G., Soniega M. P. G., Baloloy A., et al. (2021). Blue carbon ecosystem services through a vulnerability lens opportunities to reduce social vulnerability in fishing communities. Front. Mar. Sci. 8. doi: 10.3389/fmars.2021.671753
Ray R., Majumder N., Chowdhury C., Jana T. K. (2012). Wood chemistry and density: An analog for response to the change of carbon sequestration in mangroves. Carbohydr. Polymers 90, 102–108. doi: 10.1016/j.carbpol.2012.05.001
Reef R., Lovelock C. E. (2014). Historical analysis of mangrove leaf traits throughout the 19th and 20th centuries reveals differential responses to increases in atmospheric CO2. Global Ecol. Biogeogr. 23, 1209–1214. doi: 10.1111/geb.12211
Renzi J. J., He Q., Silliman B. R. (2019). Harnessing Positive species interactions to enhance coastal wetland restoration. Front. Ecol. Evolution 7. doi: 10.3389/fevo.2019.00131
Richards D. R., Friess D. A. (2017). Characterizing coastal ecosystem service trade-offs with future urban development in a tropical city. Environ. Management 60, 961–973. doi: 10.1007/s00267-017-0924-2
Riegel S., Kuhfuss L., Stojanovic T. (2023). Nature-based solutions for climate change mitigation: Assessing the Scottish Public’s preferences for saltmarsh carbon storage. Ecol. Economics 211, 107863. doi: 10.1016/j.ecolecon.2023.107863
Rogers K., Kelleway J. J., Saintilan N., Megonigal J. P., Adams J. B., Holmquist J. R., et al. (2019). Wetland carbon storage controlled by millennial-scale variation in relative sea-level rise. Nature 567, 91–95. doi: 10.1038/s41586-019-0951-7
Ross F. W. R., Boyd P. W., Filbee-Dexter K., Watanabe K., Ortega A., Krause-Jensen D., et al. (2023). Potential role of seaweeds in climate change mitigation. Sci. Total Environment 885, 163699. doi: 10.1016/j.scitotenv.2023.163699
Ruiz-Rosero J., Ramirez-Gonzalez G., Viveros-Delgado J. (2019). Software survey: ScientoPy, a scientometric tool for topics trend analysis in scientific publications. Scientometrics 121, 1165–1188. doi: 10.1007/s11192-019-03213-w
Saintilan N., Rogers K., Mazumder D., Woodroffe C. (2013). Allochthonous and autochthonous contributions to carbon accumulation and carbon store in southeastern Australian coastal wetlands. Estuar. Coast. Shelf Sci. 128, 84–92. doi: 10.1016/j.ecss.2013.05.010
Samper-Villarreal J., Mumby P. J., Saunders M. I., Roelfsema C., Lovelock C. E. (2018). Seagrass organic carbon stocks show minimal variation over short time scales in a heterogeneous subtropical seascape. Estuaries Coasts 41, 1732–1743. doi: 10.1007/s12237-018-0381-z
Sasmito S. D., Taillardat P., Clendenning J. N., Cameron C., Friess D. A., Murdiyarso D., et al. (2019). Effect of land-use and land-cover change on mangrove blue carbon: A systematic review. Global Change Biol. 25, 4291–4302. doi: 10.1111/gcb.14774
Schile L. M., Kauffman J. B., Crooks S., Fourqurean J. W., Glavan J., Megonigal J. P. (2017). Limits on carbon sequestration in arid blue carbon ecosystems. Ecol. Applications 27, 859–874. doi: 10.1002/eap.1489
Schultz M. A., Janousek C. N., Brophy L. S., Schmitt J., Bridgham S. D. (2023). How management interacts with environmental drivers to control greenhouse gas fluxes from Pacific Northwest coastal wetlands. Biogeochemistry 165, 165–190. doi: 10.1007/s10533-023-01071-6
Senger D. F., Hortua D. A. S., Engel S., Schnurawa M., Moosdorf N., Gillis L. G. (2021). Impacts of wetland dieback on carbon dynamics: A comparison between intact and degraded mangroves. Sci. Total Environment 753, 141817. doi: 10.1016/j.scitotenv.2020.141817
Serrano O., Kelleway J. J., Lovelock C., Lavery P. S. (2019). Conservation of blue carbon ecosystems for climate change mitigation and adaptation in Coastal Wetlands: an Integrated Ecosystem Approach (Elsevier), United States, 965–996. doi: 10.1016/B978-0-444-63893-9.00028-9
Serrano O., Lavery P. S., López-Merino L., Ballesteros E., Mateo M. A. (2016). Location and associated carbon storage of erosional escarpments of seagrass posidonia mats. Front. Mar. Sci. 3. doi: 10.3389/fmars.2016.00042
Serrano O., Lavery P. S., Rozaimi M., Mateo M. A. (2014). Influence of water depth on the carbon sequestration capacity of seagrasses. Global Biogeochemical Cycles 28, 950–961. doi: 10.1002/2014GB004872
Shapiro A. C., Trettin C. C., Küchly H., Alavinapanah S., Bandeira S. (2015). The mangroves of the Zambezi Delta: Increase in extent observed via satellite from 1994 to 2013. Remote Sensing 7, 16504–16518. doi: 10.3390/rs71215838
Smit A. J., Brearley A., Hyndes G. A., Lavery P. S., Walker D. I. (2005). Carbon and nitrogen stable isotope analysis of an Amphibolis griffithii seagrass bed. Estuar. Coast. And Shelf Sci. 65, 545–556. doi: 10.1016/j.ecss.2005.07.002
Stovall A. E.L., Lagomasino D., Lee S. K., Simard M., Thomas N. M., Trettin C., et al. (2020). Evaluating current and future sensor-specific biomass calibration in the tallest mangrove forest on earth. IGARSS 2020-2020 IEEE International Geoscience and Remote Sensing Symposium, 5964–5967. doi: 10.1109/IGARSS39084.2020.9324460
Sutton-Grier A. E., Moore A. K., Wiley P. C., Edwards P. E. T. (2014). Incorporating ecosystem services into the implementation of existing U.S. natural resource management regulations: Operationalizing carbon sequestration and storage. Mar. Policy 43, 246–253. doi: 10.1016/j.marpol.2013.06.003
Svane N., Lange T., Egemose S., Dalby O., Thomasberger A., Flindt M. R. (2022). Unoccupied aerial vehicle-assisted monitoring of benthic vegetation in the coastal zone enhances the quality of ecological data. Prog. Phys. Geography-Earth Environment 46, 232–249. doi: 10.1177/03091333211052005
Tanner M. K., Moity N., Costa M. T., Jarrin J. R. M., Aburto-Oropeza O., Salinas-de-León P. (2019). Mangroves in the Galapagos: Ecosystem services and their valuation. Ecol. Economics 160, 12–24. doi: 10.1016/j.ecolecon.2019.01.024
Temmink R. J. M., Lamers L. P. M., Angelini C., Bouma T. J., Fritz C., van de Koppel J., et al. (2022). Recovering wetland biogeomorphic feedbacks to restore the world’s biotic carbon hotspots. Science 376, 594. doi: 10.1126/science.abn1479
Theuerkauf S. J., Puckett B. J., Theuerkauf K. W., Theuerkauf E. J., Eggleston D. B. (2017). Density-dependent role of an invasive marsh grass, Phragmites australis, on ecosystem service provision. PloS One 12, e0173007. doi: 10.1371/journal.pone.0173007
Thomas S. (2014). Blue carbon: Knowledge gaps, critical issues, and novel approaches. Ecol. Economics 107, 22–38. doi: 10.1016/j.ecolecon.2014.07.028
Tokoro T., Hosokawa S., Miyoshi E., Tada K., Watanabe K., Montani S., et al. (2014). Net uptake of atmospheric CO2 by coastal submerged aquatic vegetation. Global Change Biol. 20, 1873–1884. doi: 10.1111/gcb.12543
Tong C., Zhang L. H., Wang W. Q., Gauci V., Marrs R., Liu B. G., et al. (2011). Contrasting nutrient stocks and litter decomposition in stands of native and invasive species in a sub-tropical estuarine marsh. Environ. Res. 111, 909–916. doi: 10.1016/j.envres.2011.05.023
Tran T. V., Reef R., Zhu X. (2022). A review of spectral indices for mangrove remote sensing. Remote Sensing 14, 4868. doi: 10.3390/rs14194868
Tu Q., Yang S. Y., Zhou Q. L., Yang J. (2015). Sediment transport and carbon sequestration characteristics along mangrove fringed coasts. Acta Oceanologica Sinica 34, 21–26. doi: 10.1007/s13131-015-0614-y
Tyllianakis E., Callaway A., Vanstaen K., Luisetti T. (2019). The value of information: Realising the economic benefits of mapping seagrass meadows in the British Virgin Islands. Sci. Total Environment 650, 2107–2116. doi: 10.1016/j.scitotenv.2018.09.296
Ullman R., Bilbao-Bastida V., Grimsditch G. (2012). Including Blue Carbon in climate market mechanisms. Ocean Coast. Management 83, 15–18. doi: 10.1016/j.ocecoaman.2012.02.009
Vanderklift M. A., Herr D., Lovelock C. E., Murdiyarso D., Raw J. L., Steven A. D. L. (2022). A guide to international climate mitigation policy and finance frameworks relevant to the protection and restoration of blue carbon ecosystems. Front. Mar. Sci. 9. doi: 10.3389/fmars.2022.872064
van Eck N. J., Waltman L. (2017). Citation-based clustering of publications using CitNetExplorer and VOSviewer. Scientometrics 111, 1053–1070. doi: 10.1007/s10811-010-9604-9
Vázquez-Lule A., Seyfferth A. L., Limmer M. A., Mey P., Guevara M., Vargas R. (2022). Hyperspectral Reflectance for measuring canopy-level nutrients and photosynthesis in a salt marsh. J. Geophysical Research-Biogeosciences 127, e2022JG007088. doi: 10.1029/2022JG007088
Vo T. Q., Kuenzer C., Oppelt N. (2015). How remote sensing supports mangrove ecosystem service valuation: A case study in Ca Mau province, Vietnam. Ecosystem Services 14, 67–75. doi: 10.1016/j.ecoser.2015.04.007
Wang F., Tang J. W., Ye S. Y., Liu J. H. (2021). Blue carbon sink function of Chinese coastal wetlands and carbon neutrality strategy. Bull. Chin. Acad. Sci. 36, 241–251. doi: 10.16418/j.issn.1000-3045.20210215101-en
Wang C. L., Zhao X. S., Chen X. Y., Xiao C., Fan X. W., Shen C., et al. (2023). Variations in CO2 and CH4 exchange in response to multiple biophysical factors from a Mangrove Wetland Park in Southeastern China. Atmosphere 14, 805. doi: 10.3390/atmos14050805
Wedding L. M., Moritsch M., Verutes G., Arkema K., Hartge E., Reiblich J., et al. (2021). Incorporating blue carbon sequestration benefits into sub-national climate policies. Global Environ. Change-Human Policy Dimensions 69, 102206. doi: 10.1016/j.gloenvcha.2020.102206
Xiong Y. M., Liao B. W., Wang F. M. (2018). Mangrove vegetation enhances soil carbon storage primarily through in situ inputs rather than increasing allochthonous sediments. Mar. pollut. Bulletin 131, 378–385. doi: 10.1016/j.marpolbul.2018.04.043
Ya C., Anderson W., Jaffé R. (2015). Assessing dissolved organic matter dynamics and source strengths in a subtropical estuary: Application of stable carbon isotopes and optical properties. Continental Shelf Res. 92, 98–107. doi: 10.1016/j.csr.2014.10.005
Yadav K. K., Gupta N., Prasad S., Malav L. C., Bhutto J. K., Ahmad A., et al. (2023). An eco-sustainable approach towards heavy metals remediation by mangroves from the coastal environment: A critical review. Mar. pollut. Bulletin 188, 114569. doi: 10.1016/j.marpolbul.2022.114569
Yang Y. S. (2017). The impacts of global environmental changes on typical ecosystems: Status, challenges and trends. Ecol. Soc. China 37, 1–11. doi: 10.5846/stxb201701050044
Yong W. T. L., Thien V. Y., Rupert R., Rodrigues K. F. (2022). Seaweed: A potential climate change solution. Renewable Sustain. Energy Rev. 159, 112222. doi: 10.1016/j.rser.2022.112222
Young M. A., Serrano O., Macreadie P. I., Lovelock C. E., Carnell P. (2021). Ierodiaconou, D. National scale predictions of contemporary and future blue carbon storage. Sci. Total Environment 800, 149573. doi: 10.1016/j.scitotenv.2021.149573
Yu Y., Wang S. Y., Yu P. F., Wang D. S., Hu B. L., Zheng P., et al. (2024). A bibliometric analysis of emerging contaminants (ECs) (2001-2021): Evolution of hotspots and research trends. Sci. Total Environment 907, 168116. doi: 10.1016/j.scitotenv.2023.168116
Zhao C. P., Sadula M., Huang X. M., Yang Y. L., Gong Y., Yang S. (2022). The Game model of blue carbon collaboration along MSR-From the regret theory perspective. Mathematics 10, 1006. doi: 10.3390/math10061006
Zheng Y. H., Jin R. J., Zhang X. J., Wang Q. X., Wu J. P. (2019). The considerable environmental benefits of seaweed aquaculture in China. Stochastic Environ. Res. Risk Assessment 33, 1203–1221. doi: 10.1007/s00477-019-01685-z
Keywords: blue carbon, carbon sequestration, climate change, ecosystem services, hot topics, research trend
Citation: Wang S, Yan D, Wang C, Wu L and Huang Y (2024) A bibliometric analysis of blue carbon (1993–2023): evolution of research hot topics and trends. Front. Mar. Sci. 11:1430545. doi: 10.3389/fmars.2024.1430545
Received: 10 May 2024; Accepted: 08 August 2024;
Published: 30 August 2024.
Edited by:
Stelios Katsanevakis, University of the Aegean, GreeceReviewed by:
Carolyn J. Ewers Lewis, Flagler College, United StatesJames Morris, University of South Carolina, United States
Copyright © 2024 Wang, Yan, Wang, Wu and Huang. This is an open-access article distributed under the terms of the Creative Commons Attribution License (CC BY). The use, distribution or reproduction in other forums is permitted, provided the original author(s) and the copyright owner(s) are credited and that the original publication in this journal is cited, in accordance with accepted academic practice. No use, distribution or reproduction is permitted which does not comply with these terms.
*Correspondence: Shanshan Wang, d3NzQGhhdXN0LmVkdS5jbg==