- 1Universidad Pontificia Bolivariana - School of Engineering, Medellín, Antioquia, Colombia
- 2Monterey Bay Aquarium Research Institute - Seafloor Processes - MBARI, Moss Landing, CA, United States
Areas of the seafloor enriched with seeping fluids host unique chemosynthetic communities, and their interactions not only linked to the presence of oil and gas resources, but directly impact global geochemical cycles. These ecosystems can be found in diverse geological settings, spanning from passive to active continental margins, and encompass environments such as mid-ocean ridges, seamounts, cold brine lakes, mud volcanoes, and carbonate pinnacles. This review aims to examine seep environments in the Western Atlantic, the Caribbean, and Colombia, with a focus on understanding: I) their nature and origin, Itheir associated seabed characteristics, III) the biological communities directly connected to them, and IV) the chemistry and flow of the emitted fluids. The review identifies the close relationship of cold seeps to various geomorphological features, including linear diapir systems, salt diapirs resulting from salt tectonics, regions of mud volcanism, and compact seafloor mounds likely associated with buried mud diapirs. However, existing data on the fauna within these environments predominantly focus on megafauna, such as vestimentiferan tubeworms (Annelida), mussels, and vesicomyid bivalves (Mollusca), overlooking the crucial role of communities of small organisms, including fungi or macrofauna. This review highlights the absence of a consistent consensus among researchers regarding the factors controlling fauna distribution and presence in seep environments. Various authors have put forth divergent factors that influence seep community structures, with some emphasizing water depth, others the geological environment, and some the relationship with geomorphological conditions and fluid emissions. These findings underscore the need for further research into environmental factors and their roles in the observed distribution, presenting a promising avenue for future investigations.
1 Introduction
Cold seeps represent locations where hydrocarbon-rich fluids, often containing methane or hydrogen sulfide, seep from the seafloor. They serve as unique seabed regions hosting chemosynthetic communities, exerting an influence on global geochemical cycles, and offering valuable insights into hydrocarbon systems (Levin, 2005; Cordes et al., 2009; Levin et al., 2016; Kennicutt, 2017). These seeps occur across both passive and active continental margins (Sibuet and Olu, 1998) and manifest in a diverse array of geological settings, encompassing continental margins, mid-ocean ridges, seamounts, cold brine lakes, and carbonate structures, among other environments (Levin et al., 2016).
Cold seeps are prevalent in active and passive continental margins across all the oceans of the world (Sibuet and Olu, 1998; Bowden et al., 2013; Joye, 2020). These seeps contribute significantly to the environmental diversity of the seafloor and sustain specialized, well-organized communities of chemosymbiotic-bearing species. Their impact on diversity is multifaceted, as they furnish substrate, sustenance, refuge, and opportunities for diverse biotic interactions (Levin, 2005; Gracia Clavijo, 2018), as in the case of communities of Bathymodiolinae (family Mytilidae) (Olu-Le Roy et al., 2007) or foraminifera such as Epistominella exigua, Reophax nodulosus, Nodellummembranaceum (Robinson et al., 2004), among others.
The most extensively studied cold seep communities comprise tubeworms, mussels, and symbiont-bearing clams (Levin, 2005), often belonging to genera also found at hydrothermal vents (Paull et al., 1984; Sibuet and Olu, 1998; Levin, 2005; Cordes et al., 2007; Levin et al., 2012). Seeps and vents share common taxa, including siboglinid tubeworms, vesicomid clams, and bathymodiolin mussels, among others (Paull et al., 1984; Sibuet and Olu, 1998; Cordes et al., 2007; Levin et al., 2012). Nevertheless, typically, these species do not overlap with more than 20 percent of their species, even when vent and seep sites are in close geographical proximity (Sibuet and Olu, 1998). Some species occur in both cold methane-rich seeps and sedimented vents, such as the siboglinid tubeworms Lamellibrachia barham and Escarpia spicata, Parascarpia echinospica, the vesicomid clams Archivesica gigas, Phreagena okutanii, and Calyptogena solidissima, as well as the bathymodioline mussels Bathymodiolus japonicus and Bathymodiolus platifrons (Craddock et al., 1995; Black et al., 1997; Gill et al., 2005; Watanabe et al., 2010).
In the literature, there are very different and contradictory findings about which factors explain the community structures of cold seeps. Various authors have differing perspectives on the factors influencing species composition in the community, with some emphasizing depth (Cordes et al., 2007; Olu et al., 2010), and others highlighting the importance of geomorphology and fluids (Gill et al., 2005; Amon et al., 2017; Duenas et al., 2021), and still others underscoring the combined influence of the marine environment and depth (Watanabe et al., 2010).
We study seepage environments in the Western Atlantic, Caribbean, and Colombia because these ecosystems serve as hotspots for unique life forms adapted to extreme conditions, and they are important for biodiversity, ecological interactions, climate change impacts, economic resources, and conservation efforts. The main objective of this study is to investigate the interconnections between community structures, geomorphology, and fluid chemistry in cold seepage environments within the area of interest. This review aims to enhance our comprehension of the following key aspects: I) the nature and origins of cold seeps; II) the geological attributes of the seabed hosting these seeps; III) the specific communities associated with them; and IV) the chemistry of the fluids they emit.
There exist inconsistencies in the terminology employed, including the usage of “cold seeps,” “methane seeps,” and “hydrocarbon seeps.” Additionally, there is confusion regarding the distinction between “hydrothermal vents” and “hydrocarbon seepage” (Campbell, 2006; Schweitzer and Feldmann, 2008). Some researchers find similarities between the environments of hydrothermal vents and hydrocarbon seeps, as both release sulfide and/or methane (Olu et al., 1996; Van Dover et al., 2002; Campbell, 2006). In this review, the terms “hydrocarbon seeps,” “cold seeps,” “seepage,” “seeps,” and “methane seeps” will collectively refer to environments where hydrocarbon release occurs in oceanic settings at temperatures similar to or “low” compared to the surrounding environment (Sibuet and Olu, 1998; Campbell, 2006). Meanwhile, the term “hydrothermal vents” pertains specifically to environments characterized by the expulsion of hot water, often reaching temperatures of up to 400°C, typically found in deep ocean regions heated by geothermal processes and enriched with various chemicals (Van Dover, 2000; Van Dover et al., 2002).
2 Methods
2.1 Study locations
For this review, our primary focus is on the Western Atlantic region, with special emphasis on the Caribbean and Colombia, given the limited existing knowledge in these areas (Gracia et al., 2012; Cortes et al., 2018; Carvajal-Arenas et al., 2020; Duenas et al., 2021). Figure 1 illustrates the studies and specific regions that have been considered for this comprehensive analysis (Table 1).
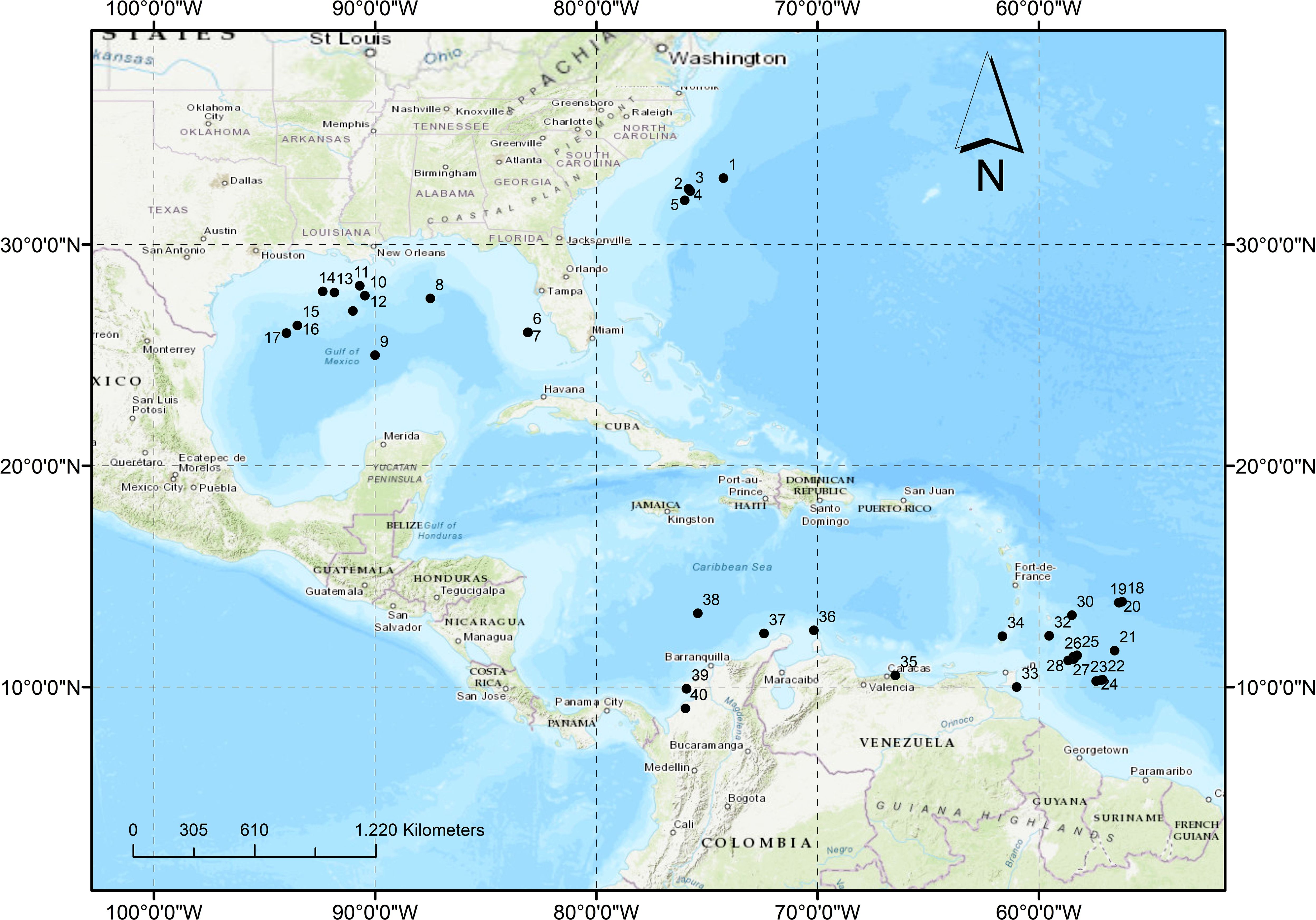
Figure 1 Location of the studied sites with cold seeps ecosystems in the western Atlantic, the Caribbean, and Colombia. The numbers are contrasted in Table 1 with their respective reference.
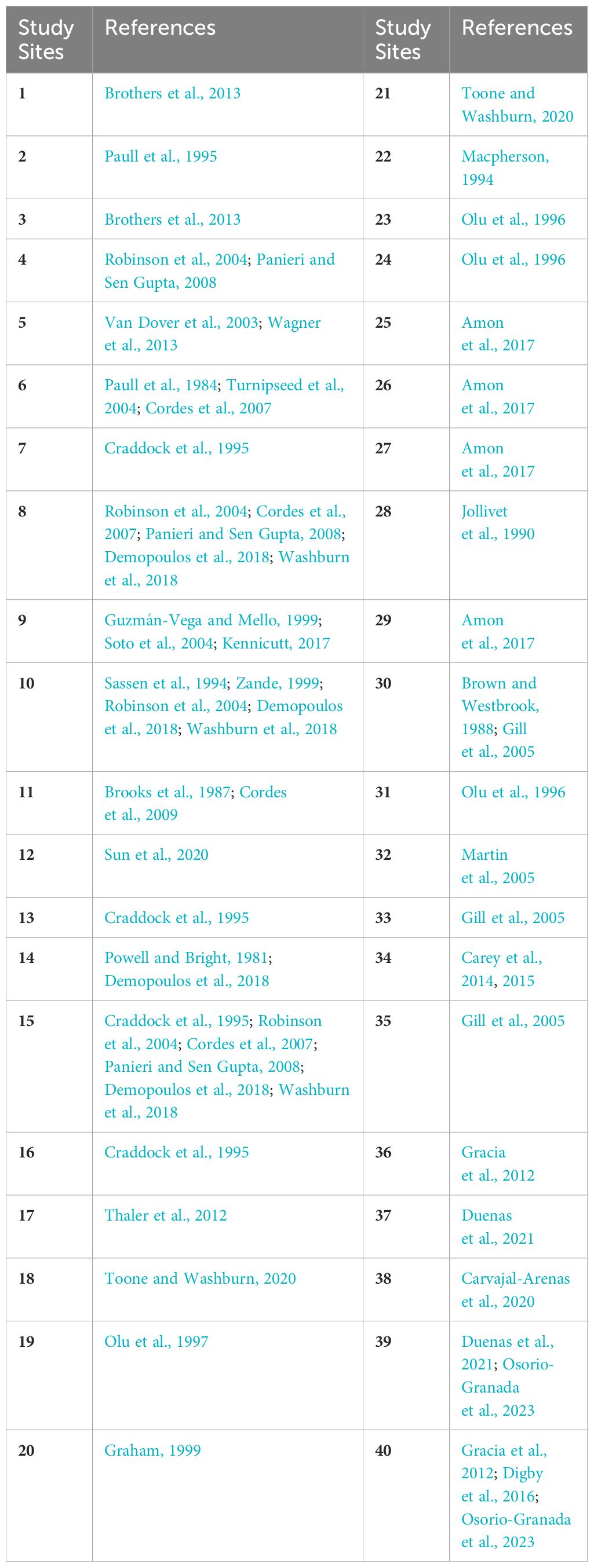
Table 1 Number of the area indicated on the map with the reference (Appendix 1 in Supplementary Material).
2.2 Methodological assessments
To comprehensively assess the diverse methodologies employed for evaluating seep environments in the region, we have compiled a detailed table outlining the specific sampling methods and the types of samples analyzed (Appendix 2). It is noteworthy that over 40% of the investigations have utilized Remotely Operated Vehicles (ROV) or Human Occupied Vehicles (HOVs) (Olu et al., 1996; Olu-Le Roy et al., 2007; Toone and Washburn, 2020), as depicted in Figure 2. Other methodologies, albeit used to a lesser extent, include: I) towed camera system (Jollivet et al., 1990; Paull et al., 1995; Duenas et al., 2021); II) box corer (Demopoulos et al., 2018), which is a sampler of benthic communities (Boland and Rowe, 1991); III) collection of individuals stored in Natural History museums (Kiel and Hansen, 2015); among others (Figure 2). Other methodologies contributed less than 1% and were categorized under the “other” category, which includes approaches such as phylogenetic analysis, semiballoon trawling net, and so forth.
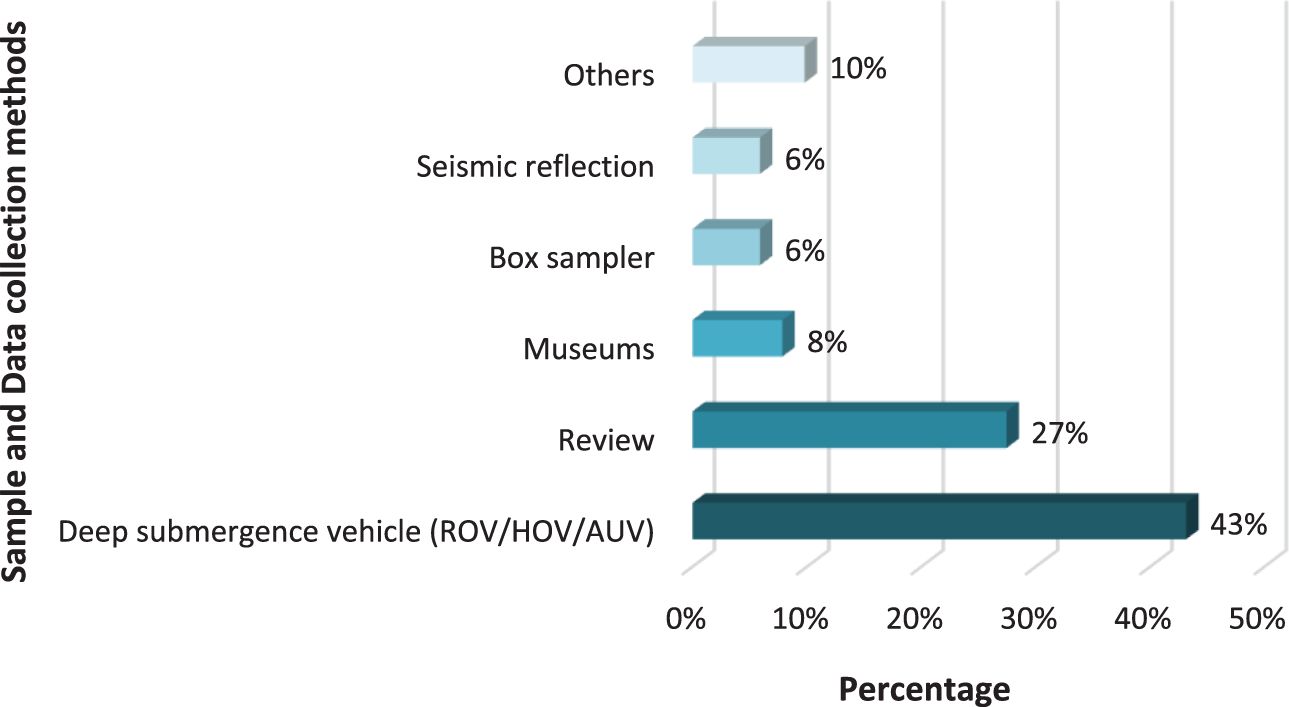
Figure 2 Percentage contribution of the different methodologies used in the research analyzed in the bibliographic review. The “Others” category includes methodologies with a contribution of less than 10%, such as Phylogenetic analysis, Semiballoon trawling net, etc (Appendix 2 in Supplementary Material).
The limited variability observed in the choice of sampling methods reflects a notable information gap within the field. Several researchers have emphasized the insufficiency of studies in addressing numerous questions regarding seeps and reducing environments overall (Van Dover and Lichtwardt, 1986; Zande, 1999; Levin, 2005; Nagano et al., 2010; Thaler et al., 2012; Levin et al., 2016). Some have also noted that small groups of individuals have often been overlooked in investigations of cold seeps and deep-sea hydrothermal vents (Graham, 1999; Levin, 2005; Amon et al., 2017). These observations show the need for conducting further research of different types, to close these information gaps and advance our understanding of these complex ecosystems.
3 Discussion
3.1 Geological settings: geographic distribution and geological context
3.1.1 North Western Atlantic
The Blake Ridge Diapir Seep, situated in the southwestern United States, occupies depths ranging from 2155 to 2600 meters. This region displays extensive areas with gas hydrates. Isotopic data strongly suggest that the methane (CH4) found in these hydrates originates from microbial processes (Paull et al., 1995; Van Dover et al., 2003; Brothers et al., 2013).
Paull et al. (1995) have documented a linear system comprising approximately 19 diapirs that rise from near the base of the Carolina Trough. Some of these diapirs extend high enough to disrupt the seafloor, contributing to the formation of accommodation faults and the mobilization of fluids (Van Dover et al., 2003). Within this area, the transport of methane and hydrogen sulfide towards the water column is recorded, likely occurring through fault systems (Paull et al., 1995; Brothers et al., 2013). In this area, multiple pockmarks have also been reported, presumably originating from methane migrating from the seabed through porous spaces and the collapse of sediments (Paull et al., 1995; Wagner et al., 2013) (See Figure 1: Studied sites 1 to 5) (Table 2).
3.1.2 Gulf of Mexico
In the Gulf of Mexico, seepage is a predominant feature. It is an area where approximately 70% of the total reserves and production of Mexico are located (Guzmán-Vega and Mello, 1999). The methane emitted in these seeps has several origins, including biogenic (resulting from methane generated by bacterial metabolism), thermogenic (arising from the heating of organic matter at high temperatures deep in the subsurface), or a combination of both. The fluids emanating from these seeps encompass a range of substances, including gases, liquids, asphalts, brines, and tars (Powell and Bright, 1981; Powell et al., 1983; Robinson et al., 2004; Turnipseed et al., 2004; Cordes et al., 2007, 2009; Kennicutt, 2017).
To the north of the GoM, the presence of seeps can be attributed to salt tectonics, a phenomenon documented by various studies (Brooks et al., 1987; Sassen et al., 1994; Soto et al., 2004; Cordes et al., 2009; Kennicutt, 2017; Roelofse et al., 2020; Sun et al., 2020). Salt tectonics give rise to saline structures within the subsurface, which in turn create pathways for fluid migration. These pathways extend not only towards the reservoir rocks but also toward the surface (Kennicutt, 2017). Many of these seeps are of petrogenic origin and tend to cluster near salt structures, for example in the “Serendipity Gas Seepage Area,” where an estimated 19,000 seeps are concentrated within a relatively small area of approximately 6,000 square kilometers on the continental shelf (DeGolyer, 1932; Watkins and Worzel, 1978; Geyer and Giammona, 1980; Hovland et al., 1993).
South of the GoM, in the Campeche area, saline diapirs rising from evaporite deposits have been reported (Guzmán-Vega and Mello, 1999; MacDonald et al., 2004; Naehr et al., 2009; Roelofse et al., 2020). Changes in seabed density, interpreted as sedimentary layers with high permeability, facilitate the upward flow of a gas-solid mixture of hydrocarbons. Additionally, bubbling at the surface is observed, likely stemming from degassing of the sediment (Soto et al., 2004; Solomon et al., 2009) (See Figure 1: Studied sites 6 to 17) (Table 3).
3.1.3 Caribbean
The seafloor in the vicinity of Trinidad and Tobago is home to an extensive region featuring mud volcanoes and cold seeps. These geological phenomena are a result of the compression of fluid-rich marine sediments occurring as the Atlantic plate subducts beneath the Caribbean plate within the forearc of the Lesser Antilles (Carey et al., 2014, 2015; Amon et al., 2017).
In Grenada, cold seepage is observed in the distal margins of a debris avalanche deposit originating from the Kick’em Jenny submarine volcano. Remarkably, this is the sole known active submarine volcano within the Caribbean Sea (Martin et al., 2005; Carey et al., 2014, 2015). These seeps are situated on steep slopes characterized by unconsolidated and deformed hemipelagic sediments, a feature potentially associated with fluid overpressure generated during the volcano’s collapse (Carey et al., 2014, 2015; Raineault and Flanders, 2019).
Gill et al. (2005) examined ancient cold seep sites in Barbados, Trinidad, and northern Venezuela. The hydrocarbon seepage sustaining chemosymbiotic ecosystems at these sites was linked to tectonic activity in the southern Caribbean region (Olu et al., 1996, 1997; Gill et al., 2005). For instance, the Scotland District in the northeast of Barbados features carbonates and fossils associated with two distinct tectonic units: the Suboceanic Fault Zone and a diapiric mix, and the Suboceanic Fault Zone, where the tectonic convergence between accretionary prism sediments and layers of overlying sediment thrust from the forearc basin takes place (Brown and Westbrook, 1988; Gill et al., 2005).
Further investigations reveal that active cold seeps in the southern Barbados Prism exhibit varying origins, with some being biogenic (Toone and Washburn, 2020) and others thermogenic (Olu et al., 1996). These seeps are typically situated on the tops and upper slopes of diapiric volcanoes and anticlinal ridges, intersected by numerous faults through which subducting fluids circulate and undergo diagenesis (Jollivet et al., 1990; Olu et al., 1996, 1997; Gill et al., 2005) (See Figure 1: Studied sites 18 to 35) (Table 4).
3.1.4 Colombian Caribbean
Located to the northeast of Colombia, the Sinú-San Jacinto Basin, also known as the Accretionary Sinú Prism (ASP), is a prominent geological feature within the Colombian-Caribbean Basin. The ASP consists of two distinct belts with closely adjacent folds: I) The Paleogene San Jacinto fold belt, which extends northward. II) The Neogene Sinú fold belt, situated along the eastern margin of the San Jacinto fold belt (Sánchez and Permanyer, 2006; Cortes et al., 2018; Rodríguez et al., 2021). The ASP is characterized by mud diapirism and hosts numerous hydrocarbon seeps. Particularly noteworthy are the very thick sedimentary sequences marked by mud diapirism near the continent and point thrust systems on the slope of the continental shelf (Gracia et al., 2012; Cortes et al., 2018; Osorio-Granada et al., 2023).
Cortes et al. (2018) conducted research indicating that the Late Cretaceous Cansona Formation and the Paleogene Arroyo Seco Formation within the basin have the potential to generate liquid hydrocarbons. Furthermore, the Neogene stratigraphic sequence exhibits significant potential for gas production. In support of these findings, both 2D and 3D seismic reflection data have revealed direct and widespread hydrocarbon indicators from deep-water successions of the Eastern Colombia Basin (BCE) and the adjacent South Caribbean Deformed Belt (SCDB). These indicators collectively suggest the existence of an extensive, albeit vaguely defined petroleum system (Carvajal-Arenas et al., 2020).
Recently, in the Sinu Fold Belt, bathymetric data have identified dome structures and active and inactive pockmarks, structures that are strongly associated with gas seepages on the continental margins (Osorio-Granada et al., 2023).
The domes were found at depths between 25 and 1300 m, among the shelf and the continental slope; ranging from 15 to 45 m in height, and with diameters at the base between 250 m and 2 km. Some show some type of plume or gas leak above the water column, revealing areas of central vents, which probably constitute the fluid source for the formation of the dome structure. The pockmarks were found mainly on the continental shelf. Due to their expression, it is very difficult to differentiate them in the slope area. These structures range in depth from 3 to 15 m and diameters ranging from 50 to 170 m. The origin of these structures is related to the expulsion of shallow gas pockets, indicating that gas is abundant in the surface sediments, demonstrated by the frequency of gas plumes detected in bottom profiles (Osorio-Granada et al., 2023) (See Figure 1: Studied sites 35 to 40) (Table 5).
3.2 Biological settings: fauna related to cold seeps
Numerous reduced chemicals are found in cold seeps and hydrothermal vents that can be used by chemosynthetic prokaryotes as an energy source for carbon fixation (McMullin et al., 2007). This presents the potential for sustaining life for organisms reliant on this primary chemosynthetic production, either by feeding on free-living bacteria or forming symbiotic relationships with chemosynthetic bacteria to obtain nutrients (Kennicutt and Burke, 1995). This review explores diverse investigations of the benthic fauna associated with cold seeps. It is important to keep in mind that the benthic fauna is divided into epifauna, the fauna that lives on the aquatic bottom, and the infauna, which lives among the sediments (Guzmán and y Ardila, 2004). Furthermore, it is classified based on size: Megafauna (>1cm) (Grassle et al., 1975; Gates and Jones, 2012); Macrobenthos or macrofauna (>500µm); Meiobenthos or meifauna (<500µm and >63µm) and Microbenthos or microfauna (<63µm) (Pech Pool and Ardisson Herrera, 2010).
3.2.1 Megafauna
The majority of publications focused on fauna within these environments primarily center on megafauna studies. Generally, the most frequently observed megafauna in seepage environments includes vestimentifers and mussels, alongside vesicomid bivalves, shrimps, and galatheid crabs (Paull et al., 1984; Macpherson, 1994; Sibuet and Olu, 1998; Van Dover et al., 2003; Levin, 2005; Cordes et al., 2007; Olu-Le Roy et al., 2007; Kiel, 2016).
These communities are usually dominated by large bivalves and, in some cases, vestimentiferous shrublands (Jollivet et al., 1990; Van Dover et al., 2003; Turnipseed et al., 2004).
Studies along the base of the Florida Escarpment have revealed the presence of cold seep communities, which include several organisms taxonomically related to those found in hydrothermal vents. Large bivalves often dominate these communities and, in some cases, vestimentiferan thickets (Jollivet et al., 1990; Van Dover et al., 2003; Turnipseed et al., 2004). In both cold seeps and hydrothermal vents, bacterial endosymbionts play a crucial role by oxidizing reduced compounds for energy while fixing carbon dioxide, thus providing an organic food source for their hosts (Brooks et al., 1987; Jollivet et al., 1990; Turnipseed et al., 2004; Kiel and Hansen, 2015). Mussel banks are considered one of the most similar habitats shared between geographically distant chemosynthetic ecosystems, although it’s noted that this similarity is only a preliminary approximation (Turnipseed et al., 2004). These findings strongly suggest that deep-sea chemosynthesis remains the most plausible explanation for the existence of highly productive deep-sea communities (Paull et al., 1984; Jollivet et al., 1990; Olu et al., 1997).
An example of this is the presence of bathymodioline mussels and vesicomyid clams reported in this area (See Figure 1: Studied sites 1 to 5). These organisms depend on symbiotic chemosynthetic bacteria for their nutrition, which limits them to areas where chemical flux is sufficient for this process (Sibuet and Olu, 1998; Wagner et al., 2013). The ‘bulls-eye’ distribution of mussels concerning the seabed pockmarks indicates that the mussels are centered on bathymetric irregularities generated by point-source methane seepage, where fluid flux and methane and sulfide concentrations are greatest (Wagner et al., 2013). However, Wagner et al. (2013) do not exclude the possibility that the chemistry of the fluids in the sediments of pockmarks may not be suitable for the survival of clams or that the fluid flux in each pockmark may vary, both over time and in relation to adjacent pockmarks, leaving questions about which specific factors control the zonation of clams and mussels.
Olu-Le Roy et al. (2007) observed a notable similarity between the communities of the Bathymodiolinae subfamily (family Mytilidae) in deep waters, specifically at depths exceeding 3,000 meters, within cold seep environments along the equatorial belt of the African Atlantic. These findings indicated a resemblance to the communities found in seeps within the GoM and Barbados. Furthermore, there was a striking similarity between deep oceanic communities in the GoM (400 to 3300m) and seep communities within the Accretionary Prism of Barbados, rather than with those localized at the upper slope of the GoM or Blake Ridge. This suggests a possible capacity for dispersal in terms of taxonomic relationships across the equatorial belt of the Atlantic, indicating the presence of amphiatlantic species (Olu-Le Roy et al., 2007; Olu et al., 2010).
In the GoM, community development is probably determined by the methane or sulfur compounds that seepages give to the benthos, as well as by the geological characteristics of the surface sediments. Seep mussels are found in areas defined by flowage patterns of methane-saturated brine at the sediment/seawater interface, suggesting that brine pools can sustain mussel communities (MacDonald et al., 1990; MacDonald, 1998; Cordes et al., 2007, 2009). Moreover, groups of tubeworms are more commonly associated with mound topography on the crests of grabens or half-grabens. Clam communities tend to be more dispersed over larger areas than tubeworm or mussel communities and can be infaunal, like the Lucinidae and Thyasiridae groups, or epibenthic, like the Vesicomyidae (MacDonald et al., 1990; MacDonald, 1998) (See Figure 1: Studied sites 6 to 17).
At the Barbados Accretion Prism, research conducted by Olu et al. (1996) and Gill et al. (2005) has provided insights into benthic communities that rely on chemosynthesis processes. These communities are primarily found in areas where geological structures are influenced by diapirism, encompassing features such as mud volcanoes, domes, and an anticline ridge. Within these mud volcanoes, symbiotic sponges have been identified. These sponges host bacteria that directly utilize the methane provided by the seeping fluids for growth and development, a phenomenon known as methanotrophic symbiosis. This process which is carried out by sponges and their symbionts, supports fluid expulsion patterns (Olu et al., 1997). It can be reasonably inferred that various types of geological features, including mud volcanoes, diatremes, diapirs, and intermediate structures within the Barbados Prism, are colonized by chemosynthetic communities. This inference is supported by the presence of gas hydrates containing significant amounts of methane throughout the region where mud volcanoes are located (Brown and Westbrook, 1988; Olu et al., 1997). There is a particularly different site where no evidence of historical seepage is present. However, the recorded thiotrophy (organisms living on sulfide or other reduced sulfur compounds) indicates the transport of chemosynthetically derived material to this site from nearby seeps or weak seepages that have never been strong enough to support a flourishing community of chemosymbiotic seep organisms dependent on methanotrophy or thiotrophy. This suggests that the influence of seep areas may extend further than previously thought (Demopoulos et al., 2018; Toone and Washburn, 2020) (See Figure 1: Study sites 18 to 35).
In the Accretionary Sinú Prism (ASP) region of Colombia, various bivalve species belonging to the families Vesicomyidae, Lucinidae, Solemyidae, and Thyasiridae have been collected as reported by Gracia et al. (2012). Additionally, the presence of live specimens of bivalves such as Calyptogena ponderosa and Vesicomya caribbea has been documented in the northern area near the mouth of the Magdalena River. This discovery suggests the existence of active methane seep sites in the region. These chemosynthetic communities are highly adapted to environments rich in sulfur compounds and often constitute the dominant taxa within these reducing environments (Gracia et al., 2012).
To the south of the ASP and the north of the La Guajira basin, the dominant megafauna inhabiting cold seep communities includes tube worms (Lamellabranchia sp. and Escarpia sp.), mussels (Bathymodiolus sp.), shrimp (Alvinocaris sp.), and lobsters (Munidopsis sp.) (Duenas et al., 2021). These communities exhibit similarities with the structures of chemosynthetic communities in the Accretionary Prism of Barbados, Trinidad and Tobago, and the GoM (Olu et al., 1996; Cordes et al., 2007; Carey et al., 2014; Amon et al., 2017, 1997; Duenas et al., 2021). Besides, in the Gran Fuerte area of the southern Colombian Caribbean, spanning approximately 14,000 km2 and designated as an Important Area for Biodiversity, Digby et al. (2016) employed a methodology to identify cold seeps and the structured benthic communities associated with them. Their study confirmed the presence of soft substrates and chemosynthetic communities, characterized by the existence of shells from Bathymodiolus spp. and Calyptogyna spp., exclusively associated with cold seep sites. These findings suggest that depth may be the primary factor explaining the community structures of seeps in the Atlantic Equatorial Belt, as previously mentioned (Cordes et al., 2007; Olu et al., 2010). Another alternative hypothesis is proposed by Levin et al. (2012), suggesting that the chemosynthetic communities in the southwestern Caribbean might share a closer affinity with those on the Pacific margin of Costa Rica. This could be due to a historical connection that existed before the closure of the Isthmus of Panama (Gracia et al., 2012).
On the other hand, the geomorphological conditions of this area make it possible to find well-developed sedimentary basins, which contribute to the formation of mud diapirs that, in turn, create optimal conditions for the occurrence of seeps. Methane-rich fluids, emerging from overpressured regions, migrate outward and support the chemosynthetic communities that inhabit these areas (Tunnicliffe et al., 2003; Gracia et al., 2012). However, in Colombia there is not enough research on cold seepage, and many of the characteristics of these reducing systems remain unknown (Gracia et al., 2012; Duenas et al., 2021) (See Figure 1: Study sites 36 to 40) (Table 6).
3.2.2 Macrofauna
Demopoulos et al. (2018) propose that the composition of communities in the northern GoM is influenced by several key factors, including their proximity to seep sites, the slope of the terrain, the geochemistry of the seep environments, and the availability of food resources. Their research indicates that most examined taxa were found within a distance of 16 meters from seep habitats, and these findings align with the idea that these communities heavily rely on organic matter originating from seeps. This supports the notion that the influence of seep ecosystems extends spatially over a considerable area (Demopoulos et al., 2018; Toone and Washburn, 2020). However, Levin and Mendoza (2007) assert that the results of their research support the idea that common properties among these types of communities are more likely to be found between habitats (microbial mats, clam beds, pogonophoran fields), regardless of location or water depth.
Studies on the structure and abundance of macrofaunal communities show that many documented taxa have been observed at different seep sites. Families of polychaetes such as Dorvilleidae, Paraonidae, Syllidae, Cirratulidae, and Ampharetidae, as well as peracarid groups like amphipods, cumaceans, and tanaidaceans, are some examples of groups shared across these investigations (Robinson et al., 2004; Levin and Mendoza, 2007; Demopoulos et al., 2018; Washburn et al., 2018)1. Nevertheless, it should be noted that the level of identification has varied between studies, making it unsurprising to find similarities at this level of taxonomic resolution. Further identification is necessary to improve diversity comparisons among these seep habitats.
Another argument reveals that many macroinfaunal taxa identified at seep sites were also present in background sediments (Washburn et al., 2018). This is because seep communities are generally composed of a subset of background taxa that are tolerant to high concentrations of hydrogen sulfide (Bernardino et al., 2010). For reasons such as this, it has been thought that seeps are extremely heterogeneous environments, representing broad ranges of depth, chemical composition, fluid flow, geomorphology, age, and benthic fauna communities (Levin, 2005; Levin and Mendoza, 2007; Washburn et al., 2018). Additionally, many researchers agree on the need for greater efforts to collect these types of organisms (Graham, 1999; Levin and Mendoza, 2007; Toone and Washburn, 2020), as well as taxonomic work, to determine the amount of endemism present at seep sites (Levin and Mendoza, 2007; Cordes et al., 2009) (Table 7).
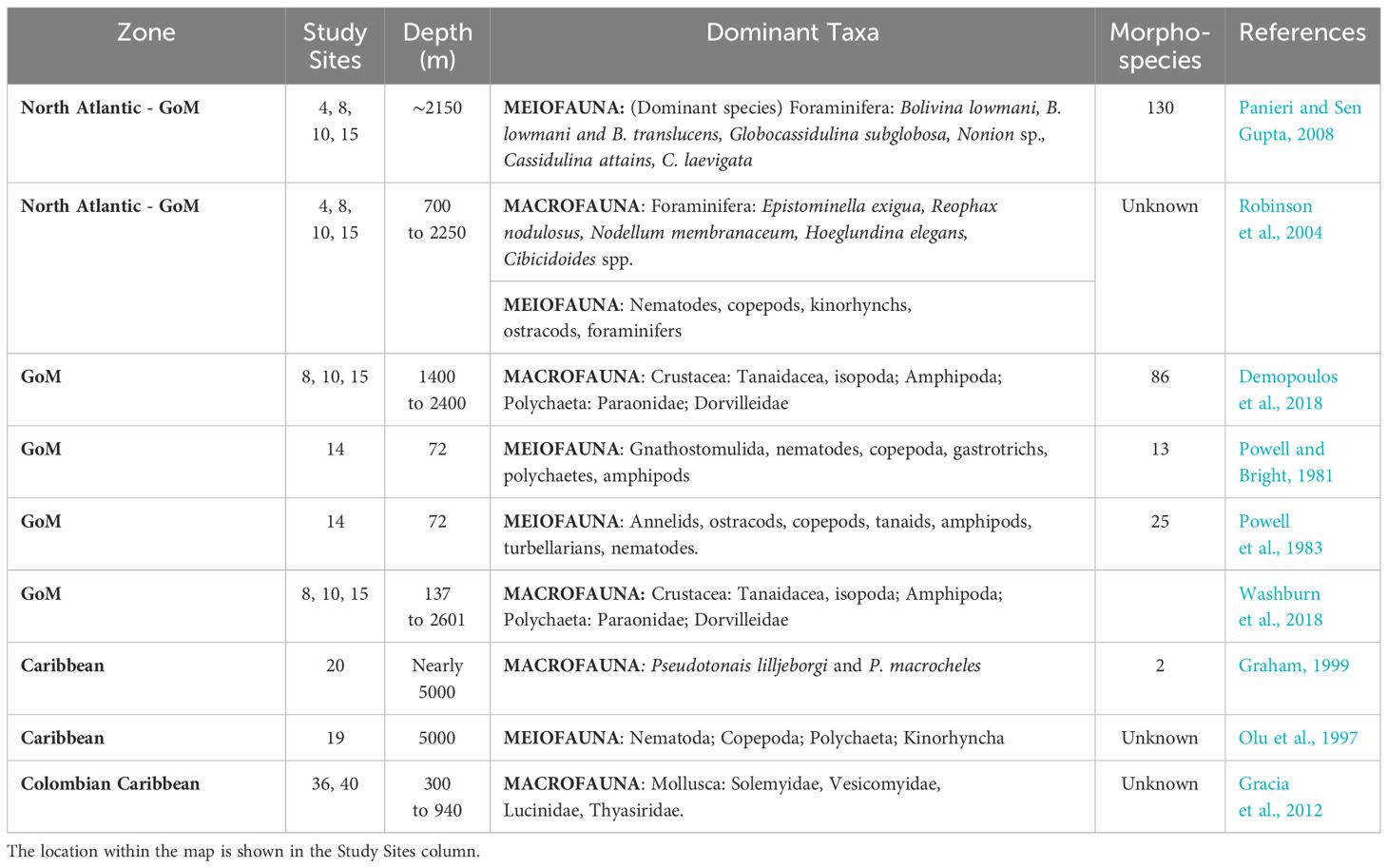
Table 7 Macrofauna and Meiofauna information including dominant taxa and morphospecies in the studied areas.
Our understanding of the relationship between macrofauna and seeps or other reducing ecosystems is limited globally, primarily because there have been relatively few comprehensive investigations in this type of research (Levin, 2005; Levin et al., 2016). Several hypotheses have been proposed to explain the presence of macrofauna in reducing ecosystems: I) Habitat Heterogeneity and Geochemistry: One premise suggests that the community structure of macrofauna is influenced by the heterogeneity of the habitat and the geochemistry of the sediments (Cordes et al., 2010; Demopoulos et al., 2018; Pereira et al., 2021). In this view, the diversity and abundance of macrofauna are shaped by the specific characteristics of the environment. II) Seep Fluid Flow: Another premise posits that the diversity and abundance of macrofauna depend on seep fluid flow rates. It suggests that these factors peak at intermediate fluid flow values and decline when the toxicity reduction from fluid flow outweighs the benefits of organic enrichment (Olu et al., 1997; Levin et al., 2003; Bernardino et al., 2010). In other words, an optimal range of fluid flow may be necessary for thriving macrofauna communities. Tryon et al. (2002) documented initial measurements of fluid flows in various locations, including the Alaska margin, the Oregon margin, Peru, and the Bush Hill seeps in the northern Gulf of Mexico. However, subsequent research suggests that these values may have been overestimated (Luff and Wallmann, 2003). This ratifies what Levin (2005) expressed that, to obtain answers to these questions, researchers must carry out coordinated in situ biological, geochemical, microbiological, and hydrogeological measurements over long periods. III) Variability in Density Comparisons: Levin (2005) noted that there is variability in the comparison of macrofauna density associated with seeps and those in non-seep environments. This suggests that the relationship between macrofauna and geochemistry is complex and may not have a straightforward correlation. Currently, there is a transition zone that is between seeps and non-seeps sites. These places may have greater local diversity by combining taxa from reducing habitats and those that are tolerant to the stressors that occur there (Cordes et al., 2009; Levin et al., 2015). However, the spatial and temporal scales at which these differences become evident, or whether proximity to other hard soil types affects the importance of filtered carbonates as hard substrate habitat, remain to be determined (Levin et al., 2016).
Contrary to the notion that all macrofauna in reducing ecosystems are highly tolerant of sulfide concentrations, some studies have revealed that many macrofauna taxa tend to avoid areas with significant sulfide concentrations. Instead, they occupy sediment layers that are relatively free of this compound (Levin et al., 2003). Furthermore, when comparing the densities of deep-sea fauna at depths greater than 3,000 meters with the macrofauna associated with hydrothermal vents, the latter often exhibits higher densities. This difference in density could be attributed to the scarcity of food at greater depths, making methane a valuable additional source of carbon for organisms in hydrothermal vent communities (Levin and Michener, 2002; Demopoulos et al., 2018).
The smaller size of certain taxa within macrofauna allows them to engage in vertical migration between different sources of oxygen, sulfide, and nitrate within sediment layers, enabling them to store one or the other as needed (Levin, 2005). This vertical movement is a crucial adaptation that helps these organisms cope with the varying conditions present in sediments of reducing ecosystems. Additionally, the presence of microbial mats can have a significant impact on the geochemistry and sediment substrate within these environments, influencing associated biota (Robinson et al., 2004). These mats can limit the availability of oxygen and create highly sulfidic conditions in seep sediments, which in turn affect food supply and other ecological factors for infaunal invertebrates (Levin, 2005) (Table 7).
3.2.3 Meiofauna
Research on meiofauna in cold seep environments remains limited, with most studies focusing on the geographical regions and depth distribution of these groups. These investigations often provide global measurements of abundance, biomass, or major taxa, but they rarely delve into patterns of species composition or diversity (Levin, 2005; Levin et al., 2016).
In the Blake Ridge area, communities of foraminifera associated with bacterial mats and mussel beds have been documented (Robinson et al., 2004; Panieri and Sen Gupta, 2008; Panieri et al., 2014). Panieri et al. (2014) indicate that the community composition of foraminifera belonging to the meiofauna is affected by methane emissions, as evidenced by the scarcity of agglutinated foraminifera at seep sites. This is possibly due to oxygen depletion caused by the presence of methane or sulfide in pore and bottom water. Generally, these agglutinated species are less tolerant to dysoxia/microxia than calcareous species (Levin, 2003). However, Panieri et al. (2014) emphasize that no segregation of foraminiferal species by the geochemistry of seep samples can be inferred, and the data obtained cannot demonstrate the possible effect of variable sulfide or methane fluxes on the community.
In this site, there was also an attempt to record differences between the densities of foraminifera associated with bacterial mats and mussel beds. It was found that the difference in association between substrates is minimal and inconsistent, as the same two species (Bolivina lowmani and Globocassidulina subglobosa) dominate these assemblages (Panieri and Sen Gupta, 2008; Panieri et al., 2014). On the other hand, Robinson et al. (2004) reported that foraminifera contributed surprisingly little to the overall seep fauna, possibly because the data were derived from a limited number of samples. However, the rest of the meiofauna reveals significant biotic heterogeneity in seep sediments, both between oceanic regions and among different seep habitats (See Figure 1: Studied sites 1 to 5).
Olu et al. (1997) made a discovery by documenting the existence of meiofaunal communities in the cold-seeping mud volcanoes of the Barbados Accretionary Prism. These mud volcanoes were found to harbor extensive fields of polychaetes and exhibited exceptionally high densities of meiofauna. The most prevalent taxa identified in this unique environment included Nematoda, Copepods, Polychaetes, and Kinorhyncha. While the study did not directly attribute the presence of polychaetes and other taxa to fluid expulsion patterns, it suggested that these organisms might be indirectly linked to increased biological production, potentially driven by bacterial activity. This hypothesis was based on the observation that the area featured gas hydrates containing substantial amounts of methane. It was theorized that these mud volcanoes could release methane from these hydrates, thereby supporting widespread biological activity in the surrounding environment. This discovery shed light on the remarkable adaptability of meiofaunal communities to thrive in extreme and dynamic deep-sea environments, such as cold-seeping mud volcanoes and hinted at the complex interplay of biological and geological factors in shaping these ecosystems (Brown and Westbrook, 1988) (See Figure 1: Studied sites 18 to 35).
In the same area, nematode density values were exceptionally high and were recorded in I) similar depths in normal deep-sea environments, II) at hydrothermal vents; and III) even higher than at shallower depths. However, nematodes are not always the drivers of density patterns. For instance, in the GoM at shallow brine seep (72 m, East Flower Garden Bank), the meiofauna was dominated by gnathostomulids, platyhelminths, aschelminths, nematodes, and amphipods (Powell and Bright, 1981; Powell et al., 1983), while in Blake Ridge – GoM, harpacticoid copepods were surprisingly well represented (Robinson et al., 2004). The Gnathostomulida was also the phylum found with a distribution center located within the sulfide system (Powell and Bright, 1981). Finally, at the Barbados Accretionary Prism, the large expanse of polychaete fields was the representative fauna (Olu et al., 1997) (Table 7).
Finally, it is possible that density of meiofauna is influenced by various factors, as noted by Gracia Clavijo (2018), including depth, habitat type, calcium carbonate content, organic matter, and food availability, among others. Giere (2009) also suggests that the relationship between abundance and biomass may be depth-dependent. However, it remains unclear which of these factors primarily controls the distribution of meio-epifauna in cold seep environments (Gracia Clavijo, 2018).
3.2.4 Fungi
Endemic deep-sea fungi represent a relatively unexplored and enigmatic aspect of the deep-sea biosphere, and their role in benthic ecosystems remains poorly understood (Thaler et al., 2012; Levin et al., 2016). Nevertheless, advances in molecular techniques have paved the way for the discovery of numerous novel deep-sea fungal groups, particularly within habitats like methane seeps and hydrothermal vents (Thaler et al., 2012).
Zande (1999) introduced the world to a commensal fungus belonging to the Ascomycetes class. This unique fungus was found within the gills of Bathynerita naticoidea, a prominent gastropod species inhabiting oil seep communities along the upper continental slope of the GoM. While the precise nature of the association between this fungus and the gastropod remains somewhat enigmatic, researchers speculate that it may serve as a detoxification agent within this specialized ecosystem. The hypothesis proposes that this fungus could play a crucial role in mitigating the harmful effects of hydrocarbons and sulfur compounds present in the seep environment. It is believed to absorb these compounds from the surrounding water, subsequently oxidizing them into alcohols, aldehydes, and fatty acids. These transformed compounds are then thought to be incorporated into the cellular lipids of the gastropod, potentially assisting in the detoxification and utilization of otherwise toxic substances.
The presence of Ascomycota in oxygen-poor environments suggests its ability to adapt to these conditions, possibly functioning as a facultative anaerobe or utilizing other organisms that thrive in such environments. However, the precise ecological role of this fungal group remains elusive despite its widespread distribution and frequent occurrence in oxygen-poor sediments (Thaler et al., 2012). This observation aligns with the findings of Nagano et al. (2010), who emphasize the existence of a globally distributed, deep-sea endemic fungal group within the Ascomycota phylum (Table 8).
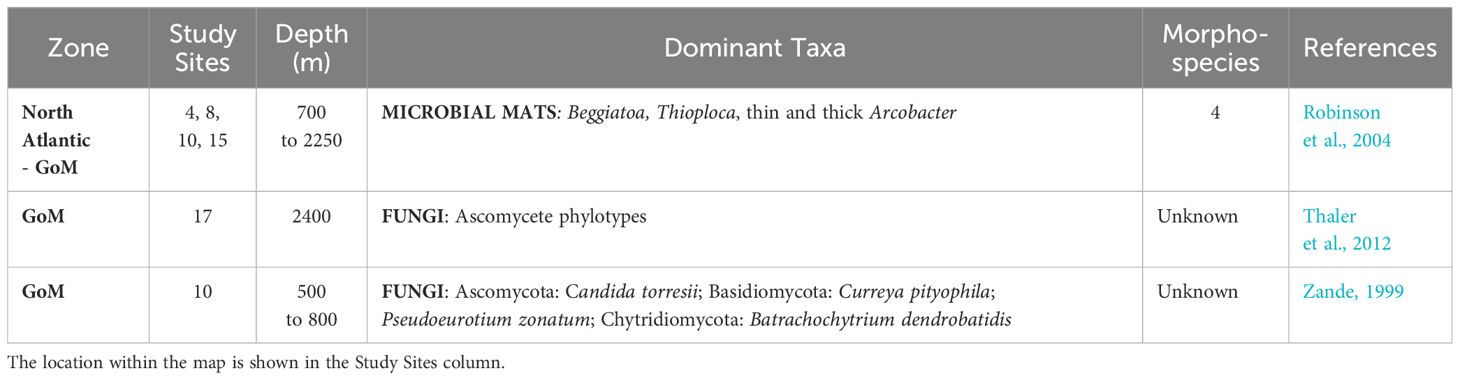
Table 8 Fungal and bacterial information including dominant taxa and morphospecies in the studied areas.
3.2.5 Microbial mats
Cold seeps are dynamic environments where the presence of methane fosters the development of dense microbial communities characterized by high metabolic rates (Levin, 2005). These ecosystems thrive due to several key factors, including an abundant food supply, the availability of substrates, and high concentrations of methane and sulfur. Within these microbial mats, a diverse array of microbial taxa coexists, with a dominant biomass comprised of filamentous sulfur-oxidizing bacteria such as Beggiatoa, Thioploca, Arcobacter, and Thiothrix (Levin, 2005). These bacteria play a crucial role in the seep ecosystem by utilizing the available sulfides. Despite the harsh geochemical conditions, particularly the elevated sulfide concentrations, cold seeps support a surprisingly diverse faunal community (Levin et al., 2003; Robinson et al., 2004; Gill et al., 2005; Levin, 2005; Panieri et al., 2014).
Robinson et al. (2004) provided the first direct comparison of seepage fauna in different types of microbial mat systems for the GoM and the Western Atlantic region (Blake Ridge). This study showed that the GoM supports higher densities and diversity in most taxa than the hydrocarbon seepage infauna of the Blake Ridge microbial mats. This could reveal significant biotic heterogeneity in seep sediments both between oceanic regions, and between different seep habitats. These differences are probably reflected in the concentrations of some compounds that favor faunal distribution and diversity patterns in hydrocarbon seeps. However, both thick and thin Arcobacter mats at Blake Ridge appear to represent the most hostile environment for all taxa. These differences in densities may also be due to the presence of oil in the area, which is common in these seeps (Robinson et al., 2004). These sediments were modified during the Pleistocene, when there were large accumulations of oil and gas that began to impact the seabed sediments Venting is thus suppressed over time, favoring slow development of complex communities that depend on abundant hydrocarbons in sediments (Sassen et al., 1994; Sun et al., 2020) (Table 8).
4 Knowledge gaps and areas for further research
This review identified some knowledge gaps that require further scientific investigation.
1. In recent years an increasing number of modern oil seeps have been identified, such as offshore southern California or the Black Sea (Sun et al., 2020). These areas are characterized by the presence of oil in the seepage fluids, contributing not only a major source of oil to the ocean but also releasing vast amounts of methane. Oil seepage accompanied by methane increases the flow of methane into the ocean and even into the atmosphere, thanks to the protection of the surface coatings of the gas bubbles (Valentine et al., 2010). However, distinguishing oil from methane seep deposits is difficult in cases where δ13Ccarb values are greater than about −30‰, due to the mixing of different carbon sources (Sun et al., 2020). These types of seeps exert significant control over the composition of the fauna that inhabits the seeps (Sun et al., 2020) since oil and accessory methane escaping from the seabed, facilitate the growth of bacterioplankton in the seawater bottom of the seeps, which is believed to provide nutrition for some organisms that inhabit these seeps (Kiel and Peckmann, 2019). Consequently, the need for unambiguous identification of this type of seep grows, to understand the adaptation of life based on chemosynthesis (Sun et al., 2020) and to better understand the impact of oil seepages, along geological time, in the carbon cycle, such as in I) microbial processes, microorganisms in seep environments play a crucial role in metabolizing hydrocarbons through processes like biodegradation; II) sedimentary carbon storage, oil seepage can also contribute to the burial of organic carbon in sediments; III) Feedback effects, changes in environmental conditions resulting from oil seepages, such as alterations in sediment composition, oxygen levels, and nutrient availability, can influence the activity of marine organisms and communities microbial; among others (Levin and Sibuet, 2012).
2. As previously mentioned, some researchers have emphasized that small communities, including bacteria and fungi, have often been overlooked in studies of cold seeps and deep-sea hydrothermal vents (Graham, 1999; Levin, 2005; Amon et al., 2017). One possible reason for this oversight could be the challenges associated with sampling at great depths using traditional samplers, without forgetting the high costs. Consequently, there is a pressing need to enhance research methods and sampling techniques that enable a more comprehensive exploration of the relationships between these small communities (e.g. bacteria and funguses) and methane formation in these unique ecosystems. This approach can help fill critical knowledge gaps and provide a deeper understanding of the intricate dynamics within these environments.
3. Some researchers suggest that conducting additional molecular studies within restricted chemoautotrophic ecosystems could unveil a previously undiscovered reservoir of fungal biodiversity (Nagano et al., 2010; Thaler et al., 2012); exploring marine bacterial diversity in the southern Colombian Caribbean Sea (Franco et al., 2020). Furthermore, further investigations into the faunal associations surrounding fungal-reducing environments, which have yet to be explored, may offer insights into the geographic extent of these associations, the nature of these relationships, and whether such associations are unique to chemical environments like seeps and vents (Van Dover and Lichtwardt, 1986; Zande, 1999). These studies have the potential to enhance our understanding of the intricate interplay between fungi, fauna, and the chemical processes in these specialized ecosystems.
5 Conclusions and finals remarks
The review highlights several key similarities among cold seep sites in the western Atlantic. One of the prominent commonalities is their geological setting, characterized by the influence of diapirism. This geological phenomenon is observed in various locations, including the Black Ridge, Northern Gulf of Mexico (GoM), Barbados Accretionary Prism, and the Colombia Basin (Brown and Westbrook, 1988; Jollivet et al., 1990; Paull et al., 1995; Olu et al., 1996, 1997; Cordes et al., 2009; Gracia et al., 2012; Cortes et al., 2018; Toone and Washburn, 2020). Diapirism plays a significant role in shaping these seep environments, as it contributes to the formation of faults and facilitates the mobilization of fluids such as methane and sulfide (Paull et al., 1995; Olu et al., 1996; Van Dover et al., 2003; Cordes et al., 2009; Gracia et al., 2012; Brothers et al., 2013; Carvajal-Arenas et al., 2020; Toone and Washburn, 2020).
The analysis of existing studies reveals several notable trends and knowledge gaps in the field of cold seep environments in the western Atlantic. Here are some key observations: I) Geographic Concentration of Studies: The majority of research on cold seep environments appears to be concentrated in two main regions: the northern GoM (Brooks et al., 1987; Robinson et al., 2004; Sun et al., 2020), and the Antilles area (Olu et al., 1996; Carey et al., 2014; Amon et al., 2017). These areas have been the primary focus of investigations, leading to a relatively robust understanding of seep ecosystems in these regions. II) Knowledge Gap in the Caribbean-Colombian Region: A significant knowledge gap is evident in the area between Colombia and the northern Gulf of Mexico. This gap suggests that limited research has been conducted in this specific region, leaving much to be explored and understood about cold seep ecosystems along this stretch of the western Atlantic (Figure 1). III) Incomplete Understanding of Fluid Dynamics and Geological Features: There is a significant knowledge gap regarding the origin and pathways of seep fluids, as well as the geological structures and processes shaping cold seep environments.
In the northern Gulf of Mexico, there is a distinctive influence of salt structures that are both unique to this region and have been exclusively documented here (Brooks et al., 1987; Cordes et al., 2009; Sun et al., 2020). These salt structures play a pivotal role in creating migration pathways for fluids (Soto et al., 2004; Cordes et al., 2009), facilitating their movement not only towards reservoir rocks but also towards the seafloor surface (Kennicutt, 2017). These geological features are closely associated with the presence of brine seeps and brine pools, which are additional geomorphological phenomena largely attributed to the processes of salt tectonics (Powell et al., 1983; Sassen et al., 1994; Cordes et al., 2009).
In this specific region of the Caribbean, there is another unique geological environment near the Kick’em Jenny underwater volcano. Seep occurrences in this area are a consequence of fluid overpressure that developed during the collapse of this ancient volcano. This event led to the burial and deformation of hemipelagic sediments, triggering debris avalanches and subsequent fluid outflow (Brown and Westbrook, 1988; Carey et al., 2014, 2015).
This review has highlighted the presence of various geological features in specific locations. For instance, it has discussed the occurrence of gas hydrates, particularly methane hydrates, which have been documented in research conducted on the Blake Ridge (Paull et al., 1995; Van Dover et al., 2003), the northern of the GoM (Cordes et al., 2009), and the Antilles (Olu et al., 1997; Amon et al., 2017). Interestingly, these formations are not commonly observed on the seafloor, even under conditions of stable pressure and temperature conducive to methane hydrate formation (Paull et al., 1995).
Mud volcanoes, while documented in research conducted in the Antilles (Brown and Westbrook, 1988; Olu et al., 1996; Toone and Washburn, 2020), have also been observed in Panama, albeit not directly associated with seeps (Reed et al., 1990). Moreover, there is a significant presence of mud volcanoes along the Colombian Caribbean coast (Díaz et al., 1996; Di Luccio et al., 2021; Lopez Ramos et al., 2022). This indicates that despite some geological studies in the area, there is still much to be understood regarding their potential relationships with cold seeps in this region.
Another noteworthy example is the presence of pockmarks, which have been reported only on the Blake Ridge (Paull et al., 1995; Brothers et al., 2013). However, these geological features are among the most common in similar environments worldwide. Pockmarks have been documented in various regions such as the Scottish Shelf (King and MacLean, 1970), Barents Sea (Solheim and Elverhøi, 1985), Canadian continental shelf (Fader, 1991), the equatorial West African region (Pilcher and Argent, 2007), the Congo Basin (Andresen and Huuse, 2011) Sinú fold belt (Osorio-Granada et al., 2023)1, and many others. The scarcity of information regarding pockmarks in this specific region suggests that there may be more undocumented occurrences in this part of the world.
It is remarkable that most studies on fauna associated with cold seeps primarily focus on megafauna (Paull et al., 1995; Cordes et al., 2009; Gracia et al., 2012; Carey et al., 2014; Duenas et al., 2021). The predominant organisms in these communities and recorded in these studies are mollusks (Lucinidae, Mytilidae, Vesicomyidae, Calyptogena), tubeworms (Siboglinidae), and squat lobsters (Galatheidae) (Turnipseed et al., 2004; Cordes et al., 2009; Carey et al., 2014; Duenas et al., 2021).
Throughout the area of interest, communities of mussels and vesicomyid clams are reported. Nevertheless, their presence is correlated with different types of environments: I) in Blake Ridge and the Florida Escarpment (Northwest Atlantic), they are associated with pockmarks (Sibuet and Olu, 1998; Wagner et al., 2013); II) in the Gulf of Mexico, they are found in areas defined by patterns of methane-saturated brine flow at the sediment/seawater interface (MacDonald et al., 1990; MacDonald, 1998; Cordes et al., 2007, 2009); III) in the Barbados accretionary prism, they are associated with various geological structures, including mud volcanoes, diatremes, diapirs, and intermediate structures within the prism (Olu et al., 1996; Gill et al., 2005); and IV) in the Sinú fold belt, they are associated with mud diapirs that allow the release of methane-rich fluids (Gracia et al., 2012; Duenas et al., 2021). However, in the same Sinú fold belt, but in areas of shallow water seepages (47 to 166m), no such organisms were found)1.
The association of megafauna with geomorphological features does not show a marked trend. As an example, in the northwestern Atlantic, there is evidence that these communities are associated with pockmarks (Paull et al., 1995; Van Dover et al., 2003; Brothers et al., 2013). However, in the Sinú Fold Belt, where extensive areas with pockmarks are recorded (Osorio-Granada et al., 2023)1, this fauna is associated with mud diapirs (Gracia et al., 2012), and note that these exhibit similarities with the structures of the chemosynthetic communities of the Barbados Accretionary Prism, Trinidad and Tobago, and the Gulf of Mexico (Gracia et al., 2012; Duenas et al., 2021). The origin of the fluid also does not show a clear association trend. Few studies record this information. Nevertheless, in the northwestern Atlantic, the documented fluid origin is biogenic (Paull et al., 1995; Van Dover et al., 2003), while in the Sinú Fold Belt, it is both biogenic and thermogenic (Osorio-Granada et al., 2023)1.
Macrofaunal studies have provided different theories about the factors influencing their association with seeps (proximity to the seep, terrain slope, geochemistry of the seep environments, and availability of food resources) (Demopoulos et al., 2018). Another theory is that the habitat where these communities are found (microbial mats, clam beds, pogonophoran fields) is likely the common factor, regardless of the proximity to the seep or depth (Levin and Mendoza, 2007). Additionally, it was impossible to observe any association trend regarding geomorphological features or the type and origin of the fluid, as there are not many studies and those did not document this type of information about the study area.
To compare data between the GoM and the Colombian Caribbean regarding the type and origin of fluid and geomorphological features associated with macrofauna, another study in the same area of the GoM, that recorded this information, was reviewed. The GoM study shows the presence of mud volcanoes, brine pools, salt diapirs, and thermogenic fluid origin (Sassen et al., 1994), while the Sinú Fold Belt study shows the presence of pockmarks, mud diapirs, and biogenic and thermogenic fluid origins1. Thus, showing that there appears to be no similarity or trend between the areas.
All studies on the structure and composition of macrofauna examined for this review, indicate that in waters deeper than 700 m, they share similar families: polychaetes such as Dorvilleidae, Paraonidae, Syllidae, Cirratulidae, and Ampharetidae, as well as peracarid groups like amphipods, cumaceans, and tanaidaceans, among others (Robinson et al., 2004; Levin and Mendoza, 2007; Demopoulos et al., 2018). Moreover, these same family similarities are found in studies in waters less than 200 m deep (Washburn et al., 2018)1. However, the levels of taxonomic identification among the studies vary and are not very specialized, so it is not uncommon to find similarities at the order or family level. This highlights the need to reach a taxonomic category such as genus or species to improve diversity comparisons among these seep habitats and to determine the amount of endemism present at the seep sites (Levin and Mendoza, 2007; Cordes et al., 2009).
Exceptionally high densities of meiofauna, primarily composed of extensive fields of polychaetes, nematodes, copepods, and kinorhynchs, were recorded in the cold-seeping mud volcanoes of the Barbados Accretionary Prism. However, the presence of this community could not be attributed to fluid expulsion patterns but rather to increased biological production, potentially driven by bacterial activity. Based on the hypothesis that mud volcanoes could release methane from gas hydrates, thus supporting biological activity, this reaffirms the remarkable adaptability of meiofaunal communities to thrive in extreme environments such as cold-seeping mud volcanoes (Brown and Westbrook, 1988; Olu et al., 1997).
The findings highlighted significant heterogeneity in cold seeps, where clearly distinguishable patterns could not be identified. Following a comprehensive analysis, no evidence was found for trends in the distribution of accompanying fauna concerning the type and origin of fluid, structural geology, depth, or geographic location.
More than 40% of investigations in these environments were conducted using remotely operated vehicles (ROVs) or Human Occupied Vehicles (HOVs), potentially leading to an underestimation of smaller fauna (Graham, 1999; Levin, 2005). This has led researchers to highlight the relative oversight of small faunal groups and the many unanswered questions surrounding seepage and reduction ecosystems (Graham, 1999; Levin, 2005; Nagano et al., 2010; Levin et al., 2016; Toone and Washburn, 2020).
This comprehensive review underscores the necessity for continued research on cold seeps, as fundamental questions such as the triggers for seep activation or deactivation and the origins and escape structures of seep fluids remain unresolved. Additionally, it highlights the geographical gaps in our understanding of seep environments across the Atlantic Ocean. Further investigations into these enigmatic ecosystems are crucial for advancing our knowledge of their dynamics and ecological significance.
Finally, despite the extensive review of studies conducted in the region of interest, which also builds upon earlier reviews (e.g. Levin, 2005), it is apparent that even with technological advancements in recent years, many of the fundamental questions raised about these environments remain unanswered (Cordes et al., 2016; Kiel, 2016; Levin et al., 2016; Toone and Washburn, 2020; Duenas et al., 2021). This underscores the pressing need for continued research in these unique and complex ecosystems.
Author contributions
MP: Writing – original draft, Writing – review & editing. PR: Writing – original draft, Writing – review & editing. AM: Conceptualization, Data curation, Formal analysis, Funding acquisition, Investigation, Methodology, Project administration, Resources, Software, Supervision, Validation, Visualization, Writing – review & editing.
Funding
The author(s) declare financial support was received for the research, authorship, and/or publication of this article. Agencia Nacional de Hidrocarburos - Colombia (ANH) y Ministerio de Ciencia Tecnología e Innovación - Colombia (MinCiencias). Project Methane seep hunting: A multi-scale and multi method approach 785/668–2019.
Conflict of interest
The authors declare that the research was conducted in the absence of any commercial or financial relationships that could be construed as a potential conflict of interest.
Publisher’s note
All claims expressed in this article are solely those of the authors and do not necessarily represent those of their affiliated organizations, or those of the publisher, the editors and the reviewers. Any product that may be evaluated in this article, or claim that may be made by its manufacturer, is not guaranteed or endorsed by the publisher.
Supplementary material
The Supplementary Material for this article can be found online at: https://www.frontiersin.org/articles/10.3389/fmars.2024.1430377/full#supplementary-material
Footnotes
- ^ Aguilar-Pérez, M. I., Zapata-Ramírez, P. A. and Micallef, A. "Macrofauna of soft sediments associated with methane seeps from the offshore Sinú Basin, Colombian Caribbean" (in preparation)
References
Amon D. J., Gobin J., Van Dover C. L., Levin L. A., Marsh L., Raineault N. A. (2017). Characterization of methane-seep communities in a deep-sea area designated for oil and natural gas exploitation off Trinidad and Tobago. Front. Mar. Sci. 4, 342. doi: 10.3389/fmars.2017.00342
Andresen K. J., Huuse M. (2011). ‘Bulls-eye’pockmarks and polygonal faulting in the Lower Congo Basin: Relative timing and implications for fluid expulsion during shallow burial. Mar. Geol. 279, 111–127. doi: 10.1016/j.margeo.2010.10.016
Bernardino A. F., Smith C. R., Baco A., Altamira I., Sumida P. Y. (2010). Macrofaunal succession in sediments around kelp and wood falls in the deep NE Pacific and community overlap with other reducing habitats. Deep Sea Res. Part I: Oceanogr. Res. Papers 57, 708–723. doi: 10.1016/j.dsr.2010.03.004
Black M. B., Halanych K. M., Maas P. A. Y., Hoeh W. R., Hashimoto J., Desbruye´res D., et al. (1997). Molecular systematics of vestimentiferan tubeworms from hydrothermal vents and cold-water seeps. Mar. Biol. 130, 141–149. doi: 10.1007/s002270050233
Boland G. S., Rowe G. T. (1991). Deep-sea benthic sampling with the GOMEX box corer. Limnol. Oceanogr. 36 (5), 1015–1020.
Bowden D. A., Rowden A. A., Thurber A. R., Baco A. R., Levin L. A., Smith C. R. (2013). Cold seep epifaunal communities on the Hikurangi Margin, New Zealand: Composition, succession, and vulnerability to human activities. PloS One 8, e76869. doi: 10.1371/journal.pone.0076869
Brooks J. M., Kennicutt M. C., Fisher C. R., Macko S. A., Cole K., Childress J. J., et al. (1987). Deep-sea hydrocarbon seep communities: evidence for energy and nutritional carbon sources. Science 238, 1138–1142. doi: 10.1126/science.238.4830.1138
Brothers L. L., Van Dover C. L., German C. R., Kaiser C. L., Yoerger D. R., Ruppel C. D., et al. (2013). Evidence for extensive methane venting on the southeastern US Atlantic margin. Geology 41, 807–810. doi: 10.1130/G34217.1
Brown K., Westbrook G. K. (1988). Mud diapirism and subcretion in the Barbados ridge accretionary complex: the role of fluids in accretionary processes. Tectonics 7, 613640. doi: 10.1029/TC007i003p00613
Campbell K. A. (2006). Hydrocarbon seep and hydrothermal vent paleoenvironments and paleontology: past developments and future research directions. Paleogeogr. Palaeoclimatol. Palaeoecol. 232, 362–407. doi: 10.1016/j.palaeo.2005.06.018
Carey S., Ballard R., Bell K. L., Bell R. J., Connally P., Dondin F., et al. (2014). Cold seeps associated with a submarine debris avalanche deposit at Kick’em Jenny volcano, Grenada (Lesser Antilles). Deep Sea Res. Part I: Oceanogr. Res. Papers 93, 156–160. doi: 10.1016/j.dsr.2014.08.002
Carey S., Bell K. L., Roman C., Dondin F., Robertson R., Gobin J., et al. (2015). Exploring Kick’em Jenny Submarine Volcano and the Barbados Cold Seep Province, Southern Lesser Antilles [in special issue: new Frontiers in Ocean Exploration: The E/V Nautilus 2014 Gulf of Mexico and Caribbean Field Season]. Oceanography 28, 38–39. doi: 10.5670/
Carvajal-Arenas L. C., Torrado L., Mann P., English J. (2020). Basin modeling of Late Cretaceous/Mio-Pliocene (.) petroleum system of the deep-water eastern Colombian Basin and South Caribbean Deformed Belt. Mar. Petroleum Geol. 121, 104511. doi: 10.1016/j.marpetgeo.2020.104511
Cordes E. E., Bergquist D. C., Fisher C. R. (2009). Macro-ecology of Gulf of Mexico cold seeps. Annu. Rev. Mar. Sci. 1, 143–168. doi: 10.1146/annurev.marine.010908.163912
Cordes E. E., Carney S. L., Hourdez S., Carney R. S., Brooks J. M., Fisher C. R. (2007). Cold seeps of the deep Gulf of Mexico: community structure and biogeographic comparisons to Atlantic equatorial belt seep communities. Deep Sea Res. Part I: Oceanogr. Res. Papers 54, 637–653. doi: 10.1016/j.dsr.2007.01.001
Cordes E. E., Cunha M. R., Galéron J., Mora C., Olu-Le Roy K., Sibuet M., et al. (2010). The influence of geological, geochemical, and biogenic habitat heterogeneity on seep biodiversity. Mar. Ecol. 31, 51–65. doi: 10.1111/j.1439-0485.2009.00334.x
Cordes E. E., Jones D. O., Schlacher T. A., Amon D. J., Bernardino A. F., Brooke S., et al. (2016). Environmental impacts of the deep-water oil and gas industry: a review to guide management strategies. Front. Environ. Sci. 4, 58. doi: 10.3389/fenvs.2016.00058
Cortes J. E., Aguilera R., Wilches O., Osorno J. F., Cortes S. I. (2018). Organic geochemical insights from oil seeps, tars, rocks, and mud volcanoes on the petroleum systems of the Sinú-San Jacinto basin, Northwestern, Colombia. J. South Am. Earth Sci. 86, 318–341. doi: 10.1016/j.jsames.2018.06.007
Craddock C., Hoeh W. R., Gustafson R. G., Lutz R. A., Hashimoto J., Vrijenhoek R. J. (1995). Evolutionary relationships among deep-sea mytilids (Bivalvia, Mytilidae) from hydrothermal vents and cold-water methane sulfide seeps. Mar. Biol. 121, 477–4485. doi: 10.1007/BF00349456
DeGolyer E. (1932). Oil associated with igneous rocks in Mexico. AAPG Bull. 16, 799–808. doi: 10.1306/3D932AC4-16B1-11D7-8645000102C1865D
Demopoulos A. W., Bourque J. R., Durkin A., Cordes E. E. (2018). The influence of seep habitats on sediment macrofaunal biodiversity and functional traits. Deep Sea Res. Part I: Oceanogr. Res. Papers 142, 77–93. doi: 10.1016/j.dsr.2018.10.004
Díaz J. M., Sánchez J. A., Díaz-Pulido G. (1996). Geomorfología y formaciones arrecifales recientes de Isla Fuerte y Bajo Bushnell, plataforma continental del Caribe Colombiano. Boletín de Investigaciones Marinas y Costeras-Invemar 25 (1), 87–105. doi: 10.25268/bimc.invemar.1996.25.0.372
Digby A., Puentes V., León J. (2016). Cold seeps associated with structured benthic communities: More accurate identification and evaluation using a new multibeam survey methodology in the offshore Southern Colombian Caribbean. Int. J. Geosci. 7, 761–774. doi: 10.4236/ijg.2016.75058
Di Luccio D., Guerra I. M. B., Valero L. E. C., Giraldo D. F. M., Maggi S., Palmisano M. (2021). Physical and geochemical characteristics of land mud volcanoes along Colombia’s Caribbean coast and their societal impacts. Sci. Total Environ. 759, 144225. doi: 10.1016/j.scitotenv.2020.144225
Duenas L. F., Puentes V., León J., Herrera S. (2021). Fauna associated with cold seeps in the deep Colombian Caribbean. Deep Sea Res. Part I: Oceanogr. Res. Papers 173, 103552. doi: 10.1016/j.dsr.2021.103552
Fader G. B. (1991). Gas-related sedimentary features from the eastern Canadian continental shelf. Continental Shelf Res. 11 (8-10), 1123–1153.
Franco N. R., Giraldo M. Á., López-Alvarez D., Gallo-Franco J. J., Dueñas L. F., Puentes V., et al. (2020). Bacterial composition and diversity in deep-sea sediments from the Southern Colombian Caribbean Sea. Diversity 13, 10. doi: 10.3390/d13010010
Gates A. R., Jones D. O. B. (2012). Recovery of benthic megafauna from anthropogenic disturbance at a hydrocarbon drilling well (380 m depth in the Norwegian Sea). PLoS ONE 7 (10), e44114. doi: 10.1371/journal.pone.0044114
Geyer R. A., Giammona C. P. (1980). “Naturally occurring hydrocarbons in the Gulf of Mexico and Caribbean Sea,” in Elsevier Oceanography Series, ed. R. A. Geyer (Elsevier), 37–106. doi: 10.1016/S0422-9894(08)71379-5
Giere O. (2009). Meiobenthology, The microscopic motile fauna of aquatic sediments. Biomedical and Life Sciences. 2nd edition. (Springer-Verlag: Berlin Heidelberg), 527.
Gill F. L., Harding I. C., Little C. T., Todd J. A. (2005). Palaeogene and Neogene cold seep communities in Barbados, Trinidad, and Venezuela: An overview. Palaeogeogr. Palaeoclimatol. Palaeoecol. 227, 191–209. doi: 10.1016/j.palaeo.2005.04.024
Gracia A., Rangel-Buitrago N., Sellanes J. (2012). Methane seep mollusks from the Sinú–San Jacinto fold belt in the Caribbean Sea of Colombia. J. Mar. Biol. Assoc. United Kingdom 92, 1367–1377. doi: 10.1017/S0025315411001421
Gracia Clavijo M. A. (2018). Meio-epifauna of Costa Rican Cold Seeps (Universidad Nacional de Colombia Facultad de Ciencias, Departamento de Biolog a Instituto de Estudios en Ciencias del Mar CECIMAR Santa Marta D.T.C.H., Colombia: Centro de Estudios de Ciencias del Mar).
Graham J. (1999). A new species of Pseudotanais (Crustacea, Tanaidacea) from cold seeps in the deep Caribbean, collected by the French submersible Nautile. Zoosystema 21 (3), 445–451.
Grassle J. P., Sanders R. R., Hessler G. T., Rowe G. T., McLellan T. (1975). Pattern and zonation: a study of the bathyal megafauna using the research submersible Alvin. Deep Sea Res. 22, 457–481. doi: 10.1016/0011-7471(75)90020-0
Guzmán A., y Ardila N. (2004). Estados de los fondos blandos en Colombia. En: Informe del estado de los ambientes marinos y costeros en Colombia: Año 2003 (Santa Marta: INVEMAR), 190 p.
Guzmán-Vega A., Mello M. (1999). Origin of oil in the Sureste Basin, Mexico. Amer. Assoc. Petrol. Geol. 83, 1068–1095.
Hovland M., Judd A. G., Burke R. A. Jr. (1993). The global flux of methane from shallow submarine sediments. Chemosphere 26, 559–578. doi: 10.1016/0045-6535(93)90442-8
Jollivet D., Faugeres J. C., Griboulard R., Desbruyers D., Blanc G. (1990). Composition and spatial organization of a cold seep community on the South Barbados accretionary prism: tectonic, geochemical and sedimentary context. Prog. Oceanogr. 24, 25–45. doi: 10.1016/0079-6611(90)90017-V
Joye S. B. (2020). The geology and biogeochemistry of hydrocarbon seeps. Annu. Rev. Earth Planet. Sci. 48, 205–231. doi: 10.1146/annurev-earth-063016-020052
Kennicutt M. C. (2017). Oil and gas seeps in the Gulf of Mexico. Habitats and biota of the Gulf of Mexico: Before the deepwater horizon oil spill: Volume 1: Water quality, sediments, sediment contaminants, oil and gas seeps, coastal habitats, offshore plankton and benthos, and shellfish. Eds. Herb C. (Houston, TX, USA: Ward Department of Civil and Environmental Engineering Rice University), 275–358.
Kennicutt M. C. II, Burke R. A. Jr. (1995). “Stable isotopes: clues to biological cycling of elements at hydrothermal vents,” in Microbiology of Deep-Sea Hydrothermal Vents. Ed. Karl D. M. (CRC Press, Boca Raton FL), 275–287.
Kiel S. (2016). A biogeographic network reveals evolutionary links between deep-sea hydrothermal vents and methane seep faunas. Proc. R. Soc. B: Biol. Sci. 283, 20162337. doi: 10.1098/rspb.2016.2337
Kiel S., Hansen B. T. (2015). Cenozoic methane-seep faunas of the Caribbean region. PloS One 10, e0140788. doi: 10.1371/journal.pone.0140788
Kiel S., Peckmann J. (2019). Resource partitioning among brachiopods and bivalves at ancient hydrocarbon seeps: A hypothesis. PloS One 14, e0221887. doi: 10.1371/journal.pone.0221887
King L. H., MacLean B. (1970). Pockmarks on the Scotian Shelf (Atlantic Oceanographic Laboratory, Bedford Institute, Dartmouth, Nova Scotia: Geological Society of America Bulletin) 81 (10), 3141–3148. doi: 10.1130/0016-7606(1970)81[3141:POTSS]2.0.CO;2
Levin L. A. (2005). Ecology of cold seep sediments: interactions of fauna with flow, chemistry, and microbes. Oceanogr. Mar. Biol.: Annu. Rev. 43, 1–46.
Levin L. A., Baco A. R., Bowden D. A., Colaco A., Cordes E. E., Cunha M. R., et al. (2016). Hydrothermal vents and methane seeps: rethinking the sphere of influence. Front. Mar. Sci. 3, 195812. doi: 10.3389/fmars.2016.00072
Levin L. A., Mendoza G. F. (2007). Community structure and nutrition of deep methane-seep macrobenthos from the North Pacific (Aleutian) Margin and the Gulf of Mexico (Florida Escarpment). Mar. Ecol. 28, 131–151. doi: 10.1111/j.1439-0485.2006.00131.x
Levin L. A., Mendoza G. F., Grupe B. M., Gonzalez J. P., Jellison B., Rouse G., et al. (2015). Biodiversity on the rocks: macrofauna inhabiting authigenic carbonate at Costa Rica methane seeps. PloS One 10, e0131080. doi: 10.1371/journal.pone.0131080
Levin L. A., Michener R. H. (2002). Isotopic evidence for chemosynthesis-based nutrition of macrobenthos: The lightness of being at Pacific methane seeps. Limnol. Oceanogr. 47, 1336–1345. doi: 10.4319/lo.2002.47.5.1336
Levin L. A., Orphan V. J., Rouse G. W., Rathburn A. E., Ussler W., Cook G. S., et al. (2012). A hydrothermal seep on the Costa Rica margin: middle ground in a continuum of reducing ecosystems. Proc. R. Soc. B 279, 2580–2588. doi: 10.1098/rspb.2012.0205
Levin L. A., Sibuet M. (2012). Understanding continental margin biodiversity: A new imperative. Annu. Rev. Mar. Sci. 4, 79–112. doi: 10.1146/annurev-marine-120709-142714
Levin L. A. (2003). Oxygen minimum zone benthos: adaptation and community response to hypoxia. Oceanography and Marine Biology: an Annual Review. 41, 1–45.
Levin L. A., Ziebis W., Mendoza G. F., Growney V. A., Tryon M. D., Brown K. M., et al. (2003). Spatial heterogeneity of macrofauna at northern California methane seeps: influence of sulfide concentration and fluid flow. Mar. Ecol. Prog. Ser. 265, 123–139. doi: 10.3354/meps265123
Lopez Ramos E., Gonzalez Penagos F., Rincón Martinez D. A., Moreno Gomez N. R. (2022). Detachment levels of Colombian Caribbean mud volcanoes. CT&F-Ciencia Tecnología y Futuro 12, 49–77. doi: 10.29047/01225383.401
Luff R., Wallmann K. (2003). Fluid flow, methane fluxes, carbonate precipitation and biogeochemical turnover in gas hydrate-bearing sediments at Hydrate Ridge, Cascadia Margin: numerical modeling and mass balances. Geochim. Cosmochimica Acta 67, 3403–3421. doi: 10.1016/S0016-7037(03)00127-3
MacDonald I. R. (1998). Habitat formation at Gulf of Mexico hydrocarbon seeps. Cahiers Biol. Mar. 39, 337–340.
MacDonald I. R., Bohrmann G., Escobar E., Abegg F., Blanchon P., Blinova V., et al. (2004). Asphalt volcanism and chemosynthetic life in the Campeche Knolls, Gulf of Mexico. Science 304, 999–1002. doi: 10.1126/science.1097154
MacDonald I. R., Guinasso N. L., Reilly J. F., Brooks J. M., Callender W. R., Gabrielle S. G. (1990). Gulf of Mexico hydrocarbon seep communities: VI. Patterns in community structure and habitat. Geo Marine Lett. 10, 244–252. doi: 10.1007/BF02431071
Macpherson E. (1994). Occurrence of two lithodid crabs (Crustacea: Decapoda: Lithodidae) in the cold seep zone of the South Barbados accretionary prism. PROCEEDINGS-BIOLOGICAL Soc. OF WASHINGTON 107, 465–465.
Martin J. W., Wishner K., Emit J. R. (2005). Caridean and sergestid shrimp from the Kick’em Jenny submarine volcano, southeastern Caribbean Sea. Crustaceana 78 (2), 215–221.
McMullin E. R., Bergquist D. C., Fisher C. R. (2007). Metazoans in extreme environments: adaptations of hydrothermal vent and hydrocarbon seep fauna. Gravitational Space Res. 13 (2), 13–23.
Naehr T. H., Birgel D., Bohrmann G., MacDonald I. R., Kasten S. (2009). Biogeochemical controls on authigenic carbonate formation at the Chapopote “asphalt volcano”, Bay of Campeche. Chem. Geol. 266, 390–402. doi: 10.1016/j.chemgeo.2009.07.002
Nagano Y., Nagahama T., Hatada Y., Nunoura T., Takami H., Miyazaki J., et al. (2010). Fungal diversity in deep-sea sediments–the presence of novel fungal groups. Fungal Ecol. 3, 316–325. doi: 10.1016/j.funeco.2010.01.002
Olu K., Cordes E. E., Fisher C. R., Brooks J. M., Sibuet M., Desbruyères D. (2010). Biogeography and potential exchanges among the Atlantic equatorial belt cold-seep faunas. PloS One 5, e11967. doi: 10.1371/journal.pone.0011967
Olu K., Lance S., Sibuet M., Henry P., Fiala-Médioni A., Dinet A. (1997). Cold seep communities as indicators of fluid expulsion patterns through mud volcanoes seaward of the Barbados accretionary prism. Deep Sea Res. Part I: Oceanogr. Res. Papers 44, 811–841. doi: 10.1016/S0967-0637(96)00123-9
Olu K., Sibuet M., Harmegnies F., Foucher J. P., Fiala-Medioni A. (1996). Spatial distribution of diverse cold seep communities living on various diapiric structures of the southern Barbados prism. Prog. Oceanogr. 38, 347–376. doi: 10.1016/S0079-6611(97)00006-2
Olu-Le Roy K., Von Cosel R., Hourdez S., Carney S. L., Jollivet D. (2007). Amphi-Atlantic cold-seep Bathymodiolus species complexes across the equatorial belt. Deep Sea Res. Part I: Oceanogr. Res. Papers 54, 1890–1911. doi: 10.1016/j.dsr.2007.07.004
Osorio-Granada A. M., Jigena-Antelo B., Vidal-Perez J., Zambianchi E., Osorio-Granada E. G., Torrecillas C., et al. (2023). Acoustic evidence of shallow gas occurrences in the offshore sinú Fold Belt, Colombian Caribbean Sea. J. Mar. Sci. Eng. 11, 2121. doi: 10.3390/jmse11112121
Panieri G., Aharon P., Sen Gupta B. K., Camerlenghi A., Ferrer F. P., Cacho I. (2014). Late Holocene foraminifera of Blake Ridge diapir: Assemblage variation and stable-isotope record in gas-hydrate bearing sediments. Mar. Geol. 353, 99–107. doi: 10.1016/j.margeo.2014.03.020
Panieri G., Sen Gupta B. K. (2008). Benthic foraminifera of the Blake ridge hydrate mound, Western North Atlantic Ocean. Mar. Micropaleontol. 66/2, 91–102. doi: 10.1016/j.marmicro.2007.08.002
Paull C. K., Hecker B., Commeau R., Freeman-Lynde R. P., Neumann C., Corso W. P., et al. (1984). Biological communities at the Florida Escarpment resemble hydrothermal vent taxa. Science 226, 965–967. doi: 10.1126/science.226.4677.965
Paull C. K., Ussler W. III, Borowski W. S., Spiess F. N. (1995). Methane-rich plumes on the Carolina continental rise: Associations with gas hydrates. Geology 23, 89–92. doi: 10.1130/0091-7613(1995)023<0089:MRPOTC>2.3.CO;2
Pech Pool D., Ardisson Herrera P. L. (2010). “Diversidad en el Bentos Marino-Costero,” in Biodiversidad y Desarrollo Humano en Yucatán. Eds. Duran R., Méndez M. (Mérida, Yucatán, Mexico: CICY; PPD-FMAM; CONABIO; SEDUMA), 144–146.
Pereira O. S., Gonzalez J., Mendoza G. F., Le J., Coscino C. L., Lee R. W., et al. (2021). The dynamic influence of methane seepage on macrofauna inhabiting authigenic carbonates. Ecosphere 12, e03744. doi: 10.1002/ecs2.3744
Pilcher R., Argent J. (2007). Mega-pockmarks and linear pockmark trains on the West African continental margin. Mar. Geol. 244 (1-4), 15–32. doi: 10.1016/j.margeo.2007.05.002
Powell E. N., Bright T. J. (1981). A thiobios does exist — gnathostomulid domination of the canyon community at the East Flower Garden Brine Seep. Internationale Rev. der Gesamten Hydrobiol. 66, 675–683. doi: 10.1002/iroh.19810660504
Powell E. N., Bright T. J., Woods A., Gittings S. (1983). Meiofauna and the thiobios in the east flower garden brine seep. Mar. Biol. 73, 269–283. doi: 10.1007/BF00392253
Raineault N. A., Flanders J. (2019). New frontiers in ocean exploration: The E/V Nautilus, NOAA Ship Okeanos Explorer, and R/V Falkor 2018 field season. Oceanography 32 (1), 150. doi: 10.5670/oceanog.2019.supplement.01
Reed D. L., Silver E. A., Tagudin J. E., Shipley T. H., Vrolijk P. (1990). Relations between mud volcanoes, thrust deformation, slope sedimentation, and gas hydrate, offshore north Panama. Mar. Petroleum Geol. 7, 44–54. doi: 10.1016/0264-8172(90)90055-L
Robinson C. A., Bernhard J. M., Levin L. A., Mendoza G. F., Blanks J. K. (2004). Surficial hydrocarbon seep infauna from the Blake Ridge (Atlantic Ocean 2150 m) and the Gulf of Mexico (690–2240 m). Mar. Ecol. 25, 313–336. doi: 10.1111/j.1439-0485.2004.00034.x
Rodríguez I., Bulnes M., Poblet J., Masini M., Flinch J. (2021). Structural style and evolution of the offshore portion of the Sinu Fold Belt (South Caribbean Deformed Belt) and adjacent part of the Colombian Basin. Mar. Petroleum Geol. 125, 104862. doi: 10.1016/j.marpetgeo.2020.104862
Roelofse C., Alves T. M., Gafeira J. (2020). Structural controls on shallow fluid flow and associated pockmark fields in the East Breaks area, northern Gulf of Mexico. Mar. Petroleum Geol. 112, 104074. doi: 10.1016/j.marpetgeo.2019.104074
Sánchez C., Permanyer A. (2006). Origin and alteration of oils and oil seeps from the Sinú-San Jacinto Basin, Colombia. Organic Geochem. 37, 1831–1845. doi: 10.1016/j.orggeochem.2006.07.012
Sassen R., MacDonald I. R., Requejo A. G., Guinasso N. L., Kennicutt M. C., Sweet S. T., et al. (1994). Organic geochemistry of sediments from chemosynthetic communities, Gulf of Mexico slope. Geo Marine Lett. 14, 110–119. doi: 10.1007/BF01203722
Schweitzer C. E., Feldmann R. M. (2008). New Eocene hydrocarbon seep decapod crustacean (Anomura: Galatheidae: Shinkaiinae) and its paleobiology. J. Paleontol. 82, 1021–1029. doi: 10.1666/08-007.1
Sibuet M., Olu K. (1998). Biogeography, biodiversity and fluid dependence of deep-sea cold-seep communities at active and passive margins. Deep Sea Res. II 45, 51–567. doi: 10.1016/S0967-0645(97)00074-X
Solheim A., Elverhøi A. (1985). A pockmark field in the Central Barents Sea; gas from a petrogenic source. Polar Res. 3, 11–19. doi: 10.1111/j.1751-8369.1985.tb00492.x
Solomon E. A., Kastner M., MacDonald I. R., Leifer I. (2009). Considerable methane fluxes to the atmosphere from hydrocarbon seeps in the Gulf of Mexico. Nat. Geosci. 2, 561–565. doi: 10.1038/ngeo574
Soto L. A., García S. S., Veneroni D. L. (2004). Ambientes influidos por emanaciones naturales de hidrocarburos y gas en el suroeste del Golfo de México Vol. I. (Universidad Juárez Autónoma de Tabasco. Villahermosa, México: Universidad y Ciencia), 51–58.
Sun Y., Gong S., Li N., Peckmann J., Jin M., Roberts H. H., et al. (2020). A new approach to discern the hydrocarbon sources (oil vs. methane) of authigenic carbonates forming at marine seeps. Mar. Petroleum Geol. 114, 104230. doi: 10.1016/j.marpetgeo.2020.104230
Thaler A. D., Van Dover C. L., Vilgalys R. (2012). Ascomycete phylotypes recovered from a Gulf of Mexico methane seep are identical to an uncultured deep-sea fungal clade from the Pacific. Fungal Ecol. 5, 270–273. doi: 10.1016/j.funeco.2011.07.002
Toone T. A., Washburn T. W. (2020). Phytodetritus, chemosynthesis, and the dark biosphere: Does depth influence trophic relationships at deep-sea Barbados seeps. Deep Sea Res. Part I: Oceanogr. Res. Papers 165, 103367. doi: 10.1016/j.dsr.2020.103367
Tryon M. D., Brown K. M., Torres M. E. (2002). Fluid and chemical fluxes in and out of sediments hosting hydrate deposits on Hydrate Ridge, OR, II: hydrological processes. Earth Planet. Sci. Lett. 201, 541–557. doi: 10.1016/S0012-821X(02)00732-X
Tunnicliffe V., Juniper S. K., Sibuet M. (2003). “Reducing environments of the deep-sea floor,” in Ecosystems of the deep oceans. Ed. Tyler P. A. (Elsevier, Amsterdam), 81–110.
Turnipseed M., Jenkins C. D., Van Dover C. L. (2004). Community structure in Florida Escarpment seep and Snake Pit (Mid-Atlantic Ridge) vent mussel beds. Mar. Biol. 145, 121–132. doi: 10.1007/s00227-004-1304-z
Valentine D. L., Reddy C. M., Farwell C., Hill T. M., Pizarro O., Yoerger D. R., et al. (2010). Asphalt volcanoes as a potential source of methane to late Pleistocene coastal waters. Nat. Geosci. 3, 345–348. doi: 10.1038/ngeo848
Van Dover C. L. (2000). The ecology of deep-sea hydrothermal vents (Princeton, New Jersey: Princeton University Press). doi: 10.1515/9780691239477
Van Dover C. L., Aharon P., Bernhard J. M., Caylor E., Doerries M., Flickinger W., et al. (2003). Blake Ridge methane seeps: characterization of a soft-sediment, chemosynthetically based ecosystem. Deep Sea Res. Part I: Oceanogr. Res. Papers 50, 281–300. doi: 10.1016/S0967-0637(02)00162-0
Van Dover C. L., German C. R., Speer K. G., Parson L. M., And R. C., Vrijenhoek (2002). Evolution and biogeography of deep-sea vent and seep invertebrates. Science 295, 1253–1257. doi: 10.1126/science.1067361
Van Dover C. L., Lichtwardt R. W. (1986). A new trichomycete commensal with a galatheid squat lobster from deep-sea hydrothermal vents. Biol. Bull. 171, 461–468. doi: 10.2307/1541687
Wagner J. K., McEntee M. H., Brothers L. L., German C. R., Kaiser C. L., Yoerger D. R., et al. (2013). Cold-seep habitat mapping: High-resolution spatial characterization of the Blake Ridge Diapir seep field. Deep Sea Res. Part II: Topical Stud. Oceanogr. 92, 183–188. doi: 10.1016/j.dsr2.2013.02.008
Washburn T. W., Demopoulos A. W., Montagna P. A. (2018). Macrobenthic infaunal communities associated with deep-sea hydrocarbon seeps in the northern Gulf of Mexico. Mar. Ecol. 39, e12508. doi: 10.1111/maec.12508
Watanabe H., Fujikura K., Kojima S., Miyazaki J. I., Fujiwara Y. (2010). “Japan: vents and seeps in close proximity,” in The vent and seep biota: aspects from microbes to ecosystems. Ed. Kiel S. (Springer, Netherlands), 379–401. doi: 10.1007/978–90-481–9572-5_12
Watkins J. S., Worzel J. L. (1978). Serendipity gas seep area, South Texas offshore: GEOLOGIC NOTES. AAPG Bull. 62, 1067–1074.
Keywords: cold seeps, diapirs, mud volcanoes, salt tectonics, and fauna
Citation: Aguilar Pérez MI, Zapata-Ramírez PA and Micallef A (2024) A review of cold seeps in the Western Atlantic, focusing on Colombia and the Caribbean. Front. Mar. Sci. 11:1430377. doi: 10.3389/fmars.2024.1430377
Received: 10 May 2024; Accepted: 17 July 2024;
Published: 01 August 2024.
Edited by:
Elva G. Escobar-Briones, National Autonomous University of Mexico, MexicoReviewed by:
Chih-Lin Wei, National Taiwan University, TaiwanChunsheng Wang, Ministry of Natural Resources, China
Copyright © 2024 Aguilar Pérez, Zapata-Ramírez and Micallef. This is an open-access article distributed under the terms of the Creative Commons Attribution License (CC BY). The use, distribution or reproduction in other forums is permitted, provided the original author(s) and the copyright owner(s) are credited and that the original publication in this journal is cited, in accordance with accepted academic practice. No use, distribution or reproduction is permitted which does not comply with these terms.
*Correspondence: Paula A. Zapata-Ramírez, paula.zapataramirez@upb.edu.co