- 1Department of Applied Ecology and Phycology, Institute of Biological Sciences, University of Rostock, Rostock, Germany
- 2Institute of Pharmacy, Pharmacognosy, University of Innsbruck, Innsbruck, Austria
- 3Thuenen Institute of Baltic Sea Fisheries, Rostock, Germany
- 4Interdisciplinary Faculty, Department of Maritime Systems, University of Rostock, Rostock, Germany
Ultraviolet radiation (UVR) is the photochemically most reactive waveband of incident solar irradiation. Despite high absorption in aquatic environments, UVR causes numerous biochemical, genetic, and cytotoxic effects in aquatic organisms. To counteract UVR stress, many of those species are able to synthesize, accumulate, or acquire UV-sunscreen compounds for photoprotection from their diet. The most abundant UV sunscreens in marine and freshwater organisms are mycosporine-like amino acids (MAAs), which exhibit high molar extinction coefficients in the UVR range along with a strong photo- and heat stability. In this study, we investigated the qualitative and quantitative MAA distribution patterns in the eyes of 39 fish species, mainly from the temperate northern hemisphere (Baltic Sea, Northern Atlantic), using state-of-the-art analytical methods. The fish eyes of the most investigated species (33 taxa) contained MAAs, between one and seven different compounds. The MAAs palythine, asterina-330, palythene, and usujirene were present (as previously reported), and three new compounds, aplysiapalythine A, porphyra-334, and shinorine, were identified. Total MAA concentrations covered a wide range from trace amounts to > 4.2 mg g−1 dry weight, thereby providing the first quantitative data on MAAs in fish eyes. The highest MAA contents were measured in Sprattus sprattus, which are comparable to those of intertidal red seaweeds. The trophic transfer of MAAs from primary producers via zooplankton to the fish is discussed, along with the localization in the fish eye as well as possible additional functions.
Introduction
Solar radiation reaching the Earth’s surface is composed of infrared, visible, and ultraviolet wavelengths that affect many biotas in aquatic ecosystems (Vincent and Neale, 2000). Although sunlight is essential as an energy source for all primary producers, excessive levels can also become detrimental to organisms. Of the incident radiation, ultraviolet radiation (UVR, 280–400 nm) has the greatest potential to negatively affect aquatic organisms given the high energy content and the fact that these damaging wavelengths are absorbed by several biomolecules that are metabolically important (Pavlov et al., 2019).
UVR in aquatic ecosystems varies in space and time, e.g., due to geographic location, season, and time of the day, clouds, waves, currents, and/or optical properties of the water column. Particularly high concentrations of chromophoric dissolved organic matter (CDOM) and/or suspended particles can lead to reduced penetration of UVR into the water column (Pavlov et al., 2019).
UVR damages living organisms by inducing DNA lesions and reactive oxygen species (ROS) formation that further trigger adverse processes such as protein degradation or lipid peroxidation (Karsten, 2008; Schuch et al., 2017). From an evolutionary perspective, UV exposure posed a limiting factor for most organisms for a geologically long time, and hence numerous protection strategies developed that include the avoidance of sunlight, shielding, and UV response mechanisms that guarantee constant repair (Cockell and Knowland, 1999). The evolution of molecular protection and repair mechanisms enabled the transfer of nonmodified genetic information to the offspring. Around 2.9 billion years ago, Cyanobacteria, as the first primary producers, already possessed extra- and intracellular UV-sunscreen secondary metabolites (Cockell and Knowland, 1999; Karsten, 2008). Some of those compounds, named mycosporines, mycosporine-like amino acids (MAAs), and related compounds, define the origin of the most potent UV filters (Robson et al., 2019).
Mycosporines and MAAs represent a group of colorless and very hydrophilic substances with a low molecular weight (smaller than 400 Da) that play an essential role in the photoprotection of diverse marine and freshwater organisms. Moreover, they exhibit antioxidative activities and are involved in stress response (Shick and Dunlap, 2002; Karsten, 2008; Peng et al., 2023). These biomolecules are characterized by a cyclohexenone (mycosporines) and cyclohexenimine (MAAs) core, respectively, that is substituted with an amino acid or its amino alcohol. The molecules exhibit extraordinarily high molar extinction coefficients in the UVR region (Ԑ = 28,100 M−1 cm−1 to 50,000 M−1 cm−1) with typical absorption maxima between 310 nm and 360 nm due to the presence of conjugated double bonds. Moreover, cyclohexenimine-type MAAs like shinorine, porphyra-334, or palythine are known to be chemically very stable and heat/photoresistant (Karsten, 2008 and references therein).
Peng et al. (2023) listed 78 MAAs, mostly occurring in primary producers such as red macroalgae but also in other algal groups and cyanobacteria. MAAs were also reported in many marine invertebrates like sponges, sea urchins, or corals, as well as in vertebrates like fish (Shick and Dunlap, 2002). Various primary producers synthesize these UV-sunscreen molecules, which are then transferred via the food web to higher trophic levels, such as zooplankton or fish. Notably, sea urchins can even transfer these compounds to their eggs (Shick and Dunlap, 2002). The MAAs palythinol, palythene, and asterina-330 were reported from the ocular lenses of tropical fish species (Dunlap et al., 1989). The ocular tissues from 52 species of tropical marine fish from the Great Barrier Reef contained variable levels of MAAs, with interspecific variations in total lens concentrations ranging from 7.96 pmol mg−1 protein (white-tip reef shark) to 2,357 nmol mg−1 protein (scarlet-breasted Maori wrasse), the latter equivalent to a concentration of about 60 mM (calculated from Dunlap et al., 1989). In warm-temperate to tropical fish species, MAAs were found in around 170 taxa (Dunlap et al., 1989; Mason et al., 1998; Siebeck and Marshall, 2001), mainly located in the eyes and the mucus (Zamzow and Losey, 2002; Zamzow, 2004, 2007; Eckes et al., 2008), as well as in eggs (Chioccara et al., 1980; Grant et al., 1980; Planck et al., 1981; Arbeloa et al., 2010). For temperate to coldwater fish, we are not aware of any study analyzing fish eyes for MAAs.
At present, only a few MAAs could be detected in the eyes of about 170 fish species screened for their composition of MAAs, out of > 34,000 known fish species (www.fishbase.org). One aspect related to the poor state of knowledge on MAAs in fish eyes might be explained by the analytical HPLC method widely applied in the previous publications (e.g., Dunlap et al., 1989; Thorpe et al., 1993), which turned out to be inaccurate due to insufficient resolution (double peaks or coelution) (Karsten et al., 2009). As a consequence, the application of this previous HPLC approach led to the detection of only four MAAs in the red alga Bostrychia scorpioides, while the use of a new state-of-the-art analytical technique resulted in 12 additional and new MAAs (Bostrychines A to L) (Orfanoudaki et al., 2019, 2023). Due to these methodological constraints, the true chemical diversity of MAAs in fish eyes from different biogeographic regions is poorly studied, and their specific functions and roles are still mostly putative, given the great species diversity in fishes.
The present study aims at chemically screening the eyes of 39 fish species from European waters for their composition of MAAs, both in terms of compounds and the amount of MAAs per dry weight. We assumed that the eyes of most fish species contain MAAs, as they are part of an aquatic food web that is based on MAA-forming primary producers.
Materials and methods
Fish samples and eye dissection
In total, 39 fish species identified by experts to species level were provided by different German institutions (Tables 1, 2), such as the Federal German Thünen-Institute of Baltic Sea Fisheries (Rostock, Germany), the German Oceanographic Museum (Stralsund, Germany), the Alfred-Wegener-Institute for Marine and Polar Research (Bremerhaven, Germany), and independent local fishermen. Of the 39 species, 26 were almost marine taxa and 13 originated from freshwater habitats (Tables 1, 2). Some species, such as Sander lucioperca, for example, widely occur in freshwater habitats (lakes, rivers) but also in the brackish water of the Baltic Sea.
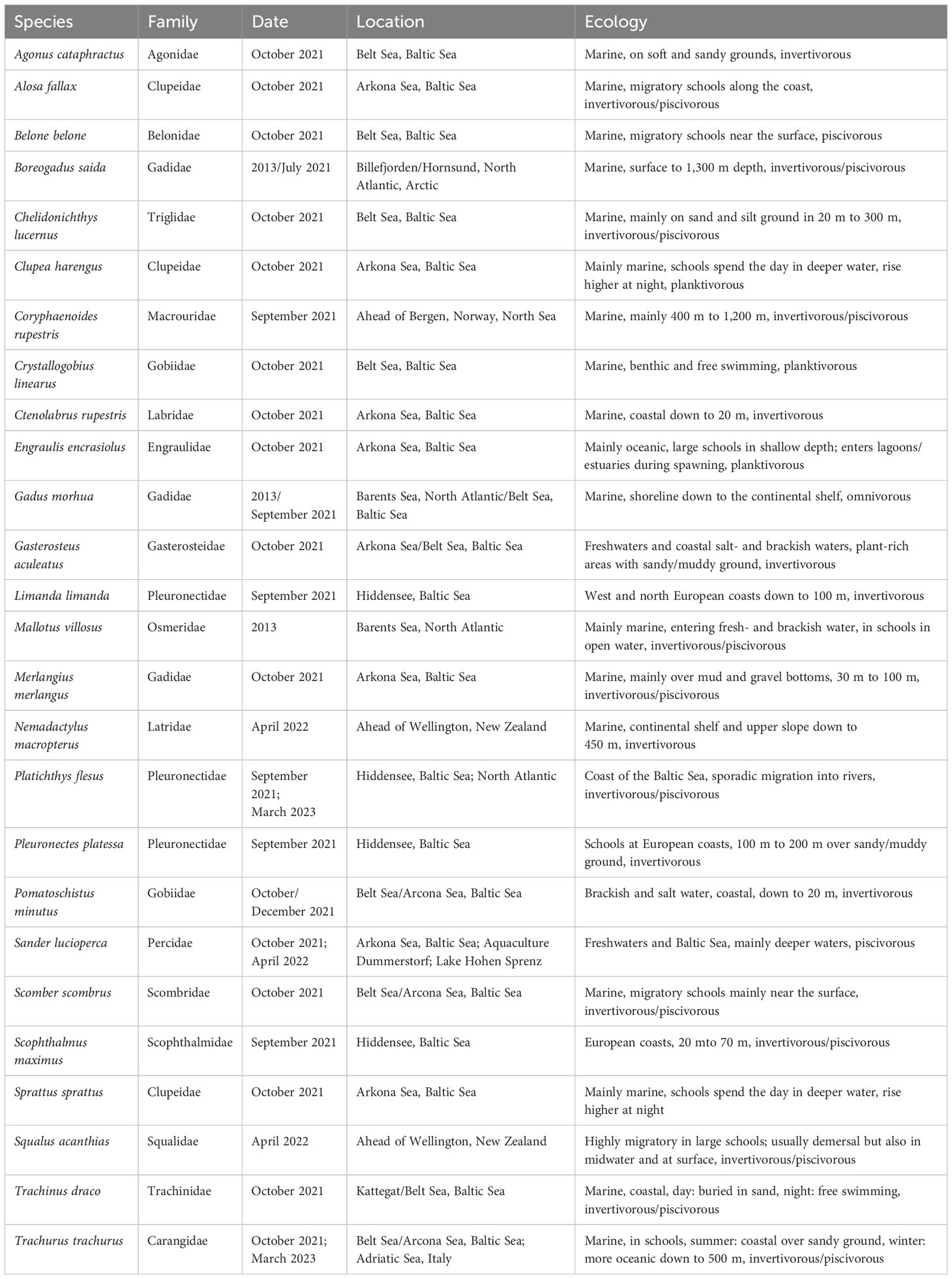
Table 1 Overview of sampled marine fish species, phylogenetic family (after Naylor et al., 2012; Hughes et al., 2018; Fricke et al., 2022), date and location of capture, behavior, and diet information according to literature.
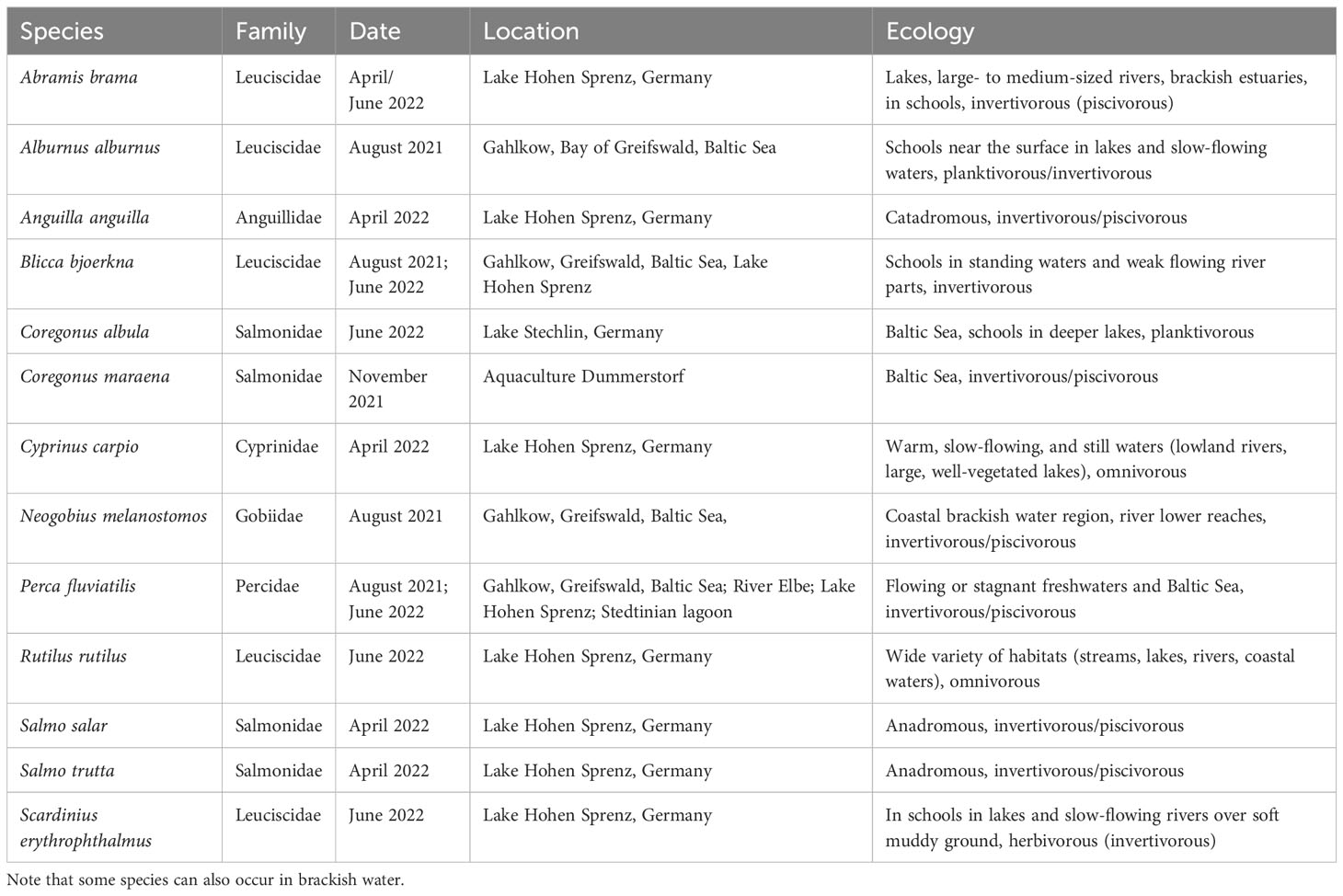
Table 2 Overview of sampled freshwater fish species, phylogenetic family (after Naylor et al., 2012; Hughes et al., 2018; Fricke et al., 2022), date and location of capture, behavior, and diet information according to literature.
Most samples were fresh, others were provided as frozen fish, and there was no difference between both conditions in the later chemical analysis. Duplicate samples were always processed.
The fish eyes were dissected by (1) cutting the retro bulbar fat tissue with blood vessels around the eye (Duke-Elder, 1958; Jurk, 2002), followed by (2) dissection of the four straight and the two oblique ocular muscles as well as the optical nerve (Demoll et al., 1964; Reimann, 2015).
After dissection, the intact fish eyes were briefly washed with distilled water to remove external tissue remains, frozen at − 18°C, and freeze-dried with a lyophiliser (Alpha 1-4 LSCPlus, Martin Christ GmbH, Osterode am Harz, Germany). The freeze-dried eyes were stored at − 18°C prior to MAA extraction.
MAA extraction
For the MAA extraction, 1 mL of aqueous methanol v/v (25%) was added to 10–15 mg of freeze-dried, pulverized fish eye sample in screw-capped vials. The samples were vortexed and left in a water bath at 45°C for 3–4 h. Extracts were vortexed after 2 h and after 3–4 h. This approach has been previously evaluated as a complete quantitative and qualitative extraction method (e.g., Carreto et al., 1990; Bandaranayake, 1998; Karsten et al., 2009). After 5 min of centrifugation (2,376×g) (Biofuge pico, Heraeus, Hanau, Germany), 800 μL of the supernatant was transferred into a new vial and evaporated overnight to dryness using a rotational vacuum concentrator (Christ RVC 2-25, Martin Christ GmbH, Osterode am Harz, Germany). The dried pellets were redissolved in 800 μL of high-pressure liquid chromatography (HPLC)-grade water, vortexed for 30 s, and centrifuged for an additional 5 min (16,058×g) (Biofuge pico, Heraeus, Hanau, Germany). The supernatant was then transferred into HPLC vials and stored in a freezer at − 18°C until being analyzed.
MAA analysis by high-pressure liquid chromatography
Solvents and chemicals used for liquid chromatography were purchased from Merck (Darmstadt, Germany). Ammonium formate was obtained from Serva (Heidelberg, Germany) and MS-grade formic acid from VWR International (Vienna, Austria). Ultra-pure water was prepared in-house with an Arium 611 UV purification system (Sartorius, Göttingen, Germany). Standards of mycosporine-like amino acids with confirmed chemical structure and purity were available from previous projects (Orfanoudaki et al., 2019, 2023).
HPLC analyses were performed on a Merck–Hitachi Elite La Chrom instrument (Tokyo, Japan) equipped with a quaternary pump, autosampler, column thermostat, and diode-array detector. Separation conditions were according to the method described by Orfanoudaki et al. (2020). The stationary phase was a YMC-Pack ODS column (250 mm × 4.60 mm, 5 μm; YMC Europe, Dinslaken, Germany), which was protected by a respective guard column. The mobile phase consisted of 20 mM ammonium formate and 0.25% (v/v) formic acid in water (A) and methanol (B). The applied gradient was as follows: 100% A from 0 min to 20 min, 80% A at 30 min, 2% A at 35 min, and held at this composition for 5 min (total runtime of 40 min), followed by 15 min of re-equilibration under the initial conditions. Flow rate, temperature, and injection volume were adjusted to 0.65 mL min−1, 9°C, and 5.0 μL. Detection wavelengths were set to 310 nm, 330 nm, and 350 nm. All samples were membrane-filtered (0.45 µm pore size) prior to analysis. The integration of target peaks was carried out with the EZChrom Elite software using the chromatograms recorded at 330 nm.
Stock solutions of shinorine, palythine, asterina-330, porphyra-334, and aplysiapalythine A originating from a previous study (Orfanoudaki et al., 2020) were used for calibration. At least six calibrator levels per compound were prepared by dilution in water, and each level was tested in triplicate. Calibration curves were prepared by plotting the peak areas versus the concentrations of each MAA. The regression parameters (intercept, slope, and determination coefficient) were calculated by linear regression analysis using Microsoft Excel. The limit of detection (LOD) and limit of quantification (LOQ) for each MAA were calculated from the regression models. The LOD was calculated as 3.3 times the residual standard deviation of the regression line divided by the slope of the calibration curve, whereas the LOQ was calculated as 10 times the residual standard deviation divided by the slope. Usujirene and palythene were quantified by using the calibration curve of aplysiapalythine A.
UHPLC-HRMS/MS experiments served to confirm the identity of the assigned/quantified MAAs and were performed according to the method described by Zwerger et al. (2023). The experiments were performed on a Vanquish system (Thermo Scientific, Waltham, MA, USA) consisting of a quaternary pump, autosampler, column oven, and variable wavelength detector connected to a Thermo Scientific Exploris 120 Orbitrap HRMS unit. Separation was carried out on a Luna Omega C18 100 Å column (100 mm × 2.1 mm; particle size, 1.6 µm; Phenomenex, Torrance, CA, USA) protected by a SecurityGuard ULTRA Guard C18 precolumn. The mobile phase comprised water with 0.25% formic acid and 20 mM ammonium formate (A) and acetonitrile (B). All other parameters and settings can be found in the aforementioned publication (Zwerger et al., 2023).
Results
Representative HPLC chromatograms with the seven MAAs found in fish eyes are given, along with their chemical structures (Figure 1). Mass spectra of the individual MAAs are provided in the Supplementary Material.
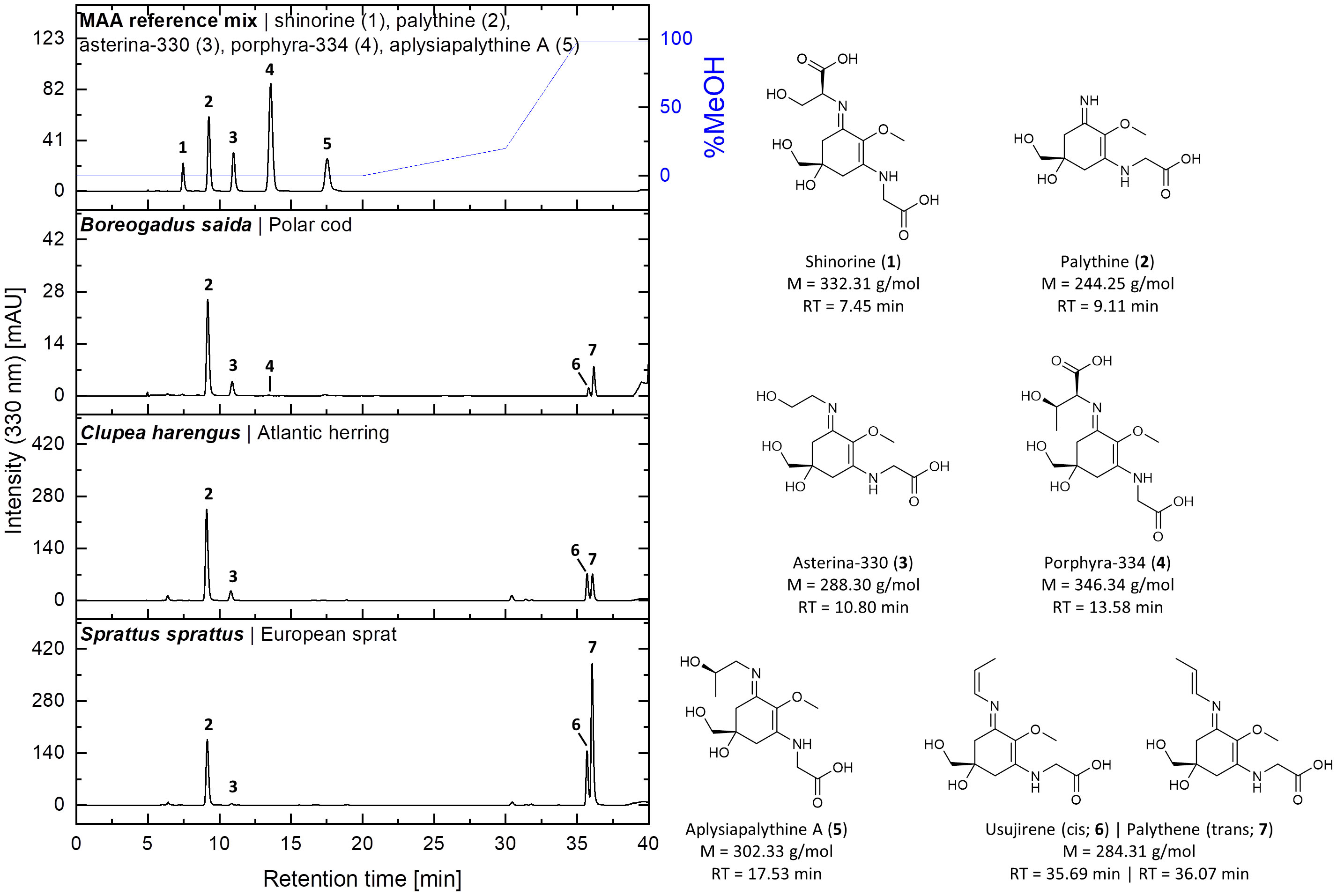
Figure 1 HPLC chromatograms of a standard mixture and aqueous-methanolic fish eye extracts of Boreogadus saida, Clupea harengus, and Sprattus sprattus recorded at 330 nm. The chemical structures, molecular weights (M), and retention times (RT) of the detected MAAs are depicted on the right. The identity of usujirene (6) and palythene (7) in the samples was confirmed by UHPLC-HRMS/MS.
UV-absorbing MAAs were found in the eyes of 33 out of 39 investigated fish species using the HPLC approach (Figure 2). The MAAs palythine, asterina-330, palythene, and usujirene were, as previously reported in the literature, present, and three new compounds, aplysiapalythine A, porphyra-334, and shinorine, could be identified. Total MAA contents covered a wide range from trace amounts up to 4.2 mg g−1 dry weight, thereby providing the first quantitative data on MAAs in fish eyes.
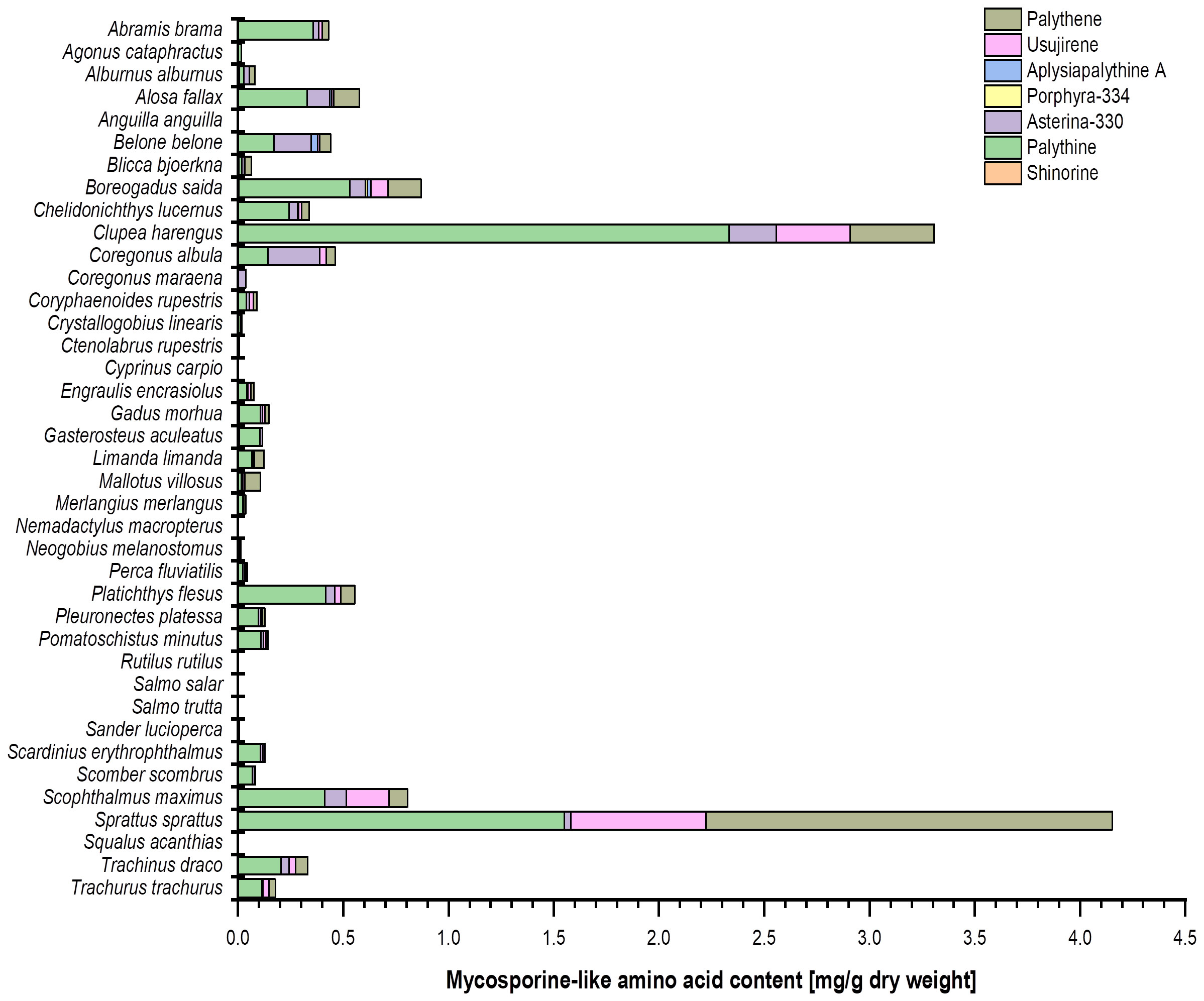
Figure 2 Bar chart representing the MAA content (mg g−1 dry weight) and composition in the investigated fish eye extracts (in alphabetical order). The values originate from (at least) biological duplicate measurements. Usujirene and palythene were quantified using the calibration curve of aplysiapalythine A. For the sake of simplicity, all mean values were used without taking the LOD and LOQ of the respective calibration curves into account.
Most of the marine fish species (24 out of 26 taxa) contained MAAs in their eyes, some even in high concentrations (Table 1; Figure 2). In contrast, 12 out of 13 freshwater species revealed only traces or lacked MAAs, except Abramis brama, which exhibited 0.36 mg palythine g−1 dry weight (Table 2; Figure 2).
The eyes of Anguilla anguilla, Cyprinus carpio, Nemadactylus macropterus, Rutilus rutilus, Salmo trutta, and Squalus acanthias did not contain any trace of MAAs (Figure 2). In their eyes, Ctenolabrus rupestris exhibited a trace of palythine and Salmo salar a trace of asterina-330. In the other species, aplysiapalythine A occurred only in Boreogadus saida (Figures 2, 3). Shinorine and porphyra-334 were measured as trace concentrations in some fish eyes and only in a relatively small number of marine species. Although usujirene occurred in a few marine species only, concentrations between 0.2 mg g−1 and 0.6 mg g−1 dry weight were detected in Clupea harengus, Scophthalmus maximus, and Sprattus sprattus (Figure 2). Palythene was determined in nine different marine species, and values up to 1.9 mg g−1 dry weight were measured, the highest amount in the eyes of Sprattus sprattus. In contrast, palythine and asterina-330 were the most abundant MAAs among all investigated fish species. The palythine concentration in the eyes of Clupea harengus reached 2.3 mg g−1 dry weight, which was the highest individual quantitative MAA value (Figure 2). Total MAA concentrations covered a wide range from trace amounts to 4.2 mg g−1 dry weight in Sprattus sprattus.
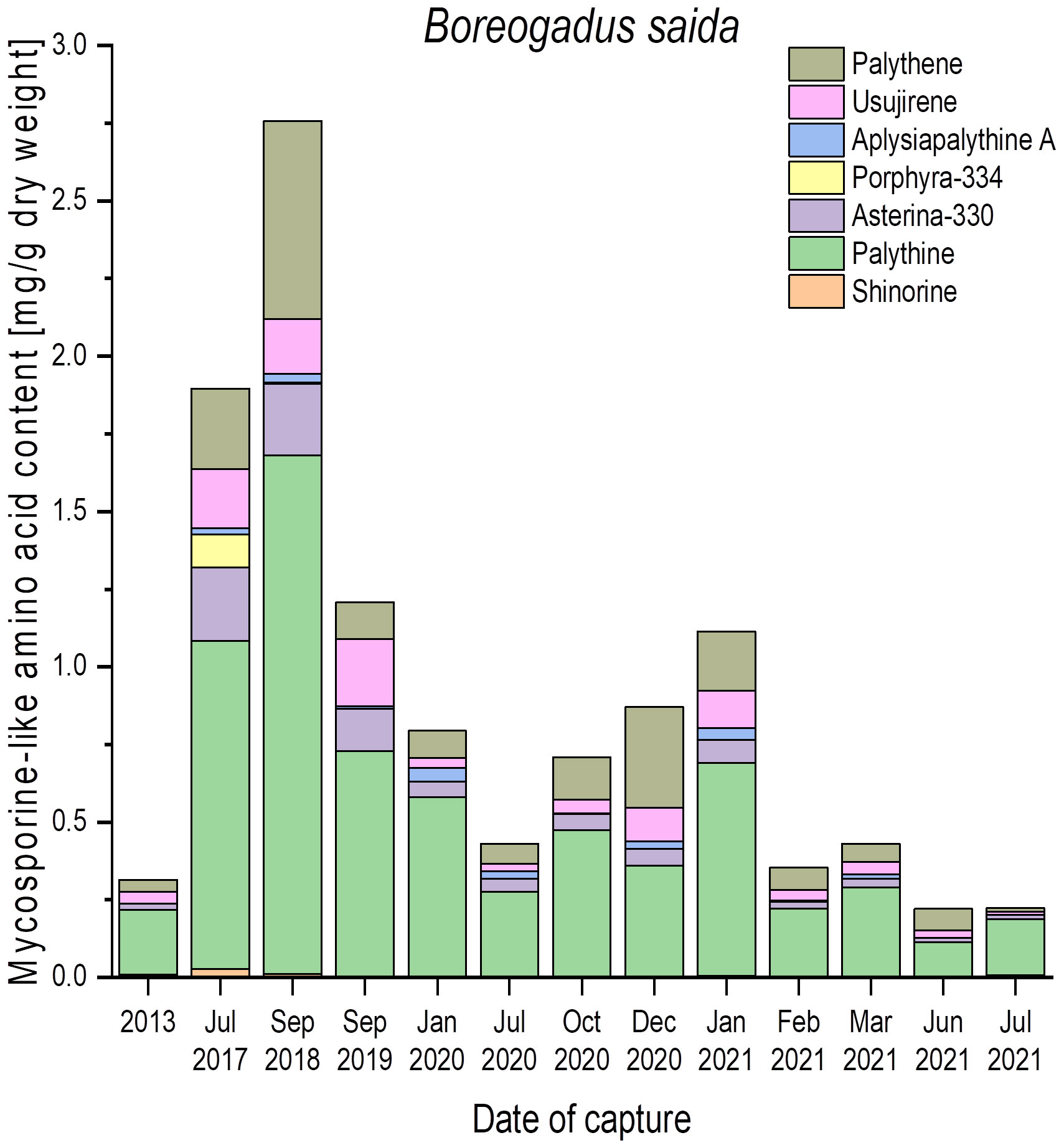
Figure 3 Qualitative and quantitative MAA contents (mg g−1 dry weight) in fish eyes of polar cod (Boreogadus saida), captured in the Billefjord, North Sea, at different dates. All values represent the means of biological duplicates. Usujirene and palythene were quantified using the calibration curve of aplysiapalythine A. For the sake of simplicity, all mean values were used without taking the LOD and LOQ of the respective calibration curves into account.
To evaluate how static or variable the MAA amounts in the fish eyes might be, numerous samples of Boreogadus saida (polar cod) were analyzed, which were all captured at different seasons in the Billefjord, North Sea, between 2013 and 2021 (Figure 3). In this species, all seven MAAs could be detected, but the qualitative and quantitative patterns exhibited a high variability. Palythine was always the most abundant MAA (> 60% of total content), ranging from 0.2 mg g−1 to 1.7 mg g−1 dry weight. The other six identified MAAs occurred in different combinations and concentrations across all samples, i.e., shinorine and porphyra-334 were not always present (Figure 3).
Trachinus draco (greater weever) was captured on four different dates in October 2021 in the Belt Sea, Baltic Sea. The eyes of this species also exhibited variable qualitative and quantitative MAA patterns. While samples from 10th October showed only trace amounts, those of 16th, 17th, and 26th October exhibited solely 0.2 mg g−1 to 0.4 mg g−1 dry weight of palythine (Figure 4). In addition, the eye samples contained asterina-330, palythene, and usujirene, resulting in total MAA concentrations between 0.3 mg g−1 and 0.6 mg g−1 dry weight (Figure 4).
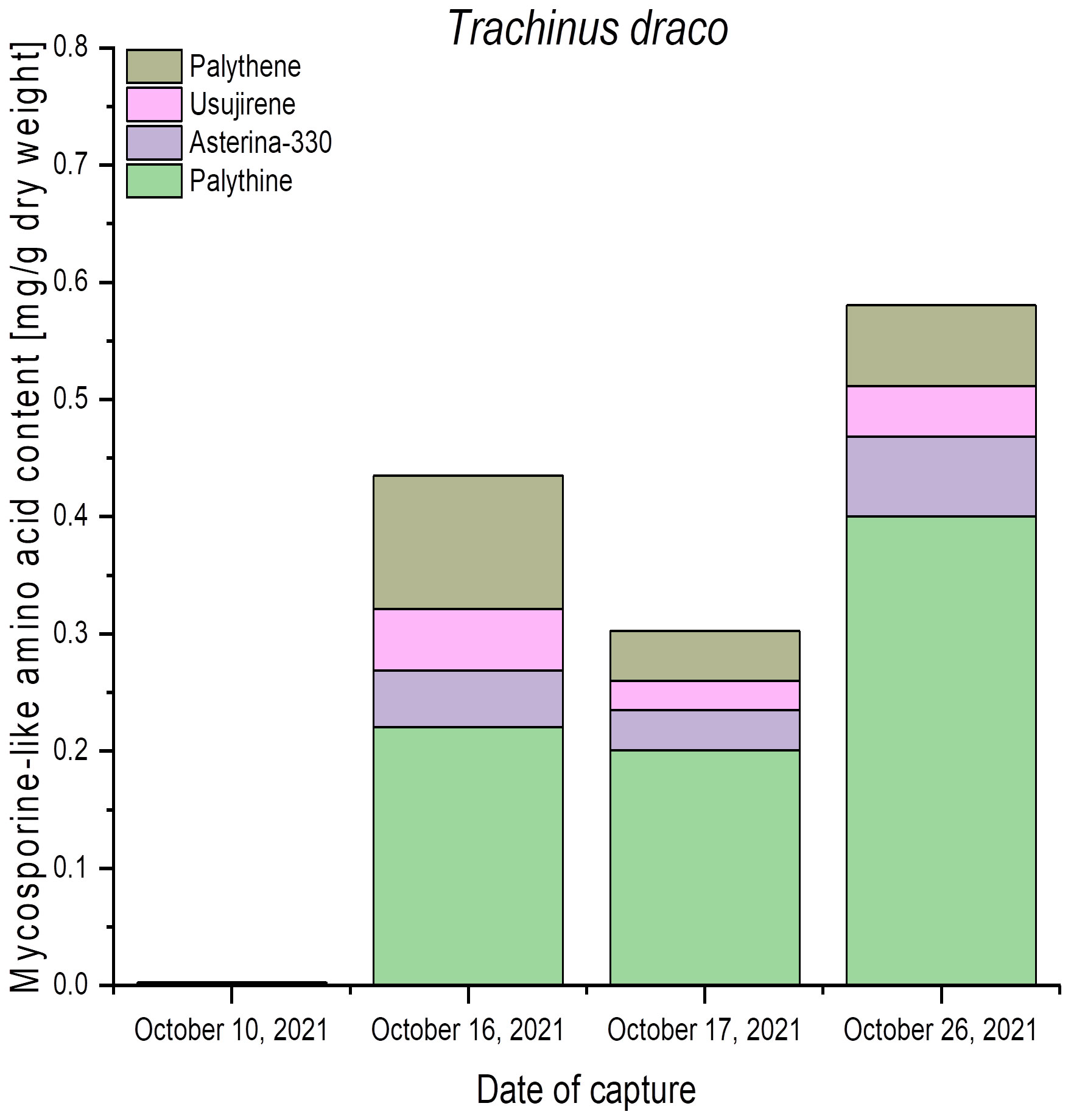
Figure 4 Qualitative and quantitative MAA contents (mg g−1 dry weight) in fish eyes of greater weever (Trachinus draco), captured in the Belt Sea, Baltic Sea, at different dates. All values represent the means of biological duplicates. Usujirene and palythene were quantified using the calibration curve of aplysiapalythine A. For the sake of simplicity, all mean values were used without taking LOD and LOQ into account.
Discussion
Mycosporines and MAAs are well-described and experimentally proven as natural UV-sunscreen compounds (Robson et al., 2019; Peng et al., 2023). They are mainly distributed among different marine algal groups and marine invertebrates. In fish, the date of the first report back to 1980 when Grant et al. (1980) and Planck et al. (1981) reported the occurrence of the MAAs palythine and palythene in the eggs of Gadus morhua (Atlantic cod). Dunlap et al. (1989) undertook the first systematic screening of MAA distribution patterns in the ocular tissues of 52 tropical fish species. They also determined palythine and palythene but additionally found asterina-330 and palythinol, and all investigated fish eyes contained at least one of these MAAs, some even all four. A few years later, Thorpe et al. (1993) studied the ocular lenses of 120 fish species from freshwater and marine habitats in temperate and tropical regions, and UV-absorbing compounds were present in the majority of examined taxa. In 10 species, the MAAs palythine, palythene, and asterina-330 could be identified but not quantified.
Overall, our qualitative and quantitative MAA data on the eyes of 39 fish species originating mainly from the southern Baltic Sea and northern Atlantic are in close agreement with these earlier studies. Nevertheless, MAAs occurred mainly in the eyes of marine or brackish fish species, while in freshwater fish, only Abramis brama out of 13 taxa exhibited a low but clearly a detectable amount of palythine (Figure 2). These data are difficult to discuss as most lakes in northern Germany are shallow, eutrophic, and hence turbid, and as a consequence, light transparency is typically low. Therefore, we expect that most freshwater fish species in these aquatic systems are rarely confronted with incident UVR, which might explain the lack of or traces of MAAs only in their eyes. In addition, to the best of our knowledge, we are not aware of MAA studies of freshwater communities in German lowland lakes, i.e., we do not know about the presence of UV-sunscreen compounds in such aquatic systems. In clear-water mountain lakes such as in the Alps and the Pyrenees, phytoplankton assemblages contain MAAs, which can be transferred via the food chain at least to zooplankton (Laurion et al., 2002), while data on fish in such water bodies are missing.
The application of a more advanced HPLC method for MAA analysis with improved separation and resolution features compared to that of Dunlap et al. (1989) and Thorpe et al. (1993) allowed the identification and confirmation of the already known UV-sunscreen compounds shinorine, palythine, asterina-330, porphyra-334, palythene, and usujirene. In addition, the so-far unreported MAA aplysiapalythine A could be unambiguously identified with our improved method and by comparison with chemically well-characterized standard compounds. Palythene is a geometric isomer of usujirene (Whittock et al., 2022), and hence both compounds exhibited a very similar retention time in previous HPLC approaches and were often treated as a pair. With our improved HPLC method, both MAAs could be separated with different retention times of 35.69 min (usujirene) and 36.07 min (palythene) (Figure 1), respectively, along with slightly different absorption maxima (357 nm versus 360 nm), which serve as a diagnostic feature.
The qualitative data presented in this study clearly indicate that the composition of MAAs in fish eyes can be more complex than considered before (Dunlap et al., 1989; Thorpe et al., 1993), as species like Boreogadus saida contained seven different MAAs (Figure 3). One explanation for this increase in the number of MAAs is of course related to the strong improvements in the development of new analytical methods over the last 20–30 years, such as in liquid chromatography and mass spectrometry, as well as the recent and successful application of feature-based molecular networking (Zwerger et al., 2023). This suggests that state-of-the-art analytical facilities and chemical networking approaches are required to qualitatively and quantitatively evaluate the “real”, mainly complex composition of MAAs of any biological sample. A good example is the red alga Bostrychia scorpioides, which grows as an epiphyte on European salt marsh plants and hence is regularly exposed to UV, salt, and desiccation stress (Orfanoudaki et al., 2019). The composition of MAAs of this species was first investigated by Karsten et al. (2000), who reported mycosporine-glycine, shinorine, porphyra-334, palythine, asterina-330, and palythinol by applying an HPLC method comparable to that of Dunlap et al. (1989). Using various chromatographic techniques in combination with nuclear magnetic resonance (NMR) spectroscopy and high-resolution mass spectrometry (HRMS), Orfanoudaki et al. (2019) reported six additional and so far unknown MAAs, bostrychines A to F, which chemical structures could also be elucidated. However, they found numerous peaks in their chromatograms that were unknown and considered putative MAAs but could not be isolated. A modified isolation protocol that included silica gel column chromatography, flash chromatography on reversed phase material, and semipreparative HPLC finally led to the purification and structural elucidation of these unknown peaks/compounds, resulting in another six MAAs, bostrychines G to L (Orfanoudaki et al., 2023). So, B. scorpioides contained at least 18 chemically identified MAAs based on progress in analytical chemistry, and for fish eyes, we also expect a plethora of UV-sunscreen compounds, most of which have yet to be isolated, purified, and elucidated.
The total MAA concentrations in the eyes of 39 fish species ranged from trace amounts to 4.2 mg g−1 dry weight. Comparison with published quantitative data on other fish eyes is difficult, as, for example, Thorpe et al. (1993) provided only qualitative information. The ocular tissues of tropical marine fish from the Great Barrier Reef contained variable levels of MAAs, with interspecific variations in total lens concentrations ranging from 7.96 pmol mg−1 protein (white-tip reef shark) to 2,357 nmol mg−1 protein (scarlet-breasted Maori wrasse) (Dunlap et al., 1989). Since these data are given on a protein basis, they are difficult to recalculate on a dry weight basis, as we do not have information on the protein content of our investigated fish eyes. If the quantitative data presented in this study are compared with those of different marine and terrestrial algae, it is obvious that 4.2 mg MAAs g−1 dry weight in Clupea harengus can be considered a relatively high amount. In many green and red algae, total MAA contents range from 1 mg g−1 to 10 mg g−1 dry weight, and 4 mg g−1 dry weight is typical for intertidal seaweeds, which are regularly confronted by high UVR during low tide (Karsten et al., 2000; Karsten, 2008).
Fish eyes are morphologically and anatomically very similar to the human eye (Reimann, 2015), which is a highly sensitive organ that can be easily damaged by UVR. UV-induced damage to ocular tissue may result in pathology to the cornea, lens, or retina. The particular combination of exposure parameters, such as wavelengths and total energy delivered, determines the primary target tissue(s). UVA can reach deeper layers of the eye, like the lens. UVB only affects the eye’s surface, but it is energy richer and consequently causes more cell damage (Zuclich, 1989; Chen et al., 2009). If the cornea remains intact, only small amounts of UVB reach the retina. Although the exact location of MAAs in fish eye tissues is unknown, it is reasonable to assume that the cornea and/or vitreous eye are of uttermost importance as the photoprotective layer/structure for the radiation-sensitive retina. Although an exact dissecting protocol is missing, Dunlap et al. (1989) provided UV absorption spectra of methanolic extracts of cornea, iris, and lens ocular tissues isolated from Makaira indica (black marlin). Each of these tissue extracts exhibited UV absorption in the range of 328 nm to 332 nm, with the highest values in the cornea and lens. These data at least support the hypothesis of MAA location primarily in the cornea.
Irrespective of the exact localization of MAAs in fish eyes, the high concentrations found in the present study suggest that they fulfill important functions. Although experimental evidence is missing for fish, studies on marine invertebrates (sea urchins, etc.) and numerous micro- and macroalgae unambiguously prove that MAAs act as natural UV-sunscreen compounds (for review, see Karsten, 2008; Peng et al., 2023, and references therein). In algae, MAAs are induced and accumulated after natural or artificial exposure to enhanced UVR (Karsten, 2008), and the presence of MAAs in terrestrial microalgae typically results in a strongly reduced UV sensitivity of growth and photosynthesis compared to related aquatic taxa without the capability for MAA biosynthesis (Karsten et al., 2007). The UV-protective function of MAAs is further supported by their chemical structure, which is based on conjugated double bonds, resulting in the highest known molar extinction coefficients of natural products in the UVR region along with impressive photostability (Whittock et al., 2022).
Apart from the sunscreen function, MAAs in the fish eye may strengthen the visual acuity by filtering out short-wavelength radiation (Dunlap et al., 1989). As a consequence, such filtering would reduce chromatic aberration caused by the misfocusing of shorter wavelengths on the retina, filter molecular scattering of short wavelength radiation (Rayleigh scattering), and improve optical contrast in the underwater blue light environment (Muntz, 1973; Dunlap et al., 1989).
In addition, various biochemical functions of MAAs, such as protecting against UVR-induced DNA damage (Peng et al., 2023) or acting as antioxidants (Dunlap and Yamamoto, 1995), have been reported. Different MAAs are capable of scavenging reactive oxygen species or inhibiting cellular damage caused by singlet oxygen, along with exerting antioxidative effects through their UVR screening (Peng et al., 2023, and references therein).
The obvious trophic transfer of MAAs from the primary producers to the consumers seems to be a rather complex but still unstudied process, as the main producers are algae, which are eaten by zooplankton, which is the diet of many fish species in the juvenile and/or adult stage. While shinorine is an abundant MAA in the algal diet, fish eyes typically contain palythine and/or asterina-330 but mainly lack shinorine (Dunlap et al., 1989; Thorpe et al., 1993; this study). These MAAs differ structurally and functionally because palythine and asterina-330 (bonded to ammonia and ethanolamine, respectively, at the C-1 iminocarbonyl position of the ring chromophore) are neutral owing to zwitterion formation, whereas shinorine (bonded to an amino acid, serine, at the C-1 position) has a net acidic character (Mason et al., 1998). Whether these intrinsic chemical differences might account for their differential uptake from the algal diet into the fish (e.g., Medaka fish (Oryzias latipes)), perhaps by distinct carriers, or whether fish have the metabolic capability to biochemically modify the sequestered MAAs as discussed for corals (Shick et al., 1999), is still an open question. In addition, the underlying molecular mechanisms, i.e., the prevention of digestion of MAAs and their transport into the eyes, are not understood. Recently, an unexpected discovery was reported, namely the de novo synthesis of gadusol in zebrafish—a compound with UVB (280–315 nm) protective activity. Gadusol is chemically related to the MAA precursor 4-deoxygadusol, but different enzymes are involved in the synthesis. Nevertheless, it is still unclear whether fish can actually synthesize MAAs or not (Osborn et al., 2015).
The presented data on Boreogadus saida and Trachinus draco indicate strong variability in the concentration and composition of MAAs across the seasons and between catches. Consequently, sufficient numbers of replicates are required for robust conclusions, and at present, it is difficult to link the MAAs detected in the fish eyes to habitat and diet information.
Many schooling zooplanktivorous fish species, such as herring or mackerel, preferentially inhabit subsurface waters, where they rely on vision for successful predation. These schooling fish species are confronted with incident UVR, and hence their eyes need protection against harmful wavelengths to avoid damage to biomolecules and cells (Dunlap et al., 1989; Thorpe et al., 1993). MAAs provide such UV-sunscreen function and hence are considered photobiological antistress metabolites for fish eyes.
Data availability statement
The original contributions presented in the study are included in the article/Supplementary Material. Further inquiries can be directed to the corresponding author.
Ethics statement
Ethical approval was not required for the studies involving animals in accordance with the local legislation and institutional requirements because we used always dead and frozen fish samples provided by our scientific network and from local fishermen. Written informed consent was not obtained from the owners for the participation of their animals in this study because all people providing samples were informed on our research, and did not ask for any written informed consent as they were interested in the data.
Author contributions
JB: Data curation, Investigation, Visualization, Writing – original draft, Writing – review & editing. FH: Investigation, Methodology, Validation, Visualization, Writing – review & editing. MG: Funding acquisition, Investigation, Methodology, Project administration, Writing – review & editing. UKr: Conceptualization, Formal analysis, Methodology, Resources, Writing – review & editing. UKa: Conceptualization, Formal analysis, Funding acquisition, Methodology, Project administration, Resources, Supervision, Validation, Writing – original draft, Writing – review & editing.
Funding
The author(s) declare financial support was received for the research, authorship, and/or publication of this article. This study was supported by the FWF-DFG DACH project UVision under grant numbers KA899/45-1 and FWF I 6122-B. Fish sampling by the Thünen Institute of Baltic Sea Fisheries was co-funded by the European Commission’s Data Collection Framework.
Acknowledgments
We thank Niklas Plag, University of Rostock, for technical support, particularly with the HPLC. Samples were provided by the Alfred-Wegener-Institute for Marine and Polar Research, Bremerhaven; the German Oceanographic Museum, Stralsund; the Thünen-Institute of Sea Fisheries in Bremerhaven; the Research Institute for Farm Animal Biology; Alexander Rixen, University of Rostock; Böttcher Fishery, Lake Stechlin; and Werner Loch, Lake Hohen Sprenz.
Conflict of interest
The authors declare that the research was conducted in the absence of any commercial or financial relationships that could be construed as a potential conflict of interest.
Publisher’s note
All claims expressed in this article are solely those of the authors and do not necessarily represent those of their affiliated organizations, or those of the publisher, the editors and the reviewers. Any product that may be evaluated in this article, or claim that may be made by its manufacturer, is not guaranteed or endorsed by the publisher.
Supplementary material
The Supplementary Material for this article can be found online at: https://www.frontiersin.org/articles/10.3389/fmars.2024.1426861/full#supplementary-material
References
Arbeloa E. M., Uez M. J., Bertolotti S. G., Churio M. S. (2010). Antioxidant activity of gadusol and occurrence in fish roes from Argentine Sea. Food Chem. 119, 586–591. doi: 10.1016/j.foodchem.2009.06.061
Bandaranayake W. M. (1998). Mycosporines: are they nature’s sunscreens? Nat. Prod. Rep. 15, 159–172. doi: 10.1039/A815159Y
Carreto J. I., Carignan M. O., Daleo G., De Marco S. G. (1990). Occurrence of mycosporine-like amino acids in the red-tide dinoflagellate Alexandrium excavatum: UV-photoprotective compounds? J. Plankton Res. 12, 909–921. doi: 10.1093/plankt/12.5.909
Chen Y., Mehta G., Vasiliou V. (2009). Antioxidant defenses in the ocular surface. Ocul. Surf 7, 176–185. doi: 10.1016/S1542-0124(12)70185-4
Chioccara F., Della Gala A., De Rosa M., Novellino E., Prota G. (1980). Mycosporine amino acids and related compounds from the eggs of fishes. Bull. Soc Chim. Belg 89, 1101–1106. doi: 10.1002/bscb.19800891212
Cockell C. S., Knowland J. (1999). Ultraviolet radiation screening compounds. Biol. Rev. Cambridge Philos. Soc. 74, 311–345. doi: 10.1017/S0006323199005356
Demoll R., Maier H. N., Wundsch H. H. (1964). Handbuch der Binnenfischerei Mitteleuropas (Stuttgart: Band II A. E. Schweizerbart’sche Verlagsbuchhandlung).
Duke-Elder S. (1958). “The Eyes of Fishes,” in System of Ophthalmology, vol. 1. (Kimpton, London, UK), 274–331.
Dunlap W. C., Williams D. M. C. B., Chalker B. E., Banaszak A. T. (1989). Biochemical photoadaptation in vision: U.V.-absorbing pigments in fish eye tissues. Comp. Biochem. Physiol. Part B Comp. Biochem. 93, 601–607. doi: 10.1016/0305-0491(89)90383-0
Dunlap W. C., Yamamoto Y. (1995). Small-molecule antioxidants in marine organisms: Antioxidant activity of mycosporine-glycine. Comp. Biochem. Physiol. Part B Comp. Biochem. 112, 105–114. doi: 10.1016/0305-0491(95)00086-N
Eckes M. J., Siebeck U. E., Dove S., Grutter A. S. (2008). Ultraviolet sunscreens in reef fish mucus. Mar. Ecol. Prog. Ser. 353, 203–211. doi: 10.3354/meps07210
Fricke R., Eschmeyer W. N., Fong J. D. (2022). Species by family/subfamily. Available online at: http://researcharchive.calacademy.org/research/ichthyology/catalog/SpeciesByFamily.asp (Accessed 29th November 2023).
Grant P. T., Planck P. A., Thomson R. H. (1980). Gadusol, a metabolite from fish eggs. Tetrahedron Lett. 21, 4043–4044. doi: 10.1016/S0040-4039(00)92866-1
Hughes L. C., Ortí G., Huang Y., Sun Y., Baldwin C. C., Thompson A. W., et al. (2018). Comprehensive phylogeny of ray-finned fishes (Actinopterygii) based on transcriptomic and genomic data. Proc. Natl. Acad. Sci. U.S.A. 115, 6249–6254. doi: 10.1073/pnas.1719358115
Jurk I. (2002). Ophthalmic disease of fish. Vet. Clin. North Am. Exot. Anim. Pract. 5, 243–260. doi: 10.1016/S1094-9194(02)00006-3
Karsten U. (2008). “Defense strategies of algae and cyanobacteria against solar ultraviolet radiation,” in Algal chemical ecology. Ed. Amsler C. D. (Springer-Verlag, Berlin), 273–296. doi: 10.1007/978-3-540-74181-7_13
Karsten U., Escoubeyrou K., Charles F. (2009). The effect of redissolution solvents and HPLC columns on the analysis of mycosporine-like amino acids (MAAs) in the macroalgal species Prasiola crispa and Porphyra umbilicalis. Helgol. Mar. Res. 63, 231–238. doi: 10.1007/s10152-009-0152-0
Karsten U., Lembcke S., Schumann R. (2007). The effects of ultraviolet radiation on photosynthetic performance, growth and sunscreen compounds in aeroterrestrial biofilm algae isolated from building facades. Planta 225, 991–1000. doi: 10.1007/s00425-006-0406-x
Karsten U., Sawall T., West J. A., Wiencke C. (2000). Ultraviolet sunscreen compounds in epiphytic red algae from mangroves. Hydrobiol 432, 159–171. doi: 10.1023/A:1004046909810
Laurion I., Lami A., Sommaruga R. (2002). Distribution of mycosporine-like amino acids and photoprotective carotenoids among freshwater phytoplankton assemblages. Aqua. Microb. Ecol. 26, 283–294. doi: 10.3354/ame026283
Mason D. S., Schafer F., Shick J. M., Dunlap W. C. (1998). Ultraviolet radiation-absorbing mycosporine-like amino acids (MAAs) are acquired from their diet by medaka fish (Oryzias latipes) but not by SKH-1 hairless mice. Comp. Biochem. Physiol. Part A Mol. Integr. Physiol. 120, 587–598. doi: 10.1016/S1095-6433(98)10069-7
Muntz W. R. A. (1973). Yellow filters and the absorption of light by the visual pigments of some Amazonian fishes. Vision Res. 13, 2235–2254. doi: 10.1016/0042-6989(73)90225-3
Naylor G. J. P., Caira J. N., Jensen K., Rosana K. A. M., Straube N., Lakner C. (2012). “Elasmobranch phylogeny: a mitochondrial estimate based on 595 species,” in Biology of Sharks and Their Relatives (Boca Raton, Florida: CRC Press), 47–72. doi: 10.1201/b11867-9
Orfanoudaki M., Alilou M., Hartmann A., Mayr J., Karsten U., Nguyen-Ngoc H., et al. (2023). Isolation and structure elucidation of novel mycosporine-like amino acids from the two intertidal red macroalgae Bostrychia scorpioides and Catenella caespitosa. Mar. Drugs 21, 543. doi: 10.3390/md21100543
Orfanoudaki M., Hartmann A., Kamiya M., West J. A., Ganzera M. (2020). Chemotaxonomic study of bostrychia spp. (Ceramiales, rhodophyta) based on their mycosporine-like amino acid content. Molecules 25, 3273. doi: 10.3390/molecules25143273
Orfanoudaki M., Hartmann A., Miladinovic H., Ngoc H. N., Karsten U., Ganzera M. (2019). Bostrychines a–f, six novel mycosporine-like amino-acids and a novel betaine from the red alga Bostrychia scorpioides. Mar. Drugs 17, 356. doi: 10.3390/md17060356
Osborn A. R., Almabruk K. H., Holzwarth G., Asamizu S., LaDu J., Kean K. M., et al. (2015). De novo synthesis of a sunscreen compound in vertebrates. ELife 4, 1–15. doi: 10.7554/eLife.05919
Pavlov A. K., Leu E., Hanelt D., Bartsch I., Karsten U., Hudson S. R., et al. (2019). “The Underwater Light Climate in Kongsfjorden and Its Ecological Implications,” in The Ecosystem of Kongsfjorden, Svalbard. Eds. Hop H., Wiencke C. (Springer, Berlin, Germany), 137–170. doi: 10.1007/978-3-319-46425-1_5
Peng J., Guo F., Liu S., Fang H., Xu Z., Wang T. (2023). Recent advances and future prospects of mycosporine-like amino acids. Molecules 28, 5588. doi: 10.3390/molecules28145588
Planck P. A., Fraser N. W., Grant P. T., Middleton C., Mitchell A. I., Thomson R. H. (1981). Gadusol, an enolic derivative of cyclohexane-1,3-dione present in the roes of cod and other marine fish. Biochem. J. 199, 741–747. doi: 10.1042/bj1990741
Reimann A. (2015). Anatomisch-makroskopische Untersuchungen von Fischaugen. Eine interaktive Anleitung zur Herstellung und Fotografie von ophthalmologischen Präparaten als Grundlage zur Fischophthalmologie. Faculty of Veterinary Medicine, Ludwig-Maximilians-University Munich, Germany.
Robson T. M., Aphalo P. J., Banaś A. K., Barnes P. W., Brelsford C. C., Jenkins G. I., et al. (2019). A perspective on ecologically relevant plant-UV research and its practical application. Photochem. Photobiol. Sci. 18, 970–988. doi: 10.1039/c8pp00526e
Schuch A. P., Moreno N. C., Schuch N. J., Menck C. F. M., Garcia C. C. M. (2017). Sunlight damage to cellular DNA: Focus on oxidatively generated lesions. Free Rad. Biol. Med. 107, 110–124. doi: 10.1016/j.freeradbiomed.2017.01.029
Shick J. M., Dunlap W. C. (2002). Mycosporine-like amino acids and related gadusols: Biosynthesis, accumulation, and UV-protective functions in aquatic organisms. Ann. Rev. Physiol. 64, 223–262. doi: 10.1146/annurev.physiol.64.081501.155802
Shick J. M., Romaine-Lioud S., Ferrier-Pages C., Gattuso J. P. (1999). Ultraviolet-B radiation stimulates shikimate pathway-dependent accumulation of mycosporine-like amino acids in the coral Stylophora pistillata despite decreases in its population of symbiotic dinoflagellates. Limnol. Oceanogr 44, 1667–1682. doi: 10.4319/lo.1999.44.7.1667
Siebeck U. E., Marshall N. J. (2001). Ocular media transmission of coral reef fish - Can coral reef fish see ultraviolet light? Vision Res. 41, 133–149. doi: 10.1016/S0042-6989(00)00240-6
Thorpe A., Douglas R. H., Truscott R. J. W. (1993). Spectral transmission and short-wave absorbing pigments in the fish lens. I. Phylogenetic distribution and identity. Vision Res. 33, 289–300. doi: 10.1016/0042-6989(93)90085-B
Vincent W. F., Neale P. J. (2000). “Mechanisms of UV damage to aquatic organisms,” in The Effects of UV Radiation in the Marine Environment. Eds. de Mora S. J., Demers, S S., Vernet M. (Cambridge University Press, Cambridge, UK), 149–176. doi: 10.1017/CBO9780511535444.007
Whittock A. L., Abiola T. T., Stavros V. G. (2022). A perspective on femtosecond pump–probe spectroscopy in the development of future sunscreens. J. Phys. Chem. A 126, 2299–2308. doi: 10.1021/acs.jpca.2c01000
Zamzow J. P. (2004). Effects of diet, ultraviolet exposure, and gender on the ultraviolet absorbance of fish mucus and ocular structures. Mar. Biol. 144, 1057–1064. doi: 10.1007/s00227-003-1286-2
Zamzow J. P. (2007). Ultraviolet-absorbing compounds in the mucus of shallow-dwelling tropical reef fishes correlate with environmental water clarity. Mar. Ecol. Prog. Ser. 343, 263–271. doi: 10.3354/meps06890
Zamzow J. P., Losey G. S. (2002). Ultraviolet radiation absorbance by coral reef fish mucus: Photo-protection and visual communication. Environ. Biol. Fish 63, 41–47. doi: 10.1023/A:1013846816869
Zuclich J. A. (1989). Ultraviolet-induced photochemical damage in ocular tissues. Health Phys. 56, 671–682. doi: 10.1097/00004032-198905000-00012
Keywords: fish eyes, freshwater, MAAs, marine environment, ultraviolet radiation, UV-sunscreens
Citation: Bonin J, Hammerle FJ, Ganzera M, Krumme U and Karsten U (2024) UV-absorbing mycosporine-like amino acids in the eyes of temperate marine and freshwater fish species. Front. Mar. Sci. 11:1426861. doi: 10.3389/fmars.2024.1426861
Received: 02 May 2024; Accepted: 03 July 2024;
Published: 22 July 2024.
Edited by:
Shailendra Pratap Singh, Banaras Hindu University, IndiaCopyright © 2024 Bonin, Hammerle, Ganzera, Krumme and Karsten. This is an open-access article distributed under the terms of the Creative Commons Attribution License (CC BY). The use, distribution or reproduction in other forums is permitted, provided the original author(s) and the copyright owner(s) are credited and that the original publication in this journal is cited, in accordance with accepted academic practice. No use, distribution or reproduction is permitted which does not comply with these terms.
*Correspondence: Ulf Karsten, dWxmLmthcnN0ZW5AdW5pLXJvc3RvY2suZGU=